- 1Department of Radiation Oncology, National Cancer Center/National Clinical Research Center for Cancer/Cancer Hospital & Shenzhen Hospital, Chinese Academy of Medical Sciences and Peking Union Medical College, Shenzhen, China
- 2Department of Radiation Oncology, Faculty of Medicine, University of Tsukuba, Tsukuba, Ibaraki, Japan
- 3Department of Radiation Oncology, National Cancer Center/National Clinical Research Center for Cancer/Cancer Hospital, Chinese Academy of Medical Sciences and Peking Union Medical College, Beijing, China
Purpose: This study aimed to describe the trends, current hotspots, and future directions in boron neutron capture therapy (BNCT) through a bibliometric analysis.
Methods: Articles related to BNCT published before 2023-12-31 were retrieved from the Web of Science Core Collection database. VOSviewer, R, and CiteSpace were used for bibliometric analysis and visualization.
Results: A total of 3347 related publications from 1975 to 2023 were retrieved. Since a burst of published documents in 1992, the past three decades have witnessed continuous investigations into BNCT-related studies. Japan was the most productive country (794, 23.72%), followed by the USA (792, 23.66%), while the latter had the most citations. Kyoto University was the most influential institution. Ono K was the most prolific author, and Applied Radiation and Isotopes was the most popular journal. Ono K was the author that had the most total citations, followed by Barth RF. “Carborane”, “boronophenylalanine”, “glioblastoma”, “sodium borocaptate”, “cancer” and “drug delivery” were the most frequent keywords. The article “Dendrimers and dendritic polymers in drug delivery” had the most citations, whereas “Boron delivery agents for neutron capture therapy of cancer” had the highest outbreak value.
Conclusion: Over the past three decades, research on BNCT has expanded significantly, with the development of novel boron carriers with improved medicinal characteristics being the most extensively investigated area. Future research will likely focus on the validation and modification of current BNCT treatment modalities using conventional boron agents in brain tumors, accelerator-based neutron sources and the application of BNCT in more clinical scenarios.
1 Introduction
Cancer remains one of the most devastating diseases affecting humans. In 2022, it was responsible for approximately one in six deaths globally, with an estimated 9.7 million fatalities (1). A major hinderance to the definitive cure of cancer is the therapeutic ratio, which has yet to increase owing to the inherent limitations of current treatment modalities. With respect to oncological surgery, the established principle of securing wide resection margins undoubtedly improved patient outcomes; however, a few residual tumor cells beyond the resection margins can lead to recurrence and metastasis (2). Systemic therapies can effectively eliminate disseminated malignant cells, but their side effects are common and sometimes lethal (3, 4). In the field of radiation therapy (RT), considerable effort has been devoted to the precise delivery of planned ionization radiation to the designed target volume and nowhere else (5, 6). Nonetheless, unintended doses to adjacent vulnerable organs at risk (OARs) limit its application to post-RT recurrences or tumors in critical locations.
Boron neutron capture therapy (BNCT), in contrast, is a unique two-step radiotherapy featuring unprecedented precision (Figure 1). In the first step, the nonradioactive isotope boron-10 (10B)-containing agent is administered and enriched in tumor cells. Second, with the selectively accumulated 10B, slow “thermal” neutrons radiated to the target volume induce neutron capture and decay reactions within a cell range (7). In this double-targeted manner, tumor cells, even those entangled with normal cells, receive a relatively high dose of irradiation and are thus susceptible to physical and biological destruction. Sparing nonmalignant components when killing tumors at a cellular resolution is a characteristic of BNCT that makes it a promising remedy for traditionally difficult-to-treat tumors.
Bibliometrics, first debuted in 1987, is a powerful tool for analyzing publication characteristics, canonical articles, and research trends in a specific field (8). By collecting most, if not all, related publications and utilizing statistical algorithms, bibliometric analysis could provide objective and comprehensive insights into the research area compared with conventional reviews that are dependent on the authors’ viewpoint. However, this useful tool has not been applied to BNCT research. Therefore, we conducted this study to fill this gap, aiming at describing the entire body of knowledge and helping researchers interested in BNCT grasp the whole picture of the past, the present and the possible future.
2 Methods
2.1 Data acquisition
The following query line was used to retrieve publications from the Web of Science core collection (WoSCC): TS= (boron neutron capture therapy) AND LA= (English) AND DT= (Article OR Review). Related articles and reviews in English published before 2023-12-31 were collected. The search results were exported in plaintext format. Two authors independently conducted the literature screening, data retrieval and analysis to reduce bias in the results. The workflow is shown in Figure 2.
2.2 Bibliometrics and visualization analysis
VOSviewer 1.6.20), R (4.4.1), and CiteSpace (6.3. R1) were used in this study. VOSviewer was used to analyze the statistical characteristics of countries, institutions, journals, authors and keywords. CiteSpace was used to identify bursts of keywords and citations. Bibliometrix, a built-in R package, was used for data visualization (9).
3 Results
3.1 Publication characteristics
In total, 3347 publications related to BNCT were retrieved from the WoSCC (see Table 1). The annual publication numbers from 1975 to 2023 are shown in Figure 3A. We observed a surge of publications in 1992, followed by a 2.73% average annual growth rate, indicating that the attention attracted to BNCT research steadily increased. The year that had the most publications was 2023 (220, 6.57%). The total number of published articles steadily and rapidly increased from 1975 to 2023 (Figure 3B). From 1992 to 2021, the annual total number of citations was greater (Figure 3C), and the annual h index was 15 or greater, while it declined afterward (Figure 3D).
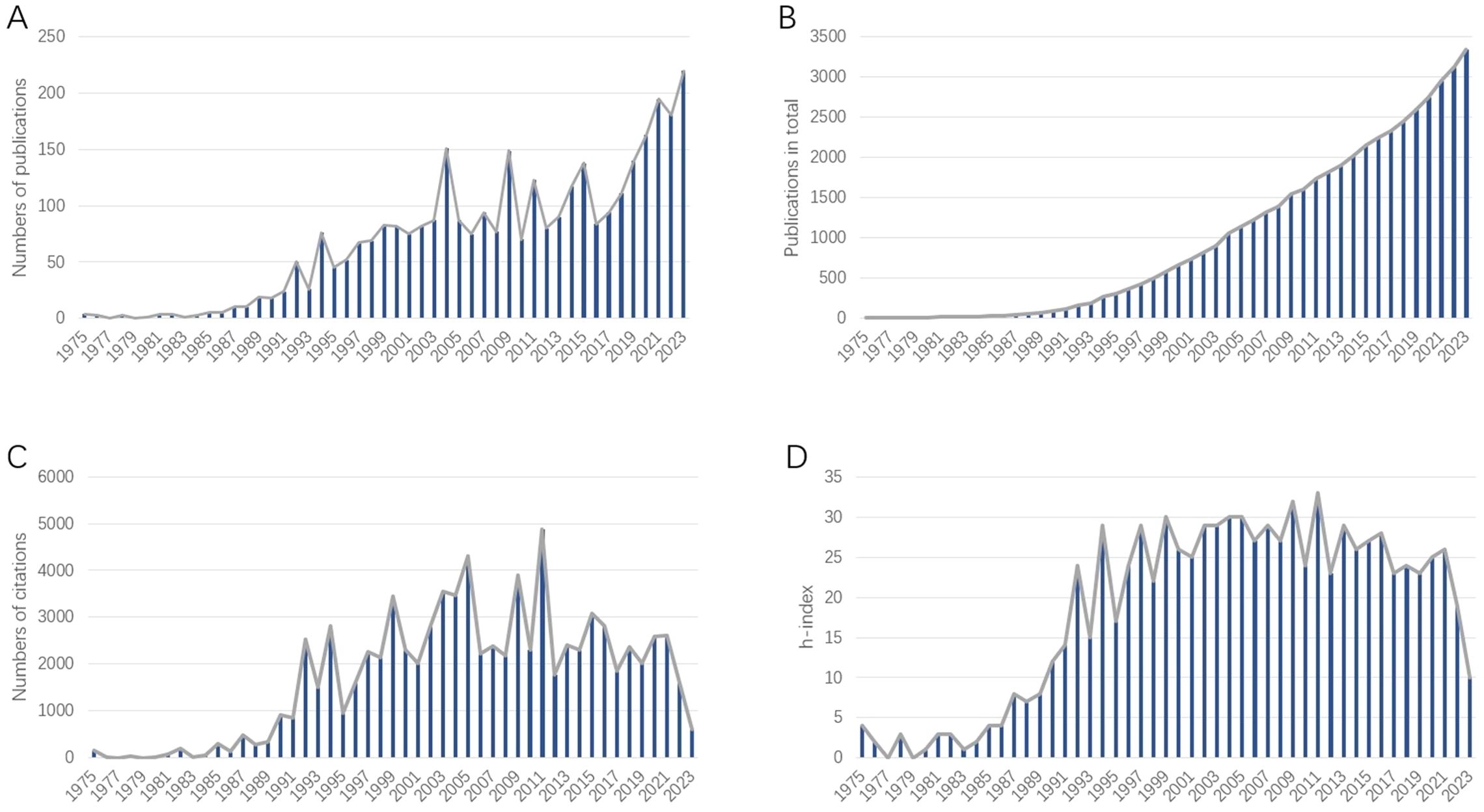
Figure 3. (A) Annual publications related to BNCT research. (B) Cumulative publications related to BNCT research. (C) Annual citations of the publications related to BNCT research. (D) Annual h-index of BNCT publications.
3.2 Countries/regions and institutions
A total of 79 counties/regions have contributed to research related to BNCT. The 38 countries/regions with more than 10 publications are shown in Figure 4A. The overlay network analysis of coauthorship reflects the number of publications by circle size and the average commencement year of study by color. In this two-dimensional diagram, strongly related nodes are located close to each other while weakly related nodes are located far away from each other. The link strength (LS) between nodes reflects the cooperation between countries, and the total link strength (TLS) is the sum of the LSs of a certain node. The USA had the strongest global cooperation (TLS=383) and cooperated the most with Argentina (LS=49). The top ten countries/regions with the highest number of publications and total citations are presented in Table 2. Japan had the most publications (794, 23.72%), followed by the USA (792, 23.66%) and Russia (274, 8.19%). Notably, the USA had the highest number of citations, followed by Japan. Figure 4B shows the geographic distribution of BNCT publications and cooperation strengths.
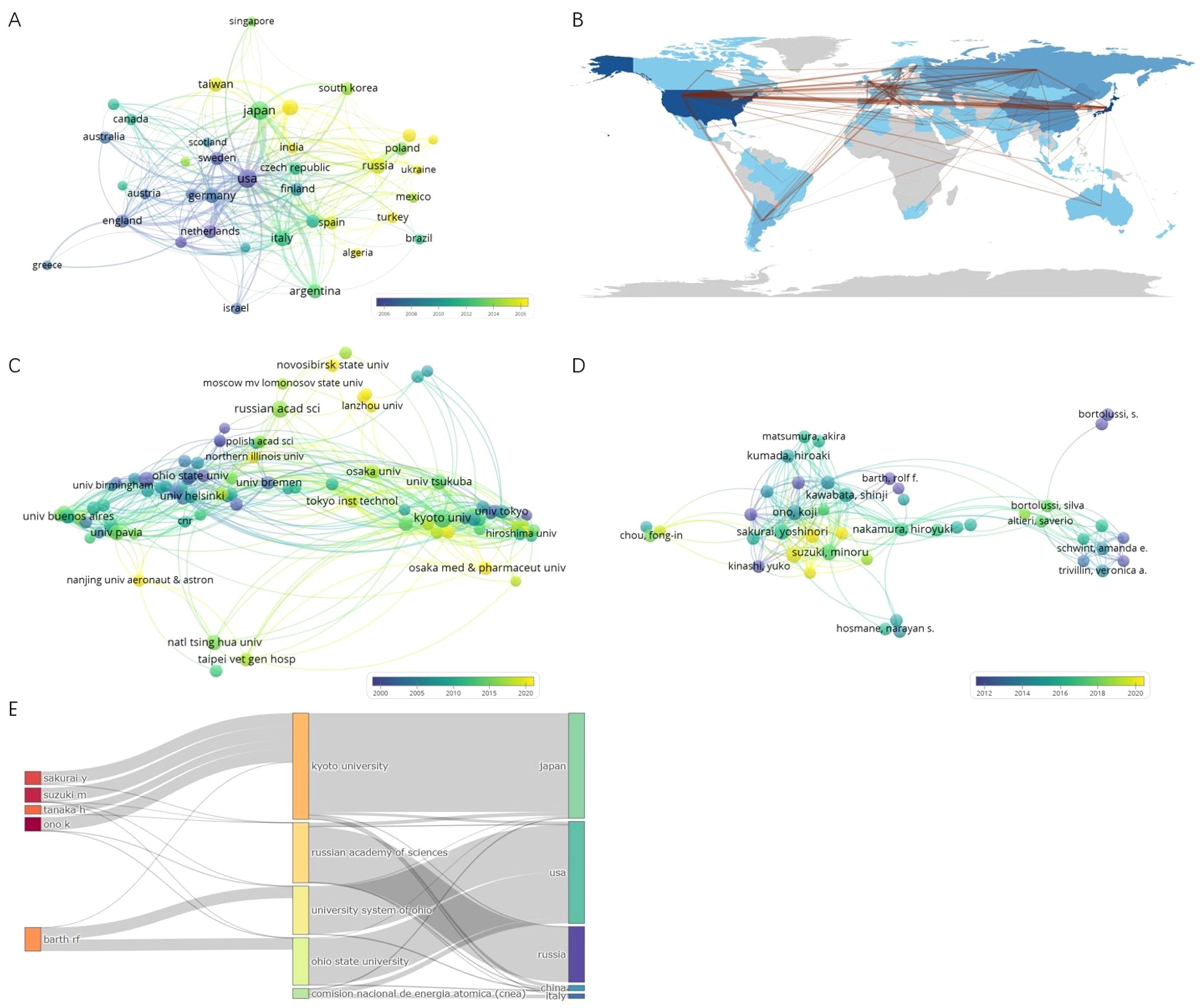
Figure 4. (A) Coauthorship overlay of countries/regions. (B) Geographic distribution of BNCT publications and cooperation strengths. (C) Coauthorship overlay of institutions. (D) Coauthorship overlay of authors. (E) Three fields plot of authors, affiliations and countries/regions.
