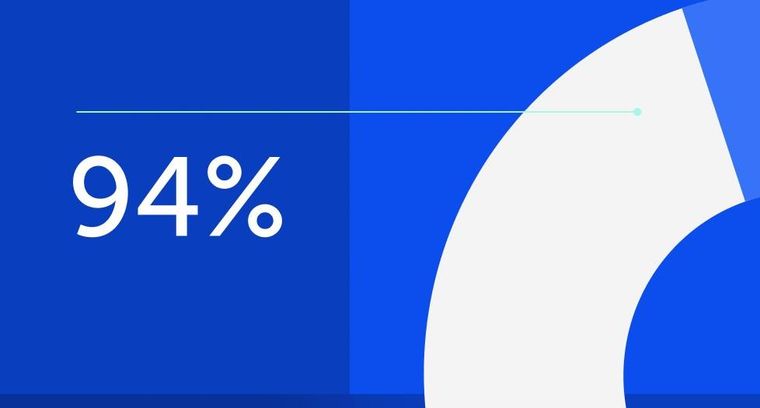
94% of researchers rate our articles as excellent or good
Learn more about the work of our research integrity team to safeguard the quality of each article we publish.
Find out more
REVIEW article
Front. Oncol., 11 April 2025
Sec. Cancer Metabolism
Volume 15 - 2025 | https://doi.org/10.3389/fonc.2025.1570288
Bilirubin, a metabolite of hemoglobin, was long thought to be a harmful waste product, but recent studies have found it to have antioxidant and anti-tumor effects. With the extensive research on the mechanism of malignant tumor development, the antioxidant effect of bilirubin is increasingly becoming a hotspot in anti-cancer research. At present, there are two main views on the relationship between bilirubin and cancer, namely, its pro-cancer and anti-cancer effects, and in recent years, studies on the relationship between bilirubin and cancer have not been systematically summarized, which is not conducive to the further investigation of the role of bilirubin on cancer. To understand the multifaceted role of bilirubin in tumorigenesis as well as to develop more effective and affordable antitumor therapies, this review provides an overview of the effects of bilirubin on tumors in terms of oxidative, inflammatory, and cellular signaling pathways, as well as the resulting therapeutic ideas and approaches.
Cancer, an increasingly prevalent disease internationally, has become one of the principal causes of morbidity and death globally, with serious economic costs to society. As reported by the International Agency for Research on Cancer, the global cancer burden has risen, resulting in 19.3 million new cancer incidences and nearly 10 million deaths from cancer in 2020 alone (1). Although there are multiple treatment options for cancer, the outcomes remain unsatisfactory. Bilirubin, an endogenous antioxidant, is a widely used biomarker for diagnosing liver diseases. For a considerable time, bilirubin was regarded as a harmful metabolic waste. However, recent studies have revealed that there is a link between cancer and bilirubin levels. Mildly elevated bilirubin levels are generally related to a lower incidence of cancer and a better prognosis, but excessively high levels are linked to a higher incidence of cancer (2, 3). This article provides an overview of the diverse impacts of bilirubin metabolism on tumor progression and its utilization in cancer therapy, aiming to offer new ideas for improving tumor treatment.
Bilirubin presents as a product of hemoglobin degradation and a bile pigment. Under physiological conditions, about three-quarters comes from senescent erythrocytes undergoing catabolism in the reticuloendothelial system, which contains splenic macrophages and hepatic Kupffer cells, and the remaining one-quarter of hemoglobin comes from ineffective red blood cell production and enzymes containing hemoglobin (4). In macrophages, heme is first decomposed to generate biliverdin by heme oxygenase-1 (HO-1), which then undergoes reduction to bilirubin by biliverdin reductase(BVR) (5, 6) (Figure 1). Bilirubin is a lipophilic molecule that accumulates in the cell, diffuses into the bloodstream, and then binds to circulating albumin to be transported to the liver. Unconjugated bilirubin (UCB, i.e., indirect bilirubin) is absorbed actively or passively by hepatocytes, mediated by the hepatic organic anion transporting polypeptides 1B1 (OATP1B1), and passes through uridine glucuronosyltransferase family1 memberA1 (UGT1A1) undergoes mono- or bi-glucuronidation (7) to form bilirubin monoglucuronide or bilirubin bi-glucuronide (i.e., direct bilirubin), which is excreted via the bile ducts by the multidrug resistance protein-2 (MRP2). It is first deposited in the gallbladder after the entry of direct bilirubin into the bile. Then it passes into the intestines along with many other bile components to promote the intake of fats and other fat-soluble chemicals. Bilirubin glucuronide is initially conjugated by microbial β-glucuronidase, and then the unconjugated bilirubin is oxidated and reduced by the intestinal flora (8). Several products can be reabsorbed back into the circulating bilirubin pool; while others are cast out through the intestines and kidneys, respectively, resulting in the characteristic colors of urine and feces.
Figure 1. Metabolism of bilirubin in the body: Heme is decomposed by HO-1 (reticuloendothelial system) to produce bilirubin, which is then converted into indirect bilirubin by bilirubin reductase. Indirect bilirubin is absorbed by hepatocytes using UGT1A1, forming direct bilirubin, which is carried into the gallbladder, excreted via the bile ducts, transported to the intestines, and finally broken down by bacteria. Part of the products are recycled to enter the circulating pool of bilirubin (i.e., the enterohepatic circulation), and the rest is excreted through the kidneys and the intestines.
For a considerable time, bilirubin was regarded as a harmful metabolic waste. However, Serum bilirubin concentrations’ mild increases, such as those in patients with Gilbert’s syndrome (GS, a benign form of unconjugated hyperbilirubinemia), as well as levels in the upper quartile of the currently accepted physiologic serum bilirubin range, have been reported to be negatively correlated with a wide range of disorders within the human body.
It has been shown that bilirubin can down-regulate NAD(P)H oxidase to prevent diabetic nephropathy by being catabolized into biliverdin(BV), which has been demonstrated in rodents (9). Several studies have shown that mildly raised UCB also reduces the incidence of obesity, Alzheimer’s disease (10), metabolic syndrome (11, 12), nonalcoholic fatty liver disease (13, 14), and diabetes mellitus (15, 16). Thus, there is strong proof that bilirubin is a clinically important biomarker for reducing the prevalence of chronic diseases (16).
The positive effects of bilirubin on other chronic diseases have inspired scholars to study its relationship with cancer. It has been shown that mildly elevated bilirubin levels are generally related to a lower incidence of cancer and a better prognosis, but excessively high levels are linked to a higher incidence of cancer. Due to the lack of statistical studies related to bilirubin covering multiple cancers, the correlation between different cancers and bilirubin cannot be quantified at present and can only be roughly analyzed through existing independent studies. Several studies have shown that cancers with a strong association with bilirubin include liver, lung, and digestive tract cancers such as gastric (17), esophageal (18), and colorectal cancers, and gynecological cancers such as breast (19–21) and cervical (22) cancers. Since the liver, as an organ directly involved in many physiological processes including bilirubin metabolism processes, has a more complex relationship with bilirubin, the details of which will be described later, the sections other than hepatocellular carcinoma (HCC) are described here. As noted in a 2019 observational study, serum bilirubin has a strong negative correlation with all-cause mortality and is primarily motivated by the impact of bilirubin on cancer (2). In 2020, Horsfall et al. conducted a Mendelian randomization study that analyzed data from over 377,000 individuals from the UK Biobank. They found that people with the rs887829 and rs4149056 genes (genes that cause elevated levels of bilirubin) had a 17% reduced risk of suffering from lung cancer, a percentage that had an even larger value in the smoker population. This is because the extra bilirubin may help to counteract some of the effects of the reactive oxygen molecules that fill the lungs (23). The association of high bilirubin with low lung tumor risk has also been shown in other studies (22, 24–28). As another example, in several studies, a low prevalence of colorectal cancer was associated with high serum bilirubin concentrations or GS genotype presentation (29–31). Serum bilirubin levels were also found to be significantly lower in CRC patients than in controls in another study of colorectal cancer (32). However, Zhang and coworkers found in a retrospective study that high DBIL was strongly associated with a poorer postoperative prognosis in patients with stage II and stage III colorectal cancer. Subsequent studies confirmed these results (33, 34). This reflects the two-sided nature of bilirubin in cancer.
Genetic factors can influence cancer by affecting bilirubin levels, and in addition to rs887829 and rs4149056 described above, the Gilbert syndrome genotype (UGT1A1*28 purebred) (2) has been associated with elevated bilirubin levels. UGT1A1*28 allele carrier status is associated with a 20% reduction in CRC risk (32). Although bilirubin levels are highly heritable and genetic variation in UGT1A1 explains a large portion of the variance, it has also been shown that enzymes involved in the production of bilirubin from hemoglobin, such as HO, may also have an effect (35). There is also the T(-413)T genotype of rs2071746, which has been shown to have significantly lower serum bilirubin levels in T(-413)T carriers in both the CRC group and the control group (32).
The above article describes the association of bilirubin with some cancers, which naturally leads to the question of whether bilirubin can be used as a way to optimize bilirubin-related cancer treatment and patient stratification, but it has been shown that, while individual serum markers are useful prognostic factors in the study of patients with cancer, individual markers may not be sufficient for predicting survival in the clinical setting. Combining multiple markers in a single index can improve their predictive ability (36–38). In addition to this, the performance of the single factor of bilirubin varies in studies targeting different cancers, e.g., in a study including male smokers as subjects, each 0.1 mg/dL decrease in bilirubin was associated with a 5% and 6% increase in the risk of lung cancer incidence and death, respectively (26), whereas an increase in bilirubin levels of 5 μmol/L in another study of smokers was associated with a lung cancer incidence rate decreased by 10.2/10,000 person-years (23). Therefore, this paper will only present the standard model related to bilirubin that has gained more acceptance. Several of these representative models will be described next.
Total bilirubin (TB or TBIL) is one of the biomarkers reflecting the development of cancer and can be abnormal in diagnostic tests for cancers such as adenocarcinoma of the jugular and stomach. Total bilirubin can be used as a valid prognostic predictor for determining cancer prognosis, and valid prognostic evaluation tools can be developed based on total bilirubin. For example, researchers have developed a new prognostic score for adenocarcinoma of the jugular abdominal region according to the preoperative ratio of total bilirubin-albumin and fibrinogen-albumin by using a controlled study approach (39). Other researchers used a prospectively trained and retrospectively validated study methodology in 778 gastric cancer patients and analyzed using X-tile software, determined that the serum levels of TBIL and albumin were independent OS forecasters in patients of gastric cancer (17), and then low serum TBIL was linked to advanced gastric cancer and worse prognosis. Another study demonstrates a strong connection between high-level DBIL and poor postoperative prognostic results in stage II and stage III colorectal cancer patients (40). A multicenter study of the association between serum TB and mortality of all causes in cancer patients with cachexia also showed that patients with high TBIL levels had worse OS in the presence of serum total bilirubin greater than the normal range of between 1.7-17.1 μmol/L (≥21.7 μmol/L) (41). The prediction of cancer prognosis by TBIL has shown different results in different studies, which requires further research to explore its internal logic.
The ALBI grade is a serviceable tool proposed by Philip J et al. in 2015 for assessing cancer status, as they ascertained objective measures of hepatic function (albumin and bilirubin) that independently affect the survival of HCC patients by utilizing data from extensive global databanks, assembling those to form a model comparable with the traditional C-P classification (42), which has a linear prediction equation of Linear prediction = (log10 bilirubin × 0.66)(albumin × -0.085), bilirubin in μmol/L and albumin in g/L. This model calculates patient-level linear predictions (xb) as well as applies cutpoints to assign every patient to any of the three prognosis divisions, now labeled ALBI classification, grades 1 to 3. Points of cut are xb ≤ -2.60 (ALBI class 1), > -2.60 ~ ≤ -1.39 (ALBI class 2), xb > -1.39 (ALBI class 3). Earlier studies have demonstrated that ALBI can help determine the prognosis of cancers such as HCC. In a study published in 2024, researchers found that ALBI and platelet-albumin-bilirubin(PALBI) grade were related to the prognosis of small cell lung cancer(SCLC) and could be utilized as simple, affordable, and a useful marker for the determination of follow-up therapies and prognosis of SCLC patients (43) by examining the association between ALBI grade and the PALBI grade and prognosis in SCLC patients, and as the grade scale increased, the mortality rate of the patients improves. Several studies published between 2021 and 2024 for HCC have shown that the ALBI grade plays a role in aiding outcome evaluation and liver reserve evaluation at several stages in stereotactic body radiotherapy of the liver, and thus it is helpful in determining the prognosis of HCC patients who undergo this therapy (44–46), and that the rate of hepatotoxicity occurs at an elevated rate as the grade increases. In addition, ALBI has been used in the assessment of the prognosis of various cancers such as squamous cell cancer of the esophagus (47) and SCLC (43). In summary, ALBI can be used as one of the factors for evaluating the prognosis of a wide range of cancers and can be a useful tool for determining prognosis as well as helping to make decisions related to cancer treatment.
Although the ALBI grade is very objective, its calculation process is quite complex. So Kariyama et al. introduced the easy-albumin-bilirubin grade (easy-albumin-bilirubin) in 2020, which is much easier to calculate in estimating hepatic functional reserve and is strongly linked with the original ALBI grade (48). A study published in 2024 in HCC has confirmed that the simple albumin-bilirubin grade is an objective and feasible prognostic model for assessing the abnormalities of liver function in HCC patients and is independent of the patient’s performance status.
Platelets play a marker role during the course of portal hypertension in cirrhosis and may reflect HCC. Thus Roayaie et al. proposed the PALBI class at the liver meeting in 2015. The PALBI classification includes bilirubin, albumin, and platelet count in serum levels to signify liver reserve in HCC. When this score rises, it usually signals a poor prognosis for the cancer. The score has been validated in the prognostic assessment of cancers such as small-cell lung cancer (43) and HCC (49–51), and it has even been pointed out that in the model of platelet-albumin (PAL), the easy (EZ)-ALBI grade and the ALBI grade, PALBI, and end-stage liver disease (MELD), which are the several hepatic reserve models, PALBI was the best model (52). In summary, PALBI is an available prognostic assessment model in HCC as well as other cancers.
In normal physiological circumstances, the presence of antioxidants counteracts the reactive oxygen species (ROS), resulting in a balance (53), while oxidative stress is a situation when the balance is disrupted. Cancer development and progression are closely associated with the processes of intracellular oxidative stress. The ROS overproduction results in oxidative impairment of lipids, proteins, and DNA (53), where the accumulation of DNA damage induced by ROS may lead to genetic destabilization in the case of cancer, thus facilitating the development of cancer (54). In addition to this, oxidative stress acts as a second messenger to promote cell proliferation (55) and angiogenesis (56), creating favorable conditions for tumor growth and development. Bilirubin is a potent free radical scavenger with antioxidant properties and reduces ROS, especially in lipid peroxidation (57, 58). In 1987, Stocker et al. proved in a study that bilirubin possesses characteristics of a natural ROS scavenger with superior antioxidant properties compared to those of vitamins C and E (59). Another study showed that every 1 mol of bilirubin scavenges 2 mol of oxygen free radicals, which in turn prevents excessive ROS from causing damage to the body (60). In addition to direct antioxidant responses, it has also been shown that bilirubin can indirectly function to the alleviation of oxidative stress, examples including activation of the nuclear factor erythroid 2-related factor 2 (Nrf2) pathway through covalently binding to Kelch-like ECH-associated protein 1 (KEAP1) (54, 61) and thus acting as an antioxidant in the cell. Thus bilirubin’s ability to scavenge ROS gives it an inhibitory function on cancer development and cancer-related inflammation. Bilirubin may also inhibit other diseases through its inhibitory effect on oxidative stress and thus inhibit tumors, for example, the occurrence of metabolic syndrome is closely related to oxidative stress (62), and some studies have also shown a negative relationship between the level of human serum total bilirubin and the incidence of metabolic syndrome (63–65), which in turn is positively correlated with the occurrence of many cancers, e.g., cancers of lung and colorectal (66–68), so bilirubin may inhibit the development of related cancers in this way. Besides that, in cancers that have already developed, bilirubin may also function to inhibit tumor progression while preserving normal cells. In studies on a variety of cancers, cancer cells have been shown to be under high levels of oxidative stress (69–75). In a model proposed in the literature, a decrease in the level of oxidative stress may contribute to the apoptosis of malignant cells and may serve as one of the mechanisms by which bilirubin screens malignant cells (76) (Figure 2).
