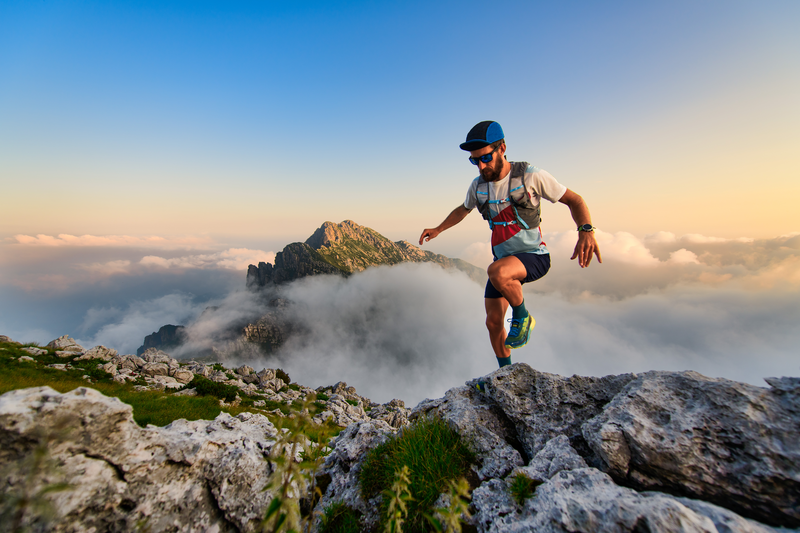
95% of researchers rate our articles as excellent or good
Learn more about the work of our research integrity team to safeguard the quality of each article we publish.
Find out more
ORIGINAL RESEARCH article
Front. Oncol. , 30 January 2025
Sec. Breast Cancer
Volume 15 - 2025 | https://doi.org/10.3389/fonc.2025.1494862
Background: To compare the diagnostic effectiveness of ultrasound (US), magnetic resonance imaging (MRI), and their combined application in distinguishing between benign and malignant breast tumors, with particular emphasis on evaluating diagnostic performance in different breast densities—fatty breast tissue, where fat predominates, and dense breast tissue, which contains a significant amount of fibroglandular tissue.
Materials and methods: A retrospective analysis was conducted on 185 patients with breast tumors, including 90 malignant and 95 benign cases. All patients underwent both US and MRI examinations within one week prior to surgery. The diagnostic accuracy of US, MRI, and their combined use in differentiating benign and malignant tumors was evaluated.
Results: The combined examination demonstrated the highest area under the curve (AUC), sensitivity, and negative predictive value (NPV) (0.904, 90%, 90.4%), outperforming US (0.830, 73.3%, 78.6%) and MRI (0.897, 89.7%, 88.8%). DeLong test results revealed statistically significant differences in AUC between US and MRI, as well as between US and the combined examination (P < 0.05). However, the difference in AUC between MRI and the combined examination was not significant (P = 0.939). In patients with fatty breast tissue, no significant differences were found between MRI and US, or between MRI and the combined examination (P = 0.708 and P = 0.317, respectively). However, the diagnostic performance between US and the combined examination was statistically significant (P < 0.05). For patients with dense breast tissue, the differences in diagnostic performance between US and MRI, and between US and the combined examination, were significant (P < 0.05), while the difference between MRI and the combined examination was not significant (P = 0.317).
Conclusion: MRI and combined examination methods significantly enhance the ability to differentiate benign and malignant breast tumors and provide important clinical value for early breast cancer detection.
Breast Cancer (BC) is the most common cancer among women worldwide and the second leading cause of cancer-related deaths (1). Timely and standardized treatment of benign breast lesions can significantly reduce the risk of cancer. However, BC is challenging to detect in its early stages and is often diagnosed in the middle or late stages, characterized by a high metastasis rate and poor prognosis (1–9). Therefore, accurate identification of breast lesions is of critical clinical importance, as it directly impacts patient management, treatment decisions, and prognosis (2). Recent studies have identified dense breasts as one of the most significant risk factors for breast cancer, with the risk being approximately two times higher in women with dense breasts compared to the average screened population (10, 11). The glandular tissue in dense breasts is more abundant, making it difficult to detect lesions against the background of normal breast parenchyma, as they may be obscured by the normal tissue (12). Consequently, patients with dense breasts require special attention. Mammography is sensitive to microcalcifications, but its sensitivity is significantly reduced in women with dense breasts, ranging from approximately 47.8% to 64.4% (13–16). This can lead to a certain degree of misdiagnosis, potentially causing patients to miss the optimal treatment window, which in turn affects survival rates and quality of life. Additionally, misdiagnosis can contribute to the wastage of medical resources, prolong hospital stays, and increase psychological stress and economic burden. Therefore, reducing misdiagnosis and improving diagnostic accuracy are crucial objectives in clinical practice. Breast magnetic resonance imaging (MRI) has high sensitivity and is not affected by breast density. It can also evaluate extramammary areas, including the contralateral breast, chest wall, axillary region, and lymph nodes (17). It can also detect occult lesions that breast ultrasound (US) and digital mammography cannot identify, aiding in lesion detection and improving the accuracy of early diagnosis, thereby reducing mortality risk. However, it has a high false-positive rate and some contraindications (8, 18, 19). The US has been used at various stages of breast cancer management, including screening dense breasts, diagnosis, chemotherapy, and prognosis, due to its non-invasive nature, lack of ionizing radiation, portability, real time capability to guide biopsies, and cost-effectiveness (20). However, the accuracy of the diagnosis heavily depends on the operator, leading to a large number of unnecessary biopsies and short-term follow-up rates (20–23).
