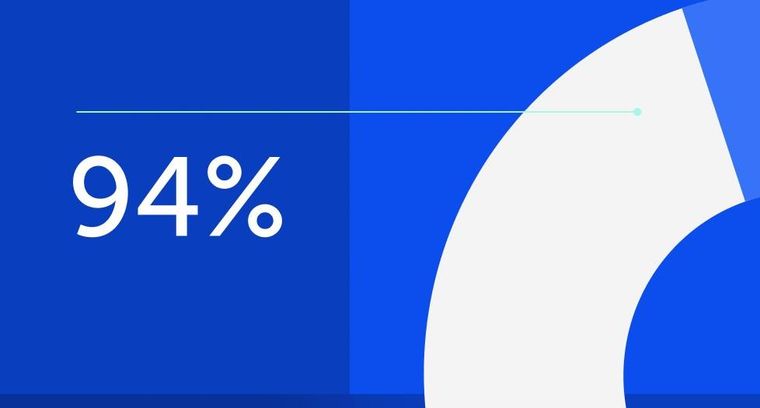
94% of researchers rate our articles as excellent or good
Learn more about the work of our research integrity team to safeguard the quality of each article we publish.
Find out more
REVIEW article
Front. Oncol., 11 April 2025
Sec. Gastrointestinal Cancers: Colorectal Cancer
Volume 15 - 2025 | https://doi.org/10.3389/fonc.2025.1554179
This article is part of the Research TopicHot Topics in Cell Death: Circular and Non-Coding Endogenous RNA in Cancer ProgressionView all 4 articles
Circular RNAs (circRNAs) are multifaceted molecules that play a pivotal role in regulating gene expression at both transcriptional and post-transcriptional levels. Their expression is highly tissue-specific and developmentally regulated, making them critical players in various physiological processes and diseases, particularly cancer. In colorectal cancer, circRNAs exhibit significantly dysregulated expression patterns and profoundly influence disease progression through diverse molecular mechanisms. Unraveling the complex roles of circRNAs in modulating colorectal cancer immunotherapy outcomes highlights their potential as both promising biomarkers and therapeutic targets. Moving forward, advancements in circRNA-based therapeutic strategies and delivery systems are poised to transform precision medicine, enabling early colorectal cancer diagnosis and improving patient prognosis.
According to the latest data from the International Agency for Research on Cancer (IARC), approximately 1.926 million new cases of colorectal cancer (CRC) were projected globally in 2022, accounting for about 9.6% of all malignant tumors. CRC-related deaths were estimated at 904,000, representing 9.3% of all cancer-related fatalities (1). CRC ranks as the third most diagnosed cancer worldwide, following lung and breast cancers, and is the second leading cause of cancer-related deaths, surpassed only by lung cancer. In China, the 2022 National Cancer Center report identified CRC as the second most frequently diagnosed cancer and the fourth leading cause of cancer-related mortality (2). Overall, CRC remains a major public health challenge, ranking among the top five cancers in both incidence and mortality globally and in China, and contributing significantly to the global disease burden.
The management of CRC combines surgical and non-surgical therapies. Surgical resection remains the cornerstone and primary curative option. However, approximately 25% of patients present with distant metastases, complicating treatment. Evidence suggests that resecting the primary tumor can improve survival (3, 4). Treatment strategies vary by disease stage. For locally advanced rectal cancer, neoadjuvant radiotherapy enhances complete resection rates and reduces recurrence risk. Patients achieving clinical complete remission (cCR) may opt for a watch-and-wait approach, avoiding surgery (5, 6). Stage III CRC patients benefit from adjuvant chemotherapy to lower recurrence rates. For metastatic CRC, personalized therapy, including RAS and BRAF mutation testing, is standard. Immunotherapy, now the fifth major treatment modality, has shown promising results, particularly in neoadjuvant settings for locally advanced and metastatic CRC (4, 7–9).
Recent studies highlight the unique expression profiles of circRNAs in various cancers, making them promising tools for early tumor detection (10). CircRNAs, a distinct class of non-coding RNAs, form closed-loop structures through back splicing, lacking a 5’ cap and 3’ poly(A) tail. This structure provides exceptional stability and conservation, as they resist exonuclease degradation (10–12). Produced via exon or intron cyclization, circRNAs play critical roles in cancer by acting as miRNA sponges, translation templates, and gene expression regulators (13, 14). In CRC, circRNAs show distinct expression patterns in serum, tissues, and exosomes, significantly influencing disease progression (15). For instance, circPTK2 promotes epithelial-mesenchymal transition (EMT) in CRC by interacting with waveform proteins, serving as a potential biomarker and therapeutic target for metastatic CRC (16). Similarly, circZNF800 enhances tumor stem cell properties and CRC progression, with CRISPR Cas13d-based knockdown showing therapeutic potential (17). Additionally, circRERE-AAV inhibits tumor growth and enhances anti-PD-1 therapy efficacy, highlighting its role in CRC immunotherapy (18). This review explores the emerging roles of circRNAs in CRC, focusing on their diagnostic, prognostic, and therapeutic applications, particularly in immunotherapy. Advancing understanding of circRNA biology may lead to innovative CRC management strategies.
In 1976, German scientists, including Heinz L. Sanger, discovered circRNA, a ring-shaped RNA molecule with a covalent bond linking its 3’ and 5’ ends, formed via back-splicing or a lasso mechanism. This circular structure enhances stability by resisting ribonuclease degradation, surpassing linear RNAs in durability. Most circRNAs are cytoplasmic, with a smaller fraction localized in the nucleus. They play key roles in neural development, tumorigenesis, immune responses, and gene regulation (19). CircRNAs possess distinctive features (10): (1) Strong stability, (2) Specificity, (3) Enrichment, (4) Evolutionary conservation, (5) Non-cap-dependent translation, and (6) Low immunogenicity. Their low immunogenicity reduces innate immune activation and dendritic cell maturation, preventing immune responses to encoded therapeutic proteins. CircRNAs are synthesized in eukaryotic cells through two pathways: (1) back-splicing, where the downstream splice donor joins the upstream splice acceptor, forming a closed loop, or (2) intronic lasso formation during linear splicing. Research highlights their critical roles in maintaining stem cell pluripotency and directing differentiation, as well as in tissue development, maintenance, and regeneration (20, 21).
CircRNAs exhibit robust and stable biological activities, playing diverse roles in physiological and pathological pathways. They can significantly influence the tumor microenvironment, affecting tumor growth and progression (22)(Figure 1). CircRNAs also impact the stem-like properties of cancer cells, contributing to cancer progression (23, 24). Dysregulated circRNA expression has been observed in various common and rare cancers (25, 26) (Figure 1). Exploring these alterations can deepen our understanding of circRNAs in cancer initiation and progression. Furthermore, specific tumor-associated circRNAs show promise as diagnostic biomarkers for cancer detection (27–29).
Figure 1. Changes in circRNA expression levels in colorectal cancer and their impact on tumor biological behavior. The left side of the figure illustrates circRNA upregulation, where the proliferation and invasion & metastasis capabilities of tumor cells are significantly enhanced. In contrast, the right side depicts circRNA downregulation, leading to the suppression of tumor cell proliferation and invasion & metastasis. Overall, the differential expression levels of circRNAs regulate the malignant phenotypes of colorectal cancer cells, providing potential targets for subsequent diagnostic and therapeutic strategies.
CircRNAs regulate gene expression through diverse mechanisms (30–32) (Figure 2): 1. miRNA Sponges: CircRNAs can act as competing endogenous RNAs (ceRNAs) by binding to miRNAs via enriched binding sites, modulating their activity. They may also serve as miRNA reservoirs or facilitate intracellular transport (10, 33). 2. Protein Sponges: Certain circRNAs bind RNA-binding proteins (RBPs), such as circMBL, which interacts specifically with MBL protein to regulate its own synthesis. This interaction depends on conserved binding sites within circMBL and its intronic sequences (19). 3. Protein Scaffolding: CircRNAs can bridge enzymes and substrates. For example, circFOXO3 binds both p53 and MDM2, enabling MDM2 to ubiquitinate p53 (34–36). 4. Template for Translation: CircRNAs with internal ribosome entry sites (IRES) can encode peptides. For instance, circPPP1R12A encodes a peptide that promotes colon cancer progression via Hippo-YAP signaling, while circFNDC3B-derived protein suppresses tumors by enhancing FBP1 activity (37–39). 5. Transcription Regulation: EIciRNAs, bound to U1snRNP, interact with RNA polymerase II to enhance transcription of their parental genes (40). 6. RNA Stability: circRNAs stabilize other RNAs, such as mRNAs and lncRNAs, often through protein interactions. For instance, circZNF609 recruits ELAV1 (HuR) to enhance mRNA stability and translation, while circXPO1 stabilizes CTNNB1 mRNA via IGF2BP recruitment, promoting lung adenocarcinoma progression (10, 41). 7. RBP Modulation: circRNAs can sequester RBPs in the cytoplasm, preventing their nuclear translocation or modulating their activity. In NSCLC, circNDUFB2 scaffolds TRIM25 and IGF2BPs to facilitate ubiquitination and degradation of IGF2BPs, activating anti-tumor immunity (42). Though most circRNAs are non-coding, some have translational potential under two conditions: the presence of open reading frames (ORFs) and IRES, or m6A modifications in their 5’UTR. Examples include circE-Cad, which encodes C-E-Cad to promote glioblastoma tumorigenicity via EGFR-STAT3 signaling, and circRNAs from oncogenic viruses like circE7, which translates the E7 oncoprotein in cervical and head and neck cancers (43–45).
