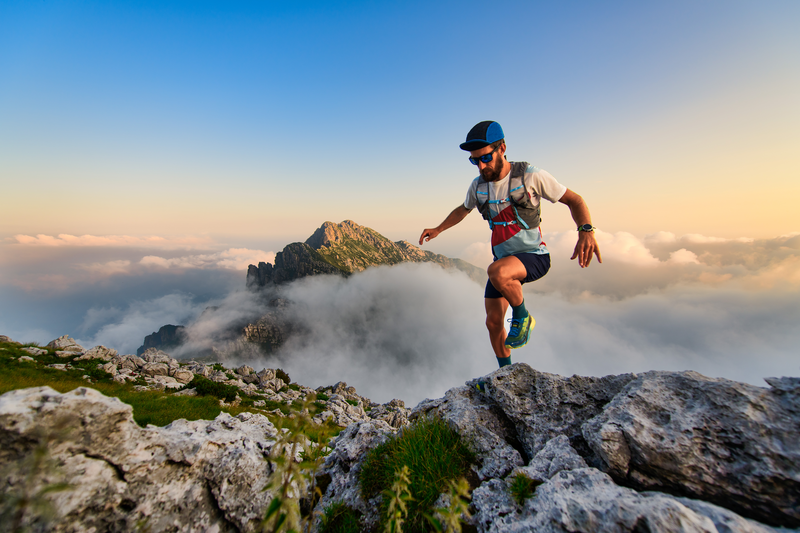
94% of researchers rate our articles as excellent or good
Learn more about the work of our research integrity team to safeguard the quality of each article we publish.
Find out more
REVIEW article
Front. Oncol.
Sec. Molecular and Cellular Oncology
Volume 15 - 2025 | doi: 10.3389/fonc.2025.1556917
The final, formatted version of the article will be published soon.
You have multiple emails registered with Frontiers:
Please enter your email address:
If you already have an account, please login
You don't have a Frontiers account ? You can register here
Cancer is a disease where cells begin to divide uncontrollably and spread to other parts of the body. Mitotic kinases play a crucial role in the initiation and progression of all human malignancies, making them common therapeutic targets. However, a significant portion of the human kinome has yet to be functionally studied in cancer systems. The NIMA-related kinase family (NEKs), consisting of 11 members distributed across different cellular regions, are important protein kinases that regulate mitotic processes. Emerging research suggests that NEK family members have potential key roles in various malignancies. This review systematically summarizes the expression and regulatory mechanisms of NEK family members in different cancer systems, highlighting that targeting NEKs holds promise as a new therapeutic approach for inhibiting cancer growth and metastasis.
Keywords: NEK Family, Mitosis, Therapeutic target, Cancer, proliferation, metastasis
Received: 07 Jan 2025; Accepted: 11 Mar 2025.
Copyright: © 2025 Li, Guo, Li, Jiao, Zhang and Cao. This is an open-access article distributed under the terms of the Creative Commons Attribution License (CC BY). The use, distribution or reproduction in other forums is permitted, provided the original author(s) or licensor are credited and that the original publication in this journal is cited, in accordance with accepted academic practice. No use, distribution or reproduction is permitted which does not comply with these terms.
* Correspondence:
Cong Cong Guo, Department of General Surgery, The First Affiliated Hospital of Shandong First Medical University, Shandong Provincial Qianfoshan Hospital, Jinan, 250014, Shandong Province, China
Ming wen Jiao, Shandong University of Traditional Chinese Medicine, Jinan, China
Disclaimer: All claims expressed in this article are solely those of the authors and do not necessarily represent those of their affiliated organizations, or those of the publisher, the editors and the reviewers. Any product that may be evaluated in this article or claim that may be made by its manufacturer is not guaranteed or endorsed by the publisher.
Research integrity at Frontiers
Learn more about the work of our research integrity team to safeguard the quality of each article we publish.