The combined use of US and MRI can fully leverage their complementary advantages, potentially improving the accuracy of differential diagnosis between benign and malignant breast tumors and increasing the early detection rate of malignant tumors. This enables timely intervention, optimizing treatment plans and patient care. Therefore, this study aims to compare the diagnostic efficacy of US, MRI, and their combination in differentiating benign and malignant breast tumors. The focus is on analyzing the diagnostic efficacy of these three methods in different breast densities (fatty and dense breasts). This research hopes to provide more precise and reliable imaging support for BC diagnosis, promoting advancements in early diagnosis and personalized treatment of breast cancer.
This retrospective study included 381 patients with breast tumors who visited the First Affiliated Hospital of Bengbu Medical University from January 2023 to May 2024. Inclusion criteria: (1) Underwent both US and MRI examinations within one week before surgery; (2) Had not received any breast surgery, radiotherapy, or chemotherapy before the study; (3) Had good quality US and MRI images; (4) Had complete histological characteristics. Exclusion criteria: (1) Age below 20 years; (2) Male patients with breast tumors; (3) Incomplete examination data or missing histopathological features. Ultimately, 185 patients aged 20-75 years (median age 47 years, range 35-54 years) were included in the study. This study adhered to the Declaration of Helsinki and used a retrospective study method, with all patients waiving informed consent. The population selection and trial overview are shown in Figure 1.
According to the 5th edition of the Breast Imaging Reporting and Data System (BI-RADS) lexicon (24), breast density is classified into four categories: a, b, c, and d. Categories a and b are considered fatty breasts, while categories c and d are considered dense breasts. The diagnostic efficacy of the three methods was considered statistically significant if P was less than or equal to 0.05. Breast density was determined using MRI as the standard, and the nature of the tumor (benign or malignant) was confirmed through pathological examination. All patients underwent both US and MRI examinations within one week before surgery.
Routine Sequences: T2WI-TRA-Fat Suppression: TR: 3200 ms; TE: 80 ms; Field of View (FOV): 340 x 340 mm; Slice thickness: 4 mm; T1WI-TRA: TR: 5.23 ms; TE: 1.8 ms; FOV: 340 x 340 mm; Slice thickness: 1.2 mm.
Diffusion-weighted Imaging (DWI): Single exponential diffusion-weighted scan (DWI): Diffusion weighting factor (b-values): 0, 1000 s/mm²; TR/TE: 6375 ms/69.8 ms; FOV: 320 mm x 320 mm; Slice thickness: 5 mm.
Dynamic Contrast-Enhanced Imaging: TR: 6.35 ms; TE: 1.3 ms; Slice thickness: 1.2 mm; FOV: 340 x 340 mm; Dynamic enhancement scan with 9 series; Repeat scan 6 times, each scan duration 60 s.
The MRI examinations were performed using a GE Signa 3.0T MRI scanner equipped with a dedicated 8-channel phased-array breast coil. Patients were positioned prone with their feet first, allowing both breasts to naturally hang within the breast coil for imaging. Images were uploaded to the workstation for analysis of lesion characteristics, primarily focusing on size, shape (regular or irregular), and margin clarity. Time-Intensity Curve analysis was conducted to assess lesion enhancement patterns. DWI was processed to generate Apparent Diffusion Coefficient (ADC) maps post-acquisition.
Ultrasound images were acquired in three planes: transverse, coronal, and sagittal, following the BI-RADS lexicon (24) lexicon. The analysis included the evaluation of characteristics such as margin clarity (clear or unclear), shape (regular or irregular), and echogenic pattern.
Both US and MRI images were assessed independently by two breast radiologists with 6 and 10 years of experience in interpreting breast ultrasound images. They were blinded to clinical information and patient age. In cases of disagreement, radiologists discussed the issue and reached a consensus.
According to the BI-RADS lexicon (24) from the American College of Radiology, breast lesions were categorized into 3-5 classes: lesions classified as 3-4a were considered benign, while those classified as 4b, 4c, and 5 were considered suspicious for malignancy.
All patients underwent pathological and immunohistochemical examinations. The expression of HER-2 gene immunohistochemistry is categorized as − or + for negative, and +++ for positive. If HER-2 expression is ++, FISH examination is conducted to determine gene amplification, with positive indicating amplification and negative indicating no amplification (25, 26).
Statistical analyses were conducted using SPSS Statistics 27.0 (IBM Corp., Chicago, Illinois, USA), R (version 3.5.2), and R Studio (version 1.1.456). Continuous variables with a normal distribution were presented as means with standard deviations, while non-normally distributed variables were represented as medians with interquartile ranges. Categorical data were shown as counts with percentages. The normality of the distributions was evaluated using the Shapiro-Wilk test. For univariate analysis, comparisons of continuous variables were made using Student’s t-test (for normally distributed data) or the Mann-Whitney U test (for non-normally distributed data), while categorical variables were compared using the Chi-square test or Fisher’s Exact test. Sensitivity, specificity, accuracy, and AUC, along with the corresponding 95% confidence intervals (CIs), were computed using the R package report ROC. The DeLong test, a statistical method frequently employed to compare differences between two or more receiver operating characteristic (ROC) curves, was used to evaluate the AUC. This method is particularly valuable for the early diagnosis of breast cancer. A p-value of less than 0.05 was considered statistically significant.