Figure 2. Bilirubin inhibits oxidative stress and thus tumor development: Bilirubin inhibits oxidative stress through two main pathways. First, it acts directly as an antioxidant to scavenge oxygen radicals, thereby inhibiting the high level of oxidative stress of cancer cells and selectively causing cancer cell apoptosis; second, it promotes the expression of several antioxidant genes by covalently binding to KEAP1 and activating the Nrf2 pathway. Through these two pathways, bilirubin also inhibits oxidative stress-induced diseases that are highly associated with cancer, such as metabolic syndrome.
The Bcl-2 protein family supervises the integrity of the cellular genome to inhibit tumor growth (77). According to their function and structure, the proteins can be classified into three categories (78). The first is the Bcl-2 subfamily, which possesses a characteristic of apoptosis resistance (79). Then there is the Bcl-2-associated X protein(Bax) subfamily, which has pro-apoptotic activity and can form holes within the mitochondrial membrane (80), resulting in mitochondrial outer membrane permeabilization (MOMP) as well as a short discharge of cytochrome c (81, 82). The third group, the BH3 domain-containing proteins subfamily, is considered to be a lethal structural domain essential for apoptosis and critical for pro-apoptotic activity. UCB causes mitochondrial ROS production, which consequently causes activating p38 and p53 downstream, ultimately leading to the increased regulation of the pro-apoptotic protein Bax and the decreased regulation of the anti-apoptotic protein Bcl-2 and phosphorylation of Bcl-xL/Bcl-2 associated death promoter (Bad) (83), thus causing apoptosis. The same results can be found in another study for breast cancer (84). It follows that bilirubin may induce apoptosis in tumor cells by upregulating Bax and downregulating Bcl-2.
Bilirubin, an endogenous antioxidant, has a dramatic antitumor impact on the carcinoma of colon and rectum cell lines. It influences cell survival and tumor cell progress, possibly by regulating the expression level of the p53 protein, and consequently controls apoptosis and autophagy. Autophagy being a complex and environmentally relevant event, has been described to be associated with the occurrence of the carcinoma of colon and rectum. Autophagy confines the proliferation of cancer cells in the early stages and promotes cancer progression under stressful situations in the later stages. In one study, researchers found that bilirubin significantly inhibited autophagy in the human colon adenocarcinoma cell lines of LS180 and SW480 cells by suppressing Beclin-1 and Microtubule-Associated Protein 1 Light Chain 3B (LC-3B) in the cells, and increased apoptosis by the upregulation of p53 in LS180 cells and downregulation of the Bcl-2 gene which inhibits apoptosis in SW480 cells (85). However, the study of bilirubin’s influence on cancer development through autophagy is still incomplete, and the above study did not address the impact of autophagy in the progression of cancer, which still needs to be demonstrated in subsequent studies.
Nuclear factor-κB (NF-κB) is a nuclear factor family consisting of multiple transcription factors involved in regulating inflammatory response, cell proliferation, tumor development, and invasion. Studies have shown that bilirubin and its metabolite biliverdin can inhibit this transcription factor.
A substantial quantity of experimental evidence demonstrates that NF-κB participates in the epithelial-mesenchymal transition (EMT) process vital for the local and distant progression of cancer by up-expressing the marker N-cadherin of mesenchymal and down-expressing the marker E-cadherin of epithelium (86). In addition, matrix metalloproteinases (MMPs), as the target of NF-κB action, play critical parts in physiological processes, e.g., organ growth and tissue metastasis (87). Studies in cervical cancer cells have found that NF-κB induces Epithelial-mesenchymal transition and stem cell-like characteristics of the tumor and promotes the tumor cells, self-renewal and migration (88). In cervical cancer cells, the modifications after translations of NF-κB were also reported to regulate tumor cell metastasis and invasion. The o-linked β-n-acetylglucosamine (o-glcnacylation) modification, dramatically elevated in cervical cancer cells, is the only intracellular glycan modification engaged in signaling. O-glcnacylation enhances the translocation of NF-κB by inhibiting the interaction of NF-κB with IκB. This enhanced the C-X-C chemokine receptor 4 (CXCR4) expression downstream, upregulated the expression of HPV E6/E7, and Ki-67, and then promoted the metastasis of uterine cervical cancer cells to the lung (89).
It is confirmed in mouse myocardial microvascular endothelial cell line H5V that UCB may influence the regulatory pathway of NF-κB via interaction with IKK proteins by inhibiting tnfα-stimulated NF-κB nuclear translocation (90). Another study also showed that astrocyte cultures showed a dramatic increase in tumor necrosis factor receptor1(TNFR1) levels, as well as subsequent activation of MAPKs p38, Jun N-terminal kinase1/2, and NF-κB, and signal-regulated kinase1/2, which is outside of the cell, when stimulated with UCB (91). The results suggest that bilirubin exerts a protective effect in intrinsic immune-related inflammation at certain doses by a mechanism associated with the NF-κB signaling pathway inhibition and the NOD-like receptor family CARD domain-containing protein 4 (NLRC4) activation, Absent in Melanoma 2 (AIM2), and NOD-like receptor family pyrin domain containing 3 (NLRP3) inflammatory vesicles, of which the inhibition contributes to the control of cancer (92–94).
Studies have shown that the metabolite of bilirubin, biliverdin, suppresses NF-κB activation caused by TNF-α, and overexpression of NF-κB stimulated by hbvr causes the cell cycle to halt in phase 1/0. This supports BVR and its substrates to regulate NF-κB, thus biliverdin can be used as a potential therapeutic tool to regulate NF-κB and is expected to inhibit the proliferation of tumor cells through this pathway (95). Biliverdin may affect NF-κB through at least two pathways. Biliverdin may inhibit NF-κB function by directly binding (96, 97). Biliverdin may also interfere with NF-κB activation signaling through its tetrapyrrole molecular structural properties (98). It has also been shown that bilirubin treatment reduced p-p65, p-IκBα, and IκBα protein levels to normal levels, suggesting that bilirubin specifically reduces NF-κB pathway activation associated with inflammation. Therefore, besides its potent anti-oxidative stress effects, bilirubin may also attenuate inflammation in osteoarthritis (OA) by inhibiting the NF-κB pathway (99), and some studies have suggested that osteoarthritis may promote the development of cancer, so bilirubin may serve to decrease the cancer development risk in this way (100).
The Ras-Raf-MEK-ERK pathway integrates signals coming from the cell surface receptors into signaling pathways downstream that promote cell growth processes and proliferation in many categories of cells.
Bilirubin exerts an inhibitory effect on multiple sites of the RAS/RAF/MEK/ERK signaling pathway. For example, in vitro bilirubin damages the Raf/ERK/MAPK pathway activation and intracellular levels of Raf and cyclin D1, leading to hypophosphorylation of amino acids S608 and S780 by retinoblastoma proteins, which prevents the release of Yin Yang 1(YY1) into the nucleus and hinders the capability of YY1 to regulate gene expression and support cell proliferation. Bilirubin was also found to promote growth arrest in the cultures of human vascular smooth muscle primary cells stimulated by serum. Researchers have proposed that this is a result of the interaction of bilirubin with the Raf/ERK/MAPK pathway, its impact on the content of cyclin D1 and Raf, altering the hypophosphorylation profile of retinoblastoma protein, calcium efflux, and YY1 protein hydrolysis (101).
As for vascular growth, which is highly correlated with tumor development, bilirubin inhibits ERK activity in damaged blood vessels, and because ERK activation is associated with the mitogenic stimulation-induced cyclin D1 expression (102, 103), the reduction of ERK activity caused by bilirubin may be responsible for the down-regulation of cyclinD1 by bilirubin after arterial injury. Furthermore, the expression of the transcription factor p53 and the cyclin-dependent kinase inhibitor p21 induced by bilirubin inhibits the proliferation of established smooth muscle cells (SMC) and neoplastic endothelial proliferation (104, 105). The anti-proliferative effects of bilirubin may involve various mechanisms. In vascular SMC cultured in vitro, bilirubin reduces the activity of ERK (101), which is a key kinase for entry into the S phase. In addition, the reduction of ERK activation by bilirubin correlates with the ability of bilirubin to block airway SMC growth. However, during the mediation of the anti-proliferative effects of bilirubin on vascular SMC, the transcription factor p53 appears to act importantly. In injured blood vessels, researchers found bilirubin to be a powerful inducer of p53 expression. Furthermore, bilirubin facilitates p53 expression in a variety of cell types, and p53 deletion eliminates the anti-proliferative influence of bilirubin in mouse SMC. What is interesting is that p53 may also promote the ability of bilirubin to cause apoptosis in SMC cultured under serum-free or serum-restricted conditions due to its stimulation of the apoptosis signaling pathway (106). Besides, as p21 overexpression in vascular SMC has been demonstrated to restrain their migration, p21 may as well be involved in the anti-migratory effects of bilirubin (107). Furthermore, bilirubin inhibits nasopharyngeal cancer cell invasion by decreasing intracellular ROS levels and inhibiting ERK1/2 activation and MMP-2 expression (108). While many associations exist between bilirubin and blood vessels and smooth muscle, studies addressing bilirubin’s effect on tumor vasculature and smooth muscle tumors are scarce and require further study.
Bilirubin inhibits PKA-catalyzed histone phosphorylation, as well as cAMP binding to PKA regulatory subunits, through both competitive (109) and noncompetitive (110) mechanisms. PKA anchoring acts importantly in pseudopod formation and chemotactic cell migration (111). Activation of the cAMP/PKA pathway induces loss of stress fibers (112), activation of regular arrangement of collecting venules (Rac) and Cell Division Cycle 42 (Cdc42) (113, 114), formation of filamentous pseudopods and lamellar pseudopods (114, 115) microfilament assembly (116), and inhibition of the Rho family of GTPases activity (117, 118), events which happen at the tip of migrating cells. PKA also affects integrin-dependent migration in a variety of cells (119) and PKA and its phosphorylated substrates are enriched in cellular protrusions (111, 120). Several types of cancers utilize the cAMP/PKA signaling pathway to behave malignant features of cancer, such as invasion, relocation, adhesion, and proliferation. PKA has been proven to inhibit the expression of malignant features of cancer by inhibiting the cAMP/PKA signaling pathway. Such correlation has been demonstrated in ovarian cancer (121–123), glioblastoma (124), colorectal cancer (125), breast cancer (126) and pituitary tumors (127), esophageal squamous cell carcinoma (128), suggesting that bilirubin may inhibit tumor progression by suppressing PKA (Figures 3, 4).
Figure 3. 1. NF-κB: (1) Bilirubin promotes tumor development by altering immune cell phenotype. (2) Bilirubin, converted from bilirubin, also inhibits IκBα and P65 associated with NF-κB activity thereby inhibiting NF-κB. (3) Bilirubin also inhibits Tnfα associated with NF-κB activation thereby inhibiting its function. 2. Raf, Bilirubin inhibits Raf, and thus retinoblastoma protein, which in turn inhibits the release of YY1 into the nucleus and blocks its proliferation-promoting process. 3. ERK, Bilirubin inhibits proliferation and cancer metastasis by inhibiting ERK, thereby inhibiting cyclin D1 and MMP-2; Bilirubin can also inhibit proliferation by directly inhibiting cyclin D1. 4. Bcl2, Bilirubin promotes the production of ROS in mitochondria, which activates P38, and its activation of P53 inhibits Bcl2, which in turn inhibits the anti-apoptotic process. 5. PKA, Bilirubin inhibits the binding of PKA to cAMP thereby inhibiting its pro-value-added effects.
Figure 4. Bilirubin promotes cancer inhibition pathway: Bilirubin promotes ROS production in mitochondria, which activates P38, and its activated P53 activates BaX, which promotes its pro-apoptotic process. Bilirubin can also directly activate P53 and P21 to play an anti-cancer role.
Bilirubin can not only have a promoting effect on the immune system, but also an inhibitory effect, and this inhibitory effect can have an inhibitory effect on tumors. Next, we will talk about the direct and indirect inhibitory influences of bilirubin on the immune system, respectively.
Bilirubin acts as an inhibitor at many sites in the immune system, including immune cell proliferation, antibody secretion, and complement activation. Counterintuitively, while bilirubin can act as a suppressor of the immune system (129), serving to increase the rate of infection (130–132), this suppression can instead act as a cancer suppressor. As one study showed (133), unconjugated bilirubin disrupts the interaction between C1q and immunoglobulins, thereby suppressing the first step of the classical pathway of complement activation, which is activated by several factors that are cancer-promoting (134–136), so this inhibition of the classical pathway may act as a cancer-inhibitor. Studies carried out on jaundiced infants have shown (137, 138) that the lymphocytes extracted from them are in a state of proliferation inhibition and their total IgA and IgM levels are reduced. In terms of bilirubin’s effects on immune cells, a 2017 study showed (139) that unconjugated bilirubin upregulates CD39 and thus inhibits Th17 immunoreactivity, this promotes several cancers, such as breast, colorectal, and ovarian cancers (140–142), and thus, the inhibition of TH17 by unconjugated bilirubin can play a role in inhibiting related cancer effects. Other than this, UCB activates both extrinsic and intrinsic pathways of apoptosis as reflected by markers such as CD95, caspase-8, Bax, MMP, cytoplasmic Ca2+, caspase-3, and DNA fragmentation (143). This literature also points out that glutathione is a key molecule in preventing UCB-induced cell death, which may help to investigate the use of bilirubin in cancer control.
Bilirubin can restrain the development of cancer by reducing inflammation and inhibiting oxidative stress. Inflammation is a pathological process featuring tissue damage or destruction resulting from a variety of chemical and cytological reactions. It usually has typical symptoms such as redness, swelling, fever, pain, and function loss. As mentioned in the previous section, ROS are pro-inflammatory, whereas bilirubin is known to scavenge ROS and reduce oxidative stress. In a 2020 study (144), Lee et al. treated acute colitis in a mouse model of inflammatory bowel disease using hyaluronic acid-bilirubin nanoparticles, which reinstated the epithelial barrier in the mice as well as modulating the intestinal microbiota, showing promising anti-inflammatory properties by increasing overall abundance and diversity. In another study done on a rat model of acute pancreatitis (145), Yao et al. used a similar approach also to find that bilirubin had an anti-inflammatory effect, and this treatment was demonstrated to protect alveolar cells from damage and alleviate acute pancreatitis. The anti-inflammatory effect of bilirubin can be side-stepped by the fact that serum bilirubin is negatively related to the markers of oxidative stress in serum (146, 147) and markers of inflammation such as C-reactive protein levels (148, 149). Many studies demonstrated that a variety of chronic inflammatory conditions are strongly associated with cancer development, such as IBD (150) and pancreatitis (151–153). This indicates that bilirubin has an anti-inflammation effect with a possible reduction in inflammation-associated cancers by this pathway.
