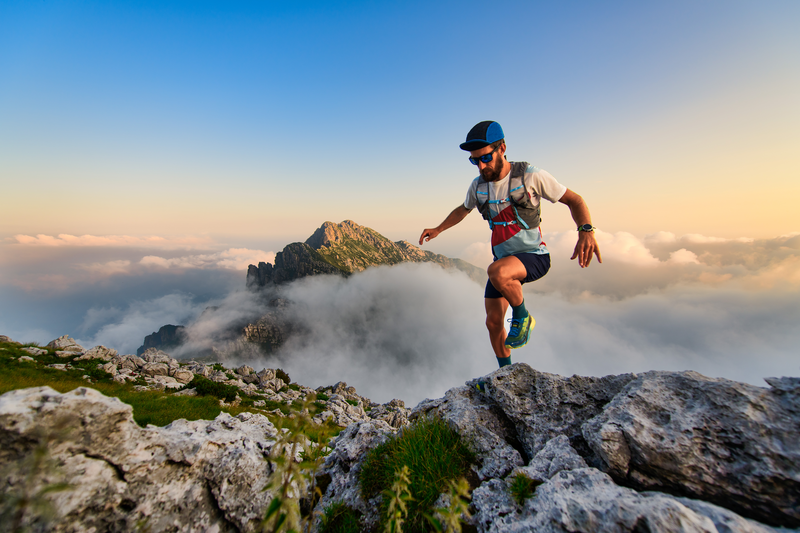
95% of researchers rate our articles as excellent or good
Learn more about the work of our research integrity team to safeguard the quality of each article we publish.
Find out more
REVIEW article
Front. Oncol. , 14 March 2025
Sec. Cancer Metabolism
Volume 15 - 2025 | https://doi.org/10.3389/fonc.2025.1524887
This article is part of the Research Topic Understanding the Impact of Microbes on Tumor Progression and Prevention: Unveiling New Avenues for Cancer therapy View all 4 articles
As cancer research advances, the intricate relationship between the microbiome and cancer is gaining heightened recognition, especially concerning tumor metastasis, where bacterial involvement becomes increasingly complex. This review seeks to systematically examine the dual roles of bacteria in the tumor metastasis process, encompassing both mechanisms that facilitate metastasis and the inhibitory effects exerted by specific microorganisms. We explore the mechanisms through which bacteria influence tumor cell migration by inducing chronic inflammation, evading host immune responses, and remodeling the ECM. Moreover, the immunomodulatory potential of probiotics and genetically engineered bacteria offers promising prospects for the prevention and treatment of tumor metastasis. This article elucidates the complexity and emerging frontiers of bacterial involvement in tumor metastasis by examining the clinical significance of bacteria as potential biomarkers and evaluating the effects of antibiotic usage on the metastatic process. We posit that comprehending the biological characteristics and clinical significance of bacteria, as a critical component of the tumor microenvironment, will offer innovative strategies and theoretical foundations for cancer treatment. Furthermore, this article explores future research directions, including the application of microbiome technologies and bacteria-based therapeutic strategies, thereby offering a valuable perspective for the development of novel anti-cancer approaches.
Cancer metastasis constitutes a principal cause of cancer-related mortality, characterized by the invasion of adjacent tissues by cancer cells originating from the primary tumor site, followed by their dissemination through the circulatory or lymphatic systems to distant organs (1). Although extensive research has elucidated the molecular mechanisms and cellular behaviors implicated in tumor metastasis, the microenvironmental factors influencing this process remain inadequately understood and warrant further investigation (1, 2). Recent research on the relationship between the microbiome and cancer has progressively revealed novel aspects of tumor biology, with particular emphasis on the role of bacteria in tumor metastasis, which has garnered significant attention (3). Bacteria constitute a crucial component of the tumor microenvironment; these microorganisms are not only present in the intestinal tract but are also widely distributed across the microbial communities of the oral cavity, cervix, and other organs (4).
Bacteria exert significant influence on the host immune system through diverse mechanisms and are integral to the initiation, progression, and metastasis of tumors. Research has identified associations between genetic predispositions within the gut microbiome and the incidence of various cancers, including breast, lung, and oral cancers (5). Dysbiosis, or imbalances in gut microbiota, can precipitate chronic inflammation, a recognized risk factor for several cancers, notably colorectal cancer (CRC) (6, 7). Certain bacteria, such as clostridium nucleatum, can directly impact the tumor microenvironment by modulating immune cell infiltration and activity (8). Tumor-associated bacteria have the potential to foster a pre-tumorigenic immune milieu, thereby facilitating tumor progression and metastasis (9). Conversely, other microorganisms, such as those within the Lachnospiraceae family, may exert inhibitory effects by eliciting anti-tumor immune responses (10).
A comprehensive investigation into the role of bacteria in tumor metastasis is expected to elucidate the intricate mechanisms underlying metastatic processes and potentially offer novel insights for clinical interventions. Notably, specific bacterial species have been identified as significantly associated with particular tumor types, exemplified by the correlation between Helicobacter pylori and gastric cancer (11), as well as the dysregulation of the intestinal microbiome in CRC (12, 13). More significantly, the composition and diversity of bacterial populations may play a crucial role in influencing the metastatic potential of tumors, thereby presenting novel opportunities for the development of bacterial-based biomarkers (14). Furthermore, bacteria and their metabolites may serve as promising targets for immunotherapy, potentially enhancing the efficacy of existing anti-tumor treatments. Nevertheless, our current understanding of the dual role of bacteria in tumor metastasis remains limited.
This review aims to examine the mechanisms by which bacteria promote or inhibit tumor metastasis, assess their clinical significance, and suggest directions for future research. We will investigate the influence of bacteria on the metastatic process through their roles in inducing inflammation, modifying immune responses, and reshaping the ECM. Additionally, we will explore the potential of bacteria as biomarkers and novel therapeutic strategies. Through this comprehensive analysis, we intend to offer new insights into the study of tumor metastasis, thereby paving the way for the development of innovative approaches in this field.
Research indicates that bacterial infections can facilitate the initiation and progression of tumors through multiple mechanisms. For instance, bacteria can induce a chronic inflammatory response, resulting in the persistent activation of the immune system. This not only promotes tumor cell proliferation but may also enhance metastasis by modifying the tumor microenvironment (15). Specifically, bacterial infections lead to the infiltration of immune cells into the tumor microenvironment, particularly the accumulation of macrophages and neutrophils, which exhibit a dual role in tumor progression by both inhibiting tumor growth and promoting metastasis (16). Certain studies have demonstrated that chronic lung infections caused by Pseudomonas aeruginosa can recruit neutrophils with highly expression of major histocompatibility complex class II, thereby altering the pulmonary microenvironment and facilitating lung metastasis of breast cancer (BC) (17). Additionally, bacterial infections may activate host inflammatory signaling pathways by releasing bacterial products, such as endotoxins, which promote tumor cell migration and invasion (18, 19). It has been observed that enterotoxigenic Bacteroides fragilis colonization in breast ducts may induce tumor cell growth and metastasis through the β-catenin and Notch1 pathways (20).
