- 1Department of Ophthalmology, Blanton Eye Institute, Houston Methodist Hospital, Houston, TX, United States
- 2Department of Neurology, Alfred Health, Melbourne, VIC, Australia
- 3Department of Neuro-Ophthalmology, Royal Victorian Eye and Ear Hospital, Melbourne, VIC, Australia
- 4Department of Neuroscience, Monash University, Clayton, VIC, Australia
- 5Department of Neurology, Austin Health, Heidelberg, VIC, Australia
- 6Department of Ophthalmology, Weill Cornell Medicine, New York, NY, United States
- 7Department of Neurology, Weill Cornell Medicine, New York, NY, United States
- 8Department of Neurosurgery, Weill Cornell Medicine, New York, NY, United States
- 9Department of Ophthalmology, University of Texas Medical Branch, Galveston, TX, United States
- 10Department of Ophthalmology, University of Texas MD Anderson Cancer Center, Houston, TX, United States
- 11Department of Ophthalmology, Texas A and M College of Medicine, Bryan, TX, United States
- 12 Department of Ophthalmology, The University of Iowa Hospitals and Clinics, Iowa City, IA, United States
Since the first description of visual snow syndrome (VSS) in 1995, there has been increasing interest particularly within the past 5-10 years in phenotyping the condition and differentiating it from conditions such as migraine with aura and hallucinogen persisting perception disorder. Structural and functional neuroimaging has provided valuable insights in this regard, yielding functional networks and anatomical regions of interest, of which the right lingual gyrus is of particular note. Various modalities, including functional magnetic resonance imaging (fMRI), positron emission tomography (PET), and single photon emission computed tomography (SPECT), have all been studied in patients with visual snow. In this article, we conduct a comprehensive literature review of neuroimaging in VSS.
1 Introduction
The clinical manifestations of visual snow syndrome (VSS) were first described by Liu et al. in 1995, who reported a cohort of ten patients with persistent positive visual phenomena that lasted from two months to five years without evidence of migrainous infarction (1). The symptoms reported included positive visual hallucinations of simple geometric forms, transient loss of vision, as well as more complex visual distortions including palinopsia and associated altered body perceptions (1). Since this first report, there has been increasing interest in the condition phenomenologically and it was first formally described as visual snow in 2014 (2, 3). The International Classification of Headache Disorders, third edition (ICHD-3) classifies VSS as a complication of migraine, however notes that further research is required, and it may not be part of the migraine spectrum per se, but rather a common comorbid disorder with shared characteristics (4). The ICHD-3 diagnostic criteria for VSS requires the presence of dynamic, continuous, tiny dots throughout the entire visual field (visual snow) for greater than three months, with the presence of at least two additional features (palinopsia, enhanced entopic