- Department of Radiotherapy Oncology, The Second Affiliated Hospital of Dalian Medical University, Dalian, China
Cervical cancer (CC) is a common malignant tumour of the female reproductive system that is highly harmful to women’s health. The efficacy of traditional surgery, radiotherapy and chemotherapy is limited, especially for recurrent and metastatic CC. With continuous progress in diagnostic and treatment technology, immunotherapy has become a new approach for treating CC and has become a new therapy for recurrent and metastatic CC. However, immunotherapy is not effective for all patients with CC. Therefore, factors related to immunotherapy efficacy in CC patients have become the focus of researchers. High-risk human papillomavirus (HPV) infection is an important factor that drives CC development and affects its progression and prognosis. Increasing attention has been given to the mechanism of the E5, E6 and E7 proteins, which are encoded by the HPV gene, in the occurrence and development of CC and their interaction with programmed cell death ligand-1/programmed cell death-1 (PD-L1/PD-1). Although some preliminary studies have been conducted on these topics, a comprehensive and systematic review of these topics is not available. This review comprehensively summarizes related articles from journals with impact factors greater than 3 and published in the past 5 years; it also reviews studies on the mechanism of HPV and CC, the mechanism of PD-L1/PD-1 axis regulation in CC, and the mechanism by which the interaction between HPV-related oncoproteins and the PD-L1/PD-1 pathway affects the development and prognosis of CC. This study provides theoretical support for the use of immunotherapies for CC, provides a basis for the selection of specific medications that target different HPV-related proteins, and provides a new perspective for the discovery of new immunotherapy targets for CC.
1 Introduction
Cervical cancer (CC) is one of the most dangerous female cancers worldwide, with more than 604 000 cases of CC reported in 2020 (1, 2). Although various screening methods for CC and the availability of human papillomavirus (HPV) vaccines have made this type of cancer largely preventable (2, 3), the incidence of CC has not decreased significantly (4). HPV is thought to be a cause of CC (5). Previous studies have reported that persistent HPV infection might increase the persistence of cervical intraepithelial neoplasia (CIN), subsequently leading to invasive CC (6–8). HPVs are divided into high-risk and low-risk types according to their level of carcinogenicity (8). Many studies have evaluated the correlation between HPV subtypes and CC progression (9–11) and the prevalent species causing the development of CC. The genes encoding HPVs produce proteins that promote viral DNA replication, cell cycle control, and tumorigenesis in the initial stages of infection (12). The E5 and E6/E7 oncogenes have the most pronounced transformation characteristics (13). Moreover, E6 and E7 are the main regulators of virus pathogenicity (14) and exert carcinogenic effects (15). E6 inhibits cell apoptosis by binding to and marking the p53 protein for degradation. This process results in an inability to repair damaged DNA in infected cells, promoting cell proliferation. E7 binds to the retinoblastoma (Rb) protein and inhibits its function, thereby releasing the inhibition of the cell cycle, allowing cells to enter S phase, and promoting cell proliferation (14, 16, 17). Failure to clear HPV infection in a timely manner can lead to the development of CC (18). Various immunotherapies against CC have been tested for their ability to eliminate viral infections and enhance the immune response. Immunotherapy targeting the programmed cell death ligand-1/programmed cell death-1 (PD-L1/PD-1) axis offers new ideas for treating a wide range of advanced human cancers (19).
In contrast to traditional tumour therapies that directly kill tumour cells, immunotherapy activates the immune system to kill tumour cells by overcoming the immunosuppression caused by tumours and the tumour microenvironment (TME). The tumour microenvironment provides abundant tumour antigens for antigen-presenting cells (APCs) to bind, which promotes their maturation (20). Activated APCs stimulate CD8+ T cells to respond to the presented tumour antigens (20, 21). When CD8+ T cells are activated, they discover and kill tumour cells, which are subsequently converted into cytotoxic T lymphocytes (CTLs) (22, 23). Notably, when a recognizable antigen expressing the major histocompatibility complex (MHC) is present, T cells are activated, and cytokine-producing cells are recruited, which triggers the inflammatory response (24). Activation of the PD-1/PD-L1 pathway is a key cause of HPV-associated CC immune escape (25). By interacting with PD-L1, PD-1 activates signalling pathways that inhibit efferent T-cell activity and act as intermediaries that facilitate tumour cell escape from T-cell killing and thus facilitate tumour cell survival (26, 27). PD-1/PD-L1 inhibitors have proven to be major new advances in anticancer drug treatment, and multiple drugs that block the PD-L1/PD-1 axis have been used to treat 13 types of cancer (28). For example, pembrolizumab has been shown to prevent cancer cells from inhibiting T-cell activation and is the only anti-PD-1 drug approved in the US as a second-line treatment for recurrent CC (29). In addition, a single-group phase 2 trial revealed antitumour activity in patients with PD-L1-positive tumours (combined positive score ≥1) but not in patients with PD-L1-negative tumours (30). Moreover, multiple clinical trials are exploring combination treatment strategies involving immunotherapy (30, 31). Studies have shown that when HPV infection worsens and leads to cervical cytopathy, PD-L1 expression is increased (32), suggesting that HPV infection may suppress the body’s immunity and increase resistance to immunotherapy (Figure 1).
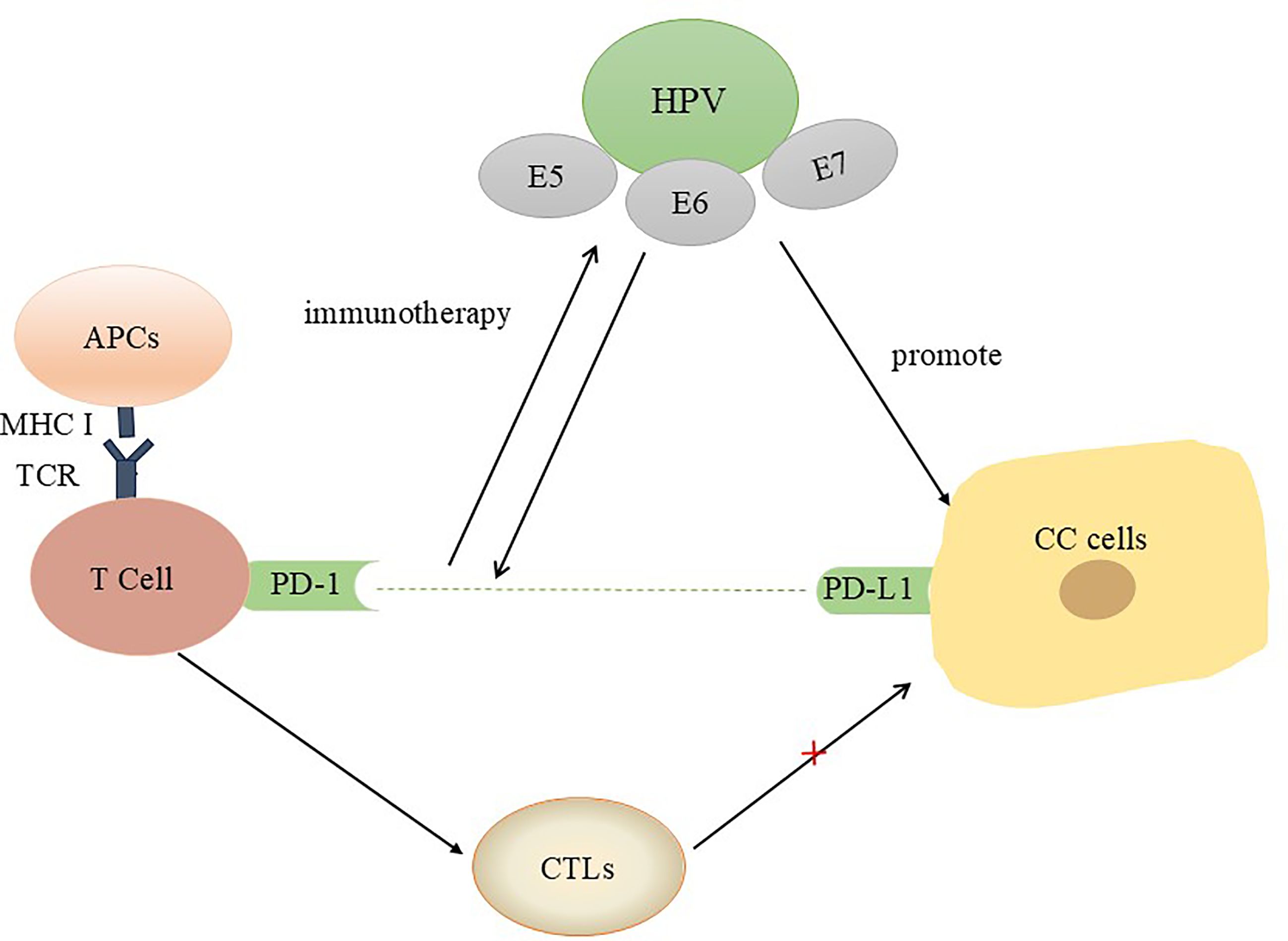
Figure 1. Interactions between HPV oncoproteins and PD-L1/PD-1 in CC patients. In the tumour microenvironment, the interaction between PD-1 and PD-L1 results in the secretion of inhibitory signals and induces T-cell apoptosis. Blocking this pathway is beneficial for activating T cells, reshaping the tumour microenvironment, and preventing immune escape.
This paper reviews a large body of literature and discusses the expression of HPV-related oncoproteins and the important role of HPV in preventing CC progression through PD-L1/PD-1 immunotherapy. This review aims to provide additional insights for future applications of immunotherapy for CC.
2 Signalling pathways associated with major HPV oncoproteins
With continuous in-depth research on the molecular mechanism of HPV carcinogenesis, the strong correlation between the activation of HPV oncogenes and cervical lesions has been further revealed. Among them, the most widely studied HPV oncoproteins are the E5, E6 and E7 oncoproteins.
2.1 E5 protein
Notably, we summarized many new ways in which CC is affected by HPV oncoproteins, mainly E5, E6 and E7. Kim et al. studied the role of E5 in the progression of CC and reported that the HPV16 E5 oncoprotein promotes CC by upregulating cyclooxygenase-2 (COX-2) expression via the EGFR signalling pathway, in which NF-κB and AP-1 play key roles (33). Moreover, E5 induces EGFR signalling, thus increasing the expression of vascular endothelial growth factor (VEGF), which plays an important role in cancer progression (34, 35). The increase in VEGF expression induced by E5 is also mediated by the activation of MEK-ERK1/2 and PI3K/Akt downstream of EGFR (33).