In total, 2234 organizations have participated in BNCT research. Figure 4C shows 148 institutions with more than 10 publications. Kyoto University had the most cooperative relationships (TLS=624). The top 10 most productive institutions are listed in Table 3. Kyoto University was the most productive (355, 10.61%), followed by the Russian Academy of Sciences (186, 5.56%) and Ohio State University (138, 4.12%). Ohio State University had the highest number of total citations (7873). Figure 4C shows that in the past 30 years, the Massachusetts Institute of Technology (MIT), Brookhaven National Laboratory, and Ohio State University conducted BNCT research earlier, whereas Kyoto University, the Russian Academy of Sciences and the University of Tsukuba commenced later.
3.3 Authors
A total of 8,574 authors have contributed to publications on BNCT. The top 10 authors with the most publications are shown in Table 4. Ono K had the highest efficiency (191, 5.71%), followed by Suzuki M (187, 5.59%) and Sakurai Y (163, 4.87%). Ono K had the highest total number of citations, whereas Barth RF had the highest h-index. Figure 4D shows a map of overlay network analysis among 236 researchers of 10 articles or more. Ono K cooperated the most with the others (TLS=1314). Figure 4E shows the correlations among the five most prolific authors, institutions, and countries that have contributed to BNCT research from 1975 to 2023 via a Sankey diagram (10).
3.4 Journals
A total of 687 journals have published articles related to BNCT. The top 10 journals in terms of BNCT publications are listed in Table 5, along with their publication counts, total citations, average citations, impact factor (IF) and Journal Citation Reports (JCR) quantile rankings. Applied Radiation and Isotopes had the most publications, followed by Medical Physics and Nuclear Instruments and Methods in Physics Research Section A. Applied Radiation and Isotopes had the highest total number of citations (5116), whereas the Journal of Neuro-Oncology had the highest average number of citations (50.49). The International Journal of Radiation Oncology Biology Physics had the highest IF (6.4), followed by Physics in Medicine & Biology (3.3) and the Journal of Neuro-Oncology (3.2). Most journals were classified as Q2 and above (90%) by JCR ranking quantiles. Journal directions included nuclear medicine, radiotherapy, oncology, and clinical neurology. A map of the cocitation network analysis is shown in Figure 5A. The top 3 cocited journals were Applied Radiation and Isotopes (4627), the Journal of the American Chemical Society (3286), and the International Journal of Radiation Oncology, Biology, Physics (3086).
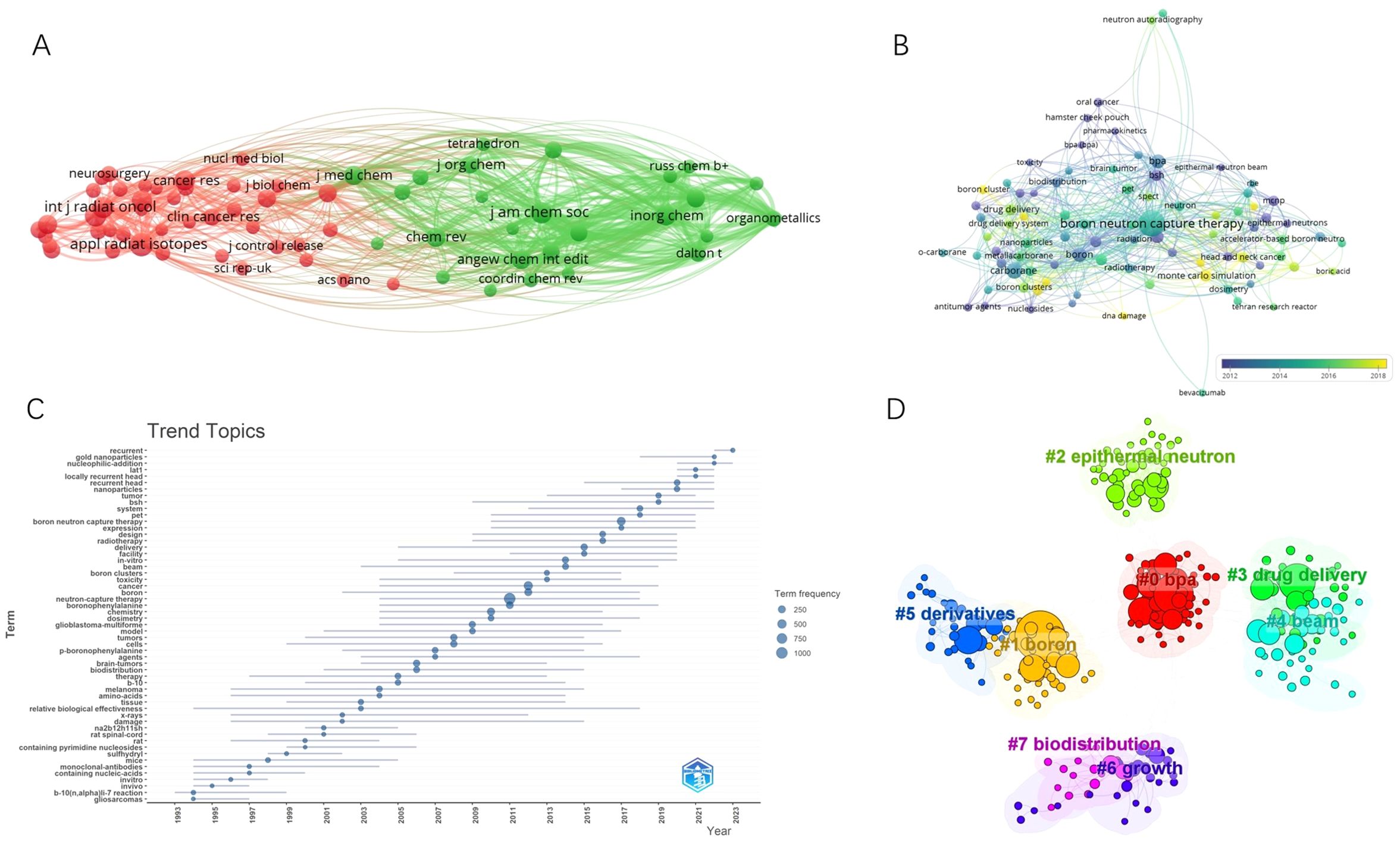
Figure 5. (A) Cocitation network map of journals. (B) Keyword co-occurrence network diagram of BNCT. (C) The trending topics. (D) Eight representative clusters of keyword clusters from 2019 to 2023.
3.5 Citation and cocitation analysis
Citation analysis is a valuable way to evaluate the most cited articles, and the number of citations can reflect the impact of an article in a particular field of research. The most cited articles by year in the realm of BNCT from 1975 to 2023 are listed in Supplementary Table 1. Among the 47 publications, 20 appeared in journals focused on organic and inorganic chemistry, 15 in journals dedicated to oncology and radiotherapy research, 7 in journals related to medicinal studies, and 5 in journals covering physics. Additionally, 30 of the 47 studies concentrated on the synthesis and properties of new boron-containing compounds, 10 on BNCT for cancer treatment, 5 on dosimetry, and 2 on the biological effects. In Supplementary Table 2, we summarize the 100 most cited articles in this field. The article “Dendrimers and Dendritic Polymers in Drug Delivery”, published in Drug Discovery Today in 2005, has received the highest citation count (1158).
3.6 Keywords
In total, 5181 keywords were proposed by the authors. A map of the overlay network analysis of keywords is shown in Figure 5B. The top 10 most common keywords were “BNCT”, “carborane”, “boronophenylalanine (BPA)”, “boron”, “glioblastoma (GBM)”, “sodium borocaptate (BSH)”, “cancer”, “drug delivery”, “neutron capture therapy”, and “Monte Carlo”. The terms marked in dark blue represent an average publication year of 2008 or earlier, whereas those in bright yellow represent 2016 or later. “BSH”, “GBM”, and “radiation” were previously the main topics. The keywords “Monte Carlo simulation”, “accelerator-based neutron source”, “cytotoxicity”, “drug delivery”, “head and neck cancer”, and “boron cluster” appeared relatively late. A similar trend is shown in Figure 5C.
To obtain the latest research hotspots of BNCT research, we analyzed the clustering of keywords within 5 years. Eight major clusters are shown in Figure 5D with their sizes and silhouette values in Supplementary Table 3. On the basis of clustering vocabulary analysis, the latest BNCT studies have focused on BPA administration, sources of neutrons, synthesis of novel boron-containing agents, their biodistribution, and the influence on tumor growth in animal models.
3.7 Citation bursts
The top 25 keywords with citation bursts are shown in Figure 6A. The red lines reflect the burst duration. The keywords “mouse” (1990-2000) and “malignant melanoma” (1990-2000) drew considerable attention at the end of the 20th century, whereas “glioma” (1996-2005), “glioblastoma multiforme” (1999-2004), “brain tumors” (2000-2008), and “BSH synonyms” (1993-2005) suggested a surge of publications related to the clinical outcomes of BNCT in the treatment of brain tumors using BSH as a boron carrier. In the second decade of the 21st century, “recurrent head” (2013-2023), “drug delivery” (2015-2021), “nanoparticles” (2017-2023), and “Monte Carlo simulation” (2017-2023) have attracted extensive attention. The top 25 references with burst citations are shown in Figure 6B. The article “Boron delivery agents for neutron capture therapy of cancer” published by RF Barth et al. in Cancer Communications had the strongest outbreak, and the burst is still ongoing. The review “The Chemistry of Neutron Capture Therapy” by Soloway AH et al. had the second highest burst strength.
3.8 Trends of contribution
The production of top 5 countries, authors, affiliations, and journals over time is shown in Figure 7, respectively. As demonstrated in Figure 7A, the cumulate contribution of Japan surpassed that of USA in 2015, while China’s production exceeded Italy’s in 2021. In the last five years, the most prolific authors are Suzuki M, Tanaka H, and Sakurai Y from Japan, while Kyoto University and Russian academy of sciences are the most active institutions worldwide (Figures 7B, C). Top five journals of BNCT research are shown in Figure 7D. From late 2000s, the journal Applied Radiation and Isotopes has been the leading source of BNCT related articles.