Out of the 381 enrolled female patients, 79 had mastitis, 6 were male patients, 91 had undergone preoperative surgery and radiotherapy/chemotherapy, 14 had incomplete pathological diagnosis data, and 6 were under 20 years old. Therefore, these were excluded, leaving a final cohort of 185 patients (median age 47 years, range 35-54 years). All patients underwent both US and MRI examinations before surgery, with pathological diagnosis and biopsy results serving as the gold standard.
There are a total of 185 breast lumps among these patients, and their clinical information is shown in Table 1. According to BI-RADS classification, BI-RADS 3-4A were assessed as benign lesions, while BI-RADS 4B-5 were assessed as malignant lesions. There were 95 benign lesions, with a median tumor diameter of 1.7 cm (range: 1.2-2.55 cm). These included 69 cases of fibroadenoma, 6 cases of benign phyllodes tumor, and 24 cases of intraductal papilloma. There were 90 cases of breast cancer, with a median tumor diameter of 2.2 cm (range: 1.8-3 cm). Among these, the majority were non-specific invasive carcinoma (74 cases), with 4 cases of invasive lobular carcinoma, 2 cases of ductal carcinoma in situ, and 1 case of malignant phyllodes tumor.
Among the 185 breast tumors, there were 95 benign tumors and 90 malignant tumors. The diagnostic results are as follows: Benign tumors: US: Detected 88 benign tumors; MRI: Detected 87 benign tumors; US + MRI (Combined): Detected 85 benign tumors; Malignant tumors: US: Detected 66 malignant tumors; MRI: Detected 79 malignant tumors; US + MRI (Combined): Detected 87 malignant tumors.
The diagnostic performance of MRI alone is superior to that of the US alone (P < 0.05). There is no significant difference in diagnostic performance between MRI alone and the combined use of US and MRI (P = 0.052). The combined diagnostic performance of US and MRI is superior to that of US alone (P < 0.05), as shown in Table 2.
Table 2. Number of cases diagnosed with benign and malignant breast tumors by US, MRI, and combined examinations (n).
Pathological and immunohistochemical examinations revealed the following histological subtypes among the 90 malignant breast tumors: Subtype Distribution: Luminal A:3 cases; Luminal B:53 cases; Triple-negative:16 cases; HER-2 overexpression:18 cases; Receptor Status: ER (Estrogen Receptor): ER-: 31 cases (34.4%); ER+: 59 cases (65.6%); PR (Progesterone Receptor): PR-: 32 cases (35.6%); PR+: 58 cases (64.4%); HER-2 (Human Epidermal Growth Factor Receptor 2): HER-2-: 63 cases (70%); HER-2+: 27 cases (30%); These distributions are illustrated in Figure 2.
The diagnostic performance metrics of US, MRI, and the combined are as follows: US: Accuracy:83.2% [95% CI: 0.773-0.876]; Sensitivity:73.3% [95% CI: 0.634-0.809]; Specificity:92.6% [95% CI: 0.874-0.979]; Positive Predictive Value (PPV):90.4% [95% CI: 0.873-0.972]; Negative Predictive Value (NPV):78.6% [95% CI: 0.71-0.862]; MRI: Accuracy:89.7% [95% CI: 0.857-0.962]; Sensitivity:87.8% [95% CI: 0.81-0.945]; Specificity:91.6% [95% CI: 0.86-0.972]; Positive Predictive Value (PPV):90.8% [95% CI: 0.847-0.969]; Negative Predictive Value (NPV): 88.8% [95%CI:0.825-0.95]; Combined: Accuracy:89.7%[95%CI:0.849-0.935]; Sensitivity:90%[95% CI: 0.838-0.962]; Specificity:89.5% [95% CI: 0.833-0.956]; Positive Predictive Value (PPV):89% [95% CI: 0.826-0.954]; Negative Predictive Value (NPV):89.72% [95% CI: 0.849-0.935]; These results are summarized in Table 3.
Table 3. Diagnostic efficacy of US, MRI, and combined examinations in differentiating benign and malignant breast tumors.
The clinical value of US, MRI, and the combined can be assessed using the area under the curve (AUC) values: AUC Values: US:0.830[95% CI: 0.769-0.976]; MRI:0.897 [95% CI: 0.854-0.936]; Combined:0.904 [95% CI: 0.845-0.964]; The differences in AUC between US and MRI, and between US and the combined examination, are statistically significant (P < 0.05).
There is no statistically significant difference in AUC between MRI and the combined examination (P = 0.939, Z = 0.076). MRI alone and combined MRI with the US have superior diagnostic performance compared to the US alone (P < 0.05). There is no significant difference in diagnostic performance between MRI alone and the combined examination (P = 0.052). These findings are summarized in Table 4.