phenomena, photophobia, and/or nyctalopia), and that the presentation is not better accounted for by another condition (2, 4). The underlying pathophysiology of VSS remains unclear, but multiple hypotheses have been proposed involving abnormal processing downstream from the primary visual cortex, and neuronal hyperexcitability (5–8). Increasing research, and employment of advanced neuro-imaging has provided invaluable insights into VSS. This article reviews the literature of neuro-imaging in VSS, providing a summary of abnormal findings (Table 1) and discusses possible insights for the condition.
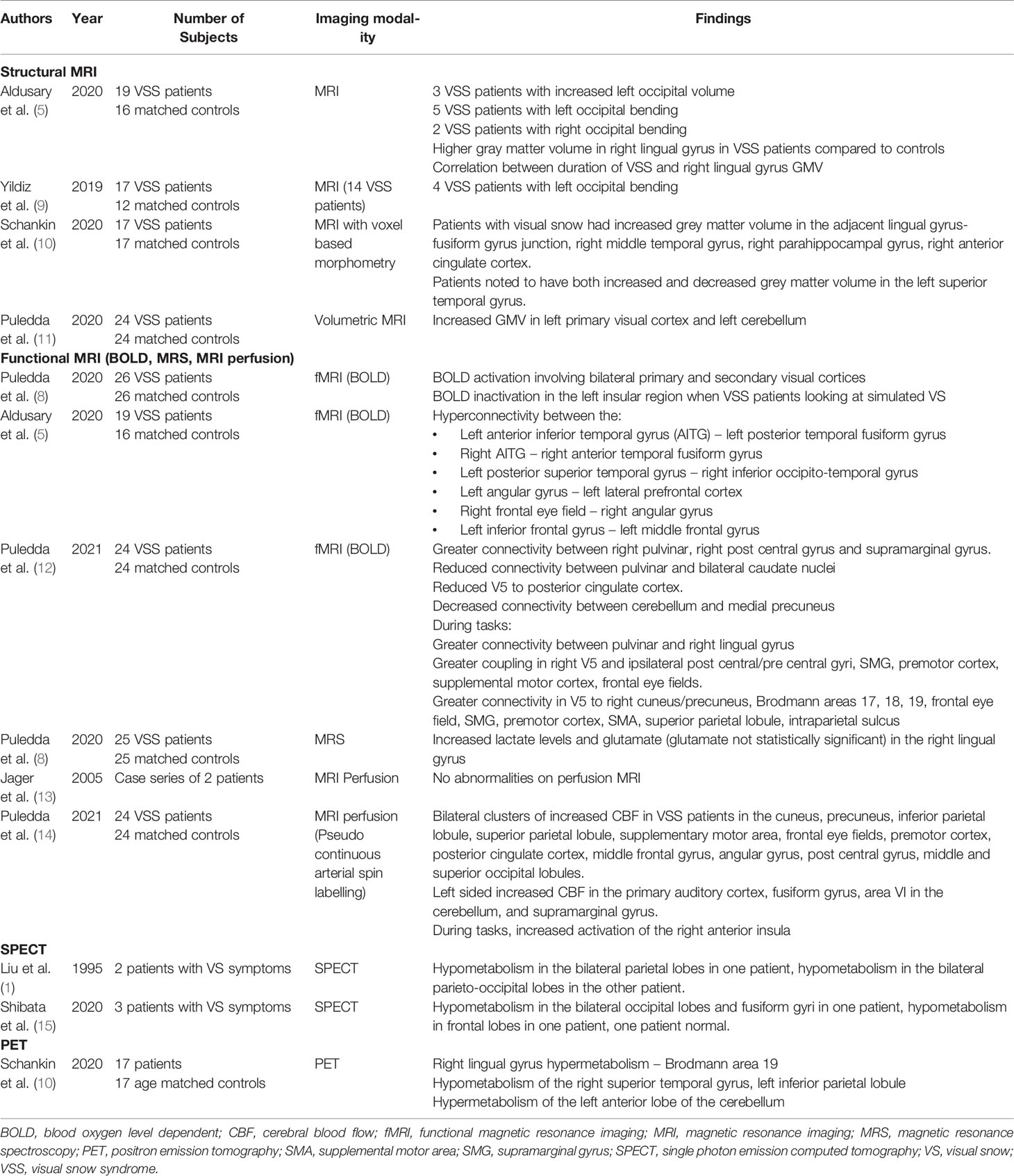
Table 1 Summary of neuroimaging studies and their findings performed in patients with visual snow syndrome.
2 Neuroimaging in VSS
2.1 Structural Neuroimaging
While investigation of VSS with cranial computerized tomography has not identified any structural abnormalities, the literature on MRI findings is more heterogeneous (16). The current literature, primarily consisting of case level evidence, includes 85 reports of patients with VSS and no evidence of structural abnormality on cranial MRI (7, 13, 16–23). In cases reported to have a structural abnormality, the most common finding related to the occipital cortex and the cerebellum (5, 9, 11, 24).
2.1.1 Occipital Bending
Changes in the occipital lobe including an asymmetric lateral ventricle can produce MRI findings where one occipital lobe wraps around the other and has been termed “occipital bending”. There are 12 case reports in the literature of occipital bending on cranial MRI (ten left, two right sided), with one case associated with hemispheric asymmetry (5, 9, 24). A case-control study compared brain MRI of 19 patients with VSS to 16 matched controls. Increased left occipital volume was noted in three patients, while occipital bending was seen in five patients on the left, and two on the right (5). Another report of 17 patients with VSS and 12 matched controls found left occipital bending in four subjects (9). The overall prevalence of this finding in VSS patients is unknown due to the relatively small populations involved in these studies, but has not been noted in any of the control patients.
Occipital bending, the phenomenon of the occipital lobe crossing the antero-posterior axis, has also been reported in several psychiatric conditions, including depression, bipolar disorder, and schizophrenia (25–29). Several theories have been postulated for the cause of this observation, including enlargement of a lateral ventricle, greater cerebrospinal fluid volumes, or increased or decreased white matter in other areas, causing secondary asymmetric different pressure in the occipital cortex. Further research is required to elucidate this. Of the twelve patients reported in the literature with VSS and occipital bending, at least four had a comorbid diagnosis of depression (the data was not available in four cases) (5, 9, 24). Further research is required therefore, to elucidate whether this finding is related to VSS or psychiatric comorbidity, as well as the underlying cause of the occipital bending.
