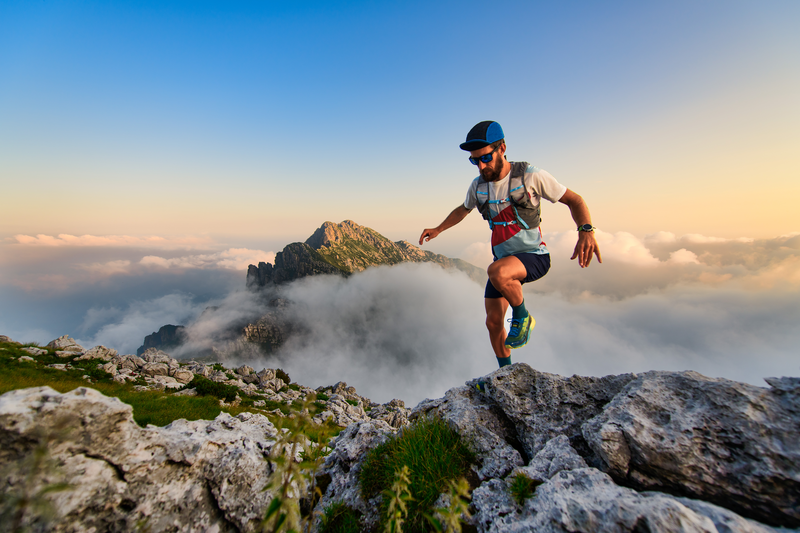
95% of researchers rate our articles as excellent or good
Learn more about the work of our research integrity team to safeguard the quality of each article we publish.
Find out more
REVIEW article
Front. Oncol. , 24 March 2025
Sec. Molecular and Cellular Oncology
Volume 15 - 2025 | https://doi.org/10.3389/fonc.2025.1513360
Rab Proteins, A Subfamily Of The Ras Superfamily Of Small Gtpases, Are Critical Regulators Of Intracellular Vesicular Trafficking, Which Is Intricately Linked To Various Cellular Processes. These Proteins Play Essential Roles Not Only In Maintaining Cellular Homeostasis But Also In Mediating The Complex Interplay Between Cancer Cells and Their Microenvironment. Rab Proteins Can Act As Either Oncogenic Factors Or Tumor Suppressors, With Their Functions Highly Dependent On The Cellular Context. Mechanistic Studies Have Revealed That Rab Proteins Are Involved In A Variety Of Processes, Including Vesicular Transport, Tumor Microenvironment Regulation, Autophagy, Drug Resistance, and Metabolic Regulation, and Play Either A Promotional Or Inhibitory Role In Cancer Development. Consequently, Targeting Rab Gtpases To Restore Dysregulated Vesicular Transport Systems May Offer A Promising Therapeutic Strategy To Inhibit Cancer Progression. However, It Is Equally Important To Consider The Potential Risks Of Disrupting Rab Functions, As Their Roles Are Highly Context-Dependent and May Have Opposing Effects In Different Malignancies. This Review Focuses On The Multifaceted Involvement Of Rab Family Proteins In Cancer Progression Underscores Their Importance As Potential Therapeutic Targets and Underscores The Need For A Deeper Understanding Of Their Complex Roles In Tumorigenesis.
RAB GTPases, the largest branch of the Ras protein superfamily, have regulatory factor functions in vesicular transport, endocytosis membrane transport, membrane targeting and fusion. In humans, more than 70 different RAB proteins are localized in different intracellular membranes (1). As master regulators of intracellular cargo transport, RAB proteins precisely control vesicle formation, movement, tethering, and fusion, thereby maintaining cellular homeostasis and signal transduction. In recent years, growing evidence has demonstrated that RAB proteins are not only essential for normal cellular physiology but also play complex and multifaceted roles in tumor initiation, progression, and metastasis.
Tumor development is a multifactorial and multistep process involving the dysregulation of various biological behaviors, including cell proliferation, apoptosis, metabolism, invasion, and migration. Studies have shown that the dysregulation in RABs level or the interaction between RABs and effectors may be associated with cancer (2). In some scenarios, RAB proteins may act as oncogenic factors, promoting tumor progression by enhancing invasiveness, metastatic potential, or drug resistance; in other contexts, they may function as tumor suppressors, limiting tumor growth by inhibiting proliferation or inducing apoptosis (3). RAB proteins influence tumor progression directly or indirectly by regulating vesicular transport, autophagy, metabolic reprogramming, and interactions with the tumor microenvironment (4).
This review aims to systematically summarize the dual roles of RAB proteins in tumor progression, with a focus on their molecular mechanisms in vesicular transport, tumor microenvironment modulation, autophagy, metabolic reprogramming, and drug resistance. By delving into the multifaceted functions of RAB proteins in cancer, we hope to provide new insights into their roles in tumorigenesis and progression, as well as a theoretical foundation for developing RAB protein-targeted therapeutic strategies.
RAB proteins primarily regulate vesicle budding, transport, tethering, and fusion through the transition between GTP- and GDP-bound states (5). Typically composed of approximately 200 amino acids, they feature highly conserved GTP/GDP-binding sites and GTPase activity domains. Structurally, RAB proteins consist of four conserved guanine nucleotide-binding domains and an effector domain. Evolutionarily, RAB proteins exhibit a high degree of conservation, with sequence homology ranging from 55% to 75% across different species (6).
RAB GTPases acts as molecular switches to regulate vesicular transport from the cell membrane as well as membrane fusion of target compartments through the regulation of GEFs and GAPs (7). By activating RAB proteins, GEFs may promote membrane transport processes in tumor cells, thereby affecting tumor invasion, metastasis, and drug resistance. GAP may have a dual role by inhibiting the activity of RAB proteins. On the one hand, it may exert an oncogenic effect by inhibiting the activity of tumor-associated RAB proteins; on the other hand, if the function of GAP is inhibited, it may lead to the aberrant activation of RAB proteins, which may promote tumor progression. GDI, by regulating the cycling of RAB proteins, may affect the metabolism and signaling of tumor cells (Figure 1).
Figure 1. RAB protein cycles between active and inactive and mediates vesicle transport via effector proteins.
The critical roles of GAP, GEF and GDI in the regulation of RAB proteins make them potential targets for cancer therapy. By inhibiting the activity of GEF, the activation of RAB proteins can be reduced, thereby inhibiting the membrane transport and invasive ability of tumor cells. The development of drugs that enhance the activity of GAP may help to inhibit the aberrant activation of RAB proteins, thereby exerting an anticancer effect. By regulating the function of GDI, the cycling and distribution of RAB proteins can be influenced, which in turn affects the metabolism and signaling of tumor cells.
The primary means of regulating the composition and arrangement of the cell surface is through vesicular trafficking, which encompasses endocytosis and endosomal circulation. RAB GTPases are part of a vast family of proteins that are highly conserved and play a crucial role in regulating vesicular trafficking (8).
The RAB GTPases can coordinate various types of responses by activating a variety of effectors at key stages of membrane traffic, such as the budding of vesicles, their delivery, tethering, docking, and their fusion (9, 10). RABs that are GTP-bound can recruit effector proteins to mediate the budding of vesicles in donor organelles, and tether them to acceptor compartments (11). As a result, RAB proteins coordinate spatially and temporally specific vesicle transport, as well as protein secretion and endocytosis. Thus, RAB proteins are known to play a crucial role in intracellular trafficking.