Figure 2. Multiple mechanisms of circRNA in tumor immunomodulation and immunotherapy. 1. miRNA Sponge: circRNAs (e.g., circIL4R) bind to specific miRNAs (e.g., miR-761), alleviating the translational repression of target mRNAs, thereby influencing tumor cell proliferation and invasion. 2. Signaling Pathway Regulation: circRNAs participate in regulating key signaling pathways (e.g., TGFβ/SMAD) by modulating the activity of transcription factors (e.g., SMAD2/3/4), further affecting cell proliferation, differentiation, and immune responses. 3. Immune Cell Polarization: Certain circRNAs (e.g., circPOLQ) can influence the polarization of macrophages into M1 or M2 phenotypes, shaping the immune landscape of the tumor microenvironment through the secretion of cytokines (e.g., IL-10, TGFβ, M-CSF, IL-35). 4. Immune Evasion: circRNAs (e.g., circ_0020397) interact with immune checkpoint pathways such as the PD-1/PD-L1 axis, promoting tumor cell evasion from immune surveillance and destruction. 5. Potential Therapeutic Targets: circRNAs (e.g., circPTK2) play a critical role in regulating tumor cell proliferation and immune responses, making them promising targets for novel strategies in tumor immunotherapy through modulation of their expression or function.
In summary, an increasing body of research is delving into the biogenesis and functionality of circRNAs, with a particular focus on the cellular processes that give rise to circRNAs and the mechanisms by which they exert their influence on development and disease. These studies underscore the potential of circRNAs to serve as valuable diagnostic and prognostic biomarkers.
Research indicates that over 70 circRNAs are significantly upregulated in CRC, actively contributing to its initiation and progression. For example, circHERC4 is highly expressed in CRC tissues and correlates with lymph node metastasis and advanced tumors (46). CircHIPK3 is overexpressed in hepatocellular carcinoma, breast, CRC, and lung cancers, with elevated levels in CRC linked to poorer prognosis (47–49). Similarly, circALG1 is associated with CRC metastasis, while circPTK2 overexpression correlates with metastasis, advanced staging, and chemotherapy resistance (16, 50, 51). Increased circ5615 expression is linked to advanced T stage and poor prognosis in CRC (52). Conversely, downregulated circRNAs often exhibit anti-tumor effects in CRC. CircPTEN1 and circLPAR1 are both under expressed in CRC tissues, with the latter linked to reduced tumor weight and size (53, 54). CircPLCE1 downregulation is associated with poorer survival and advanced staging (45, 55, 56). CircEXOC6B, significantly downregulated in CRC, negatively correlates with tumor size, lymphatic metastasis, and TNM stage (57). CircLHFPL2 is also under expressed in PIK3CA-mutated CRC, with its downregulation linked to poor prognosis (58). Overall, the abnormal expression patterns of circRNAs in CRC highlight their potential as biomarkers for diagnosis and prognosis.
CircRNAs influence cancer development through various mechanisms shaped by their sequence, stability, post-transcriptional modifications, secondary structure, and accumulation patterns under specific conditions. They play critical roles in modulating tumor signaling pathways such as PI3K/AKT, Wnt/β-catenin, JAK/STAT, GEF-H1/RhoA, and TGF-β/Smad. CircRNAs regulate these pathways by upregulating oncogenes, downregulating tumor suppressor genes, or modulating downstream protein levels (49, 54, 59–63). As a result, circRNAs hold significant potential as biomarkers for CRC diagnosis.
This study reveals for the first time that circIL4R expression is significantly elevated in CRC cells, tissues, and serum, highlighting its potential as a diagnostic and prognostic biomarker. TFAP2C transcriptionally induces circIL4R expression, which competitively binds miR-761, thereby upregulating TRIM29. This process targets PHLPP1 for ubiquitin-mediated degradation, activating the PI3K/AKT pathway and promoting CRC progression (62). In contrast, circPTEN1 acts as a CRC suppressor by interfering with the TGF-β/Smad signaling pathway. CircPTEN1 binds to the MH2 domain of Smad4, disrupting its interaction with phosphorylated Smad2/3, thereby curbing metastasis driven by TGF-β signaling. Targeting TGF-β signaling could serve as an effective therapeutic strategy, with circPTEN1 emerging as a promising candidate for preventing metastatic CRC (52). Additionally, a positive feedback loop involving HIF1A, RRAGB, and mTORC1 plays a key role in CRC development. CircEXOC6B binds to RRAGB, disrupting its interaction with RRAGC/D and inhibiting this loop. This suppression hampers CRC cell growth and enhances 5-fluorouracil (5-FU)-induced apoptosis, offering new insights into therapeutic targets involving the HIF1A and mTORC1 pathways (64). The circular RNA circGPRC5A is significantly elevated in CRC tissues compared to normal counterparts and is strongly associated with tumor size, stage, and lymph node involvement. In vitro and in vivo studies revealed that circGPRC5A enhances CRC cell proliferation, migration, and metastasis. Mechanistically, circGPRC5A binds to PPP1CA, inhibiting its ubiquitination by UBA1 and preventing proteasomal degradation. This stabilizes PPP1CA, increasing its phosphatase activity, which dephosphorylates YAP at Ser127 and Ser109. Dephosphorylated YAP translocates to the nucleus, interacts with TEAD transcription factors, and activates target gene expression, promoting tumor progression. Immunohistochemical analysis further demonstrated elevated Ki-67 and PPP1CA expression in tumors with high circGPRC5A levels, consistent with its role in enhancing cell proliferation. Silencing circGPRC5A reduced these protein levels, confirming its involvement in CRC progression and tumorigenesis (65). A study confirmed the cyclic structure and intracellular localization of circFNDC3B, highlighting its reduced expression in colon cancer and its association with poorer patient survival. The research demonstrated that lower levels of circFNDC3B suppressed colon cancer progression. Analysis through circRNADb revealed that circFNDC3B contains ORFs and IRES, enabling it to encode proteins. Specifically, circFNDC3B-218aa was shown to inhibit metastasis and EMT in colorectal cancer by modulating the Snail/FBP1 signaling pathway, thereby suppressing tumor growth. Moreover, circFNDC3B-218aa promotes a metabolic shift from glycolysis to oxidative phosphorylation, further impeding EMT progression. These findings provide new insights into the mechanisms of colorectal cancer development (39).
Recent studies have revealed several critical roles of circRNAs in CRC. CircYAP, encoding the oncogenic protein YAP-220aa, promotes liver metastasis in CRC by inhibiting LATS1-mediated YAP phosphorylation, thereby enhancing YAP activity. Notably, circYAP is overexpressed in CRC with liver metastases and correlates with poor prognosis, positioning it as a potential prognostic biomarker and therapeutic target (66). CircHERC4, another oncogenic driver, is significantly upregulated in CRC tissues and linked to increased proliferation, migration, and invasiveness of CRC cells. Elevated circHERC4 levels are associated with metastasis and poor survival outcomes. Mechanistically, circHERC4 may inhibit miR-556-5p, thereby upregulating CTBP2 and suppressing E-cadherin activation. These findings suggest that targeting circHERC4 could offer novel therapeutic strategies (46). Conversely, circFBXW4 functions as a tumor suppressor in CRC by regulating the miR-338-5p/SLC5A7 axis, presenting a new avenue for therapy (67). Additionally, exosome-derived circLPAR1 suppresses CRC growth by binding to eIF3h, disrupting the METTL3-eIF3h interaction, and reducing BRD4 translation, providing new insights into early diagnosis and disease mechanisms (53). Finally, circFMN2 promotes CRC cell proliferation and migration through the miR-1182/hTERT pathway, highlighting the intricate regulatory network between circRNAs, miRNAs, and mRNAs in CRC progression (68).
In aggregate, these findings indicate that individual circRNAs could exert both pro- and anti-cancer effects in the context of colorectal carcinogenesis, metastasis, and drug resistance, mediated by diverse molecular pathways. Consequently, they may represent promising and valuable biomarkers for the clinical diagnosis, treatment, and prognostic evaluation of cancer.