2.1.2 Increased Gray Matter Volume
Increased gray matter volume (GMV) in the lingual gyrus has been found on volumetric MRI in 19 patients with VSS compared to 16 controls (5). A statistically significant correlation between right lingual gyral gray matter volume and disease duration was also noted (5). A second study of 17 patients with VSS with matched controls also noted increased GMV in the lingual gyrus-fusiform gyrus junction, which correlated with hypermetabolism on PET (discussed in more detail below), suggesting both a structural and functional association with VSS (10). This study also found increased GMV in the right middle temporal gyrus, right parahippocampal gyrus, and right anterior cingulate cortex, though these did not have abnormal findings on PET. Both increased and decreased GMV was noted in the left superior temporal gyrus in some of these patients (10).
The lingual gyrus, located on the inferomedial aspect of the occipital lobe, has a role in V1 and V2 visual field representation of the contralateral superior quadrant, encoding complex images, processing visual information such as parts of human faces and maintaining images in working memory, which may explain its involvement in VSS (30–32). Increased GMV in the lingual gyrus has been previously reported to be associated with divergent thinking and creativity, possibly due to increased synaptic connections (33). Supporting this hypothesis of reactive GMV change, a 2018 study of 19 healthy subjects underwent an MRI pre and post 45 minutes of sensory stimulation, demonstrated increase in GMV in brain regions receiving afferent input from the stimulated body site (34). GMV in the lingual gyrus specifically, along with other areas of the visual pathway, are increased in airline pilots and athletes, further supporting the hypothesis (35, 36).
In a separate study of 24 patients with VSS compared to matched controls, increased GMV was reported in the left primary visual cortex and left cerebellum (11). The laterality of this finding was speculated to be a statistical, not a morphological observation, as increased GMV was also noted on the contralateral hemisphere on post-hoc analysis with altered significance thresholds. PET has also shown hypermetabolism in the visual cortex previously (discussed further below), further supporting the hypothesis of occipital cortex involvement (11). Of note however, migraine is a common comorbidity seen in up to 80% of patients with VSS (36, 37). GMV in migraine has been variably reported to be increased in the lingual, fusiform and parahippocampal gyri, decreased in the V1, V2, V3, and V5 visual areas, or show no different to controls (38–40). Further research is required to clarify these observations, and the significance of GMV between these two conditions.
2.2 Functional MRI
2.2.1 Blood Oxygenation Level Dependent Imaging
Functional magnetic resonance imaging (fMRI) measures changes in brain metabolism, with the intention of differentiating metabolic changes related to specific tasks from other unregulated brain processes (41). The use of blood oxygenation level dependent (BOLD) activity to quantify areas of activation during particular tasks is one sequence of fMRI. Puledda et al. investigated 24 patients with VSS and 24 controls with fMRI while looking at a dark screen or simulated visual snow images. Both groups were found to have BOLD activation in the primary and secondary visual cortices when looking at the visual snow images (8). Additionally, the VSS group also showed BOLD deactivation in the left insula when looking at the visual snow images compared to the dark screen and the control group, also noted on the right with decreased significance thresholds (8). Cluster analysis within groups also showed BOLD deactivation with the snow task in the middle frontal gyrus, superior frontal gyrus, frontal eye fields, supramarginal gyrus, frontal operculum, and right insula with clusters from both groups also showing periventricular area BOLD deactivation (8).
Puledda et al. reviewed 24 patients and matched controls with fMRI at 8 seed points – the right pulvinar, right V1, right V5, right lingual gyrus, left cerebellum nodule VI, posterior midcingulate cortex/posterior cingulate cortex; left precuneus and right insula, locations based on findings in previous studies (12). Hyperconnectivity was noted between multiple regions, including the right pulvinar to the right postcentral and supramarginal gyrus, V5 to the right cuneus, precuneus, Brodmann visual areas 17, 18, 19, frontal eye fields, supramarginal gyrus, premotor cortex, supplementary motor area, superior parietal lobule, intraparietal sulcus, left precuneus to the right precentral gyrus and frontal eye fields, posterior cingulate gyrus to the bilateral medial precuneus, and from the cerebellum to the right lateral precuneus, right superior parietal lobule, and post central gyrus. When patients were exposed to the VS simulating task, there was additional hyperconnectivity noted between the right pulvinar and right lingual gyrus. Areas of decreased connectivity between the pulvinar and bilateral caudate nuclei, right V5 to posterior cingulate cortex and the right temporo-parietal junction, and the cerebellum to the posterior cingulate cortex and medial precuneus. Post hoc analyses adjusting for migraine showed most of these cluster differences remained statistically significant, except for the cerebellar seed findings.
