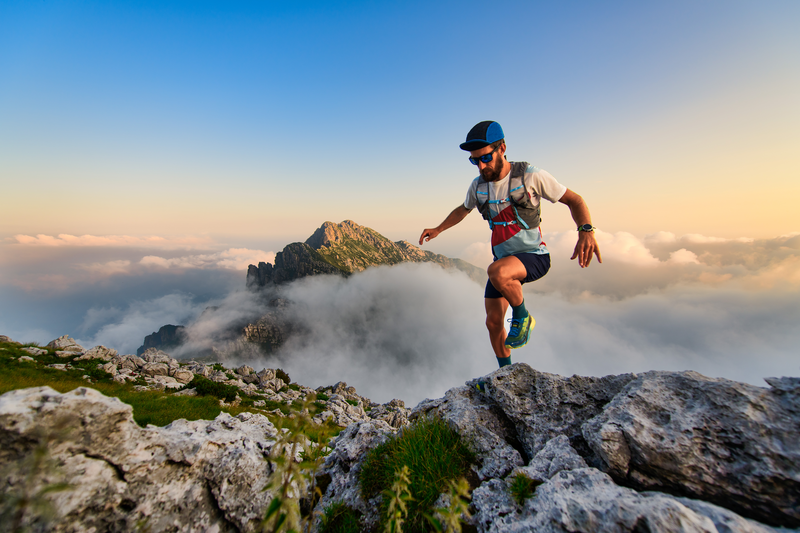
94% of researchers rate our articles as excellent or good
Learn more about the work of our research integrity team to safeguard the quality of each article we publish.
Find out more
SYSTEMATIC REVIEW article
Front. Oncol. , 21 February 2025
Sec. Molecular and Cellular Oncology
Volume 15 - 2025 | https://doi.org/10.3389/fonc.2025.1547889
Background: circHIPK3 role in cancer as oncogene or tumor suppressor is still debated, therefore, this study aimed to understand the dual role of this circRNA in different cancers. Furthermore, all available evidence of circHIPK3 interactions with sponged-miRNA and RBPs in oncological diseases were systematically gathered to better understand the its functional role in cancer.
Methods: PubMed, BioMedCentral, Web of Science, Embase and Scopus databases were searched for articles published until October 2024, following the PRISMA guideline. In computational analysis, miRNAs’ sponged target genes and RBPs were used for gene enrichment in KEGG, REACTOME and Gene Ontology, and TISSUES expression. miRTargetLink 2.0 was used to search for target genes, and STRING v.12.0 for gene enrichment.
Results: circHIPK3 can regulate 33 miRNAs which regulate 399 target genes, and that were mainly enriched in major biological pathways important for cancer development and promoting. circHIPK3/miR-124-3p/miR-637/miR-338-3p are the most well documented interactions in cancers that may control MAPK, Jak/STAT3, Wnt/β-catenin, and PI3K/Akt signaling pathways. circHIPK3 regulates miRNAs that modulate genes responsible for chemoresistance, such as ATP-binding cassette and solute carrier transporters genes, and DNA repair genes. circHIPK3 has binding sites for RBPs, which participate mainly of RNA processing and control, and gene expression regulation. Finally, we believe that it has an onco-circRNA role in most cancers, except in bladder cancer, where it has a TS-circRNA function likely due to the microenvironment permeated by high amounts of hydrogen peroxide.
Conclusion: circHIPK3 dysregulation is an important mechanism for cancer establishment, progression and chemoresistance making it an interesting molecule with a potential therapeutic target.
Bladder, breast, cervix and corpus uteri, colorectum, leukemia, liver, lung, esophagus, ovary, pancreas, prostate, stomach, and thyroid cancers stand out among the types of tumors with the highest incidence and mortality in the world population (1). Integrative genomic studies and protein analysis have characterized and identified a complex but not fully understood interaction network involved in tumors’ molecular pathogenesis, such as chromosomal instability, microsatellite instability, hypermethylation phenotypes, gene mutation, non-coding RNA (ncRNA), and protein aberrant expression (2).
A series of recent studies have reported in cancer the dysregulation of a new class of ncRNA, called circular RNA (circRNA) (3). CircRNAs are classified as long ncRNAs due to their >200 nt length, and are derived from pre-mRNA that have their 5’ and 3’ ends covalently joined in a closed loop structure through backsplicing (4). Among the hundreds of dysregulated circRNAs in cancer, circHIPK3 stands out as one of the most reported in many types (3, 5–73). circHIPK3 or hsa_circ_0000284 is derived from exon 2 (1,099 bp) of the Homeodomain Interacting Protein Kinase 3 (HIPK3) gene, located on human chromosome 11 (chr11:33278868-33378568) (5).
This circRNA was described acting as both RNA-binding proteins (RBP) and miRNA sponge (3, 5–73) in different types of cancers, but the miRNA sponge function is the most explored and understood one (3, 5, 8, 11–24, 26–28, 33–58, 60, 62, 64–73). For instance, one of the first reports for circHIPK3 found its overexpression in cancer cells and identified at least 18 binding sites for 9 different miRNAs; it was also noticed that it acts as a modulator of cancer cell growth by sponging miR-124-3p (5). In addition, circHIPK3 was predicted to have binding sites for 50 different RBPs (3, 30).
Several studies on different types of cancers reported the upregulation of circHIPK3 and its important role as a miRNAs’ sponge contributing to cancer onset and development. In blood (6, 23), bone (60), breast (11–16), cerebral (38–42), gastrointestinal (5, 19–22, 24–30, 32–36, 43–47, 64, 65), gynecological (17, 18, 61, 62), head and neck (55–58), kidney (72, 73), lung (48–53), prostate (66–70), and skin (54) cancers it is overexpressed, and is able to promote cell proliferation, migration, invasion, apoptosis inhibition, metastasis, and chemoresistance. For example, its overexpression can affect the axes miR-193a-5p/HMGB1/PI3K/AKT in breast cancer (11), miR-338-3p/HIF-1α in cervical (17), miR-637/STAT3/Bcl-2/beclin1 in colorectal (19), miR-124/B4GALT1, NF-κB in chronic myeloid leukemia (23), miR-124-3p/STAT3 in glioma (38), miR-124/SphK1/STAT3/CDK4 in lung (48), miR-215–5p/YY1 in melanoma (54) and miR-7/VEGF in ovarian (62), contributing to cancer cell growth, proliferation, invasion, migration, epithelial-mesenchymal transitions, apoptosis inhibition, and chemoresistance.
The under expression of circHIPK3 has also been reported in some studies (31, 59, 63, 71), mainly for bladder cancer (7–10). For example, its under expression can affect the miR-588/HPSE axis favoring cell migration, invasion, and angiogenesis (8). Its reduced expression is related to progression (7) and gemcitabine resistance in bladder cancer (9), and it can negatively regulate autophagy (10).
The dysregulation of circHIPK3 is undoubtedly an important mechanism for cancer development. However, its biological role as oncogenic (onco-circ) and/or tumor suppressor (TS-circ) among the different types of tumors is not clear yet and well established. In most cancers, circHIPK3 is upregulated (5, 6, 11–30, 32–58, 60–62, 64–70, 72, 73), but it is known to be downregulated in bladder cancer (7–10). Therefore, the careful searching for new pathways that converge to different tumors may improve the understanding of the mechanisms involved in the pathogenesis mediated by this circRNA.
Here, we gathered all available evidence associated with the mRNA-miRNA-circHIPK3-RBPs axis in oncological diseases in search of its functional role in cancer. The evidence demonstrates that it has a wide onco-circRNA role in cancer, except in bladder cancer, in which it likely has a H2O2-dependent TS-circRNA function. Furthermore, the dysregulation of this molecule is an important mechanism for resistance to a broad spectrum of chemotherapy drugs. In this scenario, these data open new perspectives towards its use as a potential therapeutic target in cancers.
We followed the Preferred Reporting Items for Systematic Reviews and Meta-Analyses (PRISMA) guideline to perform this study (74). This study is currently submitted to PROSPERO, under submission number: 628708.
This study is a systematic review with computational analysis on the role of circHIPK3 in cancer. This study does not require approval by Ethic Committee.
We selected only original studies that conducted experimental validation by “strong evidence” (e.g., RT-qPCR, western blot, luciferase reporter and/or cell assay) of circHIPK3 in human cancer. Papers based on computational prediction analysis without experimental validation or retracted were excluded. No language restriction was applied in the search.
A literature search was performed for studies reporting circHIPK3 in cancers in PubMed, BioMedCentral, Web of science, Embase and Scopus databases. The search was conducted until October 1, 2024, in which we used the following entries: “circHIPK3 OR hsa_circ_0000284” and “cancer” combined with boolean operators.
For eligibility, two independent reviewers (M.M.C. and L.R-das-M.) screened all records provided by the databases. Titles and abstracts were reviewed, followed by full-text reviews of potentially eligible studies. Review disagreements were resolved through consensus among reviewers and/or consultation with a third reviewer (A.L.P.).
Two reviewers (M.M.C. and L.R-das-M.) independently extracted data from the included studies using a data extraction form that was provided as Supplementary Material. Data were collected on the cancer type, sample type and size, circHIPK3 expression profile, sponged miRNAs, experimental validation methods, country of origin, biological function and/or clinical significance, first author and year of publication. Extraction disagreements were resolved through consensus among reviewers and/or consultation with a third reviewer (A.L.P.).
To understand the functional role of circHIPK3, its interactions with miRNAs and RBPs was explored. At first, sponged miRNAs were collected from the selected studies, and their target genes were found through miRTargetLink 2.0 tool (https://ccb-compute.cs.uni-saarland.de/mirtargetlink2/) (75). This tool was chosen among many others because it is directly connected to the miRTarBase database (http://miRTarBase.cuhk.edu.cn/) (76), which provides information on experimentally validated interaction between the miRNA and its target genes.