In tumorigenesis, RAB proteins are functionally impaired by mutations or post-translational modifications that disrupt vesicle trafficking, leading to dysregulated intracellular vesicular transport and contributing to tumorigenesis (2). The relevant RAB proteins are summarized in Table 1 and Figure 2. For example, RAB5 is beneficial to the early stages of endosome formation, and microtubule-dependent adhesion disassembly mediates RAB5 function in vesicle formation and early endosome formation (12). The overexpression of RAB5 promoted the capacity of invasion, migration and exosome secretion in cancer (13). The aberrant expression of RAB5 enhances tumor cell invasion by stimulating endocytosis or cytosolic recycling to promote the release of matrix met alloproteinases(MMP) (14). RAB5 can regulate EGFR vesicular recycling to promote migration in triple-negative breast cancer (TNBC) (15). High RAB2A expression has been found in human breast cancer, and RAB2A regulates most significantly post-endocytosis transport of membrane type 1 (MT1)-MMP and E-calmodulin-polarized Golgi transport by binding to VPS39, suggesting that RAB2A may be an independent predictor of disease recurrence in breast cancer patients (16).
Figure 2. The functions of RAB proteins in tumor development. RAB proteins participate in tumor genesis and development by regulating vesicle trafficking, tumor microenvironment, glucose metabolism, and autophagy.
The RAB7 protein is extensively studied as regulator of vesicular trafficking. RAB7 triggers pro-tumor or anti-tumor effects and exerts oncogenic and oncostatic functions during cancer progression (17). Studies have shown that inhibition of RAB7 expression enhances the pro-apoptotic effects of drugs on TNBC in triple-negative breast cancer, and targeting RAB7 impairs autophagic fluxes to inhibit melanoma growth; thus, targeting RAB7 may contribute to effective cancer therapy (18). RAB7 can facilitate troglitazone to prevent HGF-induced protease secretion, and prostate tumor growth and invasion (19). In the plasma membrane, RAB11 promotes endosome formation and fusion (20, 21). Alterations in RAB11 isoforms significantly affect cancer cell survival, progression and metastasis as well as accumulation of toxic substances (22). In cervical cancer, Rab11 affects tumor cell invasion and migration by regulating vesicle trafficking and the integrin αvβ3/FAK/PI3K pathway signaling pathway (23). As part of the transcytotic machinery,RAB17 docks apically and helps fuse apical vesicles (24). Researchers have found that RAB17 is recruited rapidly to efferosomes, and then migrates to the cell center, promoting cancer proliferation and migration (25). RAB17 can suppresses proliferation and migration of hepatocellular carcinoma cells and reduces the tumor growth via ERK signaling pathway (26). RAB25 can promote or block tumor growth. Integrins can be reinjected from late endosomes/lysosomes through RAB25, which facilitates cancer progression (27). In ovarian cancer, Rab25 enhances cancer cell invasiveness via the expression of the β1 integrin/EGFR/VEGF-A/Snail signaling axis and myostatin (28). In colorectal cancer, Rab25 suppressed the invasion of colon cancer cells by inactivating EGFR through up-regulation of claudin-7 expression (29). Rab34 mediates its vesicular transport within cells by interacting with integrin β3, which in turn regulates tumor cell adhesion and migration (30). RAB37 regulates vesicle trafficking from the Golgi network to the plasma membrane, so it is a regulator of vesicle trafficking and protein transportation (2). There is evidence that RAB37 regulates intracellular vesicle trafficking through GTP and reduces tumor growth/metastases and elicits an immunostimulatory TME (31). RAB39A is a RAB small GTPase located in different subcellular compartments that regulates the endocytic trafficking pathway. Rab39A inhibits cervical cancer cell migration, invasion and epithelial-to-mesenchymal transition EMT by suppressing AKT signaling (32).
The RAB protein plays a crucial role in the regulation of vesicular transport at multiple steps. There is a relationship between tumor progression and deregulation of RAB proteins and destruction of vesicle trafficking networks (5). Targeting RAB proteins can promote or inhibit the proliferation, migration ability of tumor cells by affecting the vesicular transport process. Therefore, the recovery of the dysfunctional vesicular transport system in cancer cells may provide a future direction for inhibiting tumor progression.
Extracellular vehicles (EVs) are a heterogeneous group of membrane-structured vesicles that are actively released by almost all types of cells. Small extracellular vesicles (sEVs) usually refers to EVs smaller than 200 nm in diameter and play crucial roles in tumor development, growth, and metastasis by interacting with the tumor microenvironment (33). The tumor microenvironment (TME) consists of many different cells that support or inhibit tumorigenesis and are connected to each other to promote disease progression. The tumor microenvironment can modulate antitumor immunity and contribute to heterogeneity in treatment response. Cancer cells can shape the microenvironment, and stromal components interact with tumor cells to support tumor growth and progression to help support tumorigenesis (34). The morphology and function of cancer cells in the tumor microenvironment are altered, and the diversity of cell types and metabolic patterns may be detrimental to treatment and lead to tumor recurrence.
The RAB proteins are involved in antigen presentation and play a critical role in tumor microenvironment interactions. RAB proteins can foster immunosuppressive TME by regulating the changes in cell interaction factors, thus promoting the progression of cancer. RAB effectors appear to promote tumor development or suppress tumorigenesis through oncogenic signaling pathways. The relevant RAB proteins are summarized in Table 1, Figure 2. Ectopic RAB5 is differentially expressed in various tumors, and the potential mechanisms include RAB5-regulated exosome secretion from cancer cells and stromal cells, affecting the communication between cancer cells, immune cells and the microenvironment, forming a tumor microenvironment conducive to the growth of tumor cells, and regulating cancer cell migration and invasion (13). RAB6B is a member of the RAB GTPases and has been found to be dysregulated in various tumors. RAB6B expression was found to be associated positively with immune cell infiltration in hepatocellular carcinoma (HCC), while RAB6B expression was associated with CD8+ T cell depletion, leading to an immunosuppressive microenvironment. It suggests that RAB6B may be involved in ECM remodeling in TME, which is associated with the formation of an immunosuppressive microenvironment in HCC. Targeting RAB13 can affect the relationship between cancer cells and tumor microenvironment, interfere with the establishment of stem cell niche in breast cancer, thereby providing therapeutic opportunities for targeted breast cancer treatment (35). RAB27A overexpression promotes the growth and metastasis of cancer in an exosome-dependent or independent manner. The correlation between the elevated levels of RAB27A and the presence of non-small cell lung cancer (NSCLC) and RAB27A might play a critical role in increasing chemosensitivity in NSCLC (36). Increased expression of RAB27B is linked to decreased survival rates among individuals with breast cancer, and this GTPase might impact the generation of angiogenic and prometabolic substances within the tumor microenvironment. In the tumor microenvironment, RAB27B may affect paracrine communication and play a significant role in cancer cell secretion (37). In addition, Rab27A and Rab27B are highly expressed in pancreatic cancer and are closely associated with tumor stage and vascular infiltration, and their mechanism of action may involve epithelial-mesenchymal transition (EMT) mediated by the MAPK/ERK signaling pathway, thereby promoting tumor invasion and metastasis (38, 39). The RAB35-regulated vesicle subpopulation facilitates communication between cancer cells and stromal cells, providing an environment conducive to tumor growth (40). RAB35 enhances the expression of integrin and promotes cell migration in cervical and breast cancer (41). The RAB37 protein acts as a tumor promoter in macrophages by mediating the exocytosis of IL-6 through the activation of PD-1 on CD8+ T cells, and its associated transport pathway can promote the formation of immunosuppressive TME (42).