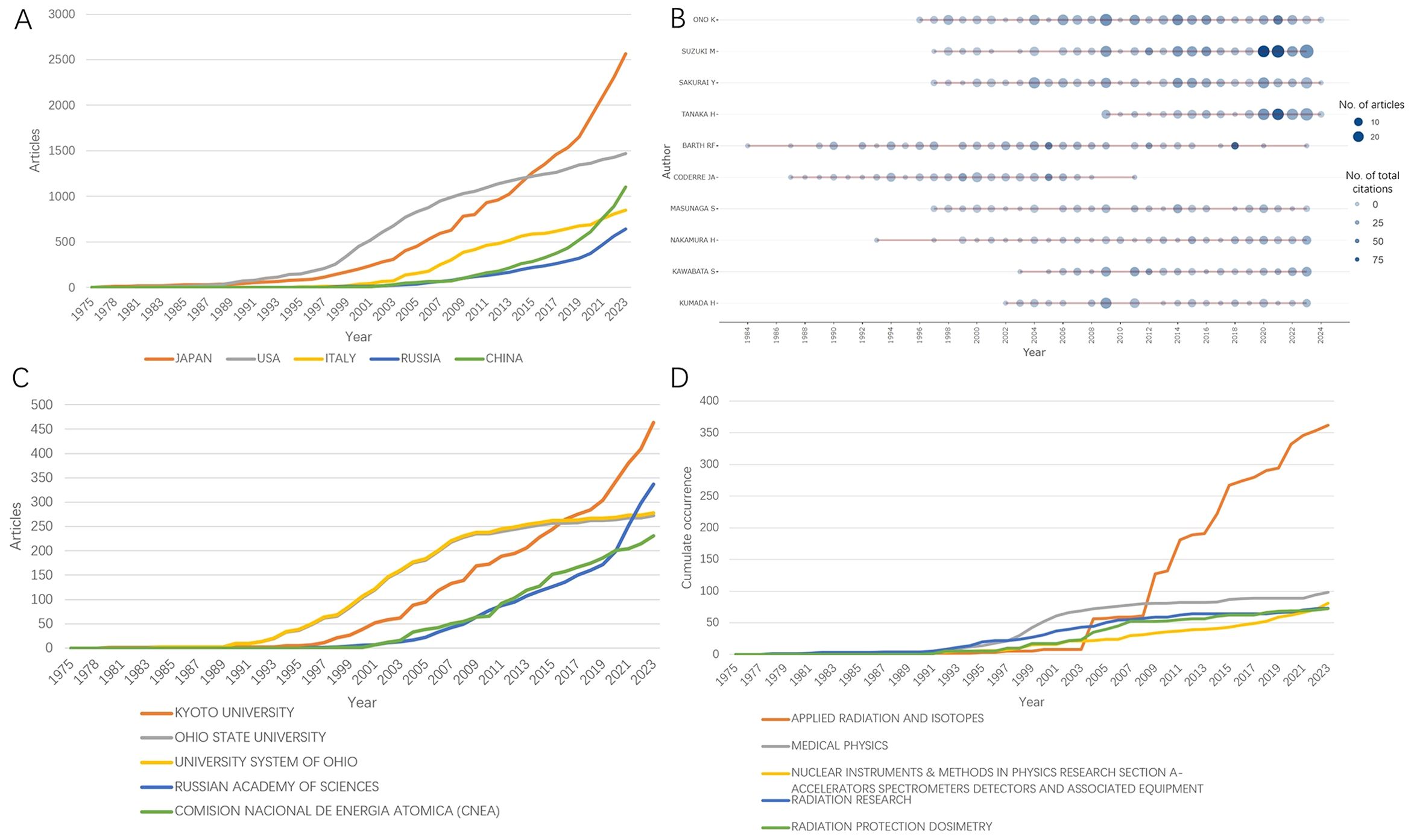
Figure 7. The production of top 5 (A) countries, (B) authors, (C) affiliations, and (D) journals over time.
4 Discussion
4.1 Trends in the development of BNCT-related research
To date, this is the first study to offer a comprehensive bibliometric analysis of BNCT-related publications in the WOSCC from 1975 to 2023. The findings show a consistent increase in scientific output in this field. The peak number of citations and the highest H-index occurred in 2011. Japan, China, and Russia are the top three most productive countries in the recent five years. The decrease in citations and the h-index since 2022 may be attributed to the proximity to the end of the collection period.
4.2 Contributions by countries, institutions, authors, and journals
We conducted this bibliometric analysis in the field of BNCT to objectively identify the most influential countries, institutions, authors, and journals. Japan has emerged as a major contributor with the highest number of publications, whereas the USA has led in citations and international collaboration. Notably, three of the top ten institutions and eight of the top ten most productive authors are Japanese. Kyoto University was the most influential institution, and Ono K was the most prolific author over the past 30 years. The journal Applied Radiation and Isotopes had the most publications and total citations. Additionally, our results indicated that the Journal of Neuro-Oncology had the highest number of citations per publication in this field, suggesting that clinical applications of BNCT for GBMs continue to attract the attention of physicians and researchers.
4.3 Research hotspots and frontiers
To find research hotspots and leading edges in the field of BNCT, we conducted an analysis of citations, cocitations, and keywords. To our knowledge, this study is the only bibliometric analysis of this topic, with several meaningful conclusions. First, the synthesis of novel boron-containing agents is a constant hotspot, varying from boronic acid derivatives lacking selectivity to boron clusters such as carboranes and then to modern boron carriers with targeting capacity. Antibody conjugates and nanoparticles are becoming more attractive to researchers. Our keyword analysis results indicate that researchers have paid increasing attention to “accelerator-based neutron source”, “cytotoxicity”, “drug delivery”, “head and neck cancer”, and “boron cluster”, with the aim of improving the neuron source, boron delivery and number of types of cancer to treat with BNCT. In contrast, previous studies focused more on BSH and GBMs. Third, the burst analysis of keywords and citations revealed “brain tumors”, “nanoparticles”, and “Monte Carlo simulation”, suggesting that they are milestones in the development of BNCT research. Finally, with the addition of cutting-edge publications, we sense prelusive therapeutic advancements using novel boron agents, with applications unrestricted to small animals in the near future.
4.3.1 Development of neutron sources
Ideal neutrons for BNCT should be radiated at high flux rates, designated energy levels and targeted volumes without unwanted heavy particles. Although the characteristics and purity of the neutrons were subpar with respect to modern standards, experimental nuclear reactors provided the first thermal neutrons that contributed to the proof of concept of BNCT. One decade after World War II, Farr LE and Sweet WH used the Massachusetts Institute of Technology nuclear reactor (MITR) as neutron sources (11). In the 1960s, Hatanaka initiated clinical trials on brain malignancies via the Hitachi Training Reactor and the Musashi Institute of Technology reactor (12). As mentioned above, the results of these trials were far from fruitful. The slow neutrons had a critical disadvantage. Owing to their low energy level, thermal neutrons can only pass through an average of 2 cm in the tissue before they lose the capacity to induce a nuclear reaction. This resulted in intracranial tumor patients often needing debulking resection and the surgical cavity being left open when receiving the external beam, which might have led to brain necrosis by increasing the boron concentration at exposed incisions (13). In addition, the short path length of thermal neutrons has limited the noninvasive application of BNCT to melanomas and other superficial neoplasms.
Reactors remained the mainstream neutron source until the early 21st century as the physical characterization of their neutrons improved. The epithermal neutrons from MITR II and Brookhaven Medical Research Reactor (BMRR) with modified beam parameters came into clinical use for melanoma and GBM patients in the 1990s (14, 15). The relatively high-energy epithermal neutrons (0.5 eV < En < 10 keV) from reactors had better penetration of approximately 6 cm beneath the tissue surface, comparing to 2 cm for thermal (En < 0.5 eV) neutrons, which significantly increased the body volume in reach and made craniotomy unnecessary (14, 16). However, several limitations of reactor-based BNCT exist. First, the rareness of a nuclear reactor is a major impediment to BNCT research. The technical requirements and financial burdens of building and maintaining a nuclear reactor dwarf the benefits of this promising solution as definitive precision medicine. In addition, the reactors need to be shared with basic physics researchers and shut down for maintenance for one third of a whole year (17). Finally, nuclear accidents are always a concern.
Alternative neutron sources have been under development since the 1980s (18). Accelerators at that time for physical research had a neutron density far less than the requirement of BNCT, which is more than 1×1011 to 1012 (n/cm2·s) (19). In recent years, accelerator-based (AB) systems have provided comparable intensities of epithermal neutrons with the whole system in a compact form suitable for in-hospital installation (20). In short, protons are accelerated by cyclotrons (21) or linear accelerators (22) to hit the target metal materials, such as lithium (23) or beryllium (24). Nuclear reactions, e.g., 7Li(p, n)7Be or 9Be(p, n)9B, occur, and then, epithermal neutrons are emitted from the target. By 2023, AB-BNCT has become standard clinical therapy covered by medical insurance in 5 hospitals in Japan (25). Currently, several clinical studies in China (26) and Korea (27) in which several manufacturers use accelerator-based neutron sources (ABNSs) are in progress. The clinical application of ABNS in the new era warrants further investigation.
4.3.2 Development of boron delivery agents
The development of boron carriers has spanned over 70 years and remains critical to the success of BNCT. The general requirements for boron agents include low systemic toxicity, high selectivity, and durable persistence in tumors during BNCT (28). The challenge lies in achieving at least 20 μg/g 10B in tumors for effective radiation, while less than one-third of the boron is present in blood or normal tissue. Advances in synthetic techniques and biological targeting have led to the emergence of several promising boron delivery strategies.
The first boron carrier used in early BNCT studies was borax. In Farr’s procedure, sodium tetraborate was infused intravenously, followed by 30 min of irradiation of given neutron counts at the skin surface, and a few derivatives of it were used in subsequent trials (11, 29). These first-generation compounds have poor selectivity, a low tumor tissue ratio, short persistence in brain tumors and high systemic toxicity (29). BSH (30) and BPA (31) are second-generation boron agents that are more selective and have been widely used in clinical trials. BSH is a polyhedral borane anion commonly used in Japanese clinical trials. The EORTC 11961 study revealed that the tumor-to-brain ratio of BSH was greater than 40 in GBM patients (32). BPA is a hydrophobic dihydroxyboryl derivative of phenylalanine that can be actively transported into cells via the L-amino acid transport system and is upregulated in most tumors (33). The safety of intravenous administration of BSH and BPA has been clinically tested in Japan, the USA, Europe, and Argentina (34), although neither meets all of the requirements. The main limitations of BSH are its lack of receptor-mediated tumor selectivity, low tumor-to-blood ratio, and significant side effects during treatment (35). While some researchers have addressed this by conjugating BSH with specific ligands (36, 37), its high cost remains a barrier to its use as an ideal boron carrier. The low boron content of BPA requires high doses, increasing treatment costs and straining liver and kidney metabolism. Its poor solubility and instability lead to a reduced boron concentration at the tumor site (38), whereas its short retention time, possibly influenced by LAT1’s anti-transport mechanism, further limits its effectiveness.
The next generation of boron compounds features the conjugation of boron agents to tumor-targeting molecules to achieve the selective accumulation of boron in tumors. The targeting relies on the binding of linked ligands to corresponding receptors that are overexpressed by tumor cells. These novel boron agents generally consist of a stable boron cluster or moiety of high boron concentration and tumor-targeting molecules linked together. Amino acids, peptides, carbohydrates, nucleosides, antibodies, and nanoparticles as targeting or carrier moieties have attracted considerable attention.
Low-molecular-weight agents as targeting moieties. In addition to BPA, boronated amino acids, along with their derivatives, have been widely investigated. These include natural amino acids and unnatural cyclic amino acids (39, 40). However, none of these compounds possess better in vitro characteristics than BPA derivatives do (35). Peptide ligands have been demonstrated through the functionalization of carriers with high affinity and selectivity (41). Michiue et al. reported that when connected to arginine repeats, BSH has increased intracellular accumulation in both the cytoplasm and nucleus (42). In vivo positron emission tomography (PET) confirmed the selective retention of BSH-3R in tumor tissue. Nagasawa et al. developed and evaluated a novel boron carrier by conjugating BSH to cell-membrane penetrating peptides (CPPs) (43). Compared with BSH, the CPP-conjugated form presented a greater intracellular concentration and better eradication of T98G cells when exposed to neutrons. Barth’s group proposed a strategy to target vascular endothelial growth factor receptor (VEGFR) and epidermal growth factor receptor (EGFR), which are often overexpressed in tumors (44, 45). However, indirect tumoricidal activity via ischemic necrosis and the expression of EGFR on normal glial cells have limited their success.