2.2 E6 protein
HPV E6-mediated activation of c-Jun N-terminal kinase (JNK) drives EGFR signalling to promote CC cell proliferation. In this pathway, JNK activated by HPV E6 subsequently phosphorylates and activates c-Jun, which in turn induces the expression of host cell regulatory genes, including EGFR. The pathway is activated when the EGFR-driven signal, in turn, activates JNK. As a result, a complex positive feedback loop is formed in HPV-positive CC cells (36). Furthermore, Shu et al. analysed another regulatory pathway for HPV16 E6, which inhibits p53 transcription by recruiting the coinhibitory factor NCOR1 to activate octamer-binding transcription factor 4 (OCT4) expression (37). Additionally, E6 can promote the degradation of p53 by interacting with the cellular ubiquitin ligase E6AP. This pathway has a neoplastic effect, resulting in escape from cell death (38, 39).
2.3 E6 and E7 proteins
Another study analysed another regulatory pathway of p53 through WB assays to detect the expression of p53. Hypoxia-inducible factor-1 alpha antisense RNA-2 (HIF1A-AS2) was found to significantly induce CC cell apoptosis via the P53/caspase9/caspase3 axis mediated by HPV16 E6/E7 (40). Surprisingly, many other possible therapeutic targets are also regulated by the high-risk HPV oncoproteins E6/E7. For example, in knockdown and overexpressing cell culture models, the role of T-box transcription factor 3 (TBX3) in promoting the proliferation and migration of HPV-positive cells was validated, and the tumour-promoting activity of TBX3 in CC was shown to be influenced by HPV E6 and E7 signalling (41). In addition, using RNA-Seq data, Trujillo-Cirilo et al. confirmed that IL-2 receptor (IL-2R) expression was significantly higher in CC tumours than in normal tissues. The HPV E6 and E7 genes increase the activity of the functional IL-2R on CC cells, promoting the onset of CC (42).
Many microRNAs (miRs), which are noncoding regulatory RNA molecules, interact with high-risk HPV oncoproteins (43, 44). For example, miR-34a may be upregulated in E6/E7-expressing cells to promote the development of CC (45). Additionally, E6/E7 may increase the expression of miR-18a to induce SKT4 expression (46). In contrast, another miR, miR-424, is underexpressed in CC tissues and high-grade cervical intraepithelial neoplasia (47, 48). Interestingly, Hong S et al. reported that miR-424 levels were reduced by a factor of 10 in cells expressing E6 and E7. In addition, they confirmed that high expression of miR-424 led to decreased checkpoint kinase 1 (CHK1) levels. A previous study highlighted the important role of CHK1 activation in the genome using general inhibitors (49). Therefore, HPV E6/E7 indirectly leads to high CHK1 expression by inhibiting miR-424, thus affecting viral replication (50). Furthermore, Olmedo-Nieva, L et al. first reported that E6/E7 inhibited RHO 2 family-interacting cell polarization regulator (RIPOR2) expression and increased PFKFB4 expression. These findings, supported by multiple experimental methods, demonstrated that the E6 oncoprotein inhibits RIPOR2 transcription and promotes its ubiquitination, leading to RIPOR2 downregulation (51). Moreover, in a Mexican cohort, RIPOR2 expression decreased with the progression of precancerous cervical lesions, suggesting that downregulated RIPOR2 expression was closely associated with shorter overall survival (OS) (51).
2.4 E7 protein
Another study revealed that pyruvate kinase M2 (PKM2) is regulated by the E7 oncoprotein to affect the occurrence of CC (52). HPV16 E7 increases the expression of PKM2 and enhances its nonglycolytic function to promote CC growth, which was confirmed by data from The Cancer Genome Atlas (TCGA) and cell models (52).
With the discovery of an increasing number of targets, the role of HPV oncogenic proteins in CC at the molecular level has been confirmed. This finding also reemphasizes the decisive causative role of HPV in CC. The oncogenic proteins E6 and E7 of HPV could influence the process of malignant progression via several common cancer pathways, as shown in Figure 2.
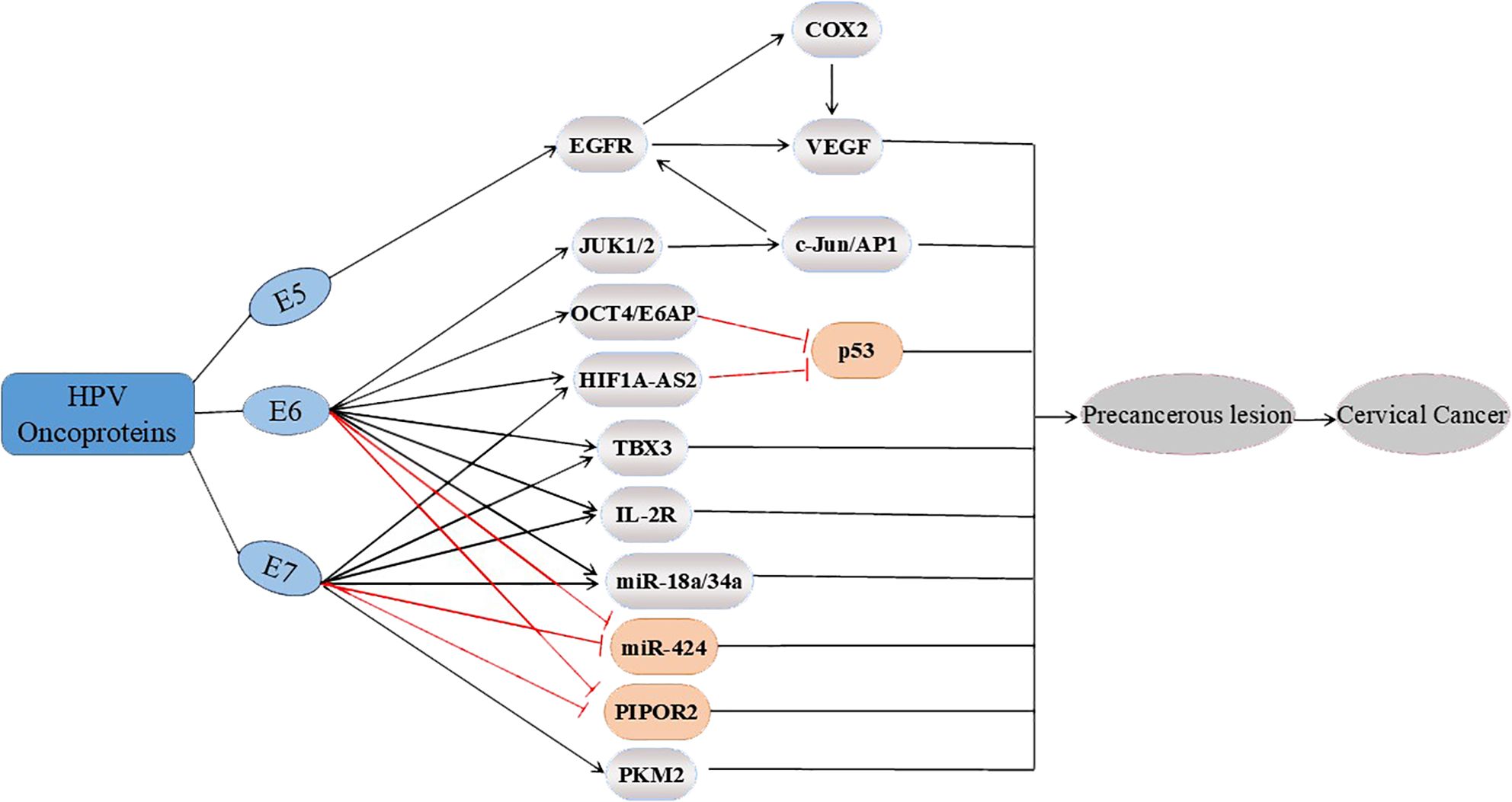
Figure 2. Three major oncoproteins (E5, E6 and E7) affect multiple potential targets involved in the progression of cervical cancer. E5 upregulates EGFR, thereby regulating the expression of COX2 and VEGF; E6 also affects the EGFR pathway by upregulating the expression of JUK1/2 and c-Jun/AP1. In addition, E6 upregulates OCT4/E6AP, and E6 and E7 upregulate HIF1A-AS2, TBX3, IL-2R and miR-18a/34a. OCT4/E6AP and HIF1A-AS2 both affect p53. In addition, E6 and E7 inhibit PIPOR2 and miR-424, whereas E7 upregulates the expression of PKM2, ultimately regulating the progression of cervical cancer.
3 Factors affecting the PD-1/PD-L1 axis in CC
In recent years, breakthroughs in immunotherapy have been achieved in patients with a variety of malignant tumours. Immunotherapy has been used to activate the immune response, which is conducive to the elimination of HPV infection and the early prevention of cancer (53–56). PD-1 and its ligand PD-L1 are immune checkpoints that inhibit tumour-induced immunity (27, 57). PD-1 is found on the surface of diverse immune cells (58). PD-L1 interacts with PD-1 on T lymphocytes and transmits an inhibitory signal (59–61). The inhibition of PD-L1/PD-1 can effectively rescue T cells and improve the prognosis of patients with tumours.
A direct correlation has been reported between elevated PD-L1 expression and shorter overall survival of patients with CC (62). In addition, CC patients who have poor survival outcomes express the PD-L1 protein at high levels; specifically, approximately 96% of tumour samples express PD-L1 (63). In addition, compared with that of PD-1, the expression of PD-L1 can increase in a wider range of cell types in response to inflammatory cytokines and other stimuli. In the TME, PD-L1 expression is regulated by a variety of factors, including inflammatory stimuli and carcinogenic pathways, at the transcriptional, posttranscriptional, and posttranslational levels (64). The therapeutic effects of current immune checkpoint inhibitors can be further enhanced by modulating PD-L1 expression. Exploring the factors affecting the expression of PD-1 and PD-L1 could provide broader ideas for immunotherapy (Table 1).