Certain bacterial species have the capacity to enhance the proliferation of regulatory T cells through the production of immunosuppressive factors, thereby attenuating the anti-tumor immune response (21). Notably, Campylobacter is markedly enriched in metastatic lesions of primary CRC, and its cytolethal distending toxin has been implicated in promoting CRC metastasis via the JAK2-STAT3-MMP9 signaling pathway in murine models of hepatic or pulmonary metastasis (22). Empirical evidence underscores the pivotal role of the gut microbiota in modulating host immune responses, with specific beneficial bacteria, such as Akkermansia muciniphila and Bifidobacterium longum, being identified as enhancers of anti-tumor immunity (23, 24). In the context of colon cancer, dysregulation of the intestinal microbiota occurs, wherein bacterial inflammatory factors can facilitate tumorigenesis and metastasis by activating NF-κB and other pro-inflammatory signaling pathways (25). Furthermore, certain tumor cells, including melanoma, can exploit metabolites produced by intestinal bacteria to modulate their immune evasion mechanisms, thereby augmenting their survival (26). In addition, bacteria have the capability to influence immune cell function through the production of extracellular vesicles, which may transport tumor antigens or immunosuppressive factors, thereby facilitating immune evasion (27, 28). Research has demonstrated that Fusobacterium nucleatum can enhance cancer growth and metastasis by releasing toll-like receptor 4 (TLR4), which interacts with BC cells via extracellular vesicles (29). Conversely, Lactobacillus casei and Lactobacillus reuteri have been shown to inhibit the proliferation and migration of pancreatic cancer cells by suppressing TLR4. These bacteria also impede the induction of M2 macrophages by pancreatic cancer cells and promote the differentiation of M1 macrophages (30).
Bacteria are integral to the establishment of niches at sites of tumor metastasis. Research has demonstrated the presence of viable bacteria within murine breast tumors, which preemptively migrate to the lungs to facilitate the metastasis of BC cells. Notably, the removal of these bacteria does not impact the growth of the primary tumor but significantly reduces lung metastasis (31). Similarly, Escherichia coli residing in CRC disrupts the gut vascular barrier and metastasizes to the liver, thereby promoting the formation of pre-metastatic niches and facilitating the metastasis of CRC cells (32). These findings offer novel insights and potential therapeutic targets for the development of innovative anti-tumor metastasis strategies.
The ECM constitutes a critical element of the tumor microenvironment, serving not only as a structural scaffold for tumor cells but also modulating their proliferation and metastatic potential through interactions with these cells.
Bacteria possess the ability to degrade ECM components through the secretion of autocrine or induced enzymes, consequently modifying the physical and chemical properties of the tumor microenvironment. For example, Helicobacter pylori can stimulate the secretion of cellular matrix metalloproteinase (MMPs) to degrade collagen and other ECM components via MAPK signaling pathways, a mechanism that may facilitate tumor cell migration and enhance tumor aggressiveness (33). Moreover, the introduction of excessive Escherichia coli in antibiotic-treated murine models of CRC has been observed to upregulate cathepsin K expression in tumors, a factor associated with increased liver metastasis (34). Additionally, bacterial presence can trigger local inflammatory responses, thereby promoting ECM remodeling. Lourdes et al. provided a comprehensive analysis demonstrating that the infiltration of inflammatory cells induces the rearrangement and synthesis of ECM components in BC, thereby creating a microenvironment conducive to tumor growth (35). Furthermore, bacterial prostatitis is associated with ERG+ pre-cancerous changes, suggesting that bacterial infection may activate oncogenic driver genes in prostate tissue, a process that involves modifications to the ECM (36).
In recent years, bacteria-based cancer therapies targeting the ECM have demonstrated significant potential. One study revealed that engineered Salmonella typhimurium could degrade the ECM within tumors by secreting the HysA protein, thereby enhancing the delivery of chemotherapeutic agents (37). Another study introduced the engineered bacterium VNPNKase, which was designed to sustainably degrade the ECM, inactivate cancer-associated fibroblasts, and facilitate the recruitment of dendritic cells and T cells (38). Although the application of bacteria and ECM in tumor therapy remains in its nascent stages, initial investigations have highlighted the promise of this approach. Future research is expected to advance the field by exploring more effective treatment modalities and underlying mechanisms. It is crucial to emphasize that ensuring the safety and efficacy of bacterial therapies, along with understanding their impact on the host immune system, is essential for the development of clinical applications.
The intricate interplay between bacteria-induced chronic inflammation and the ECM remodeling is characterized by a series of complex interactions. Chronic infection triggers the activation of the immune system, resulting in the release of inflammatory mediators and an upregulation of MMPs, which subsequently lead to the degradation and remodeling of ECM components. This remodeling process alters the tissue and tumor microenvironment, thereby facilitating tumor cell migration and invasion and elevating the risk of cancer metastasis (Figure 1, by figdraw.com, UWSSOc4e14). A comprehensive investigation of this mechanism is crucial not only for enhancing our understanding of the pathological processes underlying related diseases but also for potentially providing valuable insights for the development of innovative therapeutic strategies.
Research indicates that certain bacterial species can facilitate tumor metastasis, whereas others can inhibit this process by activating the host’s immune response, thereby enhancing the efficacy of cancer immunotherapy (Tables 1, 2). Notably, lactic acid bacteria have demonstrated significant potential in suppressing tumor metastasis (39). A study investigated the application of Veillonella parvula in treating BC in mice, revealing a reduction in mean tumor volume and liver metastases by 44.3% and 51.6%, respectively. This effect may be attributed to the decreased lactic acid levels in the tumor microenvironment (40). Additionally, milk fermented with Lactobacillus casei CRL431 was found to mitigate capecitabine-induced side effects and reduce tumor metastasis across various mouse models of BC (41). Furthermore, while Porphyromonas gingivalis has been shown to promote pancreatic intraepithelial neoplasia (PanIN), Lactobacillus species have been observed to impede the progression of these lesions and inhibit epithelial-mesenchymal transition (EMT) (42).
A study has demonstrated that Clostridium butyricum can mitigate MYC-mediated resistance to 5-fluorouracil and enhance the efficacy of anti-PD1 immunotherapy by facilitating the ubiquitination and subsequent degradation of MYC. This process ultimately inhibits the proliferation and metastasis of CRC (43). Additionally, research indicates that Clostridium butyrate downregulates the expression of METTL3 in CRC cells, leading to decreased levels of vimentin and VEGFR2. This downregulation consequently reduces EMT and angiogenesis, thereby inhibiting tumor metastasis in nude mice (44). Furthermore, Salmonella has been shown to impede tumor metastasis by downregulating the expression of epithelial cell adhesion molecules via the phosphorylated AKT/mTOR signaling pathway (45).
Mixed probiotics have been employed in research to impede tumor metastasis. In particular, a multi-species probiotic formulation was administered to treat CRC in rats, yielding results that demonstrated a significant reduction in tumor angiogenesis and inhibition of CRC liver metastasis (46). Furthermore, the administration of probiotic supplements in mice with melanoma has been shown to enhance the population of short-chain fatty acid-producing bacteria in the gut, elevate the levels of short-chain fatty acids (SCFAs) in the bloodstream, and promote the recruitment of helper T cell 17 by upregulating the expression of chemokine (C-C motif) ligand 20 (CCL20) in lung endothelial cells. These effects collectively contribute to a reduction in lung metastases (47).