Cancer cells have an increased rate of glucose uptake and glycolysis, known as the Warburg effect (46), which renders carbohydrates an ideal ligand for boron conjugates. Aoki et al. synthesized highly hydrophilic 2-borylsugars that were actively transported into cancer cells by glucose transporter 1 (GLUT1). Then, 2-borylsugar is phosphorylated and stored intracellularly, as confirmed by in vitro assays (47). Ekholm et al. developed 6-O-carboranylmethyl glycoconjugates with high affinities for GLUT1 (48). Compared with second-generation boron agents, this compound has a 40-fold greater boron delivery capacity. Tsurubuchi et al. reported the synthesis of an α-D-mannopyranoside derivative named MMT1242 with prolonged intracellular retention compared with that of BPA (49). Notably, both mannose receptors (MRs) and GLUT1 mediate the uptake of MMT1242. Given that MRs are upregulated on the cell membranes of tumors and correlate with tumorigenesis (50), mannose conjugates hold potential for improving the therapeutic effects of BNCT. In summary, despite their high water solubility and rapid clearance from metabolically active tumor cells, leading to poor intracellular boron retention, these studies underscore the potential of carbohydrate-based boron carriers. Their enhanced uptake, high tumor affinity, and growth inhibition offer valuable insights for developing new boron agents for BNCT.
Other small molecules, such as nucleosides, porphyrins, folic acid (FA), hyaluronic acid (HA), and COX-2 substrates, have also been widely investigated in recent years. Thymidine kinase 1 (TK1) is highly expressed in the cytosol of proliferating tumor cells (51). To target TK1, Barth et al. synthesized a 3-carboranyl thymidine analog (3CTA), N5–2OH, with high tumor-to-brain and tumor-to-blood ratios (52). In vivo studies demonstrated a significantly prolonged mean survival time (MST) in rats bearing RG2 gliomas, whereas validation of N5–2OH in an F98 glioma model revealed minimal MST improvement (53). The underlying cause of this phenomenon needs further investigation. FA receptors are highly expressed in tumor cell lines and are correlated with a metastatic tendency (54, 55). In 2020, Nakagawa’s group synthesized several hydrophilic FA derivatives with IC50 values of less than 3 mM in the U87 MG glioma cell line (56). The HA receptor CD44 is upregulated in cancers of numerous origins (57). In 2008, Crescenzi’s group designed an HA-conjugated carborane, named HapACB, with high affinity for CD44 in vitro (58). COX-2 is highly expressed in oral squamous carcinoma cells (59). Boronated COX-2 inhibitors demonstrated great radiosensitization capacity in CAL27 cells by suppressing the PI3K/Akt and MAPK signaling pathways (60). The conjugation of boron-enriched moieties with well-validated targeting molecules might be a productive avenue in the future.
High-molecular-weight agents as targeting moieties. Monoclonal antibodies possess unparalleled targeting selectivity, and antibody-based therapies are achieving significant clinical success across various cancers (61). With respect to BNCT, the first antibody-assisted localization was achieved by conjugating benzenediazonium ions to antibodies against carcinoembryonic antigen (CEA) in 1984 (62). This conjugate contained 30 boron atoms per IgG molecule and demonstrated a high degree of selective accumulation in CEA-positive human colonic carcinomas grown in hamsters. Recently, EGFR and CD133 have been investigated as targets for antibody conjugates. Barth and colleagues developed dendrimers with high boron concentrations linked to cetuximab, a widely used monoclonal antibody that targets EGFR (63); or to L8A4, which targets EGFRvIII (64); or to EGF (45) per se. These EGFR-binding boron agents demonstrated significant tumoricidal effects in a rat model bearing F98 gliomas transfected with the corresponding genes (63–66). Nakase’s group also targeted EGFR via the conjugation of dodecaborate to cetuximab through the Z33 peptide (67). EGFR-expressing cells internalize the conjugates via the macropinocytotic pathway, but further validation of BNCT therapeutics involving this compound is necessary. Sun’s group reported that with CD133 on SU2 glioma cells targeted by boron−antibody conjugates, the elongation of experimental mouse survival time was remarkable (68). To date, the key challenges of antibody-based boron delivery strategies include (1) coping with variable expression of the target across tumors (2); elucidating the mechanisms for entering cancerous cells; and (3) achieving uniform boron distribution or selective enrichment within critical compartments of the tumor.
As the burst analysis indicated, extensive investigations have recently been conducted on the use of nanocarriers for boron delivery. Nanoparticles are materials with specialized functions formed by atoms or molecules at the nanoscale. Recent progress in materials chemistry, biology, and related areas has rapidly advanced the use of nanoparticles in fields such as industry and medicine (69, 70). These nanoparticle-based systems have demonstrated numerous advantages in drug delivery, e.g., high stability, water solubility, tumor accumulation, and low preparation requirements (71), due to the selective accumulation of nanoparticles in tumors, known as the enhanced permeability and retention (EPR) effect (72). Additionally, nanocarriers can easily deliver other therapeutic agents alongside boron compounds to tumors. As a result, nanostructures have emerged as a focus of BNCT research.
Polymers represent a prominent class of nanocarriers that have been extensively studied in recent years, such as dendrimers (73) and polymer micelles (74), which have the ability to extend drug retention in tumors and enhance the hydrophilicity of drugs. The boron-containing compounds are either conjugated to or encapsulated by the polymers. In 2012, Sumitani et al. reported significant suppression of colon-26 tumor (CT26) growth in mice treated with carboranes embedded by their poly(ethylene glycol)-block-poly(lactide) copolymer (PEG-b-PLA) (75). Five years later, Makino and coworkers reported that poly(L-lactide-co-glycolide) (PLLGA)-encapsulated carboranes maintained a tumor-to-blood ratio of boron concentration over 5 for more than 8 hours after administration (76). In 2019, Chen’s group developed a polymer-based tumor-targeting boron delivery system, named iRGD-PEG-PCCL-B (77). When wrapped by this polymer, BSH had six times higher uptake by A549 cells than its original form. In addition, iRGD-PEG-PCCL-B could simultaneously pack the BSH with doxorubicin, enabling highly targeted BNCT combined with chemotherapy for synergistic tumor suppression. A year later, Nomoto and colleagues prepared a novel polyvinyl alcohol-BPA complex that demonstrated improved uptake mediated by LAT1, prolonged cellular retention, and substantial CT26 growth suppression with high biocompatibility (78). In 2023, Dai et al. synthesized novel BPA-containing polydopamine (B-PDA) nanoparticles that had significant glioma ablation capacity in mice exposed to neutron radiation, with a tumor-to-brain ratio of 6.83 ± 1.75 (79). Overall, the use of polymers as boron carriers has provided substantial preclinical evidence, demonstrating their potential as promising candidates for further investigations.
Liposomes and inorganic boron-containing nanoparticles have undergone extensive investigation, as examined in two recent reviews (80, 81). These inorganic nanoparticles can be classified into boron nitride (82, 83) (BN) nanotubes (84–86), boron-doped carbon dots (87), magnetic nanomaterials (88, 89), gold nanoparticles (90, 91), and mesoporous silica nanoparticles (92, 93), although a more detailed review is beyond the scope of this study. Owing to their high biocompatibility, low systemic toxicity, improved physiochemical stability, controlled release and EPR effect, the majority of these multifunctional nanocomposites demonstrated improved selectivity for tumor cells and increased boron uptake in both in vivo and in vitro experiments through ligand conjugations, surface modifications or structural alterations, rendering these categories of nanocarriers promising blueprints for innovative boron delivery materials.
4.3.3 Enhanced imaging and dosimetry
Traditional imaging techniques have offered limited insight into the distribution of boron compounds and the dose delivered to tissues. To analyze the boron concentration, various methods are available, including colorimetry, prompt γ-ray analysis (PGRA), inductively coupled plasma atomic emission spectrometry (ICP−AES), direct-current plasma atomic emission spectroscopy (DCP-AES), inductively coupled plasma−mass spectrometry (ICP-MS), quantitative neutron capture radiography (QNCR), electron energy loss spectroscopy (EELS), flow injection combined with ESI-MS/MS (FI/ESI-MS/MS), sputter-initiated resonance ionization microprobe (SIRIMP), laser atomization resonance ionization microprobe (LARIMP), secondary ion mass spectrometry (SIMS), single-photon emission computed tomography (SPECT), PET, nuclear magnetic resonance (NMR), and magnetic resonance imaging (MRI). All of the current boron drugs and their imaging techniques are presented in Table 6.
Currently, not all of these technologies are utilized in clinical settings. Many remain at the cellular level or in research stages, such as QNCR and SIRIMP, whereas newer methods such as nano-SIMS require additional development before clinical use. Presently, four primary methods are employed in clinical practice, all of which are based on modern imaging techniques: positron emission tomography (PET) and magnetic resonance imaging (MRI) provide enhanced visualization of the boron distribution and tumor response. Additionally, advanced dosimetry tools now enable precise measurement of radiation doses, ensuring accurate treatment planning.
4.3.4 Clinical advances
Early clinical trials had mixed results due to technology and compound limitations. More recent clinical trials have demonstrated promising results, particularly with new boron compounds and improved neutron sources. Trials are exploring the effectiveness of BNCT for various cancers, including those resistant to conventional therapies. The evidence suggests better outcomes and fewer side effects in some cases.
Glioblastoma multiforme: The use of BNCT for treating brain gliomas can be traced back to 1951-1961 (111). During this period, Brookhaven National Laboratory conducted a clinical trial using 10B-enriched borax and recruited 28 patients with brain cancers, including GBM and other types of gliomas. However, these trials failed because the first generation of boron drugs could damage normal tissue and be cleared from tumor cells too quickly, resulting in insufficient boron concentrations in tumors. With the subsequent development of borocaptate sodium (BSH) and boronophenylalanine (BPA) compounds, a series of clinical findings emerged after the 1990s. Five trials conducted in Japan from 1998 to 2007 (112), 1998 to 2008 (113), 1999 to 2002 (114), and 2002 to 2007 (115) reported median survival times (MSTs) of 25.7 months, 19.5 months, 23.2 months, and 10.8 months, respectively. These trials involved 15, 23, 9, and 21 patients with brain cancer, and they used various medication regimens and radiation doses. This series of studies revealed that BNCT combined with X-ray and temozolomide could prolong the median survival time of patients with GBM. In the past 20 years, with the development of new boron drugs and advancements in dosimetry, seven clinical studies on GBM have been registered with the JRCT and NCT. A summary of the results is shown in Table 7. Two studies have reported preliminary results, namely, BNCT with temozolomide (TMZ) for GBM, with an MST of 15.6 months in patients with newly diagnosed GBM and 8.6 months in patients with recurrent GBM, with the remaining studies ongoing. Other studies are in the recruiting stage, and it is worthwhile to follow the results of these studies to better guide BNCT in treating GBM.
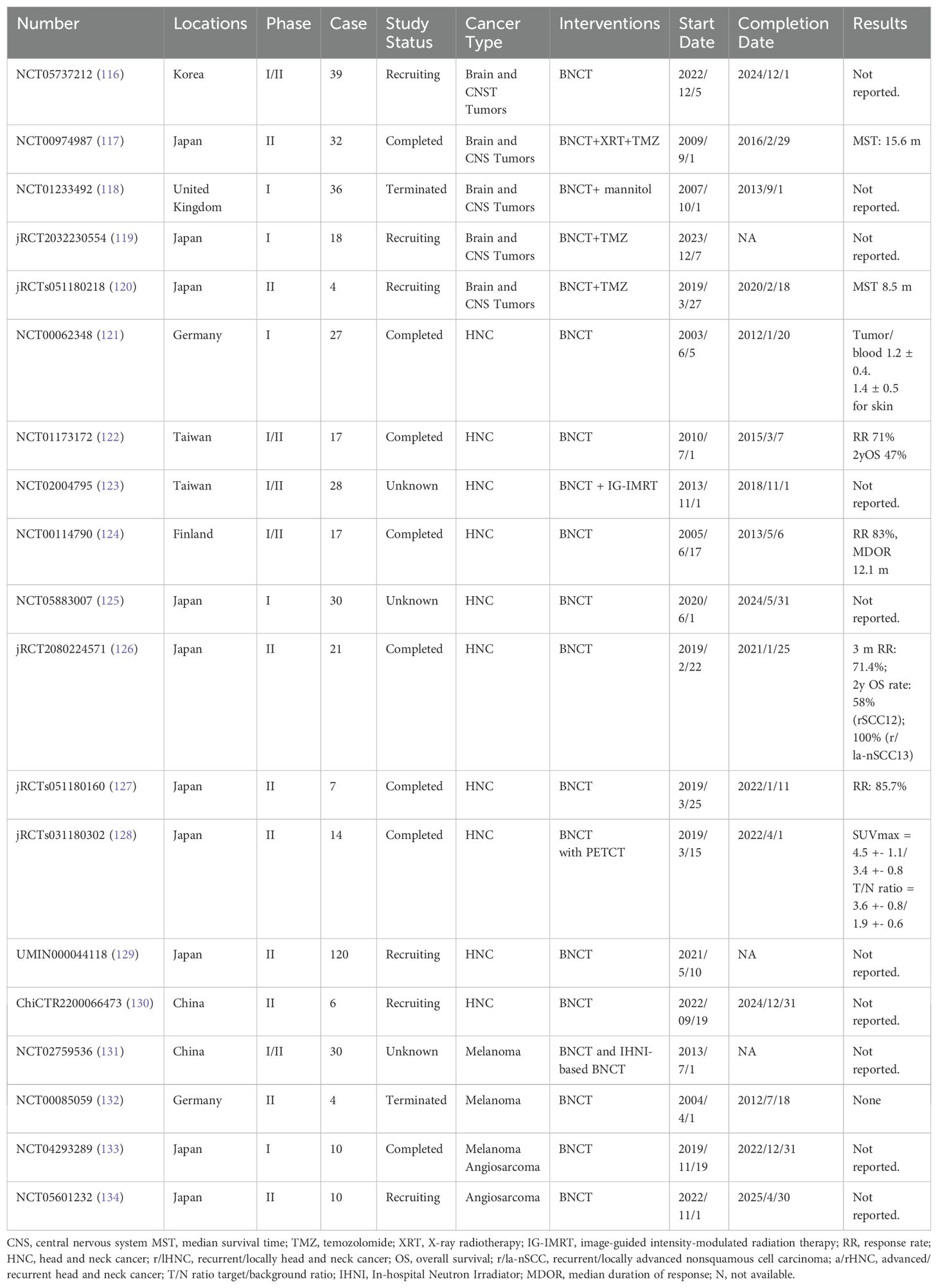
Table 7. List of Registered Clinical Studies of BNCT for the Treatment of Tumors in the Last 20 Years.