3.1 SPOP
Nuclear spotted poxvirus and zinc finger protein (SPOP) affects the development of multiple malignancies, including but not limited to lung, colon, stomach, and prostate cancers (65). The downregulation of SPOP helps prevent the metastasis of CC and is beneficial for improving the prognosis of CC patients, as shown through in vivo and in vitro experiments (26). SPOP plays a dual role in CC. SPOP mutations may lead to a decreased ability to degrade the HPV E6 and E7 oncoproteins, thereby increasing genomic stability and allowing cancer cells to survive (66, 67). Additionally, m-IF and HALO analyses revealed that aberrant expression of SPOP reduces the expression of PD-L1 on the cell surface, promoting spatial separation between PD-1 and PD-L1 and thereby promoting immune suppression in the tumour microenvironment (26). CXCL16-related rescue experiments verified that CXCL16 is an intermediate by which SPOP mediates PD-1 immune tolerance (26).
3.2 GSK-3
Glycogen synthase kinase 3 (GSK-3, isoforms α and β) is a serine-threonine kinase associated with tumour growth, cell invasion and metastasis (68). Surprisingly, the PI3K-AKT pathway is involved in the regulatory process of GSK-3, further leading to its inactivation (69). Importantly, Taylor et al. reported that inhibition of the enzyme GSK-3 activates the transcription factor Tbx21 (Tbet), which is responsible for the downregulation of PD-1 (70). Therefore, GSK-3 inhibitors may be widely used to modulate immune responses because of their powerful regulatory effect on PD-1. These findings suggest that GSK-3α/β is a regulatory factor involved in PD-1 transcription.
3.3 MicroRNA-21
MicroRNA-21 (miR-21) inhibits the STAT1 signalling pathway required for the IFN-γ-induced M1 polarization of macrophages by targeting JAK2 and STAT1, which also regulate apoptosis and carcinogenic transformation (71). Therefore, miR-21 depletion enhances the efficacy of PD-1 antibody immunotherapy through the M1 polarization of TAMs (71). In addition, STAT1 signalling has been shown to transcriptionally modulate PD-L1 expression via IFN-γ in head and neck cancers (72). JAK2/STAT1 signalling is a major coregulator of PD-L1 transcription that is driven by the IFN-γ and EGFR pathways (72).
3.4 IL-1β
Xu et al. reported that lactic acid released by tumours can trigger the secretion of IL-1β from Mφs by activating the NLRP3 inflammasome; in turn, Mφ-derived IL-1β suppresses the immune response by activating the NF-κB signalling pathway to drive an increase in PD-L1 levels (73). IL-1β can reduce PD-L1 levels in tumour cells and suppress the infiltration of Mφs in vivo, increasing the antitumour efficacy (73).
3.5 Yin-yang 1
Overexpression of the transcription factor Yin-yang 1 (YY1) is directly or indirectly involved in the regulation of PD-L1 expression. Many signalling pathways, such as the p53 pathway, are involved in the regulation of YY1 and PD-L1 expression (74). Therefore, targeting YY1 directly or through various pathways engaged in crosstalk may lead to the downregulation of PD-L1 expression in tumour cells, thereby enhancing the cell-mediated antitumour response (74).
3.6 DENR
The expression of PD-L1 can be regulated by targeting DENR, which is an RNA-binding protein (RBP) that affects RNA metabolism. Control experiments have shown that in cancer cells lacking DENR expression, the translation of JAK2 and the interferon-γ-JAK-STAT signalling pathway are disrupted, and the level of PD-L1 expression is decreased (75). In summary, DENR is a factor regulating PD-L1 that promotes tumour immune escape. The possibility of DENR as a potential target is an important discovery in immunotherapy.
3.7 TAZ
The Hippo pathway interferes with immune cell function and promotes tumorigenesis (76, 77). Transcriptional regulatory factor 1 (TAZ) plays a role in the Hippo pathway. TAZ mRNA expression was significantly increased in the CC group compared with the control group. In addition, a high level of TAZ in CC increases the activity of the PD-L1 promoter (78). The PD-L1 mRNA level in HeLa cells transfected with the TAZ gene was also significantly increased (78). A statistically significant positive correlation was detected between TAZ and PD-L1 protein expression in CC tissues. TAZ targeting of PD-L1 promotes the proliferation and invasion of cancer cells, and TAZ is a major regulator of PD-L1 expression.
3.8 FAT4
FAT atypical cadherin 4 (FAT4) functions as a tumour suppressor and has been detected on the cell membrane of mammalian cells (79). FAT4 is abnormally expressed in many tumours due to mutation or deletion, especially in squamous cell carcinoma. The antagonistic effect of FAT4 on the nuclear localization of β-catenin inhibits the β-catenin/STT3/PD-L1 signalling pathway, which is necessary for activating antitumour immunity. In addition, FAT4 induces CTL activation after downregulating PD-L1 expression (80). These findings increase our understanding of PD-L1-related regulation in tumours at the molecular level (81).
3.9 TFAP2A
The transcription factor AP−2 alpha (TFAP2A) plays a role in promoting apoptosis in CC. In vitro and in vivo experiments confirmed that the expression of the TFAP2A gene in CC is higher than that in normal people and is related to the tumour stage and local metastasis. IHC staining was performed for 30 normal cervical tissues and 91 CC tissues, and a higher percentage of PD-L1-positive cells was observed in cancer tissues than in normal tissues (82). The binding of TFAP2A to the PD-L1 promoter region is beneficial for its high expression, thereby forming a positive feedback loop during tumour growth. In colon cancer, the PI3K‒AKT pathway has been shown to be an intermediary for positive feedback (83). However, TFAP2A is also a tumour suppressor; for example, it is underexpressed in hepatocellular carcinoma (84). Therefore, additional studies on the dual role of TFAP2A need to be performed.
Due to the complexity of CC progression and the highly challenging nature of the immunotherapy field (85), the discovery of new therapeutic targets may improve the response rate to immunotherapy. Research on the expression of the PD-1/PD-L1 axis in severe viral infections and HPV-induced CC will be a hot topic.
4 Potential roles of HPV oncoproteins in the response to PD-L1/PD-1 immunotherapy
Research on the importance of the PD-L1/PD-1 axis in the aetiology of CC has become increasingly extensive. A study of 25 patients with cervical lesions revealed higher PD-L1 expression in HPV-positive CC patients than in HPV-negative CC patients according to an analysis of a tissue microarray of tumour cores from 25 patients with cervical lesions (P<0.05) (86). In addition, the significant correlation between HPV positivity and high PD-L1 expression has been widely studied (32, 87, 88). HPV oncoproteins affect the expression of PD-L1 through a variety of molecular signals (Figure 3).
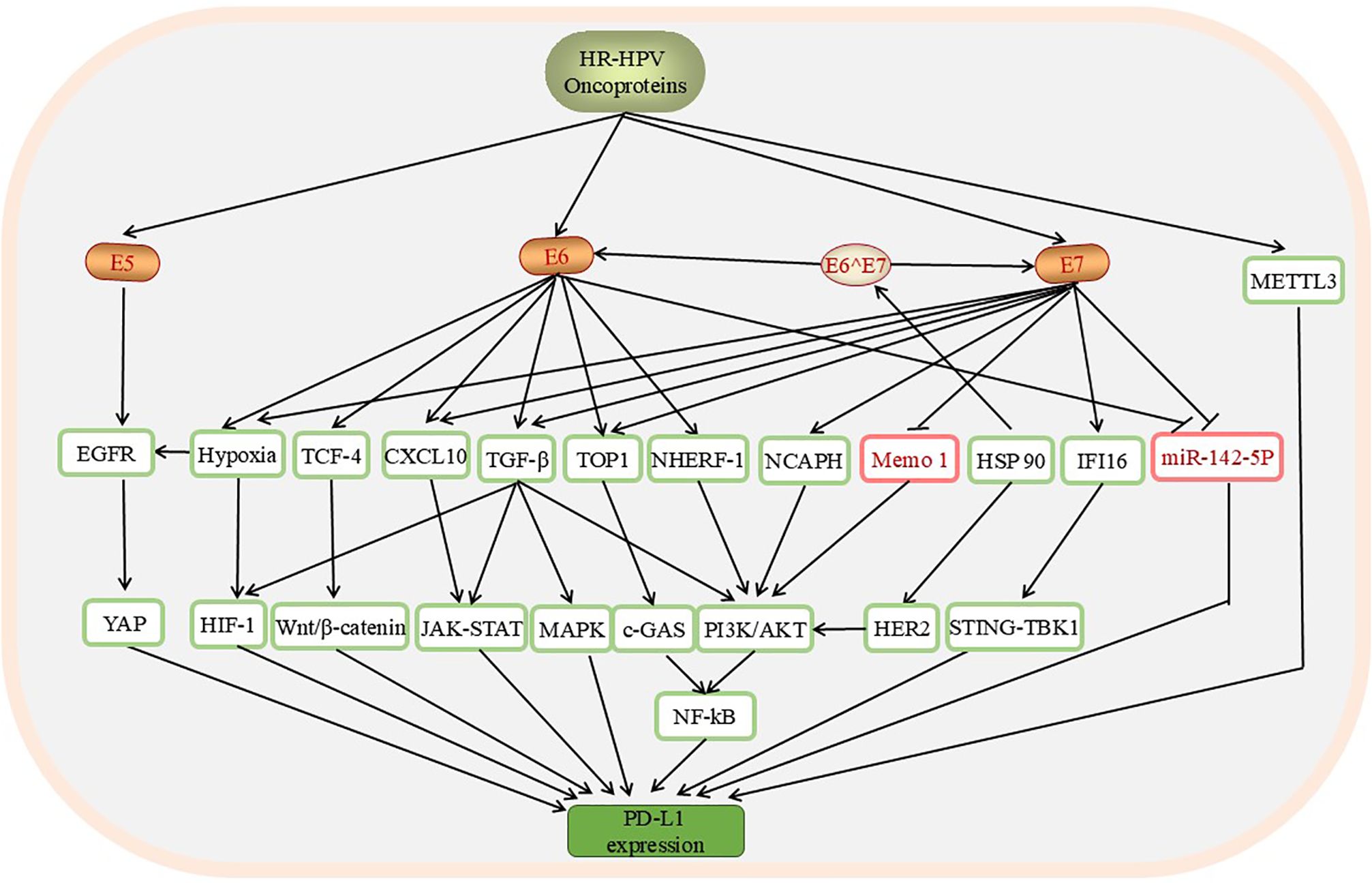
Figure 3. The pathways through which HPV affects the levels of PD-L1/PD-1 in CC include the YAP, HIF-1, Wnt/β-catenin, JAK-STAT, MAPK, c-GAS, PI3K/AKT, and STING-TBK1 pathways. These pathways are involved mainly in promoting PD-L1 mRNA expression. HPV oncoproteins are positive regulators of these downstream factors, except Memo-1, whose expression is downregulated by E7. E6 and E7 inhibit the expression of miR-142-5p, upregulate the expression of PD-L1, and induce the expression of PD-L1. In addition, the upregulation of METTL3 by HPV can increase the expression of PD-L1.