In miRTargetLink 2.0, only target genes whose interaction with their target miRNA was experimentally validated by “strong evidence” (e.g., RT-qPCR, western blot, luciferase reporter and/or cell assay) were included in the analyses. The RBPs that were used in functional enrichment, were obtained from previous studies that predicted the circHIPK3-RBPs interactions (3, 30).
miRNAs-target genes and RBPs were used to perform functional analyses in Kyoto Encyclopedia of Genes and Genomes – KEGG (https://www.genome.jp/kegg/), Reactome (https://reactome.org/) pathways, Gene Ontology – GO (http://geneontology.org/), and TISSUES expression database 2.0 (https://tissues.jensenlab.org) through STRING: functional protein association networks v.12.0 tool (https://string-db.org/) (77). For the statistical significance of gene enrichment, STRING v.12.0 default was applied for p-value adjustment to false discovery rate (FDRadj), using Benjamini-Hochberg correction method, and FDRadj<0.05.
Systematic search in the five literature databases allowed the selection of 69 eligible studies, which were considered during the analyses (Figure 1).
circHIPK3 dysregulation was reported by 69 studies (8 downregulated and 61 upregulated study report) in 21 different cancers, being able to sponge 33 different miRNAs experimentally validated (Table 1 and Supplementary Table S1). Details of the studies included in the analyses are contained in Table 1 and Supplementary Table S1.
Eight studies found this circRNA downregulated (7–10, 31, 59, 63, 71). However, some of them used paraffin preserved tissue (31), samples from patients receiving chemotherapy (59), controls samples from patients with another type of tumor (63), and normal samples collected very close to the tumor (e.g., normal tissues ≥ 1cm far away to tumor) (71). These factors may cause biases for the evaluation of gene expression. For instance, studies demonstrated that long RNAs are more susceptible to degradation than small RNAs ones in paraffin preserved samples (78, 79). In addition, samples collected adjacent to the tumor (and used as normal tissue) can be influenced by the tumor microenvironment and have their gene expression profile altered when compared to a truly healthy tissue (field cancerization phenomenon) (79, 80).
The interaction of circHIPK3 and six sponged miRNAs have been reported in more than one cancer type, for example, circHIPK3/miR-124-3p was reported in 13 different studies, circHIPK3/miR-637 in eight studies, circHIPK3/miR-338-3p in four studies, and circHIPK3/miR-326, circHIPK3/miR-485-3p circHIPK3/miR-508-3p and circHIPK3/miR-582-3p in two studies each (Table 1; Supplementary Table S1).
The prognostic and diagnosis value of circHIPK3 in different cancers has been investigated. For example, low expression levels are correlated with high pathological grade, risk of progression, lymph node metastasis and gemcitabine resistance in bladder cancer (7, 9). On the other hand, high expression levels are correlated with a worse prognosis (11), as well as paclitaxel (14) and trastuzumab (16) resistance in breast cancer. In colorectal cancer, its upregulation is correlated with tumor size, lymph node metastasis, distant metastasis, recurrence, poor survival and oxaliplatin resistance (19). It is correlated to poor overall survival rate in cholangiocarcinoma (23), chronic myeloid leukemia (24) and ovarian cancer (62). In osteosarcoma, it is correlated to shorter overall survival times and poor prognosis, serving as a biomarker (AUC = 0.783; 0.875) (60, 61). In lung cancer, it has been suggested as a biomarker (AUC = 0.897) (54). In gastric cancer, it is correlated to poor overall survival rate (33), T stage, Ming’s classification and infiltrative type (34), and cisplatin resistance (37). In glioma, it is correlated with unfavorable prognosis (40) and temozolomide resistance (42, 43). In nasopharyngeal carcinoma, it is correlated to lower overall survival and distant metastasis‐free survival rate (56). In oral cancer, it is correlated to distant metastasis, higher tumor staging and shorter survival (59). In pancreatic cancer, it is correlated to gemcitabine resistance (65). In renal cancer, it is correlated to lymph node metastasis and shorter survival rates (73, 74). Therefore, circHIPK3 appears to be potentially useful as a prognostic and diagnostic marker.
Considering ethnic populations, the dysregulation of this circRNA in cancer has been reported mainly in the Asian population, however, it was found in the European population and in the mixed Brazilian population, in South America (Supplementary Table S1).
Except for miR-4524-5p, the other 33 miRNAs can regulate a total of 399 genes experimentally validated by strong evidences (Supplementary Table S2). Enrichment analysis of the 399 target genes in Gene Ontology showed that many of them were enriched in biological processes, cellular structural composition and molecular functions related to cell differentiation, fate, adhesion, motility, migration, growth and death. Furthermore, many have been related to epithelial to mesenchymal transition, inflammation, immunity, gene expression, RNA processing and interaction to messenger and non-coding RNAs (Figure 2; Supplementary Table S3).
In KEGG pathways, gene enrichment was organized into four distinct classes, such as 1) major cancer-related biological pathways, 2) cancer pathways, 3) risk factors-related pathways for cancer development, and 4) chemoresistance-related pathways in cancer (Figure 3). For example, of the 25 highlighted pathways from the “major cancer-related biological pathways” class, we highlight the PI3K-Akt signaling pathway [hsa04151] which has 64 enriched target genes (Supplementary Table S4). Many target genes were also enriched in 18 different cancer pathways, 15 pathways associated to risk factors for the development of different types of cancer, and at least four pathways directly associated to chemoresistance (Figure 3; Supplementary Table S4).
Considering pathways associated to anti-cancer agent resistance treatments, some target genes were enrichment in antifolate resistance (8 target genes), platinum drug resistance (18 target genes), PD-L1 expression and PD-1 checkpoint (26 target genes), and EGFR tyrosine kinase inhibitor resistance (34 target genes) pathways (Supplementary Table S4). The target genes such as ABCC1 (regulated by miR-7-5p) and ABCC4 (target of miR-124-3p), ABCG2 (target of miR-212-3p) and GJA1 (target of miR-381-3p) are involved in “efflux transmembrane transporter activity” (GO:0015562; four target genes) (Supplementary Table S3) and platinum drug resistance (hsa01524; 26 target genes; (Supplementary Table S4). In addition, some of these miRNAs also regulate solute transport genes, such as SLC7A5 (target of miR-7-5p), SLC16A1 (target of miR-124-3p), and SLC40A1 (target of miR-485-3p) (Supplementary Table S2). These genes are involved in transmembrane transport activity and chemoresistance (81–83).
We observed that RAD51 (recombinase) and MGMT (O-6-methylguanine-DNA methyltransferase) genes, responsible for repairing DNA damage caused by chemotherapeutic alkylating and platinum derivatives, can be regulated by miR-107 and miR-124-3p, respectively (Supplementary Table S2).
In REACTOME pathways, gene target enrichment was organized into three classes, such as 1) cell cycle-related pathways, 2) “other” – pathways related to immune system, TP53 modulation and extracellular matrix remodeling (important to support cancer invasion and metastasis), and 3) cell death-related pathways (Figure 4; Supplementary Table S5).
Seventy-six target genes can be regulated by at least two different miRNAs, of which we highlighted oncogenes and tumor suppressors that can be regulated by at least three (BCL2, CDH2, CCND1, CD274, DNMT3B, DNMT1, GRN, MYC, MMP9, SP1, PI3KR3, KLF4 and IGF1R), and four (CDK6, CD151 and PTEN) different sponged miRNAs (Supplementary Table S6).
To better understand the importance of these 76 target genes, we performed their gene enrichment and selected biological processes from Gene Ontology that presented a number ≥40 enriched target genes, and in KEGG and REACTOME pathways with a number ≥10 enriched target genes (Figure 5; Supplementary Table S7). These target genes were enriched in functions related to cell control (e.g., differentiation, adhesion, motility, migration, growth and death), to epithelial to mesenchymal transition, inflammation, immunity, and oncogenic viral infection (Figure 5).
Gene enrichment (molecular process) of the 50 RBPs that have binding sites in circHIPK3 (3, 30), showed that these proteins participate of biological processes, cellular structural composition and molecular functions related mainly to RNA metabolism and regulation of gene expression (Figure 6A). We highlight the DDX54, EIF4A3, FMR1, IGF2BP1, IGF2BP2, LIN28B and MOV10, since they have more than 10 circHIPK3 binding sites (Supplementary Table S8).
Figure 6. RBPs enrichment. (A) RBPs enrichment in Gene Ontology. (B) RBPs enrichment in TISSUE expression database.
We highlight the molecular function of six RBPs (HNRNPA2B1, IGF2BP1, IGF2BP2, IGF2BP3, YTHDF1, and YTHDC1) related to N6-methyladenosine-(m6A)-containing RNA binding [GO:1990247] (Figure 6A; Supplementary Table S8). The m6A markers are important degradation signals for several classes of RNA, which are recognized by m6A-binding proteins (“readers”), such as HNRNPA2B1, IGF2BP1/2/3, YTHDF1/2/3 and YTHDC1/2 (84).
Some RBPs compose the Cajal body (DKC1, FBL, FMR1, HNRNPA2B1, NOP58, and SMNDC1) and P-body (CAPRIN1, ELAVL1, IGF2BP1, IGF2BP2, IGF2BP3, LIN28A, MOV10, UPF1, and YTHDF1) organelle-like structures (Figure 6A; Supplementary Table S8). These non-membrane organelle-like structures are associated to RNA storage and decomposition, and cancer (85, 86). Additionally, we found that many of these RBPs are abundantly expressed in cancer cells and in human tissues (Figure 6B).
In recent years, studies have demonstrated and reinforced the importance of circRNAs in cancer pathogenesis, and the clinical impact of mRNA-target-miRNA/circHIPK3/RBP interaction network in cell biological processes (3).
Sixty-seven studies in cancer report the dysregulation of circHIPK3 in cancer, and that it may interfere in 33 miRNAs activity by sponging them (5, 8, 11–24, 26–28, 33–58, 60, 62, 64–73). These studies show that the homeostasis break of circHIPK3/33-miRNAs/mRNA-targets results in disturbance of pathways that lead to the loss of cellular control, causing proliferation, migration, invasion, evasion of apoptosis and autophagy, EMT, metastasis and chemoresistance, and its overexpression was associated to poor prognosis (5–73).