RAB proteins transport a variety of cargoes and substrates to govern conventional and non-conventional vesicular secretion pathways thereby playing a role in remodeling TME. RAB GTPases are tightly controlled by cytokines in the microenvironment and enable aggressive cancer growth by delivering key factors to the tumor microenvironment. Therefore, there is a need to further understand how RAB regulates conventional vesicular transport during tumorigenesis and its role in tumor immunity and targeting RAB proteins could affect the relationship between cancer cells and the tumor microenvironment, thereby influencing tumor progression and providing therapeutic opportunities for targeted cancer therapies.
As a highly conservative process of eukaryotic cellular recycling, autophagy breaks down cytoplasmic organelles, proteins, and macromolecules and circulates their breakdown products. Autophagy plays a key role in the development and progression of cancer (43), participates in the regulation of the immune microenvironment during the initiation and progression of tumors, and can promote tumor cell death, thereby playing a tumor suppressive role, and therefore can be used as a potential target to improve cancer treatment (44, 45). Dysregulated autophagy has an impact on health and disease.
There are several RAB proteins that are involved in autophagosome formation, including RAB1, RAB2, RAB5, RAB7, RAB9A, RAB11, RAB23, RAB32, RAB10, and RAB39A (Table 1, Figure 2). RAB1 produces dependent autophagy, which selectively inhibits this process through the GAS pathway (46). RAB1 has important regulatory functions in the growth, migration and survival of cancers. RAB1 affects neuroblastoma progression through interaction with the autophagy receptor protein optineurin (OPTN), regulation of autophagy-related signaling pathways, and influencing metabolic adaptations in tumor cells (47). In mammalian cells, Studies have shown that RAB2 is involved in regulating autophagosome formation. RAB2 is also linked to autophagy pathways and the Golgi apparatus through transport membranes and participates in different autophagy mechanisms. Study reports that RAB2 is required for autophagosome clearance in human breast cancer cells (48). RAB2 gene amplification occurs in a wide variety of human cancers and may contribute to tumorigenesis (49). RAB5 forms complexes that contribute to the early stages of autophagy (50). RAB5 can influence cancer cell progression by regulating autophagosome formation and maturation, influencing cancer cell adaptation to metabolic stress, and serving as a biomarker of antibody-drug coupling (ADC) efficacy. RAB11 is an endosomal recycling protein that contributes to the formation and maturation of autophagosome. Researchers reported that RAB11 loss can prevent autophagosomes from fusing with late endosomes, hinder autophagy, and eventually lead to apoptosis and promote tumorigenesis (51). Autophagosome formation and maturation can be regulated by RAB10. RAB10 can bind to the autophagy receptor OPTN, promoting mitochondrial autophagy (52). Down-regulation of miRNA targets RAB10 to activate the AMPK signaling pathway and inhibit proliferation, inducing autophagy and apoptosis in human hepatocellular carcinoma cells (53). Circular RNAs (circRNAs) regulates apoptosis and autophagy in gastric cancer by affecting microRNA expression of RAB10 (54). RAB39A regulates cancer stemness and tumorigenesis in sarcoma models (55), probably by regulating autophagy and lysosomal fusion. RAB7, RAB8B and RAB24 play a crucial role in the maturation of autophagosome. By regulating lysosomal fusion, the RAB7 effector can promote the degradation of neuronal autophagy lysosome (56). RAB7 induces mitophagy and plays a pivotal tumor-suppressing role in gastric cancer (57). Reducing the expression of RAB7 inhibited the autophagy level of gastric cancer cells, suppressed the inhibitory effect in the proliferation, migration and invasion of gastric cancer cells, and promoted cell apoptosis (58). RAB23 is localized to autophagosomes and inhibits cell growth and proliferation and induces apoptosis in breast cancer cells (59). Furthermore, RAB22A was shown to facilitate anti-tumor immunity by mediating the formation of non-typical autophagosomes (60). Depletion of RAB escort protein 1 (REP1) blocks autophagosome formation, increases megacellular drinking, and modulates intracellular nutrient levels and mTOR activity. These suggest that REP1-mediated intracellular metabolism and degradation processes are key regulators of cancer cell survival and are expected to be potential targets for cancer therapy (61).
As key regulators of vesicular transport and molecular “switches” for vesicle trafficking, many RAB GTPases play a critical role in the autophagy process. Autophagy, a cellular degradation and recycling mechanism, has a dual role in cancer biology. On one hand, it acts as a protective mechanism by preventing early tumor development; on the other hand, it supports metabolic adaptation, maintenance, and survival of established and metastatic tumors (62). This dual nature of autophagy, both promoting and inhibiting cancer, highlights its significant contribution to tumor initiation and progression. Furthermore, autophagy is involved in shaping an immunosuppressive tumor microenvironment, making it a potential therapeutic target for cancer treatment. RAB proteins, through their regulation of autophagy and related vesicular transport processes, are emerging as crucial players in cancer development. For instance, specific RAB proteins modulate autophagosome formation, maturation, and fusion with lysosomes, thereby influencing autophagic flux. Dysregulation of these RAB-mediated processes can lead to either excessive or insufficient autophagy, both of which can drive tumor progression.
Drug resistance refers to the tolerance of microorganisms, parasites and tumor cells to the effects of chemotherapy drugs. Once drug resistance occurs, the chemotherapy effects of drugs will decrease significantly. At present, tumor drug resistance is the main problem that limits the efficacy of tumor chemotherapy drugs. Cancer cells are also resistant to immunotherapeutic drugs, which may lead to the rapid recurrence of cancer/disease progression and ultimately cause the death of patients.
For example, the miRNA expression can increase cisplatin resistance in lung cancer stem cells by inhibiting RAB6 (63). RAB6B and RAB6C are associated with drug sensitivity. The knockdown of RAB6B inhibits liver cancer cell proliferation and promotes apoptosis, thus improving drug sensitivity. Similarly, the overexpression of RAB6c induces the intracellular accumulation of several anticancer drugs. This may contribute to the development of drug resistance in breast cancer cells (64). Moreover, RAB7 can mediate resistance to cisplatin chemotherapy in extracellular vesicles, and RAB7 expression is downregulated in many cisplatin-resistant cell lines compared to sensitive cell lines (65). RAB7 affects drug chemotherapy resistance in cervical cancer cells by regulating advanced endocytosis pathways and extracellular vesicle secretion (17). RAB27A has also been related to resistance to some conventional chemotherapy drugs. A downregulation of RAB27A can increase chemotherapy sensitivity in lung cancer cells (36). Rab27B enhances chemoresistance of tumor cells by promoting exosome release in hepatocellular carcinoma (66). In addition, secretory RAB8 is another RAB involved in chemotherapeutic drug resistance. Cells resistant to platinum compounds generally express high levels of RAB8, and overexpression of RAB8 can enhance cisplatin resistance by increasing its secretion of cisplatin-resistant proteins, a potential target for cancer chemotherapy. RAB-like protein 1 A (RBEL1A) is up-regulated in breast tumor cells, promotes cell proliferation, cell cycle and invasion, induces cisplatin sensitivity, and RBEL1A induction after cisplatin treatment inhibits chemosensitivity (67).