Another study by Aldusary et al. assessed resting state functional connectivity of the BOLD signal comparing 19 patients with VSS to 16 controls (5). Following adjustment for multiple covariates, the authors found hyperconnectivity in the resting state between multiple brain regions. These included the left anterior inferior temporal gyrus (AITG) to left posterior temporal fusiform gyrus, right AITG to the right anterior temporal fusiform gyrus, left posterior superior temporal gyrus to the right inferior occipital temporal gyrus, left angular gyrus to the left lateral prefrontal cortex, right frontal eye field to the right angular gyrus, and the left inferior frontal gyrus to the left middle frontal gyrus (5). Of note, no hyperconnectivity to the right lingual gyrus was noted.
The findings of the within-group VSS cluster analysis by Puledda showing areas of BOLD deactivation with activity in areas where there was BOLD activation in Aldusary’s cohort of patients at rest (frontal eye field, middle frontal gyrus) might suggest these areas are of more significance.
2.2.2 MRI Perfusion
MRI perfusion has been used in migraine, malignancy, and stroke to identify functional abnormalities not visible on structural CT or MR imaging (42). No abnormalities on contrast enhanced perfusion MRI have been noted in VSS patients, though only one small case series of two patients has been reported (13).
Cerebral blood flow (CBF) maps with three-dimensional pseudo-continuous arterial spin labelling (pASL) have also been used to assess areas of functional activation in patients with VSS. Twenty-four VSS patients, matched with twenty-four age and sex matched controls underwent perfusion imaging with pASL during both a visual task (simulated visual snow image) and a control image (12). At baseline, multiple clusters of increased CBF were seen bilaterally in the VSS patients in the cuneus, precuneus, inferior parietal lobule, superior parietal lobule, supplementary motor area, frontal eye fields, premotor cortex, posterior cingulate cortex, middle frontal gyrus, angular gyrus, post central gyrus, middle and superior occipital lobules. Increased CBF were also noted on the left hemisphere in the primary auditory cortex, fusiform gyrus, area VI in the cerebellum, and supramarginal gyrus. After the visual snow stimulus, the posterior cingulate cortex and the left inferior parietal lobule did not show a significant difference, but the other listed locations did. Post hoc analysis in VSS patients without migraines only revealed increased cerebral blood flow in the left superior temporal gyrus, right superior parietal lobule, cuneus, and precuneus, and comparisons to migraine patients previously studied with similar imaging protocols showed right precentral gyrus and right precuneus activation (14). When performing tasks, the VSS patients had increased CBF to the right anterior insula compared to the control group, and that the control group had deactivation of this region (12).
The insula, the most consistently implicated structure in functional MRI, has been shown to have multiple roles in processing of visual stimuli, including initiation of visual motion imagery neurofeedback in patients without VSS (14). Activation of the insular cortex has been shown to be involved in the determination of the orientation of object movement, and noted in speech processing of lip movements without audible sounds (43). Similarly, remodeling of the anterior and posterior insula on fMRI have been noted in patients with blindness, both congenital and acquired (44). Within the VSS populations, as discussed above, findings of BOLD deactivation primarily in the left insula, as well as increased CBF in the right anterior insula have been noted. One hypothesis is that insular inactivation during visual snow like tasks may relate to baseline increased activation of the insula, or reduction in activity with the task; the former explanation is thought to better match with the insula involvement in the salience network (8). It was also noted that external stimulation of visual snow may not have the same neuroimaging correlates as the intrinsic snow perceived by the VSS cohort, and this may also explain the insula findings.
2.2.3 MR Spectroscopy
Puledda et al. also utilized MR spectroscopy (MRS) to evaluate the metabolic profile of patients with VSS. The authors studied 25 patients who had prior fMRI BOLD imaging and 25 matched controls who underwent MRS while looking at simulated visual snow and control images. The study found a significant increase in lactate levels in the right lingual gyrus in the VSS group, and a non-significant increase in glutamate levels (p=0.06) (8). These findings were postulated to be related to inefficiencies in cellular metabolism, resulting in increased anaerobic glycolysis, possibly coupled with increased energy demand from activation of the region, thereby raising lactate levels (8). The uptrend in glutamate, an excitatory neurotransmitter, which trended towards statistical significance, was also thought to represent hyperexcitability of the region. Combined with the BOLD fMRI, this was thought to represent increased activation of the lingual gyrus.
2.3 Single Photon Emission Computed Tomography
Hypometabolism on SPECT was reported in two of the patients in the initial series of patients reported by Liu. One patient was reported to have bilateral parietal, and the other bilateral parieto-occipital hypometabolism (1). A second case series of VSS patients reported one patient with bilateral occipital and fusiform gyri hypometabolism, one with mild frontal hypoperfusion, and a third patient with a normal SPECT scan (15). The relative heterogeneity of these findings and lack of age-matched controls limits the interpretation of this data.