Indeed, our functional analyses show that many of the target genes of sponged miRNAs can modulate pathways that control these biological and cellular processes. For example, in our analyses PI3K-Akt signaling pathway was highlighted due to the involvement of the large number of target genes identified, of which we highlight the AKT1, AKT3, BDNF, CCND2, CDK4, CDK6, COL4A1 and PIK3R1. These genes are directly affected by circHIPK3 in gastric cancer [miR-124/miR-29b/COL4A1/CDK6 (33), miR-876-5p/PIK3R1 (35) and miR-637/AKT1 (36)], gallbladder cancer [miR-124/CDK6 (28)], breast cancer [circHIPK3/AKT (11)], glioma [miR-124/CCND2 (40)], lung cancer [miR-124/CDK4 (48) and miR-107/BDNF (50)] and oral squamous cell carcinoma [miR-637/AKT (58)]. PI3K-Akt is a highly conserved and extremely important pathway for cellular homeostasis, as it is responsible for promotes cell proliferation, survival, metabolism, growth, apoptosis and angiogenesis in response to extracellular signals (87).
Dysregulation of circHIPK3/miR-124-3p has been reported in breast (15), chronic myeloid leukemia (23), esophageal (26), gallbladder (28), gastric (33), glioma (38, 40), hepatocellular carcinoma (43, 46, 47), lung (48, 49), and oral squamous cell carcinoma (56) cancers, demonstrating it to be an important interaction for cancer development. Indeed, miR-124-3p is a tumor suppressor miRNA in cancers and is able to control PI3K-Akt signaling pathway (88). circHIPK3/miR-637 has been reported in colorectal (19), cholangiocarcinoma (21), esophageal (27), gastric (36), lung (53), oral squamous cell carcinoma (58), osteosarcoma (60), and renal carcinoma (71) cancers. miR-637 is able to modulating the Jak/STAT3, Wnt/β-catenin, and PI3K/Akt signaling pathways; it is downregulated in cancer and is associated to larger tumors and later tumor node metastasis staging in cancer patients (89). circHIPK3/miR-338-3p has been reported in cervical (17), gastric (34), hepatocellular carcinoma (44), and prostate (66, 68) cancers. miR-338-3p is downregulated in cancer and has an important role during tumor progression by modulation of Wnt, MAPK, and PI3K/Akt signaling pathways (90). Interestingly, MAPK, Jak/STAT3, Wnt/β-catenin, and PI3K/Akt signaling pathways were abundantly enriched by the target genes analyzed (Figure 2). Therefore, these evidence together suggest that circHIPK3 acts in the functional silencing of these tumor suppressor miRNAs, acting as an onco-cirRNA and favoring cancer development.
circHIPK3 functional role and expression in cancer remains dubious, for example, a massive number of studies demonstrate that circHIPK3 is upregulated (5, 6, 11–30, 32–58, 60–62, 64–70, 72, 73), while few studies it is downregulated (7–10, 31, 59, 63, 71). This circRNA was found downregulated in bladder cancer (7–10), and its expression is even lower in muscle invasive bladder cancer (e.g., tumor invades the lamina propria and detrusor muscle) when compared with non-muscle invasive bladder cancer (e.g., tumor limited to the urothelium) (91). An interesting aspect associated to the normal bladder physiology is the existence of a urinary microenvironment permeated by the presence of considerable amounts of hydrogen peroxide (H2O2), a reactive oxygen species (ROS) (92). It was also identified that in cancer patients (e.g., breast, cervical, esophagus and laryngeal carcinoma) the urine concentration of H2O2 is two- to three-fold higher than in healthy people (92). In human bladder cancer (urothelial carcinoma), was showed that elevated levels of ROS induced by Nox4 enzyme (a H2O2-generator enzyme) are required for tumor initiation and progression (93). It was also observed that H2O2 can induce a metastatic phenotype in bladder cancer cells (94). In mouse model, was observed that healthy urothelial cell produce H2O2 in response to calcium signaling (95). Curiously, H2O2 is able to put down circHIPK3 expression in osteoblast (96), cardiomyocyte (97) and lens epithelial (98) cell human. Therefore, the circHIPK3 downregulation observed in bladder cancer may be a consequence of the normal and tumoral H2O2-elevated milieu characteristic of this organ.
Many target genes were enriched in pathways related to risk factors associated to the cancer, such as type II diabetes mellitus, inflammatory bowel disease and tuberculosis (Figure 2). Indeed, circHIPK3 contributes to hyperglycemia and insulin resistance by disturbing the miR-192-5p/FOXO1 axis homeostasis (99) and is upregulated in type II diabetes patients (100), and it was found upregulated in acute pancreatitis (101), an important risk factor for the pancreatic cancer development (102).
This circRNA was observed to be downregulated in Crohn’s disease and ulcerative colitis (103), conditions that lead to atrophy of the intestinal epithelium (104) and favors the onset of colorectal cancer (105). However, its expression increases the proliferation of intestinal cells by sponging miR-29b, contributing to the renewal of the intestinal mucosa after injury caused by these diseases (103). Therefore, circHIPK3 acts on the proliferation of intestinal mucosa cells, maintaining their renewal and homeostasis (103), however, when dysregulated (e.g., upregulated) it can lead to colorectal cancer (19, 20). In addition, circHIPK3 can sponge miR-29b-3p and abolish its function in gastric cancer (33), so this interaction may be an important mechanism for epithelial mucosal proliferation in gastrointestinal cancers. Interestingly, the circHIPK3 downregulation observed in Crohn’s disease and ulcerative colitis (91), may be a consequence of the high levels of H2O2 produced during the development of these diseases (106, 107).
In lung cancer, circHIPK3 is overexpressed (48–52). Its overexpression also induces pulmonary fibrosis by interfering with the circHIPK3/miR-30a-3p/FOXK2 axis (108) and by inducing fibroblast-to-myofibroblast transition via regulation of circHIPK3/miR-338-3p/SOX4/COLA1A1 (109), this condition is a factor in risk for lung cancer (110). Furthermore, its overexpression is related to pulmonary arterial hypertension development (111), whose prevalence is higher in patients with lung cancer (112).
circHIPK3 is upregulated in acute kidney injury and affects the activity of miR-93-5p (113), miR-124-3p (114), miR-148b-3b (114, 115) and miR-338-3p (116). Upregulation of circHIPK3 is also associated to chronic tubulointerstitial nephritis and renal tubulointerstitial fibrosis by regulating miR-30a/profibrotic-proteins axis (117). In renal tubular epithelial cell, its upregulation induces cell proliferation and inhibition of the apoptosis by miR-326 and miR-487a-3p modulation (118). It was also found overexpressed in podocytes subjected to injury caused by high-glucose concentrations (119), this injury is a common condition in diabetes mellitus. These conditions are an important risk factor for the development of kidney cancer (120), since it is also overexpressed in this type of cancer (72, 73). Interestingly, circHIPK3 regulates the miR-124-3p (15, 23, 26, 28, 33, 38, 40, 43, 46–49, 56), miR-326 (12, 13) and miR-338-3p (17, 34, 44, 66, 68) activity in cancer.
circHIPK3 plays an important role in resistance to many anti-cancer agents. For example, its overexpression favors oxaplatin resistance, apoptosis and autophagy inhibition in colorectal cancer by abolishing the regulatory function of miR-637 on STAT3/Bcl-2/beclin1 signaling pathway (19). In gastric cancer, it promotes cisplatin resistance as a consequence of apoptosis, autophagy, and ferroptosis inhibition by abolishing the regulatory power of miR-508-3p on the ↑Bcl-2/beclin1/SLC7A11 axis (37). The effect of circHIPK3 on gemcitabine resistance is attributed to its negative regulatory role on miR-330-5p which enables RASSF1 activity, favoring proliferation, invasiveness, migration, EMT, and apoptosis inhibition in pancreatic cancer (64). In breast cancer, paclitaxel resistance occurs due to circHIPK3/miR-1286/HK2 modulation and consequent cell cycle progression and apoptosis inhibition (14); and trastuzumab resistance occurs through the transmission of circHIPK3 via exosomes and miR-582-3p/RNF11 axis regulation, which promotes cell proliferation, invasion, and apoptosis inhibition (16). Temozolomide resistance arises as a consequence of the modulation of the cell concentration (IC50) of temozolomide and apoptosis inhibition by circHIPK3/miR-524-5p/KIF2A (41) and exosomal-circHIPK3/miR-421/ZIC5 (42) axis regulation in glioblastoma. Therefore, although the details of the molecular mechanisms leading to circHIPK3-mediated chemoresistance are not fully understood, interference by this circRNA in distinct and complex pathways resulting in the inhibition of cell death may be a key mechanism in this process.
In bladder cancer, it has been observed that circHIPK3 downregulation favors gemcitabine resistance (9), although the underlying mechanism remains unknown. NXPH4 overexpression has been shown to induce gemcitabine resistance in bladder cancer by increasing ROS and glycolysis levels (121). Since ROS is a known factor that can downregulate circHIPK3 (96–98), this suggests a potential link. Furthermore, low expression levels of LOXL4 and SRSF2 – genes regulated by miR-29b-3p and miR-193a-3p (see Supplementary Table S2) – have been associated with inhibited apoptosis and enhanced multi-drug resistance in bladder cancer (122). Therefore, the ROS-induced NXPH4/↓circHIPK3/↑miR-29b-3p/↑miR-193a-3p/↓LOXL4/↓SRSF2 axis may represent a potential mechanism through which circHIPK3 downregulation contributes to gemcitabine resistance in bladder cancer.