Table 4. Comparison of diagnostic efficacy of US, MRI, and combined examinations in differentiating benign and malignant breast tumors.
The diagnostic performance of US, MRI, and their combined use can vary based on different fibroglandular tissue (FGT) categories in breast density. In Fatty Breast Tissue, the diagnostic performance differences between MRI and US alone, and between MRI and the combined examination, are not statistically significant (P = 0.708, P = 0.317 respectively). The difference between the US alone and the combined examination is statistically significant (P < 0.05). In Dense Breast Tissue, the diagnostic performance differences between the US and MRI alone, and between the US and the combined examination, are statistically significant (P < 0.05). There is no statistically significant difference in diagnostic performance between MRI alone and the combined examination (P = 0.317). These results are summarized in Table 5.
Table 5. Pairwise comparison of FGT classification of benign and malignant breast tumors by US, MRI, and combined examinations at different breast densities.
BC originating from the epithelial cells of the mammary gland, is a primary cause of cancer-related deaths in women (27). Research (28) indicates that the five-year survival rate surpasses 95% for patients diagnosed at stages I or II, but significantly drops for those diagnosed at later stages. As a result, accurately distinguishing breast lesions is critically important, as it directly influences patient survival, management strategies, treatment options, and overall prognosis (2). his study therefore examines the diagnostic performance of US, MRI, and their combined use in differentiating benign from malignant breast tumors. It is also the first to investigate the effectiveness of US, MRI, and their combination in distinguishing between benign and malignant lesions.
The results demonstrate that the combined examination of US and MRI achieved the highest values for AUC at 0.904, sensitivity at 90%, NPV at 90.4%, and accuracy at 89.7% (US: AUC 0.830, sensitivity 73.3%, NPV 78.6%, accuracy 83.2%; MRI: AUC 0.897, sensitivity 89.7%, NPV 88.8%, accuracy 89.7%). Both MRI alone and the combined examination showed consistently superior performance compared to the US alone in distinguishing benign from malignant breast tumors. Moreover, the addition of MRI to the US significantly increased sensitivity from 73.3% to 90% in diagnostic assessments. Schmidt et al. (29) found that the PPV of US for residual tumor areas was 77.22%, compared to 74.36% for mammography. Our study demonstrates the PPV of 90.4% for breast US, indicating higher PPV compared to mammography. Jing Chen et al. (30) reported the NPV of 53.98% for breast US, while Schaeffer et al. (31) reported an NPV of 48.1%. In contrast, our study shows an NPV of 78.6% and an accuracy of 83.2%, with the AUC of 0.830 (0.769-0.976), indicating that breast US exhibits good discriminatory performance in distinguishing benign from malignant breast tumors.
These findings provide valuable insights into early detection of breast tumors and personalized treatment strategies. BC poses a serious threat to women’s health globally, and early detection and accurate diagnosis are crucial for improving patient outcomes (9, 30). MRI offers advantages such as high soft tissue contrast and high resolution, potentially providing a new method for distinguishing between benign and malignant breast tumors (32). DWI utilizes the restricted diffusion of water molecules, with malignant tumors typically exhibiting lower ADC values compared to benign tumors (33). Dynamic contrast enhanced (DCE)scanning can obtain semi-quantitative parameters and time-intensity curves, which can better distinguish between benign and malignant breast tumors (34). In future studies, we will place greater emphasis on multi-modal MRI research, specifically analyzing the diagnostic efficacy of DWI and DCE for distinguishing between benign and malignant breast tumors.
The cost-effectiveness of MRI is a widely debated issue. Recent studies have demonstrated that UF-DCE-MRI provides diagnostic performance that is either comparable to or even exceeds that of conventional DCE-MRI (34–43). Kuhl et al. introduced a simplified MRI protocol (44), and in a study involving 443 women and 606 MRI exams, they found that the diagnostic accuracy of the simplified protocol was equal to that of the full protocol. The development of these faster sequences is expected to lower the cost of MRI exams and improve their cost-effectiveness.
In this study, MRI demonstrated an accuracy of 89.7%, sensitivity of 87.8%, specificity of 89.7%, PPV of 90.8%, NPV of 88.8%, and an AUC of 0.897 (0.854-0.936). Compared to US alone, MRI exhibited superior diagnostic performance. When combined with US, MRI showed similar diagnostic results. Our findings suggest that MRI is more effective in distinguishing the nature of breast tumors, underscoring its clinical importance in the early detection of BC.
Early detection is closely linked to improved BC survival rates. Systematic mammography has been used for decades to enhance early BC detection, yet breast cancer continues to be a leading cause of cancer-related deaths among women (45). Research indicates that dense breast tissue is an independent risk factor for BC, potentially masking tumors on mammograms and resulting in missed diagnoses (46–48). Therefore, novel imaging techniques are needed to improve the early differentiation of benign and malignant breast tumors, reduce the likelihood of benign tumors turning malignant, and ultimately lower BC mortality rates.