4.1 The E5 oncoprotein
Lee et al. reported that yes-associated protein (YAP) affects the activation of the PD-L1/PD-1 pathway by regulating the transcription of PD-L1 (89). The E5 oncoprotein activates EGFR, which in turn enhances the in vivo regulation of YAP, thereby increasing the therapeutic potential of YAP and illuminating the complex impact of the EGFR–YAP signalling pathway on PD-L1 in CC progression. In summary, therapy targeting this pathway may downregulate PD-L1 expression and may be a potential mechanism for inhibiting T-cell apoptosis and persistent HPV infection (85, 89–92). Interestingly, Ping-Chih Hsu et al. reported that in NSCLC, PD-L1 expression is also regulated by the EGFR pathway. Activating the EGFR kinase domain is beneficial for further inducing PD-L1 expression, possibly through the activation of the Hippo/YAP signalling pathway, which causes T-cell apoptosis (93). These studies could guide studies of the E5 oncoprotein.
4.2 E6 and E7 oncoproteins
The pathways that affect the expression of the PD-L1/PD-1 axis through the E6 and E7 oncoproteins have been studied more extensively.
4.2.1 The HIF-1α pathway
Hypoxia, a physiological stimulus, induces hypoxia inducible factor 2α (HIF-2α) to upregulate the EGFR protein (94, 95). In almost all cancers, the presence of a hypoxic environment increases HIF-1α expression (96, 97). In hypoxic HPV-transformed cells, the expression of the E6 and E7 oncoproteins is downregulated at the transcriptional level via the selective regulation of the PI3K/mTORC2/Akt axis (98–100). More importantly, the E6 and E7 oncogenes increase HIF-1α levels and stability, respectively, in anoxic environments (101). Interestingly, more than one study illustrated that hypoxia increases the expression of PD-L1, and a positive correlation has been observed between HIF-1α and PD-L1 expression in tumours (90, 102, 103).
Under hypoxic conditions, transforming growth factor β (TGF-β) also participates in the HIF-1α signalling pathway and increases the level of PD-L1 in HPV-driven cancers (104). TGF-β can indirectly promote the transcription and upregulation of PD-L1 by activating various non-SMAD-dependent signalling pathways, such as the PI3K/Akt, MAPK/ERK, and JAK/STAT pathways (105). Similarly, in late-stage tumours, the increase in the levels of the HPV E6 and E7 oncoproteins can also activate TGF-β signalling in CC, leading to immunosuppression and tumour progression (106). TGF-β has a dual role. In the early stages of tumour development, E6 and E7 enhancement interferes with the growth inhibition of transformed cells by TGF-β (107). Ultimately, an immunosuppressive microenvironment is established that is conducive to tumour cell escape from the supervision of the immune system. Inhibitors targeting the TGF-β pathway are being used in combination with PD-1/PD-L1 inhibitors to achieve the precise application of immunotherapy. Drugs such as the anti-TGF-β/PD-L1 bispecific antibody YM101 (108) and BITP have seen increased clinical application (109). However, whether HIF-1α-mediated upregulation of PD-L1 is associated with a poor prognosis for CC patients has yet to be investigated.
4.2.2 The Wnt/β-catenin pathway
Overactivation of the Wnt signalling pathway in the progression of CC has been shown to occur in previous studies (110–112). Furthermore, the important role of HPV E6 in this activation pathway has been recognized with the help of cellular models (113). T-cell factor 4 (TCF-4) has been shown to exhibit abnormal mutations in various types of cancer (114). Both E6 and E7 promote Wnt/β-catenin expression and increase TCF-mediated transcription (115, 116). Similarly, Munoz-Bello et al. reported that E6 and E6*I upregulate the TCF-4 transcription factor to regulate the activity of the Wnt/β-catenin pathway, promote the proliferation of cancer cells, induce the stabilization of TCF-4, and help maintain the transformation and immortalization of cancer cells (116). After β-catenin enters the nucleus, it binds to TCF-4, activating the transcription of downstream genes, including MYC (117), which is an important step in driving high PD-L1 expression. Furthermore, both Wnt/β-catenin and PD-L1/PD-1 inactivate CD8+ T-cell function by influencing the c-MYC gene during carcinogenesis and tumour formation, enabling tumour cells to evade the immune system (118). Therefore, inhibition of the Wnt signalling pathway in cancer cells can disrupt PD-L1 expression by affecting MYC signalling, thereby enhancing the immune defence against tumours (119). By targeting various components of the TCF-4-Wnt/β-catenin-MYC-PD-L1 pathway, new diagnostic and therapeutic strategies can be developed to enhance the immune defence against tumours.
4.2.3 The JAK-STAT pathway
CXC motif chemokine 10 (CXCL10) is an important mediator of cancer intracellular signalling pathways and cell transport (120). CXCL10 is secreted by CC cells after HPV infection and subsequently binds to CXCR3 in surrounding fibroblasts (121). Along with the activation of the JAK-STAT pathway, the CXCL10-CXCR3 axis increases extracellular PD-L1 levels (86). These mechanisms may promote virus incubation and help HPVs escape the immune response. Moreover, in gene-edited cells, knocking out E6/E7 leads to a significant reduction in CXCL10 expression, indicating the regulatory effect of HPV E6/E7 on CXCL10 expression in human cervical cells (86, 122). Furthermore, another study revealed that the serum CXCL10 concentration is significantly higher in patients than in healthy controls, which is consistent with previous conclusions (123). Therefore, CXCL10 may be a predictive biomarker for diagnosing CC.
4.2.4 The c-GAS pathway
The upregulation of topoisomerase I (TOP1) triggers DNA repair, acting as a key DDR protein (124). In CC, TOP1 promotes an increase in NF-κB expression by regulating cyclic GMP-AMP synthase (c-GAS), which is an important step in increasing PD-L1 expression. Conversely, a low level of TOP1 inhibits tumour cell growth. Therefore, TOP1 and c-GAS together form a signalling pathway that promotes tumour cell growth (125, 126). Interestingly, the HPV oncogenic protein E6 has been shown to regulate c-GAS (127). Luo et al. further highlighted the roles of the oncogenic proteins E6 and E7 in increasing TOP1 expression. The use of specific shRNAs to suppress the expression of the oncogenic proteins HPV E6 and E7 in CC cells led to the inhibition of TOP1 and PD-L1, with E7 resulting in a greater response rate. Additionally, co-IP experiments revealed that E6 and E7 can promote the interaction between cGAS and TOP1, leading to the accumulation of the PD-L1 protein in cervical cancer patients (128). Furthermore, studies have confirmed that the inhibition of TOP1, such as with topotecan (TPT) or camptothecin (CPT), has antitumour effects (124), driving the activation of the cGAS-NF-κB-PD-L1 pathway in cancer therapy.
4.2.5 The PI3K/AKT pathway
In HPV-induced cancers, NF-κB induces an increase in PD-L1/PD-1 expression, and PI3K/AKT signalling is activated by the E6 and E7 genes (129–131). The continuous NF-κB and PI3K/AKT pathways connect HPV oncogenes with the PD-L1/PD-1 pathway. In vitro, the association between the loss of Na+/H+ exchanger regulatory factor-1 (NHERF-1) in CC and ERK signalling stimulated by EGFR has been preliminarily verified (132, 133). Moreover, persistent activation of EGFR by NHERF-1 also results in a poor prognosis for CC patients (133). Moreover, NHERF1 is degraded by E6 and E7, which triggers the PI3K/AKT pathway (134). The direct binding of E2F1 to the nonstructural maintenance of chromosomes (SMC) condensin I complex subunit H (NCAPH) gene promoter provides evidence that E7 increases the expression of the NCAPH gene. When the expression of NCAPH decreases, the expression of PDK1 decreases, which indicates that the activation of the AKT pathway by NCAPH may depend on the upregulation of PDK1 expression (135). Furthermore, NCAPH can transmodulate the transcription of E7 (135).
Unlike NCAPH, Trejo-Cerro et al. discovered the effect of HPV16 E7 on Memo1 through proteomic research and reported that the inhibition of Memo1 promoted the growth of HPV-positive cervical cancer cells, which was accompanied by the activation of the Akt pathway (136). Moreover, the effects of heat shock protein 90 (HSP90) overexpression on E6/E7 cells have been widely analysed. Specifically, HSP90 does not interact directly with E6/E7 but rather stabilizes the viral proteins E6/E7 to exert its effects (137). Furthermore, by exploring the expression pattern of HSP90, Zeng, J et al. confirmed the inhibitory effect of HSP90 knockdown on the proliferation of CaSki and SiHa cells (138). Notably, these findings are consistent with those of previous studies in this field (139). Notably, the downregulation of HSP90 inhibits the HER2/PI3K/AKT pathway, thereby effectively inhibiting the proliferation and migration of HPV16+ cancer cells (138).
4.2.6 The STING-TBK1 pathway
Interferon-gamma inducible factor 16 (IFI16) is an essential protein involved in DNA virus infection that stimulates cGAMP expression (140, 141). HPV E7 facilitates the ubiquitin‒proteasome-mediated degradation of IFI16 through the E3 ligase TRIM21, inhibiting pyroptosis during infection (142). Furthermore, Cai, H et al. injected SiHa cells into NOD/SCID mice to establish tumour xenografts and verify the roles of PD-L1 and IFI16 in CC development. Both PD-L1 and IFI16 knockdown significantly suppressed the growth of SiHa-derived tumours in vivo (143). The expression and distribution of IFI16 were analysed using different methods, such as western blotting. The cytoplasmic translocation of IFI16 was affected by IFN-γ stimulation, and IFI16 participated in STING pathway activation through this pathway (144). IFI16 activated STING-TBK1-mediated immunoregulation and the downstream NF-κB pathway, which upregulated PD-L1 in the immune microenvironment, thus promoting CC progression (143). Therefore, STING-TBK1 may constitute a new pathway to inhibit the metastasis of CC cells, which is worth exploring.