2.4 Positron Emission Tomography
One case-control study has been published of patients with VSS who have undergone PET. In the study, 17 patients, and 17 matched controls underwent a PET scan. None of the control patients had comorbid migraine, however 82% of patients with visual snow also had migraine (10). The visual snow group was found to have right lingual gyrus hypermetabolism, as well as a trend towards hypermetabolism of the anterior lobe of the left cerebellum, after adjusting for the presence of typical migraine aura within the groups (10).
3 Discussion
3.1 Current Proposed Models of VSS
Whilst the definite underlying pathophysiology of visual snow is still not completely clear, the studies above support the hypothesis of multiple network involvement, including the visual, attentional, and salience networks. Abnormalities in all 3 of these pathways, both at rest, and during tasks, are consistent with a multi-domain aetiology, however need to be taken into consideration with the limitations below.
3.2 Limitations Within the Literature
Whilst some consistent findings have been noted through multiple studies, in particular the involvement of the right lingual gyrus, methodological challenges and differences within these studies need to be acknowledged, and are also summarised in Table 2.
3.2.1 Migraine as a Comorbidity
Whilst the prospective matched neuroimaging studies utilize age and sex matched controls, the approach to the migraine comorbidity is more variable. The prevalence of migraine in visual snow patients is reported as high as 80% in some studied populations, and whether this could be a potential confounder is still unclear. Studies by Puledda et al, which contribute a substantial proportion of the neuroimaging literature in VSS patients, excluded migraineurs from the control group, although some of the studies utilised a second model with migraine as a covariate (8, 11, 12, 14). Schankin et al. also excluded migraineurs/frequent migraineurs from the control group, whereas Aldusary et al. had migraineurs in both the VSS and control groups, and Yildiz et al. had a VSS group with migraine and one without migraine (5, 9, 10). Although there were no statistically significant differences in clinical characteristics in the VSS with and without migraine groups in the Yildiz study, larger cohorts of VSS patients have noticed different clinical characteristics within the migraineurs and non migraineurs, strengthening the argument for this being a potential confounder (9, 45).
3.2.2 Relationship to Hallucinogen Persistent Perception Disorder/Recreational Drug Use
Hallucinogen persistent perceptual disorder (HPPD) and recreational drug use, can both produce symptoms similar to VSS. By the ICHD-3 diagnostic criteria for VSS, if HPPD is suspected, a diagnosis of VSS cannot be made. Most studies excluded all patients with a history of recreational drug use, to minimize HPPD patients from being included in the patient cohort, however Aldusary et al. did not have this as an exclusion criteria, again highlighting the heterogeneity of the populations within the studies (5).
3.2.3 Age/Comorbidities
There were slight variations in inclusion criteria for age, but most studies including patients 18 – 20 years to 50 – 60 years in age. Similarly, minor variations in acceptable comorbidities existed between studies, but generally patients with major comorbidities, especially with neurological, ophthalmic, or psychiatric manifestations were excluded. Pregnant patients were excluded from all groups except in studies by Puledda et al, where pregnancy status was not specifically discussed, although patients who could not have MRI were excluded from these trials, suggesting pregnant patients may have been excluded.
4 Conclusion
Our current understanding of VSS remains ill-defined but multiple potential mechanisms and localizations have been proposed. Some of these hypotheses appear to have clinico-radiologic correlation on functional and structural neuro-imaging studies including fMRI, PET, and SPECT. To date, the current literature describes mainly heterogeneous imaging findings based upon case reports, small case series, and a few case-control studies. The most consistently reported neuroimaging findings in VSS involve the lingual gyrus and occipital cortex and supports the hypothesis that VSS is a downstream visual processing disorder. We recognize that the lingual gyrus contains the V1 and V2 visual field representation of the contralateral superior quadrant for most of its extent but also encodes complex images, processes specific visual information including human faces, and maintains images in working memory. Although the majority of anatomical findings described in the literature reference the entire lingual gyrus without reference to the exact subregion of the gyrus, we believe that future study is necessary to provide putative structure and function correlation in VSS.
Increased GMV, which is observed both in VSS and in normal populations, may be either a marker, or consequence of, the condition. Studies thus far are also somewhat heterogeneous in the patient population, allowing for possible other confounders, especially migraine.
Further research is now required to undertake larger, controlled studies to delineate these early observations, while controlling for confounding conditions such as migraine or psychiatric comorbidity. Longitudinal studies are required to determine if GMV can be correlated with clinical phenotype, duration or severity of symptoms, or response to treatment. Future neuroimaging study of VSS including fMRI, PET, and SPECT will be necessary to define the disorder better and to assess the potential efficacy of any new treatments for VSS.