Several target genes were enriched in the platinum drug resistance pathway (hsa01524), including the BCL2 oncogene, which can be regulated by at least three miRNAs sponged by circHIPK3 (miR-7-5p, miR-29b-3p, and miR-448). In fact, circHIPK3 overexpression can affect the activity this gene and induce resistance to oxaliplatin in colorectal cancer (19) and to cisplatin in gastric cancer (37). Genes belonging to ATP-binding cassette (ABC) transportes, such as ABCC1 (regulated by miR-7-5p), ABCC4 (regulated by miR-124-3p), ABCG2 (regulated by miR-212-3p), and GJA1 (regulated by miR-381-3p) were enriched in efflux transmembrane transporter activity (GO:0015562). In cancer cells that have multidrug resistance generally upregulate these genes, which are associated with the efflux of chemotherapeutics out of cells and, therefore, decrease chemosensitivity to anticancer drugs (82, 83). In addition, SLC7A5 (regulated by miR-7-5p), SLC16A1 (regulated by miR-124-3p), and SLC40A1 (regulated by miR-485-3p) genes, belong to the transmembrane solute transport (SLC) and are involved in chemoresistance in many of the cancers studied here (81). Interestingly, circHIPK3/miR508-3p interaction can modulate SLC7A11 activity and induce cisplatin resistance in gastric cancer (37). Therefore, the modulation of these genes by sponging these miRNAs could be another important mechanism associated to chemoresistance involving circHIPK3.
miR-124-3p can regulate the MGMT gene, responsible for repairing DNA damage caused by chemotherapeutic alkylating and alkylating-like agents (e.g., gemcitabine, temozolomide and platinum-based drugs). Indeed, MGMT overexpression can lead to apoptosis escape induced by alkylating agents (123). For example, MGMT overexpression induces resistance to gemcitabine in pancreatic cancer (124), to cisplatin in colorectal cancer (125) and to temozolomide in glioma (126, 127) and estrogen receptor positive breast cancer (128). Thus, circHIPK3/miR-124-3p/MGMT may modulate resistance to multi-drugs in these cancers. On the other hand, the RAD51 recombinase gene (which encodes a protein that is essential for repairing damaged DNA) is regulated by miR-107. Interestingly, overexpression of this protein is associated with gemcitabine resistance in lung (129) and contributes to chemotherapy-induced damage and the destabilization of genetic material in cancer cells (130). Therefore, the circHIPK3/miR-107/RAD51 axis may be another mechanism that induces chemotherapy resistance in cancer.
N6-methyladenosine (m6A) is a modification that occurs internally in long RNAs (e.g., circRNA) that are recognized by “readers” (e.g., IGF2BPs), and when deregulated they can lead to cancer (83). Interestingly, circHIPK3 appears to be a peculiar molecule, because despite not having any m6A modification in its structure that signals for the binding of readers such as IGF2BPs (131), it surprisingly maintains many binding sites for RNA-binding proteins (RBPs), including for IGF2BPs group (Supplementary Table S8). IGF2BP1, IGF2BP2 and IGF2BP3 are dysregulated in many tumor types (84), being associated with chemoresistance in glioblastoma (132), ovarian (133) and colorectal (134) cancer. In fact, circHIPK3 sequesters IGF2BP2 allowing the target gene of this RBP, STAT3 oncogene (responsible for controlling cell proliferation and survival), to be expressed. Therefore, circHIPK3 would act as competing endogenous RNA (ceRNA) for IGF2BP2 (92). It has also been reported that this circRNA can act as a scaffold for E3 ubequitin ligase (135). Interestingly, the existence of m6A and recognition by YTHDF2 “readers” is a pathway by which circRNA are degraded (136), so the absence of m6A in circHIPK3 may have conferred more stability to this complex molecule.
FMR1 is an interesting RBP, since it is associated with cancer cell growth, metastasis, EMT, apoptosis, and angiogenesis (137), and has 35 binding sites in circHIPK3 (Supplementary Table S8). Recent evidence demonstrates that circHIPK3 binds to BRCA1 messenger RNA, conferring stability and protection against FRM1 protein (a negative regulator of BRCA1), allowing the effective expression of this oncogene. Therefore, circHIPK3 acts as ceRNA by binding to BRCA1 messenger RNA using the same binding site used by FRM1, and this interaction causes chemoresistance to DNA-damaging drugs (138).
Other RBPs such as DDXR54, EIF4A, LIN28B and MOV10 have multiple binding sites for circHIPK3. These RBPs are important for stability and functional role of circRNAs in several types of cancers. For example, DDXR54 binding to long non-coding RNAs and confers stability to genes that contribute to cell growth in gastric cancer (139) and that facilitate stemness and EMT of osteosarcoma cells (140). EIF4A modulates the expression of some circRNAs by flanking specific sequences of pre-mRNA of its target gene, contributing to cisplatin resistance in bladder cancer (141). circHIPK3/miR-107/LIN28B axis may be a mechanism of chemoresistance in gastric (3) and ovarian (133) cancers. MOV10 can bind to circ-DICER1 and modulate the cell viability, migration, and angiogenesis in glioma (142). Therefore, the interaction of circHIPK3 with these RBPs may participate in important mechanisms for the development of cancer and chemoresistance.
Our strategy of combining experimentally validated public data and robust bioinformatics tools for functional analysis made it possible to identify alternative routes that explain the diversity of functions exerted by circHIPK3 and its implication in multidrug resistance. A limitation of this study is that we did not experimentally test the axes modulated by this circRNA suggested here, therefore, future experimental validations of these pathways are necessary. We highlight the need for more studies on gallbladder cancer, leukemia, melanoma, nasopharyngeal carcinoma and thyroid cancer to strengthen the amount of evidence, as well as expanding the representation of other ethical populations not yet studied regarding the expression of this circRNA. Finally, our results undoubtedly open new perspectives towards understanding how circHIPK3 can exert a modulating role on establishment, progression and chemoresistance in different cancers.
In this study, we observed that circHIPK3 is dysregulated and that it can regulate 33 miRNAs in different types of cancer, whose target genes control important processes and biological pathways for the cancer establishment and maintenance. circHIPK3/miR-124-3p/miR-637/miR-338-3p are the most well documented interactions in various cancers types, and can control MAPK, Jak/STAT3, Wnt/β-catenin, and PI3K/Akt signaling pathways, and may be important for support the initiation and establishment of the cancer. miR-7-5p/ABCC1/SLC7A5, miR-107/RAD51, miR-124-3p/ABCC4/SLC16A1/MGMT, miR-212-3p/ABCG2, miR-381-3p/GJA1 and miR-485-3p/SLC40A1 may modulate pathways that confer chemoresistance to cancer cells. circHIPK3 contains multiple sites for the same RBPs (e.g., DDX54, EIF4A3, FMR1, IGF2BP1, IGF2BP2, LIN28B and MOV10), many of which are involved in chemoresistance and organelle-like structures, such as Cajal body and P-body which are associated with cancer. Additionally, circHIPK3 is upregulated in cancer in general acting as an onco-circRNA, except in bladder cancer, which has a likely TS-circRNA function due to the microenvironment with large amounts of H2O2 present in this organ. Therefore, circHIPK3 is a complex and multifunctional molecule that favors the establishment, progression and chemoresistance of cancers, making it an interesting molecule with a potential therapeutic target.
The original contributions presented in the study are included in the article/Supplementary Material, further inquiries can be directed to the corresponding author/s.
MC: Data curation, Formal analysis, Writing – original draft. LR-M: Data curation, Formal analysis, Writing – original draft. AV: Data curation, Visualization, Writing – review & editing. FS: Methodology, Resources, Writing – review & editing. AD: Data curation, Visualization, Writing – review & editing. JM: Data curation, Visualization, Writing – review & editing. CC: Data curation, Investigation, Writing – review & editing. RN: Writing – review & editing. AP: Conceptualization, Data curation, Funding acquisition, Investigation, Project administration, Writing – review & editing.
The author(s) declare financial support was received for the research, authorship, and/or publication of this article. This study was funded by Programa Institucional de Bolsas de Iniciação Científica da UFPA (PROPESP/PIBIC/UFPA). MC was supported by scholarship PIBIC/UFPA.
We thank to Pró-Reitoria de Pesquisa e Pós-Graduação da Universidade Federal do Pará (PROPESP-UFPA).
The authors declare that the research was conducted in the absence of any commercial or financial relationships that could be construed as a potential conflict of interest.
The author(s) declare that no Generative AI was used in the creation of this manuscript.
All claims expressed in this article are solely those of the authors and do not necessarily represent those of their affiliated organizations, or those of the publisher, the editors and the reviewers. Any product that may be evaluated in this article, or claim that may be made by its manufacturer, is not guaranteed or endorsed by the publisher.
The Supplementary Material for this article can be found online at: https://www.frontiersin.org/articles/10.3389/fonc.2025.1547889/full#supplementary-material
1. Ferlay J, Ervik M, Lam F, Laversanne M, Colombet M, Mery L, et al. Global cancer observatory: cancer today (2024). Lyon, France: International Agency for Research on Cancer. Available online at: https://gco.iarc.who.int/today (Accessed January 11, 2024).