4.2.7 MicroRNAs regulate the PD-L1/PD-1 axis
Researchers assume that microRNAs are intermediaries that regulate the expression of other genes. In addition to the miRNAs mentioned above, in recent studies, miR-142-5p has been found to act as a tumour suppressor in CC, mediating the regulatory effect of E6/E7 on PD-L1 (145, 146). The inverse relationship between miR-142-5p and PD-L1 expression was confirmed by the transfection of the E6 and E7 oncoproteins (146). In addition, the upregulation of miR-142-5pweakens the effect of E6/E7 on PD-L1 and inhibits the occurrence of tumours. These results were further confirmed via in vivo experiments (146). Therefore, using miR-142-5p as a tumour suppressor to increase the efficacy of tumour immunotherapy is feasible.
4.2.8 Possible factors related to PD-L1 expression in CC
Interestingly, another target that directly affects HPV infection and PD-L1 expression was identified. METTL3 plays an immunomodulatory role in HPV-associated cancers by inhibiting the infiltration of immune cells (147). METTL3, a key regulator of N6-adenosine methylation (m6A), is associated with the HPV status and expression in tumours and is correlated with a poor prognosis (147). A high level of METTL3 promotes the formation of an immunosuppressive tumour microenvironment (147, 148). Additionally, high levels of METTL3 and YTHDF1 may lead to a poor prognosis for patients with CC (149, 150). Moreover, METTL3 accelerates the Warburg effect or aerobic glycolysis (149). Further research by Ji H et al. revealed that METTL3 increased HK2 protein expression by increasing the stability of the HK2 mRNA, thus accelerating glycolysis. Notably, the expression of PD-L1 in CC was negatively correlated with the expression of ALKBH5, FTO, METTL3, RBM15B, YTHDF1, YTHDF3, and ZC3H1 (148). This study has several limitations. For example, how HPV infection affects METTL3 expression remains to be further elucidated. Thus, additional possible interactions between HPV oncoproteins and PD-L1 are worth exploring.
Building on previous research, the continuous enrichment of pathways such as the YAP, HIF-1, Wnt/β-catenin, JAK-STAT, PI3K/AKT, and STING-TBK1 pathways provides new perspectives for trends in personalized immunotherapy and holds promise for addressing the issue of low response rates to immunotherapy.
5 Conclusions
HPV infection is one of the important factors leading to the occurrence of CC, particularly high-risk HPV types 16/18, which are the most common. Continuous viral infection is a driving factor in tumour progression, and the HPV oncoproteins E6/E7 can evade host immune surveillance in various ways, ultimately leading to cancer. Numerous studies have confirmed that the expression of PD-L1/PD-1 is related to the treatment and prognosis of CC. However, verification of whether the expression of PD-L1/PD-1 is associated with infection with different types of HPV related to CC is urgently needed. Therefore, understanding the many unelucidated pathogenic mechanisms of HPV, the untapped potential of the PD-L1/PD-1 axis, and the impact of high-risk HPV oncoproteins on the PD-L1/PD-1 pathway is crucial for guiding clinical decisions in selecting effective immunotherapy options for patients with CC. For this purpose, this paper summarizes the high-quality literature published in the past 5 years and elaborates in detail how the E5, E6 and E7 oncoproteins affect the expression of PD-L1 through YAP, HIF-1, Wnt/β-catenin, JAK-STAT, PI3K/AKT and STINT-Tbk1 signalling and promote the occurrence of CC. EGFR, hypoxia, TCF-4, CXCL10, NHERF-1, NCAPH, HSP90, IFI16 and other targets involved in these pathways are positively regulated by oncoproteins. In particular, the Memo1 and miR-142-5p regulatory factors are negatively regulated by the E7 oncoprotein. Targeting or knocking out these factors can effectively hinder the progression of CC cells, significantly enhancing the effect of immunotherapy. Moreover, the expression of PD-L1 itself might predict the clinical outcomes of CC patients. Therefore, we believe that infection with different high-risk HPV types leads to differences in the sensitivity of patients with CC to PD-1/PD-L1 immunotherapy, which provides a reference for individualized and specific clinical treatment and a basis for the development of more effective immune checkpoint inhibitors. Elucidating the roles of different regulatory pathways can help evaluate therapeutic effects and facilitate the prediction of whether the use of a specific immunotherapeutic drug will be effective. Moreover, immunotherapies are expected to largely avoid immune-related side effects and overcome resistance mechanisms. Although the current research is limited, HPV-positive patients are undoubtedly more likely to benefit from the precise application of immunotherapy. The identification of an increasing number of pathways has expanded our understanding of HPV-related CC and, more importantly, strongly validated that immunotherapy may have a wider range of antitumour applications in the future.
Author contributions
JiL: Conceptualization, Writing – original draft. YM: Resources, Writing – original draft. QW: Investigation, Writing – review & editing. PP: Formal Analysis, Writing – review & editing. JuL: Writing – review & editing. XX: Supervision, Writing – review & editing.
Funding
The author(s) declare that no financial support was received for the research, authorship, and/or publication of this article.
Conflict of interest
The authors declare that the research was conducted in the absence of any commercial or financial relationships that could be construed as a potential conflict of interest.
Publisher’s note
All claims expressed in this article are solely those of the authors and do not necessarily represent those of their affiliated organizations, or those of the publisher, the editors and the reviewers. Any product that may be evaluated in this article, or claim that may be made by its manufacturer, is not guaranteed or endorsed by the publisher.
References
1. Arbyn M, Weiderpass E, Bruni L, de Sanjose S, Saraiya M, Ferlay J, et al. Estimates of incidence and mortality of cervical cancer in 2018: a worldwide analysis. Lancet Glob Health. (2020) 8:e191–203. doi: 10.1016/S2214-109X(19)30482-6
2. Cohen PA, Jhingran A, Oaknin A, Denny L. Cervical cancer. Lancet. (2019) 393:169–82. doi: 10.1016/S0140-6736(18)32470-X
3. The Lancet Infectious D. A blueprint for elimination of cervical cancer. Lancet Infect Dis. (2024) 24:1. doi: 10.1016/s1473-3099(23)00764-8
4. Fontham ETH, Wolf AMD, Church TR, Etzioni R, Flowers CR, Herzig A, et al. Cervical cancer screening for individuals at average risk: 2020 guideline update from the American Cancer Society. CA Cancer J Clin. (2020) 70:321–46. doi: 10.3322/caac.21628
5. Hansen BT, Campbell S, Nygard M. Regional differences in cervical cancer incidence and associated risk behaviors among Norwegian women: a population-based study. BMC Cancer. (2021) 21:935. doi: 10.1186/s12885-021-08614-w
6. Burk RD, Chen ZG, Saller C, Tarvin K, Carvalho AL, Scapulatempo-Neto C, et al. Integrated genomic and molecular characterization of cervical cancer. Nature. (2017) 543:378–84. doi: 10.1038/nature21386
7. Quist KM, Solorzano I, Wendel SO, Chintala S, Wu C, Wallace NA, et al. Cervical cancer development: implications of HPV16 E6E7-NFX1-123 regulated genes. Cancers (Basel). (2021) 13(24):6182. doi: 10.3390/cancers13246182
8. Kombe AJK, Zoa-Assoumou S, Bounda GA, Nsole-Biteghe FA, Jin T, Zoure AA. Advances in etiopathological role and control of HPV in cervical cancer oncogenesis. Front Biosci (Landmark Ed). (2023) 28:245. doi: 10.31083/j.fbl2810245
9. Chaturvedi AK. Beyond cervical cancer: burden of other HPV-related cancers among men and women. J Adolesc Health. (2010) 46:S20–26. doi: 10.1016/j.jadohealth.2010.01.016
10. Tian R, Liu J, Fan W, Li R, Cui Z, Jin Z, et al. Gene knock-out chain reaction enables high disruption efficiency of HPV18 E6/E7 genes in cervical cancer cells. Mol Ther Oncolytics. (2022) 24:171–9. doi: 10.1016/j.omto.2021.12.011
11. Fani M, Mahmoodi P, Emadzadeh M, Avan A, Karimi E, Ferns GA, et al. Correlation of human papillomavirus 16 and 18 with cervical cancer and their diagnosis methods in Iranian women: A systematic review and meta-analysis. Curr Probl Cancer. (2020) 44:100493. doi: 10.1016/j.currproblcancer.2019.06.008
12. Chen B, Zhao L, Yang R, Xu T. Advances in molecular mechanism of HPV16 E5 oncoprotein carcinogenesis. Arch Biochem Biophys. (2023) 745:109716. doi: 10.1016/j.abb.2023.109716
13. Vallejo-Ruiz V, Gutiérrez-Xicotencatl L, Medina-Contreras O, Lizano M. Molecular aspects of cervical cancer: a pathogenesis update. Front Oncol. (2024) 14:1356581. doi: 10.3389/fonc.2024.1356581
14. Singini MG, Singh E, Bradshaw D, Ramaliba T, Chen WC, Motlhale M, et al. Usefulness of high-risk HPV early oncoprotein (E6 and E7) serological markers in the detection of cervical cancer: A systematic review and meta-analysis. J Med Virol. (2022) 95:e27900. doi: 10.1002/jmv.27900
15. Guo N, Niu Z, Yan Z, Liu W, Shi L, Li C, et al. Immunoinformatics design and in vivo immunogenicity evaluation of a conserved CTL multi-epitope vaccine targeting HPV16 E5, E6, and E7 proteins. Vaccines. (2024) 12:392. doi: 10.3390/vaccines12040392
16. Bhattacharjee R, Das SS, Biswal SS, Nath A, Das D, Basu A, et al. Mechanistic role of HPV-associated early proteins in cervical cancer: Molecular pathways and targeted therapeutic strategies. Crit Rev Oncology/Hematology. (2022) 174:103675. doi: 10.1016/j.critrevonc.2022.103675
17. Wang Y, Li G. PD-1/PD-L1 blockade in cervical cancer: current studies and perspectives. Front Med. (2019) 13:438–50. doi: 10.1007/s11684-018-0674-4
18. Ye J, Zheng L, He Y, Qi X. Human papillomavirus associated cervical lesion: pathogenesis and therapeutic interventions. MedComm (2020). (2023) 4:e368. doi: 10.1002/mco2.368
19. Attademo L, Tuninetti V, Pisano C, Cecere SC, Di Napoli M, Tambaro R, et al. Immunotherapy in cervix cancer. Cancer Treat Rev. (2020) 90:102088. doi: 10.1016/j.ctrv.2020.102088
20. Jiang X, Wang J, Deng X, Xiong F, Ge J, Xiang B, et al. Role of the tumor microenvironment in PD-L1/PD-1-mediated tumor immune escape. Mol Cancer. (2019) 18:10. doi: 10.1186/s12943-018-0928-4
21. Huang Q, Wu X, Wang Z, Chen X, Wang L, Lu Y, et al. The primordial differentiation of tumor-specific memory CD8(+) T cells as bona fide responders to PD-1/PD-L1 blockade in draining lymph nodes. Cell. (2022) 185 4049–4066:e4025. doi: 10.1016/j.cell.2022.09.020
22. Awadasseid A, Zhou Y, Zhang K, Tian K, Wu Y, Zhang W. Current studies and future promises of PD-1 signal inhibitors in cervical cancer therapy. BioMed Pharmacother. (2023) 157:114057. doi: 10.1016/j.biopha.2022.114057
23. Wu Y, Ye S, Goswami S, Pei X, Xiang L, Zhang X, et al. Clinical significance of peripheral blood and tumor tissue lymphocyte subsets in cervical cancer patients. BMC Cancer. (2020) 20:173. doi: 10.1186/s12885-020-6633-x
24. Mahoney KM, Rennert PD, Freeman GJ. Combination cancer immunotherapy and new immunomodulatory targets. Nat Rev Drug Discovery. (2015) 14:561–84. doi: 10.1038/nrd4591
25. Hrudka J, Hojný J, Prouzová Z, Kendall Bártů M, Čapka D, Zavillová N, et al. High tumour mutational burden is associated with strong PD-L1 expression, HPV negativity, and worse survival in penile squamous cell carcinoma: an analysis of 165 cases. Pathology. (2024) 56:357–66. doi: 10.1016/j.pathol.2023.10.010
26. Wu J, Wu Y, Guo Q, Chen S, Wang S, Wu X, et al. SPOP promotes cervical cancer progression by inducing the movement of PD-1 away from PD-L1 in spatial localization. J Transl Med. (2022) 20:384. doi: 10.1186/s12967-022-03574-6