Head and neck cancers: BNCT has been used for various head and neck cancers, including squamous cell carcinoma (SCC). It provides a viable alternative to conventional therapies, especially for patients who have not responded well to traditional treatments or those with recurrent head and neck cancer (HNC). In the past 20 years, approximately 10 clinical trials have investigated BNCT for HNC. The earliest trial, EORTC 11011 (NCT00062348) (121), began in Essen, Germany, in 2003 and involved six patients with advanced SCC of the head and neck. These patients received an injection of either BPA or BSH, and the researchers assessed the distribution of boron-10 (B10) in both tumor and normal tissues. The mucosa and skin were identified as the most critical organs at risk.
Between 2010 and 2015, researchers in Taiwan conducted studies involving 17 patients who underwent BNCT with BPA at a dose of 400 mg/kg, delivering a prescribed dose of 12–35 Gy. Among these patients, six achieved a complete response, and six achieved a partial response, resulting in a 2-year overall survival (OS) rate of 47%. This study demonstrated that fractionated BNCT, administered at 30-day intervals with adaptive planning, is both effective and safe (122). Another study at the same center (NCT02004795) (135) focused on combining BNCT with image-guided intensity-modulated radiotherapy (IG-IMRT). The trial involved nine participants, and the combined BNCT+IMRT plan showed a significantly better conformity index for gross tumor volume (GTV) than did the BNCT-alone plan (P = 0.003). This improvement was particularly notable for tumors larger than 100 cm³, indicating that combining BNCT with IG-IMRT enhances homogeneity and conformity in treating larger tumors. This study aimed to enroll 28 patients, and the final survival-related results are anticipated.
A phase I/II trial (NCT00114790) from Finland involved 12 patients with locally advanced (rT3, rT4, or rN2) head and neck cancer that had recurred and was inoperable (124). This study revealed that 83% of patients responded positively to treatment, and 17% experienced tumor growth stabilization for 5.5 to 7.6 months. The median duration of response was 12.1 months, highlighting the effectiveness and favorable safety profile of BNCT for treating inoperable, locally advanced head and neck carcinomas, including those with recurrence at previously irradiated sites.
In Japan, BNCT research for HNC has also been substantial. Over the past 20 years, five trials have been registered. Hirose et al. conducted a trial (jRCT2080224571) using BNCT with a cyclotron-based epithermal neutron source (C-BENS). The study included 21 patients, with eight recurrent SCC (rSCC) and 13 non-SCC (r/la-nSCC) cases. The response rates were 42.9% at one month, 57.1% at two months, and 71.4% at three months (126). The two-year OS rates were 58% for the rSCC group and 100% for the non-SCC group. Additionally, two small-sample phase II studies initiated in 2019 have yet to publish any results. By 2023, two other trials (UMIN000044118 and ChiCTR2200066473) established by Japanese and Chinese researchers were registered for HNC and BNCT. The Japanese study is expected to include 120 patients with SCC of the head and neck, providing valuable insights from larger clinical research outcomes.
Melanoma: Boron–Neutron capture therapy (BNCT) has demonstrated efficacy in treating melanoma, particularly in advanced stages. Research suggests that BNCT is effective in reducing tumor size and controlling metastases. Since 2013, five studies have investigated BNCT for skin tumors, but only one study has published results. A phase I/II trial conducted by Kamitani (136) (jRCTs061180066) and completed in June 2020 included three patients with recurrent skin malignancies who had not undergone surgical treatment. The patients were intravenously administered the BPA-F complex (200 mg/kg body weight) for 2.5 to 3 hours before irradiation. The results revealed a 100% tumor control rate (CR+PR) with no adverse effects exceeding Grade 3. These findings indicate the effectiveness of BNCT against recurrent skin cancers and malignancies. However, the study included only three patients. The largest ongoing study is being conducted by researchers from Xiangya Third Hospital in China. This study aimed to enroll 30 patients, use 350 mg/kg BPA and deliver 20.0 Gy of radiation biological effectiveness (RBE). The results of this study are anticipated and may provide further insights into the efficacy of BNCT for the treatment of melanoma.
In addition to the tumors mentioned above, BNCT has also been investigated in other cancers, such as liver cancer, sarcoma, angiosarcoma, and refractory breast cancer, demonstrating objective efficacy. For example, a phase I/II clinical study published in 2015 (137) reported the treatment of malignant peripheral nerve sheath tumors (MPNSTs) with 500 mg/kg of boronophenylalanine (BPA) and 24.3 Gy-Eq, resulting in a two-year stable disease (SD) period. Other nonregistered studies are not discussed here.
4.4 Future research trends
Although BNCT was theoretically established more than 80 years ago, its clinical development has been limited by neutron source technology. Recent advancements in accelerator-driven neutron sources have revitalized BNCT, enabling practical applications. On the basis of the analysis of recent keyword clusters, future research will likely focus on several key areas: enhancing targeting and specificity through new boron carriers that selectively accumulate in tumors, improving molecular targeting strategies, and developing more accurate assays for boron uptake. Additionally, synergistic approaches that combine BNCT with other therapies, such as immunotherapy and advanced delivery systems using nanoparticles, will be explored to improve treatment efficacy. Establishing standardized radiation dose guidelines through clinical trials is essential for diverse cancer types, alongside efforts to increase international collaboration and innovation in neutron source development to increase the accessibility and cost effectiveness of BNCT.
4.5 Advantages and limitations
The primary strength of our research lies in the comprehensive analysis of global publications on BNCT from a scientific literature perspective. However, there are several limitations. First, the papers in this study were exclusively sourced from WOSCC, which may have resulted in some omissions in the literature, especially considering the long-time frame. Second, since our findings were primarily based on common bibliometric indicators, we may have overlooked important metrics, potentially missing valuable insights. Third, as the BNCT field is rapidly advancing, the most recent publications were not included in our analysis; these publications will be incorporated in future studies. Fourth, the retrieval strategy focused solely on publications written in English, potentially introducing selection bias by excluding studies in other languages. Finally, owing to the large sample size, our findings provide a general overview of the BNCT field, but some potentially valuable research directions may have been overlooked.
5 Conclusion
To summarize, we conducted a bibliometric analysis using VOSviewer, R, and CiteSpace to outline the current research situation and development trend of BNCT. This article demonstrates the characteristics of the publications; identifies the most influential countries, institutions, authors, journals, and articles; and analyzes the bursts of keywords and references. In addition, we also discussed the research hotspots and trends of BNCT. At present, basic and preclinical research on novel boron agents, as well as the clinical application of BNCT in brain tumors, head and neck malignancies and melanomas, are current research hotspots. Future research trends will include improving the treatment regimen, examining the application of accelerator-based neutron sources, refining the dosimetry, and exploring the fitness of BNCT in other clinical settings, such as multiple liver or lung metastases. In addition, the synergistic effects and cost effectiveness of BNCT are largely unstudied. Finally, the pursuit of a better boron delivery solution is still far from complete.
Data availability statement
The original contributions presented in the study are included in the article/Supplementary Material. Further inquiries can be directed to the corresponding authors.
Author contributions
YC: Conceptualization, Data curation, Formal analysis, Methodology, Visualization, Writing – original draft, Investigation, Validation, Writing – review & editing. MA: Data curation, Formal analysis, Writing – original draft, Validation. YM: Writing – review & editing, Supervision. JJ: Funding acquisition, Writing – review & editing, Project administration, Supervision.
Funding
The author(s) declare financial support was received for the research, authorship, and/or publication of this article. This project was supported by Sanming Project of Medicine in Shenzhen (No. SZSM202211030), Shenzhen Key Medical Discipline Construction Fund (No. SZXK013), Shenzhen High-level Hospital Construction Fund, and Shenzhen Clinical Research Center for Cancer (No. (2021)287). All from the Shenzhen government for the research, authorship, and/or publication of this article.
Conflict of interest
The authors declare that the research was conducted in the absence of any commercial or financial relationships that could be construed as a potential conflict of interest.
Generative AI statement
The author(s) declare that no Generative AI was used in the creation of this manuscript.
Publisher’s note
All claims expressed in this article are solely those of the authors and do not necessarily represent those of their affiliated organizations, or those of the publisher, the editors and the reviewers. Any product that may be evaluated in this article, or claim that may be made by its manufacturer, is not guaranteed or endorsed by the publisher.
Supplementary material
The Supplementary Material for this article can be found online at: https://www.frontiersin.org/articles/10.3389/fonc.2024.1507157/full#supplementary-material
References
1. Bray F, Laversanne M, Sung H, Ferlay J, Siegel RL, Soerjomataram I, et al. Global cancer statistics 2022: globocan estimates of incidence and mortality worldwide for 36 cancers in 185 countries. CA Cancer J Clin. (2024) 74:229–63. doi: 10.3322/caac.21834
2. Seyfried TN, Huysentruyt LC. On the origin of cancer metastasis. Crit Rev Oncog. (2013) 18:43–73. doi: 10.1615/critrevoncog.v18.i1-2.40
3. Love RR, Leventhal H, Easterling DV, Nerenz DR. Side effects and emotional distress during cancer chemotherapy. Cancer. (1989) 63:604–12. doi: 10.1002/1097-0142(19890201)63:3<604::AID-CNCR2820630334>3.0.CO;2-2
4. Kroschinsky F, Stolzel F, von Bonin S, Beutel G, Kochanek M, Kiehl M, et al. New drugs, new toxicities: severe side effects of modern targeted and immunotherapy of cancer and their management. Crit Care. (2017) 21:89. doi: 10.1186/s13054-017-1678-1
5. Connell PP, Hellman S. Advances in radiotherapy and implications for the next century: A historical perspective. Cancer Res. (2009) 69:383–92. doi: 10.1158/0008-5472.CAN-07-6871
6. Frazier RC, Vicini FA, Sharpe MB, Yan D, Fayad J, Baglan KL, et al. Impact of breathing motion on whole breast radiotherapy: A dosimetric analysis using active breathing control. Int J Radiat Oncol Biol Phys. (2004) 58:1041–7. doi: 10.1016/j.ijrobp.2003.07.005
7. Taylor HJ, Goldhaber M. Detection of nuclear disintegration in a photographic emulsion. Nature. (1935) 135:341–. doi: 10.1038/135341a0
8. Garfield E. 100 citation classics from the journal of the American medical association. JAMA. (1987) 257:52–9. doi: 10.1001/jama.1987.03390010056028
9. Aria M, Cuccurullo C. Bibliometrix: an R-tool for comprehensive science mapping analysis. J Informetrics. (2017) 11:959–75. doi: 10.1016/j.joi.2017.08.007
10. Riehmann P, Hanfler M, Fröhlich B. Interactive sankey diagrams. IEEE Symposium on Information Visualization (InfoVis 05); Minneapolis. Hoboken, New Jersey, USA: John Wiley & Sons (2005). p. 233–40.
11. Farr LE. Neutron capture therapy with boron in the treatment of glioblastoma multiforme. Am J Roentgenol Radium Ther Nucl Med. (1954) 71:279–93.