CircRNAs play a crucial role in modulating immune responses within the tumor microenvironment (TME) (69). Studies show that various immune cells, including monocytes, neutrophils, B-cells, and platelets, can internalize extracellular circRNAs, with monocytes exhibiting the highest uptake efficiency. The uptake of circRNAs is particularly enhanced in differentiated macrophages and dendritic cells (DCs), indicating their key role in recognizing and processing circRNAs (70). CircRNAs positively regulate macrophage function and, when derived from tumor cells, can induce M2 polarization in macrophages (71). This polarization is governed by pathways such as JAK1/STAT3 and PI3K-AKT, with circRNAs actively participating in these processes (72, 73). M2 macrophages, in response to tumor-associated cytokines, lose their antitumor function and instead secrete immunosuppressive factors like IL-10, TGF-β, and IDO, promoting immune evasion (74). Additionally, exosomal circRNAs from macrophages, such as circMERTK, enhance IL-10 production in tumor-associated macrophages (TAMs), which suppresses CD8+ T-cell function and contributes to the immunosuppressive TME. Targeting this mechanism could offer therapeutic potential in colorectal cancer (75).
CircRNAs play a key role in enhancing tumor immunity by promoting T cell recruitment and activation (Figure 3). For example, circDNA2v, frequently overexpressed in CRC, prevents its own ubiquitination and degradation by binding to IGF2BP3, stabilizing c-Myc mRNA and influencing the oncogenic traits of CRC cells. Knockdown of circDNA2v activates the JAK-STAT1 pathway and increases the secretion of CXCL10 and IL-9, which enhance the chemotactic and cytotoxic functions of CD8+ T cells, boosting anti-tumor immunity, as shown in in vitro and in vivo models (76). On the other hand, certain circRNAs, such as circRNF216, can inhibit CRC progression and enhance tumor immunity. circRNF216 promotes CD8+ T cell infiltration by upregulating ZC3H12C, triggering an immune response that helps limit tumor growth (77). Thus, circRNAs may serve as potential biomarkers for CRC treatment by modulating tumor immunity.
Figure 3. Multifunctionality of circRNAs in colorectal cancer immunotherapy. 1. Biomarkers: circRNAs can be utilized for early diagnosis and prognosis evaluation. For instance, by detecting circRNA expression levels in blood or tissue samples and correlating them with clinical survival curves, patients can be stratified into high-risk and low-risk groups. 2. Immune Regulation: circRNAs influence the balance of the tumor immune microenvironment by modulating the functions of various immune cells, including regulatory T cells (Tregs), macrophages, and natural killer cells (NK cells). They also promote or suppress tumor progression by inducing or inhibiting the secretion of cytokines (e.g., IL-10, TGF-β). 3. Therapeutic Targets: Molecular interventions targeting circRNAs hold promise for improving tumor treatment efficacy. The figure contrasts "Non-response" and "Response," illustrating that some patients achieve better therapeutic outcomes under circRNA-targeted strategies. This highlights the potential of circRNAs as emerging targets for precision therapy in colorectal cancer.
A study found that transfection of circARGL into CRC cells enhances their proliferation and migration. Exosomal circARGL also affects TGF-β expression, suggesting its role in tumor progression (78, 79). TGF-β, a multifunctional cytokine, regulates immune functions and is linked to tumorigenesis and metastasis. In the tumor microenvironment, TGF-β suppresses N1 neutrophil differentiation while promoting N2 neutrophils, aiding tumor progression (80, 81). Exosomal circRNAs from tumors modulate TGF-β expression through miRNA sponging, contributing to the N1 to N2 switch and supporting tumor growth (82).
Natural Killer (NK) cells, crucial to immune defense, are impacted by exosomal circRNAs such as circFOXO3 and circRHOT1, which sequester miRNAs, leading to NK cell senescence and tumor progression (83). Exosomes from cancer cells, carrying circUHRF1, impair NK cell function and contribute to resistance to anti-PD1 therapy in hepatocellular carcinoma. Additionally, elevated circFAT1 expression activates STAT3, reducing CD8+ T cell infiltration and diminishing PD1-blocking immunotherapy efficacy, promoting immune evasion (84). Targeting circRNAs and modulating immune cells in the tumor microenvironment could enhance immunotherapy efficacy and improve patient survival. In summary, circRNAs play a central role in regulating tumor-associated macrophages, regulatory T cells, CD8+ T cells, neutrophils, and NK cells in the tumor microenvironment.
The TME enables tumor cells to infiltrate blood and lymphatic vessels, evading immune surveillance and resisting T cell cytotoxicity, leading to metastasis and tumor growth (64). In CRC, circ_0020397 sponges miR-138, upregulating TERT and PD-L1 expression (85). CiR7 increases PD-L1 levels through miRNA-independent modulation of CMTM4 and CMTM6 (64), while hsa_circ_0136666 and circ-KRT6C enhance PD-L1 expression by targeting miR-497 and miR-485-3p, respectively (86, 87). Elevated TERT expression promotes CRC cell proliferation, while PD-L1 suppresses T cell activation, aiding immune evasion and cancer progression (88). Silencing circPGPEP1 boosts T cell proliferation and inhibits CRC tumor growth (64). Overexpression of circQSOX1 promotes glycolysis and reduces the effectiveness of anti-CTLA-4 therapy, aiding immune escape (89). These findings suggest that circRNAs could serve as promising diagnostic biomarkers for CRC.
CircRNAs have emerged as critical regulators in colorectal cancer (CRC), serving as both therapeutic targets and immunomodulators. Oncogenic circRNAs, such as CDR1as and circHIPK3, promote CRC progression by sponging tumor-suppressive miRNAs (e.g., miR-7) and activating pathways like EGFR/IGF1R and FAK/YY1 (90, 91). Silencing these circRNAs using siRNAs or shRNAs (e.g., against circPTK2 and circMETTL3) effectively suppresses tumor growth and metastasis (15, 92–94). Additionally, circRNAs like circKRT6C and circQSOX1 modulate immune evasion mechanisms, such as the miR-485-3p/PD-L1 axis and Treg-mediated immunosuppression, highlighting their potential to enhance immunotherapy (87, 89). Tumor-suppressive circRNAs, including circDDX17 and circ-FBXW7, inhibit CRC proliferation when overexpressed, offering alternative therapeutic strategies (90).
CircRNA-based immunotherapies are showing promising preliminary outcomes (Table 1). For instance, combining PD-1/PD-L1 blockade with CDR1as targeting enhances immunotherapy efficacy by upregulating PD-L1 expression (15, 92). Small molecules YAP inhibitors suppress circPPP1R12A-73aa impairing tumor metastasis (95). Exosome-derived circRNAs and radiation-induced circRNA modulation (e.g., carbon ion irradiation) provide novel diagnostic and therapeutic biomarkers (96, 97). Furthermore, CRISPR-based editing (e.g., circZNF800 knockdown) and circRNA cloning into plasmid vectors demonstrate potential in CRC treatment (17, 92).
These advancements underscore the dual role of circRNAs as therapeutic targets and immunomodulators. By targeting oncogenic circRNAs and leveraging tumor-suppressive circRNAs, researchers are developing innovative strategies to improve CRC outcomes. The integration of circRNA-based therapies with existing treatments, such as immune checkpoint inhibitors and small molecules, holds significant promise for advancing CRC immunotherapy and reducing disease burden (64, 86, 88, 89, 93, 98–101).
CircRNAs are emerging as promising biomarkers for cancer prognosis due to their high abundance and stability in cancer cells, solid tumors, and body fluids, including serum, plasma, and urine (15). Certain circRNAs correlate with clinicopathological features like lymphatic metastasis, distant metastasis, and recurrence, making them potential prognostic biomarkers for CRC (92). For example, upregulation of circ3823 is linked to enhanced proliferation, metastasis, and angiogenesis, while circ5615 correlates with T-staging (15). CircHIPK3, when upregulated, boosts CRC cell proliferation, migration, invasion, and apoptosis. circSPARC’s overexpression associates with larger tumors, deeper infiltration, and poor survival (15). Conversely, downregulation of circPTEN1 promotes metastasis and invasion, serving as an independent predictor of poor survival outcomes (102). Other circRNAs, including circ_0009361, can suppress CRC growth and metastasis, indicating their potential as prognostic biomarkers (15). CircRNAs like circCCDC66, circPPP1R12A, ciRS-7, and circ_0014717 correlate with reduced survival rates, emphasizing their prognostic value in CRC (90). A circular RNA-based classifier (cirScore) using four circRNAs (hsa_circ_0122319, hsa_circ_0087391, hsa_circ_0079480, hsa_circ_0008039) has been developed to predict CRC recurrence (92, 103). Additionally, hsa_circ_0005075 and circFADS2 serve as independent predictors of CRC prognosis (104, 105). CircHIPK3 and circCCDC66 are particularly promising, with roles in CRC cell growth and metastasis, and their expression is inversely related to clinical outcomes (106). Finally, hsa_circRNA_102958 promotes CRC cell proliferation and invasion, suggesting it may also be a biomarker for poor prognosis. Overall, circRNAs offer exciting potential for prognostic assessment and therapeutic targeting in CRC (107).