2. Hoadley KA, Yau C, Hinoue T, Wolf DM, Lazar AJ, Drill E, et al. Cell-of-origin patterns dominate the molecular classification of 10,000 tumors from 33 types of cancer. Cell. (2018) 173:291–304.e6. doi: 10.1016/j.cell.2018.03.022
3. Pereira AL, Magalhães L, Pantoja RP, Araújo G, Ribeiro-dos-Santos Â, Vidal AF. The biological role of sponge circular RNAs in gastric cancer: main players or coadjuvants? Cancers. (2020) 12:1982. doi: 10.3390/cancers12071982
4. Xiao M-S, Ai Y, Wilusz JE. Biogenesis and functions of circular RNAs come into focus. Trends Cell Biol. (2020) 30:226–40. doi: 10.1016/j.tcb.2019.12.004
5. Zheng Q, Bao C, Guo W, Li S, Chen J, Chen B, et al. Circular RNA profiling reveals an abundant circHIPK3 that regulates cell growth by sponging multiple miRNAs. Nat Commun. (2016) 7:11215. doi: 10.1038/ncomms11215
6. Gaffo E, Boldrin E, Molin AD, Bresolin S, Bonizzato A, Trentin L, et al. Circular RNA differential expression in blood cell populations and exploration of circRNA deregulation in pediatric acute lymphoblastic leukemia. Sci Rep. (2019) 9:14670. doi: 10.1038/s41598-019-50864-z
7. Okholm TLH, Nielsen MM, Hamilton MP, Christensen L-L, Vang S, Hedegaard J, et al. Circular RNA expression is abundant and correlated to aggressiveness in early-stage bladder cancer. NPJ Genom Med. (2017) 2:36. doi: 10.1038/s41525-017-0038-z
8. Li Y, Zheng F, Xiao X, Xie F, Tao D, Huang C, et al. CircHIPK3 sponges miR-558 to suppress heparanase expression in bladder cancer cells. EMBO Rep. (2017) 18:1646–59. doi: 10.15252/embr.201643581
9. Xie F, Zhao N, Zhang H, Xie D. Circular RNA circHIPK3 promotes gemcitabine sensitivity in bladder cancer. J Cancer. (2020) 11:1907–12. doi: 10.7150/jca.39722
10. Wang C, Liu T, Wang J, Cheng C, Zhang Z, Zhang J, et al. CircHIPK3 negatively regulates autophagy by blocking VCP binding to the Beclin 1 complex in bladder cancer. Discovery Oncol. (2023) 14:86. doi: 10.1007/s12672-023-00689-0
11. Chen Z-G, Zhao H-J, Lin L, Liu J-B, Bai J-Z, Wang G-S. Circular RNA CirCHIPK3 promotes cell proliferation and invasion of breast cancer by sponging miR-193a/HMGB1/PI3K/AKT axis. Thorac Cancer. (2020) 11:2660–71. doi: 10.1111/1759-7714.13603
12. Luo N, Liu S, Li X, Hu Y, Zhang K. Circular RNA circHIPK3 promotes breast cancer progression via sponging MiR-326. Cell Cycle. (2021) 20:1320–33. doi: 10.1080/15384101.2021.1939476
13. Qi L, Sun B, Yang B, Lu S. circHIPK3 (hsa_circ_0000284) Promotes Proliferation, Migration and Invasion of Breast Cancer Cells via miR-326. Onco Targets Ther. (2021) 14:3671–85. doi: 10.2147/OTT.S299190
14. Ni J, Xi X, Xiao S, Xiao X. Silencing of circHIPK3 Sensitizes Paclitaxel-Resistant Breast Cancer Cells to Chemotherapy by Regulating HK2 Through Targeting miR-1286. Cancer Manag Res. (2021) 13:5573–85. doi: 10.2147/CMAR.S307595
15. Shi P, Liu Y, Yang H, Hu B. Breast cancer derived exosomes promoted angiogenesis of endothelial cells in microenvironment via circHIPK3/miR-124-3p/MTDH axis. Cell Signal. (2022) 95:110338. doi: 10.1016/j.cellsig.2022.110338
16. Zhang H, Yan C, Wang Y. Exosome-mediated transfer of circHIPK3 promotes trastuzumab chemoresistance in breast cancer. J Drug Targeting. (2021) 29:1004–15. doi: 10.1080/1061186X.2021.1906882
17. Qian W, Huang T, Feng W. Circular RNA HIPK3 promotes EMT of cervical cancer through sponging miR-338-3p to up-regulate HIF-1α. Cancer Manag Res. (2020) 12:177–87. doi: 10.2147/CMAR.S232235
18. Wu S, Liu S, Song H, Xia J. Circular RNA HIPK3 plays a carcinogenic role in cervical cancer progression via regulating miR-485-3p/FGF2 axis. J Investig Med. (2021) 69:768–74. doi: 10.1136/jim-2020-001537
19. Zhang Y, Li C, Liu X, Wang Y, Zhao R, Yang Y, et al. circHIPK3 promotes oxaliplatin-resistance in colorectal cancer through autophagy by sponging miR-637. EbioMedicine. (2019) 48:277–88. doi: 10.1016/j.ebiom.2019.09.051
20. Yan Y, Su M, Qin B. CircHIPK3 promotes colorectal cancer cells proliferation and metastasis via modulating of miR-1207-5p/FMNL2 signal. Biochem Biophys Res Commun. (2020) 524:839–46. doi: 10.1016/j.bbrc.2020.01.055
21. Wang S, Hu Y, Lv X, Li B, Gu D, Li Y, et al. Circ-0000284 arouses Malignant phenotype of cholangiocarcinoma cells and regulates the biological functions of peripheral cells through cellular communication. Clin Sci (Lond). (2019) 133:1935–53. doi: 10.1042/CS20190589
22. Sun J, Feng M, Zou H, Mao Y, Yu W. Circ_0000284 facilitates the growth, metastasis and glycolysis of intrahepatic cholangiocarcinoma through miR-152-3p-mediated PDK1 expression. Histol Histopathol. (2023) 38:1129–43. doi: 10.14670/HH-18-544
23. Feng XQ, Nie SM, Huang JX, Li TL, Zhou JJ, Wang W, et al. Circular RNA circHIPK3 serves as a prognostic marker to promote chronic myeloid leukemia progression. Neoplasma. (2020) 67:171–7. doi: 10.4149/neo_2018_181129N908
24. Ba Y, Liu Y, Li C, Zhu Y, Xing W. HIPK3 Promotes Growth and Metastasis of Esophageal Squamous Cell Carcinoma via Regulation of miR-599/c-MYC Axis. Onco Targets Ther. (2020) 13:1967–78. doi: 10.2147/OTT.S217087
25. Gou Y, Ma J, Han S, Jin D, Chen M, Wang B, et al. CircHIPK3 affects Malignant phenotypes in esophageal squamous cell carcinoma by regulating p53-akt-mdm2 signaling pathways. Cancer Res Prev Treat. (2019) 46:11. doi: 10.3971/j.issn.1000-8578.2019.19.0386
26. Yao D, Lin S, Chen S, Wang Z. circHIPK3 regulates cell proliferation and migration by sponging microRNA-124 and regulating serine/threonine kinase 3 expression in esophageal squamous cell carcinoma. Bioengineered. (2022) 13:9767–80. doi: 10.1080/21655979.2022.2060776
27. Cao S-Q, Xue S-T, Li W-J, Hu G-S, Wu Z-G, Zheng J-C, et al. CircHIPK3 regulates fatty acid metabolism through miR-637/FASN axis to promote esophageal squamous cell carcinoma. Cell Death Discovery. (2024) 10:110. doi: 10.1038/s41420-024-01881-z
28. Kai D, Yannian L, Yitian C, Dinghao G, Xin Z, Wu J. Circular RNA HIPK3 promotes gallbladder cancer cell growth by sponging microRNA-124. Biochem Biophys Res Commun. (2018) 503:863–9. doi: 10.1016/j.bbrc.2018.06.088
29. Vidal AF, Ribeiro-dos-Santos AM, Vinasco-Sandoval T, Magalhães L, Pinto P, Anaissi AKM, et al. The comprehensive expression analysis of circular RNAs in gastric cancer and its association with field cancerization. Sci Rep. (2017) 7:14551. doi: 10.1038/s41598-017-15061-w
30. Reis-das-Mercês L, Vinasco-Sandoval T, Pompeu R, Ramos AC, Anaissi AKM, Demachki S, et al. CircRNAs as potential blood biomarkers and key elements in regulatory networks in gastric cancer. Int J Mol Sci. (2022) 23:650. doi: 10.3390/ijms23020650
31. Ghasemi S, Emadi-Baygi M, Nikpour P. Down-regulation of circular RNA ITCH and circHIPK3 in gastric cancer tissues. Turk J Med Sci. (2019) 49:687–95. doi: 10.3906/sag-1806-50
32. Liu W-G, Xu Q. Upregulation of circHIPK3 promotes the progression of gastric cancer via Wnt/β-catenin pathway and indicates a poor prognosis. Eur Ver Med Pharmacol Sci. (2019) 23:7905–12. doi: 10.26355/eurrev_201909_19004
33. Cheng J, Zhuo H, Xu M, Wang L, Xu H, Peng J, et al. Regulatory network of circRNA-miRNA-mRNA contributes to the histological classification and disease progression in gastric cancer. J Transl Med. (2018) 16:216. doi: 10.1186/s12967-018-1582-8
34. Jin Y, Che X, Qu X, Li X, Lu W, Wu J, et al. CircHIPK3 Promotes Metastasis of Gastric Cancer via miR-653-5p/miR-338-3p-NRP1 Axis Under a Long-Term Hypoxic Microenvironment. Front Oncol. (2020) 10:1612. doi: 10.3389/fonc.2020.01612
35. Li Q, Tian Y, Liang Y, Li C. CircHIPK3/miR-876-5p/PIK3R1 axis regulates regulation proliferation, migration, invasion, and glutaminolysis in gastric cancer cells. Cancer Cell Int. (2020) 20:391. doi: 10.1186/s12935-020-01455-w
36. Yang D, Hu Z, Zhang Y, Zhang X, Xu J, Fu H, et al. CircHIPK3 promotes the tumorigenesis and development of gastric cancer through miR-637/AKT1 pathway. Front Oncol. (2021) 11:637761. doi: 10.3389/fonc.2021.637761
37. Shang Z, Luo Z, Wang Y, Liu Q, Xin Y, Zhang M, et al. CircHIPK3 contributes to cisplatin resistance in gastric cancer by blocking autophagy-dependent ferroptosis. J Cell Physiol. (2023) 238:2407–24. doi: 10.1002/jcp.31093
38. Hu D, Zhang Y. Circular RNA HIPK3 promotes glioma progression by binding to miR-124-3p. Gene. (2019) 690:81–9. doi: 10.1016/j.gene.2018.11.073
39. Jin P, Huang Y, Zhu P, Zou Y, Shao T, Wang O. CircRNA circHIPK3 serves as a prognostic marker to promote glioma progression by regulating miR-654/IGF2BP3 signaling. Biochem Biophys Res Commun. (2018) 503:1570–4. doi: 10.1016/j.bbrc.2018.07.081