The effect of bilirubin on cancer has long been thought to be primarily associated with its strong antioxidant properties. However, in recent years, a growing amount of studies have shown that bilirubin acts as a ligand role in cellular signaling processes and through this role has an impact on cancer development.
Receptors that can bind bilirubin include aryl hydrocarbon receptor (AhR), peroxisome proliferator-activated receptors α (PPARα), constitutive androstane receptor (CAR), fatty acid-binding proteins (FABPs), etc., which will be described below.
AhR is a widespread receptor found in many organs, body tissues, and cell groups (154), exhibiting both anticancer and oncogenic properties (155). Experiments carried out on cells cultured in vitro have shown that both bilirubin and biliverdin are endogenous agonists of the AhR (97). In vitro experiments in human colon cancer cells, agonist stimulation of the AhR may mediate cancer cell cycle arrest (156). In contrast, bilirubin and biliverdin, as endogenous agonists of the AhR, may reduce the proliferation of cancer cells due to the AhR stimulation.
PPARs are multi-structural domain proteins belonging to the nuclear receptor superfamily, acting mainly as ligand-activated transcription factors (157). PPARα can upregulate genes involved in fatty acid transport, as well as the fatty acid β-oxidation process related to the mitochondria and peroxisomal, to facilitate the intake, utilization, and catabolism of fatty acid (13). Experimental studies carried out in rodents have also shown that bilirubin reduces liver fat accumulation by binding to PPARα (13, 158–160), and the mechanism behind this may be an increase in both number and function of mitochondria, leading to a reduction in lipid accumulation (161–163). Several studies in different human patient populations have also demonstrated that bilirubin levels in serum, especially direct bilirubin, are negatively correlated with the prevalence of nonalcoholic steatohepatitis (64, 164–167). Because bilirubin protects the liver by reducing the incidence of nonalcoholic steatohepatitis, which another study showed may progress to cirrhosis and HCC (168, 169), bilirubin may inhibit cancer by binding to PPARα.
CAR and FABPs are two cellular receptors that are capable of binding to and exerting effects on bilirubin, and the mechanisms of action of both are related to PPARα as mentioned above. Firstly, CAR is introduced, belonging to the nuclear receptor superfamily (170), and is enriched in the liver (171). It has been shown that intracellular bilirubin has ligand transcription factors for CAR (172, 173), which may enhance its transcriptional levels. In contrast, CAR crosstalks with peroxisome proliferator-activated receptor (PPAR) (174), and this crosstalk may allow bilirubin to reduce the incidence of nonalcoholic steatohepatitis through the interaction between CAR and PPAR (175), which in turn reduces the incidence of HCC. Then there are FABPs, FABPs are a class of low molecular weight intracellular proteins that bind efficiently to bilirubin (176) and deliver it to the PPAR in the nucleus (177), and this delivering action contributes to bilirubin’s binding to and functioning on the PPAR. In contrast, bilirubin, which is a strong regulator of PPARα (13) and PPARγ (178), can also trigger the expression of FABP1 through the regulation of PPAR. In studies of cancers such as HCC (179, 180) and colorectal cancer (181, 182), it has also been found that a high incidence of cancer is correlated with low expression of FABP1, which might be associated with the bilirubin-FABP1 interactions described previously (Figure 5).
Figure 5. Bilirubin acts as a ligand that binds to cellular receptors and produces oncogenic effects: 1. Bilirubin activates PPARα via two pathways to inhibit fat accumulation and thus related cancers. (1) Bilirubin is delivered to the nucleus by binding to FABP1 thereby binding to PPARα. (2) Bilirubin inhibits fat accumulation by promoting CAR expression and thereby contributing to its crosstalk with PPARα. 2. Bilirubin binds to AhR and promotes cell cycle arrest thereby inhibiting cancer.
When at high values in the normal range, bilirubin may inhibit cancer, but bilirubin may promote cancer under certain circumstances. For instance, a study in 2024 showed that when the concentration of bilirubin in serum reaches a certain value, the antioxidant effect of bilirubin begins to reduce, and even induces oxidative stress and thus promotes the development of cancer (183). In other studies, bilirubin has shown some promoting effects in cancers such as the cancers in the liver (18, 184) and lung (185). This indicates that the relationship between bilirubin levels and cancer risk is not simply linear and that both too low and too high concentrations may increase cancer risk (22, 186, 187). For the use of bilirubin in cancer therapy, research on the dual effects of bilirubin on cancer is a must, fewer studies have been conducted on its cancer-promoting mechanisms. The cancer-promoting effects of bilirubin may work through the following mechanisms.
Bilirubin can have a promoting effect on some sites of the immune system and thus on tumors. As shown in an earlier publication, unconjugated bilirubin stimulates microglia primary cultures to respond by acquiring a phagocytic phenotype, which is transformed into an inflammatory response featuring secretion of the pro-inflammatory cytokines interleukin (IL)-1β, IL-6, and tumor necrosis factor (TNF)-α, increased regulation of cyclooxygenase (COX)-2, and enhanced the activities of MMP- 9 and -2 (188). In another study, an ϵ-polylysine-bilirubin (PLL-BR) coupling was designed and synthesized to act as a method for bilirubin solubility and delivery. In vitro experiments, it enhanced M2-type macrophage polarization, resulting in elevated cytoprotective effects against antioxidant and inflammatory conditions; in vivo experiments in diabetic mice, compared to untreated islets, PLL-BR-coated islets led to the induction of an anti-inflammatory response featuring higher M2 macrophage markers levels and localized vascularization (189).
In the above description, cancer development has been associated with inflammation in several ways, and the narrative here suggests that bilirubin can also promote inflammation in several ways that may promote cancer. Whereas M2 macrophages can exert cancer-promoting effects in several ways, such as directly promoting cancer cell metastasis (190), promoting angiogenesis (191), and inhibiting tumor-killer cell activity (192), this suggests that bilirubin may promote cancer through this mechanism.
Although it has been shown that bilirubin is mainly inhibitory in its effect on the proliferation of tumors, it can also exhibit promotional properties in some aspects, and further studies on its inhibitory properties will help to generate new therapies for tumors, while studies on its promotional properties can give us a better knowledge of the two-faced nature of bilirubin, which can be used to aid in the side-by-side development of new treatments for tumors.
As mentioned above, bilirubin is a substance with two sides: it is cytotoxic at high concentrations. Still, when bilirubin is only mildly elevated, such as in GS, bilirubin substantially benefits humans in maintaining good health and reducing the incidence of various oxidative stress-mediated diseases(including tumors). That is why there have been attempts to increase bilirubin levels in the body (mimicking the GS state) to prevent or treat cancer. Here are a few ways to increase the concentration of bilirubin in your body.
Since bilirubin-like structures in plants share similar structure and function with bilirubin, complementing natural bilirubin-like structures through dietary supplementation is an effective way to elevate bilirubin levels, and one of the inexpensive and most studied bilirubin-like structures is Phycocyanobilin (PCB).PCB is a natural blue-coloring chromone with a linear tetrapyrrole structure in cyanobacteria and red algae and binds to phycocyanin (PC). It has antioxidant, anti-inflammatory, immunomodulatory, and anticancer activities due to its similarity to biliverdin or bilirubin (193). Currently, several animal experiments are proving the efficacy of PC in preventing or treating various diseases, as well as clinical trials of PC in cancer.
Spirulina, a cyanobacterium with various nutritional and therapeutic properties (194), is an important PC and allophycocyanin (APC) source in the phycobiliprotein family. There is research proving that the proliferation of pancreatic cancer cells was greatly inhibited in vitro by PC purified from Spirulina and that the inhibitory effect on the growth of a broad-spectrum cancer cell was enhanced with increasing doses of PC (195). Yang et al. found that the consumption of Spirulina by cancer patients during the first two cycles of chemotherapy increased IgM levels and CD8+T cell counts in vivo. This suggests that spirulina can reduce the incidence of myelosuppression and enhance immune function in tumor patients (196). Another study indicated that taking Spirulina reduced HBsAg levels in patients with chronic hepatitis B who were on continuous nucleoside analogs, and relieved liver inflammation, hepatic steatosis, and cirrhosis, thereby potentially lowering the probability of HCC (197). The above experiments show that PC has a widely suppressive effect on various tumor cells, which has crucial clinical significance.
Therefore, scientists’ research has become the direction of isolating and purifying PCB from natural algae to obtain a high concentration and maintain biological activity. Brião et al. produced food-grade PCs from spirulina by increasing the purity of PCs from 0.53 to 0.76 using phosphate buffer extraction combined with ultrafiltration and diafiltration (198). Furthermore, an investigator conducted ultrafiltration experiments using a 0.02g/mL Spirulina water extract sample. The purification/fractionation steps were carried out utilizing a polyethersulfone membrane. After the procedure, the purity of the rough extract improved from 0.74 to 0.93 due to the elimination of approximately 91.7% of the DNA, and the purification procedure was enhanced by applying six percolation cycles, forming the PC extract whose purity is up to 1.16, making it more favorable for food intake and biomedical applications (199).
In addition, it has been shown that the addition of 3 mM succinic acid to Spirulina medium increased biomass production rates to 164.05 mg/L(2-fold higher than the control)and increased PC production rates to 26.70 mg/L(3-fold higher than the control) (200). Moreover, the use of organic acids not only boosted the thermal stability of the PC but also increased the purity to 2.2.
It remains a challenge to develop a green and sustainable method to purify PCBs from algae to obtain high purity, suitable for biomedical applications.
Various drugs can affect key enzymes in bilirubin metabolism, resulting in mildly elevated serum bilirubin levels (mimicking a GS state) (Figure 6). This can potentially prevent and treat cancer to some extent. In addition, pharmacologic hyperbilirubinemia is typically benign and reversible, without underlying severe liver lesions such as cholestasis or hepatocyte necrosis. Therefore, drug induction can be considered a safe and reliable approach.
Figure 6. Targets of mildly elevated bilirubin concentrations induced by various drugs: The sharp red arrow suggests a promoting effect, while the blunt red one indicates inhibition. Class A drugs act by inhibiting OATP1B1, preventing the entry of UCB into the hepatocyte. Class B drugs inhibit OATP1B1, OATP1B3, and UGT1A1.Class C drugs inhibit UGT1A1 which affects the aldolization of bilirubin. Class D drugs inhibit bilirubin excretion through MRP2.Class E drugs induce HO-1 expression to promote bilirubin production.
As is well known, ursodeoxycholic acid can hinder UCB from entering liver cells for transformation by inhibiting OATP1B1, leading to a notable increase in bilirubin concentration. A randomized controlled trial showed that low-dose ursodeoxycholic acid (500 mg) increased serum bilirubin concentrations by 30% (201). Furthermore, it has been reported that the use of ursodeoxycholic acid seems to have a positive influence on the prevention of radiation-induced liver disease after radioembolization in patients with liver metastases from breast cancer (202).
Atazanavir is an HIV protease inhibitor. A retrospective cohort study of 1,020 HIV patients demonstrated that HIV patients taking atazanavir had mildly increased serum bilirubin levels and a significantly decreased risk of developing new-onset cardiovascular disease (203). This effect appears to be reliably induced by indirect hyperbilirubinemia mediated by competitive inhibition of UGT1A1, which induces a Gilbert phenotype. In addition, atazanavir also inhibits OATP1B1 and OATP1B3, and indinavir, amprenavir, saquinavir, rifampicin, cyclosporin A, and rifamycin SV have the same mechanism (204, 205).
The suppression function of octreotide on OATP1B1-mediated transport is stronger, while it exhibits weaker inhibitory effects on OATP1B3 and MRP2, which is consistent with clinical observations. Hyperbilirubinemia occurs in a small portion of patients accepting octreotide treatment (206). If properly applied, it may inhibit cancer development by mimicking the GS state.
Clinically widely used NSAIDs, such as celecoxib (207) or acetylsalicylic acid (208), can induce HO-1. According to reports, after 24 hours of treatment with celecoxib, an increase in its concentration can enhance the levels of HO-1 mRNA and protein expression in endothelial cells of humans, and the bilirubin content reaches its highest level after 48 hours of celecoxib treatment.
A Mendelian randomization analysis study (209) detected that lowering LDL-C and PCSK9 protein levels by PCSK9 variants was relevant to an increase in CB, demonstrating that direct bilirubin is increased with the use of newer lipid-lowering therapies like the preprotein convertase chytokeratase/kexin type 9 (PCSK9), monoclonal antibody inhibitors: alirocumab and evolocumab, and hepatic PCSK9 siRNA inhibitor inclisiran. PCSK9 is inversely related to liver function and bilirubin (210, 211). Similarly, the drugs of the fibrates (212, 213) upregulate the expression of HO-1 mRNA and protein to increase bilirubin levels. Whereas statins (214–216), and niacin (217) in addition to the HO-1 induction mentioned above, include inhibition of basolateral transport of unconjugated bilirubin, the latter may be more important.
Kinase inhibitors such as erlotinib, nilotinib, pazopanib, regorafenib, sorafenib, and verofenib inhibit UGT1A1. Since the clinical steady-state Cmax concentration is higher than their IC50, these TKI agents can make patients with a high incidence of hyperbilirubinemia by effectively inhibiting UGT1A1 (218, 219). In addition, it has been shown that most of the FDA-approved tyrosine kinases (TKIs) dramatically inhibit OATP1B1 for its function.
Irinotecan (220), methotrexate (221), cyclosporine A (222), and rifampicin (204) share similar UGT1A1 inhibitory activity in the treatment of cancer.
It is worth noting that hyperbilirubinemia caused by a variety of drugs is often seen as a symbol of deferred drug biotransformation and signs of drug-induced hepatic injury, so care should be taken to control the bilirubin concentration during the clinical application of drugs to induce a GS state; excessively high levels may be counterproductive. Bilirubin concentration levels and drug side effects should still be monitored several times while using these drugs.
Tumors generate large amounts of ROS during rapid metabolic proliferation, which can promote tumor cell survival, invasion, proliferation, and metastasis by regulating various signaling pathways (223, 224). Therefore, bilirubin can exert anticancer effects by scavenging reactive oxygen radicals in cancer cells. Bilirubin nanoparticles (BRNPs) can preferentially accumulate in tumors due to enhanced permeability and retention (EPR) effects (225). In addition, bilirubin’s rapid ROS and light response, efficient photothermal conversion, biodegradability, and biocompatibility make it more suitable than other liposomes as a carrier for encapsulating anticancer drugs (Table 1).
Bilirubin is insoluble in water because of the formation of intramolecular hydrogen bonds by its hydrophilic groups (carboxylic acid and amide bonds). However, bilirubin can target ROS and be oxidized to biliverdin, resulting in increased water solubility. When irradiated with light of a suitable wavelength, bilirubin can also occur in photoisomerization (through intramolecular hydrogen bonds breaking) and be converted to the more water-soluble photoisomer, which is readily disposed of and eliminated by the liver and kidneys (226, 235). This ability to respond to both ROS and external light stimuli demonstrates that bilirubin can be used as a multi-stimulus response system to construct nanomedicines.
In 2016, Lee et al. first developed BRNPs for cancer therapy (226). They combined bilirubin and polyethylene glycol into a coupling via a steady amide bond and loaded the anticancer drug doxorubicin (DOX) into BRNPs via film-forming and rehydration to form DOX-loaded BRNPs (DOX@BRNPs). In vivo, experiments in xenograft mice bearing human lung adenocarcinoma cells indicated that tumor growth was restrained by 27.8% in mice treated with free DOX, 55.0% in the DOX@BRNPs group, and even as high as 71.9% in the laser-irradiated DOX@BRNPs group. This indicates that the use of BRNPs can greatly enhance the anti-tumor efficacy of DOX chemotherapy drugs. In addition, 38.1% of tumor growth was inhibited when treated with BRNPs individually, suggesting that BRNPs, as well as free bilirubins, also have inherent anticancer efficacy.