In this study, we utilized MRI’s high sensitivity, which remains unaffected by breast density, alongside the real-time evaluation offered by US. The combination of both methods yielded promising results. In dense breast tissue, the diagnostic performance of MRI and the combined approach surpassed that of US alone, while the performance of standalone MRI was similar to the combined approach. In fatty breast tissue, the diagnostic results from US and MRI were generally consistent, detecting 66/90 and 79/90 malignant tumors, and 88/95 and 87/95 benign tumors, respectively. In the study by Wienbeck et al. (49), the diagnostic accuracy of contrast-enhanced cone-beam breast CT was assessed in dense breast tissue and compared with non-contrast cone-beam breast CT, mammography, and MRI. The results demonstrated that MRI provided superior accuracy and AUC, with values of 88% and 0.89, respectively. In this study, MRI achieved an accuracy of 89.7% and an AUC of 0.897, aligning closely with the findings of Wienbeck et al. (49). A recent study using a deep learning model based on multimodal imaging for differentiating benign and malignant breast lesions (27) reported an AUC of 0.943 (95% CI 0.792–0.995). In comparison, the AUC for combined US and MRI diagnosis in our study was 0.904 (0.845–0.964), while the AUC for US alone was 0.830 (0.769–0.976). When compared to the performance of combined imaging and deep learning models, the diagnostic effectiveness of US alone was significantly lower. However, the combined diagnostic approach demonstrated accuracy comparable to that of artificial intelligence models, suggesting its potential for providing practical recommendations in the early differential diagnosis of breast tumors.
This study has several limitations. First, it is a single-center study with a relatively small sample size, so future research will involve multi-center, large-scale studies with more extensive sample sizes. Second, the sample distribution is uneven, with malignant tumors predominantly consisting of non-specific invasive breast cancer, which may introduce selection bias. To address this, future studies will include a broader variety of tumor types, such as ductal carcinoma in situ and lobular carcinoma. Third, this study included various types of benign and malignant breast tumors, but for the analysis of the diagnostic value of US, MRI, and their combined use, these lesions were simply divided into two groups without further subgroup analysis based on molecular subtypes. Fourth, since this study focused on comparing the diagnostic performance of US, MRI, and combined diagnostics in differentiating between benign and malignant breast tumors, dynamic contrast-enhanced sequences and DWI were not analyzed separately. We plan to explore this aspect in future research. Finally, this study primarily included BI-RADS 3 and BI-RADS 5 lesions, resulting in a larger average tumor size. Future studies will include smaller lesions to validate the findings of this study.
The original contributions presented in the study are included in the article/supplementary material. Further inquiries can be directed to the corresponding author.
The studies involving humans were approved by the institutional review board of The First Affiliated Hospital of Bengbu Medical College (Reference number:2023-441), The studies were conducted in accordance with the local legislation and institutional requirements. The ethics committee/institutional review board waived the requirement of written informed consent for participation from the participants or the participants’ legal guardians/next of kin because This is a retrospective study. This study adhered to the Declaration of Helsinki and used a retrospective study method, with all patients waiving informed consent. Written informed consent was not obtained from the individual(s) for the publication of any potentially identifiable images or data included in this article because This retrospective study was approved by the institutional review board of our hospital (Reference number:2023-441), and the requirement for the patient’s informed consent was waived. This study adhered to the Declaration of Helsinki.
DM: Conceptualization, Data curation, Formal analysis, Investigation, Methodology, Project administration, Software, Supervision, Validation, Writing – original draft, Writing – review & editing. CW: Conceptualization, Data curation, Formal analysis, Investigation, Methodology, Software, Supervision, Writing – review & editing. JL: Conceptualization, Data curation, Formal analysis, Investigation, Methodology, Project administration, Software, Writing – review & editing. XH: Conceptualization, Data curation, Investigation, Methodology, Software, Supervision, Writing – review & editing. YZ: Conceptualization, Investigation, Methodology, Software, Supervision, Writing – review & editing. ZG: Conceptualization, Formal analysis, Investigation, Methodology, Project administration, Supervision, Writing – review & editing. CLi: Conceptualization, Formal analysis, Investigation, Software, Writing – review & editing. CLu: Software, Writing – review & editing. YH: Supervision, Validation, Writing – original draft, Software, Writing – review & editing, Conceptualization, Data curation, Formal analysis, Investigation, Methodology, Project administration.
The author(s) declare financial support was received for the research, authorship, and/or publication of this article. This work was supported by the Natural Science University-Level Key Project (2021byzd027) and the Key Project of Natural Science Research at Anhui Province Universities (2022AH051473).
The authors extend their gratitude to the patients for their willingness to participate in the study. This research work was funded by the Key Project of Natural Science Research at Anhui Province Universities (2022AH051473), and the Natural Science University-Level Key Project (2021byzd027).
Author XH, CLu were employed by the company Anhui Fu qing Medical Equipment Co. LTD.
The remaining authors declare that the research was conducted in the absence of any commercial or financial relationships that could be constructed as a potential conflict of interest.
All claims expressed in this article are solely those of the authors and do not necessarily represent those of their affiliated organizations, or those of the publisher, the editors and the reviewers. Any product that may be evaluated in this article, or claim that may be made by its manufacturer, is not guaranteed or endorsed by the publisher.