Certain bacterial species exert an inhibitory influence within the tumor microenvironment by competing with tumor cells for essential nutrients and spatial niches. The metabolites produced by these bacteria may directly impede tumor cell proliferation and migration (48). For instance, a study demonstrated enhanced chemotherapy efficacy against advanced melanoma B16 metastasis through the aerosol inhalation of antibiotics (vancomycin/neomycin) or Bacillus rhamnosus, resulting in the inhibition of lung metastasis (49). Furthermore, research has indicated that SCFAs generated by intestinal bacteria, such as valerate and butyrate, can enhance tumor immune efficacy by modulating the immune response (50). Notably, butyrate, a prevalent SCFA, has been shown to inhibit tumor growth in murine models and potentially reverse immunosuppression induced by elevated levels of tumor-associated immune cells, including PD-L1 and IL-10 (51). These findings offer novel insights into the potential application of bacteria and their metabolites as anti-tumor therapeutic strategies.
Bacteria-based immunotherapy strategies have garnered significant attention in recent years owing to their promising potential in cancer treatment. Bacteria possess the capability to selectively localize at tumor sites and augment the anti-tumor response by activating the host immune system (52). Intravenous administration of Escherichia coli has been shown to colonize necrotic or hypoxic tumor regions. In a study conducted by Jiang et al., Escherichia coli was utilized as an engineered bacterial carrier in conjunction with radiotherapy, resulting in a significant inhibition of CRC growth and metastasis in murine models (53). The application of engineered bacteria presents considerable potential in the field of bacteria-based immunotherapy. Recent advancements in synthetic biology and nanotechnology have facilitated the development of bacteria with improved tumor specificity, enabling the targeted delivery of therapeutic proteins, cytokines, or drugs directly to tumor sites (54). Certain studies have involved the engineering of Escherichia coli to express human CXCL16, which has been employed in the treatment of various tumor models in mice. These engineered bacteria have the capacity to release chemokines within the tumor microenvironment, thereby attracting an influx of immune cells to the tumor site and augmenting the anti-tumor immune response (55). Bacterial membranes have been extensively investigated as potent adjuvants for tumor vaccines, attributed to their inherent capacity to stimulate the immune system. Several studies have employed nanoparticles encapsulated within double-membrane vesicles derived from the Salmonella strain VNP20009 to treat malignant melanoma tumors in murine models, resulting in a marked enhancement of therapeutic efficacy and the promotion of anti-tumor immunity (56). The incorporation of cancer vaccines within bacterial outer membranes has been shown to effectively activate the murine immune system, facilitating the production of robust immune protection upon the presentation of tumor antigens (57).
The combined application of bacterial therapy and immunotherapy is also an emerging treatment strategy. In a clinical trial involving 20 patients with advanced melanoma who had not previously undergone treatment, a combination therapy of fecal microbiota transplantation (FMT) and PD-1 inhibitors was administered. The FMT treatment facilitated the reduction of harmful bacteria without inducing adverse events (58). Additionally, a study investigating the use of Lactobacillus paracasei and cytokine-induced killer cells in the treatment of CRC in nude mice demonstrated that Lactobacillus paracasei effectively inhibited tumor growth and liver metastasis, with the synergistic treatment yielding superior outcomes (59).
While bacteria-based immunotherapy and probiotic strategies hold significant promise for inhibiting tumor metastasis, several challenges remain, including variability in individual efficacy, as well as concerns regarding the safety and stability of probiotics. To address these issues, future research should prioritize the development of personalized treatment regimens, optimize the screening and application processes for probiotics, and conduct in-depth investigations into their mechanisms of action. Furthermore, integrating these approaches with other immunotherapy and precision medicine strategies may enhance therapeutic outcomes and improve the prognosis for patients with tumors (Figure 2, by figdraw.com, SSWPY088bd).
In recent years, increasing attention has been directed towards the relationship between bacteria and tumor metastasis. Bo et al. discovered that bacteria present in BC cells can accompany the metastasis and colonization of these cells. These bacteria are capable of modulating the actin cytoskeleton of host cells, thereby enhancing the survival of tumor cells in the circulatory system under conditions of fluid shear stress. Furthermore, the removal of tumor-associated microorganisms impedes metastasis, although it does not inhibit the growth of the primary tumor (31). In the tumor microbiome of BC patients, there is an increased infiltration of Bacillus, Pseudomonas, Brevibacterium, and Mycobacterium. Notably, a heightened abundance of Paenibacillus in these patients correlates with a reduced survival rate (60). Furthermore, the composition of the gut microbiota is considered a crucial factor influencing the response to immune checkpoint inhibitors (ICPI), offering novel insights for personalized cancer immunotherapy (61). In CRC research, it was observed that the predominant bacteria in male CRC patients were Bacteroides, Eubacterium, and Faecalibacterium, whereas in female CRC patients, Bacteroides, Subdoligranulum, and Eubacterium were most prevalent (62). A meta-analysis indicated a significant association between F. nucleatum and CRC metastasis (63), with F. nucleatum promoting CRC metastasis via the miR-1322/CCL20 axis and M2 macrophage polarization (64). Additional studies have identified that the dominant bacterial species in periodontitis, Porphyromonas gingivalis, can facilitate the onset and progression of pancreatic cancer in murine models (65). Concurrently, a clinical trial revealed that a low abundance of Eubacterium infirmum, Actinobaculum, and Selenomonas is linked to a poor response in oral squamous cell carcinoma (OSCC) patients undergoing neoadjuvant therapy (66). Variations in bacterial exosomes between healthy individuals and cancer patients may represent a novel biomarker, demonstrating significant diagnostic potential across a range of diseases. The molecular content of bacterial exosomes can provide insights into the host’s health status and may facilitate the early detection of diseases (67).
The aforementioned findings position bacterial profiling as a promising instrument for assessing metastasis risk. Utilizing high-throughput sequencing technology enables a comprehensive analysis of gut microbiota composition, thereby elucidating associations between specific bacterial profiles and tumor metastasis. This analytical approach not only facilitates personalized risk assessments for individual patients but also lays the theoretical groundwork for the development of targeted interventions. As technology continues to advance and improve, bacterial profiling is anticipated to become a standard methodology in clinical practice.
The administration of antibiotics notably influences the risk of metastasis during cancer treatment. While antibiotics can modify the gut microbiome by decreasing the abandance of pathogenic bacteria, they may concurrently disturb the equilibrium of beneficial microbial populations, potentially exerting adverse effects on tumor metastasis. An epidemiological investigation encompassing various tumors, such as non-small cell lung cancer and melanoma, revealed that patients receiving ICPI who were exposed to antibiotics exhibited reduced progression-free survival, overall survival, and response rates (68). Research indicates that the intestinal microbiota plays a role in the treatment of HER2-positive BC with trastuzumab, whereas the administration of antibiotics diminishes the efficacy of this treatment (69). In murine models of metastasis using LLC and B16-F10 cells, SPF mice treated with the antibiotic cocktail (ABX) exhibited increased lung metastasis. Conversely, the transplantation of bifidobacterium into germ-free mice markedly inhibited lung metastasis (70). The administration of ABX can significantly disrupt the balance of intestinal microbiota and exacerbate cancer metastasis in Lewis lung cancer mouse models (71). These findings underscore the critical importance of maintaining microbial ecological balance in cancer therapy and suggest that broad-spectrum antibiotics should be employed with caution in future clinical settings, particularly for patients at risk of tumor progression and metastasis.