Another interesting experiment was published (227). The bilirubin-chitosan conjugate (BR-Chitosan) was labeled with Sulfo-Cyanine7 fluorescent dye and injected into HeLa tumor-bearing mice. Eight hours later, it primarily accumulated in the tumor region, whereas free Sulfo-Cyanine7 exhibited a systemic distribution. Experimental results indicate that BR-Chitosan exhibits significant tumor-targeting properties. In an exception to the catabolism of the BR-Chitosan at the tumor site to release the internal hypoxia-activated prodrug HAPTH-302, it also exhibited intrinsic oxygen-consuming properties in the tumor region in the presence of overexpression of ROS, including H2O2, which greatly increased the degree of hypoxia around the tumor tissues and converted HAPTH-302 from nontoxic to toxic in a hypoxic environment, exerting selective antitumor effects. Bilirubin not only compensates for the limitation of HAPTH-302’s inability to function in the region of normoxic tumors but also has a strong absorbance that allows for chemo-thermal synergistic treatment. It also has a simpler and safer composition than other deoxidizers and carriers. In HeLa tumor-bearing mice, drug cytotoxicity was significantly increased and tumor hypoxia was enhanced.
To enhance the antitumor effects, Lee et al. sought to further refine BRNPs by incorporating immune responses. Hyaluronic acid (HA) has targeted CD44 and immunomodulatory properties. Recently, they developed a hyaluronic acid-bilirubin nanoparticles loaded with SC144(SC144@HABN) (228). Experimental data showed that intravenously injected HABN accumulated in tumor cells and tumor-associated myeloid cells, (possibly as a result of the enhanced permeability and retention effect and targeting of CD44, ROS effect) allowing SC144 to act: radicalizing macrophages into the M1 phenotype, inducing killing effect of CD8+ T cells, enhanced PD-L1 expression in tumor cells and inducing immunogenic cell death in vitro. The significant tumor-targeting and lysis-releasing effects of HABN vectors attenuated the toxicity of SC144 on human normal cells and CD8+ T cells, and enhanced the synergistic anti-cancer efficacy of immunotherapy. In addition, Animal experiments have shown that the SC144@HABN+anti-PD-L1 combination treatment can complementarily increase anti-neoplastic efficiency and effectively eliminate MC38 colon tumors and immune checkpoint blockade-resistant 4T1 breast tumors. Subsequently, articles have also reported that HABN containing adriamycin (DOX@HABN) showed significant tumor targeting and synergistic anti-tumor capabilities in HeLa-loaded mice (229).
In addition, a Ce6/BR-FFVLK-PEG nanomedicine encapsulating dimeric paclitaxel (PTX) & indolimod (IND) was reported (230) Its surface-covered macrophage membrane facilitates nanomedicine circulation and tumor targeting. Chlorin e6 (Ce6) or BR can generate ROS under laser beam irradiation, which triggers the conversion of micelles into nanofibers and facilitates the release of core anticancer agents. Growth and lung metastasis of breast cancer in situ were considerably inhibited by this nanomedicine through BR-induced photodynamic therapy, PTX-induced typical immunogenic cell death (ICD) effect, and activation of immune response in combination with IND.
Due to bilirubin’s high photosensitivity (PS), blue light is often utilized to reduce unconjugated bilirubin levels in neonates or preterm infants. When laser light irradiates accumulated PS reagents (e.g., bilirubin), photoactivation generates toxic ROS and induces cell death to provide local antitumor therapy. Moreover, the liganding of bilirubin with particular metal ions may enhance its effectiveness in photoacoustic imaging (PAI) and photothermal therapy (PTT).
Inspired by the binding mode of melanin gallstones, Lee et al. exploited cisplatin-chelated bilirubin nanoparticles (cisPt@BRNPs) (231). They found that under laser irradiation, with its great photothermal conversion efficiency, it could generate considerable heat, causing cell death in more than 70% of HT-29 human colorectal adenocarcinoma cells (while cisplatin exerted a small anti-tumor effect). Interestingly, substantial photoacoustic activity was generated by cisPt@BRNPs, enabling tumor visualization, which can be used as a PAI probe for cancer diagnosis and treatment.
Because of the shallow depth of light penetration in the tissue, it cannot treat tumors deeper in. So novel PEGylated bilirubin-encapsulated CaWO4 nanoparticles(PEG-BR/CWO NPs) were reported by Pizzuti et al (232). When the nanoparticles were irradiated by X-rays, the CaWO4 core emitted ultraviolet and blue light, which was absorbed by bilirubin to produce a photodynamic effect, generating ROS to enhance cancer cell death. Animal studies of head and neck cancer transplants have shown that combining radiation and photodynamic therapy enhances the anti-cancer treatment effect.
Recently, Mn2+-chelated, biotinylated bilirubin nanoparticles (Mn@bt-BRNPs) were reported. Unlike conventional MR contrast agents, BRNPs release internal Mn2+ in response to ROS stimulation, which allows for better display of ROS in the tumor and tumor microenvironment, thereby inducing T1-weighted MRI signal enhancement. Moreover, experimental data indicated that adriamycin-loaded Mn@bt-BRNPs could effectively inhibit the growth of A549 lung cancer cells (233). This combined antitumor effect and tumor visualization in one of the BRNPs is a significant advantage of them as nanocarriers. Similar bilirubin nanomedicines have been reported to monitor the progression of cirrhosis (236).
In addition, BV nanoparticles with long-term fixity were constructed by Xing et al. through supramolecular self-assembly of BV and metal-binding short peptides. Metal ions, being incorporated into the BV nanoparticles such as Mn2+, can strengthen their photostability and endow them with magnetic resonance. Data from in vivo studies show that its selective accumulation in tumors can locally raise the temperature of tumors under near-infrared illumination, thus leading to the induction of effective photothermal tumor ablation for selective treatment (234). In addition, BV nanoparticles can also be used as multimodal contrast agents for better imaging of tumors in ultrasound and magnetic resonance, thus having great potential in precision medicine.
It is worth noting that the anticancer and immunomodulatory role of bilirubin in nanomedicines was overlooked in some of the above experiments, which focused on its high photothermal conversion efficiency as a photosensitizer on tumors, without noting that the anticancer role of bilirubin/biliverdin as a ROS scavenger in its own right should not be underestimated. Moreover, BRNPs have better efficacy in ROS-overproducing tumor cells than in ROS-low-expressing tumors. The therapeutic efficacy of passively targeted BRNPs dependent on the EPR effect is largely influenced by differences in the strength of the EPR effect exhibited within different tumors (225). It remains a challenge to effectively utilize the benefits of bilirubin to target tumor cells highly selectively.
Bilirubin has a complex interaction with cancer, and bilirubin can inhibit tumorigenesis and development through a variety of mechanisms, including influencing cellular redox reactions, signaling, and the immune system, while the effects of cancer on bilirubin have derived several prognostic models. Current research on the effects of bilirubin on tumors has some contradictory results and perhaps unexplored underlying mechanisms. Some studies have found antiproliferative effects of bilirubin in specific human tissue cells, but have not gone on to investigate its role in the corresponding tumors. The effect of bilirubin on the intestinal flora, which plays a key role in its metabolism, has also not been explored.
Dietary supplementation with PC and drug induction can elevate serum bilirubin concentrations, but only modest elevations are strongly associated with reduced cancer incidence. In constructing BRNPs, bilirubin is a versatile carrier for antitumor drugs and a photosensitizer for diagnosing and treating cancer, enabling better imaging of tumors. However, the antitumor effects of bilirubin itself were overlooked in most experiments. The efficacy of BRNPs in ROS overproducing tumors was better than that in ROS low-expressing tumors, which might be the limitation of BRNPs. Despite this, bilirubin-targeted nanomedicines remain a suitable approach for future cancer prevention and treatment.
FY: Writing – original draft, Conceptualization, Data curation, Formal analysis, Investigation, Methodology, Project administration, Software, Supervision. ST: Writing – original draft, Conceptualization, Data curation, Formal analysis, Investigation, Methodology, Project administration, Software, Supervision. HW: Writing – review & editing.
The author(s) declare that no financial support was received for the research and/or publication of this article.
The authors declare that the research was conducted in the absence of any commercial or financial relationships that could be construed as a potential conflict of interest.
The author(s) declare that no Generative AI was used in the creation of this manuscript.
All claims expressed in this article are solely those of the authors and do not necessarily represent those of their affiliated organizations, or those of the publisher, the editors and the reviewers. Any product that may be evaluated in this article, or claim that may be made by its manufacturer, is not guaranteed or endorsed by the publisher.
1. Sung H, Ferlay J, Siegel RL, Laversanne M, Soerjomataram I, Jemal A, et al. Global cancer statistics 2020: GLOBOCAN estimates of incidence and mortality worldwide for 36 cancers in 185 countries. CA Cancer J Clin. (2021) 71:209–49. doi: 10.3322/caac.21660
2. Vitek L, Hubacek JA, Pajak A, Doryńska A, Kozela M, Eremiasova L, et al. Association between plasma bilirubin and mortality. Ann Hepatol. (2019) 18:379–85. doi: 10.1016/j.aohep.2019.02.001
3. Aoyama T, Ju M, Machida D, Komori K, Tamagawa H, Tamagawa A, et al. Clinical impact of preoperative albumin-bilirubin status in esophageal cancer patients who receive curative treatment. In Vivo. (2022) 36:1424–31. doi: 10.21873/invivo.12847
4. Berk PD, Blaschke TF, Scharschmidt BF, Waggoner JG, Berlin NI. A new approach to quantitation of the various sources of bilrubin in man. J Lab Clin Med. (1976) 87:767–80.
5. Pimstone NR, Tenhunen R, Seitz PT, Marver HS, Schmid R. The enzymatic degradation of hemoglobin to bile pigments by macrophages. J Exp Med. (1971) 133:1264–81. doi: 10.1084/jem.133.6.1264
6. Wegiel B, Baty CJ, Gallo D, Csizmadia E, Scott JR, Akhavan A, et al. Cell surface biliverdin reductase mediates biliverdin-induced anti-inflammatory effects via phosphatidylinositol 3-kinase and Akt. J Biol Chem. (2009) 284:21369–78. doi: 10.1074/jbc.M109.027433
7. Kamisako T, Kobayashi Y, Takeuchi K, Ishihara T, Higuchi K, Tanaka Y, et al. Recent advances in bilirubin metabolism research: the molecular mechanism of hepatocyte bilirubin transport and its clinical relevance. J Gastroenterol. (2000) 35:659–64. doi: 10.1007/s005350070044
8. Vítek L, Majer F, Muchová L, Zelenka J, Jirásková A, Branný P, et al. Identification of bilirubin reduction products formed by Clostridium perfringens isolated from human neonatal fecal flora. J Chromatography. B Analytical Technol In Biomed Life Sci. (2006) 833:149–57. doi: 10.1016/j.jchromb.2006.01.032
9. Fujii M, Inoguchi T, Sasaki S, Maeda Y, Zheng J, Kobayashi K, et al. Bilirubin and biliverdin protect rodents against diabetic nephropathy by downregulating NAD(P)H oxidase. Kidney Int. (2010) 78:905–19. doi: 10.1038/ki.2010.265
10. Kim TS, Pae CU, Yoon SJ, Jang WY, Lee NJ, Kim JJ, et al. Decreased plasma antioxidants in patients with Alzheimer’s disease. Int J Geriatr Psychiatry. (2006) 21:344–8. doi: 10.1002/gps.1469
11. Kwon KM, Kam JH, Kim MY, Kim MY, Chung CH, Kim JK, et al. Inverse association between total bilirubin and metabolic syndrome in rural korean women. J Womens Health (Larchmt). (2011) 20:963–9. doi: 10.1089/jwh.2010.2453
12. Lin LY, Kuo HK, Hwang JJ, Lai LP, Chiang FT, Tseng CD, et al. Serum bilirubin is inversely associated with insulin resistance and metabolic syndrome among children and adolescents. Atherosclerosis. (2009) 203:563–8. doi: 10.1016/j.atherosclerosis.2008.07.021
13. Stec DE, John K, Trabbic CJ, Luniwal A, Hankins MW, Baum J, et al. Bilirubin binding to PPARα Inhibits lipid accumulation. PLoS One. (2016) 11:e0153427. doi: 10.1371/journal.pone.0153427
14. Weaver L, Hamoud AR, Stec DE, . Hinds TD Jr. Biliverdin reductase and bilirubin in hepatic disease. Am J Physiol Gastrointest Liver Physiol. (2018) 314:G668–g676. doi: 10.1152/ajpgi.00026.2018
15. Vítek L. The role of bilirubin in diabetes, metabolic syndrome, and cardiovascular diseases. Front Pharmacol. (2012) 3:55. doi: 10.3389/fphar.2012.00055
16. Wagner KH, Wallner M, Mölzer C, Gazzin S, Bulmer AC, Tiribelli C, et al. Looking to the horizon: the role of bilirubin in the development and prevention of age-related chronic diseases. Clin Sci (Lond). (2015) 129:1–25. doi: 10.1042/cs20140566
17. Sun H, He B, Nie Z, Pan Y, Lin K, Peng H, et al. A nomogram based on serum bilirubin and albumin levels predicts survival in gastric cancer patients. Oncotarget. (2017) 8:41305–18. doi: 10.18632/oncotarget.17181
18. Seyed Khoei N, Wagner K-H, Carreras-Torres R, Gunter MJ, Murphy N, Freisling H. Associations between prediagnostic circulating bilirubin levels and risk of gastrointestinal cancers in the UK biobank. Cancers. (2021) 13:2749. doi: 10.3390/cancers13112749
19. Alexandre J, Bleuzen P, Bonneterre J, Sutherland W, Misset JL, Guastalla J, et al. Factors predicting for efficacy and safety of docetaxel in a compassionate-use cohort of 825 heavily pretreated advanced breast cancer patients. J Clin Oncology: Off J Am Soc Clin Oncol. (2000) 18:562–73. doi: 10.1200/JCO.2000.18.3.562
20. Liu X, Meng QH, Ye Y, Hildebrandt MAT, Gu J, Wu X. Prognostic significance of pretreatment serum levels of albumin, LDH and total bilirubin in patients with non-metastatic breast cancer. Carcinogenesis. (2015) 36:243–8. doi: 10.1093/carcin/bgu247
21. Wyld L, Gutteridge E, Pinder SE, James JJ, Chan SY, Cheung KL, et al. Prognostic factors for patients with hepatic metastases from breast cancer. Br J Cancer. (2003) 89:284–90. doi: 10.1038/sj.bjc.6601038