40. Liu Z, Guo S, Sun H, Bai Y, Song Z, Liu X. Circular RNA circHIPK3 elevates CCND2 expression and promotes cell proliferation and invasion through miR-124 in glioma. Front Genet. (2020) 11:1013. doi: 10.3389/fgene.2020.01013
41. Yin H, Cui X. Knockdown of circHIPK3 Facilitates Temozolomide Sensitivity in Glioma by Regulating Cellular Behaviors Through miR-524-5p/KIF2A-Mediated PI3K/AKT Pathway. Cancer Biother Radiopharm. (2021) 36:556–67. doi: 10.1089/cbr.2020.3575
42. Han C, Wang S, Wang H, Zhang J. Exosomal circ-HIPK3 Facilitates Tumor Progression and Temozolomide Resistance by Regulating miR-421/ZIC5 Axis in Glioma. Cancer Biother Radiopharm. (2021) 36:537–48. doi: 10.1089/cbr.2019.3492
43. Chen G, Shi Y, Liu M, Sun J. circHIPK3 regulates cell proliferation and migration by sponging miR-124 and regulating AQP3 expression in Hapatocellular carcinoma. Cell Death Dis. (2018) 9:175. doi: 10.1038/s41419-017-0204-3
44. Li W, Xue H, Li Y, Li P, Ma F, Liu M, et al. HIPK3 circular RNA promotes metastases of HCC through sponging miR-338-3p to induce ZEB2 expression. Dig Dis Sci. (2021) 66:3439–47. doi: 10.1007/s10620-020-06688-3
45. Zhang H, Dai Q, Zheng L, Yuan X, Pan S, Deng J. Knockdown of circ_HIPK3 inhibits tumorigenesis of Hepatocellular carcinoma via the miR-582-3p/DLX2 axis. Biochem Biophys Res Commun. (2020) 533:501–9. doi: 10.1016/j.bbrc.2020.09.050
46. Hu H, Wang Y, Qin Z, Sun W, Chen Y, Wang J, et al. Regulation of MRP4 Expression by circHIPK3 via Sponging miR-124-3p/miR-4524-5p in Hepatocellular Carcinoma. Biomedicines. (2021) 9:497. doi: 10.3390/biomedicines9050497
47. Yu Q, Chen W, Li Y, He J, Wang Y, Yang S, et al. The novel circular RNA HIPK3 accelerates the proliferation and invasion of hepatocellular carcinoma cells by sponging the micro RNA-124 or micro RNA-506/pyruvate dehydrogenase kinase 2 axis. Bioengineered. (2022) 13:4717–29. doi: 10.1080/21655979.2022.2031398
48. Yu H, Chen Y, Jiang P. Circular RNA HIPK3 exerts oncogenic properties through suppression of miR-124 in lung cancer. Biochem Biophys Res Commun. (2018) 506:455–62. doi: 10.1016/j.bbrc.2018.10.087
49. Chen X, Mao R, Su W, Yang X, Geng Q, Guo C, et al. circHIPK3 modulates autophagy via MIR124-3p-STAT3-PRKAA/AMPKα signaling in STK11 mutant lung cancer. Autophagy. (2020) 16:659–71. doi: 10.1080/15548627.2019.1634945
50. Hong W, Zhang Y, Ding J, Yang Q, Xie H, Gao X. circHIPK3 Acts as Competing Endogenous RNA and Promotes Non-Small-Cell Lung Cancer Progression through the miR-107/BDNF Signaling Pathway. BioMed Res Int. (2020) 2020:6075902. doi: 10.1155/2020/6075902
51. Lu H, Han X, Ren J, Ren K, Li Z, Sun Z. Circular RNA HIPK3 induces cell proliferation and inhibits apoptosis in non-small cell lung cancer through sponging miR-149. Cancer Biol Ther. (2020) 21:113–21. doi: 10.1080/15384047.2019.1669995
52. Li L, Zhang Q, Lian K. Circular RNA circ_0000284 plays an oncogenic role in the progression of non-small cell lung cancer through the miR-377-3p-mediated PD-L1 promotion. Cancer Cell Int. (2020) 20:247. doi: 10.1186/s12935-020-01310-y
53. Zhu Y, Shen L, Xia Q, Tao H, Liu Z, Wang M, et al. Extracellular vesicle-derived circHIPK3: Novel diagnostic biomarker for lung câncer. Adv Med Sci. (2023) 68:426–32. doi: 10.1016/j.advms.2023.10.003
54. Zhu X, Sun J. CircHIPK3 regulates melanoma cell behaviors by binding with miR-215-5p to upregulate YY1. Mol Cell Probes. (2020) 53:101644. doi: 10.1016/j.mcp.2020.101644
55. Ke Z, Xie F, Zheng C, Chen D. CircHIPK3 promotes proliferation and invasion in nasopharyngeal carcinoma by abrogating miR-4288-induced ELF3 inhibition. J Cell Physiol. (2019) 234:1699–706. doi: 10.1002/jcp.27041
56. Wang J, Zhao SY, Ouyang SS, Huang ZK, Luo Q, Liao L. Circular RNA circHIPK3 acts as the sponge of microRNA-124 to promote human oral squamous cell carcinoma cells proliferation. Zhonghua Kou Qiang Yi Xue Za Zhi. (2018) 53:546–51. doi: 10.3760/cma.j.issn.1002-0098.2018.08.009
57. Bi L, Zhang C, Yao Y, He Z. Circ-HIPK3 regulates YAP1 expression by sponging miR-381-3p to promote oral squamous cell carcinoma development. J Biosci. (2021) 46:20. doi: 10.1007/s12038-021-00142-w
58. Jiang W, Zhang C, Zhang X, Sun L, Li J, Zuo J. CircRNA HIPK3 promotes the progression of oral squamous cell carcinoma through upregulation of the NUPR1/PI3K/AKT pathway by sponging miR-637. Ann Transl Med. (2021) 9:860. doi: 10.21037/atm-21-1908
59. Xiao-Long M, Kun-Peng Z, Chun-Lin Z. Circular RNA circ_HIPK3 is down-regulated and suppresses cell proliferation, migration and invasion in osteosarcoma. J Cancer. (2018) 9:1856–62. doi: 10.7150/jca.24619
60. Wen Y, Li B, He M, Teng S, Sun Y, Wang G. circHIPK3 promotes proliferation and migration and invasion via regulation of miR-637/HDAC4 signaling in osteosarcoma cells. Oncol Rep. (2021) 45:169–79. doi: 10.3892/or.2020.7833
61. Liu N, Zhang J, Zhang L-Y, Wang L. CircHIPK3 is upregulated and predicts a poor prognosis in epithelial ovarian cancer. Eur Rev Med Pharmacol Sci. (2018) 22:3713–8. doi: 10.26355/eurrev_201806_15250
62. Zhou H, Li J, Lai X, Wang K, Zhou W, Wang J. CircHIPK3 modulates VEGF through MiR-7 to affect ovarian cancer cell proliferation and apoptosis. J BUON. (2021) 26:691–7.
63. Teng F, Xu J, Zhang M, Liu S, Gu Y, Zhang M, et al. Comprehensive circular RNA expression profiles and the tumor-suppressive function of circHIPK3 in ovarian cancer. Int J Biochem Cell Biol. (2019) 112:8–17. doi: 10.1016/j.biocel.2019.04.011
64. Liu Y, Xia L, Dong L, Wang J, Xiao Q, Yu X, et al. CircHIPK3 promotes gemcitabine (GEM) resistance in pancreatic cancer cells by sponging miR-330-5p and targets RASSF1. Cancer Manag Res. (2020) 12:921–9. doi: 10.2147/CMAR.S239326
65. Zhang J, Li J, Xiong Y, Li R. Circ_0000284 upregulates RHPN2 to facilitate pancreatic cancer proliferation, metastasis, and angiogenesis through sponging miR-1179. J Biochem Mol Toxicol. (2023) 37:e23274. doi: 10.1002/jbt.23274
66. Cai C, Zhi Y, Wang K, Zhang P, Ji Z, Xie C, et al. CircHIPK3 overexpression accelerates the proliferation and invasion of prostate cancer cells through regulating miRNA-338-3p. Onco Targets Ther. (2019) 12:3363–72. doi: 10.2147/OTT.S196931
67. Chen D, Lu X, Yang F, Xing N. Circular RNA circHIPK3 promotes cell proliferation and invasion of prostate cancer by sponging miR-193a-3p and regulating MCL1 expression. Cancer Manag Res. (2019) 11:1415–23. doi: 10.2147/CMAR.S190669
68. Liu F, Fan Y, Ou L, Li T, Fan J, Duan L, et al. CircHIPK3 facilitates the G2/M transition in prostate cancer cells by sponging miR-338-3p. Onco Targets Ther. (2020) 13:4545–58. doi: 10.2147/OTT.S242482
69. Liu DC, Song LL, Li XZ, Liang Q, Zhang ZG, Han CH. Circular RNA circHIPK3 modulates prostate cancer progression via targeting miR-448/MTDH signaling. Clin Transl Oncol. (2021) 23:2497–506. doi: 10.1007/s12094-021-02650-5
70. Tang Y, Liu J, Li X, Wang W. Exosomal circRNA HIPK3 knockdown inhibited cell proliferation and metastasis in prostate cancer by regulating miR-212/BMI-1 pathway. J Biosci. (2021) 46:69. doi: 10.1007/s12038-021-00190-2
71. Li H, Heng B, Ouyang P, Xie X, Zhang T, Chen G, et al. Comprehensive profiling of circRNAs and the tumor suppressor function of circHIPK3 in clear cell renal carcinoma. J Mol Histol. (2020) 51:317–27. doi: 10.1007/s10735-020-09882-9
72. Lai J, Xin J, Fu C, Zhang W. CircHIPK3 promotes proliferation and metastasis and inhibits apoptosis of renal cancer cells by inhibiting MiR-485-3p. Cancer Cell Int. (2020) 20:248. doi: 10.1186/s12935-020-01319-3
73. Han B, Shaolong E, Luan L, Li N, Liu X. CircHIPK3 Promotes Clear Cell Renal Cell Carcinoma (ccRCC) Cells Proliferation and Metastasis via Altering of miR-508-3p/CXCL13 Signal. Onco Targets Ther. (2020) 13:6051–62. doi: 10.2147/OTT.S251436
74. Page MJ, McKenzie JE, Bossuyt PM, Boutron I, Hoffmann TC, Mulrow CD, et al. The PRISMA 2020 statement: an updated guideline for reporting systematic reviews. BMJ. (2021) 372:n71. doi: 10.1136/bmj.n71
75. Kern F, Aparicio-Puerta E, Li Y, Fehlmann T, Kehl T, Wagner V, et al. miRTargetLink 2.0—interactive miRNA target gene and target pathway networks. Nucleic Acids Res. (2021) 49:W409–16. doi: 10.1093/nar/gkab297
76. Huang H-Y, Lin Y-C-D, Cui S, Huang Y, Tang Y, Xu J, et al. miRTarBase update 2022: an informative resource for experimentally validated miRNA–target interactions. Nucleic Acids Res. (2022) 50:D222–30. doi: 10.1093/nar/gkab1079