Author Contributions
Category 1: Conception and design: SR, JR, PM, and AL. Acquisition of data: SR, JR, PM, and AL. Analysis and interpretation of data: SR, JR, PM, and AL. Category 2: Drafting the manuscript: SR, JR, PM, and AL. Revising it for intellectual content: SR, JR, PM, and AL. Category 3: Final approval of the completed manuscript: SR, JR, PM, and AL. All authors contributed to the article and approved the submitted version.
Conflict of Interest
The authors declare that the research was conducted in the absence of any commercial or financial relationships that could be construed as a potential conflict of interest.
Publisher’s Note
All claims expressed in this article are solely those of the authors and do not necessarily represent those of their affiliated organizations, or those of the publisher, the editors and the reviewers. Any product that may be evaluated in this article, or claim that may be made by its manufacturer, is not guaranteed or endorsed by the publisher.
References
1. Liu GT, Schatz NJ, Galetta SL, Volpe NJ, Skobieranda F, Kosmorsky GS. Persistent Positive Visual Phenomena in Migraine. Neurology (1995) 45:664–8. doi: 10.1212/WNL.45.4.664
2. Schankin CJ, Maniyar FH, Digre KB, Goadsby PJ. “Visual Snow” - A Disorder Distinct From Persistent Migraine Aura. Brain (2014) 137:1419–28. doi: 10.1093/brain/awu050
3. Ffytche DH. Visual Hallucinatory Syndromes: Past, Present, and Future. Dialogues Clin Neurosci (2007) 9:173–89. doi: 10.31887/DCNS.2007.9.2/dffytche
4. International Headache Society. IHS Classification ICHD-3. A1.4.6 Visual Snow (2018). Available at: https://ichd-3.org/appendix/a1-migraine/a1-4-complications-of-migraine/a1-4-6-visual-snow/.
5. Aldusary N, Traber GL, Freund P, Fierz FC, Weber KP, Baeshen A, et al. Abnormal Connectivity and Brain Structure in Patients With Visual Snow. Front Hum Neurosci (2020) 14:582031. doi: 10.3389/fnhum.2020.582031
6. Bou Ghannam A, Pelak VS. Visual Snow: A Potential Cortical Hyperexcitability Syndrome. Curr Treat Options Neurol (2017) 19:9. doi: 10.1007/s11940-017-0448-3
7. Lauschke JL, Plant GT, Fraser CL. Visual Snow: A Thalamocortical Dysrhythmia of the Visual Pathway? J Clin Neurosci (2016) 28:123–7. doi: 10.1016/j.jocn.2015.12.001
8. Puledda F, Ffytche D, Lythgoe DJ, O’Daly O, Schankin C, Williams SCR, et al. Insular and Occipital Changes in Visual Snow Syndrome: A BOLD fMRI and MRS Study. Ann Clin Trans Neurol (2020) 7:296–306. doi: 10.1002/acn3.50986
9. Yildiz FG, Turkyilmaz U, Unal-Cevik I. The Clinical Characteristics and Neurophysiological Assessments of the Occipital Cortex in Visual Snow Syndrome With or Without Migraine. Headache (2019) 59:484–94. doi: 10.1111/head.13494
10. Schankin CJ, Maniyar FH, Chou DE, Eller M, Sprenger T, Goadsby PJ. Structural and Functional Footprint of Visual Snow Syndrome. Brain (2020) 143:1106–13. doi: 10.1093/brain/awaa053
11. Puledda F, Bruchhage M, O’Daly O, Ffytche D, Williams SCR, Goadsby PJ. Occipital Cortex and Cerebellum Gray Matter Changes in Visual Snow Syndrome. Neurology (2020) 95:e1792–9. doi: 10.1212/WNL.0000000000010530
12. Puledda F, O’Daly O, Schankin C, Ffytche D, Williams SC, Goadsby PJ. Disrupted Connectivity Within Visual, Attentional and Salience Networks in the Visual Snow Syndrome. Hum Brain Mapp (2021) 42(7):hbm.25343. doi: 10.1002/hbm.25343
13. Jäger HR, Giffin NJ, Goadsby PJ. Diffusion- and Perfusion-Weighted MR Imaging in Persistent Migrainous Visual Disturbances. Cephalalgia (2005) 25:323–32. doi: 10.1111/j.1468-2982.2004.00858.x
14. Puledda F, Schankin CJ, O’Daly O, Ffytche D, Eren O, Karsan N, et al. Localised Increase in Regional Cerebral Perfusion in Patients With Visual Snow Syndrome: A Pseudo-Continuous Arterial Spin Labelling Study. J Neurology Neurosurgery Psychiatry (2021) 92:918–26. doi: 10.1136/jnnp-2020-325881