A study has demonstrated that porphyromonas gingivalis enhances OSCC cell proliferation, promotes angiogenesis, induces epithelial-to-mesenchymal transition, and generates carcinogenic metabolites. In contrast, oral Streptococcus species, such as Streptococcus gordonii, counteract the pro-tumor epithelial cell phenotype induced by Porphyromonas gingivalis (72). Furthermore, other researchers have identified that Fusobacterium nucleatum utilizes the lectin FAP2 to colonize the surface of BC cells, thereby facilitating tumor growth and metastasis. The application of antibiotics and anti-FAP2 treatments has been shown to mitigate the tumor-promoting effects of F. nucleatum (8). These findings suggest that early targeted bacterial therapies may play a crucial role in preventing metastasis and tumor dissemination. Additionally, research has explored the use of non-absorbable antibiotics to decrease CRC proliferation and metastasis by reducing the population of bacteria responsible for producing deoxycholic acid (DCA) (73). Moreover, salicycin has been found to inhibit tumor growth and metastasis by reversing the immunosuppressive microenvironment (74).
The role of bacteria as biomarkers for tumor metastasis is garnering increasing attention. The presence of specific bacterial species has been linked to the risk of tumor metastasis, offering a novel perspective for early screening and personalized treatment approaches. Concurrently, the administration of antibiotics has the potential to alter the microbial community, which may, in turn, influence the metastatic process. These observations underscore the significant role of microbial ecology in tumor development and treatment. Consequently, future research should focus on elucidating the interactions between bacteria and tumors to optimize anti-tumor strategies and enhance treatment efficacy.
The advent of high-throughput sequencing technology has facilitated unprecedented advancements in microbiome research. This methodology allows for the rapid and efficient analysis of large-scale samples, thereby elucidating the diversity and composition of both gut and tumor microbiomes (75, 76). Through the application of whole genome sequencing, 16S rRNA gene amplification sequencing, and metagenomic studies, researchers have been able to investigate the associations between specific bacterial populations and tumor metastasis. By integrating these sophisticated sequencing technologies, a comprehensive understanding of the microbiome’s role can be achieved. For instance, research has demonstrated that gut microbiota can affect the effectiveness of cancer therapies and the broader tumor microenvironment, underscoring the significance of microbial diversity in cancer prognosis (77). Furthermore, the identification of specific bacterial taxa linked to various cancer types, including CRC and esophageal squamous cell carcinoma, underscores the potential of microbiome analysis as both a diagnostic tool and a therapeutic target (78, 79). Through the application of metagenomic methodologies, researchers are able to elucidate the functional capabilities of microbial communities, thereby offering insights into their roles in tumor biology and metastasis (80, 81).
This comprehensive approach not only enhances our understanding of cancer biology but also informs the development of microbiome-based therapeutic interventions for cancer. As the field of microbiome research progresses, the interactions between microbial communities and cancer may unveil novel therapeutic targets and strategies for managing tumor metastasis.
In recent years, bacterial genetic engineering has emerged as a significant advancement in cancer therapy. Utilizing synthetic biology, researchers have developed methodologies to engineer bacteria capable of identifying and eradicating tumor cells. A frequently employed engineered bacterium is Escherichia coli Nissle 1917. Certain researchers have modified this bacterium to function as a temperature-sensitive entity, which can be activated through focused ultrasound to facilitate sustained tumor treatment (82). Moreover, recent studies have successfully engineered Escherichia coli to convert ammonia within tumors into L-arginine, thereby augmenting the anti-tumor efficacy of ICPI (83). Concurrently, other investigations are examining the potential of bacteria as drug delivery vectors, which can facilitate the targeted release of anti-cancer agents within the tumor microenvironment, thereby enhancing the specificity and efficacy of these therapeutic agents (84). This cutting-edge research holds promise for introducing novel perspectives and methodologies in cancer treatment.
The integration of bacterial agents with tumor immunotherapy represents a significant area of research interest. Certain bacterial species have the capacity to activate the host immune system, thereby augmenting the effectiveness of immunotherapeutic interventions. For instance, specific bacterial taxa, such as Bifidobacterium pseudolongum, Lactobacillus johnsonii, and Olsenella species, have been demonstrated to enhance the efficacy of ICPIs, facilitating improved recognition and destruction of cancer cells by the immune system (85). Research has demonstrated that specific gut bacteria, including Bifidobacterium and Ackermannia, have the capacity to stimulate the immune system by promoting T cell activation and augmenting cytokine production, which are essential for immune signal transduction (23, 86, 87).
In summary, elucidating the relationship between bacteria and the immune system presents novel opportunities for advancing cancer immunotherapy. This suggests that modulating the microbiome could be a promising strategy to enhance treatment outcomes for cancer patients.
As bacteria-based therapeutic strategies advance towards clinical implementation, it is imperative to meticulously address ethical considerations and conduct comprehensive safety assessments. The utilization of genetically modified bacteria presents potential biosafety concerns, including possible effects on the environment and the human microbiome. Concurrently, ethical issues such as informed consent, privacy, and the equitable distribution of treatment warrant careful attention. In the development of novel therapeutic interventions, it is imperative to institute a robust ethical review framework to guarantee research transparency and safeguard patient interests. Furthermore, the implementation of thorough clinical trials and safety evaluations is essential to advance bacteria-based treatment strategies that minimize risk while ensuring the efficacy of new therapies and the safety of patients.
The intersection of microbiome research and tumor metastasis has emerged as a significant area of focus within cancer research. Empirical studies have demonstrated a close association between the bacterial composition of the tumor microenvironment and the risk of tumor metastasis. By analyzing the microbiome of patients, researchers aim to identify specific bacterial taxa as potential biomarkers, thereby facilitating early detection and personalized therapeutic strategies. Nonetheless, the practical application of these findings is hindered by challenges such as individual variability and the inherent complexity of the microbiome. These factors may impact the reproducibility and relevance of study outcomes, complicating their clinical translation. Furthermore, effectively integrating these research insights into clinical practice, alongside reconciling conventional treatment paradigms with emerging technologies, represents a critical challenge that must be addressed in future research endeavors.
Despite advancements in emerging technologies such as microbial engineering, which includes sequencing and bacterial innovations offering novel approaches to cancer treatment, their practical application continues to encounter numerous challenges. These challenges encompass the high costs associated with these technologies, the complexity of data processing, and the need for regulatory compliance. Moreover, ethical and safety evaluations are critical when implementing new therapies. For instance, while probiotic therapies have the potential to modulate the microbiome and enhance immune responses, ensuring their long-term safety for patients remains imperative. Consequently, the successful implementation of these technologies necessitates multidisciplinary collaboration, fostering comprehensive research in relevant fields, developing viable solutions, and undertaking rigorous clinical trials. This approach is essential to ensure that these innovative technologies can be effectively integrated into real-world settings, ultimately offering improved treatment options for cancer patients.