Cancer cells undergo dramatic metabolic adaptations to meet increased bioenergetic demands, leading to resistance to treatment. Tumor cells can influence the proliferative, migratory, and apoptotic stages of a tumor by developing drug resistance. Several RAB proteins have been associated with resistance to anticancer drugs, and the cytosolic action of RAB proteins, whose dysregulation affects tumor cell resistance and alterations in the tumor microenvironment in tumorigenesis. Targeting RAB proteins further provides an opportunity for therapeutic strategies to reduce cancer progression and anticancer resistance, providing new targets for cancer therapy.
It has been shown that transport and metabolic signaling pathways intersect, meaning that vesicular transport can influence the regulation of metabolic signaling (68). RAB GTPases play an essential role in the regulation of vesicular transport and also influence and participate in cellular metabolic processes. RAB GTPases can regulate the transport of GLUT (glucose transporter) and the formation of lipid droplets (LD) as well as the processes of glucose metabolism and lipid metabolism in cancer cells.
For muscle contractions to occur, glucose is an important fuel, and glucose metabolism plays an important role in health. The glucose transporter (GLUT) is necessary for muscle and fat to utilize dietary glucose. We know that glucose transporter (GLUT), a transmembrane protein family that affects the cell’s ability to absorb extracellular glucose, is involved in glucose metabolism, inflammatory reaction, and immune response.
The primary glucose transporter GLUT4 transports glucose from intracellular vesicles to the cytoplasm (69). The RAB protein plays an important role in metabolic reprogramming, and studies have demonstrated that GLUT4 transport is mediated by RAB proteins. A RAB-GTPase is a critical regulator of GLUT4 transport, coordinating its translocation to the plasma membrane (70). Some RAB proteins participate in GLUT4 transport, such as RAB8A, RAB10, RAB20, RAB13 (Table 1, Figure 2). Overexpression of RAB8A inhibits insulin-induced GLUT4 transport processes in skelet al muscle cells by regulating exocytosis of GLUT4 vesicles (69). Reduction of RAB8A protein inhibits breast cancer proliferation, migration, and invasion, thereby decreasing the survival of cancer patients (71). The RAB10 GTPase plays an important role in glucose metabolism in cells, mainly related to GLUT4 exocytosis in fat cells (72). An insufficient expression of RAB20 in hepatoma cells leads to the release of EVs, and a decrease in triosephosphate isomerase 1 (TPI1) levels promotes aerobic glycolysis and promotes the development of liver cancer (73). Glucose transport and autophagy are important functions of RAB21, a small GTPase. RAB21 depletion affects the glucose uptake process, thereby increasing autophagy flux (74), while also inhibiting tumor progression in vivo, indicating RAB21 plays a role in carcinogenicity. In addition, RAB-GAP can act on RAB35 to regulate GLUT4 transport in insulin-stimulated adipocytes (75) and it plays a role in cancer invasion, metastasis and immune escape. A study showed that RAB7 depletion reduced mTOR overactivation, decreased glucose consumption and ROS overproduction, and increased the number of healthy mitochondria (76). Gamma-glutamyl transferase 7 suppresses gastric cancer by cooperating with RAB7 to induce mitophagy (57). Therefore, we hypothesized that RAB7 may play an important role in cancer progression through cellular metabolism. RAB32 supports mTORC1 signaling to regulate proliferation and metabolism in hepatocellular carcinoma by binding to lysosomes (68).
The RAB proteins are involved in GLUT4 transport, which controls glucose metabolism, allowing tumor cells to grow and proliferate (77). GLUT4 acts as an insulin-responsive glucose transporter from GLUT4 storage vesicles to the cell membrane. So, we speculate that GLUT4 transport is mediated by RAB proteins, affecting the development of glucose metabolism in tumors. We can target glycolytic pathways by modulating RAB proteins as a therapeutic approach to inhibit cancer progression. RAB protein is involved in tumor cell autophagy, tumor microenvironment, and glucose metabolism, and these processes are involved in the tumors (68).
The RAB family, the largest group within the RAS superfamily, plays a pivotal role in cancer progression by regulating vesicular trafficking, tumor microenvironment, autophagy, and glucose metabolism, thereby influencing metastasis, proliferation, and cell cycle control (Figures 2, 3). Unlike other RAS superfamily members, such as RAS, RHO, and ARF, which primarily mediate signal transduction and cytoskeletal dynamics, RAB proteins exert their unique functions through the modulation of vesicle transport. For instance, while RAS mutations often drive uncontrolled cell proliferation via pathways like MAPK signaling, RAB proteins impact tumor development by altering vesicular trafficking and secretion, which in turn affects the tumor microenvironment, cellular metabolism, and metastatic processes.
In recent years, the role of the RAB family in cancer has garnered increasing attention, with its critical function in membrane trafficking offering unique therapeutic targets, particularly in strategies aimed at altering cancer cell behavior by modulating intracellular transport. RAB proteins play a pivotal role in the tumor microenvironment by regulating vesicular trafficking and exosome secretion. Exosomes, containing DNA, RNA, proteins, lipids, and lipoproteins, are essential for intercellular communication, influencing gene expression and functionality in recipient cells. For instance, mRNA and miRNA can serve as tumor-specific biomarkers for diagnosis, while modulating the activity of specific RNAs may offer novel therapeutic approaches. Exosomal proteins, such as growth factors, cytokines, and enzymes, may promote tumor angiogenesis or immune evasion. Targeting these RNAs and proteins to develop specific diagnostic markers or small-molecule inhibitors could enhance early tumor detection, improve treatment precision, and provide deeper insights into RAB-mediated tumor progression. Furthermore, integrating multi-omics technologies to comprehensively analyze these components will help unravel the complex roles of RAB proteins in the tumor microenvironment.
RAB proteins are essential for normal cellular physiology, but their dysregulation in cancer can lead to detrimental outcomes, closely linked to tumor initiation, progression, and metastasis. Depending on the cellular context, RAB proteins can function as either oncogenes or tumor suppressors (Figure 3). This dual role makes the RAB family particularly intriguing for understanding the complex mechanisms of cancer progression, though it also complicates therapeutic strategies. For instance, RAB3B has been identified as a hub gene for diagnosis and prognosis prediction in various cancers, including lung adenocarcinoma, glioma, and colorectal cancer. Additionally, RAB22A has been shown to promote anti-tumor immunity by mediating the formation of atypical autophagosomes (60).
The functional diversity of RAB proteins across different cancers is closely tied to their intracellular mechanisms. For instance, RAB27A and RAB27B exhibit distinct roles in various cancers: in liver cancer, RAB27B enhances chemotherapy resistance by promoting exosome release, while in pancreatic cancer, their overexpression correlates with tumor stage and vascular invasion, demonstrating oncogenic properties. However, in certain digestive system tumors, they may act as tumor suppressors (39, 66). These functional differences may arise from variations in expression levels, interactions with other signaling pathways, and differences in the cellular microenvironment. Tissue specificity and cancer subtypes also influence RAB protein functions. For example, RAB25 acts as an oncogene in ovarian, breast, and gastric cancers but suppresses tumor growth in colon and head-neck cancers, likely due to its subcellular localization and roles in different tissues (78). Additionally, some RAB proteins exhibit dual functions depending on the cellular context and dynamic changes during tumor progression. For instance, RAB27A not only promotes metastasis in pancreatic cancer but also modulates the tumor immune microenvironment by regulating exosome secretion in immune cells (38). These complexities underscore the necessity of in-depth research into the mechanism-dependent roles of RAB proteins in cancer progression.