Numerous downregulated circRNAs play a critical role in negatively regulating CRC growth and metastasis. Due to their stability and long half-life, these tumor suppressor circRNAs could have substantial antitumor effects when expressed in CRC cells or tissues. Zheng et al. found that circLPAR1 expression was significantly reduced in CRC tissues, and its overexpression decreased tumor weight and size, suggesting its potential as a biomarker for poor prognosis (15). Similarly, circRNF216, downregulated in CRC, inhibits metastasis when overexpressed, both in vitro and in vivo (77). CircRERE suppresses CRC malignancy by sequestering miR-6837-3p, upregulating MAVS, and enhancing the type I IFN signaling pathway, thus stimulating anti-tumor immunity. This effect is further potentiated when combined with anti-PD-1 therapy (18). Additionally, hsa_circRNA _00004677, upregulated in CRC tissues, contributes to tumor progression by promoting eIF4A3-driven translation of the c-Myc oncogene, correlating with poor patient prognosis (108). In conclusion, circRNAs are crucial modulators of tumorigenesis in various cancers. Their stability and tissue-specific expression highlight their potential as molecular biomarkers and therapeutic targets, meriting further investigation for early diagnosis, treatment, and prognostic assessment in CRC (89).
The integration of bioinformatics tools has significantly advanced the study of circRNAs in CRC, providing robust platforms to analyze circRNA-miRNA-mRNA networks and regulatory interactions. Tools such as CircNet 2.0 and CircNetVis enable comprehensive visualization and reconstruction of circRNA-centered regulatory networks, identifying key sponging interactions and downstream mRNA targets (109, 110). CircScan and EasyCircR facilitate accurate circRNA identification and quantification from RNA-seq data, distinguishing circRNAs from linear isoforms with high precision (111). Specialized tools like circRNA-sponging and CRAFT predict miRNA-binding sites and functional enrichment, aiding in prioritizing circRNAs with high sponging potential or disease relevance (112). Additionally, circMine and riboCIRC offer user-friendly interfaces for exploring circRNA expression profiles, clinical correlations, and cross-species conservation, streamlining hypothesis generation (113, 114). These tools collectively enhance the systematic exploration of circRNA roles in CRC pathogenesis.
Bioinformatics tools bridge the gap between high-throughput data and mechanistic insights, complementing wet-lab experiments. For example, CircNet 2.0 can validate experimentally observed circRNA-miRNA interactions by mapping them to established networks, reinforcing their biological significance (91). CRAFT and circRNA-sponging predict novel interactions that guide targeted functional studies, reducing trial-and-error approaches (87). Tools like riboCIRC integrate ribo-seq data to assess circRNA translatability, supporting findings on oncogenic circRNA-encoded peptides (95). Furthermore, circMine links circRNA expression with patient survival data, helping prioritize biomarkers for clinical validation (93, 94). By contextualizing experimental results within larger regulatory frameworks, these tools enhance the reproducibility and translational relevance of circRNA studies.
The synergy between bioinformatics tools and experimental research accelerates the discovery of circRNA-based diagnostics and therapies. EasyCircR and CircScan standardize circRNA detection pipelines, enabling consistent analysis across studies and cohorts (111). CircNetVis generates interactive networks to visualize circRNA-driven immune evasion mechanisms, aiding in the design of combination therapies targeting circRNAs and immune checkpoints (110). Databases like circMine provide pre-processed multi-omics datasets, allowing researchers to explore circRNA-drug interactions or repurpose existing therapies (115). Moreover, riboCIRC’s integration of translatome data supports the development of circRNA-encoded peptide-targeted therapies (114). By offering scalable, data-driven insights, these tools reduce research bottlenecks and foster innovation in CRC circRNA biology, ultimately accelerating the translation of circRNA discoveries into clinical applications.
The development of CRC is a multifactorial process driven by genetic, environmental, dietary factors, and dysregulated gene expression (Figure 4). As a novel class of non-coding RNAs, circRNAs exert pleiotropic effects in the CRC tumor microenvironment by regulating cell signaling, epithelial-mesenchymal transition, angiogenesis, and immune evasion, demonstrating potential as diagnostic/prognostic biomarkers (88, 106). Although research on circulating RNAs in CRC remains nascent, circRNAs have shown unique therapeutic value: 1) acting as competitive inhibitors of microRNAs to modulate immune responses (77); 2) serving as stable vaccine vectors encoding tumor antigens to enhance anti-tumor immunity via activation of CD8+/CD4+ T cells and dendritic cells (116–118); and 3) synergizing with combination therapies such as CAR-T (chimeric antigen receptor T-cell) (119, 120).
Figure 4. Future perspectives of circRNA in colorectal cancer immunotherapy. This framework outlines the multifaceted landscape of circRNA research and its applications. Basic research focuses on functional exploration, particularly the roles of circRNAs in the tumor microenvironment (TME) and the identification of therapeutic targets. Technology development encompasses optimizing RNA modification and delivery systems to enhance circRNA stability, designing circRNA-based vaccines, and discovering combination therapies to amplify treatment efficacy. Clinical translation advances circRNAs into practical use through biomarker-driven diagnostic and prognostic tests, as well as clinical trials evaluating the safety and efficacy of circRNA-targeted therapies. Looking forward, future directions emphasize deepening mechanistic understanding through enhanced basic research, expanding clinical trials to validate therapeutic potential, and updating technologies to refine circRNA applications. Collectively, these efforts bridge fundamental discoveries to clinical innovations, positioning circRNAs as pivotal tools in advancing cancer diagnosis and precision therapy.
Current limitations and challenges include:
1. Predominant reliance on in vitro models for mechanistic studies and a lack of clinical-grade delivery systems;
2. Constraints in validating in vivo effects due to interspecies microenvironmental disparities;
3. Clinical translation risks.
Proposed strategies for advancement:
1. Development of novel vectors to enable sustained circRNA expression;
2. Integration of single-cell sequencing and spatial omics to dissect spatiotemporal regulatory networks;
3. Establishment of standardized protocols for circRNA synthesis, purification, and delivery.
Although current studies have preliminarily revealed the potential of circRNAs in regulating immune responses in CRC, the hierarchy of evidence and clinical applicability still require cautious evaluation. Most mechanistic investigations rely on in vitro cell models, which fail to recapitulate the dynamic interactions between immune cells and stromal components in the tumor microenvironment. While animal experiments partially validate in vivo effects, interspecies differences in immune microenvironments may compromise the clinical extrapolation of findings. Furthermore, clinical data directly linking circRNAs to immunotherapy responses in CRC remain scarce. Some mechanisms are extrapolated from other cancer types, and existing clinical studies are predominantly limited by retrospective designs and small sample sizes, rendering them prone to confounding factors. Future research should integrate single-cell sequencing, spatial transcriptomics, and prospective cohorts to systematically dissect the spatiotemporal-specific roles of circRNAs in the human CRC immune microenvironment. Additionally, establishing standardized circRNA detection and functional validation protocols will be critical to enhance the translational value of these findings.
YC: Writing – original draft, Writing – review & editing. YH: Data curation, Writing – original draft. LL: Writing – original draft, Writing – review & editing. LX: Writing – original draft, Writing – review & editing.
The author(s) declare that financial support was received for the research and/or publication of this article. This study was funded by the science and technology innovation Program of Changde city (2023ZD69).
The authors declare that the research was conducted in the absence of any commercial or financial relationships that could be construed as a potential conflict of interest.
The author(s) declare that no Generative AI was used in the creation of this manuscript.
All claims expressed in this article are solely those of the authors and do not necessarily represent those of their affiliated organizations, or those of the publisher, the editors and the reviewers. Any product that may be evaluated in this article, or claim that may be made by its manufacturer, is not guaranteed or endorsed by the publisher.