27. Han Y, Liu D, Li L. PD-1/PD-L1 pathway: current researches in cancer. Am J Cancer Res. (2020) 10:727–42.
28. Guo Z, Yu J, Chen Z, Chen S, Wang L. Immunological mechanisms behind anti-PD-1/PD-L1 immune checkpoint blockade: intratumoral reinvigoration or systemic induction? Biomedicines. (2024) 12:764. doi: 10.3390/biomedicines12040764
29. Colombo N, Dubot C, Lorusso D, Caceres MV, Hasegawa K, Shapira-Frommer R, et al. Pembrolizumab for persistent, recurrent, or metastatic cervical cancer. N Engl J Med. (2021) 385:1856–67. doi: 10.1056/NEJMoa2112435
30. Tewari KS, Monk BJ, Vergote I, Miller A, de Melo AC, Kim HS, et al. Survival with cemiplimab in recurrent cervical cancer. N Engl J Med. (2022) 386:544–55. doi: 10.1056/NEJMoa2112187
31. Xu Q, Wang J, Sun Y, Lin Y, Liu J, Zhuo Y, et al. Efficacy and safety of sintilimab plus anlotinib for PD-L1-positive recurrent or metastatic cervical cancer: A multicenter, single-arm, prospective phase II trial. J Clin Oncol. (2022) 40:1795–805. doi: 10.1200/JCO.21.02091
32. Yang-Chun F, Zhen-Zhen C, Yan-Chun H, Xiu-Min M. Association between PD-L1 and HPV status and the prognostic value for HPV treatment in premalignant cervical lesion patients. Med (Baltimore). (2017) 96:e7270. doi: 10.1097/MD.0000000000007270
33. Kim SH, Juhnn YS, Kang S, Park SW, Sung MW, Bang YJ, et al. Human papillomavirus 16 E5 up-regulates the expression of vascular endothelial growth factor through the activation of epidermal growth factor receptor, MEK/ ERK1,2 and PI3K/Akt. Cell Mol Life Sci. (2006) 63:930–8. doi: 10.1007/s00018-005-5561-x
34. Kim SH, Oh JM, No JH, Bang YJ, Juhnn YS, Song YS. Involvement of NF-kappaB and AP-1 in COX-2 upregulation by human papillomavirus 16 E5 oncoprotein. Carcinogenesis. (2009) 30:753–7. doi: 10.1093/carcin/bgp066
35. Wasson CW, Morgan EL, Muller M, Ross RL, Hartley M, Roberts S, et al. Human papillomavirus type 18 E5 oncogene supports cell cycle progression and impairs epithelial differentiation by modulating growth factor receptor signalling during the virus life cycle. Oncotarget. (2017) 8:103581–600. doi: 10.18632/oncotarget.21658
36. Morgan EL, Scarth JA, Patterson MR, Wasson CW, Hemingway GC, Barba-Moreno D, et al. E6-mediated activation of JNK drives EGFR signalling to promote proliferation and viral oncoprotein expression in cervical cancer. Cell Death Differ. (2021) 28:1669–87. doi: 10.1038/s41418-020-00693-9
37. Shu S, Li Z, Liu L, Ying X, Zhang Y, Wang T, et al. HPV16 E6-Activated OCT4 Promotes Cervical Cancer Progression by Suppressing p53 Expression via Co-Repressor NCOR1. Front Oncol. (2022) 12:900856. doi: 10.3389/fonc.2022.900856
38. Soumia M, Hajji H, El Mzibri M, Younes FZ, Mohammed B, Mohamed B, et al. In-silico molecular modeling studies to identify novel potential inhibitors of HPV E6 protein. Vaccines (Basel). (2022) 10:1452. doi: 10.3390/vaccines10091452
39. Celegato M, Messa L, Bertagnin C, Mercorelli B, Loregian A. Targeted disruption of E6/p53 binding exerts broad activity and synergism with paclitaxel and topotecan against HPV-transformed cancer cells. Cancers (Basel). (2021) 14:193. doi: 10.3390/cancers14010193
40. Guan HM, Li WQ, Liu J, Zhou JY. LncRNA HIF1A-AS2 modulated by HPV16 E6 regulates apoptosis of cervical cancer cells via P53/caspase9/caspase3 axis. Cell Signal. (2022) 97:110390. doi: 10.1016/j.cellsig.2022.110390
41. Khan SF, Burmeister CA, Scott DJ, Sinkala M, Ramburan A, Wu HT, et al. TBX3 promotes cervical cancer proliferation and migration via HPV E6 and E7 signaling. Mol Cancer Res. (2023) 21:345–58. doi: 10.1158/1541-7786.MCR-22-0598
42. Trujillo-Cirilo L, Torres-Corioriles EI, Rangel-Corona R, Corona-Ortega MT, Weiss-Steider B. Evidence that the viral oncoproteins E6 and E7 of HPV induce the expression of a functional IL-2R on cervical cancer cells. Cytokine. (2021) 148:155592. doi: 10.1016/j.cyto.2021.155592
43. Satapathy S, Batra J, Jeet V, Thompson EW, Punyadeera C. MicroRNAs in HPV associated cancers: small players with big consequences. Expert Rev Mol Diagnostics. (2017) 17:711–22. doi: 10.1080/14737159.2017.1339603
44. Di Martino MT, Arbitrio M, Caracciolo D, Cordua A, Cuomo O, Grillone K, et al. miR-221/222 as biomarkers and targets for therapeutic intervention on cancer and other diseases: A systematic review. Mol Ther Nucleic Acids. (2022) 27:1191–224. doi: 10.1016/j.omtn.2022.02.005
45. Liu M, Wang W, Chen H, Lu Y, Yuan D, Deng Y, et al. miR-9, miR-21, miR-27b, and miR-34a Expression in HPV16/58/52-Infected Cervical Cancer. BioMed Res Int. (2020) 2020:2474235. doi: 10.1155/2020/2474235
46. Morgan EL, Patterson MR, Ryder EL, Lee SY, Wasson CW, Harper KL, et al. MicroRNA-18a targeting of the STK4/MST1 tumour suppressor is necessary for transformation in HPV positive cervical cancer. PloS Pathog. (2020) 16:e1008624. doi: 10.1371/journal.ppat.1008624
47. Xu J, Li Y, Wang F, Wang X, Cheng B, Ye F, et al. Suppressed miR-424 expression via upregulation of target gene Chk1 contributes to the progression of cervical cancer. Oncogene. (2013) 32:976–87. doi: 10.1038/onc.2012.121
48. Tian Q, Li Y, Wang F, Li Y, Xu J, Shen Y, et al. MicroRNA detection in cervical exfoliated cells as a triage for human papillomavirus-positive women. J Natl Cancer Inst. (2014) 106:dju241. doi: 10.1093/jnci/dju241
49. Hong S, Cheng S, Iovane A, Laimins LA. STAT-5 regulates transcription of the topoisomerase IIbeta-binding protein 1 (TopBP1) gene to activate the ATR pathway and promote human papillomavirus replication. mBio. (2015) 6:e02006–02015. doi: 10.1128/mBio.02006-15
50. Hong S, Cheng S, Songock W, Bodily J, Laimins LA. Suppression of microRNA 424 levels by human papillomaviruses is necessary for differentiation-dependent genome amplification. J Virol. (2017) 91:e01712-17. doi: 10.1128/JVI.01712-17
51. Olmedo-Nieva L, Munoz-Bello JO, Martinez-Ramirez I, Martinez-Gutierrez AD, Ortiz-Pedraza Y, Gonzalez-Espinosa C, et al. RIPOR2 expression decreased by HPV-16 E6 and E7 oncoproteins: an opportunity in the search for prognostic biomarkers in cervical cancer. Cells. (2022) 11:3942. doi: 10.3390/cells11233942
52. Lee SA, Ho C, Troxler M, Lin CY, Chung SH. Non-metabolic functions of PKM2 contribute to cervical cancer cell proliferation induced by the HPV16 E7 oncoprotein. Viruses. (2021) 13:433. doi: 10.3390/v13030433
53. Haddad RI, Seiwert TY, Chow LQM, Gupta S, Weiss J, Gluck I, et al. Influence of tumor mutational burden, inflammatory gene expression profile, and PD-L1 expression on response to pembrolizumab in head and neck squamous cell carcinoma. J Immunother Cancer. (2022) 10:e003026. doi: 10.1136/jitc-2021-003026
54. Li H, Zhu SW, Zhou JJ, Chen DR, Liu J, Wu ZZ, et al. Tertiary lymphoid structure raises survival and immunotherapy in HPV(-) HNSCC. J Dent Res. (2023) 102:678–88. doi: 10.1177/00220345231151685
55. Dorta-Estremera S, Hegde VL, Slay RB, Sun R, Yanamandra AV, Nicholas C, et al. Targeting interferon signaling and CTLA-4 enhance the therapeutic efficacy of anti-PD-1 immunotherapy in preclinical model of HPV(+) oral cancer. J Immunother Cancer. (2019) 7:252. doi: 10.1186/s40425-019-0728-4
56. Galvis MM, Borges GA, Oliveira TB, Toledo IP, Castilho RM, Guerra ENS, et al. Immunotherapy improves efficacy and safety of patients with HPV positive and negative head and neck cancer: A systematic review and meta-analysis. Crit Rev Oncol Hematol. (2020) 150:102966. doi: 10.1016/j.critrevonc.2020.102966