Despite the significant potential of the RAB family in cancer therapy, targeting RAB proteins presents unique challenges. Unlike the RAS family, which primarily targets mutant proteins, RAB proteins share high sequence and structural similarity, exhibit functional complexity, and lack distinct binding sites, making specific targeting difficult. Additionally, RAB protein activity is regulated by multiple upstream regulators and downstream effectors, meaning targeting a single RAB protein may yield limited therapeutic effects. Currently, the absence of well-defined small-molecule inhibitors for RAB proteins further restricts the development of targeted therapies. However, the critical role of RAB proteins in vesicular trafficking offers a promising avenue for cancer treatment, such as improving drug delivery or enhancing immune cell function by modulating vesicle transport.
Given the complexity of RAB proteins and the challenges in targeting them directly, focusing on their effector proteins or downstream signaling pathways may prove more effective. RAB proteins exert their functions through interactions with effectors, such as SAND-1, which mediates vesicle transport from late endosomes to lysosomes in the case of RAB7 (79). Moreover, downstream pathways of RAB proteins play significant roles in cancer; for example, RAB34 regulates tumor cell adhesion and migration by interacting with integrin β3 (30). Targeting these effectors or pathways could overcome the limitations of single RAB protein targeting, though their dual functionality in upstream and downstream contexts necessitates personalized treatment strategies tailored to specific tissues and cellular backgrounds. Combining RAB-targeted therapies with existing treatments, such as immunotherapy or chemotherapy, through synergistic approaches may enhance therapeutic outcomes. Additionally, leveraging CRISPR/Cas9 or RNA interference to selectively knock down oncogenic RAB proteins or their regulators, or developing highly selective small-molecule inhibitors, could be viable strategies, particularly in cancers with specific RAB protein overexpression. While targeting RAB proteins represents a novel therapeutic approach, their potential dual roles require careful evaluation. Addressing these challenges could provide valuable insights for cancer research and treatment.
YJ: Conceptualization, Methodology, Writing – original draft. RL: Writing – original draft, Conceptualization. GT: Writing – original draft, Investigation. WW: Writing – review & editing, Resources. CC: Writing – review & editing, Resources. QY: Resources, Writing – review & editing.
The author(s) declare that financial support was received for the research and/or publication of this article. This study was supported by the University Synergy Innovation Program of Anhui Province (GXXT-2022-064), excellent Scientific Research and Innovation Team of Anhui Universities (2024AH010021), the Major Program of Anhui Educational Committee (No.: KJ2019ZD28), the Program for graduate’s research of Bengbu Medical College (Byycx22042, Byycx23013).
We would like to thank Institute of Health and Medicine, Hefei Comprehensive National Science for help providing writing assistance.
The authors declare that the research was conducted in the absence of any commercial or financial relationships that could be construed as a potential conflict of interest.
The author(s) declare that no Generative AI was used in the creation of this manuscript.
All claims expressed in this article are solely those of the authors and do not necessarily represent those of their affiliated organizations, or those of the publisher, the editors and the reviewers. Any product that may be evaluated in this article, or claim that may be made by its manufacturer, is not guaranteed or endorsed by the publisher.
1. Schwartz SL, Cao C, Pylypenko O, Rak A, Wandinger-Ness A. Rab GTPases at a glance. J Cell Sci. (2007) 120(Pt 22):3905–10. doi: 10.1242/jcs.015909
2. Tzeng HT, Wang YC. Rab-mediated vesicle trafficking in cancer. J BioMed Sci. (2016) 23(1):70. doi: 10.1186/s12929-016-0287-7
3. Kuo IY, Hsieh CH, Kuo WT, Chang CP, Wang YC. Recent advances in conventional and unconventional vesicular secretion pathways in the tumor microenvironment. J BioMed Sci. (2022) 29:56. doi: 10.1186/s12929-022-00837-8
4. Li G, Marlin MC. Rab family of GTPases. Methods Mol Biol. (2015) 1298:1–15. doi: 10.1007/978-1-4939-2569-8_1
5. Chano T, Avnet S. RAB39A: a Rab small GTPase with a prominent role in cancer stemness. J Biochem. (2018) 164(1):9–14. doi: 10.1093/jb/mvy041
6. Coppola U, Ristoratore F, Albalat R, D’Aniello S. The evolutionary landscape of the Rab family in chordates. Cell Mol Life Sci. (2019) 76(20):4117–30. doi: 10.1007/s00018-019-03103-7
7. Burk K, Pasterkamp RJ. Disrupted neuronal trafficking in amyotrophic lateral sclerosis. Acta Neuropathol. (2019) 137(6):859–77. doi: 10.1007/s00401-019-01964-7
8. Sinha D, Roy S, Saha P, Chatterjee N, Bishayee A. Trends in research on exosomes in cancer progression and anticancer therapy. Cancers (Basel). (2021) 13(2):326. doi: 10.3390/cancers13020326
9. Jin H, Tang Y, Yang L, Peng X, Li B, Fan Q, et al. Rab GTPases: Central coordinators of membrane trafficking in cancer. Front Cell Dev Biol. (2021) 9:648384. doi: 10.3389/fcell.2021.648384
10. Sneeggen M, Guadagno NA, Progida C. Intracellular transport in cancer metabolic reprogramming. Front Cell Dev Biol. (2020) 8:597608. doi: 10.3389/fcell.2020.597608
11. Stenmark H. Rab GTPases as coordinators of vesicle traffic. Nat Rev Mol Cell Biol. (2009) 10(8):513–25. doi: 10.1038/nrm2728
12. Howe EN, Burnette MD, Justice ME, Schnepp PM, Hedrick V, Clancy JW, et al. Rab11b-mediated integrin recycling promotes brain metastatic adaptation and outgrowth. Nat Commun. (2020) 5:11:3017. doi: 10.1038/s41467-020-16832-2
13. Qiao L, Dong C, Zhang J, Sun G. The expression of Rab5 and its effect on invasion, migration and exosome secretion in triple negative breast cancer. Korean J Physiol Pharmacol. (2023) 27(2):157–65. doi: 10.4196/kjpp.2023.27.2.157