1. Siegel RL, Giaquinto AN, Jemal A. Cancer statistics, 2024. CA Cancer J Clin. (2024) 74:12–49. doi: 10.3322/caac.21820
2. Xu L, Zhao J, Li Z, Sun J, Lu Y, Zhang R, et al. National and subnational incidence, mortality and associated factors of colorectal cancer in China: A systematic analysis and modelling study. J Glob Health. (2023) 13:4096. doi: 10.7189/jogh.13.04096
3. Dohrn N, Klein MF. Colorectal cancer: current management and future perspectives. Br J Surg. (2023) 110:1256–9. doi: 10.1093/bjs/znad095
4. Morris VK, Kennedy EB, Baxter NN, Benson AB 3rd, Cercek A, Cho M, et al. Treatment of metastatic colorectal cancer: ASCO guideline. J Clin Oncol. (2023) 41:678–700. doi: 10.1200/jco.22.01690
5. Conroy T, Castan F, Etienne PL, Rio E, Mesgouez-Nebout N, Evesque L, et al. Total neoadjuvant therapy with mFOLFIRINOX versus preoperative chemoradiotherapy in patients with locally advanced rectal cancer: long-term results of the UNICANCER-PRODIGE 23 trial. Ann Oncol. (2024) 35:873–81. doi: 10.1016/j.annonc.2024.06.019
6. Fernandez LM, São Julião GP, Figueiredo NL, Beets GL, van der Valk MJM, Bahadoer RR, et al. Conditional recurrence-free survival of clinical complete responders managed by watch and wait after neoadjuvant chemoradiotherapy for rectal cancer in the International Watch & Wait Database: a retrospective, international, multicentre registry study. Lancet Oncol. (2021) 22:43–50. doi: 10.1016/s1470-2045(20)30557-x
7. Chalabi M, Fanchi LF, Dijkstra KK, Van den Berg JG, Aalbers AG, Sikorska K, et al. Neoadjuvant immunotherapy leads to pathological responses in MMR-proficient and MMR-deficient early-stage colon cancers. Nat Med. (2020) 26:566–76. doi: 10.1038/s41591-020-0805-8
8. Cercek A, Lumish M, Sinopoli J, Weiss J, Shia J, Lamendola-Essel M, et al. PD-1 blockade in mismatch repair-deficient, locally advanced rectal cancer. N Engl J Med. (2022) 386:2363–76. doi: 10.1056/NEJMoa2201445
9. Lin ZY, Zhang P, Chi P, Xiao Y, Xu XM, Zhang AM, et al. Neoadjuvant short-course radiotherapy followed by camrelizumab and chemotherapy in locally advanced rectal cancer (UNION): early outcomes of a multicenter randomized phase III trial. Ann Oncol. (2024) 35:882–91. doi: 10.1016/j.annonc.2024.06.015
10. Pisignano G, Michael DC, Visal TH, Pirlog R, Ladomery M, Calin GA. Going circular: history, present, and future of circRNAs in cancer. Oncogene. (2023) 42:2783–800. doi: 10.1038/s41388-023-02780-w
11. Zhuo CJ, Hou WH, Jiang DG, Tian HJ, Wang LN, Jia F, et al. Circular RNAs in early brain development and their influence and clinical significance in neuropsychiatric disorders. Neural Regener Res. (2020) 15:817–23. doi: 10.4103/1673-5374.268969
12. Huang X, Song C, Zhang J, Zhu L, Tang H. Circular RNAs in breast cancer diagnosis, treatment and prognosis. Oncol Res. (2023) 32:241–9. doi: 10.32604/or.2023.046582
13. Li H, Peng K, Yang K, Ma W, Qi S, Yu X, et al. Circular RNA cancer vaccines drive immunity in hard-to-treat Malignancies. Theranostics. (2022) 12:6422–36. doi: 10.7150/thno.77350
14. Zhou WY, Cai ZR, Liu J, Wang DS, Ju HQ, Xu RH. Circular RNA: metabolism, functions and interactions with proteins. Mol Cancer. (2020) 19:172. doi: 10.1186/s12943-020-01286-3
15. Zhang Y, Luo J, Yang W, Ye WC. CircRNAs in colorectal cancer: potential biomarkers and therapeutic targets. Cell Death Dis. (2023) 14:353. doi: 10.1038/s41419-023-05881-2
16. Yang H, Li X, Meng Q, Sun H, Wu S, Hu W, et al. Correction: CircPTK2 (hsa_circ_0005273) as a novel therapeutic target for metastatic colorectal cancer. Mol Cancer. (2024) 23:69. doi: 10.1186/s12943-024-01983-3
17. Rengganaten V, Huang CJ, Wang ML, Chien Y, Tsai PH, Lan YT, et al. Circular RNA ZNF800 (hsa_circ_0082096) regulates cancer stem cell properties and tumor growth in colorectal cancer. BMC Cancer. (2023) 23:1088. doi: 10.1186/s12885-023-11571-1
18. Ding N, You AB, Yang H, Hu GS, Lai CP, Liu W, et al. A tumor-suppressive molecular axis EP300/circRERE/miR-6837-3p/MAVS activates type I IFN pathway and antitumor immunity to suppress colorectal cancer. Clin Cancer Res. (2023) 29:2095–109. doi: 10.1158/1078-0432.Ccr-22-3836
19. Chen X, Yang T, Wang W, Xi W, Zhang T, Li Q, et al. Circular RNAs in immune responses and immune diseases. Theranostics. (2019) 9:588–607. doi: 10.7150/thno.29678
20. Kristensen LS, Andersen MS, Stagsted LVW, Ebbesen KK, Hansen TB, Kjems J. The biogenesis, biology and characterization of circular RNAs. Nat Rev Genet. (2019) 20:675–91. doi: 10.1038/s41576-019-0158-7
21. Patop IL, Wüst S, Kadener S. Past, present, and future of circRNAs. EMBO J. (2019) 38:e100836. doi: 10.15252/embj.2018100836
22. Tang LL, Guo R, Zhang N, Deng B, Chen L, Cheng ZB, et al. Effect of radiotherapy alone vs radiotherapy with concurrent chemoradiotherapy on survival without disease relapse in patients with low-risk nasopharyngeal carcinoma: A randomized clinical trial. JAMA. (2022) 328:728–36. doi: 10.1001/jama.2022.13997
23. Loh JJ, Ma S. Hallmarks of cancer stemness. Cell Stem Cell. (2024) 31:617–39. doi: 10.1016/j.stem.2024.04.004
24. Liu CX, Chen LL. Circular RNAs: Characterization, cellular roles, and applications. Cell. (2022) 185:2016–34. doi: 10.1016/j.cell.2022.04.021
25. Kristensen LS, Jakobsen T, Hager H, Kjems J. The emerging roles of circRNAs in cancer and oncology. Nat Rev Clin Oncol. (2022) 19:188–206. doi: 10.1038/s41571-021-00585-y
26. Liu J, Zhao F, Chen LL, Su S. Dysregulation of circular RNAs in inflammation and cancers. Fundam Res. (2023) 3:683–91. doi: 10.1016/j.fmre.2023.04.019
27. Dong Y, Gao Q, Chen Y, Zhang Z, Du Y, Liu Y, et al. Identification of CircRNA signature associated with tumor immune infiltration to predict therapeutic efficacy of immunotherapy. Nat Commun. (2023) 14:2540. doi: 10.1038/s41467-023-38232-y
28. Zhang W, Xu C, Yang Z, Zhou J, Peng W, Zhang X, et al. Circular RNAs in tumor immunity and immunotherapy. Mol Cancer. (2024) 23:171. doi: 10.1186/s12943-024-02082-z
29. Yi J, Li B, Yin X, Liu L, Song C, Zhao Y, et al. CircMYBL2 facilitates hepatocellular carcinoma progression by regulating E2F1 expression. Oncol Res. (2024) 32:1129–39. doi: 10.32604/or.2024.047524
30. Sun J, Li B, Shu C, Ma Q, Wang J. Functions and clinical significance of circular RNAs in glioma. Mol Cancer. (2020) 19:34. doi: 10.1186/s12943-019-1121-0
31. Wei J, Li M, Xue C, Chen S, Zheng L, Deng H, et al. Understanding the roles and regulation patterns of circRNA on its host gene in tumorigenesis and tumor progression. J Exp Clin Cancer Res. (2023) 42:86. doi: 10.1186/s13046-023-02657-6
32. Xu L, Ma X, Zhang X, Zhang C, Zhang Y, Gong S, et al. hsa_circ_0007919 induces LIG1 transcription by binding to FOXA1/TET1 to enhance the DNA damage response and promote gemcitabine resistance in pancreatic ductal adenocarcinoma. Mol Cancer. (2023) 22:195. doi: 10.1186/s12943-023-01887-8
33. Deng G, Mou T, He J, Chen D, Lv D, Liu H, et al. Circular RNA circRHOBTB3 acts as a sponge for miR-654-3p inhibiting gastric cancer growth. J Exp Clin Cancer Res. (2020) 39:1. doi: 10.1186/s13046-019-1487-2