Bacteria exhibit a multifaceted and significant influence on the process of tumor metastasis, demonstrating a clear dual role. On one hand, certain bacterial species can facilitate the metastasis and invasion of tumor cells by enhancing inflammatory responses, modifying the tumor microenvironment, and modulating the immune response. Conversely, specific bacteria and their metabolites have the potential to inhibit tumor growth, indicating that the role of bacteria in tumor development is not exclusively detrimental. This dual effect offers a novel perspective, implying that bacteria and their associated mechanisms could emerge as innovative targets for therapeutic intervention.
In the future, the industry ought to intensify the comprehensive investigation of the biological characteristics of bacteria, with particular emphasis on elucidating the mechanisms underlying their role in the regulation of tumor metastasis. The exploration of bacteria-tumor cell interactions should be further integrated with high-throughput techniques and bioinformatics to effectively address the complexity and uncertainty inherent in current research. Furthermore, multidisciplinary collaboration is anticipated to facilitate significant advancements in tumor treatment through the development of novel therapeutic strategies, such as bacterial combined immunotherapy and bacteria-based genetic engineering therapy. Overall, the investigation of bacteria as a regulatory factor in tumor metastasis is projected to offer innovative insights and directions for more precise and effective cancer therapies in the future.
LL: Visualization, Writing – original draft. DZ: Visualization, Writing – review & editing.
The author(s) declare that financial support was received for the research and/or publication of this article. This study was supported by Medicine and Health Development Plan Project of Shandong Province (202408011108); Project supported by affiliated hospital (teaching hospital) research and development foundation of Shandong Second Medical University (2024FYM118); Key R&D Program Policy Guidance Project of Liaocheng City (2023YD39), awarded to D.Z.
The authors declare that the research was conducted in the absence of any commercial or financial relationships that could be construed as a potential conflict of interest.
The author(s) declare that no Generative AI was used in the creation of this manuscript.
All claims expressed in this article are solely those of the authors and do not necessarily represent those of their affiliated organizations, or those of the publisher, the editors and the reviewers. Any product that may be evaluated in this article, or claim that may be made by its manufacturer, is not guaranteed or endorsed by the publisher.
1. Fares J, Fares MY, Khachfe HH, Salhab HA, Fares Y. Molecular principles of metastasis: A hallmark of cancer revisited. Signal transduction targeted Ther. (2020) 5:28. doi: 10.1038/s41392-020-0134-x
2. Lyden D, Ghajar CM, Correia AL, Aguirre-Ghiso JA, Cai S, Rescigno M, et al. Metastasis. Cancer Cell. (2022) 40:787–91. doi: 10.1016/j.ccell.2022.07.010
3. Fu A, Yao B, Dong T, Cai S. Emerging roles of intratumor microbiota in cancer metastasis. Trends Cell Biol. (2023) 33:583–93. doi: 10.1016/j.tcb.2022.11.007
4. Nejman D, Livyatan I, Fuks G, Gavert N, Zwang Y, Geller LT, et al. The human tumor microbiome is composed of tumor type-specific intracellular bacteria. Science. (2020) 368:973–80. doi: 10.1126/science.aay9189
5. Gao XF, Zhang CG, Huang K, Zhao XL, Liu YQ, Wang ZK, et al. An oral microbiota-based deep neural network model for risk stratification and prognosis prediction in gastric cancer. J Oral Microbiol. (2025) 17:2451921. doi: 10.1080/20002297.2025.2451921
6. Yang Y, Li L, Xu C, Wang Y, Wang Z, Chen M, et al. Cross-talk between the gut microbiota and monocyte-like macrophages mediates an inflammatory response to promote colitis-associated tumourigenesis. Gut. (2020) 70:1495–506. doi: 10.1136/gutjnl-2020-320777
7. Song X, Gao H, Lin Y, Yao Y, Zhu S, Wang J, et al. Alterations in the microbiota drive interleukin-17c production from intestinal epithelial cells to promote tumorigenesis. Immunity. (2014) 40:140–52. doi: 10.1016/j.immuni.2013.11.018
8. Parhi L, Alon-Maimon T, Sol A, Nejman D, Shhadeh A, Fainsod-Levi T, et al. Breast cancer colonization by fusobacterium nucleatum accelerates tumor growth and metastatic progression. Nat Commun. (2020) 11:3259. doi: 10.1038/s41467-020-16967-2
9. Sheng D, Yue K, Li H, Zhao L, Zhao G, Jin C, et al. The interaction between intratumoral microbiome and immunity is related to the prognosis of ovarian cancer. Microbiol Spectr. (2023) 11:e0354922. doi: 10.1128/spectrum.03549-22
10. Zhang X, Yu D, Wu D, Gao X, Shao F, Zhao M, et al. Tissue-resident lachnospiraceae family bacteria protect against colorectal carcinogenesis by promoting tumor immune surveillance. Cell Host Microbe. (2023) 31:418–32.e8. doi: 10.1016/j.chom.2023.01.013
11. Chen YC, Malfertheiner P, Yu HT, Kuo CL, Chang YY, Meng FT, et al. Global prevalence of helicobacter pylori infection and incidence of gastric cancer between 1980 and 2022. Gastroenterology. (2024) 166:605–19. doi: 10.1053/j.gastro.2023.12.022
12. Bai X, Wei H, Liu W, Coker OO, Gou H, Liu C, et al. Cigarette smoke promotes colorectal cancer through modulation of gut microbiota and related metabolites. Gut. (2022) 71:2439–50. doi: 10.1136/gutjnl-2021-325021
13. Gou H, Su H, Liu D, Wong CC, Shang H, Fang Y, et al. Traditional medicine pien tze huang suppresses colorectal tumorigenesis through restoring gut microbiota and metabolites. Gastroenterology. (2023) 165:1404–19. doi: 10.1053/j.gastro.2023.08.052
14. Battaglia TW, Mimpen IL, Traets JJH, van Hoeck A, Zeverijn LJ, Geurts BS, et al. A pan-cancer analysis of the microbiome in metastatic cancer. Cell. (2024) 187:2324–35.e19. doi: 10.1016/j.cell.2024.03.021
15. Wen Y, Zhu Y, Zhang C, Yang X, Gao Y, Li M, et al. Chronic inflammation, cancer development and immunotherapy. Front Pharmacol. (2022) 13:1040163. doi: 10.3389/fphar.2022.1040163