14. Frittoli E, Palamidessi A, Marighetti P, Confalonieri S, Bianchi F, Malinverno C, et al. A RAB5/RAB4 recycling circuitry induces a proteolytic invasive program and promotes tumor dissemination. J Cell Biol. (2014) 206(2):307–28. doi: 10.1083/jcb.201403127
15. Stallaert W, Brüggemann Y, Sabet O, Baak L, Gattiglio M, Bastiaens PIH. Contact inhibitory eph signaling suppresses EGF-promoted cell migration by decoupling EGFR activity from vesicular recycling. Sci Signal. (2018) 11(541):eaat0114. doi: 10.1126/scisignal.aat0114
16. Kajiho H, Kajiho Y, Frittoli E, Confalonieri S, Bertalot G, Viale G, et al. RAB2A controls MT1-MMP endocytic and e-cadherin polarized golgi trafficking to promote invasive breast cancer programs. EMBO Rep. (2016) 17(7):1061–80. doi: 10.15252/embr.201642032
17. Guerra F, Bucci C. Role of the RAB7 protein in tumor progression and cisplatin chemoresistance. Cancers (Basel). (2019) 11(8):1096. doi: 10.3390/cancers11081096
18. Ranieri R, Ciaglia E, Amodio G, Picardi P, Proto MC, Gazzerro P, et al. N6-isopentenyladenosine dual targeting of AMPK and Rab7 prenylation inhibits melanoma growth through the impairment of autophagic flux. Cell Death Differ. (2018) 25(2):353–67. doi: 10.1038/cdd.2017.165
19. Steffan JJ, Dykes SS, Coleman DT, Adams LK, Rogers D, Carroll JL, et al. Supporting a role for the GTPase Rab7 in prostate cancer progression. PloS One. (2014) 9(2):e87882. doi: 10.1371/journal.pone.0087882
21. Grant BD, Donaldson JG. Pathways and mechanisms of endocytic recycling. Nat Rev Mol Cell Biol. (2009) 10(9):597–608. doi: 10.1038/nrm2755
22. Ferro E, Bosia C, Campa CC. RAB11-mediated trafficking and human cancers: An updated review. Biol (Basel). (2021) 10(1):26. doi: 10.3390/biology10010026
23. Xu H, Yuan Y, Wu W, Zhou M, Jiang Q, Niu L, et al. Hypoxia stimulates invasion and migration of human cervical cancer cell lines HeLa/SiHa through the Rab11 trafficking of integrin αvβ3/FAK/PI3K pathway-mediated Rac1 activation. J Biosci. (2017) 42(3):491–9. doi: 10.1007/s12038-017-9699-0
24. Striz AC, Stephan AP, López-Coral A, Tuma PL. Rab17 regulates apical delivery of hepatic transcytotic vesicles. Mol Biol Cell. (2018) 29(23):2887–97. doi: 10.1091/mbc.E18-07-0433
25. Yin C, Argintaru D, Heit B. Rab17 mediates intermixing of phagocytosed apoptotic cells with recycling endosomes. Small GTPases. (2019) 10(3):218–26. doi: 10.1080/21541248.2017.1308852
26. Wang K, Mao Z, Liu L, Zhang R, Liang Q, Xiong Y, et al. Rab17 inhibits the tumourigenic properties of hepatocellular carcinomas via the erk pathway. Tumour Biol. (2015) 36(8):5815–24. doi: 10.1007/s13277-015-3251-3
27. Dozynkiewicz MA, Jamieson NB, Macpherson I, Grindlay J, van den Berghe PVE, von Thun A, et al. Rab25 and CLIC3 collaborate to promote integrin recycling from late endosomes/lysosomes and drive cancer progression. Dev Cell. (2012) 22(1):131–45. doi: 10.1016/j.devcel.2011.11.008
28. Jeong BY, Cho KH, Jeong KJ, Park YY, Kim JM, Rha SY, et al. Rab25 augments cancer cell invasiveness through a β1 integrin/EGFR/VEGF-A/Snail signaling axis and expression of fascin. Exp Mol Med. (2018) 50(1):e435. doi: 10.1038/emm.2017.248
29. Cho SJ, Jeong BY, Yoon SH, Park CG, Lee HY. Rab25 suppresses colon cancer cell invasion through upregulating claudin–7 expression. Oncol Rep. (2024) 51(2):26. doi: 10.3892/or.2023.8685
30. Sun L, Xu X, Chen Y, Zhou Y, Tan R, Qiu H, et al. Rab34 regulates adhesion, migration, and invasion of breast cancer cells. Oncogene. (2018) 37(27):3698–714. doi: 10.1038/s41388-018-0202-7
31. Yang PS, Yu MH, Hou YC, Chang CP, Lin SC, Kuo IY, et al. Targeting protumor factor chitinase-3-like-1 secreted by Rab37 vesicles for cancer immunotherapy. Theranostics. (2022) 12(1):340–61. doi: 10.7150/thno.65522
32. Zou C, Fan J, He M, Xu Y, Wang K, Cai Y, et al. Epigenetic silencing of Rab39a promotes epithelial to mesenchymal transition of cervical cancer through AKT signaling. Exp Cell Res. (2019) 378(2):139–48. doi: 10.1016/j.yexcr.2019.02.025
33. Hinger SA, Abner JJ, Franklin JL, Jeppesen DK, Coffey RJ, Patton JG. Rab13 regulates sEV secretion in mutant KRAS colorectal cancer cells. Sci Rep. (2020) 10:15804. doi: 10.1038/s41598-020-72503-8
34. Lu C, Rong D, Zhang B, Zheng W, Wang X, Chen Z, et al. Current perspectives on the immunosuppressive tumor microenvironment in hepatocellular carcinoma: challenges and opportunities. Mol Cancer. (2019) 18(1):130. doi: 10.1186/s12943-019-1047-6
35. Wang H, Xu H, Chen W, Cheng M, Zou L, Yang Q, et al. Rab13 sustains breast cancer stem cells by supporting tumor-stroma cross-talk. Cancer Res. (2022) 82(11):2124–40. doi: 10.1158/0008-5472.CAN-21-4097
36. Li X, Wang H, Ni Q, Tang Z, Ni J, Xu L, et al. Effects of silencing Rab27a gene on biological characteristics and chemosensitivity of non-small cell lung cancer. Oncotarget. (2017) 8(55):94481–92. doi: 10.18632/oncotarget.21782
37. Zavala-Barrera C, del-Río-Robles JE, García-Jiménez I, Egusquiza-Alvarez CA, Hernández-Maldonado JP, Vázquez-Prado J, et al. The calcium sensing receptor (CaSR) promotes Rab27B expression and activity to control secretion in breast cancer cells. Biochim Biophys Acta (BBA) - Mol Cell Res. (2021) 1868(7):119026. doi: 10.1016/j.bbamcr.2021.119026
38. Kren N, Michaud D, Bagchi S, Greene K, Pylayeva-Gupta Y. Rab27a plays a dual role in metastatic propensity of pancreatic cancer. Sci Rep. (2020) 10(1):7390. doi: 10.1038/s41598-020-64248-1
39. Li TY, Qin C, Zhao BB, Li ZR, Wang YY, Zhao YT, et al. Construction of a prognostic model with exosome biogenesis- and release-related genes and identification of RAB27B in immune infiltration of pancreatic cancer. Transl Cancer Res. (2024) 13(9):4846–65. doi: 10.21037/tcr-24-54
40. Yeung V, Webber JP, Dunlop EA, Morgan H, Hutton J, Gurney M, et al. Rab35-dependent extracellular nanovesicles are required for induction of tumour supporting stroma. Nanoscale. (2018) 10(18):8547–59. doi: 10.1039/C8NR02417K
