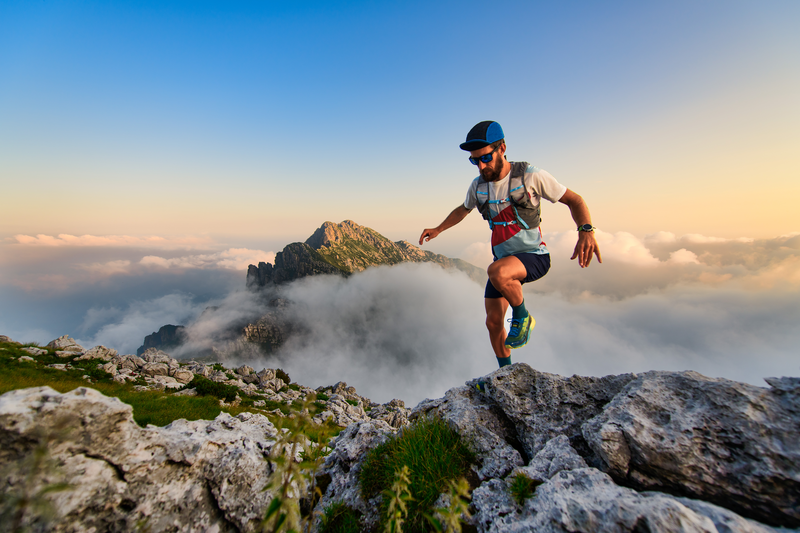
94% of researchers rate our articles as excellent or good
Learn more about the work of our research integrity team to safeguard the quality of each article we publish.
Find out more
ORIGINAL RESEARCH article
Front. Oncol.
Sec. Pharmacology of Anti-Cancer Drugs
Volume 15 - 2025 | doi: 10.3389/fonc.2025.1565638
This article is part of the Research Topic Genomic Discoveries and Pharmaceutical Development in Urologic Tumors - Volume II View all articles
The final, formatted version of the article will be published soon.
You have multiple emails registered with Frontiers:
Please enter your email address:
If you already have an account, please login
You don't have a Frontiers account ? You can register here
MiR-146b has been identified as being overexpressed in human bladder cancer (BCa) and implicated in promoting cancer cell invasion. However, its specific involvement in BCa cell growth remains unclear. In this study, we demonstrate that downregulation of miR-146b significantly suppresses tumorigenic growth of human BCa cells both in vitro and in vivo by inducing G0/G1 cell cycle arrest. Specifically, miR-146b inhibition resulted in a significant reduction in colony formation (p < 0.05) and anchorage-independent growth in both UMUC3 and T24T cells, as measured by soft agar assays, with three independent replicates for each experiment. Notably, Cyclin D1 protein plays a crucial role in miR-146b-induced BCa cell proliferation, as confirmed by Western blot analysis (p < 0.05), with each experiment performed in triplicate. Mechanistic investigations reveal that miR-146b reduces mothers against decapentaplegic homolog 4 (SMAD4) mRNA stability by directly binding to its 3' untranslated region (3'-UTR), leading to decreased SMAD4 expression. This reduction in SMAD4 levels promotes cellular myelocytomatosis (C-MYC) transcription, which in turn enhances Cyclin D1 transcription, ultimately facilitating BCa cell proliferation. The findings unveil a novel regulatory axis involving SMAD4/C-MYC/Cyclin D1 in mediating the oncogenic role of miR-146b in BCa cells. Statistical significance was determined using Student's t-test, with p-values < 0.05 considered significant. Together with its previously established function in BCa invasion, the results highlight the potential for developing miR-146b-based therapeutic strategies for treating human BCa patients.
Keywords: MiR-146b, bladder cancer cell growth, Smad4, c-Myc, Cyclin D1
Received: 23 Jan 2025; Accepted: 03 Mar 2025.
Copyright: © 2025 Zhu, Zheng, Yin, Ding, Li, Wang, Shu, Zhou, Liu and Liu. This is an open-access article distributed under the terms of the Creative Commons Attribution License (CC BY). The use, distribution or reproduction in other forums is permitted, provided the original author(s) or licensor are credited and that the original publication in this journal is cited, in accordance with accepted academic practice. No use, distribution or reproduction is permitted which does not comply with these terms.
* Correspondence:
Junlan Zhu, Precision Medicine Laboratory, Beilun People's Hospital, Beilun Branch of the First Affiliated Hospital, School of Medicine, Zhejiang University, Ningbo, Zhejiang Province, China
Weihua Liu, Department of Urology, Beilun People's Hospital, Beilun Branch of the First Affiliated Hospital, School of Medicine, Zheji, Ningbo, Zhejiang Province, China
Jian Liu, Precision Medicine Laboratory, Beilun People's Hospital, Beilun Branch of the First Affiliated Hospital, School of Medicine, Zhejiang University, Ningbo, Zhejiang Province, China
Disclaimer: All claims expressed in this article are solely those of the authors and do not necessarily represent those of their affiliated organizations, or those of the publisher, the editors and the reviewers. Any product that may be evaluated in this article or claim that may be made by its manufacturer is not guaranteed or endorsed by the publisher.
Research integrity at Frontiers
Learn more about the work of our research integrity team to safeguard the quality of each article we publish.