77. Szklarczyk D, Kirsch R, Koutrouli M, Nastou K, Mehryary F, Hachilif R, et al. The STRING database in 2023: protein-protein association networks and functional enrichment analyses for any sequenced genome of interest. Nucleic Acids Res. (2023) 51:D638–46. doi: 10.1093/nar/gkac1000
78. Zhang P, Lehmann BD, Shyr Y, Guo Y. The utilization of formalin fixed-paraffin-embedded specimens in high throughput genomic studies. Int J Genomics. (2017) 2017:1926304. doi: 10.1155/2017/1926304
79. Pereira AL, Magalhães L, Moreira FC, Reis-das-Mercês L, Vidal AF, Ribeiro-Dos-Santos AM, et al. Epigenetic field cancerization in gastric cancer: microRNAs as promising biomarkers. J Cancer. (2019) 10:1560–9. doi: 10.7150/jca.27457
80. Pereira A, Moreira F, Vinasco-Sandoval T, Cunha A, Vidal A, Ribeiro-Dos-Santos AM, et al. miRNome reveals new insights into the molecular biology of field cancerization in gastric cancer. Front Genet. (2019) 10:592. doi: 10.3389/fgene.2019.00592
81. Payen VL, Mina E, Hée VFV, Porporato PE, Sonveaux P. Monocarboxylate transporters in cancer. Mol Metab. (2020) 33:48–66. doi: 10.1016/j.molmet.2019.07.006
82. Pridham KJ, Shah F, Hutchings KR, Sheng KL, Guo S, Liu M, et al. Connexin 43 confers chemoresistance through activating PI3K. Oncogenesis. (2022) 11:2. doi: 10.1038/s41389-022-00378-7
83. Duvivier L, Gerard L, Diaz A, Gillet J-P. Linking ABC transporters to the hallmarks of cancer. Trends Cancer. (2024) 10:124–34. doi: 10.1016/j.trecan.2023.09.013
84. Deng X, Qing Y, Horne D, Huang H, Chen J. The roles and implications of RNA m6A modification in cancer. Nat Rev Clin Oncol. (2023) 20:507–26. doi: 10.1038/s41571-023-00774-x
85. Ronchetti D, Todoerti K, Tuana G, Agnelli L, Mosca L, Lionetti M, et al. The expression pattern of small nucleolar and small Cajal body-specific RNAs characterizes distinct molecular subtypes of multiple myeloma. Blood Cancer J. (2012) 2:e96. doi: 10.1038/bcj.2012.41
86. Kodali S, Proietti L, Valcarcel G, López-Rubio AV, Pessina P, Eder T, et al. RNA sequestration in P-bodies sustains myeloid leukaemia. Nat Cell Biol. (2024) 26:1745–58. doi: 10.1038/s41556-024-01489-6
87. Hemmings AB, Restuccia FD. PI3K-PKB/akt pathway. Cold Spring Harb Perspect Biol. (2012) 4:a011189. doi: 10.1101/cshperspect.a011189
88. Wang C, Fu R, Wang Y, Wei J, Yu Y, Hu L, et al. miR-124-3p and miR-194-5p regulation of the PI3K/AKT pathway via ROR2 in medulloblastoma progression. Cancer Gene Ther. (2024) 31:941–54. doi: 10.1038/s41417-024-00762-y
89. Shen J, Liang C, Su X, Wang Q, Ke Y, Fang J, et al. Dysfunction and ceRNA network of the tumor suppressor miR-637 in cancer development and prognosis. biomark Res. (2022) 10:72. doi: 10.1186/s40364-022-00419-8
90. Moghbeli M. Molecular interactions of miR-338 during tumor progression and metastasis. Cell Mol Biol Lett. (2021) 26:13. doi: 10.1186/s11658-021-00257-w
91. Okholm TLH, Kamstrup AB, Nielsen MM, Hollensen AK, Graversgaard ML, Kristensen LS, et al. circHIPK3 nucleates IGF2BP2 and functions as a competing endogenous RNA. eLife. (2024) 13:RP91783. doi: 10.7554/eLife.91783.4
92. Wang D-H, Ogino K, Sato Y, Sakano N, Kubo M, Takemoto K, et al. Urinary hydrogen peroxide as biomarker. In: Preedy V, Patel V, editors. General methods in biomarker research and their applications. Biomarkers in disease: methods, discoveries and applications. Springer, Dordrecht (2015). doi: 10.1016/S0009-8981(03)00236-5
93. Shimada K, Fujii T, Anai S, Fujimoto K, Konishi N. ROS generation via NOX4 and its utility in the cytological diagnosis of urothelial carcinoma of the urinary bladder. BMC Urol. (2011) 11:22. doi: 10.1186/1471-2490-11-22
94. Hempel N, Bartling TR, Mian B, Melendez JA. Acquisition of the metastatic phenotype is accompanied by H2O2-dependent activation of the p130Cas signaling complex. Mol Cancer Res. (2013) 11:303–12. doi: 10.1158/1541-7786.MCR-12-0478
95. Donkó A, Ruisanchez E, Orient A, Enyedi B, Kapui R, Péterfi Z, et al. Urothelial cells produce hydrogen peroxide through the activation of Duox1. Free Radic Biol Med. (2010) 49:2040–8. doi: 10.1016/j.freeradbiomed.2010.09.027
96. Liang J, Shen Y-C, Zhang X-Y, Chen C, Zhao H, Hu J. Circular RNA HIPK3 downregulation mediates hydrogen peroxide-induced cytotoxicity in human osteoblasts. Aging (Albany NY). (2020) 12:1159–70. doi: 10.18632/aging.102674
97. Fan M, Liang T, Xie F, Ma P, Li J. Exosomal circ_HIPK3 reduces apoptosis in H2O2-induced AC16 cardiomyocytes through miR-33a-5p/IRS1 axis. Transpl Immunol. (2023) 80:101862. doi: 10.1016/j.trim.2023.101862
98. Chen S, Wang M, Jian R, Li H, Liu G, Zhou C, et al. Circ_HIPK3 inhibits H2O2-induced lens epithelial cell injury in age-related cataract depending on the regulation of miR-495-3p/HDAC4 pathway. Biochem Genet. (2023) 61:565–77. doi: 10.1007/s10528-022-10266-9
99. Cai H, Jiang Z, Yang X, Lin J, Cai Q, Li X. Circular RNA HIPK3 contributes to hyperglycemia and insulin homeostasis by sponging miR-192-5p and upregulating transcription factor forkhead box O1. Endocr J. (2020) 67:397–408. doi: 10.1507/endocrj.EJ19-0271
100. Rezaeinejad F, Mirzaei A, Khalvati B, Sabz G, Alipoor B. Circulating expression levels of CircHIPK3 and CDR1as circular-RNAs in type 2 diabetes patients. Mol Biol Rep. (2022) 49:131–8. doi: 10.1007/s11033-021-06850-8
101. Wang J, Li X, Liu Y, Peng C, Zhu H, Tu G, et al. CircHIPK3 promotes pyroptosis in acinar cells through regulation of the miR-193a-5p/GSDMD axis. Front Med (Lausanne). (2020) 7:88. doi: 10.3389/fmed.2020.00088
102. Kirkegård J, Cronin-Fenton D, Heide-Jørgensen U, Mortensen FV. Acute pancreatitis and pancreatic cancer risk: A nationwide matched-cohort study in Denmark. Gastroenterology. (2018) 154:1729–36. doi: 10.1053/j.gastro.2018.02.011
103. Xiao L, Ma X-X, Luo J, Chung HK, Kwon MS, Yu T-X, et al. Circular RNA circHIPK3 promotes homeostasis of the intestinal epithelium by reducing microRNA 29b function. Gastroenterology. (2021) 161:1303–1317.e3. doi: 10.1053/j.gastro.2021.05.060
104. Villanacci V, Del Sordo R, Parigi TL, Leoncini G, Bassotti G. Inflammatory bowel diseases: does one histological score fit all? Diagnostics (Basel). (2023) 13:2112. doi: 10.3390/diagnostics13122112
105. Porter RJ, Arends MJ, Churchhouse AMD, Din S. Inflammatory bowel disease-associated colorectal cancer: translational risks from mechanisms to medicines. J Crohns Colitis. (2021) 15:2131–41. doi: 10.1093/ecco-jcc/jjab102
106. Santhanam S, Venkatraman A, Ramakrishna BS. Impairment of mitochondrial acetoacetyl CoA thiolase activity in the colonic mucosa of patients with ulcerative colitis. Gut. (2007) 56:1543–9. doi: 10.1136/gut.2006.108449
107. Alemany-Cosme E, Sáez-González E, Moret I, Mateos B, Iborra M, Nos P, et al. Oxidative stress in the pathogenesis of crohn’s disease and the interconnection with immunological response, microbiota, external environmental factors, and epigenetics. Antioxidants (Basel). (2021) 10:64. doi: 10.3390/antiox10010064
108. Xu Q, Cheng D, Li G, Liu Y, Li P, Sun W, et al. CircHIPK3 regulates pulmonary fibrosis by facilitating glycolysis in miR-30a-3p/FOXK2-dependent manner. Int J Biol Sci. (2021) 17:2294–307. doi: 10.7150/ijbs.57915
109. Zhang J-X, Lu J, Xie H, Wang D-P, Ni H-E, Zhu Y, et al. circHIPK3 regulates lung fibroblast-to-myofibroblast transition by functioning as a competing endogenous RNA. Cell Death Dis. (2019) 10:182. doi: 10.1038/s41419-019-1430-7
110. Jeganathan N, Cleland D, Sathananthan M. The association of lung cancer with pulmonary fibrosis. ERJ Open Res. (2022) 8:00505–2021. doi: 10.1183/23120541.00505-2021
111. Hong L, Ma X, Liu J, Luo Y, Lin J, Shen Y, et al. Circular RNA-HIPK3 regulates human pulmonary artery endothelial cells function and vessel growth by regulating microRNA-328-3p/STAT3 axis. Pulm Circ. (2021) 11:20458940211000234. doi: 10.1177/20458940211000234
112. Eul B, Cekay M, Pullamsetti SS, Tello K, Wilhelm J, Gattenlöhner S, et al. Noninvasive surrogate markers of pulmonary hypertension are associated with poor survival in patients with lung cancer. Am J Respir Crit Care Med. (2021) 204:1316–9. doi: 10.1164/rccm.202005-2023LE
113. Zhengbiao Z, Liang C, Zhi Z, Youmin P. Circular RNA_HIPK3-targeting miR-93-5p regulates KLF9 expression level to control acute kidney injury. Comput Math Methods Med. (2023) 2023:1318817. doi: 10.1155/2023/1318817