41. Argenzio E, Margadant C, Leyton-Puig D, Janssen H, Jalink K, Sonnenberg A, et al. CLIC4 regulates cell adhesion and β1 integrin trafficking. J Cell Sci. (2014) 127(Pt 24):5189–203. doi: 10.1242/jcs.150623
42. Kuo IY, Yang YE, Yang PS, Tsai YJ, Tzeng HT, Cheng HC, et al. Converged Rab37/IL-6 trafficking and STAT3/PD-1 transcription axes elicit an immunosuppressive lung tumor microenvironment. Theranostics. (2021) 11(14):7029–44. doi: 10.7150/thno.60040
43. Hou L, Zhao Y, Song GQ, Ma YH, Jin XH, Jin SL, et al. Interfering cellular lactate homeostasis overcomes taxol resistance of breast cancer cells through the microRNA-124-mediated lactate transporter (MCT1) inhibition. Cancer Cell Int. (2019) 19:193. doi: 10.1186/s12935-019-0904-0
44. Lei Y, Zhang E, Bai L, Li Y. Autophagy in cancer immunotherapy. Cells. (2022) 11(19):2996. doi: 10.3390/cells11192996
45. Rakesh R, PriyaDharshini LC, Sakthivel KM, Rasmi RR. Role and regulation of autophagy in cancer. Biochim Biophys Acta Mol Basis Dis. (2022) 1868(7):166400. doi: 10.1016/j.bbadis.2022.166400
46. Toh H, Nozawa T, Minowa-Nozawa A, Hikichi M, Nakajima S, Aikawa C, et al. Group a streptococcus modulates RAB1- and PIK3C3 complex-dependent autophagy. Autophagy. (2020) 16(2):334–46. doi: 10.1080/15548627.2019.1628539
47. Song GJ, Jeon H, Seo M, Jo M, Suk K. Interaction between optineurin and Rab1a regulates autophagosome formation in neuroblastoma cells. J Neurosci Res. (2018) 96(3):407–15. doi: 10.1002/jnr.24143
48. Lőrincz P, Tóth S, Benkő P, Lakatos Z, Boda A, Glatz G, et al. Rab2 promotes autophagic and endocytic lysosomal degradation. J Cell Biol. (2017) 216(7):1937–47. doi: 10.1083/jcb.201611027
49. Tisdale E J, Artalejo C R. Rab2 stimulates LC3 lipidation on secretory membranes by noncanonical autophagy. Exp Cell Res. (2023) 429(1):113635. doi: 10.1016/j.yexcr.2023.113635
50. Liu B, Lu Y, Zhang T, Yu X, Wang Q, Chi Y, et al. CMTM7 as a novel molecule of ATG14L-Beclin1-VPS34 complex enhances autophagy by Rab5 to regulate tumorigenicity. Cell Commun Signal. (2021) 19(1):77. doi: 10.1186/s12964-021-00720-3
51. Rai P, Kumar Roy J. Endosomal recycling protein Rab11 in parkin and Pink1 signaling in drosophila model of parkinson’s disease. Exp Cell Res. (2022) 420(2):113357. doi: 10.1016/J.Yexcr.2022.113357
52. Wauters F, Cornelissen T, Imberechts D, Martin S, Koentjoro B, Sue C, et al. LRRK2 mutations impair depolarization-induced mitophagy through inhibition of mitochondrial accumulation of RAB10. Autophagy. (2020) 16(2):203–22. doi: 10.1080/15548627.2019.1603548
53. Zhang YJ, Pan Q, Yu Y, Zhong XP. microRNA-519d induces autophagy and apoptosis of human hepatocellular carcinoma cells through activation of the AMPK signaling pathway via Rab10. Cancer Manag Res. (2020) 12:2589–602. doi: 10.2147/CMAR.S207548
54. Duan X, Yu X, Li Z. Circular RNA hsacirc0001658 regulates apoptosis and autophagy in gastric cancer through microRNA-182/Ras-related protein rab-10 signaling axis. Bioengineered. (2022) 13(2):2387–97. doi: 10.1080/21655979.2021.2024637
55. Chano T, Kita H, Avnet S, Lemma S, Baldini N. Prominent role of RAB39A-RXRB axis in cancer development and stemness. Oncotarget. (2018) 9(11):9852–66. doi: 10.18632/oncotarget.23955
56. Xing R, Zhou H, Jian Y, Li L, Wang M, Liu N, et al. The Rab7 effector WDR91 promotes autophagy-lysosome degradation in neurons by regulating lysosome fusion. J Cell Biol. (2021) 220(8):e202007061. doi: 10.1083/jcb.202007061
57. Wang X, Zhang L, Chan FKL, Ji J, Yu J, Liang JQ. Gamma-glutamyltransferase 7 suppresses gastric cancer by cooperating with RAB7 to induce mitophagy. Oncogene. (2022) 41(26):3485–97. doi: 10.1038/s41388-022-02339-1
58. Zhu M, Wei C, Wang H, Han S, Cai L, Li X, et al. SIRT1 mediated gastric cancer progression under glucose deprivation through the FoxO1-Rab7-autophagy axis. Front Oncol. (2023) 13:1175151. doi: 10.3389/fonc.2023.1175151
59. Liu Y, Zeng C, Bao N, Zhao J, Hu Y, Li C, et al. Effect of Rab23 on the proliferation and apoptosis in breast cancer. Oncol Rep. (2015) 34(4):1835–44. doi: 10.3892/or.2015.4152
60. Gao Y, Zheng X, Chang B, Lin Y, Huang X, Wang W, et al. Intercellular transfer of activated STING triggered by RAB22A-mediated non-canonical autophagy promotes antitumor immunity. Cell Res. (2022) 32(12):1086–104. doi: 10.1038/s41422-022-00731-w
61. Choi J, Kim H, Bae YK, Cheong H. REP1 modulates autophagy and macropinocytosis to enhance cancer cell survival. Int J Mol Sci. (2017) 18(9):1866. doi: 10.3390/ijms18091866
62. Debnath J, Gammoh N, Ryan KM. Autophagy and autophagy-related pathways in cancer. Nat Rev Mol Cell Biol. (2023) 24(8):560–75. doi: 10.1038/s41580-023-00585-z
63. Yang L, Lin Z, Wang Y, Gao S, Li Q, Li C, et al. MiR-5100 increases the cisplatin resistance of the lung cancer stem cells by inhibiting the Rab6. Mol Carcinog. (2018) 57(3):419–28. doi: 10.1002/mc.22765
64. Recchi C, Seabra MC. Novel functions for Rab GTPases in multiple aspects of tumour progression. Biochem Soc Trans. (2012) 40(Pt 6):1398–403. doi: 10.1042/BST20120199
65. Guerra F, Paiano A, Migoni D, Girolimetti G, Perrone AM, De Iaco P, et al. Modulation of RAB7A protein expression determines resistance to cisplatin through late endocytic pathway impairment and extracellular vesicular secretion. Cancers (Basel). (2019) 11(1):52. doi: 10.3390/cancers11010052
66. Li R, Dong C, Jiang K, Sun R, Zhou Y, Yin Z, et al. Rab27B enhances drug resistance in hepatocellular carcinoma by promoting exosome-mediated drug efflux. Carcinogenesis. (2020) 41(11):1583–91. doi: 10.1093/carcin/bgaa029
67. Chen C, Zhao Z, Tang S, Zhang C. Rab-like protein 1 a is upregulated by cisplatin treatment and partially inhibits chemoresistance by regulating p53 activity. Oncol Lett. (2018) 16(4):4593–9. doi: 10.3892/ol.2018.9205
68. Drizyte-Miller K, Chen J, Cao H, Schott MB, McNiven MA. The small GTPase Rab32 resides on lysosomes to regulate mTORC1 signaling. J Cell Sci. (2020) 133(11):jcs236661. doi: 10.1242/jcs.236661
69. Wu J, Cheng D, Liu L, Lv Z, Liu K. TBC1D15 affects glucose uptake by regulating GLUT4 translocation. Gene. (2019) 683:210–5. doi: 10.1016/j.gene.2018.10.025