12. Nakagawa Y, Pooh K, Kobayashi T, Kageji T, Uyama S, Matsumura A, et al. Clinical review of the Japanese experience with boron neutron capture therapy and a proposed strategy using epithermal neutron beams. J Neurooncol. (2003) 62:87–99. doi: 10.1007/bf02699936
13. Sweet WH. Practical problems of the past in the use of Boron: Slow neutron capture therapy in the treatment of glioblastoma multiforme. The first international symposium on neutron capture therapy. United States: International Atomic Energy Agency (IAEA) (1982).
14. Busse PM, Harling OK, Palmer MR, Kiger WS 3rd, Kaplan J, Kaplan I, et al. A critical examination of the results from the Harvard-mit nct program phase I clinical trial of neutron capture therapy for intracranial disease. J Neurooncol. (2003) 62:111–21. doi: 10.1007/bf02699938
15. Chadha M, Capala J, Coderre JA, Elowitz EH, Iwai J, Joel DD, et al. Boron neutron-capture therapy (Bnct) for glioblastoma multiforme (Gbm) using the epithermal neutron beam at the Brookhaven national laboratory. Int J Radiat Oncol Biol Phys. (1998) 40:829–34. doi: 10.1016/s0360-3016(97)00891-2
16. Pignol JP, Paquis P, Breteau N, Chauvel P, Sauerwein W, Group EBS. Boron Neutron Capture Enhancement of Fast Neutron for Nonremoved Glioblastomas: Rationale of a Clinical Trial. In: Wiegel T, Hinkelbein W, Brock M, Hoell T, editors. Controversies in Neuro-Oncology: 3rd International Symposium on Special Aspects of Radiotherapy, Berlin, April/May 1998, vol. 33. Basel, Switzerland: S. Karger AG (1999).
17. Suzuki M. Boron neutron capture therapy (Bnct): A unique role in radiotherapy with a view to entering the accelerator-based bnct era. Int J Clin Oncol. (2020) 25:43–50. doi: 10.1007/s10147-019-01480-4
18. Blue TE, Yanch JC. Accelerator-based epithermal neutron sources for boron neutron capture therapy of brain tumors. J Neurooncol. (2003) 62:19–31. doi: 10.1007/bf02699931
19. Amin Shokr AA, Auterinen C, Binney I, Blaumann S, Blue H, Burian T, et al. Current Status of Neutron Capture Therapy. Vienna: INTERNATIONAL ATOMIC ENERGY AGENCY (2001).
20. Kumada H, Sakae T, Sakurai H. Current development status of accelerator-based neutron source for boron neutron capture therapy. EPJ Techn Instrument. (2023) 10:18. doi: 10.1140/epjti/s40485-023-00105-5
21. Tanaka H, Sakurai Y, Suzuki M, Masunaga S, Kinashi Y, Kashino G, et al. Characteristics comparison between a cyclotron-based neutron source and kur-hwnif for boron neutron capture therapy. Nucl Instruments Methods Phys Res Section B: Beam Interact Mater Atoms. (2009) 267:1970–7. doi: 10.1016/j.nimb.2009.03.095
22. Nakamura S, Igaki H, Ito M, Imamichi S, Kashihara T, Okamoto H, et al. Neutron flux evaluation model provided in the accelerator-based boron neutron capture therapy system employing a solid-state lithium target. Sci Rep. (2021) 11:8090. doi: 10.1038/s41598-021-87627-8
23. Horiike H, Murata I, Iida T, Yoshihashi S, Hoashi E, Kato I, et al. Liquid li based neutron source for bnct and science application. Appl Radiat Isotopes. (2015) 106:92–4. doi: 10.1016/j.apradiso.2015.07.026
24. Kumada H, Kurihara T, Yoshioka M, Kobayashi H, Matsumoto H, Sugano T, et al. Development of beryllium-based neutron target system with three-layer structure for accelerator-based neutron source for boron neutron capture therapy. Appl Radiat Isotopes. (2015) 106:78–83. doi: 10.1016/j.apradiso.2015.07.033
25. Matsumura A, Asano T, Hirose K, Igaki H, Kawabata S, Kumada H. Initiatives toward clinical boron neutron capture therapy in Japan. Cancer Biother Radiopharm. (2023) 38:201–7. doi: 10.1089/cbr.2022.0056
26. Zhang Z, Chong Y, Liu Y, Pan J, Huang C, Sun Q, et al. A review of planned, ongoing clinical studies and recent development of bnct in mainland of China. Cancers (Basel). (2023) 15:16. doi: 10.3390/cancers15164060
27. Bae Y-s, Kim D-S, Seo HJ, Han J-U, Yoon HJ, Hwang JJ, et al. Advances of linac-based boron neutron capture therapy in Korea. AAPPS Bull. (2022) 32:34. doi: 10.1007/s43673-022-00063-2
28. Barth RF, Coderre JA, Vicente MG, Blue TE. Boron neutron capture therapy of cancer: current status and future prospects. Clin Cancer Res. (2005) 11:3987–4002. doi: 10.1158/1078-0432.Ccr-05-0035
29. Goodwin JT, Farr LE, Sweet WH, Robertson JS. Pathological study of eight patients with glioblastoma multiforme treated by neutron-capture therapy using boron 10. Cancer. (1955) 8:601–15. doi: 10.1002/1097-0142(1955)8:3<601::aid-cncr2820080326>3.0.co;2-r
30. Soloway AH, Hatanaka H, Davis MA. Penetration of brain and brain tumor. Vii. Tumor-binding sulfhydryl boron compounds. J Medicinal Chem. (1967) 10:714–7. doi: 10.1021/jm00316a042
31. Snyder H, Reedy AJ, Lennarz WJ. Synthesis of aromatic boronic acids. Aldehydo boronic acids and a boronic acid analog of tyrosine1. J Am Chem Soc. (1958) 80:835–8. doi: 10.1021/ja01537a021
32. Hideghéty K, Sauerwein W, Wittig A, Götz C, Paquis P, Grochulla F, et al. Tissue uptake of bsh in patients with glioblastoma in the eortc 11961 phase I bnct trial. J neuro-oncol. (2003) 62:145–56. doi: 10.1007/BF02699941
33. Wittig A, Sauerwein WA, Coderre JA. Mechanisms of transport of P-borono-phenylalanine through the cell membrane in vitro. Radiat Res. (2000) 153:173–80. doi: 10.1667/0033-7587(2000)153[0173:motopb]2.0.co;2
34. Barth RF, Vicente MG, Harling OK, Kiger WS 3rd, Riley KJ, Binns PJ, et al. Current status of boron neutron capture therapy of high grade gliomas and recurrent head and neck cancer. Radiat Oncol. (2012) 7:146. doi: 10.1186/1748-717x-7-146
35. Barth RF, Mi P, Yang W. Boron delivery agents for neutron capture therapy of cancer. Cancer Commun (Lond). (2018) 38:35. doi: 10.1186/s40880-018-0299-7
36. Kalot G, Godard A, Busser B, Pliquett J, Broekgaarden M, Motto-Ros V, et al. Aza-bodipy: A new vector for enhanced theranostic boron neutron capture therapy applications. Cells. (2020) 9:1953. doi: 10.3390/cells9091953
37. Lamba M, Goswami A, Bandyopadhyay A. A periodic development of bpa and bsh based derivatives in boron neutron capture therapy (Bnct). Chem Commun. (2021) 57:827–39. doi: 10.1039/D0CC06557A
38. Watanabe T, Hattori Y, Ohta Y, Ishimura M, Nakagawa Y, Sanada Y, et al. Comparison of the pharmacokinetics between L-bpa and L-fbpa using the same administration dose and protocol: A validation study for the theranostic approach using [18 F]-L-fbpa positron emission tomography in boron neutron capture therapy. BMC Cancer. (2016) 16:1–10. doi: 10.1186/s12885-016-2913-x
39. Kabalka G, Wu Z, Yao ML. Synthesis of a series of boronated unnatural cyclic amino acids as potential boron neutron capture therapy agents. Appl Organometal Chem. (2008) 22:516–22. doi: 10.1002/aoc.1435
40. Semioshkin A, Nizhnik E, Godovikov I, Starikova Z, Bregadze V. Reactions of oxonium derivatives of [B12h12]2– with amines: synthesis and structure of novel B12-based ammonium salts and amino acids. J Organometal Chem. (2007) 692:4020–8. doi: 10.1016/j.jorganchem.2007.06.001
41. Mäde V, Els-Heindl S, Beck-Sickinger AG. Automated solid-phase peptide synthesis to obtain therapeutic peptides. Beilstein J Organ Chem. (2014) 10:1197–212. doi: 10.3762/bjoc.10.118
42. Iguchi Y, Michiue H, Kitamatsu M, Hayashi Y, Takenaka F, Nishiki T-i, et al. Tumor-specific delivery of bsh-3r for boron neutron capture therapy and positron emission tomography imaging in a mouse brain tumor model. Biomaterials. (2015) 56:10–7. doi: 10.1016/j.biomaterials.2015.03.061
43. Isono A, Tsuji M, Sanada Y, Matsushita A, Masunaga S, Hirayama T, et al. Design, synthesis, and evaluation of lipopeptide conjugates of mercaptoundecahydrododecaborate for boron neutron capture therapy. ChemMedChem. (2019) 14:823–32. doi: 10.1002/cmdc.201800793
44. Backer MV, Gaynutdinov TI, Patel V, Bandyopadhyaya AK, Thirumamagal BTS, Tjarks W, et al. Vascular endothelial growth factor selectively targets boronated dendrimers to tumor vasculature. Mol Cancer Ther. (2005) 4:1423–9. doi: 10.1158/1535-7163.Mct-05-0161
45. Yang W, Barth RF, Wu G, Huo T, Tjarks W, Ciesielski M, et al. Convection enhanced delivery of boronated egf as a molecular targeting agent for neutron capture therapy of brain tumors. J Neuro-Oncol. (2009) 95:355–65. doi: 10.1007/s11060-009-9945-x
46. Koppenol WH, Bounds PL, Dang CV. Otto Warburg’s contributions to current concepts of cancer metabolism. Nat Rev Cancer. (2011) 11:325–37. doi: 10.1038/nrc3038
47. Itoh T, Tamura K, Ueda H, Tanaka T, Sato K, Kuroda R, et al. Design and synthesis of boron containing monosaccharides by the hydroboration of D-glucal for use in boron neutron capture therapy (Bnct). Bioorgan Medicinal Chem. (2018) 26:5922–33. doi: 10.1016/j.bmc.2018.10.041
48. Matović J, Järvinen J, Bland HC, Sokka IK, Imlimthan S, Ferrando RM, et al. Addressing the biochemical foundations of a glucose-based “Trojan horse”-strategy to boron neutron capture therapy: from chemical synthesis to in vitro assessment. Mol Pharma. (2020) 17:3885–99. doi: 10.1021/acs.molpharmaceut.0c00630
49. Tsurubuchi T, Shirakawa M, Kurosawa W, Matsumoto K, Ubagai R, Umishio H, et al. Evaluation of a novel boron-containing A-D-mannopyranoside for bnct. Cells. (2020) 9:1277. doi: 10.3390/cells9051277
50. Xiong M, Lei Q, You X, Gao T, Song X, Xia Y, et al. Mannosylated liposomes improve therapeutic effects of paclitaxel in colon cancer models. J Microencapsul. (2017) 34:513–21. doi: 10.1080/02652048.2017.1339739
51. Arnér ESJ, Eriksson S. Mammalian deoxyribonucleoside kinases. Pharmacol Ther. (1995) 67:155–86. doi: 10.1016/0163-7258(95)00015-9
52. Tjarks W, Tiwari R, Byun Y, Narayanasamy S, Barth RF. Carboranyl thymidine analogues for neutron capture therapy. Chem Commun. (2007) 47:4978–91. doi: 10.1039/b707257k
53. Barth RF, Yang W, Nakkula RJ, Byun Y, Tjarks W, Chu Wu L, et al. Evaluation of tk1 targeting carboranyl thymidine analogs as potential delivery agents for neutron capture therapy of brain tumors. Appl Radiat Isotopes. (2015) 106:251–5. doi: 10.1016/j.apradiso.2015.06.031
54. Parker N, Turk MJ, Westrick E, Lewis JD, Low PS, Leamon CP. Folate receptor expression in carcinomas and normal tissues determined by a quantitative radioligand binding assay. Anal Biochem. (2005) 338:284–93. doi: 10.1016/j.ab.2004.12.026
55. Zwicke GL, Ali Mansoori G, Jeffery CJ. Utilizing the folate receptor for active targeting of cancer nanotherapeutics. Nano Rev. (2012) 3:18496. doi: 10.3402/nano.v3i0.18496