22. Inoguchi T, Nohara Y, Nojiri C, Nakashima N. Association of serum bilirubin levels with risk of cancer development and total death. Sci Rep. (2021) 11:13224. doi: 10.1038/s41598-021-92442-2
23. Horsfall LJ, Burgess S, Hall I, Nazareth I. Genetically raised serum bilirubin levels and lung cancer: a cohort study and Mendelian randomisation using UK Biobank. Thorax. (2020) 75:955–64. doi: 10.1136/thoraxjnl-2020-214756
24. Freisling H, Seyed Khoei N, Viallon V, Wagner K-H. Gilbert’s syndrome, circulating bilirubin and lung cancer: a genetic advantage? Thorax. (2020) 75:916–7. doi: 10.1136/thoraxjnl-2020-215642
25. Horsfall LJ, Rait G, Walters K, Swallow DM, Pereira SP, Nazareth I, et al. Serum bilirubin and risk of respiratory disease and death. JAMA. (2011) 305:691–7. doi: 10.1001/jama.2011.124
26. Wen C-P, Zhang F, Liang D, Wen C, Gu J, Skinner H, et al. The ability of bilirubin in identifying smokers with higher risk of lung cancer: a large cohort study in conjunction with global metabolomic profiling. Clin Cancer Research: an Off J Am Assoc For Cancer Res. (2015) 21:193–200. doi: 10.1158/1078-0432.CCR-14-0748
27. Monroy-Iglesias MJ, Moss C, Beckmann K, Hammar N, Walldius G, Bosco C, et al. Serum total bilirubin and risk of cancer: A swedish cohort study and meta-analysis. Cancers. (2021) 13:5540. doi: 10.3390/cancers13215540
28. Li N, Xu M, Cai M-Y, Zhou F, Li C-F, Wang B-X, et al. Elevated serum bilirubin levels are associated with improved survival in patients with curatively resected non-small-cell lung cancer. Cancer Epidemiol. (2015) 39:763–8. doi: 10.1016/j.canep.2015.06.007
29. Wang Y, Yi C, Wang Y, Li H, Li B, Wang D, et al. Distribution of uridine diphosphate glucuronosyltransferase 1A polymorphisms and their role in irinotecan-induced toxicity in patients with cancer. Oncol Lett. (2017) 14:5743–52. doi: 10.3892/ol.2017.6933
30. Zucker SD, Horn PS, Sherman KE. Serum bilirubin levels in the U.S. population: gender effect and inverse correlation with colorectal cancer. Hepatol (Baltimore Md.). (2004) 40:827–35. doi: 10.1002/hep.20407
31. Liu C-Y, Chen P-M, Chiou T-J, Liu J-H, Lin J-K, Lin T-C, et al. UGT1A1*28 polymorphism predicts irinotecan-induced severe toxicities without affecting treatment outcome and survival in patients with metastatic colorectal carcinoma. Cancer. (2008) 112:1932–40. doi: 10.1002/cncr.23370
32. Jirásková A, Novotný J, Novotný L, Vodicka P, Pardini B, Naccarati A, et al. Association of serum bilirubin and promoter variations in HMOX1 and UGT1A1 genes with sporadic colorectal cancer. Int J Cancer. (2012) 131:1549–55. doi: 10.1002/ijc.27412
33. Yu Q-Q, Qiu H, Zhang M-S, Hu G-Y, Liu B, Huang L, et al. Predictive effects of bilirubin on response of colorectal cancer to irinotecan-based chemotherapy. World J Gastroenterol. (2016) 22:4250–8. doi: 10.3748/wjg.v22.i16.4250
34. Gao C, Fang L, Li J-T, Zhao H-C. Significance and prognostic value of increased serum direct bilirubin level for lymph node metastasis in Chinese rectal cancer patients. World J Gastroenterol. (2016) 22:2576–84. doi: 10.3748/wjg.v22.i8.2576
35. Lin R, Wang X, Wang Y, Zhang F, Wang Y, Fu W, et al. Association of polymorphisms in four bilirubin metabolism genes with serum bilirubin in three Asian populations. Hum Mutat. (2009) 30:609–15. doi: 10.1002/humu.20895
36. Deng Q, He B, Gao T, Pan Y, Sun H, Xu Y, et al. Up-regulation of 91H promotes tumor metastasis and predicts poor prognosis for patients with colorectal cancer. PLoS One. (2014) 9:e103022. doi: 10.1371/journal.pone.0103022
37. Deng Q, He B, Liu X, Yue J, Ying H, Pan Y, et al. Prognostic value of pre-operative inflammatory response biomarkers in gastric cancer patients and the construction of a predictive model. J Trans Med. (2015) 13:66. doi: 10.1186/s12967-015-0409-0
38. Boonpipattanapong T, Chewatanakornkul S. Preoperative carcinoembryonic antigen and albumin in predicting survival in patients with colon and rectal carcinomas. J Clin Gastroenterol. (2006) 40:592–5. doi: 10.1097/00004836-200608000-00006
39. Zhang X-J, Fei H, Sun C-Y, Li Z-F, Li Z, Guo C-G, et al. Novel prognostic score based on the preoperative total bilirubin-albumin ratio and fibrinogen-albumin ratio in ampullary adenocarcinoma. World J Gastrointestinal Surg. (2023) 15:2247–58. doi: 10.4240/wjgs.v15.i10.2247
40. Zhang Q, Ma X, Xu Q, Qin J, Wang Y, Liu Q, et al. Nomograms incorporated serum direct bilirubin level for predicting prognosis in stages II and III colorectal cancer after radical resection. Oncotarget. (2017) 8:71138–46. doi: 10.18632/oncotarget.11424
41. Li X-R, Zhang Q, Zhang K-P, Zhang X, Ruan G-T, Song M-M, et al. Association of serum total bilirubin with survival outcomes in patients with cancer cachexia: A prospective, multicenter cohort study. Nutr (Burbank Los Angeles County Calif.). (2022) 102:111711. doi: 10.1016/j.nut.2022.111711
42. Johnson PJ, Berhane S, Kagebayashi C, Satomura S, Teng M, Reeves HL, et al. Assessment of liver function in patients with hepatocellular carcinoma: a new evidence-based approach-the ALBI grade. J Clin Oncology: Off J Am Soc Clin Oncol. (2015) 33:550–8. doi: 10.1200/JCO.2014.57.9151
43. Kut E, Menekse S. Prognostic significance of pretreatment albumin-bilirubin (ALBI) grade and platelet-albumin-bilirubin (PALBI) grade in patients with small cell lung cancer. Sci Rep. (2024) 14:1371. doi: 10.1038/s41598-024-51375-2
44. Joo J, Jeon H, Kim D, Kim W, Nam J, Kim D, et al. Predictive power of the albumin-bilirubin score for hepatotoxicity in stereotactic ablative radiation therapy for hepatocellular carcinoma. Cancers. (2023) 15:3777. doi: 10.3390/cancers15153777
45. Jackson WC, Hartman HE, Gharzai LA, Maurino C, Karnak DM, Mendiratta-Lala M, et al. The potential for midtreatment albumin-bilirubin (ALBI) score to individualize liver stereotactic body radiation therapy. Int J Radiat Oncology Biology Phys. (2021) 111:127–34. doi: 10.1016/j.ijrobp.2021.04.012
46. Yoshino Y, Suzuki G, Shiomi H, Kimoto T, Seri S, Yamazaki H, et al. Albumin-bilirubin score is a useful predictor of worsening liver reserve after stereotactic body radiation therapy in elderly Japanese patients with hepatocellular carcinoma. J Radiat Res. (2024) 65:244–50. doi: 10.1093/jrr/rrae006
47. Kitahama T, Ishii K, Haneda R, Inoue M, Mayanagi S, Tsubosa Y. Clinical significance of albumin-bilirubin grade in thoracic esophageal squamous cell carcinoma. J Surg Res. (2024) 295:673–82. doi: 10.1016/j.jss.2023.08.056
48. Kariyama K, Nouso K, Hiraoka A, Wakuta A, Oonishi A, Kuzuya T, et al. EZ-ALBI score for predicting hepatocellular carcinoma prognosis. Liver Cancer. (2020) 9:734–43. doi: 10.1159/000508971
49. Yang C, Wu X, Liu J, Wang H, Jiang Y, Wei Z, et al. Nomogram based on platelet-albumin-bilirubin for predicting tumor recurrence after surgery in alpha-fetoprotein-negative hepatocellular carcinoma patients. J Hepatocellular Carcinoma. (2023) 10:43–55. doi: 10.2147/JHC.S396433
50. Pang Q, Liu S, Wang L, Pan H, Wang C, Zhou L, et al. The significance of platelet-albumin-bilirubin (PALBI) grade in hepatocellular carcinoma patients stratified according to platelet count. Cancer Manage Res. (2020) 12:12811–22. doi: 10.2147/CMAR.S277013
51. Morandi A, Risaliti M, Montori M, Buccianti S, Bartolini I, Moraldi L. Predicting post-hepatectomy liver failure in HCC patients: A review of liver function assessment based on laboratory tests scores. Medicina (Kaunas Lithuania). (2023) 59:1099. doi: 10.3390/medicina59061099
52. Ho S-Y, Liu P-H, Hsu C-Y, Huang Y-H, Liao J-I, Su C-W, et al. Comparison of four albumin-based liver reserve models (ALBI/EZ-ALBI/PALBI/PAL) against MELD for patients with hepatocellular carcinoma undergoing transarterial chemoembolization. Cancers. (2023) 15:1925. doi: 10.3390/cancers15071925
53. Gupte A, Mumper RJ. Elevated copper and oxidative stress in cancer cells as a target for cancer treatment. Cancer Treat Rev. (2009) 35:32–46. doi: 10.1016/j.ctrv.2008.07.004
54. Rizvi A, Farhan M, Nabi F, Khan RH, Adil M, Ahmad A. Transcriptional control of the oxidative stress response and implications of using plant derived molecules for therapeutic interventions in cancer. Curr Medicinal Chem. (2021) 28:8480–95. doi: 10.2174/0929867328666210218110550
55. Wang Y, Qi H, Liu Y, Duan C, Liu X, Xia T, et al. The double-edged roles of ROS in cancer prevention and therapy. Theranostics. (2021) 11:4839–57. doi: 10.7150/thno.56747
56. Xia C, Meng Q, Liu L-Z, Rojanasakul Y, Wang X-R, Jiang B-H. Reactive oxygen species regulate angiogenesis and tumor growth through vascular endothelial growth factor. Cancer Res. (2007) 67:10823–30. doi: 10.1158/0008-5472.CAN-07-0783
57. Tomaro ML, Batlle AMDC. Bilirubin: its role in cytoprotection against oxidative stress. Int J Biochem Cell Biol. (2002) 34:216–20. doi: 10.1016/S1357-2725(01)00130-3
58. Sedlak TW, Saleh M, Higginson DS, Paul BD, Juluri KR, Snyder SH. Bilirubin and glutathione have complementary antioxidant and cytoprotective roles. Proc Natl Acad Sci United States America. (2009) 106:5171–6. doi: 10.1073/pnas.0813132106
59. Stocker R, Yamamoto Y, McDonagh AF, Glazer AN, Ames BN. Bilirubin is an antioxidant of possible physiological importance. Sci (New York N.Y.). (1987) 235:1043–6. doi: 10.1126/science.3029864
60. Stocker R, Glazer AN, Ames BN. Antioxidant activity of albumin-bound bilirubin. Proc Natl Acad Sci United States America. (1987) 84:5918–22. doi: 10.1073/pnas.84.16.5918
61. Nam J, Lee Y, Yang Y, Jeong S, Kim W, Yoo J-W, et al. Is it worth expending energy to convert biliverdin into bilirubin? Free Radical Biol Med. (2018) 124:232–40. doi: 10.1016/j.freeradbiomed.2018.06.010
62. Roberts CK, Sindhu KK. Oxidative stress and metabolic syndrome. Life Sci. (2009) 84:705–12. doi: 10.1016/j.lfs.2009.02.026
63. Kim AH, Son D-H, Moon M-E, Jeon S, Lee HS, Lee Y-J. Sex differences in the relationship between serum total bilirubin and risk of incident metabolic syndrome in community-dwelling adults: Propensity score analysis using longitudinal cohort data over 16 years. Cardiovasc Diabetol. (2024) 23:92. doi: 10.1186/s12933-024-02182-6
64. Liang C, Yu Z, Bai L, Hou W, Tang S, Zhang W, et al. Association of serum bilirubin with metabolic syndrome and non-alcoholic fatty liver disease: A systematic review and meta-analysis. Front In Endocrinol. (2022) 13:869579. doi: 10.3389/fendo.2022.869579
65. Hao H, Guo H, Ma R-L, Yan Y-Z, Hu Y-H, Ma J-L, et al. Association of total bilirubin and indirect bilirubin content with metabolic syndrome among Kazakhs in Xinjiang. BMC Endocrine Disord. (2020) 20:110. doi: 10.1186/s12902-020-00563-y
66. Liu T, Liu C-A, Zhang Q-S, Zhang Q, Wang Y-M, Song M-M, et al. Association of the age of onset of metabolic syndrome with the risk of all cancer types. Diabetes Metab Syndrome. (2023) 17:102896. doi: 10.1016/j.dsx.2023.102896
67. Zhang Z, Liu Q, Huang C, Wu J, Wen Y. Association between metabolic syndrome and the risk of lung cancer: A meta-analysis. Hormone Metab Res = Hormon- Und Stoffwechselforschung = Hormones Et Metabolisme. (2023) 55:846–54. doi: 10.1055/a-2179-0809
68. Zhang C, Zhang L, Tian Y, Guan B, Li S. Association between metabolic syndrome and early-stage colorectal cancer. BMC Cancer. (2023) 23:1020. doi: 10.1186/s12885-023-11537-3
69. Navarro J, Obrador E, Carretero J, Petschen I, Aviñó J, Perez P, et al. Changes in glutathione status and the antioxidant system in blood and in cancer cells associate with tumour growth in vivo. Free Radical Biol Med. (1999) 26:410–8. doi: 10.1016/S0891-5849(98)00213-5
70. Ho JC, Chan-Yeung M, Ho SP, Mak JC, Ip MS, Ooi GC, et al. Disturbance of systemic antioxidant profile in nonsmall cell lung carcinoma. Eur Respir J. (2007) 29:273–8. doi: 10.1183/09031936.00000106
71. Güven M, Oztürk B, Sayal A, Ozet A. Lipid peroxidation and antioxidant system in the blood of patients with Hodgkin’s disease. Clin Biochem. (2000) 33:209–12. doi: 10.1016/s0009-9120(00)00062-x
72. Aydin A, Arsova-Sarafinovska Z, Sayal A, Eken A, Erdem O, Erten K, et al. Oxidative stress and antioxidant status in non-metastatic prostate cancer and benign prostatic hyperplasia. Clin Biochem. (2006) 39:176–9. doi: 10.1016/j.clinbiochem.2005.11.018
73. Senthil K, Aranganathan S, Nalini N. Evidence of oxidative stress in the circulation of ovarian cancer patients. Clinica Chimica Acta; Int J Clin Chem. (2004) 339:27–32. doi: 10.1016/j.cccn.2003.08.017
74. Beevi SS, Rasheed MH, Geetha A. Evidence of oxidative and nitrosative stress in patients with cervical squamous cell carcinoma. Clinica Chimica Acta; Int J Clin Chem. (2007) 375:119–23. doi: 10.1016/j.cca.2006.06.028
75. Batcioglu K, Mehmet N, Ozturk IC, Yilmaz M, Aydogdu N, Erguvan R, et al. Lipid peroxidation and antioxidant status in stomach cancer. Cancer Invest. (2006) 24:18–21. doi: 10.1080/07357900500449603
76. Fruehauf JP, Meyskens FL. Reactive oxygen species: a breath of life or death? Clin Cancer Research: an Off J Am Assoc For Cancer Res. (2007) 13:789–94. doi: 10.1158/1078-0432.ccr-06-2082
77. Siddiqui WA, Ahad A, Ahsan H. The mystery of BCL2 family: Bcl-2 proteins and apoptosis: an update. Arch Toxicol. (2015) 89:289–317. doi: 10.1007/s00204-014-1448-7
78. Adams JM, Cory S. Bcl-2-regulated apoptosis: mechanism and therapeutic potential. Curr Opin In Immunol. (2007) 19:488–96. doi: 10.1016/j.coi.2007.05.004
79. Reed JC. Proapoptotic multidomain Bcl-2/Bax-family proteins: mechanisms, physiological roles, and therapeutic opportunities. Cell Death Differentiation. (2006) 13:1378–86. doi: 10.1038/sj.cdd.4401975
80. Gross A, Jockel J, Wei MC, Korsmeyer SJ. Enforced dimerization of BAX results in its translocation, mitochondrial dysfunction and apoptosis. EMBO J. (1998) 17:3878–85. doi: 10.1093/emboj/17.14.3878
81. Antonsson B, Montessuit S, Sanchez B, Martinou JC. Bax is present as a high molecular weight oligomer/complex in the mitochondrial membrane of apoptotic cells. J Biol Chem. (2001) 276:11615–23. doi: 10.1074/jbc.M010810200
82. Zha H, Aimé-Sempé C, Sato T, Reed JC. Proapoptotic protein Bax heterodimerizes with Bcl-2 and homodimerizes with Bax via a novel domain (BH3) distinct from BH1 and BH2. J Biol Chem. (1996) 271:7440–4. doi: 10.1074/jbc.271.13.7440
83. NaveenKumar SK, Thushara RM, Sundaram MS, Hemshekhar M, Paul M, Thirunavukkarasu C, et al. Unconjugated Bilirubin exerts Pro-Apoptotic Effect on Platelets via p38-MAPK activation. Sci Rep. (2015) 5:15045. doi: 10.1038/srep15045
84. Shahrokhi SZ, Tehrani FSK, Salami S. Molecular mechanisms of bilirubin induced G1 cell cycle arrest and apoptosis in human breast cancer cell lines: involvement of the intrinsic pathway. Mol Biol Rep. (2022) 49:10421–9. doi: 10.1007/s11033-022-07757-8