57. Yi M, Zheng X, Niu M, Zhu S, Ge H, Wu K. Combination strategies with PD-1/PD-L1 blockade: current advances and future directions. Mol Cancer. (2022) 21:28. doi: 10.1186/s12943-021-01489-2
58. Agata Y, Kawasaki A, Nishimura H, Ishida Y, Tsubata T, Yagita H, et al. Expression of the PD-1 antigen on the surface of stimulated mouse T and B lymphocytes. Int Immunol. (1996) 8:765–72. doi: 10.1093/intimm/8.5.765
59. Keir ME, Butte MJ, Freeman GJ, Sharpe AH. PD-1 and its ligands in tolerance and immunity. Annu Rev Immunol. (2008) 26:677–704. doi: 10.1146/annurev.immunol.26.021607.090331
60. Fife BT, Pauken KE, Eagar TN, Obu T, Wu J, Tang Q, et al. Interactions between PD-1 and PD-L1 promote tolerance by blocking the TCR-induced stop signal. Nat Immunol. (2009) 10:1185–92. doi: 10.1038/ni.1790
61. Dong Y, Sun Q, Zhang X. PD-1 and its ligands are important immune checkpoints in cancer. Oncotarget. (2017) 8:2171–86. doi: 10.18632/oncotarget.13895
62. Zhang L, Zhao Y, Tu Q, Xue X, Zhu X, Zhao KN. The roles of programmed cell death ligand-1/ programmed cell death-1 (PD-L1/PD-1) in HPV-induced cervical cancer and potential for their use in blockade therapy. Curr Med Chem. (2021) 28:893–909. doi: 10.2174/0929867327666200128105459
63. Enwere EK, Kornaga EN, Dean M, Koulis TA, Phan T, Kalantarian M, et al. Expression of PD-L1 and presence of CD8-positive T cells in pre-treatment specimens of locally advanced cervical cancer. Mod Pathol. (2017) 30:577–86. doi: 10.1038/modpathol.2016.221
64. Yi M, Niu M, Xu L, Luo S, Wu K. Regulation of PD-L1 expression in the tumor microenvironment. J Hematol Oncol. (2021) 14:10. doi: 10.1186/s13045-020-01027-5
65. Song Y, Xu Y, Pan C, Yan L, Wang ZW, Zhu X. The emerging role of SPOP protein in tumorigenesis and cancer therapy. Mol Cancer. (2020) 19:2. doi: 10.1186/s12943-019-1124-x
66. Byun B, Tak H, Joe CO. BTB/POZ domain of speckle-type POZ protein (SPOP) confers proapoptotic function in HeLa cells. Biofactors. (2007) 31:165–9. doi: 10.1002/biof.5520310303
67. Pang K, Lee J, Kim J, Park J, Park Y, Hong E, et al. Degradation of DRAK1 by CUL3/SPOP E3 Ubiquitin ligase promotes tumor growth of paclitaxel-resistant cervical cancer cells. Cell Death Dis. (2022) 13:169. doi: 10.1038/s41419-022-04619-w
68. Shaw G, Cavalcante L, Giles FJ, Taylor A. Elraglusib (9-ING-41), a selective small-molecule inhibitor of glycogen synthase kinase-3 beta, reduces expression of immune checkpoint molecules PD-1, TIGIT and LAG-3 and enhances CD8+ T cell cytolytic killing of melanoma cells. J Hematol Oncol. (2022) 15:134. doi: 10.1186/s13045-022-01352-x
69. Krueger J, Rudd CE, Taylor A. Glycogen synthase 3 (GSK-3) regulation of PD-1 expression and and its therapeutic implications. Semin Immunol. (2019) 42:101295. doi: 10.1016/j.smim.2019.101295
70. Taylor A, Harker JA, Chanthong K, Stevenson PG, Zuniga EI, Rudd CE. Glycogen synthase kinase 3 inactivation drives T-bet-mediated downregulation of co-receptor PD-1 to enhance CD8+ Cytolytic T cell responses. Immunity. (2016) 44:274–86. doi: 10.1016/j.immuni.2016.01.018
71. Xi J, Huang Q, Wang L, Ma X, Deng Q, Kumar M, et al. miR-21 depletion in macrophages promotes tumoricidal polarization and enhances PD-1 immunotherapy. Oncogene. (2018) 37:3151–65. doi: 10.1038/s41388-018-0178-3
72. Concha-Benavente F, Srivastava RM, Trivedi S, Lei Y, Chandran U, Seethala RR, et al. Identification of the cell-intrinsic and -extrinsic pathways downstream of EGFR and IFNgamma that induce PD-L1 expression in head and neck cancer. Cancer Res. (2016) 76:1031–43. doi: 10.1158/0008-5472.CAN-15-2001
73. Xu C, Xia Y, Zhang BW, Drokow EK, Li HY, Xu S, et al. Macrophages facilitate tumor cell PD-L1 expression via an IL-1beta-centered loop to attenuate immune checkpoint blockade. MedComm (2020). (2023) 4:e242. doi: 10.1002/mco2.242
74. Hays E, Bonavida B. YY1 regulates cancer cell immune resistance by modulating PD-L1 expression. Drug Resist Update. (2019) 43:10–28. doi: 10.1016/j.drup.2019.04.001
75. Chen B, Hu J, Hu X, Chen H, Bao R, Zhou Y, et al. DENR controls JAK2 translation to induce PD-L1 expression for tumor immune evasion. Nat Commun. (2022) 13:2059. doi: 10.1038/s41467-022-29754-y
76. Guo X, Zhao Y, Yan H, Yang Y, Shen S, Dai X, et al. Single tumor-initiating cells evade immune clearance by recruiting type II macrophages. Genes Dev. (2017) 31:247–59. doi: 10.1101/gad.294348.116
77. Murakami S, Shahbazian D, Surana R, Zhang W, Chen H, Graham GT, et al. Yes-associated protein mediates immune reprogramming in pancreatic ductal adenocarcinoma. Oncogene. (2016) 36:1232–44. doi: 10.1038/onc.2016.288
78. Janse van Rensburg HJ, Azad T, Ling M, Hao Y, Snetsinger B, Khanal P, et al. The hippo pathway component TAZ promotes immune evasion in human cancer through PD-L1. Cancer Res. (2018) 78:1457–70. doi: 10.1158/0008-5472.Can-17-3139
79. Mao W, Zhou J, Hu J, Zhao K, Fu Z, Wang J, et al. A pan-cancer analysis of FAT atypical cadherin 4 (FAT4) in human tumors. Front Public Health. (2022) 10:969070. doi: 10.3389/fpubh.2022.969070
80. Wang D, Wu S, He J, Sun L, Zhu H, Zhang Y, et al. FAT4 overexpression promotes antitumor immunity by regulating the β-catenin/STT3/PD-L1 axis in cervical cancer. J Exp Clin Cancer Res. (2023) 42:222. doi: 10.1186/s13046-023-02758-2
81. Huang F-Y, Wong DK-H, Mak L-Y, Cheung T-T, Zhang S-S, Chau H-T, et al. FAT4 loss initiates hepatocarcinogenesis through the switching of canonical to noncanonical WNT signaling pathways. Hepatol Commun. (2023) 7:e0338. doi: 10.1097/hc9.0000000000000338
82. Yang J, Gao Y, Yao S, Wan S, Cai H. TFAP2A promotes cervical cancer via a positive feedback pathway with PD−L1. Oncol Rep. (2023) 49:114. doi: 10.3892/or.2023.8551
83. Beck AC, Cho E, White JR, Paemka L, Li T, Gu VW, et al. AP-2α Regulates S-phase and is a marker for sensitivity to PI3K inhibitor buparlisib in colon cancer. Mol Cancer Res. (2021) 19:1156–67. doi: 10.1158/1541-7786.Mcr-20-0867
84. Kołat D, Bednarek Andrzej K, Płuciennik E. The biological characteristics of transcription factors AP-2α and AP-2γ and their importance in various types of cancers. Bioscience Rep. (2019) 39:BSR20181928. doi: 10.1042/bsr20181928
85. Yang W, Song Y, Lu YL, Sun JZ, Wang HW. Increased expression of programmed death (PD)-1 and its ligand PD-L1 correlates with impaired cell-mediated immunity in high-risk human papillomavirus-related cervical intraepithelial neoplasia. Immunology. (2013) 139:513–22. doi: 10.1111/imm.12101
86. Chen X, He H, Xiao Y, Hasim A, Yuan J, Ye M, et al. CXCL10 produced by HPV-positive cervical cancer cells stimulates exosomal PDL1 expression by fibroblasts via CXCR3 and JAK-STAT pathways. Front Oncol. (2021) 11:629350. doi: 10.3389/fonc.2021.629350
87. Meng Y, Liang H, Hu J, Liu S, Hao X, Wong MSK, et al. PD-L1 expression correlates with tumor infiltrating lymphocytes and response to neoadjuvant chemotherapy in cervical cancer. J Cancer. (2018) 9:2938–45. doi: 10.7150/jca.22532
88. Choschzick M, Gut A, Fink D. PD-L1 receptor expression in vulvar carcinomas is HPV-independent. Virchows Archiv. (2018) 473:513–6. doi: 10.1007/s00428-018-2364-7