114. Han J, Li W, Zhang J, Guan Y, Huang Y, Li X. Mechanism of circHIPK3-miRNA-124-3p/miRNA-148b-3p-Mediated Inflammatory Responses and Cell Senescence in Candida albicans-Induced Septic Acute Kidney Injury. Gerontology. (2022) 68:1145–65. doi: 10.1159/000523910
115. Tang L, Zhang J, Han J, Zhang D, Zhang H, Liu J, et al. Molecular mechanism of circHIPK3 in mitochondrial function in septic acute kidney injury. Environ Toxicol. (2024) 39:2596–609. doi: 10.1002/tox.24127
116. Lu H, Chen Y, Wang X, Yang Y, Ding M, Qiu F. Circular RNA HIPK3 aggravates sepsis-induced acute kidney injury via modulating the microRNA-338/forkhead box A1 axis. Bioengineered. (2022) 13:4798–809. doi: 10.1080/21655979.2022.2032974
117. Wu Y, Luan J, Jiao C, Zhang S, Ma C, Zhang Y, et al. circHIPK3 exacerbates folic acid-induced renal tubulointerstitial fibrosis by sponging miR-30a. Front Physiol. (2022) 12:715567. doi: 10.3389/fphys.2021.715567
118. Zhuang L, Wang Z, Hu X, Yang Q, Pei X, Jin G. CircHIPK3 alleviates high glucose toxicity to human renal tubular epithelial HK-2 cells through regulation of miR-326/miR-487a-3p/SIRT1. Diabetes Metab Syndr Obes. (2021) 14:729–40. doi: 10.2147/DMSO.S289624
119. Liu F, Huang J, Zhang C, Xie Y, Cao Y, Tao L, et al. Regulation of podocyte injury by circHIPK3/FUS complex in diabetic kidney disease. Int J Biol Sci. (2022) 18:5624–40. doi: 10.7150/ijbs.75994
120. Peired AJ, Antonelli G, Angelotti ML, Allinovi M, Guzzi F, Sisti A, et al. Acute kidney injury promotes development of papillary renal cell adenoma and carcinoma from renal progenitor cells. Sci Transl Med. (2020) 12:eaaw6003. doi: 10.1126/scitranslmed.aaw6003
121. Wang D, Zhang P, Liu Z, Xing Y, Xiao Y. NXPH4 promotes gemcitabine resistance in bladder cancer by enhancing reactive oxygen species and glycolysis activation through modulating NDUFA4L2. Cancers (Basel). (2022) 14:3782. doi: 10.3390/cancers14153782
122. Deng H, Lv L, Li Y, Zhang C, Meng F, Pu Y, et al. miR-193a-3p regulates the multi-drug resistance of bladder cancer by targeting the LOXL4 gene and the oxidative stress pathway. Mol Cancer. (2014) 13:234. doi: 10.1186/1476-4598-13-234
123. Bai P, Fan T, Sun G, Wang X, Zhao L, Zhong R. The dual role of DNA repair protein MGMT in cancer prevention and treatment. DNA Repair (Amst). (2023) 123:103449. doi: 10.1016/j.dnarep.2023.103449
124. Shi Y, Wang Y, Qian J, Yan X, Han Y, Yao N, et al. MGMT expression affects the gemcitabine resistance of pancreatic cancer cells. Life Sci. (2020) 259:118148. doi: 10.1016/j.lfs.2020.118148
125. Zhang H, Li Q, Guo X, Wu H, Hu C, Liu G, et al. MGMT activated by Wnt pathway promotes cisplatin tolerance through inducing slow-cycling cells and nonhomologous end joining in colorectal cancer. J Pharm Anal. (2024) 14:100950. doi: 10.1016/j.jpha.2024.02.004
126. Chen X, Zhang M, Gan H, Wang H, Lee J-H, Fang D, et al. A novel enhancer regulates MGMT expression and promotes temozolomide resistance in glioblastoma. Nat Commun. (2018) 9:2949. doi: 10.1038/s41467-018-05373-4
127. Fan C-H, Liu W-L, Cao H, Wen C, Chen L, Jiang G. O6-methylguanine DNA methyltransferase as a promising target for the treatment of temozolomide-resistant gliomas. Cell Death Dis. (2013) 4:e876. doi: 10.1038/cddis.2013.388
128. Bobustuc GC, Kassam AB, Rovin RA, Jeudy S, Smith JS, Isley B, et al. MGMT inhibition in ER positive breast cancer leads to CDC2, TOP2A, AURKB, CDC20, KIF20A, Cyclin A2, Cyclin B2, Cyclin D1, ERα and Survivin inhibition and enhances response to temozolomide. Oncotarget. (2018) 9:29727–42. doi: 10.18632/oncotarget.25696
129. Tsai M-S, Kuo Y-H, Chiu Y-F, Su Y-C, Lin Y-W. Down-regulation of Rad51 expression overcomes drug resistance to gemcitabine in human non-small-cell lung cancer cells. J Pharmacol Exp Ther. (2010) 335:830–40. doi: 10.1124/jpet.110.173146
130. Gao X, Liu J, Fan D, Li X, Fang Z, Yan K, et al. Berberine enhances gemcitabine-induced cytotoxicity in bladder cancer by downregulating Rad51 expression through inactivating the PI3K/Akt pathway. Oncol Rep. (2022) 47:33. doi: 10.3892/or.2021.8244
131. Zhao W, Liu J, Wu J, Ma X, Wang X, Zhang L, et al. High-throughput microarray reveals the epitranscriptome-wide landscape of m6A-modified circRNA in oral squamous cell carcinoma. BMC Genomics. (2022) 23:611. doi: 10.1186/s12864-022-08806-z
132. Li P, Richard HT, Zhu K, Li L, Huang S. The roles and regulation of m6A modification in glioblastoma stem cells and tumorigenesis. Biomedicines. (2022) 10:969. doi: 10.3390/biomedicines10050969
133. Hsu K-F, Shen M-R, Huang Y-F, Cheng Y-M, Lin S-H, Chow N-H, et al. Overexpression of the RNA-binding proteins Lin28B and IGF2BP3 (IMP3) is associated with chemoresistance and poor disease outcome in ovarian cancer. Br J Cancer. (2015) 113:414–24. doi: 10.1038/bjc.2015.254
134. Kendzia S, Franke S, Kröhler T, Golob-Schwarzl N, Schweiger C, Toeglhofer AM, et al. A combined computational and functional approach identifies IGF2BP2 as a driver of chemoresistance in a wide array of pre-clinical models of colorectal cancer. Mol Cancer. (2023) 22:89. doi: 10.1186/s12943-023-01787-x
135. Ding F, Lu L, Wu C, Pan X, Liu B, Zhang Y, et al. circHIPK3 prevents cardiac senescence by acting as a scaffold to recruit ubiquitin ligase to degrade HuR. Theranostics. (2022) 12:7550–66. doi: 10.7150/thno.77630
136. Ren L, Jiang Q, Mo L, Tan L, Dong Q, Meng L, et al. Mechanisms of circular RNA degradation. Commun Biol. (2022) 5:1355. doi: 10.1038/s42003-022-04262-3
137. Khan FA, Fang N, Zhang W, Ji S. The multifaceted role of Fragile X-Related Protein 1 (FXR1) in cellular processes: an updated review on cancer and clinical applications. Cell Death Dis. (2024) 15:72. doi: 10.1038/s41419-023-06413-8
138. Grelloni C, Garraffo R, Setti A, Rossi F, Peruzzi G, Cinquanta M, et al. BRCA1 levels and DNA-damage response are controlled by the competitive binding of circHIPK3 or FMRP to the BRCA1 mRNA. Mol Cell. (2024) 84(21):4079–94.e10. doi: 10.1016/j.molcel.2024.09.016
139. Zhang Y, Guo H, Zhang H. SNHG10/DDX54/PBX3 feedback loop contributes to gastric cancer cell growth. Dig Dis Sci. (2021) 66:1875–84. doi: 10.1007/s10620-020-06488-9
140. Yao W, Hou J, Liu G, Wu F, Yan Q, Guo L, et al. LncRNA CBR3-AS1 promotes osteosarcoma progression through the network of miR-140-5p/DDX54-NUCKS1-mTOR signaling pathway. Mol Ther Oncolytics. (2022) 25:189–200. doi: 10.1016/j.omto.2022.03.001
141. Wei W, Liu K, Huang X, Tian S, Wang H, Zhang C, et al. EIF4A3-mediated biogenesis of circSTX6 promotes bladder cancer metastasis and cisplatin resistance. J Exp Clin Cancer Res. (2024) 43:2. doi: 10.1186/s13046-023-02932-6
Keywords: circular RNA, CircHIPK3, RBP, microRNA, bladder cancer, chemoresistance
Citation: Campelo MM, Reis-das-Mercês L, Vidal AF, da Silva FRP, de Oliveira ACA, Monteiro JRdS, Cabral CG, Noronha RCR and Pereira AL (2025) The dual role of circHIPK3 in cancer and its implications for multiple drugs resistance: a systematic review and computational approach. Front. Oncol. 15:1547889. doi: 10.3389/fonc.2025.1547889
Received: 18 December 2024; Accepted: 31 January 2025;
Published: 21 February 2025.
Edited by:
Tao Liu, University of New South Wales, AustraliaCopyright © 2025 Campelo, Reis-das-Mercês, Vidal, da Silva, de Oliveira, Monteiro, Cabral, Noronha and Pereira. This is an open-access article distributed under the terms of the Creative Commons Attribution License (CC BY). The use, distribution or reproduction in other forums is permitted, provided the original author(s) and the copyright owner(s) are credited and that the original publication in this journal is cited, in accordance with accepted academic practice. No use, distribution or reproduction is permitted which does not comply with these terms.
*Correspondence: Adenilson Leão Pereira, YWRlbmlsc29ubHBAdWZwYS5icg==
†These authors have contributed equally to this work
Disclaimer: All claims expressed in this article are solely those of the authors and do not necessarily represent those of their affiliated organizations, or those of the publisher, the editors and the reviewers. Any product that may be evaluated in this article or claim that may be made by its manufacturer is not guaranteed or endorsed by the publisher.
Research integrity at Frontiers
Learn more about the work of our research integrity team to safeguard the quality of each article we publish.