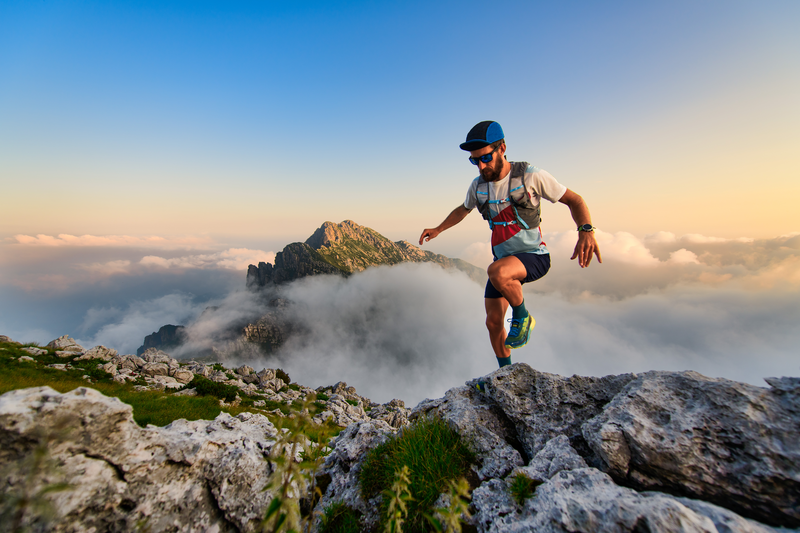
94% of researchers rate our articles as excellent or good
Learn more about the work of our research integrity team to safeguard the quality of each article we publish.
Find out more
REVIEW article
Front. Oncol.
Sec. Head and Neck Cancer
Volume 15 - 2025 | doi: 10.3389/fonc.2025.1529225
The final, formatted version of the article will be published soon.
You have multiple emails registered with Frontiers:
Please enter your email address:
If you already have an account, please login
You don't have a Frontiers account ? You can register here
Head and neck tumors represent a prevalent category of oral and maxillofacial malignancies, posing significant therapeutic and prognostic challenges due to their complex anatomical structure, tumor heterogeneity, and resistance to conventional therapies. Recent studies have highlighted the strong association between tumor progression and neoangiogenesis, with the angiopoietin (ANG) family playing a central role in this process. Comprising ANG1, ANG2, ANG3, and ANG4, these factors regulate multiple signaling pathways that promote cellular growth, differentiation, and proliferation, thereby driving angiogenesis and accelerating tumor growth and metastasis. Therefore, a comprehensive investigation of the ANG family’s role in head and neck tumors may offer critical insights into tumorigenesis mechanisms and unveil novel therapeutic targets. Such research has the potential to improve treatment outcomes and enhance the quality of life for patients.
Keywords: head and neck tumors, Angiopoietins (Ang-1 and Ang-2), TieReceptors, tumor invasion, Anti-Angiogenic Therapy (AAT)
Received: 19 Nov 2024; Accepted: 21 Feb 2025.
Copyright: © 2025 Zhong, Fei, Zhao, Chen, Gao, Huang and Fei. This is an open-access article distributed under the terms of the Creative Commons Attribution License (CC BY). The use, distribution or reproduction in other forums is permitted, provided the original author(s) or licensor are credited and that the original publication in this journal is cited, in accordance with accepted academic practice. No use, distribution or reproduction is permitted which does not comply with these terms.
* Correspondence:
Yi Huang, Sichuan Academy of Medical Sciences and Sichuan Provincial People's Hospital, Chengdu, China
Wei Fei, Sichuan Academy of Medical Sciences and Sichuan Provincial People's Hospital, Chengdu, China
Disclaimer: All claims expressed in this article are solely those of the authors and do not necessarily represent those of their affiliated organizations, or those of the publisher, the editors and the reviewers. Any product that may be evaluated in this article or claim that may be made by its manufacturer is not guaranteed or endorsed by the publisher.
Research integrity at Frontiers
Learn more about the work of our research integrity team to safeguard the quality of each article we publish.