85. Niknam M, Maleki MH, Khakshournia S, Rasouli M, Vakili O, Shafiee SM. Bilirubin, an endogenous antioxidant that affects p53 protein and its downstream apoptosis/autophagy-related genes in LS180 and SW480 cell culture models of colorectal cancer. Biochem Biophys Res Commun. (2023) 672:161–7. doi: 10.1016/j.bbrc.2023.06.050
86. Mirzaei S, Saghari S, Bassiri F, Raesi R, Zarrabi A, Hushmandi K, et al. NF-κB as a regulator of cancer metastasis and therapy response: A focus on epithelial-mesenchymal transition. J Cell Physiol. (2022) 237:2770–95. doi: 10.1002/jcp.30759
87. Tune BXJ, Sim MS, Poh CL, Guad RM, Woon CK, Hazarika I, et al. Matrix metalloproteinases in chemoresistance: regulatory roles, molecular interactions, and potential inhibitors. J Oncol. (2022) 2022:3249766. doi: 10.1155/2022/3249766
88. Dong W, Sun S, Cao X, Cui Y, Chen A, Li X, et al. Exposure to TNF−α combined with TGF−β induces carcinogenesis in vitro via NF-κB/Twist axis. Oncol Rep. (2017) 37:1873–82. doi: 10.3892/or.2017.5369
89. Ali A, Kim SH, Kim MJ, Choi MY, Kang SS, Cho GJ, et al. O-glcNAcylation of NF-κB promotes lung metastasis of cervical cancer cells via upregulation of CXCR4 expression. Molecules Cells. (2017) 40:476–84. doi: 10.14348/molcells.2017.2309
90. Malek S, Chen Y, Huxford T, Ghosh G. IkappaBbeta, but not IkappaBalpha, functions as a classical cytoplasmic inhibitor of NF-kappaB dimers by masking both NF-kappaB nuclear localization sequences in resting cells. J Biol Chem. (2001) 276:45225–35. doi: 10.1074/jbc.M105865200
91. Fernandes A, Falcão AS, Silva RFM, Gordo AC, Gama MJ, Brito MA, et al. Inflammatory signalling pathways involved in astroglial activation by unconjugated bilirubin. J Neurochemistry. (2006) 96:1667–79. doi: 10.1111/j.1471-4159.2006.03680.x
92. Chiu H-W, Lee H-L, Lee H-H, Lu H-W, Lin KY-H, Lin Y-F, et al. AIM2 promotes irradiation resistance, migration ability and PD-L1 expression through STAT1/NF-κB activation in oral squamous cell carcinoma. J Trans Med. (2024) 22:13. doi: 10.1186/s12967-023-04825-w
93. Domblides C, Crampton S, Liu H, Bartleson JM, Nguyen A, Champagne C, et al. Human NLRC4 expression promotes cancer survival and associates with type I interferon signaling and immune infiltration. J Clin Invest. (2024) 134:e166085. doi: 10.1172/JCI166085
94. Zhao H, Xu J, Zhong YN, He S, Hao Z, Zhang B, et al. Mammary hydroxylated oestrogen activates the NLRP3 inflammasome in tumor-associated macrophages to promote breast cancer progression and metastasis. Int Immunopharmacol. (2024) 142:113034. doi: 10.1016/j.intimp.2024.113034
95. Gibbs PEM, Maines MD. Biliverdin inhibits activation of NF-kappaB: reversal of inhibition by human biliverdin reductase. Int J Cancer. (2007) 121:2567–74. doi: 10.1002/ijc.v121:11
96. Sinal CJ, Bend JR. Aryl hydrocarbon receptor-dependent induction of cyp1a1 by bilirubin in mouse hepatoma hepa 1c1c7 cells. Mol Pharmacol. (1997) 52:590–9. doi: 10.1124/mol.52.4.590
97. Phelan D, Winter GM, Rogers WJ, Lam JC, Denison MS. Activation of the Ah receptor signal transduction pathway by bilirubin and biliverdin. Arch Biochem Biophysics. (1998) 357:155–63. doi: 10.1006/abbi.1998.0814
98. Hansen TWR. Recent advances in the pharmacotherapy for hyperbilirubinaemia in the neonate. Expert Opin On Pharmacotherapy. (2003) 4:1939–48. doi: 10.1517/14656566.4.11.1939
99. Zhao X, Duan B, Wu J, Huang L, Dai S, Ding J, et al. Bilirubin ameliorates osteoarthritis via activating Nrf2/HO-1 pathway and suppressing NF-κB signalling. J Cell Mol Med. (2024) 28:e18173. doi: 10.1111/jcmm.18173
100. Rosas S, Kwok A, Moore J, Shi L, Smith TL, Tallant EA, et al. Osteoarthritis as a systemic disease promoted prostate cancer in vivo and in vitro. Int J Mol Sci. (2024) 25:6014. doi: 10.3390/ijms25116014
101. Stoeckius M, Erat A, Fujikawa T, Hiromura M, Koulova A, Otterbein L, et al. Essential roles of Raf/extracellular signal-regulated kinase/mitogen-activated protein kinase pathway, YY1, and Ca2+ influx in growth arrest of human vascular smooth muscle cells by bilirubin. J Biol Chem. (2012) 287:15418–26. doi: 10.1074/jbc.M111.266510
102. Weber JD, Raben DM, Phillips PJ, Baldassare JJ. Sustained activation of extracellular-signal-regulated kinase 1 (ERK1) is required for the continued expression of cyclin D1 in G1 phase. Biochem J. (1997) 326:61–8. doi: 10.1042/bj3260061
103. Villanueva J, Yung Y, Walker JL, Assoian RK. ERK activity and G1 phase progression: identifying dispensable versus essential activities and primary versus secondary targets. Mol Biol Cell. (2007) 18:1457–63. doi: 10.1091/mbc.e06-10-0908
104. Yang ZY, Simari RD, Perkins ND, San H, Gordon D, Nabel GJ, et al. Role of the p21 cyclin-dependent kinase inhibitor in limiting intimal cell proliferation in response to arterial injury. Proc Natl Acad Sci United States America. (1996) 93:7905–10. doi: 10.1073/pnas.93.15.7905
105. Sanz-González SM, Barquín L, García-Cao I, Roque M, González JM, Fuster JJ, et al. Increased p53 gene dosage reduces neointimal thickening induced by mechanical injury but has no effect on native atherosclerosis. Cardiovasc Res. (2007) 75:803–12. doi: 10.1016/j.cardiores.2007.05.002
106. Taillé C, Almolki A, Benhamed M, Zedda C, Mégret J, Berger P, et al. Heme oxygenase inhibits human airway smooth muscle proliferation via a bilirubin-dependent modulation of ERK1/2 phosphorylation. J Biol Chem. (2003) 278:27160–8. doi: 10.1074/jbc.M300364200
107. Fukui R, Shibata N, Kohbayashi E, Amakawa M, Furutama D, Hoshiga M, et al. Inhibition of smooth muscle cell migration by the p21 cyclin-dependent kinase inhibitor (Cip1). Atherosclerosis. (1997) 132:53–9. doi: 10.1016/S0021-9150(97)00086-5
108. Deng C-C, Xu M, Li J, Luo X-L, Zhu Y-J, Jiang R, et al. Unconjugated bilirubin is a novel prognostic biomarker for nasopharyngeal carcinoma and inhibits its metastasis via antioxidation activity. Cancer Prev Res (Philadelphia Pa.). (2016) 9:180–8. doi: 10.1158/1940-6207.CAPR-15-0257
109. Constantopoulos A, Matsaniotis N. Bilirubin inhibition of protein kinase: its prevention by cyclic AMP. Cytobios. (1976) 17:17–20.
110. Sano K, Nakamura H, Matsuo T. Mode of inhibitory action of bilirubin on protein kinase C. Pediatr Res. (1985) 19:587–90. doi: 10.1203/00006450-198506000-00017
111. Howe AK, Baldor LC, Hogan BP. Spatial regulation of the cAMP-dependent protein kinase during chemotactic cell migration. Proc Natl Acad Sci United States America. (2005) 102:14320–5. doi: 10.1073/pnas.0507072102
112. Dong JM, Leung T, Manser E, Lim L. cAMP-induced morphological changes are counteracted by the activated RhoA small GTPase and the Rho kinase ROKalpha. J Biol Chem. (1998) 273:22554–62. doi: 10.1074/jbc.273.35.22554
113. Feoktistov I, Goldstein AE, Biaggioni I. Cyclic AMP and protein kinase A stimulate Cdc42: role of A(2) adenosine receptors in human mast cells. Mol Pharmacol. (2000) 58:903–10. doi: 10.1016/S0026-895X(24)12461-3
114. O’Connor KL, Mercurio AM. Protein kinase A regulates Rac and is required for the growth factor-stimulated migration of carcinoma cells. J Biol Chem. (2001) 276:47895–900. doi: 10.1074/jbc.M107235200
115. Nishiya N, Kiosses WB, Han J, Ginsberg MH. An alpha4 integrin-paxillin-Arf-GAP complex restricts Rac activation to the leading edge of migrating cells. Nat Cell Biol. (2005) 7:343–52. doi: 10.1038/ncb1234
116. Whittard JD, Akiyama SK. Positive regulation of cell-cell and cell-substrate adhesion by protein kinase A. J Cell Sci. (2001) 114:3265–72. doi: 10.1242/jcs.114.18.3265
117. Lang P, Gesbert F, Delespine-Carmagnat M, Stancou R, Pouchelet M, Bertoglio J. Protein kinase A phosphorylation of RhoA mediates the morphological and functional effects of cyclic AMP in cytotoxic lymphocytes. EMBO J. (1996) 15:510–9. doi: 10.1002/j.1460-2075.1996.tb00383.x
118. Ellerbroek SM, Wennerberg K, Burridge K. Serine phosphorylation negatively regulates RhoA in vivo. J Biol Chem. (2003) 278:19023–31. doi: 10.1074/jbc.M213066200
119. Howe AK. Regulation of actin-based cell migration by cAMP/PKA. Biochim Et Biophys Acta. (2004) 1692:159–74. doi: 10.1016/j.bbamcr.2004.03.005
120. Lim CJ, Han J, Yousefi N, Ma Y, Amieux PS, McKnight GS, et al. Alpha4 integrins are type I cAMP-dependent protein kinase-anchoring proteins. Nat Cell Biol. (2007) 9:415–21. doi: 10.1038/ncb1561
121. Kilanowska A, Ziółkowska A, Stasiak P, Gibas-Dorna M. cAMP-dependent signaling and ovarian cancer. Cells. (2022) 11:3835. doi: 10.3390/cells11233835
122. McKenzie AJ, Campbell SL, Howe AK. Protein kinase A activity and anchoring are required for ovarian cancer cell migration and invasion. PLoS One. (2011) 6:e26552. doi: 10.1371/journal.pone.0026552
123. Gong S, Chen Y, Meng F, Zhang Y, Wu H, Wu F. Roflumilast restores cAMP/PKA/CREB signaling axis for FtMt-mediated tumor inhibition of ovarian cancer. Oncotarget. (2017) 8:112341–53. doi: 10.18632/oncotarget.22866
124. Kwiecinska P, Ptak A, Wrobel A, Gregoraszczuk EL. Hydroxylated estrogens (2-OH-E2 AND 4-OH-E2) do not activate cAMP/PKA and ERK1/2 pathways activation in a breast cancer MCF-7 cell line. Endocrine Regulations. (2012) 46:3–12. doi: 10.4149/endo_2012_01_3
125. Peverelli E, Giardino E, Mangili F, Treppiedi D, Catalano R, Ferrante E, et al. cAMP/PKA-induced filamin A (FLNA) phosphorylation inhibits SST2 signal transduction in GH-secreting pituitary tumor cells. Cancer Lett. (2018) 435:101–9. doi: 10.1016/j.canlet.2018.08.002
126. Wang S, Zhang Z, Qian W, Ji D, Wang Q, Ji B, et al. Angiogenesis and vasculogenic mimicry are inhibited by 8-Br-cAMP through activation of the cAMP/PKA pathway in colorectal cancer. OncoTargets Ther. (2018) 11:3765–74. doi: 10.2147/OTT.S164982
127. Jiang K, Yao G, Hu L, Yan Y, Liu J, Shi J, et al. MOB2 suppresses GBM cell migration and invasion via regulation of FAK/Akt and cAMP/PKA signaling. Cell Death Dis. (2020) 11:230. doi: 10.1038/s41419-020-2381-8
128. Huang F, Ma G, Zhou X, Zhu X, Yu X, Ding F, et al. Depletion of LAMP3 enhances PKA-mediated VASP phosphorylation to suppress invasion and metastasis in esophageal squamous cell carcinoma. Cancer Lett. (2020) 479:100–11. doi: 10.1016/j.canlet.2020.03.014
129. Haga Y, Tempero MA, Kay D, Zetterman RK. Intracellular accumulation of unconjugated bilirubin inhibits phytohemagglutin-induced proliferation and interleukin-2 production of human lymphocytes. Digestive Dis Sci. (1996) 41:1468–74. doi: 10.1007/BF02088574
130. Wu J-F, Chiang B-L, Chen H-L, Lai H-S, Chang M-H, Ni Y-H. Impaired T-lymphocyte proliferation function in biliary atresia patients with chronic cholestatic jaundice after a Kasai operation. Pediatr Res. (2006) 60:602–6. doi: 10.1203/01.PDR.0000242270.91973.ff
131. Ahlfors CE, Wennberg RP, Ostrow JD, Tiribelli C. Unbound (free) bilirubin: improving the paradigm for evaluating neonatal jaundice. Clin Chem. (2009) 55:1288–99. doi: 10.1373/clinchem.2008.121269
132. Field E, Horst HM, Rubinfeld IS, Copeland CF, Waheed U, Jordan J, et al. Hyperbilirubinemia: a risk factor for infection in the surgical intensive care unit. Am J Surg. (2008) 195:304–6. doi: 10.1016/j.amjsurg.2007.12.010
133. Basiglio CL, Arriaga SM, Pelusa F, Almará AM, Kapitulnik J, Mottino AD. Complement activation and disease: protective effects of hyperbilirubinaemia. Clin Sci (London England: 1979). (2009) 118:99–113. doi: 10.1042/CS20080540
134. Bulla R, Tripodo C, Rami D, Ling GS, Agostinis C, Guarnotta C, et al. C1q acts in the tumour microenvironment as a cancer-promoting factor independently of complement activation. Nat Commun. (2016) 7:10346. doi: 10.1038/ncomms10346
135. Cao Q, McIsaac SM, Stadnyk AW. Human colonic epithelial cells detect and respond to C5a via apically expressed C5aR through the ERK pathway. Am J Physiol Cell Physiol. (2012) 302:C1731–40. doi: 10.1152/ajpcell.00213.2011
136. Kurihara R, Yamaoka K, Sawamukai N, Shimajiri S, Oshita K, Yukawa S, et al. C5a promotes migration, proliferation, and vessel formation in endothelial cells. Inflammation Research: Off J Eur Histamine Res Soc. (2010) 59:659–66. doi: 10.1007/s00011-010-0178-4
137. Nejedlá Z. The development of immunological factors in infants with hyperbilirubinemia. Pediatrics. (1970) 45:102–4. doi: 10.1542/peds.45.1.102
138. Rola-Plezczynski M, Hensen SA, Vincent MM, Bellanti JA. Inhibitory effects of bilirubin on cellular immune responses in man. J Pediatr. (1975) 86:690–6. doi: 10.1016/S0022-3476(75)80352-0
139. Longhi MS, Vuerich M, Kalbasi A, Kenison JE, Yeste A, Csizmadia E, et al. Bilirubin suppresses Th17 immunity in colitis by upregulating CD39. JCI Insight. (2017) 2:e92791. doi: 10.1172/jci.insight.92791
140. Liu X, Wang X, Yang Q, Luo L, Liu Z, Ren X, et al. Th17 cells secrete TWEAK to trigger epithelial-mesenchymal transition and promote colorectal cancer liver metastasis. Cancer Res. (2024) 84:1352–71. doi: 10.1158/0008-5472.CAN-23-2123
141. Neuhaus F, Lieber S, Shinkevich V, Steitz AM, Raifer H, Roth K, et al. Reciprocal crosstalk between Th17 and mesothelial cells promotes metastasis-associated adhesion of ovarian cancer cells. Clin Trans Med. (2024) 14:e1604. doi: 10.1002/ctm2.1604
142. Shibabaw T, Teferi B, Ayelign B. The role of Th-17 cells and IL-17 in the metastatic spread of breast cancer: As a means of prognosis and therapeutic target. Front In Immunol. (2023) 14:1094823. doi: 10.3389/fimmu.2023.1094823
143. Khan NM, Poduval TB. Immunomodulatory and immunotoxic effects of bilirubin: molecular mechanisms. J Leukocyte Biol. (2011) 90:997–1015. doi: 10.1189/jlb.0211070
144. Lee Y, Sugihara K, Gillilland MG, Jon S, Kamada N, Moon JJ. Hyaluronic acid-bilirubin nanomedicine for targeted modulation of dysregulated intestinal barrier, microbiome and immune responses in colitis. Nat Materials. (2020) 19:118–26. doi: 10.1038/s41563-019-0462-9
145. Yao Q, Jiang X, Zhai Y-Y, Luo L-Z, Xu H-L, Xiao J, et al. Protective effects and mechanisms of bilirubin nanomedicine against acute pancreatitis. J Controlled Release: Off J Controlled Release Soc. (2020) 322:312–25. doi: 10.1016/j.jconrel.2020.03.034
146. Vítek L, Kráslová I, Muchová L, Novotný L, Yamaguchi T. Urinary excretion of oxidative metabolites of bilirubin in subjects with Gilbert syndrome. J Gastroenterol Hepatol. (2007) 22:841–5. doi: 10.1111/j.1440-1746.2006.04564.x
147. Kalousová M, Novotny L, Zima T, Braun M, Vítek L. Decreased levels of advanced glycation end-products in patients with Gilbert syndrome. Cell Mol Biol (Noisy-le-Grand France). (2005) 51:387–92.
