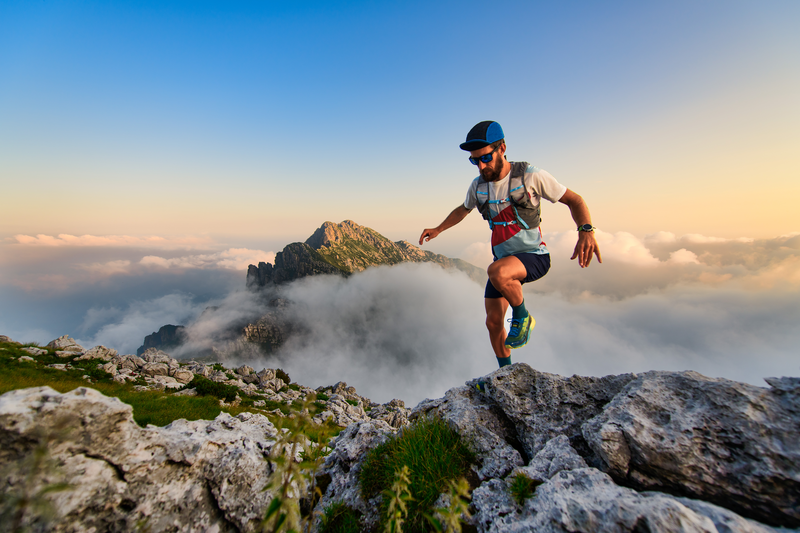
94% of researchers rate our articles as excellent or good
Learn more about the work of our research integrity team to safeguard the quality of each article we publish.
Find out more
ORIGINAL RESEARCH article
Front. Oncol.
Sec. Cancer Cell Signaling
Volume 15 - 2025 | doi: 10.3389/fonc.2025.1538649
The final, formatted version of the article will be published soon.
You have multiple emails registered with Frontiers:
Please enter your email address:
If you already have an account, please login
You don't have a Frontiers account ? You can register here
Introduction: Verify whether Onconstatin M receptor (OSMR) plays a regulatory role in the growth of glioblastoma (GBM) and explore its specific regulatory mechanism. Methods: In vitro investigations were carried out using OSMR knockdown and treatment with JAK agonist Butyzamide (JAKa). Evaluate cell proliferation rate through CCK-8; Colony formation experiment to detect cell proliferation; Transwell experiment evaluates cell invasion; Cell scratch assay to detect cell migration; WB detects the expression levels of pathway related proteins JAK, p-JAK, STAT3, p-STAT3, and CCL-2; Flow cytometry analysis of apoptosis rate, cell cycle arrest rate, and proportion of M2 macrophages; RT-qPCR was implemented to identify the expression of M2 polarization factors CD206, CD163 and IL-10 in macrophages. In the in vivo experiment, SF188 cells were subcutaneously injected into mice's right sides and divided into two groups: those with knocked down OSMR or those without. The knocked down OSMR group was divided into subgroups treated with DMSO containing or not containing JAKa. Subsequently, the tumor volume and weight of the mice were measured. RT-qPCR was utilized to assess the level of M2 polarization-related components in tumor tissues, while flow cytometry was employed to determine the M2 polarization ratio of macrophages in tumor tissues. Results: Knocking down OSMR dramatically reduces tumor cell proliferation, invasion, and migration, accelerates cell death and cell cycle arrest, and lowers JAK and STAT3 phosphorylation as well as CCL-2 expression levels, all while decreasing the fraction of M2 macrophages. Furthermore, knocking down OSMR drastically lowered tumor development and M2 polarization levels of monocytes in tumor tissue. JAKa reversed the inhibitory effect of OSMR knockdown on GBM malignant development and macrophage M2 polarization in both in vitro and in vivo studies. Conclusion: OSMR promotes the JAK/STAT3 signaling pathway, which promotes malignant glioblastoma growth and macrophages M2 polarization.
Keywords: OSMR, Glioblastoma, JAK/STAT3 signaling pathway, macrophage, M2 polarization
Received: 07 Jan 2025; Accepted: 25 Feb 2025.
Copyright: © 2025 Xiao, Tan, Liu, Zhou, Chen, Wang and Wang. This is an open-access article distributed under the terms of the Creative Commons Attribution License (CC BY). The use, distribution or reproduction in other forums is permitted, provided the original author(s) or licensor are credited and that the original publication in this journal is cited, in accordance with accepted academic practice. No use, distribution or reproduction is permitted which does not comply with these terms.
* Correspondence:
Bing Wang, The Second Affiliated Hospital, Hengyang Medical School, University of South China, Hengyang, Hunan Province, China
Disclaimer: All claims expressed in this article are solely those of the authors and do not necessarily represent those of their affiliated organizations, or those of the publisher, the editors and the reviewers. Any product that may be evaluated in this article or claim that may be made by its manufacturer is not guaranteed or endorsed by the publisher.
Research integrity at Frontiers
Learn more about the work of our research integrity team to safeguard the quality of each article we publish.