16. Wen Y, Wang X, Meng W, Guo W, Duan C, Cao J, et al. Tnf-A-dependent lung inflammation upregulates pd-L1 in monocyte-derived macrophages to contribute to lung tumorigenesis. FASEB J. (2022) 36:e22595. doi: 10.1096/fj.202200434RR
17. Ma T, Tang Y, Wang T, Yang Y, Zhang Y, Wang R, et al. Chronic pulmonary bacterial infection facilitates breast cancer lung metastasis by recruiting tumor-promoting Mhcii(Hi) neutrophils. Signal transduction targeted Ther. (2023) 8:296. doi: 10.1038/s41392-023-01542-0
18. Wang S, Kuang J, Zhang H, Chen W, Zheng X, Wang J, et al. Bile acid-microbiome interaction promotes gastric carcinogenesis. Adv Sci (Weinh). (2022) 9:e2200263. doi: 10.1002/advs.202200263
19. Wang J, Liu Z, Xu Y, Wang Y, Wang F, Zhang Q, et al. Enterobacterial lps-inducible linc00152 is regulated by histone lactylation and promotes cancer cells invasion and migration. Front Cell Infect Microbiol. (2022) 12:913815. doi: 10.3389/fcimb.2022.913815
20. Parida S, Wu S, Siddharth S, Wang G, Muniraj N, Nagalingam A, et al. A procarcinogenic colon microbe promotes breast tumorigenesis and metastatic progression and concomitantly activates notch and B-catenin axes. Cancer Discovery. (2021) 11:1138–57. doi: 10.1158/2159-8290.cd-20-0537
21. Du X, Jie Z, Zou Q. Microbiota alert: proteobacteria consume arginine to dampen omental antitumor immunity. Cell Host Microbe. (2024) 32:1045–7. doi: 10.1016/j.chom.2024.05.020
22. He Z, Yu J, Gong J, Wu J, Zong X, Luo Z, et al. Campylobacter jejuni-derived cytolethal distending toxin promotes colorectal cancer metastasis. Cell Host Microbe. (2024) 32:2080–91.e6. doi: 10.1016/j.chom.2024.11.006
23. Jiang Y, Xu Y, Zheng C, Ye L, Jiang P, Malik S, et al. Acetyltransferase from akkermansia muciniphila blunts colorectal tumourigenesis by reprogramming tumour microenvironment. Gut. (2023) 72:1308–18. doi: 10.1136/gutjnl-2022-327853
24. Shang F, Jiang X, Wang H, Guo S, Kang S, Xu B, et al. Bifidobacterium longum suppresses colorectal cancer through the modulation of intestinal microbes and immune function. Front Microbiol. (2024) 15:1327464. doi: 10.3389/fmicb.2024.1327464
25. Zhang Y, Zhang L, Zheng S, Li M, Xu C, Jia D, et al. Fusobacterium nucleatum promotes colorectal cancer cells adhesion to endothelial cells and facilitates extravasation and metastasis by inducing Alpk1/Nf-Kb/Icam1 axis. Gut Microbes. (2022) 14:2038852. doi: 10.1080/19490976.2022.2038852
26. Gopalakrishnan V, Spencer CN, Nezi L, Reuben A, Andrews MC, Karpinets TV, et al. Gut microbiome modulates response to anti-Pd-1 immunotherapy in melanoma patients. Science. (2018) 359:97–103. doi: 10.1126/science.aan4236
27. Chen G, Gao C, Jiang S, Cai Q, Li R, Sun Q, et al. Fusobacterium nucleatum outer membrane vesicles activate autophagy to promote oral cancer metastasis. J Adv Res. (2024) 56:167–79. doi: 10.1016/j.jare.2023.04.002
28. Choi HI, Choi JP, Seo J, Kim BJ, Rho M, Han JK, et al. Helicobacter pylori-derived extracellular vesicles increased in the gastric juices of gastric adenocarcinoma patients and induced inflammation mainly via specific targeting of gastric epithelial cells. Exp Mol Med. (2017) 49:e330. doi: 10.1038/emm.2017.47
29. Li G, Sun Y, Huang Y, Lian J, Wu S, Luo D, et al. Fusobacterium nucleatum-derived small extracellular vesicles facilitate tumor growth and metastasis via tlr4 in breast cancer. BMC Cancer. (2023) 23:473. doi: 10.1186/s12885-023-10844-z
30. Zhu Z, Yi B, Tang Z, Chen X, Li M, Xu T, et al. Lactobacillus casei combined with lactobacillus reuteri alleviate pancreatic cancer by inhibiting Tlr4 to promote macrophage M1 polarization and regulate gut microbial homeostasis. BMC Cancer. (2023) 23:1044. doi: 10.1186/s12885-023-11557-z
31. Fu A, Yao B, Dong T, Chen Y, Yao J, Liu Y, et al. Tumor-resident intracellular microbiota promotes metastatic colonization in breast cancer. Cell. (2022) 185:1356–72.e26. doi: 10.1016/j.cell.2022.02.027
32. Bertocchi A, Carloni S, Ravenda PS, Bertalot G, Spadoni I, Lo Cascio A, et al. Gut vascular barrier impairment leads to intestinal bacteria dissemination and colorectal cancer metastasis to liver. Cancer Cell. (2021) 39:708–24 e11. doi: 10.1016/j.ccell.2021.03.004
33. Karayiannis I, Martinez-Gonzalez B, Kontizas E, Kokkota AV, Petraki K, Mentis A, et al. Induction of mmp-3 and mmp-9 expression during helicobacter pylori infection via mapk signaling pathways. Helicobacter. (2023) 28:e12987. doi: 10.1111/hel.12987
34. Li R, Zhou R, Wang H, Li W, Pan M, Yao X, et al. Gut microbiota-stimulated cathepsin K secretion mediates Tlr4-dependent M2 macrophage polarization and promotes tumor metastasis in colorectal cancer. Cell Death differentiation. (2019) 26:2447–63. doi: 10.1038/s41418-019-0312-y
35. Herrera-Quintana L, Vázquez-Lorente H, Plaza-Diaz J. Breast cancer: extracellular matrix and microbiome interactions. Int J Mol Sci. (2024) 25(13):7226. doi: 10.3390/ijms25137226
36. Shrestha E, Coulter JB, Guzman W, Ozbek B, Hess MM, Mummert L, et al. Oncogenic gene fusions in nonneoplastic precursors as evidence that bacterial infection can initiate prostate cancer. Proc Natl Acad Sci U.S.A. (2021) 118(32):e2018976118. doi: 10.1073/pnas.2018976118
37. Kim JS, Park JE, Choi SH, Kang SW, Lee JH, Lee JS, et al. Ecm-targeting bacteria enhance chemotherapeutic drug efficacy by lowering ifp in tumor mouse models. J Control Release. (2023) 355:199–210. doi: 10.1016/j.jconrel.2023.02.001
38. Zhang Y, Liu Y, Li T, Yang X, Lang S, Pei P, et al. Engineered bacteria breach tumor physical barriers to enhance radio-immunotherapy. J Control Release. (2024) 373:867–78. doi: 10.1016/j.jconrel.2024.07.076
39. Badgeley A, Anwar H, Modi K, Murphy P, Lakshmikuttyamma A. Effect of probiotics and gut microbiota on anti-cancer drugs: mechanistic perspectives. Biochim Biophys Acta Rev Cancer. (2021) 1875:188494. doi: 10.1016/j.bbcan.2020.188494