34. Du WW, Zhang C, Yang W, Yong T, Awan FM, Yang BB. Identifying and characterizing circRNA-protein interaction. Theranostics. (2017) 7:4183–91. doi: 10.7150/thno.21299
35. Liu Y, Su Z, Tavana O, Gu W. Understanding the complexity of p53 in a new era of tumor suppression. Cancer Cell. (2024) 42:946–67. doi: 10.1016/j.ccell.2024.04.009
36. Orea-Soufi A, Paik J, Bragança J, Donlon TA, Willcox BJ, Link W. FOXO transcription factors as therapeutic targets in human diseases. Trends Pharmacol Sci. (2022) 43:1070–84. doi: 10.1016/j.tips.2022.09.010
37. Hwang HJ, Kim YK. Molecular mechanisms of circular RNA translation. Exp Mol Med. (2024) 56:1272–80. doi: 10.1038/s12276-024-01220-3
38. Zheng X, Chen L, Zhou Y, Wang Q, Zheng Z, Xu B, et al. Correction to: A novel protein encoded by a circular RNA circPPP1R12A promotes tumor pathogenesis and metastasis of colon cancer via Hippo-YAP signaling. Mol Cancer. (2021) 20:42. doi: 10.1186/s12943-021-01337-3
39. Pan Z, Cai J, Lin J, Zhou H, Peng J, Liang J, et al. A novel protein encoded by circFNDC3B inhibits tumor progression and EMT through regulating Snail in colon cancer. Mol Cancer. (2020) 19:71. doi: 10.1186/s12943-020-01179-5
40. Zhong Y, Yang Y, Wang X, Ren B, Wang X, Shan G, et al. Systematic identification and characterization of exon-intron circRNAs. Genome Res. (2024) 34:376–93. doi: 10.1101/gr.278590.123
41. Rossi F, Beltran M, Damizia M, Grelloni C, Colantoni A, Setti A, et al. Circular RNA ZNF609/CKAP5 mRNA interaction regulates microtubule dynamics and tumorigenicity. Mol Cell. (2022) 82:75–89.e9. doi: 10.1016/j.molcel.2021.11.032
42. Li B, Zhu L, Lu C, Wang C, Wang H, Jin H, et al. circNDUFB2 inhibits non-small cell lung cancer progression via destabilizing IGF2BPs and activating anti-tumor immunity. Nat Commun. (2021) 12:295. doi: 10.1038/s41467-020-20527-z
43. Zhong J, Wu X, Gao Y, Chen J, Zhang M, Zhou H, et al. Circular RNA encoded MET variant promotes glioblastoma tumorigenesis. Nat Commun. (2023) 14:4467. doi: 10.1038/s41467-023-40212-1
44. Gao X, Xia X, Li F, Zhang M, Zhou H, Wu X, et al. Circular RNA-encoded oncogenic E-cadherin variant promotes glioblastoma tumorigenicity through activation of EGFR-STAT3 signalling. Nat Cell Biol. (2021) 23:278–91. doi: 10.1038/s41556-021-00639-4
45. Liang ZX, Liu HS, Xiong L, Yang X, Wang FW, Zeng ZW, et al. A novel NF-κB regulator encoded by circPLCE1 inhibits colorectal carcinoma progression by promoting RPS3 ubiquitin-dependent degradation. Mol Cancer. (2021) 20:103. doi: 10.1186/s12943-021-01404-9
46. He J, Chu Z, Lai W, Lan Q, Zeng Y, Lu D, et al. Circular RNA circHERC4 as a novel oncogenic driver to promote tumor metastasis via the miR-556-5p/CTBP2/E-cadherin axis in colorectal cancer. J Hematol Oncol. (2021) 14:194. doi: 10.1186/s13045-021-01210-2
47. Yuan Y, Zhang X, Fan X, Peng Y, Jin Z. The emerging roles of circular RNA-mediated autophagy in tumorigenesis and cancer progression. Cell Death Discovery. (2022) 8:385. doi: 10.1038/s41420-022-01172-5
48. Huang Y, Zhang C, Xiong J, Ren H. Emerging important roles of circRNAs in human cancer and other diseases. Genes Dis. (2021) 8:412–23. doi: 10.1016/j.gendis.2020.07.012
49. Xue C, Li G, Zheng Q, Gu X, Bao Z, Lu J, et al. The functional roles of the circRNA/Wnt axis in cancer. Mol Cancer. (2022) 21:108. doi: 10.1186/s12943-022-01582-0
50. Lin C, Ma M, Zhang Y, Li L, Long F, Xie C, et al. The N(6)-methyladenosine modification of circALG1 promotes the metastasis of colorectal cancer mediated by the miR-342-5p/PGF signalling pathway. Mol Cancer. (2022) 21:80. doi: 10.1186/s12943-022-01560-6
51. Gong SX, Yang FS, Qiu DD. CircPTK2 accelerates tumorigenesis of colorectal cancer by upregulating AKT2 expression via miR-506-3p. Kaohsiung J Med Sci. (2022) 38:1060–9. doi: 10.1002/kjm2.12589
52. Ma Z, Han C, Xia W, Wang S, Li X, Fang P, et al. circ5615 functions as a ceRNA to promote colorectal cancer progression by upregulating TNKS. Cell Death Dis. (2020) 11:356. doi: 10.1038/s41419-020-2514-0
53. Zheng R, Zhang K, Tan S, Gao F, Zhang Y, Xu W, et al. Exosomal circLPAR1 functions in colorectal cancer diagnosis and tumorigenesis through suppressing BRD4 via METTL3-eIF3h interaction. Mol Cancer. (2022) 21:49. doi: 10.1186/s12943-021-01471-y
54. Zheng L, Liang H, Zhang Q, Shen Z, Sun Y, Zhao X, et al. circPTEN1, a circular RNA generated from PTEN, suppresses cancer progression through inhibition of TGF-β/Smad signaling. Mol Cancer. (2022) 21:41. doi: 10.1186/s12943-022-01495-y
55. Yi B, Dai K, Yan Z, Yin Z. Circular RNA PLCE1 promotes epithelial mesenchymal transformation, glycolysis in colorectal cancer and M2 polarization of tumor-associated macrophages. Bioengineered. (2022) 13:6243–56. doi: 10.1080/21655979.2021.2003929
56. Chen Z, Chen H, Yang L, Li X, Wang Z. CircPLCE1 facilitates the Malignant progression of colorectal cancer by repressing the SRSF2-dependent PLCE1 pre-RNA splicing. J Cell Mol Med. (2021) 25:7244–56. doi: 10.1111/jcmm.16753
57. Lin SC, Liao YC, Chen PM, Yang YY, Wang YH, Tung SL, et al. Periostin promotes ovarian cancer metastasis by enhancing M2 macrophages and cancer-associated fibroblasts via integrin-mediated NF-kappaB and TGF-beta2 signaling. J BioMed Sci. (2022) 29:109. doi: 10.1186/s12929-022-00888-x
58. Chong X, Chen J, Zheng N, Zhou Z, Hai Y, Chen S, et al. PIK3CA mutations-mediated downregulation of circLHFPL2 inhibits colorectal cancer progression via upregulating PTEN. Mol Cancer. (2022) 21:118. doi: 10.1186/s12943-022-01531-x
59. Lee YJ, Kim WR, Park EG, Lee DH, Kim JM, Shin HJ, et al. Exploring the key signaling pathways and ncRNAs in colorectal cancer. Int J Mol Sci. (2024) 25:4548. doi: 10.3390/ijms25084548
60. Song J, Liu Q, Han L, Song T, Huang S, Zhang X, et al. Hsa_circ_0009092/miR-665/NLK signaling axis suppresses colorectal cancer progression via recruiting TAMs in the tumor microenvironment. J Exp Clin Cancer Res. (2023) 42:319. doi: 10.1186/s13046-023-02887-8
61. Wang J, Zhang Y, Song H, Yin H, Jiang T, Xu Y, et al. The circular RNA circSPARC enhances the migration and proliferation of colorectal cancer by regulating the JAK/STAT pathway. Mol Cancer. (2021) 20:81. doi: 10.1186/s12943-021-01375-x
62. Yang H, Zhang H, Yang Y, Wang X, Deng T, Liu R, et al. Hypoxia induced exosomal circRNA promotes metastasis of Colorectal Cancer via targeting GEF-H1/RhoA axis. Theranostics. (2020) 10:8211–26. doi: 10.7150/thno.44419
63. Yu JH, Tan JN, Zhong GY, Zhong L, Hou D, Ma S, et al. Hsa_circ_0020134 promotes liver metastasis of colorectal cancer through the miR-183-5p-PFN2-TGF-β/Smad axis. Transl Oncol. (2024) 39:101823. doi: 10.1016/j.tranon.2023.101823
64. Zhang C, Zhang C, Liu X, Sun W, Liu H. Circular RNA PGPEP1 induces colorectal cancer Malignancy and immune escape. Cell Cycle. (2023) 22:1743–58. doi: 10.1080/15384101.2023.2225923
65. Chen Z, Li Y, He K, Yang J, Deng Q, Chen Y, et al. CircGPRC5A enhances colorectal cancer progress by stabilizing PPP1CA and inducing YAP dephosphorylation. J Exp Clin Cancer Res. (2023) 42:334. doi: 10.1186/s13046-023-02915-7