148. Peng Y-F, Xie L-Q, Xiang Y, Xu G-D. Serum bilirubin and their association with C-reactive protein in patients with migraine. J Clin Lab Anal. (2016) 30:982–5. doi: 10.1002/jcla.21967
149. Zhao X, Li L, Li X, Li J, Wang D, Zhang H. The relationship between serum bilirubin and inflammatory bowel disease. Mediators Inflammation. (2019) 2019:5256460. doi: 10.1155/2019/5256460
150. Ullman TA, Itzkowitz SH. Intestinal inflammation and cancer. Gastroenterology. (2011) 140:1807–16. doi: 10.1053/j.gastro.2011.01.057
151. Lerch MM, Gorelick FS. Models of acute and chronic pancreatitis. Gastroenterology. (2013) 144:1180–93. doi: 10.1053/j.gastro.2012.12.043
152. Malka D, Hammel P, Maire F, Rufat P, Madeira I, Pessione F, et al. Risk of pancreatic adenocarcinoma in chronic pancreatitis. Gut. (2002) 51:849–52. doi: 10.1136/gut.51.6.849
153. Lowenfels AB, Maisonneuve P, Cavallini G, Ammann RW, Lankisch PG, Andersen JR, et al. Pancreatitis and the risk of pancreatic cancer. New Engl J Med. (1993) 328:1433–7. doi: 10.1056/NEJM199305203282001
154. Hahn ME. Aryl hydrocarbon receptors: diversity and evolution. Chemico-biological Interact. (2002) 141:131–60. doi: 10.1016/S0009-2797(02)00070-4
155. Murray IA, Patterson AD, Perdew GH. Aryl hydrocarbon receptor ligands in cancer: friend and foe. Nat Rev Cancer. (2014) 14:801–14. doi: 10.1038/nrc3846
156. Yin J, Sheng B, Han B, Pu A, Yang K, Li P, et al. The AhR is involved in the regulation of LoVo cell proliferation through cell cycle-associated proteins. Cell Biol Int. (2016) 40:560–8. doi: 10.1002/cbin.10592
157. Lamas Bervejillo M, Ferreira AM. Understanding peroxisome proliferator-activated receptors: from the structure to the regulatory actions on metabolism. Adv In Exp Med Biol. (2019) 1127:39–57. doi: 10.1007/978-3-030-11488-6_3
158. Gordon DM, Blomquist TM, Miruzzi SA, McCullumsmith R, Stec DE, Hinds TD. RNA sequencing in human HepG2 hepatocytes reveals PPAR-α mediates transcriptome responsiveness of bilirubin. Physiol Genomics. (2019) 51:234–40. doi: 10.1152/physiolgenomics.00028.2019
159. Hinds TD, Hosick PA, Chen S, Tukey RH, Hankins MW, Nestor-Kalinoski A, et al. Mice with hyperbilirubinemia due to Gilbert’s syndrome polymorphism are resistant to hepatic steatosis by decreased serine 73 phosphorylation of PPARα. Am J Physiol Endocrinol Metab. (2017) 312:E244–52. doi: 10.1152/ajpendo.00396.2016
160. Hinds TD, Burns KA, Hosick PA, McBeth L, Nestor-Kalinoski A, Drummond HA, et al. Biliverdin reductase A attenuates hepatic steatosis by inhibition of glycogen synthase kinase (GSK) 3β Phosphorylation of serine 73 of peroxisome proliferator-activated receptor (PPAR) α. J Biol Chem. (2016) 291:25179–91. doi: 10.1074/jbc.M116.731703
161. Mölzer C, Wallner M, Kern C, Tosevska A, Schwarz U, Zadnikar R, et al. Features of an altered AMPK metabolic pathway in Gilbert’s Syndrome, and its role in metabolic health. Sci Rep. (2016) 6:30051. doi: 10.1038/srep30051
162. Gordon DM, Adeosun SO, Ngwudike SI, Anderson CD, Hall JE, Hinds TD, et al. CRISPR Cas9-mediated deletion of biliverdin reductase A (BVRA) in mouse liver cells induces oxidative stress and lipid accumulation. Arch Biochem Biophysics. (2019) 672:108072. doi: 10.1016/j.abb.2019.108072
163. Montaigne D, Butruille L, Staels B. PPAR control of metabolism and cardiovascular functions. Nat Rev Cardiol. (2021) 18:809–23. doi: 10.1038/s41569-021-00569-6
164. Jang BK. Elevated serum bilirubin levels are inversely associated with nonalcoholic fatty liver disease. Clin Mol Hepatol. (2012) 18:357–9. doi: 10.3350/cmh.2012.18.4.357
165. Kwak M-S, Kim D, Chung GE, Kang SJ, Park MJ, Kim YJ, et al. Serum bilirubin levels are inversely associated with nonalcoholic fatty liver disease. Clin Mol Hepatol. (2012) 18:383–90. doi: 10.3350/cmh.2012.18.4.383
166. Tian J, Zhong R, Liu C, Tang Y, Gong J, Chang J, et al. Association between bilirubin and risk of Non-Alcoholic Fatty Liver Disease based on a prospective cohort study. Sci Rep. (2016) 6:31006. doi: 10.1038/srep31006
167. Chang Y, Ryu S, Zhang Y, Son HJ, Kim J-Y, Cho J, et al. A cohort study of serum bilirubin levels and incident non-alcoholic fatty liver disease in middle aged Korean workers. PLoS One. (2012) 7:e37241. doi: 10.1371/journal.pone.0037241
168. Adams LA, Lymp JF, St Sauver J, Sanderson SO, Lindor KD, Feldstein A, et al. The natural history of nonalcoholic fatty liver disease: a population-based cohort study. Gastroenterology. (2005) 129:113–21. doi: 10.1053/j.gastro.2005.04.014
169. White DL, Kanwal F, El-Serag HB. Association between nonalcoholic fatty liver disease and risk for hepatocellular cancer, based on systematic review. Clin Gastroenterol Hepatology: Off Clin Pract J Am Gastroenterological Assoc. (2012) 10:1342–59. doi: 10.1016/j.cgh.2012.10.001
170. Uhlén M, Fagerberg L, Hallström BM, Lindskog C, Oksvold P, Mardinoglu A, et al. Proteomics. Tissue-based map of the human proteome. Sci (New York N.Y.). (2015) 347:1260419. doi: 10.1126/science.1260419
171. Kachaylo EM, Pustylnyak VO, Lyakhovich VV, Gulyaeva LF. Constitutive androstane receptor (CAR) is a xenosensor and target for therapy. Biochem Biokhimiia. (2011) 76:1087–97. doi: 10.1134/S0006297911100026
172. Huang W, Zhang J, Chua SS, Qatanani M, Han Y, Granata R, et al. Induction of bilirubin clearance by the constitutive androstane receptor (CAR). Proc Natl Acad Sci United States America. (2003) 100:4156–61. doi: 10.1073/pnas.0630614100
173. Handschin C, Meyer UA. Regulatory network of lipid-sensing nuclear receptors: roles for CAR, PXR, LXR, and FXR. Arch Biochem Biophysics. (2005) 433:387–96. doi: 10.1016/j.abb.2004.08.030
174. Xu P, Zhai Y, Wang J. The role of PPAR and its cross-talk with CAR and LXR in obesity and atherosclerosis. Int J Mol Sci. (2018) 19:1260. doi: 10.3390/ijms19041260
175. Dong B, Saha PK, Huang W, Chen W, Abu-Elheiga LA, Wakil SJ, et al. Activation of nuclear receptor CAR ameliorates diabetes and fatty liver disease. Proc Natl Acad Sci United States America. (2009) 106:18831–6. doi: 10.1073/pnas.0909731106
176. Levi AJ, Gatmaitan Z, Arias IM. Two hepatic cytoplasmic protein fractions, Y and Z, and their possible role in the hepatic uptake of bilirubin, sulfobromophthalein, and other anions. J Clin Invest. (1969) 48:2156–67. doi: 10.1172/JCI106182
177. Wolfrum C, Borrmann CM, Borchers T, Spener F. Fatty acids and hypolipidemic drugs regulate peroxisome proliferator-activated receptors alpha - and gamma-mediated gene expression via liver fatty acid binding protein: a signaling path to the nucleus. Proc Natl Acad Sci United States America. (2001) 98:2323–8. doi: 10.1073/pnas.051619898
178. Andersson C, Weeke P, Fosbøl EL, Brendorp B, Køber L, Coutinho W, et al. Acute effect of weight loss on levels of total bilirubin in obese, cardiovascular high-risk patients: an analysis from the lead-in period of the Sibutramine Cardiovascular Outcome trial. Metabolism: Clin Exp. (2009) 58:1109–15. doi: 10.1016/j.metabol.2009.04.003
179. Inoue M, Takahashi Y, Fujii T, Kitagawa M, Fukusato T. Significance of downregulation of liver fatty acid-binding protein in hepatocellular carcinoma. World J Gastroenterol. (2014) 20:17541–51. doi: 10.3748/wjg.v20.i46.17541
180. Margolskee E, Bao F, de Gonzalez AK, Moreira RK, Lagana S, Sireci AN, et al. Hepatocellular adenoma classification: a comparative evaluation of immunohistochemistry and targeted mutational analysis. Diagn Pathol. (2016) 11:27. doi: 10.1186/s13000-016-0475-5
181. Wood SM, Gill AJ, Brodsky AS, Lu S, Friedman K, Karashchuk G, et al. Fatty acid-binding protein 1 is preferentially lost in microsatellite instable colorectal carcinomas and is immune modulated via the interferon γ pathway. Modern Pathology: an Off J United States Can Acad Pathology Inc. (2017) 30:123–33. doi: 10.1038/modpathol.2016.170
182. Lawrie LC, Dundas SR, Curran S, Murray GI. Liver fatty acid binding protein expression in colorectal neoplasia. Br J Cancer. (2004) 90:1955–60. doi: 10.1038/sj.bjc.6601828
183. Shin JW, Kim N, Minh NT, Chapagain DD, Jee SH. Serum bilirubin subgroups and cancer risk: Insights with a focus on lung cancer. Cancer Epidemiol. (2025), 94:102727. doi: 10.1016/j.canep.2024.102727
184. Ihira H, Nakano S, Yamaji T, Katagiri R, Sawada N, Inoue M, et al. Plasma albumin, bilirubin, and uric acid and the subsequent risk of cancer: a case-cohort study in the Japan Public Health Center-based Prospective Study. Am J Epidemiol. (2024) 193:1460–9. doi: 10.1093/aje/kwae092
185. Yoon H-S, Shu X-O, Shidal C, Wu J, Blot WJ, Zheng W, et al. Associations of pre-diagnostic serum levels of total bilirubin and albumin with lung cancer risk: results from the southern community cohort study. Front In Oncol. (2022) 12:895479. doi: 10.3389/fonc.2022.895479
186. Seya M, Aokage T, Nojima T, Nakao A, Naito H. Bile pigments in emergency and critical care medicine. Eur J Med Res. (2022) 27:224. doi: 10.1186/s40001-022-00863-0
187. Bellarosa C, Tiribelli C. Bilirubin 2022: the past, the present and the future. Antioxidants (Basel Switzerland). (2022) 11:1632. doi: 10.3390/antiox11091632
188. Silva SL, Vaz AR, Barateiro A, Falcão AS, Fernandes A, Brito MA, et al. Features of bilirubin-induced reactive microglia: from phagocytosis to inflammation. Neurobiol Dis. (2010) 40:663–75. doi: 10.1016/j.nbd.2010.08.010
189. Zhao Y-Z, Huang Z-W, Zhai Y-Y, Shi Y, Du C-C, Zhai J, et al. Polylysine-bilirubin conjugates maintain functional islets and promote M2 macrophage polarization. Acta Biomaterialia. (2021) 122:172–85. doi: 10.1016/j.actbio.2020.12.047
190. Tu D, Dou J, Wang M, Zhuang H, Zhang X. M2 macrophages contribute to cell proliferation and migration of breast cancer. Cell Biol Int. (2021) 45:831–8. doi: 10.1002/cbin.11528
191. Mantovani A, Marchesi F, Malesci A, Laghi L, Allavena P. Tumour-associated macrophages as treatment targets in oncology. Nat Rev Clin Oncol. (2017) 14:399–416. doi: 10.1038/nrclinonc.2016.217
192. Krneta T, Gillgrass A, Poznanski S, Chew M, Lee AJ, Kolb M, et al. M2-polarized and tumor-associated macrophages alter NK cell phenotype and function in a contact-dependent manner. J Leukocyte Biol. (2017) 101:285–95. doi: 10.1189/jlb.3A1215-552R
193. Caroppo C, Pagliara P. Microalgae: A promising future. Microorganisms. (2022) 10:1488. doi: 10.3390/microorganisms10081488
194. Grosshagauer S, Kraemer K, Somoza V. The true value of spirulina. J Agric Food Chem. (2020) 68:4109–15. doi: 10.1021/acs.jafc.9b08251
195. Liao G, Gao B, Gao Y, Yang X, Cheng X, Ou Y. Phycocyanin inhibits tumorigenic potential of pancreatic cancer cells: role of apoptosis and autophagy. Sci Rep. (2016) 6:34564. doi: 10.1038/srep34564
196. Ge Y, Kang YK, Dong L, Liu LH, An GY. The efficacy of dietary Spirulina as an adjunct to chemotherapy to improve immune function and reduce myelosuppression in patients with Malignant tumors. Transl Cancer Res. (2019) 8:1065–73. doi: 10.21037/tcr.2019.06.13
197. Shiue S-J, Cheng C-L, Shiue H-S, Chen C-N, Cheng S-W, Wu L-W, et al. Arthrospira enhances seroclearance in patients with chronic hepatitis B receiving nucleos(t)ide analogue through modulation of TNF-α/IFN-γ Profile. Nutrients. (2022) 14:2790. doi: 10.3390/nu14142790