40. Kefayat A, Bahrami M, Karami M, Rostami S, Ghahremani F. Veillonella parvula as an anaerobic lactate-fermenting bacterium for inhibition of tumor growth and metastasis through tumor-specific colonization and decrease of tumor’s lactate level. Sci Rep. (2024) 14:21008. doi: 10.1038/s41598-024-71140-9
41. Méndez Utz VE, Pérez Visñuk D, Perdigón G, de Moreno de LeBlanc A. Milk fermented by lactobacillus casei Crl431 administered as an immune adjuvant in models of breast cancer and metastasis under chemotherapy. Appl Microbiol Biotechnol. (2021) 105:327–40. doi: 10.1007/s00253-020-11007-x
42. Chen SM, Hsu LJ, Lee HL, Lin CP, Huang SW, Lai CJ, et al. Lactobacillus attenuate the progression of pancreatic cancer promoted by porphyromonas gingivalis in K-Ras(G12d) transgenic mice. Cancers. (2020) 12(12):3522. doi: 10.3390/cancers12123522
43. Xu H, Luo H, Zhang J, Li K, Lee MH. Therapeutic potential of clostridium butyricum anticancer effects in colorectal cancer. Gut Microbes. (2023) 15:2186114. doi: 10.1080/19490976.2023.2186114
44. Zhang K, Dong Y, Li M, Zhang W, Ding Y, Wang X, et al. Clostridium butyricum inhibits epithelial-mesenchymal transition of intestinal carcinogenesis through downregulating Mettl3. Cancer Sci. (2023) 114:3114–27. doi: 10.1111/cas.15839
45. Yen WC, Li QZ, Wu LH, Lee WY, Chang WW, Chien PJ, et al. Salmonella inhibits tumor metastasis by downregulating epithelial cell adhesion molecules through the protein kinase-B/mammalian target of rapamycin signaling pathway. Int J Biol macromolecules. (2023) 253:126913. doi: 10.1016/j.ijbiomac.2023.126913
46. Jakubauskas M, Jakubauskiene L, Leber B, Horvath A, Strupas K, Stiegler P, et al. Probiotic supplementation suppresses tumor growth in an experimental colorectal cancer liver metastasis model. Int J Mol Sci. (2022) 23(14):7674. doi: 10.3390/ijms23147674
47. Chen L, Zhou X, Wang Y, Wang D, Ke Y, Zeng X. Propionate and butyrate produced by gut microbiota after probiotic supplementation attenuate lung metastasis of melanoma cells in mice. Mol Nutr Food Res. (2021) 65:e2100096. doi: 10.1002/mnfr.202100096
48. Sukumar M, Roychoudhuri R, Restifo NP. Nutrient competition: A new axis of tumor immunosuppression. Cell. (2015) 162:1206–8. doi: 10.1016/j.cell.2015.08.064
49. Le Noci V, Guglielmetti S, Arioli S, Camisaschi C, Bianchi F, Sommariva M, et al. Modulation of pulmonary microbiota by antibiotic or probiotic aerosol therapy: A strategy to promote immunosurveillance against lung metastases. Cell Rep. (2018) 24:3528–38. doi: 10.1016/j.celrep.2018.08.090
50. Luu M, Riester Z, Baldrich A, Reichardt N, Yuille S, Busetti A, et al. Microbial short-chain fatty acids modulate Cd8(+) T cell responses and improve adoptive immunotherapy for cancer. Nat Commun. (2021) 12:4077. doi: 10.1038/s41467-021-24331-1
51. Lee SY, Jhun J, Woo JS, Lee KH, Hwang SH, Moon J, et al. Gut microbiome-derived butyrate inhibits the immunosuppressive factors Pd-L1 and Il-10 in tumor-associated macrophages in gastric cancer. Gut Microbes. (2024) 16:2300846. doi: 10.1080/19490976.2023.2300846
52. Gurbatri CR, Arpaia N, Danino T. Engineering bacteria as interactive cancer therapies. Science. (2022) 378:858–64. doi: 10.1126/science.add9667
53. Jiang SN, Phan TX, Nam TK, Nguyen VH, Kim HS, Bom HS, et al. Inhibition of tumor growth and metastasis by a combination of escherichia coli-mediated cytolytic therapy and radiotherapy. Mol therapy: J Am Soc Gene Ther. (2010) 18:635–42. doi: 10.1038/mt.2009.295
54. Liu J, He C, Tan W, Zheng JH. Path to bacteriotherapy: from bacterial engineering to therapeutic perspectives. Life Sci. (2024) 352:122897. doi: 10.1016/j.lfs.2024.122897
55. Savage TM, Vincent RL, Rae SS, Huang LH, Ahn A, Pu K, et al. Chemokines expressed by engineered bacteria recruit and orchestrate antitumor immunity. Sci Adv. (2023) 9:eadc9436. doi: 10.1126/sciadv.adc9436
56. Chen W, Song Y, Bai S, He C, Guo Z, Zhu Y, et al. Cloaking mesoporous polydopamine with bacterial membrane vesicles to amplify local and systemic antitumor immunity. ACS nano. (2023) 17:7733–49. doi: 10.1021/acsnano.3c00363
57. Liu G, Ma N, Cheng K, Feng Q, Ma X, Yue Y, et al. Bacteria-derived nanovesicles enhance tumour vaccination by trained immunity. Nat nanotechnology. (2024) 19:387–98. doi: 10.1038/s41565-023-01553-6
58. Routy B, Lenehan JG, Miller WH Jr., Jamal R, Messaoudene M, Daisley BA, et al. Fecal microbiota transplantation plus anti-Pd-1 immunotherapy in advanced melanoma: A phase I trial. Nat Med. (2023) 29:2121–32. doi: 10.1038/s41591-023-02453-x
59. Chen HH, Luo CW, Chen YL, Chiang JY, Huang CR, Wang YT, et al. Probiotic-facilitated cytokine-induced killer cells suppress peritoneal carcinomatosis and liver metastasis in colorectal cancer cells. Int J Biol Sci. (2024) 20:6162–80. doi: 10.7150/ijbs.101051
60. D’Afonseca V, Muñoz EV, Leal AL, Soto P, Parra-Cid C. Implications of the microbiome and metabolic intermediaries produced by bacteria in breast cancer. Genet Mol Biol. (2024) 47Suppl 1:e20230316. doi: 10.1590/1678-4685-gmb-2023-0316
61. Dzutsev AK, Goldszmid RS. Towards enhancing the predictive value of the microbiota for cancer immunotherapy. Trends Cancer. (2024) 10:771–3. doi: 10.1016/j.trecan.2024.07.007
62. Yang X, Li P, Qu Z, Zhuang J, Wu Y, Wu W, et al. Gut bacteria and sex differences in colorectal cancer. J Med Microbiol. (2023) 72. doi: 10.1099/jmm.0.001706
63. Chen WD, Zhang X, Zhang YP, Yue CB, Wang YL, Pan HW, et al. Fusobacterium nucleatum is a risk factor for metastatic colorectal cancer. Curr Med Sci. (2022) 42:538–47. doi: 10.1007/s11596-022-2597-1
64. Xu C, Fan L, Lin Y, Shen W, Qi Y, Zhang Y, et al. Fusobacterium nucleatum promotes colorectal cancer metastasis through Mir-1322/Ccl20 axis and M2 polarization. Gut Microbes. (2021) 13:1980347. doi: 10.1080/19490976.2021.1980347
65. Saba E, Farhat M, Daoud A, Khashan A, Forkush E, Menahem NH, et al. Oral bacteria accelerate pancreatic cancer development in mice. Gut. (2024) 73:770–86. doi: 10.1136/gutjnl-2023-330941