66. Zeng K, Peng J, Xing Y, Zhang L, Zeng P, Li W, et al. A positive feedback circuit driven by m(6)A-modified circular RNA facilitates colorectal cancer liver metastasis. Mol Cancer. (2023) 22:202. doi: 10.1186/s12943-023-01848-1
67. Song W, Fu J, Wu J, Ren J, Xiang R, Kong C, et al. CircFBXW4 suppresses colorectal cancer progression by regulating the miR-338-5p/SLC5A7 axis. Adv Sci (Weinh). (2024) 11:e2300129. doi: 10.1002/advs.202300129
68. Li Y, Li C, Xu R, Wang Y, Li D, Zhang B. A novel circFMN2 promotes tumor proliferation in CRC by regulating the miR-1182/hTERT signaling pathways. Clin Sci (Lond). (2019) 133:2463–79. doi: 10.1042/cs20190715
69. Xu Z, Li P, Fan L, Wu M. The potential role of circRNA in tumor immunity regulation and immunotherapy. Front Immunol. (2018) 9:9. doi: 10.3389/fimmu.2018.00009
70. Granstein RD, Ding W, Ozawa H. Induction of anti-tumor immunity with epidermal cells pulsed with tumor-derived RNA or intradermal administration of RNA. J Invest Dermatol. (2000) 114:632–6. doi: 10.1046/j.1523-1747.2000.00929.x
71. Yao Y, Chen C, Wang J, Xuan H, Chen X, Li Z, et al. Circular RNA circATP9A promotes non-small cell lung cancer progression by interacting with HuR and by promoting extracellular vesicles-mediated macrophage M2 polarization. J Exp Clin Cancer Res. (2023) 42:330. doi: 10.1186/s13046-023-02916-6
72. Huang X, Wang J, Guan J, Zheng Z, Hao J, Sheng Z, et al. Exosomal circsafb2 reshaping tumor environment to promote renal cell carcinoma progression by mediating M2 macrophage polarization. Front Oncol. (2022) 12:808888. doi: 10.3389/fonc.2022.808888
73. Chen T, Liu Y, Li C, Xu C, Ding C, Chen J, et al. Tumor-derived exosomal circFARSA mediates M2 macrophage polarization via the PTEN/PI3K/AKT pathway to promote non-small cell lung cancer metastasis. Cancer Treat Res Commun. (2021) 28:100412. doi: 10.1016/j.ctarc.2021.100412
74. Sun Z, Xu Y, Shao B, Dang P, Hu S, Sun H, et al. Exosomal circPOLQ promotes macrophage M2 polarization via activating IL-10/STAT3 axis in a colorectal cancer model. J Immunother Cancer. (2024) 12:e008491. doi: 10.1136/jitc-2023-008491
75. Zhu M, Zhu Z, Jiang P, Zheng J, Yan F, Feng J. CircMERTK modulates the suppressive capacity of tumor-associated macrophage via targeting IL-10 in colorectal cancer. Hum Cell. (2023) 36:276–85. doi: 10.1007/s13577-022-00792-4
76. Wu S, Dai X, Xia Y, Zhao Q, Zhao H, Shi Z, et al. Targeting high circDNA2v levels in colorectal cancer induces cellular senescence and elicits an anti-tumor secretome. Cell Rep. (2024) 43:114111. doi: 10.1016/j.celrep.2024.114111
77. Du W, Quan X, Wang C, Song Q, Mou J, Pei D. Regulation of tumor metastasis and CD8(+) T cells infiltration by circRNF216/miR-576-5p/ZC3H12C axis in colorectal cancer. Cell Mol Biol Lett. (2024) 29:19. doi: 10.1186/s11658-024-00539-z
78. Deng Z, Fan T, Xiao C, Tian H, Zheng Y, Li C, et al. TGF-β signaling in health, disease, and therapeutics. Signal Transduct Target Ther. (2024) 9:61. doi: 10.1038/s41392-024-01764-w
79. Massagué J, Sheppard D. TGF-β signaling in health and disease. Cell. (2023) 186:4007–37. doi: 10.1016/j.cell.2023.07.036
80. Jaillon S, Ponzetta A, Di Mitri D, Santoni A, Bonecchi R, Mantovani A. Neutrophil diversity and plasticity in tumour progression and therapy. Nat Rev Cancer. (2020) 20:485–503. doi: 10.1038/s41568-020-0281-y
81. Zhao Y, Rahmy S, Liu Z, Zhang C, Lu X. Rational targeting of immunosuppressive neutrophils in cancer. Pharmacol Ther. (2020) 212:107556. doi: 10.1016/j.pharmthera.2020.107556
82. Shang A, Gu C, Wang W, Wang X, Sun J, Zeng B, et al. Exosomal circPACRGL promotes progression of colorectal cancer via the miR-142-3p/miR-506-3p- TGF-β1 axis. Mol Cancer. (2020) 19:117. doi: 10.1186/s12943-020-01235-0
83. Maia A, Tarannum M, Lérias JR, Piccinelli S, Borrego LM, Maeurer M, et al. Building a better defense: expanding and improving natural killer cells for adoptive cell therapy. Cells. (2024) 13:451. doi: 10.3390/cells13050451
84. Jia L, Wang Y, Wang CY. circFAT1 promotes cancer stemness and immune evasion by promoting STAT3 activation. Adv Sci (Weinh). (2021) 8:2003376. doi: 10.1002/advs.202003376
85. Zhang XL, Xu LL, Wang F. Hsa_circ_0020397 regulates colorectal cancer cell viability, apoptosis and invasion by promoting the expression of the miR-138 targets TERT and PD-L1. Cell Biol Int. (2017) 41:1056–64. doi: 10.1002/cbin.10826
86. Xu YJ, Zhao JM, Gao C, Ni XF, Wang W, Hu WW, et al. Hsa_circ_0136666 activates Treg-mediated immune escape of colorectal cancer via miR-497/PD-L1 pathway. Cell Signal. (2021) 86:110095. doi: 10.1016/j.cellsig.2021.110095
87. Jiang Z, Hou Z, Liu W, Yu Z, Liang Z, Chen S. circ-Keratin 6c Promotes Malignant Progression and Immune Evasion of Colorectal Cancer through microRNA-485-3p/Programmed Cell Death Receptor Ligand 1 Axis. J Pharmacol Exp Ther. (2021) 377:358–67. doi: 10.1124/jpet.121.000518
88. Viralippurath Ashraf J, Sasidharan Nair V, Saleh R, Elkord E. Role of circular RNAs in colorectal tumor microenvironment. BioMed Pharmacother. (2021) 137:111351. doi: 10.1016/j.biopha.2021.111351
89. Liu Z, Zheng N, Li J, Li C, Zheng D, Jiang X, et al. N6-methyladenosine-modified circular RNA QSOX1 promotes colorectal cancer resistance to anti-CTLA-4 therapy through induction of intratumoral regulatory T cells. Drug Resist Updat. (2022) 65:100886. doi: 10.1016/j.drup.2022.100886
90. Li T, Wang WC, McAlister V, Zhou Q, Zheng X. Circular RNA in colorectal cancer. J Cell Mol Med. (2021) 25:3667–79. doi: 10.1111/jcmm.16380
91. Zeng K, Chen X, Xu M, Liu X, Hu X, Xu T, et al. CircHIPK3 promotes colorectal cancer growth and metastasis by sponging miR-7. Cell Death Dis. (2018) 9:417. doi: 10.1038/s41419-018-0454-8
92. Long F, Lin Z, Li L, Ma M, Lu Z, Jing L, et al. Comprehensive landscape and future perspectives of circular RNAs in colorectal cancer. Mol Cancer. (2021) 20:26. doi: 10.1186/s12943-021-01318-6
93. Zhang F, Su T, Xiao M. RUNX3-regulated circRNA METTL3 inhibits colorectal cancer proliferation and metastasis via miR-107/PER3 axis. Cell Death Dis. (2022) 13:550. doi: 10.1038/s41419-022-04750-8
94. Yang H, Li X, Meng Q, Sun H, Wu S, Hu W, et al. CircPTK2 (hsa_circ_0005273) as a novel therapeutic target for metastatic colorectal cancer. Mol Cancer. (2020) 19:13. doi: 10.1186/s12943-020-1139-3
95. Zheng X, Chen L, Zhou Y, Wang Q, Zheng Z, Xu B, et al. A novel protein encoded by a circular RNA circPPP1R12A promotes tumor pathogenesis and metastasis of colon cancer via Hippo-YAP signaling. Mol Cancer. (2019) 18:47. doi: 10.1186/s12943-019-1010-6
96. Li H, Jin X, Liu B, Zhang P, Chen W, Li Q. CircRNA CBL.11 suppresses cell proliferation by sponging miR-6778-5p in colorectal cancer. BMC Cancer. (2019) 19:826. doi: 10.1186/s12885-019-6017-2
97. Gao Y, Zhou Y, Wei L, Feng Z, Chen Y, Liu P, et al. Hsa_Circ_0066351 acts as a prognostic and immunotherapeutic biomarker in colorectal cancer. Front Immunol. (2022) 13:927811. doi: 10.3389/fimmu.2022.927811
