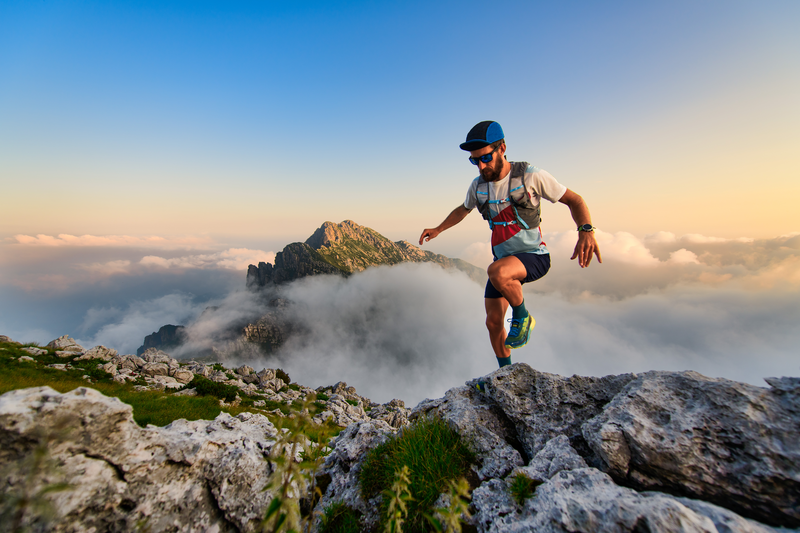
94% of researchers rate our articles as excellent or good
Learn more about the work of our research integrity team to safeguard the quality of each article we publish.
Find out more
ORIGINAL RESEARCH article
Front. Oncol.
Sec. Thoracic Oncology
Volume 15 - 2025 | doi: 10.3389/fonc.2025.1461530
This article is part of the Research Topic Advancing Precision Medicine in Lung Cancer: Integrating Genomics, Liquid Biopsy and Novel Diagnostic Tools View all 3 articles
The final, formatted version of the article will be published soon.
You have multiple emails registered with Frontiers:
Please enter your email address:
If you already have an account, please login
You don't have a Frontiers account ? You can register here
Purpose: JUNIPER, a randomized, phase III trial of patients with stage IV non–small cell lung cancer and a detectable Kristen rat sarcoma (KRAS) mutation in codons 12 or 13 whose condition progressed after platinum-based chemotherapy and up to 1 additional therapy (could include immune checkpoint inhibitors), reported prolonged progression-free survival (PFS) but not overall survival (OS) among patients who were receiving abemaciclib versus those who were receiving erlotinib. To establish whether certain patient subgroups received an OS benefit from the addition of abemaciclib to best supportive care, JUNIPER patients were retrospectively evaluated for KRAS co-mutation gene expression subtype, and abemaciclib efficacy was assessed for each patient subgroup.Materials and Methods: Of the 453 patients enrolled in the JUNIPER trial, tumor specimens for biomarker analysis were available for 148 (abemaciclib arm, n=79; erlotinib arm, n=69). Samples were profiled for gene expression and classified into 3 previously identified expression subtypes (KL, KP, and K). Tumor response, OS, and PFS were assessed within each subtype.Results: Retrospective analyses of expression subtypes revealed an OS advantage for patients with KL subtype tumors who were receiving abemaciclib versus those with the KL subtype who were receiving erlotinib (median, 13.05 vs 5.65 months; hazard ratio, 0.25; 95% confidence interval, 0.09–0.73; P=.011). KL and KP expression subtype groups derived a PFS benefit from abemaciclib versus erlotinib (KL median, 6.64 vs 2.1 months; hazard ratio, 0.12; 95% confidence interval, 0.03–0.41; P=.001, and KP median, 5.52 vs 2.24 months; hazard ratio, 0.44; 95% confidence interval, 0.23–0.84; P=.013). Patients with K subtype tumors received no OS or PFS benefit from abemaciclib treatment.Conclusions: Patients with KL expression subtype tumors may derive better OS and PFS from abemaciclib versus erlotinib in KRAS-mutated non–small cell lung cancer. These results should be further validated in an independent dataset.
Keywords: biomarkers, cdk4, Cdk6, KRAS, non-small cell lung cancer Clinical Trial registration: NCT02152631
Received: 08 Jul 2024; Accepted: 03 Mar 2025.
Copyright: © 2025 Liu, Wang, Aggarwal, Ortiz-Ruiz, Zapatero, Lallena, Peregrina, Martinez Del Hoyo, Velasco, Ebert, Gong, Hossain and Estrem. This is an open-access article distributed under the terms of the Creative Commons Attribution License (CC BY). The use, distribution or reproduction in other forums is permitted, provided the original author(s) or licensor are credited and that the original publication in this journal is cited, in accordance with accepted academic practice. No use, distribution or reproduction is permitted which does not comply with these terms.
* Correspondence:
Shawn Estrem, Lilly Research Laboratories, Eli Lilly (United States), Indianapolis, 46225, Indiana, United States
Disclaimer: All claims expressed in this article are solely those of the authors and do not necessarily represent those of their affiliated organizations, or those of the publisher, the editors and the reviewers. Any product that may be evaluated in this article or claim that may be made by its manufacturer is not guaranteed or endorsed by the publisher.
Research integrity at Frontiers
Learn more about the work of our research integrity team to safeguard the quality of each article we publish.