66. Wang XX, Liu YT, Ren JG, Liu HM, Fu Q, Yang Y, et al. Salivary microbiome relates to neoadjuvant immunotherapy response in oscc. J Dent Res. (2024) 103:988–98. doi: 10.1177/00220345241262759
67. Su KY, Koh Kok JY, Chua YW, Ong SD, Ser HL, Pusparajah P, et al. Bacterial extracellular vesicles in biofluids as potential diagnostic biomarkers. Expert Rev Mol diagnostics. (2022) 22:1057–62. doi: 10.1080/14737159.2022.2166403
68. Pinato DJ, Gramenitskaya D, Altmann DM, Boyton RJ, Mullish BH, Marchesi JR, et al. Antibiotic therapy and outcome from immune-checkpoint inhibitors. J immunotherapy Cancer. (2019) 7:287. doi: 10.1186/s40425-019-0775-x
69. Di Modica M, Gargari G, Regondi V, Bonizzi A, Arioli S, Belmonte B, et al. Gut microbiota condition the therapeutic efficacy of trastuzumab in Her2-positive breast cancer. Cancer Res. (2021) 81:2195–206. doi: 10.1158/0008-5472.can-20-1659
70. Zhu Z, Huang J, Li X, Xing J, Chen Q, Liu R, et al. Gut microbiota regulate tumor metastasis via Circrna/Mirna networks. Gut Microbes. (2020) 12:1788891. doi: 10.1080/19490976.2020.1788891
71. Li J, Shi B, Ren X, Hu J, Li Y, He S, et al. Lung-intestinal axis, shuangshen granules attenuate lung metastasis by regulating the intestinal microbiota and related metabolites. Phytomedicine: Int J phytotherapy phytopharmacology. (2024) 132:155831. doi: 10.1016/j.phymed.2024.155831
72. Lamont RJ, Fitzsimonds ZR, Wang H, Gao S. Role of porphyromonas gingivalis in oral and orodigestive squamous cell carcinoma. Periodontol 2000. (2022) 89:154–65. doi: 10.1111/prd.12425
73. Deng J, Yuan W, Tan Q, Wei X, Ma J. Non-absorbable antibiotic treatment inhibits colorectal cancer liver metastasis by modulating deoxycholic acid metabolism by intestinal microbes. J Cancer. (2022) 13:764–74. doi: 10.7150/jca.63490
74. Antoszczak M. A medicinal chemistry perspective on salinomycin as a potent anticancer and anti-cscs agent. Eur J Med Chem. (2019) 164:366–77. doi: 10.1016/j.ejmech.2018.12.057
75. Yen S, Johnson JS. Metagenomics: A path to understanding the gut microbiome. Mamm Genome. (2021) 32:282–96. doi: 10.1007/s00335-021-09889-x
76. Vitorino R. Transforming clinical research: the power of high-throughput omics integration. Proteomes. (2024) 12(3):25. doi: 10.3390/proteomes12030025
77. Kim N, Ma J, Kim W, Kim J, Belenky P, Lee I. Genome-resolved metagenomics: A game changer for microbiome medicine. Exp Mol Med. (2024) 56(7):1501–12. doi: 10.1038/s12276-024-01262-7
78. Sasaki T, Matsumoto Y, Murakami K, Endo S, Toyozumi T, Otsuka R, et al. Gut microbiome can predict chemoradiotherapy efficacy in patients with esophageal squamous cell carcinoma. Esophagus: Off J Japan Esophageal Soc. (2023) 20:691–703. doi: 10.1007/s10388-023-01004-0
79. Jiang Z, Wang J, Qian X, Zhang Z, Wang S. Oral microbiota may predict the presence of esophageal squamous cell carcinoma. J Cancer Res Clin Oncol. (2023) 149:4731–9. doi: 10.1007/s00432-022-04393-4
80. Qin X, Bi L, Yang W, He Y, Gu Y, Yang Y, et al. Dysbiosis of the gut microbiome is associated with histopathology of lung cancer. Front Microbiol. (2022) 13:918823. doi: 10.3389/fmicb.2022.918823
81. Liu F, Li J, Guan Y, Lou Y, Chen H, Xu M, et al. Dysbiosis of the gut microbiome is associated with tumor biomarkers in lung cancer. Int J Biol Sci. (2019) 15:2381–92. doi: 10.7150/ijbs.35980
82. Abedi MH, Yao MS, Mittelstein DR, Bar-Zion A, Swift MB, Lee-Gosselin A, et al. Ultrasound-controllable engineered bacteria for cancer immunotherapy. Nat Commun. (2022) 13:1585. doi: 10.1038/s41467-022-29065-2
83. Canale FP, Basso C, Antonini G, Perotti M, Li N, Sokolovska A, et al. Metabolic modulation of tumours with engineered bacteria for immunotherapy. Nature. (2021) 598:662–6. doi: 10.1038/s41586-021-04003-2
84. Chen L, Zhao R, Shen J, Liu N, Zheng Z, Miao Y, et al. Antibacterial fusobacterium nucleatum-mimicking nanomedicine to selectively eliminate tumor-colonized bacteria and enhance immunotherapy against colorectal cancer. Advanced materials (Deerfield Beach Fla). (2023) 35:e2306281. doi: 10.1002/adma.202306281
85. Mager LF, Burkhard R, Pett N, Cooke NCA, Brown K, Ramay H, et al. Microbiome-derived inosine modulates response to checkpoint inhibitor immunotherapy. Science. (2020) 369:1481–9. doi: 10.1126/science.abc3421
86. Sivan A, Corrales L, Hubert N, Williams JB, Aquino-Michaels K, Earley ZM, et al. Commensal bifidobacterium promotes antitumor immunity and facilitates anti-Pd-L1 efficacy. Science. (2015) 350:1084–9. doi: 10.1126/science.aac4255
Keywords: tumor metastasis, bacteria, chronic inflammation, immune escape, ECM remodeling, immunotherapy, antibiotics
Citation: Lin L and Zhang D (2025) Unveiling the microbial influence: bacteria’s dual role in tumor metastasis. Front. Oncol. 15:1524887. doi: 10.3389/fonc.2025.1524887
Received: 11 November 2024; Accepted: 28 February 2025;
Published: 14 March 2025.
Edited by:
Ali Ata Moazzami, Swedish University of Agricultural Sciences, SwedenReviewed by:
Khalid Omer Alfarouk, Alfarouk Biomedical Research LLC, United StatesCopyright © 2025 Lin and Zhang. This is an open-access article distributed under the terms of the Creative Commons Attribution License (CC BY). The use, distribution or reproduction in other forums is permitted, provided the original author(s) and the copyright owner(s) are credited and that the original publication in this journal is cited, in accordance with accepted academic practice. No use, distribution or reproduction is permitted which does not comply with these terms.
*Correspondence: Dongyan Zhang, ZG9uZ3lhbl8xMTI2QDEyNi5jb20=
Disclaimer: All claims expressed in this article are solely those of the authors and do not necessarily represent those of their affiliated organizations, or those of the publisher, the editors and the reviewers. Any product that may be evaluated in this article or claim that may be made by its manufacturer is not guaranteed or endorsed by the publisher.
Research integrity at Frontiers
Learn more about the work of our research integrity team to safeguard the quality of each article we publish.