15. Shibata M, Tsutsumi K, Iwabuchi Y, Kameyama M, Takizawa T, Nakahara T, et al. [123i]-IMP Single-Photon Emission Computed Tomography Imaging in Visual Snow Syndrome: A Case Series. Cephalalgia (2020) 40:1671–5. doi: 10.1177/0333102420950454
16. Bessero A-C, Plant GT. Should “Visual Snow” and Persistence of After-Images be Recognised as a New Visual Syndrome? J Neurology Neurosurgery Psychiatry (2014) 85:1057–8. doi: 10.1136/jnnp-2013-306827
17. Rastogi RG, VanderPluym J, Lewis KS. Migrainous Aura, Visual Snow, and “Alice in Wonderland” Syndrome in Childhood. Semin Pediatr Neurol (2016) 23:14–7. doi: 10.1016/j.spen.2016.01.006
18. Sastre-Ibáñez M, Santos-Bueso E, Porta-Etessam J, García-Feijoo J. Visual Snow: Report of Three Cases. J Fr Ophtalmol (2015) 38:e157–8. doi: 10.1016/j.jfo.2014.06.030
19. Berkowitz E, River Y, Digre K, Tiosano B, Kesler A. Visual Snow: A Case Series From Israel. Case Rep Ophthalmol (2020) 11:205–11. doi: 10.1159/000508602
20. Eren OE, Ruscheweyh R, Straube A, Schankin CJ. Quantification of Photophobia in Visual Snow Syndrome: A Case-Control Study. Cephalalgia (2020) 40:393–8. doi: 10.1177/0333102419896780
21. Eren O, Rauschel V, Ruscheweyh R, Straube A, Schankin CJ. Evidence of Dysfunction in the Visual Association Cortex in Visual Snow Syndrome. Ann Neurol (2018) 84:946–9. doi: 10.1002/ana.25372
22. Eren OE, Schöberl F, Schankin CJ, Straube A. Visual Snow Syndrome After Start of Citalopram-Novel Insights Into Underlying Pathophysiology. European J Clin Pharmacol (2021) 77:271–2. doi: 10.1007/s00228-020-02996-9
23. Simpson JC, Goadsby PJ, Prabhakar P. Positive Persistent Visual Symptoms (Visual Snow) Presenting as a Migraine Variant in a 12-Year-Old Girl. Pediatr Neurol (2013) 49:361–3. doi: 10.1016/j.pediatrneurol.2013.07.005
24. Unal-Cevik I, Yildiz FG. Visual Snow in Migraine With Aura: Further Characterization by Brain Imaging, Electrophysiology, and Treatment - Case Report. Headache (2015) 55:1436–41. doi: 10.1111/head.12628
25. Maller JJ, Thomson RHS, Rosenfeld JV, Anderson R, Daskalakis ZJ, Fitzgerald PB. Occipital Bending in Depression. Brain (2014) 137:1830–7. doi: 10.1093/brain/awu072
26. Maller JJ, Anderson R, Thomson RH, Rosenfeld JV, Daskalakis ZJ, Fitzgerald PB. Occipital Bending (Yakovlevian Torque) in Bipolar Depression. Psychiatry Res - Neuroimaging (2015) 231:8–14. doi: 10.1016/j.pscychresns.2014.11.008
27. Maller JJ, Anderson RJ, Thomson RH, Daskalakis ZJ, Rosenfeld JV, Fitzgerald PB. Occipital Bending in Schizophrenia. Aust New Z J Psychiatry (2017) 51:32–41. doi: 10.1177/0004867416642023
28. Fullard K, Maller JJ, Welton T, Lyon M, Gordon E, Koslow SH, et al. Is Occipital Bending a Structural Biomarker of Risk for Depression and Sensitivity to Treatment? J Clin Neurosci (2019) 63:55–61. doi: 10.1016/j.jocn.2019.02.007
29. Koch K, Schultz CC. Clinical and Pathogenetic Implications of Occipital Bending in Depression. Brain (2014) 137:1576–8. doi: 10.1093/brain/awu106
30. Kozlovskiy SA, Pyasik MM, Korotkova AV, Vartanov AV, Glozman JM, Kiselnikoc AA. Activation of Left Lingual Gyrus Related to Working Memory for Schematic Faces. Int J Psychophysiol (2014) 94:241–2. doi: 10.1016/j.ijpsycho.2014.08.927
31. Mechelh A, Humphreys GW, Mayall K, Olson A, Price CJ. Differential Effects of Word Length and Visual Contrast in the Fusiform and Lingual Gyri During Reading. Proc R Soc B: Biol Sci (2000) 267:1909–13. doi: 10.1098/rspb.2000.1229
32. Machielsen WCM, Rombouts SARB, Barkhof F, Scheltens P, Witter MP. fMRI of Visual Encoding: Reproducibility of Activation. Hum Brain Mapp (2000) 9:156–64. doi: 10.1002/(SICI)1097-0193(200003)9:3<156::AID-HBM4>3.0.CO;2-Q