1. Siegel RL, Miller KD, Wagle NS, Jemal A. Cancer statistics, 2023. CA Cancer J Clin. (2023) 73.1:17–48. doi: 10.3322/caac.21763
2. Cao Y, Huang Y, Chen X, Wang W, Chen H, Yin T, et al. Optimizing ultrafast dynamic contrast-enhanced MRI scan duration in the differentiation of benign and Malignant breast lesions. Insights Imaging. (2024) 15:112. doi: 10.1186/s13244-024-01697-6
3. Verdial FC, Bartek MA, Anderson BO, Javid SH. Genetic testing and surgical treatment after breast cancer diagnosis: Results from a national online cohort. J Surg Oncol. (2021) 123:1504–12. doi: 10.1002/jso.26372
4. Zhang Y, Chen J, Mi D, Ling J, Li H, He P, et al. Discovery of YH677 as a cancer stemness inhibitor that suppresses triple-negative breast cancer growth and metastasis by regulating the TGFβ signaling pathway. Cancer Lett. (2023) 560:216142. doi: 10.1016/j.canlet.2023.216142
5. Bernardo G, Le Noci V, Ottaviano E, De Cecco L, Camisaschi C, Guglielmetti S, et al. Reduction of Staphylococcus epidermidis in the mammary tumor microbiota induces antitumor immunity and decreases breast cancer aggressiveness. Cancer Lett. (2023) 555:216041. doi: 10.1016/j.canlet.2022.216041
6. Xiao Q, Xia M, Tang W, Zhao H, Chen Y, Zhong J. The lipid metabolism remodeling: A hurdle in breast cancer therapy. Cancer Lett. (2024) 582:216512. doi: 10.1016/j.canlet.2023.216512
7. Liang Y, Lei R, Tan J, Fang J, Yu L, Tan S, et al. HER2-targeting peptide drug conjugate with better penetrability for effective breast cancer therapy. Bio Integration. (2023) 4:170–9. doi: 10.15212/bioi-2023-0006
8. Sung H, Ferlay J, Siegel RL, Ferlay J, Siegel RL, Laversanne M, Soerjomataram I, Jemal A, et al. Global cancer statistics 2020: GLOBOCAN estimates of incidence and mortality worldwide for 36 cancers in 185 countries. CA Cancer J Clin. (2021) 71:209–49. doi: 10.3322/caac.21660
9. Lin Z, Chen L, Wang Y, Zhang T, Huang P. Improving ultrasound diagnostic precision for breast cancer and adenosis with modality-specific enhancement (MSE)-breast net. Cancer Lett. (2024) 596:216977. doi: 10.1016/j.canlet.2024.216977
10. Fan L, Zheng Y, Yu KD, et al. Breast cancer in a transitional society over 18 years: trends and present status in Shanghai, China[J. Breast Cancer Res Treat. (2009) 117:409–16. doi: 10.1007/s10549-008-0303-z
11. Price ER, Hargreaves J, Lipson JA, Sickles EA, Brenner RJ, Lindfors KK, et al. The California breast density information group: a collaborative response to the issues of breast density, breast cancer risk, and breast density notification legislation. Radiology. (2013) 269:887–92. doi: 10.1148/radiol.13131217
12. Luiten JD, Voogd AC, Luiten EJT, Duijm LEM. Trends in incidence and tumour grade in screen-detected ductal carcinoma in situ and invasive breast cancer. Breast Cancer Res Treat. (2017) 166:307–14. doi: 10.1007/s10549-017-4412-4
13. Hofvind S, Moshina N, Holen ÅS, Danielsen AS, Lee CI, Houssami N, et al. Interval and subsequent round breast cancer in a randomized controlled trial comparing digital breast tomosynthesis and digital mammography screening. Radiology. (2021) 300:66–76. doi: 10.1148/radiol.2021203936
14. Comstock CE, Gatsonis C, Newstead GM, Snyder BS, Gareen IF, Bergin JT, et al. Comparison of abbreviated breast MRI vs digital breast tomosynthesis for breast cancer detection among women with dense breasts undergoing screening [published correction appears in JAMA. JAMA. (2020) 323:1194. doi: 10.1001/jama.2020.2991. 2020;323(8):746-756. doi:10.1001/jama.2020.0572.
15. Brem RF, Lenihan MJ, Lieberman J, Torrente J. Screening breast ultrasound: past, present, and future. AJR Am J Roentgenol. (2015) 204:234–40. doi: 10.2214/AJR.13.12072
16. Johnson K, Lång K, Ikeda DM, Åkesson A, Andersson I, Zackrisson S. Interval breast cancer rates and tumor characteristics in the prospective population-based Malmö Breast Tomosynthesis Screening Trial. Radiology. (2021) 299.3:559–67. doi: 10.1148/radiol.2021204106
17. Kuhl C. The current status of breast MR imaging. Part I. Choice of technique, image interpretation, diagnostic accuracy, and transfer to clinical practice. Radiology. (2007) 244:356–78. doi: 10.1148/radiol.2442051620
18. Peters C, White R, Brown S. Magnetic resonance imaging for breast cancer diagnosis: advancements and clinical applications. J Clin Oncol. (2019) 37:1123–35.