98. Chen Z, He L, Zhao L, Zhang G, Wang Z, Zhu P, et al. circREEP3 drives colorectal cancer progression via activation of FKBP10 transcription and restriction of antitumor immunity. Adv Sci (Weinh). (2022) 9:e2105160. doi: 10.1002/advs.202105160
99. Chen Q, Tang P, Huang H, Qiu X. Establishment of a circular RNA regulatory stemness-related gene pair signature for predicting prognosis and therapeutic response in colorectal cancer. Front Immunol. (2022) 13:934124. doi: 10.3389/fimmu.2022.934124
100. Deng J, Liao S, Chen C, Han F, Lei S, Lai X, et al. Specific intracellular retention of circSKA3 promotes colorectal cancer metastasis by attenuating ubiquitination and degradation of SLUG. Cell Death Dis. (2023) 14:750. doi: 10.1038/s41419-023-06279-w
101. Zeng X, Tang J, Zhang Q, Wang C, Qi J, Wei Y, et al. CircHIPK2 contributes cell growth in intestinal epithelial of colitis and colorectal cancer through promoting TAZ translation. Adv Sci (Weinh). (2024) 11:e2401588. doi: 10.1002/advs.202401588
102. Zheng L, Liang H, Zhang Q, Shen Z, Sun Y, Zhao X, et al. circPTEN1, a circular RNA generated from PTEN, suppresses cancer progression through inhibition of TGF-beta/Smad signaling. Mol Cancer. (2022) 21:41. doi: 10.1186/s12943-022-01495-y
103. Ju HQ, Zhao Q, Wang F, Lan P, Wang Z, Zuo ZX, et al. A circRNA signature predicts postoperative recurrence in stage II/III colon cancer. EMBO Mol Med. (2019) 11:e10168. doi: 10.15252/emmm.201810168
104. Ghafouri-Fard S, Taheri M, Hussen BM, Vafaeimanesh J, Abak A, Vafaee R. Function of circular RNAs in the pathogenesis of colorectal cancer. BioMed Pharmacother. (2021) 140:111721. doi: 10.1016/j.biopha.2021.111721
105. Dong JX, Zhang LF, Liu DB, Li ZG, Gao F, Wang LP, et al. Circular ribonucleic acid circ-FADS2 promotes colorectal cancer cell proliferation and invasion by regulating miR-498/S100A16. J Physiol Pharmacol. (2022) 73. doi: 10.26402/jpp.2022.4.04
106. Xu Y, Xu X, Ocansey DKW, Cao H, Qiu W, Tu Q, et al. CircRNAs as promising biomarkers of inflammatory bowel disease and its associated-colorectal cancer. Am J Transl Res. (2021) 13:1580–93.
107. Verduci L, Tarcitano E, Strano S, Yarden Y, Blandino G. CircRNAs: role in human diseases and potential use as biomarkers. Cell Death Dis. (2021) 12:468. doi: 10.1038/s41419-021-03743-3
108. Jiang X, Peng M, Liu Q, Peng Q, Oyang L, Li S, et al. Circular RNA hsa_circ_0000467 promotes colorectal cancer progression by promoting eIF4A3-mediated c-Myc translation. Mol Cancer. (2024) 23:151. doi: 10.1186/s12943-024-02052-5
109. Chen Y, Yao L, Tang Y, Jhong JH, Wan J, Chang J, et al. CircNet 2.0: an updated database for exploring circular RNA regulatory networks in cancers. Nucleic Acids Res. (2022) 50:D93–D101. doi: 10.1093/nar/gkab1036
110. Nguyen TH, Nguyen HN, Vu TN. CircNetVis: an interactive web application for visualizing interaction networks of circular RNAs. BMC Bioinf. (2024) 25:31. doi: 10.1186/s12859-024-05646-4
111. Aparo A, Avesani S, Parmigiani L, Napoli S, Bertoni F, Bonnici V, et al. EasyCircR: Detection and reconstruction of circular RNAs post-transcriptional regulatory interaction networks. Comput Biol Med. (2025) 188:109846. doi: 10.1016/j.compbiomed.2025.109846
112. Dal Molin A, Gaffo E, Difilippo V, Buratin A, Tretti Parenzan C, Bresolin S, et al. CRAFT: a bioinformatics software for custom prediction of circular RNA functions. Brief Bioinform. (2022) 23:bbab601. doi: 10.1093/bib/bbab601
113. Zhang W, Liu Y, Min Z, Liang G, Mo J, Ju Z, et al. circMine: a comprehensive database to integrate, analyze and visualize human disease-related circRNA transcriptome. Nucleic Acids Res. (2022) 50:D83–92. doi: 10.1093/nar/gkab809
114. Li H, Xie M, Wang Y, Yang L, Xie Z, Wang H. riboCIRC: a comprehensive database of translatable circRNAs. Genome Biol. (2021) 22:79. doi: 10.1186/s13059-021-02300-7
115. Du J, Zhang L, Ma H, Wang Y, Wang P. Lidocaine suppresses cell proliferation and aerobic glycolysis by regulating circHOMER1/miR-138-5p/HEY1 axis in colorectal cancer. Cancer Manag Res. (2020) 12:5009–22. doi: 10.2147/CMAR.S244973
116. Ren Y, Manoharan T, Liu B, Cheng CZM, En Siew B, Cheong WK, et al. Circular RNA as a source of neoantigens for cancer vaccines. J Immunother Cancer. (2024) 12:e008402. doi: 10.1136/jitc-2023-008402
117. Huang D, Zhu X, Ye S, Zhang J, Liao J, Zhang N, et al. Tumour circular RNAs elicit anti-tumour immunity by encoding cryptic peptides. Nature. (2024) 625:593–602. doi: 10.1038/s41586-023-06834-7
118. Yu H, Wen Y, Yu W, Lu L, Yang Y, Liu C, et al. Optimized circular RNA vaccines for superior cancer immunotherapy. Theranostics. (2025) 15:1420–38. doi: 10.7150/thno.104698
119. Amaya L, Grigoryan L, Li Z, Lee A, Wender PA, Pulendran B, et al. Circular RNA vaccine induces potent T cell responses. Proc Natl Acad Sci U S A. (2023) 120:e2302191120. doi: 10.1073/pnas.2302191120
120. Feng Z, Zhang X, Zhou J, Li Q, Chu L, Di G, et al. An in vitro-transcribed circular RNA targets the mitochondrial inner membrane cardiolipin to ablate EIF4G2(+)/PTBP1(+) pan-adenocarcinoma. Nat Cancer. (2024) 5:30–46. doi: 10.1038/s43018-023-00650-8
121. Jiang T, Wang H, Liu L, Song H, Zhang Y, Wang J, et al. CircIL4R activates the PI3K/AKT signaling pathway via the miR-761/TRIM29/PHLPP1 axis and promotes proliferation and metastasis in colorectal cancer. Mol Cancer. (2021) 20:167. doi: 10.1186/s12943-021-01474-9
Keywords: circular RNA, colorectal cancer, immunotherapy, diagnosis, precision medicine
Citation: Cao Y, He Y, Liao L and Xu L (2025) Circular RNAs perspective: exploring the direction of immunotherapy for colorectal cancer. Front. Oncol. 15:1554179. doi: 10.3389/fonc.2025.1554179
Received: 01 January 2025; Accepted: 24 March 2025;
Published: 11 April 2025.
Edited by:
Luciano Cascione, Institute of Oncology Research (IOR), SwitzerlandReviewed by:
Antonino Aparo, University of Verona, ItalyCopyright © 2025 Cao, He, Liao and Xu. This is an open-access article distributed under the terms of the Creative Commons Attribution License (CC BY). The use, distribution or reproduction in other forums is permitted, provided the original author(s) and the copyright owner(s) are credited and that the original publication in this journal is cited, in accordance with accepted academic practice. No use, distribution or reproduction is permitted which does not comply with these terms.
*Correspondence: Lingshan Liao, bW9tbzI3OUAxNjMuY29t; Lixin Xu, eGx4bWQyMDYwQGhvdG1haWwuY29t
Disclaimer: All claims expressed in this article are solely those of the authors and do not necessarily represent those of their affiliated organizations, or those of the publisher, the editors and the reviewers. Any product that may be evaluated in this article or claim that may be made by its manufacturer is not guaranteed or endorsed by the publisher.
Research integrity at Frontiers
Learn more about the work of our research integrity team to safeguard the quality of each article we publish.