70. Jaldin-Fincati JR, Pavarotti M, Frendo-Cumbo S, Bilan PJ, Klip A. Update on GLUT4 vesicle traffic: A cornerstone of insulin action. Trends Endocrinol Metab. (2017) 28(8):597–611. doi: 10.1016/j.tem.2017.05.002
71. Liu Y, Zhang Z, Gao X, Ma Q, Yu Z, Huang S. Rab8A promotes breast cancer progression by increasing surface expression of tropomyosin-related kinase b. Cancer Lett. (2022) 535:215629. doi: 10.1016/j.canlet.2022.215629
72. Brewer PD, Habtemichael EN, Romenskaia I, Mastick CC, Coster ACF. Glut4 is sorted from a Rab10 GTPase-independent constitutive recycling pathway into a highly insulin-responsive Rab10 GTPase-dependent sequestration pathway after adipocyte differentiation. J Biol Chem. (2016) 291(2):773–89. doi: 10.1074/jbc.M115.694919
73. Liu BHM, Tey SK, Mao X, Ma APY, Yeung CLS, Wong SWK, et al. TPI1-reduced extracellular vesicles mediated by Rab20 downregulation promotes aerobic glycolysis to drive hepatocarcinogenesis. J Extracellular Vesicles. (2021) 10(10):e12135. doi: 10.1002/jev2.12135
74. Pei Y, Lv S, Shi Y, Jia J, Ma M, Han H, et al. RAB21 controls autophagy and cellular energy homeostasis by regulating retromer-mediated recycling of SLC2A1/GLUT1. Autophagy. (2023) 19(4):1070–86. doi: 10.1080/15548627.2022.2114271
75. Davey JR, Humphrey SJ, Junutula JR, Mishra AK, Lambright DG, James DE, et al. TBC1D13 is a RAB35 specific GAP that plays an important role in GLUT4 trafficking in adipocytes. Traffic. (2012) 13(10):1429–41. doi: 10.1111/j.1600-0854.2012.01397.x
76. Ding X, Zhang W, Zhao T, Yan C, Du H. Rab7 GTPase controls lipid metabolic signaling in myeloid-derived suppressor cells. Oncotarget. (2017) 8(18):30123–37. doi: 10.18632/oncotarget.16280
77. Tang M, Ren X, Fu C, Ding M, Meng X. Regulating glucose metabolism using nanomedicines for cancer therapy. J Mater Chem B. (2021) 9(29):5749–64. doi: 10.1039/D1TB00218J
78. Wang S, Hu C, Wu F, He S. Rab25 GTPase: Functional roles in cancer. Oncotarget. (2017) 8(38):64591–9. doi: 10.18632/oncotarget.19571
79. Poteryaev D, Fares H, Bowerman B, Spang A. Caenorhabditis elegans SAND-1 is essential for RAB-7 function in endosomal traffic. EMBO J. (2007) 26(2):301–12. doi: 10.1038/sj.emboj.7601498
80. Yoon SO, Shin S, Mercurio AM. Hypoxia stimulates carcinoma invasion by stabilizing microtubules and promoting the Rab11 trafficking of the alpha6beta4 integrin. Cancer Res. (2005) 65(7):2761–9. doi: 10.1158/0008-5472.CAN-04-4122
81. Dorayappan KDP, Wanner R, Wallbillich JJ, Saini U, Zingarelli R, Suarez AA, et al. Hypoxia-induced exosomes contribute to a more aggressive and chemoresistant ovarian cancer phenotype: A novel mechanism linking STAT3/Rab proteins. Oncogene. (2018) 37(28):3806–21. doi: 10.1038/s41388-018-0189-0
82. Rai A, Singh AK, Bleimling N, Posern G, Vetter IR, Goody RS. Rep15 interacts with several Rab GTPases and has a distinct fold for a Rab effector. Nat Commun. (2022) 13(1):4262. doi: 10.1038/s41467-022-31831-1
83. Zeng Z, Zhang Z, Cheng X, Yang H, Gong B, Zhou X, et al. Downregulation of RAB17 have a poor prognosis in kidney renal clear cell carcinoma and its expression correlates with DNA methylation and immune infiltration. Cell Signal. (2023) 109:110743. doi: 10.1016/j.cellsig.2023.110743
84. Hu ZQ, Rao CL, Tang ML, Zhang Y, Lu XX, Chen JG, et al. Rab32 GTPase, as a direct target of miR-30b/c, controls the intracellular survival of burkholderia pseudomallei by regulating phagosome maturation. PloS Pathog. (2019) 15(6):e1007879. doi: 10.1371/journal.ppat.1007879
85. Sheng Y, Song Y, Li Z, Wang Y, Lin H, Cheng H, et al. RAB37 interacts directly with ATG5 and promotes autophagosome formation via regulating ATG5-12-16 complex assembly. Cell Death Differ. (2018) 25(5):918–34. doi: 10.1038/s41418-017-0023-1
86. Li Z, Yue Y, Hu F, Zhang C, Ma X, Li N, et al. Electrical pulse stimulation induces GLUT4 translocation in C2C12 myotubes that depends on Rab8A, Rab13, and Rab14. Am J Physiol Endocrinol Metab. (2018) 314(5):E478–93. doi: 10.1152/ajpendo.00103.2017
87. Li Y, Wang Y, Zou L, Tang X, Yang Y, Ma L, et al. Analysis of the Rab GTPase interactome in dendritic cells reveals anti-microbial functions of the Rab32 complex in bacterial containment. Immunity. (2016) 44(2):422–37. doi: 10.1016/j.immuni.2016.01.027
Keywords: RAB proteins, cancer progression, vesicular trafficking, glucose metabolism, autophagy, drug resistance
Citation: Ji Y, Li R, Tang G, Wang W, Chen C and Yang Q (2025) The interrelated roles of RAB family proteins in the advancement of neoplastic growth. Front. Oncol. 15:1513360. doi: 10.3389/fonc.2025.1513360
Received: 18 October 2024; Accepted: 26 February 2025;
Published: 24 March 2025.
Edited by:
Yuanyuan Zeng, The First Affiliated Hospital of Soochow University, ChinaReviewed by:
Arunkumar Venkatesan, Upstate Medical University, United StatesCopyright © 2025 Ji, Li, Tang, Wang, Chen and Yang. This is an open-access article distributed under the terms of the Creative Commons Attribution License (CC BY). The use, distribution or reproduction in other forums is permitted, provided the original author(s) and the copyright owner(s) are credited and that the original publication in this journal is cited, in accordance with accepted academic practice. No use, distribution or reproduction is permitted which does not comply with these terms.
*Correspondence: Wenrui Wang, d2VucnVpLXdhbmcxOTgzQDE2My5jb20=; Changjie Chen, dHRvY2hlbmNoYW5namllQDE2My5jb20=; Qingling Yang, eXFsbWltaUAxNjMuY29t
Disclaimer: All claims expressed in this article are solely those of the authors and do not necessarily represent those of their affiliated organizations, or those of the publisher, the editors and the reviewers. Any product that may be evaluated in this article or claim that may be made by its manufacturer is not guaranteed or endorsed by the publisher.
Research integrity at Frontiers
Learn more about the work of our research integrity team to safeguard the quality of each article we publish.