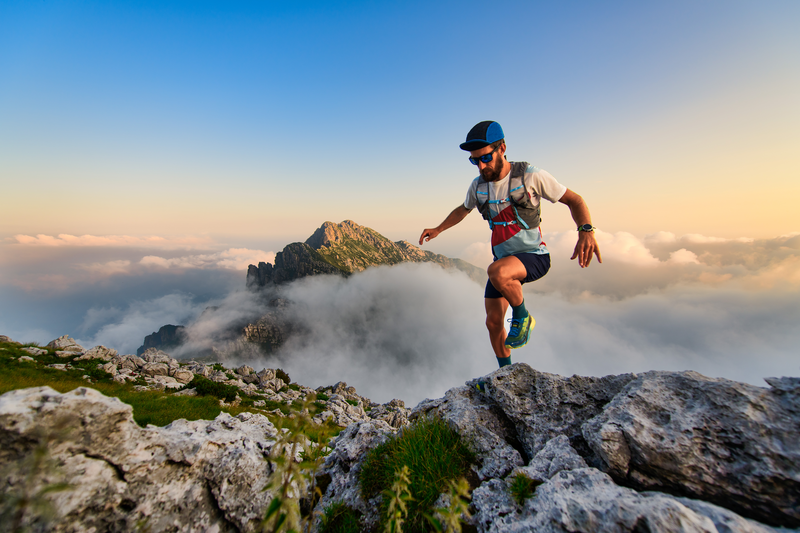
94% of researchers rate our articles as excellent or good
Learn more about the work of our research integrity team to safeguard the quality of each article we publish.
Find out more
REVIEW article
Front. Oncol.
Sec. Radiation Oncology
Volume 15 - 2025 | doi: 10.3389/fonc.2025.1511261
This article is part of the Research Topic Use of Radiation Therapy for Hematological Malignancies View all 6 articles
The final, formatted version of the article will be published soon.
You have multiple emails registered with Frontiers:
Please enter your email address:
If you already have an account, please login
You don't have a Frontiers account ? You can register here
Hematologic malignancies, including leukemias, lymphomas, and myeloma, can involve the central nervous system (CNS) at the time of diagnosis or later in relapse. CNS involvement can lead to acute neurologic symptoms or signs that need prompt evaluation and treatment. Radiotherapy (RT) can lead to quick disease response, but how it can best be incorporated early into multi-modality treatment in the urgent clinical setting is often unclear.Here, we outline a practical approach to planning and incorporating urgent RT in patients with hematologic malignancies involving the CNS. We provide a review of the literature to inform RT indications, timing, dosing, and treatment volumes by histology and clinical scenario. We also highlight evolving controversies in this field and growing indications for RT in conjunction with novel therapeutics.RT is often the quickest-acting, most reliable tool to salvage cranial neuropathies or neurologic deficits and should be considered early. If systemic or intrathecal therapy are expected to achieve swift response as upfront treatment, simulation should still be planned in the event response is delayed and RT is needed. RT in combination with certain systemic or intrathecal therapies can lead to unacceptable neurotoxicity; therefore, early multidisciplinary discussion to appropriately sequence therapies is critical. Thorough work-up with systemic imaging, complete neuroaxis MRI, ophthalmologic exam, and cerebrospinal fluid sampling can dictate target volumes from focal RT to comprehensive craniospinal irradiation (CSI). Dosing can range from as low as 4 Gray (Gy) for indolent disease to 36-50 Gy for more aggressive or refractory disease. Often, mid-treatment replanning can be considered to address swift volume reduction to improve the therapeutic window. RT plays a promising role of bridging symptomatic patients to novel therapeutics (e.g., CAR T-cell therapy), but optimal dosing and treatment volumes are an evolving topic that requires further prospective evaluation.RT is a powerful tool for achieving rapid responses in hematologic malignancies and therefore should be considered early in urgent neurologic settings. Thorough workup and discussions with the multi-disciplinary team are critical to best incorporate RT in the context of other CNSpenetrating therapies. Further work is warranted on defining RT target volumes in the context of novel therapeutics.
Keywords: Central Nervous System, Lymphoma, Radiotherapy, emergency, urgency
Received: 14 Oct 2024; Accepted: 25 Feb 2025.
Copyright: © 2025 Tringale, Imber, Cederquist, Yahalom, Moore, Hoppe, Binkley, Ross, Wijetunga, Sanghvi, Casey and Hiniker. This is an open-access article distributed under the terms of the Creative Commons Attribution License (CC BY). The use, distribution or reproduction in other forums is permitted, provided the original author(s) or licensor are credited and that the original publication in this journal is cited, in accordance with accepted academic practice. No use, distribution or reproduction is permitted which does not comply with these terms.
* Correspondence:
Kathryn Tringale, University of California, San Diego, La Jolla, United States
Disclaimer: All claims expressed in this article are solely those of the authors and do not necessarily represent those of their affiliated organizations, or those of the publisher, the editors and the reviewers. Any product that may be evaluated in this article or claim that may be made by its manufacturer is not guaranteed or endorsed by the publisher.
Research integrity at Frontiers
Learn more about the work of our research integrity team to safeguard the quality of each article we publish.