198. Brião VB, Sbeghen AL, Colla LM, Castoldi V, Seguenka B, Schimidt G, et al. Is downstream ultrafiltration enough for production of food-grade phycocyanin from Arthrospira platensis? J Appl Phycology. (2020) 32:1129–40. doi: 10.1007/s10811-019-02006-1
199. Nisticò DM, Piro A, Oliva D, Osso V, Mazzuca S, Fagà FA, et al. A combination of aqueous extraction and ultrafiltration for the purification of phycocyanin from arthrospira maxima. Microorganisms. (2022) 10:308. doi: 10.3390/microorganisms10020308
200. Gomaa M, Ali SA, Hifney AF. Enhancement of phycocyanin productivity and thermostability from Arthrospira platensis using organic acids. Microb Cell Fact. (2023) 22:248. doi: 10.1186/s12934-023-02256-2
201. He YJ, Zhang W, Tu JH, Kirchheiner J, Chen Y, Guo D, et al. Hepatic nuclear factor 1alpha inhibitor ursodeoxycholic acid influences pharmacokinetics of the organic anion transporting polypeptide 1B1 substrate rosuvastatin and bilirubin. Drug Metab Dispos. (2008) 36:1453–6. doi: 10.1124/dmd.108.020503
202. Seidensticker M, Fabritius MP, Beller J, Seidensticker R, Todica A, Ilhan H, et al. Impact of pharmaceutical prophylaxis on radiation-induced liver disease following radioembolization. Cancers. (2021) 13:1992. doi: 10.3390/cancers13091992
203. Li M, Chan WW, Zucker SD. Association between atazanavir-induced hyperbilirubinemia and cardiovascular disease in patients infected with HIV. J Am Heart Assoc. (2020) 9:e016310. doi: 10.1161/jaha.120.016310
204. Chiou WJ, de Morais SM, Kikuchi R, Voorman RL, Li X, Bow DA. In vitro OATP1B1 and OATP1B3 inhibition is associated with observations of benign clinical unconjugated hyperbilirubinemia. Xenobiotica. (2014) 44:276–82. doi: 10.3109/00498254.2013.820006
205. Campbell SD, de Morais SM, Xu JJ. Inhibition of human organic anion transporting polypeptide OATP 1B1 as a mechanism of drug-induced hyperbilirubinemia. Chem Biol Interact. (2004) 150:179–87. doi: 10.1016/j.cbi.2004.08.008
206. Visentin M, Stieger B, Merz M, Kullak-Ublick GA. Octreotide inhibits the bilirubin carriers organic anion transporting polypeptides 1B1 and 1B3 and the multidrug resistance-associated protein 2. J Pharmacol Exp Ther. (2015) 355:145–51. doi: 10.1124/jpet.115.227546
207. Hamdulay SS, Wang B, Birdsey GM, Ali F, Dumont O, Evans PC, et al. Celecoxib activates PI-3K/Akt and mitochondrial redox signaling to enhance heme oxygenase-1-mediated anti-inflammatory activity in vascular endothelium. Free Radic Biol Med. (2010) 48:1013–23. doi: 10.1016/j.freeradbiomed.2010.01.017
208. Ju Y, Feng Y, Hou X, Wu L, Yang H, Zhang H, et al. Combined apocyanin and aspirin treatment activates the PI3K/Nrf2/HO-1 signaling pathway and ameliorates preeclampsia symptoms in rats. Hypertens Pregnancy. (2022) 41:39–50. doi: 10.1080/10641955.2021.2014518
209. Rosoff DB, Bell AS, Wagner J, Mavromatis LA, Hamandi A, Park L, et al. Assessing the impact of PCSK9 and HMGCR inhibition on liver function: drug-target mendelian randomization analyses in four ancestries. Cell Mol Gastroenterol Hepatol. (2024) 17:29–40. doi: 10.1016/j.jcmgh.2023.09.001
210. Paquette M, Gauthier D, Chamberland A, Prat A, De Lucia Rolfe E, Rasmussen JJ, et al. Circulating PCSK9 is associated with liver biomarkers and hepatic steatosis. Clin Biochem. (2020) 77:20–5. doi: 10.1016/j.clinbiochem.2020.01.003
211. Schlegel V, Treuner-Kaueroff T, Seehofer D, Berg T, Becker S, Ceglarek U, et al. Low PCSK9 levels are correlated with mortality in patients with end-stage liver disease. PLoS One. (2017) 12:e0181540. doi: 10.1371/journal.pone.0181540
212. Bigo C, Kaeding J, El Husseini D, Rudkowska I, Verreault M, Vohl MC, et al. PPARα: A master regulator of bilirubin homeostasis. PPAR Res 2014. (2014), 747014. doi: 10.1155/2014/747014. Available online at: https://www.hindawi.com/journals/ppar/2014/747014.
213. Wang Y, Yu M, Ma Y, Wang R, Liu W, Xia W, et al. Fenofibrate increases heme oxygenase 1 expression and astrocyte proliferation while limits neuronal injury during intracerebral hemorrhage. Curr Neurovasc Res. (2017) 14:11–8. doi: 10.2174/1567202613666161014161943
214. Muchova L, Wong RJ, Hsu M, Morioka I, Vitek L, Zelenka J, et al. Statin treatment increases formation of carbon monoxide and bilirubin in mice: a novel mechanism of in vivo antioxidant protection. Can J Physiol Pharmacol. (2007) 85:800–10. doi: 10.1139/y07-077
215. Hu M, Tomlinson B. Effects of statin treatments and polymorphisms in UGT1A1 and SLCO1B1 on serum bilirubin levels in Chinese patients with hypercholesterolaemia. Atherosclerosis. (2012) 223:427–32. doi: 10.1016/j.atherosclerosis.2012.06.002
216. Balasubramanian R, Maideen NMP. HMG-coA reductase inhibitors (Statins) and their drug interactions involving CYP enzymes, P-glycoprotein and OATP transporters-an overview. Curr Drug Metab. (2021) 22:328–41. doi: 10.2174/1389200222666210114122729
217. Röllinghoff W, Paumgartner G, Preisig R. Nicotinic acid test in the diagnosis of Gilbert’s syndrome: correlation with bilirubin clearance. Gut. (1981) 22:663–8. doi: 10.1136/gut.22.8.663
218. Qosa H, Avaritt BR, Hartman NR, Volpe DA. In vitro UGT1A1 inhibition by tyrosine kinase inhibitors and association with drug-induced hyperbilirubinemia. Cancer Chemother Pharmacol. (2018) 82:795–802. doi: 10.1007/s00280-018-3665-x
219. Liu Y, Ramírez J, Ratain MJ. Inhibition of paracetamol glucuronidation by tyrosine kinase inhibitors. Br J Clin Pharmacol. (2011) 71:917–20. doi: 10.1111/j.1365-2125.2011.03911.x
220. Gupta B, LeVea C, Litwin A, Fakih MG. Reversible grade 4 hyperbilirubinemia in a patient with UGT1A1 7/7 genotype treated with irinotecan and cetuximab. Clin Colorectal Cancer. (2007) 6:447–9. doi: 10.3816/CCC.2007.n.015
221. Niemi M, Pasanen MK, Neuvonen PJ. Organic anion transporting polypeptide 1B1: a genetically polymorphic transporter of major importance for hepatic drug uptake. Pharmacol Rev. (2011) 63:157–81. doi: 10.1124/pr.110.002857
222. Mochizuki T, Zamek-Gliszczynski MJ, Yoshida K, Mao J, Taskar K, Hirabayashi H, et al. Effect of cyclosporin A and impact of dose staggering on OATP1B1/1B3 endogenous substrates and drug probes for assessing clinical drug interactions. Clin Pharmacol Ther. (2022) 111:1315–23. doi: 10.1002/cpt.2584
223. Prasad S, Gupta SC, Tyagi AK. Reactive oxygen species (ROS) and cancer: Role of antioxidative nutraceuticals. Cancer Lett. (2017) 387:95–105. doi: 10.1016/j.canlet.2016.03.042
224. Moloney JN, Cotter TG. ROS signalling in the biology of cancer. Semin Cell Dev Biol. (2018) 80:50–64. doi: 10.1016/j.semcdb.2017.05.023
225. Park J, Choi Y, Chang H, Um W, Ryu JH, Kwon IC. Alliance with EPR effect: combined strategies to improve the EPR effect in the tumor microenvironment. Theranostics. (2019) 9:8073–90. doi: 10.7150/thno.37198
226. Lee Y, Lee S, Lee DY, Yu B, Miao W, Jon S. Multistimuli-responsive bilirubin nanoparticles for anticancer therapy. Angew Chem Int Ed Engl. (2016) 55:10676–80. doi: 10.1002/anie.201604858
227. Chen SX, Xue F, Kuang Y, Chen S, Sheng D, Chen H. A self-activating nanovesicle with oxygen-depleting capability for efficient hypoxia-responsive chemo-thermo cancer therapy. Biomaterials. (2021) 269:120533. doi: 10.1016/j.biomaterials.2020.120533
228. Lee Y, Shinn J, Xu C, Dobson HE, Neamati N, Moon JJ. Hyaluronic acid-bilirubin nanomedicine-based combination chemoimmunotherapy. Nat Commun. (2023) 14:4771. doi: 10.1038/s41467-023-40270-5
229. Lee S, Lee SA, Shinn J, Lee Y. Hyaluronic acid-bilirubin nanoparticles as a tumor microenvironment reactive oxygen species-responsive nanomedicine for targeted cancer therapy. Int J Nanomedicine. (2024) 19:4893–906. doi: 10.2147/ijn.S460468
230. Liu R, An Y, Jia W, Wang Y, Wu Y, Zhen Y, et al. Macrophage-mimic shape changeable nanomedicine retained in tumor for multimodal therapy of breast cancer. J Control Release. (2020) 321:589–601. doi: 10.1016/j.jconrel.2020.02.043
231. Lee DY, Kim JY, Lee Y, Lee S, Miao W, Kim HS, et al. Black pigment gallstone inspired platinum-chelated bilirubin nanoparticles for combined photoacoustic imaging and photothermal therapy of cancers. Angew Chem Int Ed Engl. (2017) 56:13684–8. doi: 10.1002/anie.201707137
232. Pizzuti VJ, Viswanath D, Torregrosa-Allen SE, Currie MP, Elzey BD, Won YY. Bilirubin-coated radioluminescent particles for radiation-induced photodynamic therapy. ACS Appl Bio Mater. (2020) 3:4858–72. doi: 10.1021/acsabm.0c00354
233. Jung W, Asaduddin M, Yoo D, Lee DY, Son Y, Kim D, et al. Noninvasive ROS imaging and drug delivery monitoring in the tumor microenvironment. Biomaterials. (2024) 310:122633. doi: 10.1016/j.biomaterials.2024.122633
234. Xing R, Zou Q, Yuan C, Zhao L, Chang R, Yan X. Self-assembling endogenous biliverdin as a versatile near-infrared photothermal nanoagent for cancer theranostics. Adv Mater. (2019) 31:e1900822. doi: 10.1002/adma.201900822
235. Cardoso LC, Savedra RM, Silva MM, Ferreira GR, Bianchi RF, Siqueira MF. Effect of blue light on the electronic and structural properties of bilirubin isomers: insights into the photoisomerization and photooxidation processes. J Phys Chem A. (2015) 119:9037–42. doi: 10.1021/acs.jpca.5b04225
236. Jung W, Asaduddin M, Keum H, Son Y, Yoo D, Kim D, et al. Longitudinal magnetic resonance imaging with ROS-responsive bilirubin nanoparticles enables monitoring of nonalcoholic steatohepatitis progression to cirrhosis. Advanced Materials (Deerfield Beach Fla.). (2024) 36:e2305830. doi: 10.1002/adma.202305830
Keywords: bilirubin, tumor, metabolism, oxidation, inflammation, signal transduction, nanomedicine
Citation: Yi F, Tao S and Wu H (2025) Bilirubin metabolism in relation to cancer. Front. Oncol. 15:1570288. doi: 10.3389/fonc.2025.1570288
Received: 03 February 2025; Accepted: 11 March 2025;
Published: 11 April 2025.
Edited by:
Alvaro Marín Hernández, Instituto Nacional de Cardiología, MexicoReviewed by:
Qingkai Xue, Jiangsu Institute of Parasitic Diseases (JIPD), ChinaCopyright © 2025 Yi, Tao and Wu. This is an open-access article distributed under the terms of the Creative Commons Attribution License (CC BY). The use, distribution or reproduction in other forums is permitted, provided the original author(s) and the copyright owner(s) are credited and that the original publication in this journal is cited, in accordance with accepted academic practice. No use, distribution or reproduction is permitted which does not comply with these terms.
*Correspondence: Hongze Wu, d3Vob25nemUxMDM2QDE2My5jb20=
†These authors have contributed equally to this work
Disclaimer: All claims expressed in this article are solely those of the authors and do not necessarily represent those of their affiliated organizations, or those of the publisher, the editors and the reviewers. Any product that may be evaluated in this article or claim that may be made by its manufacturer is not guaranteed or endorsed by the publisher.
Research integrity at Frontiers
Learn more about the work of our research integrity team to safeguard the quality of each article we publish.