19. Grigoryants NF, Sass S, Alexander J. Novel technologies in breast imaging: A scoping review. Cureus. (2023) 15:e44061. doi: 10.7759/cureus.44061
20. Afrin H, Larson NB, Fatemi M, Alizad A. Deep learning in different ultrasound methods for breast cancer, from diagnosis to prognosis: current trends, challenges, and an analysis. Cancers (Basel). (2023) 15:3139. doi: 10.3390/cancers15123139
21. Berg WA, Blume JD, Cormack JB, Mendelson EB, Lehrer D, Böhm-Vélez M, et al. Combined screening with ultrasound and mammography vs mammography alone in women at elevated risk of breast cancer. JAMA. (2008) 299:2151–63. doi: 10.1001/jama.299.18.2151
22. Hooley RJ, Greenberg KL, Stackhouse RM, Geisel JL, Butler RS, Philpotts LE. Screening US in patients with mammographically dense breasts: initial experience with Connecticut Public Act 09-41. Radiology. (2012) 265:59–69. doi: 10.1148/radiol.12120621
23. Rahmat K, Mumin NA, Hamid MTR, Hamid SA, Ng WL. MRI breast: current imaging trends, clinical applications, and future research directions. Curr Med Imaging. (2022) 18.13:1347–61. doi: 10.2174/1573405618666220415130131
24. D’Orsi CJ, Sickles EA, Bassett LW, Mendelson EB, Morris EA. ACR BI-RADS® mammography ACR BI-RADS® Atlas[J]. Breast Imaging Reporting and Data System. (2013) 5:2013.
25. Wolff AC, Somerfield MR, Dowsett M, Hammond MEH, Hayes DF, McShane LM, et al. Human epidermal growth factor receptor 2 testing in breast cancer: ASCO-college of american pathologists guideline update. J Clin Oncol. (2023) 41:3867–72. doi: 10.1200/JCO.22.02864
26. Wolff AC, Hammond MEH, Allison KH, Wolff AC, Hammond MEH, Allison KH, et al. Human epidermal growth factor receptor 2 testing in breast cancer: American Society of Clinical Oncology/College of American Pathologists clinical practice guideline focused update. Arch Pathol Lab Med. (2018) 36142:2105–221364–82. doi: 10.1200/JCO.2018.77.8738
27. Du Y, Wang D, Liu M, Zhang X, Ren W, Sun J, et al. Study on the differential diagnosis of benign and Malignant breast lesions using a deep learning model based on multimodal images. J Cancer Res Ther. (2024) 20.2:625–32. doi: 10.4103/jcrt.jcrt_1796_23
28. Wong JZY, Chai JH, Yeoh YS, Mohamed Riza NK, Liu J, Teo YY, et al. Cost effectiveness analysis of a polygenic risk tailored breast cancer screening programme in Singapore. BMC Health Serv Res. (2021) 21.1:909–16. doi: 10.1186/s12913-021-06396-2
29. Schmidt G, Findeklee S, Del Sol Martinez G, Georgescu MT, Gerlinger C, Nemat S, et al. Accuracy of breast ultrasonography and mammography in comparison with postoperative histopathology in breast cancer patients after neoadjuvant chemotherapy. Diagnostics (Basel). (2023) 13:2811. doi: 10.3390/diagnostics13172811
30. Chen J, Huang Z, Luo H, Li G, Ding Z, Tian H, et al. Development and validation of nomograms using photoacoustic imaging and 2D ultrasound to predict breast nodule benignity and Malignancy. Postgrad Med J. (2024) 100:309–18. doi: 10.1093/postmj/qgad146
31. Schaefgen B, Mati M, Sinn HP, Golatta M, Stieber A, Rauch G, et al. Can routine imaging after neoadjuvant chemotherapy in breast cancer predict pathologic complete response? Ann Surg Oncol. (2016) 23:789–95. doi: 10.1245/s10434-015-4918-0
32. Rahmat K, Mumin NA, Hamid MTR, et al. MRI breast: current imaging trends, clinical applications, and future research directions[J. Curr Med Imaging. (2022) 18:1347–61. doi: 10.2174/1573405618666220415130131
33. Gültekin MA, Yabul FÇ, Temur HO, Sari L, Yilmaz TF, Toprak H, et al. Papillary lesions of the breast: addition of DWI and TIRM sequences to routine breast MRI could help in differentiation benign from Malignant. Curr Med Imaging. (2022) 18:962i. doi: 10.2174/1573405618666220218101931
34. Choi BB. Dynamic contrast enhanced-MRI and diffusion-weighted image as predictors of lymphovascular invasion in node-negative invasive breast cancer. World J Surg Oncol. (2021) 19:1–10. doi: 10.1186/s12957-021-02189-3
35. Kim JIH, Kim MJ, Yoon JH. Ultrafast dynamic contrast-enhanced breast MRI: Association with pathologic complete response in neoadjuvant treatment of breast cancer. Eur Radiol. (2022) 32.7:4823–33. doi: 10.1007/s00330-021-08530-4
36. Yamaguchi K, Nakazono T, Egashira R, Fukui S, Baba K, Hamamoto T, et al. Time to enhancement of breast lesions and normal breast parenchyma in light of menopausal status and menstrual cycle for ultrafast dynamic contrast-enhanced MRI using compressed sensing. Magnetic Resonance Imaging. (2023) 96:102–7. doi: 10.1016/j.mri.2022.11.006
37. Honda M, et al. Visual evaluation of ultrafast MRI in the assessment of residual breast cancer after neoadjuvant systemic therapy: A preliminary study association with subtype. Tomography. (2022) 8.3:1522–33. doi: 10.3390/tomography8030125
38. Ohashi A, Kataoka M, Iima M, Ota R, Ohashi A, Kishimoto AO, et al. Comparison of ultrafast dynamic contrast-enhanced (DCE) MRI with conventional DCE MRI in the morphological assessment of Malignant breast lesions. Diagnostics (Basel). (2023) 13:1105. doi: 10.3390/diagnostics13061105
39. Kataoka M, Honda M, Ohashi A, Yamaguchi K, Mori N, Goto M, et al. Ultrafast dynamic contrast-enhanced MRI of the breast: How is it used? Magnetic Resonance Med Sci. (2022) 21.1:83–94. doi: 10.2463/mrms.rev.2021-0157
40. Onishi N, Sadinski M, Hughes MC, Ko ES, Gibbs P, Gallagher KM, et al. Ultrafast dynamic contrast-enhanced breast MRI may generate prognostic imaging markers of breast cancer. Breast Cancer Res. (2020) 22:58. doi: 10.1186/s13058-020-01292-9
41. Ohashi A, Kataoka M, Iima M, Honda M, Ota R, Urushibata Y, et al. Prediction of Ki-67 expression of breast cancer with a multi-parametric model using MRI parameters from ultrafast DCE-MRI and DWI. In: 16th international workshop on breast imaging (IWBI2022), vol. 12286. Bellingham, Washington, USA: SPIE (2022) 12286:62–9.