33. Zhang L, Qiao L, Chen Q, Yang W, Xu M, Yao X, et al. Gray Matter Volume of the Lingual Gyrus Mediates the Relationship Between Inhibition Function and Divergent Thinking. Front Psychol (2016) 7:1532. doi: 10.3389/fpsyg.2016.01532
34. Schmidt-Wilcke T, Wulms N, Heba S, Pleger B, Puts NA, Glaubitz B, et al. Structural Changes in Brain Morphology Induced by Brief Periods of Repetitive Sensory Stimulation. NeuroImage (2018) 165:148–57. doi: 10.1016/j.neuroimage.2017.10.016
35. Qiu C, Zhao C, Hu G, Zhang Y, Zhu Y, Wu X, et al. Brain Structural Plasticity in Visual and Sensorimotor Areas of Airline Pilots: A Voxel-Based Morphometric Study. Behav Brain Res (2021) 411:113377. doi: 10.1016/j.bbr.2021.113377
36. Zhang Q, Li L, Guo X, Zheng L, Wu Y, Zhou C. Implicit Learning of Symmetry of Human Movement and Gray Matter Density: Evidence Against Pure Domain General and Pure Domain Specific Theories of Implicit Learning. Int J Psychophysiol (2020) 147:60–71. doi: 10.1016/j.ijpsycho.2019.10.008
37. Puledda F, Schankin C, Goadsby PJ. Visual Snow Syndrome: A Clinical and Phenotypical Description of 1,100 Cases. Neurology (2020) 94:e564–74. doi: 10.1212/WNL.0000000000008909
38. Zhang J, Wu YL, Su J, Yao Q, Wang M, Li GF, et al. Assessment of Gray and White Matter Structural Alterations in Migraineurs Without Aura. J Headache Pain (2017) 18:1–7. doi: 10.1186/s10194-017-0783-5
39. Coppola G, Petolicchio B, Renzo AD, Tinelli E, Lorenzo CD, Parisi V, et al. Cerebral Gray Matter Volume in Patients With Chronic Migraine: Correlations With Clinical Features. J Headache Pain (2017) 18:1–9. doi: 10.1186/s10194-017-0825-z
40. Palm-Meinders IH, Arkink EB, Koppen H, Amlal S, Terwindt GM, Launer LJ, et al. Volumetric Brain Changes in Migraineurs From the General Population. Neurology (2017) 89:2066–74. doi: 10.1212/WNL.0000000000004640
41. Glover GH. Overview of Functional Magnetic Resonance Imaging. Neurosurgery Clinics North America (2011) 22:133–9. doi: 10.1016/j.nec.2010.11.001
42. McGehee BE, Pollock JM, Maldjian JA. Brain Perfusion Imaging: How Does it Work and What Should I Use? J Magn Reson Imaging (2012) 36:1257–72. doi: 10.1002/jmri.23645
43. Protas M. Role of the Insula in Visual and Auditory Perception. In: Turgut M, Yurttaş C, Tubbs RS, editors. Island of Reil (Insula) in the Human Brain. Cham: Springer International Publishing (2018). p. 151–6. doi: 10.1007/978-3-319-75468-0_16
44. Liu L, Yuan C, Ding H, Xu Y, Long M, Li Y, et al. Visual Deprivation Selectively Reshapes the Intrinsic Functional Architecture of the Anterior Insula Subregions. Sci Rep (2017) 7:1–11. doi: 10.1038/srep45675
Keywords: visual snow, neuroimaging, lingual gyrus, occipital cortex, visual cortex
Citation: Raviskanthan S, Ray JC, Mortensen PW and Lee AG (2022) Neuroimaging in Visual Snow - A Review of the Literature. Front. Ophthalmol. 2:758963. doi: 10.3389/fopht.2022.758963
Received: 15 August 2021; Accepted: 17 February 2022;
Published: 11 March 2022.
Edited by:
Victoria Susan Pelak, University of Colorado, United StatesReviewed by:
Michael S. Vaphiades, University of Alabama at Birmingham, United StatesDominic Ffytche, King’s College London, United Kingdom
Copyright © 2022 Raviskanthan, Ray, Mortensen and Lee. This is an open-access article distributed under the terms of the Creative Commons Attribution License (CC BY). The use, distribution or reproduction in other forums is permitted, provided the original author(s) and the copyright owner(s) are credited and that the original publication in this journal is cited, in accordance with accepted academic practice. No use, distribution or reproduction is permitted which does not comply with these terms.
*Correspondence: Andrew G. Lee, YWdsZWVAaG91c3Rvbm1ldGhvZGlzdC5vcmc=