56. Nakagawa F, Kawashima H, Morita T, Nakamura H. Water-soluble closo-docecaborate-containing pteroyl derivatives targeting folate receptor-positive tumors for boron neutron capture therapy. Cells. (2020) 9:1615. doi: 10.3390/cells9071615
57. Leonelli F, La Bella A, Francescangeli A, Joudioux R, Capodilupo A-L, Quagliariello M, et al. A new and simply available class of hydrosoluble bioconjugates by coupling paclitaxel to hyaluronic acid through a 4-hydroxybutanoic acid derived linker. Helv Chimica Acta. (2005) 88:154–9. doi: 10.1002/hlca.200490289
58. Di Meo C, Panza L, Campo F, Capitani D, Mannina L, Banzato A, et al. Novel types of carborane-carrier hyaluronan derivatives via “Click chemistry. Macromol Biosci. (2008) 8:670–81. doi: 10.1002/mabi.200700304
59. Yu X, Li Z. Microrna expression and its implications for diagnosis and therapy of tongue squamous cell carcinoma. J Cell Mol Med. (2016) 20:10–6. doi: 10.1111/jcmm.12650
60. Zhang T, Du S, Wang Y, Guo Y, Yi Y, Liu B, et al. Novel carborane compounds based on cyclooxygenase-2 inhibitors for effective boron neutron capture therapy of tongue squamous cell carcinoma. ChemistrySelect. (2020) 5:14652–60. doi: 10.1002/slct.202004077
61. Xenaki KT, Oliveira S, van Bergen en Henegouwen PMP. Antibody or antibody fragments: implications for molecular imaging and targeted therapy of solid tumors. Front Immunol. (2017) 8:1287. doi: 10.3389/fimmu.2017.01287
62. Goldenberg DM, Sharkey RM, Primus FJ, Mizusawa E, Hawthorne MF. Neutron-capture therapy of human cancer: in vivo results on tumor localization of boron-10-labeled antibodies to carcinoembryonic antigen in the gw-39 tumor model system. Proc Natl Acad Sci. (1984) 81:560–3. doi: 10.1073/pnas.81.2.560
63. Wu G, Yang W, Barth RF, Kawabata S, Swindall M, Bandyopadhyaya AK, et al. Molecular targeting and treatment of an epidermal growth factor receptor–positive glioma using boronated cetuximab. Clin Cancer Res. (2007) 13:1260–8. doi: 10.1158/1078-0432.Ccr-06-2399
64. Yang W, Barth RF, Wu G, Kawabata S, Sferra TJ, Bandyopadhyaya AK, et al. Molecular targeting and treatment of egfrviii-positive gliomas using boronated monoclonal antibody L8a4. Clin Cancer Res. (2006) 12:3792–802. doi: 10.1158/1078-0432.Ccr-06-0141
65. Yang W, Barth RF, Wu G, Tjarks W, Binns P, Riley K. Boron neutron capture therapy of egfr or egfrviii positive gliomas using either boronated monoclonal antibodies or epidermal growth factor as molecular targeting agents. Appl Radiat Isotopes. (2009) 67:S328–S31. doi: 10.1016/j.apradiso.2009.03.030
66. Yang W, Wu G, Barth RF, Swindall MR, Bandyopadhyaya AK, Tjarks W, et al. Molecular targeting and treatment of composite egfr and egfrviii-positive gliomas using boronated monoclonal antibodies. Clin Cancer Res. (2008) 14:883–91. doi: 10.1158/1078-0432.Ccr-07-1968
67. Nakase I, Aoki A, Sakai Y, Hirase S, Ishimura M, Takatani-Nakase T, et al. Antibody-based receptor targeting using an fc-binding peptide-dodecaborate conjugate and macropinocytosis induction for boron neutron capture therapy. ACS Omega. (2020) 5:22731–8. doi: 10.1021/acsomega.0c01377
68. Sun T, Li Y, Huang Y, Zhang Z, Yang W, Du Z, et al. Targeting glioma stem cells enhances anti-tumor effect of boron neutron capture therapy. Oncotarget. (2016) 7:43095. doi: 10.18632/oncotarget.v7i28
69. Yoon T-J, Lee W, Oh Y-S, Lee J-K. Magnetic nanoparticles as a catalyst vehicle for simple and easy recycling. New J Chem. (2003) 27:227–9. doi: 10.1039/b209391j
70. Bhatia SN, Chen X, Dobrovolskaia MA, Lammers T. Cancer nanomedicine. Nat Rev Cancer. (2022) 22:550–6. doi: 10.1038/s41568-022-00496-9
71. Zhu Y, Hosmane NS. Nanostructured boron compounds for cancer therapy. Pure Appl Chem. (2018) 90:653–63. doi: 10.1515/pac-2017-0903
72. Wu J. The enhanced permeability and retention (Epr) effect: the significance of the concept and methods to enhance its application. J personal Med. (2021) 11:771. doi: 10.3390/jpm11080771
73. Shukla S, Wu G, Chatterjee M, Yang W, Sekido M, Diop LA, et al. Synthesis and biological evaluation of folate receptor-targeted boronated pamam dendrimers as potential agents for neutron capture therapy. Bioconjugate Chem. (2003) 14:158–67. doi: 10.1021/bc025586o
74. Ruan Z, Liu L, Fu L, Xing T, Yan L. An amphiphilic block copolymer conjugated with carborane and a nir fluorescent probe for potential imaging-guided bnct therapy. Polymer Chem. (2016) 7:4411–8. doi: 10.1039/C6PY00799F
75. Sumitani S, Oishi M, Yaguchi T, Murotani H, Horiguchi Y, Suzuki M, et al. Pharmacokinetics of core-polymerized, boron-conjugated micelles designed for boron neutron capture therapy for cancer. Biomaterials. (2012) 33:3568–77. doi: 10.1016/j.biomaterials.2012.01.039
76. Takeuchi I, Nomura K, Makino K. Hydrophobic boron compound-loaded poly(L-lactide-co-glycolide) nanoparticles for boron neutron capture therapy. Colloids Surf B: Biointerf. (2017) 159:360–5. doi: 10.1016/j.colsurfb.2017.08.002
77. Chen J, Yang Q, Liu M, Lin M, Wang T, Zhang Z, et al. Remarkable boron delivery of irgd-modified polymeric nanoparticles for boron neutron capture therapy. Int J Nanomed. (2019) 14:8161–77. doi: 10.2147/IJN.S214224
78. Nomoto T, Inoue Y, Yao Y, Suzuki M, Kanamori K, Takemoto H, et al. Poly (Vinyl alcohol) boosting therapeutic potential of P-boronophenylalanine in neutron capture therapy by modulating metabolism. Sci Adv. (2020) 6:eaaz1722. doi: 10.1126/sciadv.aaz1722
79. Dai L, Liu J, Zhao X, Li Y, Zhou S, Yuan L, et al. Bpa-containing polydopamine nanoparticles for boron neutron capture therapy in a U87 glioma orthotopic model. Adv Funct Mater. (2023) 33:2214145. doi: 10.1002/adfm.202214145
80. Xu H, Liu J, Li R, Lin J, Gui L, Wang Y, et al. Novel promising boron agents for boron neutron capture therapy: current status and outlook on the future. Coordin Chem Rev. (2024) 511:215795. doi: 10.1016/j.ccr.2024.215795
81. Luo T, Huang W, Chu F, Zhu T, Feng B, Huang S, et al. The dawn of a new era: tumor-targeting boron agents for neutron capture therapy. Mol Pharm. (2023) 20:4942–70. doi: 10.1021/acs.molpharmaceut.3c00701
82. Li L, Li J, Shi Y, Du P, Zhang Z, Liu T, et al. On-demand biodegradable boron nitride nanoparticles for treating triple negative breast cancer with boron neutron capture therapy. ACS Nano. (2019) 13:13843–52. doi: 10.1021/acsnano.9b04303
83. Kaur M, Singh P, Singh K, Gaharwar US, Meena R, Kumar M, et al. Boron nitride (10bn) a prospective material for treatment of cancer by boron neutron capture therapy (Bnct). Mater Lett. (2020) 259:126832. doi: 10.1016/j.matlet.2019.126832
84. Ciofani G, Raffa V, Menciassi A, Cuschieri A. Boron nitride nanotubes: an innovative tool for nanomedicine. Nano Today. (2009) 4:8–10. doi: 10.1016/j.nantod.2008.09.001
85. Nakamura H, Koganei H, Miyoshi T, Sakurai Y, Ono K, Suzuki M. Antitumor effect of boron nitride nanotubes in combination with thermal neutron irradiation on bnct. Bioorgan med Chem Lett. (2015) 25:172–4. doi: 10.1016/j.bmcl.2014.12.005
86. Pan J, Li F, Choi JH. Single-walled carbon nanotubes as optical probes for bio-sensing and imaging. J Mater Chem B. (2017) 5:6511–22. doi: 10.1039/C7TB00748E
87. Li J, Kong J, Ma S, Li J, Mao M, Chen K, et al. Exosome-coated 10b carbon dots for precise boron neutron capture therapy in a mouse model of glioma in situ. Adv Funct Mater. (2021) 31:2100969. doi: 10.1002/adfm.202100969
88. Korolkov IV, Ludzik K, Kozlovskiy AL, Fadeev MS, Shumskaya AE, Gorin YG, et al. Carboranes immobilization on fe3o4 nanocomposites for targeted delivery. Mater Today Commun. (2020) 24:101247. doi: 10.1016/j.mtcomm.2020.101247
89. Zhu Y, Stubbs LP, Ho F, Liu R, Ship CP, Maguire JA, et al. Magnetic nanocomposites: A new perspective in catalysis. ChemCatChem. (2010) 2:365–74. doi: 10.1002/cctc.200900314
90. Wu C-Y, Hsieh H-H, Chang T-Y, Lin J-J, Wu C-C, Hsu M-H, et al. Development of mri-detectable boron-containing gold nanoparticle-encapsulated biodegradable polymeric matrix for boron neutron capture therapy (Bnct). Int J Mol Sci. (2021) 22:8050. doi: 10.3390/ijms22158050
91. Hawthorne MF. The role of chemistry in the development of boron neutron capture therapy of cancer. Angew Chem Int Ed Engl. (1993) 32:950. doi: 10.1002/anie.199309501
92. Tamanoi F, Chinnathambi S, Laird M, Komatsu A, Birault A, Takata T, et al. Construction of boronophenylalanine-loaded biodegradable periodic mesoporous organosilica nanoparticles for bnct cancer therapy. Int J Mol Sci. (2021) 22:2251. doi: 10.3390/ijms22052251
93. Laird M, Matsumoto K, Higashi Y, Komatsu A, Raitano A, Morrison K, et al. Organosilica nanoparticles containing sodium borocaptate (Bsh) provide new prospects for boron neutron capture therapy (Bnct): efficient cellular uptake and enhanced bnct efficacy. Nanoscale Adv. (2023) 5:2537–46. doi: 10.1039/D2NA00839D
94. Barth RF, Adams DM, Soloway AH, Mechetner EB, Alam F, Anisuzzaman AKM. Determination of boron in tissues and cells using direct-current plasma atomic emission spectroscopy. Anal Chem. (1991) 63:890–3. doi: 10.1021/ac00009a010
95. Mukai K, Nakagawa Y, Matsumoto K. Prompt gamma ray spectrometry for in vivo measurement of boron-10 concentration in rabbit brain tissue. Neurol medico-chirurgica. (1995) 35:855–60. doi: 10.2176/nmc.35.855
96. Arlinghaus HF, Spaar MT, Switzer RC, Kabalka GW. Imaging of boron in tissue at the cellular level for boron neutron capture therapy. Anal Chem. (1997) 69:3169–76. doi: 10.1021/ac970036i
97. Michel J, Sauerwein W, Wittig A, Balossier G, Zierold K. Subcellular localization of boron in cultured melanoma cells by electron energy-loss spectroscopy of freeze-dried cryosections. J Microsc. (2003) 210:25–34. doi: 10.1046/j.1365-2818.2003.01172.x
98. Amemiya K, Takahashi H, Kajimoto Y, Nakazawa M, Yanagie H, Hisa T, et al. High-resolution nuclear track mapping in detailed cellular histology using cr-39 with the contact microscopy technique. Radiat Measure. (2005) 40:283–8. doi: 10.1016/j.radmeas.2005.01.010
99. Basilico F, Sauerwein W, Pozzi F, Wittig A, Moss R, Mauri PL. Analysis of 10b antitumoral compounds by means of flow-injection into esi-ms/ms. J Mass Spectrom. (2005) 40:1546–9. doi: 10.1002/jms.909
100. Masunaga S-i, Sakurai Y, Suzuki M, Nagata K, Maruhashi A, Kinash Y, et al. Combination of the vascular targeting agent zd6126 with boron neutron capture therapy. Int J Radiat OncologyBiologyPhysics. (2004) 60:920–7. doi: 10.1016/j.ijrobp.2004.07.672
101. Yokoyama K, Miyatake S-I, Kajimoto Y, Kawabata S, Doi A, Yoshida T, et al. Pharmacokinetic study of bsh and bpa in simultaneous use for bnct. J Neuro-oncol. (2006) 78:227–32. doi: 10.1007/s11060-005-9099-4