42. Yamaguchi K, Nakazono T, Egashira R, Fukui S, Baba K, Hamamoto T, et al. Maximum slope of ultrafast dynamic contrast-enhanced MRI of the breast: Comparisons with prognostic factors of breast cancer. Japanese J Radiol. (2021) 39:246–53. doi: 10.1007/s11604-020-01049-6
43. Ayatollahi F, Shokouhi SB, Mann RM, Teuwen J. Automatic breast lesion detection in ultrafast DCE-MRI using deep learning. Med Phys. (2021) 48:5897–907. doi: 10.1002/mp.15156
44. Kuhl CK, Schrading S, Strobel K, Schild HH, Hilgers RD, Bieling HB. Abbreviated breast magnetic resonance imaging (MRI): first postcontrast subtracted images and maximum-intensity projection-a novel approach to breast cancer screening with MRI. J Clin Oncol. (2014) 32:2304–10. doi: 10.1200/JCO.2013.52.5386
45. Smith RA, Andrews KS, Brooks D, Fedewa SA, Manassaram-Baptiste D, Saslow D, et al. Cancer screening in the United States, 2018: A review of current American Cancer Society guidelines and current issues in cancer screening. CA Cancer J Clin. (2018) 68:297–316. doi: 10.1177/0969141314563632,25
46. Wilkerson AD, Obi M, Ortega C, Sebikali-Potts A, Wei W, Pederson HJ, et al. Correction: young black women may be more likely to have first mammogram cancers: A new perspective in breast cancer disparities. Ann Surg Oncol. (2023) 30:2175. doi: 10.1245/s10434-023-13111-4
47. Salameh JP, Kashif Al-Ghita M, McInnes MDF, Seely JM. Screening with breast cancer mammography: re-evaluation of current evidence. Can Assoc Radiol J. (2023) 74:596–9. doi: 10.1177/08465371221148134
48. Dan Q, Zheng T, Liu L, Sun D, Chen Y. Ultrasound for breast cancer screening in resource-limited settings: current practice and future directions. Cancers (Basel). (2023) 15:2112. doi: 10.3390/cancers15072112
Keywords: magnetic resonance imaging, ultrasound, differential diagnosis, breast cancer, breast tumors
Citation: Ma D, Wang C, Li J, Hao X, Zhu Y, Gao Z, Liu C, Luo C and Huang Y (2025) Analysis of the diagnostic efficacy of ultrasound, MRI, and combined examination in benign and malignant breast tumors. Front. Oncol. 15:1494862. doi: 10.3389/fonc.2025.1494862
Received: 11 September 2024; Accepted: 13 January 2025;
Published: 30 January 2025.
Edited by:
Sharon R. Pine, University of Colorado Anschutz Medical Campus, United StatesReviewed by:
Matteo Ferrante, University of Rome Tor Vergata, ItalyCopyright © 2025 Ma, Wang, Li, Hao, Zhu, Gao, Liu, Luo and Huang. This is an open-access article distributed under the terms of the Creative Commons Attribution License (CC BY). The use, distribution or reproduction in other forums is permitted, provided the original author(s) and the copyright owner(s) are credited and that the original publication in this journal is cited, in accordance with accepted academic practice. No use, distribution or reproduction is permitted which does not comply with these terms.
*Correspondence: Yu Huang, eXVodWFuZ0B1c3RjLmVkdS5jbg==
Disclaimer: All claims expressed in this article are solely those of the authors and do not necessarily represent those of their affiliated organizations, or those of the publisher, the editors and the reviewers. Any product that may be evaluated in this article or claim that may be made by its manufacturer is not guaranteed or endorsed by the publisher.
Research integrity at Frontiers
Learn more about the work of our research integrity team to safeguard the quality of each article we publish.