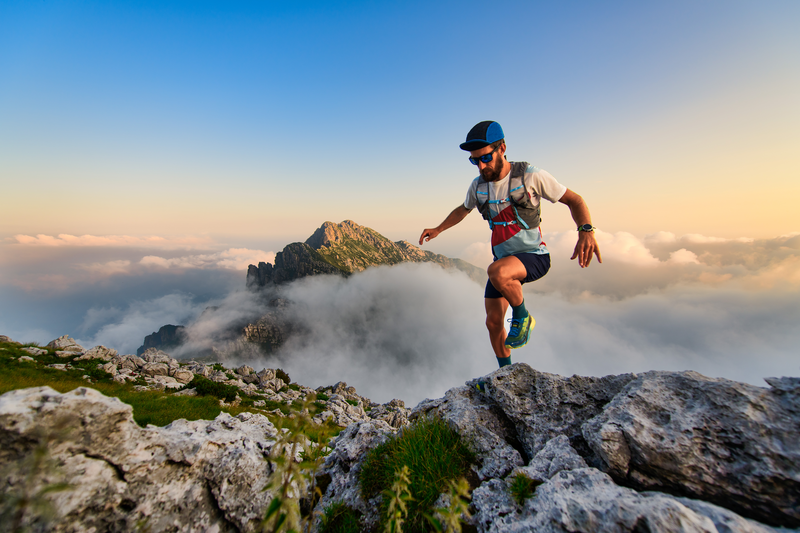
94% of researchers rate our articles as excellent or good
Learn more about the work of our research integrity team to safeguard the quality of each article we publish.
Find out more
ORIGINAL RESEARCH article
Front. Oncol.
Sec. Gastrointestinal Cancers: Hepato Pancreatic Biliary Cancers
Volume 15 - 2025 | doi: 10.3389/fonc.2025.1555649
The final, formatted version of the article will be published soon.
You have multiple emails registered with Frontiers:
Please enter your email address:
If you already have an account, please login
You don't have a Frontiers account ? You can register here
The ubiquitin proteasome system is involved in the regulation of cellular gene transcription and cellular receptor function through the degradation of proteins, thus affecting tumorigenesis and development. In this study, bioinformatics analysis revealed the expression of PSMD11 and PSMD14 in pancreatic ductal adenocarcinoma, which can be used as biomarkers for the prognosis of patients with PDAC. This study provides new targets for the prognostic assessment and targeted therapy of pancreatic ductal adenocarcinoma.The expression levels and prognostic value of PSMD11 and PSMD14 in pancreatic ductal adenocarcinoma patients were analyzed using the GEPIA2, GEO, TCGA and GTEx databases, and the relationships between these expression levels and clinical case data and the survival and prognosis of patients with pancreatic ductal adenocarcinoma were analyzed. The effects of PSMD11 and PSMD14 on the malignant biological behaviors of pancreatic cancer cells, such as proliferation, migration and invasion, were investigated by in vitro experiments.Bioinformatics analysis revealed that the expression levels of PSMD11 and PSMD14 mRNAs were significantly higher in pancreatic ductal adenocarcinoma (PDAC) tissues than in normal pancreatic tissues and that this high expression was correlated with a poor prognosis in patients with PDAC. Further evaluation of the expression of PSMD11 and PSMD14 and correlation of the results with the clinical characteristics and survival of patients with PDAC revealed that high expression of PSMD11 and PSMD14 was associated with lymph node metastasis, TNM grade, degree of differentiation, and poor prognosis in patients with PDAC. Knockdown of PSMD11 and PSMD14 significantly inhibited the proliferation, migration, and invasion ability of pancreatic cancer cells.CONCLUSION: PSMD11 and PSMD14 are highly expressed in pancreatic ductal adenocarcinoma tissues and are correlated with the degree of malignancy of pancreatic ductal adenocarcinoma; thus, PSMD11 and PSMD14 can be used as potential prognostic biomarkers and therapeutic targets for PDAC patients.
Keywords: PSMD11, PSMD14, Poor prognosis, Malignant behavior, Pancreatic Ductal Adenocarcinoma
Received: 05 Jan 2025; Accepted: 28 Feb 2025.
Copyright: © 2025 Yang, Xing, Wang, Zhang, Liu, Bai, Liu, Liu, Yang, Li and Fan. This is an open-access article distributed under the terms of the Creative Commons Attribution License (CC BY). The use, distribution or reproduction in other forums is permitted, provided the original author(s) or licensor are credited and that the original publication in this journal is cited, in accordance with accepted academic practice. No use, distribution or reproduction is permitted which does not comply with these terms.
* Correspondence:
Hua Fan, Office of Research & Innovation, The First Affiliated Hospital, College of Clinical Medicine of Henan University of Science and Technology, Luoyang, China
Disclaimer: All claims expressed in this article are solely those of the authors and do not necessarily represent those of their affiliated organizations, or those of the publisher, the editors and the reviewers. Any product that may be evaluated in this article or claim that may be made by its manufacturer is not guaranteed or endorsed by the publisher.
Research integrity at Frontiers
Learn more about the work of our research integrity team to safeguard the quality of each article we publish.