102. Chandra S, Barth RF, Haider SA, Yang W, Huo T, Shaikh AL, et al. Biodistribution and subcellular localization of an unnatural boron-containing amino acid (Cis-abcpc) by imaging secondary ion mass spectrometry for neutron capture therapy of melanomas and gliomas. PloS One. (2013) 8:e75377. doi: 10.1371/journal.pone.0075377
103. Chandra S, Lorey DR II, Smith DR. Quantitative subcellular secondary ion mass spectrometry (Sims) imaging of boron-10 and boron-11 isotopes in the same cell delivered by two combined bnct drugs: in vitro studies on human glioblastoma T98g cells. Radiat Res. (2002) 157:700–10, 11. doi: 10.1667/0033-7587(2002)157[0700:QSSIMS]2.0.CO;2
104. Wittig A, Arlinghaus HF, Kriegeskotte C, Moss RL, Appelman K, Schmid KW, et al. Laser postionization secondary neutral mass spectrometry in tissue: A powerful tool for elemental and molecular imaging in the development of targeted drugs. Mol Cancer Ther. (2008) 7:1763–71. doi: 10.1158/1535-7163.MCT-08-0191
105. Aldossari S, McMahon G, Lockyer NP, Moore KL. Microdistribution and quantification of the boron neutron capture therapy drug bpa in primary cell cultures of human glioblastoma tumour by nanosims. Analyst. (2019) 144:6214–24. doi: 10.1039/C9AN01336A
106. Reifschneider O, Schütz CL, Brochhausen C, Hampel G, Ross T, Sperling M, et al. Quantitative bioimaging of P-boronophenylalanine in thin liver tissue sections as a tool for treatment planning in boron neutron capture therapy. Anal Bioanal Chem. (2015) 407:2365–71. doi: 10.1007/s00216-014-8012-4
107. Kobayashi T, Sakurai Y, Ishikawa M. A noninvasive dose estimation system for clinical bnct based on pg-spect—Conceptual study and fundamental experiments using hpge and cdte semiconductor detectors. Med Phys. (2000) 27:2124–32. doi: 10.1118/1.1288243
108. Morita T, Kurihara H, Hiroi K, Honda N, Igaki H, Hatazawa J, et al. Dynamic changes in 18f-borono-L-phenylalanine uptake in unresectable, advanced, or recurrent squamous cell carcinoma of the head and neck and Malignant melanoma during boron neutron capture therapy patient selection. Radiat Oncol. (2018) 13:4. doi: 10.1186/s13014-017-0949-y
109. Nakamura H, Fukuda H, Girald F, Kobayashi T, Hiratsuka J, Akaizawa T, et al. In vivo evaluation of carborane gadolinium-dtpa complex as an mr imaging boron carrier. Chem Pharm Bull. (2000) 48:1034–8. doi: 10.1248/cpb.48.1034
110. Takahashi K, Nakamura H, Furumoto S, Yamamoto K, Fukuda H, Matsumura A, et al. Synthesis and in vivo biodistribution of bpa–gd–dtpa complex as a potential mri contrast carrier for neutron capture therapy. Bioorgan Medicinal Chem. (2005) 13:735–43. doi: 10.1016/j.bmc.2004.10.046
111. Slatkin DN. A history of boron neutron capture therapy of brain tumours: postulation of a brain radiation dose tolerance limit. Brain. (1991) 114:1609–29. doi: 10.1093/brain/114.4.1609
112. Kawabata S, Miyatake S-I, Kuroiwa T, Yokoyama K, Doi A, Iida K, et al. Boron neutron capture therapy for newly diagnosed glioblastoma. J Radiat Res. (2008) 50:51–60. doi: 10.1269/jrr.08043
113. Kageji T, Mizobuchi Y, Nagahiro S, Nakagawa Y, Kumada H. Long-survivors of glioblatoma treated with boron neutron capture therapy (Bnct). Appl Radiat Isotopes. (2011) 69:1800–2. doi: 10.1016/j.apradiso.2011.03.021
114. Yamamoto T, Matsumura A, Nakai K, Shibata Y, Endo K, Sakurai F, et al. Current clinical results of the Tsukuba bnct trial. Appl Radiat Isotopes. (2004) 61:1089–93. doi: 10.1016/j.apradiso.2004.05.010
115. Miyatake S-I, Kawabata S, Yokoyama K, Kuroiwa T, Michiue H, Sakurai Y, et al. Survival benefit of boron neutron capture therapy for recurrent Malignant gliomas. J neuro-oncol. (2009) 91:199–206. doi: 10.1007/s11060-008-9699-x
116. Studying the Safety, Efficacy, and Pharmacokinetic Characteristics of Bnct in Patients with Recurrent High-Grade Gliomas. Available online at: https://clinicaltrials.gov/study/NCT05737212?term=NCT05737212&rank=1 (Accessed September 07, 2024).
117. Kawabata S, Miyatake S-I, Hiramatsu R, Hirota Y, Miyata S, Takekita Y, et al. Phase ii clinical study of boron neutron capture therapy combined with X-ray radiotherapy/temozolomide in patients with newly diagnosed glioblastoma multiforme—Study design and current status report. Appl Radiat Isotopes. (2011) 69:1796–9. doi: 10.1016/j.apradiso.2011.03.014
118. Boron Phenylalanine with or without Mannitol in Treating Patients with Glioblastoma Multiforme. Available online at: https://clinicaltrials.gov/study/NCT01233492?term=NCT01233492&rank=1 (Accessed September 07, 2024).
119. Phase I Clinical Bnct Trial to Evaluate the Safty of ibnct001 and spm-011, accelerator-based novel high-power neutron source in patient with newly diagnosed glioblastoma (Ibnct-gbm-01). Available online at: https://jrct.niph.go.jp/latest-detail/jRCT2032230554 (Accessed September 07, 2024).
120. Phase ii clinical trial on clinical efficacy and safety of combination therapy of boron neutron capture therapy and bevacizumab for recurrent glioma with poor prognosis. Available online at: https://jrct.niph.go.jp/en-latest-detail/jRCTs051180218 (Accessed September 07, 2024).
121. Wittig A, Collette L, Appelman K, Bührmann S, Jäckel MC, Jöckel KH, et al. Eortc trial 11001: distribution of two 10b-compounds in patients with squamous cell carcinoma of head and neck, a translational research/phase 1 trial. J Cell Mol Med. (2009) 13:1653–65. doi: 10.1111/j.1582-4934.2009.00856.x
122. Wang L-W, Chen Y-W, Ho C-Y, Liu Y-WH, Chou F-I, Liu Y-H, et al. Fractionated boron neutron capture therapy in locally recurrent head and neck cancer: A prospective phase I/ii trial. Int J Radiat Oncol Biol Phys. (2016) 95:396–403. doi: 10.1016/j.ijrobp.2016.02.028
123. Bnct and ig-imrt for recurrent head and neck cancer. Available online at: https://clinicaltrials.gov/study/NCT02004795?term=NCT02004795&rank=1 (Accessed September 07, 2024).
124. Kankaanranta L, Seppälä T, Koivunoro H, Saarilahti K, Atula T, Collan J, et al. Boron neutron capture therapy in the treatment of locally recurred head-and-neck cancer: final analysis of a phase I/ii trial. Int J Radiat Oncol Biol Phys. (2012) 82:e67–75. doi: 10.1016/j.ijrobp.2010.09.057
125. Dose optimized bnct for head and neck cancer (St-bnct2001). Available online at: https://clinicaltrials.gov/study/NCT05883007?term=NCT05883007&rank=1 (Accessed September 07, 2024).
126. Hirose K, Konno A, Hiratsuka J, Yoshimoto S, Kato T, Ono K, et al. Boron neutron capture therapy using cyclotron-based epithermal neutron source and borofalan (10b) for recurrent or locally advanced head and neck cancer (Jhn002): an open-label phase ii trial. Radiother Oncol. (2021) 155:182–7. doi: 10.1016/j.radonc.2020.11.001
127. Establishment of protocol for neutron capture therapy for head and neck cancer (Bnct for hnc). Available online at: https://jrct.niph.go.jp/en-latest-detail/jRCTs051180160 (Accessed September 07, 2024).
128. Clinical study to evaluate the usefulness of 18F-FBPA-PET for patient selection and expanding indication for BNCT. Available online at: https://jrct.niph.go.jp/en-latest-detail/jRCTs031180302 (Accessed September 07, 2024).
129. Boron neutron capture therapy using cyclotron-based epithermal neutron source for squamous cell carcinoma of the head and neck refractory to standard treatments: an open-label phase ii trial. Available online at: https://rctportal.niph.go.jp/s/detail/um?trial_id=UMIN000044118 (Accessed September 07, 2024).
130. A single-center, single-arm clinical trial of the safety and efficacy of boron neutron capture therapy (Bnct) for advanced refractory Malignant tumors. Available online at: https://www.chictr.org.cn/showproj.html?proj=181032 (Accessed September 07, 2024).
131. In-hospital neutron irradiator (Ihni)-based boron neutron capture therapy (Bnct) in the treatment of Malignant melanoma. Available online at: https://clinicaltrials.gov/study/NCT02759536?intr=NCT02759536&rank=1 (Accessed September 07, 2024).
132. Boron neutron capture therapy using boronophenylalanine-fructose complex in treating patients with metastatic melanoma. Available online at: https://clinicaltrials.gov/study/NCT00085059?term=NCT00085059&rank=1 (Accessed September 07, 2024).
133. Boron neutron capture therapy using cics-1 and spm-011 for Malignant melanoma and angiosarcoma. Available online at: https://clinicaltrials.gov/study/NCT04293289?term=NCT04293289&rank=1 (Accessed September 07, 2024).
134. A phase ii study by using cics-1 and spm-011 commissioned by cics and stella pharma. Available online at: https://clinicaltrials.gov/study/NCT05601232?term=NCT05601232&rank=1 (Accessed September 07, 2024).
135. Lee J-C, Chuang K-S, Hsueh Liu Y-W, Lin T-Y, Teng Y-C, Wang L-W. A comparison of dose distributions in gross tumor volume between boron neutron capture therapy alone and combined boron neutron capture therapy plus intensity modulation radiation therapy for head and neck cancer. PloS One. (2019) 14:e0210626. doi: 10.1371/journal.pone.0210626
136. Bnct Phase I/Ii Trial for Skin Malignancies. Available online at: https://jrct.niph.go.jp/latest-detail/jRCTs061180066 (Accessed September 07, 2024).
Keywords: boron neutron capture therapy, bibliometric analysis, research trends, VOSviewer, CiteSpace
Citation: Cong Y, Abulimiti M, Matsumoto Y and Jin J (2024) Current research trends and hotspots of boron neutron capture therapy: a bibliometric and visualization analysis. Front. Oncol. 14:1507157. doi: 10.3389/fonc.2024.1507157
Received: 07 October 2024; Accepted: 25 November 2024;
Published: 12 December 2024.
Edited by:
Francesca Ballarini, University of Pavia, ItalyReviewed by:
Elvira V. Grigorieva, Institute of Molecular Biology and Biophysics FRC FTM, RussiaJiejian Chen, Guangzhou First People’s Hospital, China
Naoki Komatsu, Kyoto University, Japan
Copyright © 2024 Cong, Abulimiti, Matsumoto and Jin. This is an open-access article distributed under the terms of the Creative Commons Attribution License (CC BY). The use, distribution or reproduction in other forums is permitted, provided the original author(s) and the copyright owner(s) are credited and that the original publication in this journal is cited, in accordance with accepted academic practice. No use, distribution or reproduction is permitted which does not comply with these terms.
*Correspondence: Jing Jin, amluZ2ppbjEwMjVAMTYzLmNvbQ==; Yoshitaka Matsumoto, eW1hdHN1bW90b0BwbXJjLnRzdWt1YmEuYWMuanA=