89. Lee BS, Park DI, Lee DH, Lee JE, Yeo MK, Park YH, et al. Hippo effector YAP directly regulates the expression of PD-L1 transcripts in EGFR-TKI-resistant lung adenocarcinoma. Biochem Biophys Res Commun. (2017) 491:493–9. doi: 10.1016/j.bbrc.2017.07.007
90. Wang R, Zhang Y, Shan F. PD-L1: Can it be a biomarker for the prognosis or a promising therapeutic target in cervical cancer? Int Immunopharmacol. (2022) 103:108484. doi: 10.1016/j.intimp.2021.108484
91. He C, Mao D, Hua G, Lv X, Chen X, Angeletti PC, et al. The Hippo/YAP pathway interacts with EGFR signaling and HPV oncoproteins to regulate cervical cancer progression. EMBO Mol Med. (2015) 7:1426–49. doi: 10.15252/emmm.201404976
92. Nguyen CDK, Yi C. YAP/TAZ signaling and resistance to cancer therapy. Trends Cancer. (2019) 5:283–96. doi: 10.1016/j.trecan.2019.02.010
93. Hsu PC, Jablons DM, Yang CT, You L. Epidermal growth factor receptor (EGFR) pathway, yes-associated protein (YAP) and the regulation of programmed death-ligand 1 (PD-L1) in non-small cell lung cancer (NSCLC). Int J Mol Sci. (2019) 20:3821. doi: 10.3390/ijms20153821
94. Liu B, Han D, Zhang T, Cheng G, Lu Y, Wang J, et al. Hypoxia-induced autophagy promotes EGFR loss in specific cell contexts, which leads to cell death and enhanced radiosensitivity. Int J Biochem Cell Biol. (2019) 111:12–8. doi: 10.1016/j.biocel.2018.09.013
95. Zahnreich S, Gebrekidan S, Multhoff G, Vaupel P, Schmidberger H, Mayer A. Oxygen deprivation modulates EGFR and PD-L1 in squamous cell carcinomas of the head and neck. Front Oncol. (2021) 11:623964. doi: 10.3389/fonc.2021.623964
96. Ortmann B, Druker J, Rocha S. Cell cycle progression in response to oxygen levels. Cell Mol Life Sci. (2014) 71:3569–82. doi: 10.1007/s00018-014-1645-9
97. Wilson WR, Hay MP. Targeting hypoxia in cancer therapy. Nat Rev Cancer. (2011) 11:393–410. doi: 10.1038/nrc3064
98. Bossler F, Kuhn BJ, Günther T, Kraemer SJ, Khalkar P, Adrian S, et al. Repression of human papillomavirus oncogene expression under hypoxia is mediated by PI3K/mTORC2/AKT signaling. mBio. (2019) 10:e02323-18. doi: 10.1128/mBio.02323-18
99. Hoppe-Seyler K, Bossler F, Lohrey C, Bulkescher J, Rosl F, Jansen L, et al. Induction of dormancy in hypoxic human papillomavirus-positive cancer cells. Proc Natl Acad Sci U.S.A. (2017) 114:E990–8. doi: 10.1073/pnas.1615758114
100. Hoppe-Seyler K, Mändl J, Adrian S, Kuhn B, Hoppe-Seyler F. Virus/host cell crosstalk in hypoxic HPV-positive cancer cells. Viruses. (2017) 9:174. doi: 10.3390/v9070174
101. Shurin MR, Umansky V. Cross-talk between HIF and PD-1/PD-L1 pathways in carcinogenesis and therapy. J Clin Invest. (2022) 132:e159473. doi: 10.1172/JCI159473
102. Noman MZ, Chouaib S. Targeting hypoxia at the forefront of anticancer immune responses. Oncoimmunology. (2014) 3:e954463. doi: 10.4161/21624011.2014.954463
103. Ding XC, Wang LL, Zhang XD, Xu JL, Li PF, Liang H, et al. The relationship between expression of PD-L1 and HIF-1alpha in glioma cells under hypoxia. J Hematol Oncol. (2021) 14:92. doi: 10.1186/s13045-021-01102-5
104. Lin Y-T, Wu K-J. Epigenetic regulation of epithelial-mesenchymal transition: focusing on hypoxia and TGF-β signaling. J Biomed Sci. (2020) 27:39. doi: 10.1186/s12929-020-00632-3
105. Fang Z, Meng Q, Xu J, Wang W, Zhang B, Liu J, et al. Signaling pathways in cancer-associated fibroblasts: recent advances and future perspectives. Cancer Commun. (2022) 43:3–41. doi: 10.1002/cac2.12392
106. Trugilo KP, Cebinelli GCM, Castilha EP, da Silva MR, Berti FCB, de Oliveira KB. The role of transforming growth factor β in cervical carcinogenesis. Cytokine Growth Factor Rev. (2024) S1359-6101(24)00086-8.. doi: 10.1016/j.cytogfr.2024.10.006
107. Chen J, Deng Y, Ao L, Song Y, Xu Y, Wang CC, et al. The high-risk HPV oncogene E7 upregulates miR-182 expression through the TGF-β/Smad pathway in cervical cancer. Cancer Lett. (2019) 460:75–85. doi: 10.1016/j.canlet.2019.06.015
108. Yi M, Zhang J, Li A, Niu M, Yan Y, Jiao Y, et al. The construction, expression, and enhanced anti-tumor activity of YM101: a bispecific antibody simultaneously targeting TGF-β and PD-L1. J Hematol Oncol. (2021) 14:27. doi: 10.1186/s13045-021-01045-x
109. Zhang H, Chen J, Bai J, Zhang J, Huang S, Zeng L, et al. Single dual-specific anti-PD-L1/TGF-β Antibody synergizes with chemotherapy as neoadjuvant treatment for pancreatic ductal adenocarcinoma: a preclinical experimental study. Int J Surg. (2024) 110:2679–91. doi: 10.1097/js9.0000000000001226
110. Chi C, Hou W, Zhang Y, Chen J, Shen Z, Chen Y, et al. PDHB-AS suppresses cervical cancer progression and cisplatin resistance via inhibition on Wnt/β-catenin pathway. Cell Death Dis. (2023) 14:90. doi: 10.1038/s41419-022-05547-5
111. Wang B, Li X, Liu L, Wang M. β-Catenin: oncogenic role and therapeutic target in cervical cancer. Biol Res. (2020) 53:33. doi: 10.1186/s40659-020-00301-7
112. Zhang C, Liu L, Li W, Li M, Zhang X, Zhang C, et al. Upregulation of FAM83F by c-Myc promotes cervical cancer growth and aerobic glycolysis via Wnt/β-catenin signaling activation. Cell Death Dis. (2023) 14:837. doi: 10.1038/s41419-023-06377-9
113. Galloway DA, Drews CM, Case S, Vande Pol SB. E6 proteins from high-risk HPV, low-risk HPV, and animal papillomaviruses activate the Wnt/β-catenin pathway through E6AP-dependent degradation of NHERF1. PloS Pathog. (2019) 15:e1007575. doi: 10.1371/journal.ppat.1007575
114. Anwar M, Malhotra P, Kochhar R, Bhatia A, Mahmood A, Singh R, et al. TCF 4 tumor suppressor: a molecular target in the prognosis of sporadic colorectal cancer in humans. Cell Mol Biol Lett. (2020) 25:24. doi: 10.1186/s11658-020-00217-w
115. Bello J, Nieva L, Paredes A, Gonzalez A, Zavaleta L, Lizano M. Regulation of the wnt/β-catenin signaling pathway by human papillomavirus E6 and E7 oncoproteins. Viruses. (2015) 7:4734–55. doi: 10.3390/v7082842
116. Munoz-Bello JO, Olmedo-Nieva L, Castro-Munoz LJ, Manzo-Merino J, Contreras-Paredes A, Gonzalez-Espinosa C, et al. HPV-18 E6 oncoprotein and its spliced isoform E6*I regulate the wnt/beta-catenin cell signaling pathway through the TCF-4 transcriptional factor. Int J Mol Sci. (2018) 19:3153. doi: 10.3390/ijms19103153
117. Ma S, Lee T, Katoh M, Katoh M. WNT signaling and cancer stemness. Essays Biochem. (2022) 66:319–31. doi: 10.1042/ebc20220016
118. Aghbash PS, Hemmat N, Baradaran B, Mokhtarzadeh A, Poortahmasebi V, Oskuee MA, et al. The effect of Wnt/beta-catenin signaling on PD-1/PDL-1 axis in HPV-related cervical cancer. Oncol Res. (2022) 30:99–116. doi: 10.32604/or.2022.026776
119. Wong C, Chen C, Wu Q, Liu Y, Zheng P. A critical role for the regulated wnt–myc pathway in naive T cell survival. J Immunol. (2015) 194:158–67. doi: 10.4049/jimmunol.1401238
120. Reschke R, Gajewski TF. CXCL9 and CXCL10 bring the heat to tumors. Sci Immunol. (2022) 7:eabq6509. doi: 10.1126/sciimmunol.abq6509
121. Mitsuhashi A, Kondoh K, Horikawa K, Koyama K, Nguyen NT, Afroj T, et al. Programmed death (PD)-1/PD-ligand 1 blockade mediates antiangiogenic effects by tumor-derived CXCL10/11 as a potential predictive biomarker. Cancer Sci. (2021) 112:4853–66. doi: 10.1111/cas.15161
122. Xu J, Huang Z, Wang Y, Xiang Z, Xiong B. Identification of novel tumor microenvironment regulating factor that facilitates tumor immune infiltration in cervical cancer. Front Oncol. (2022) 12:846786. doi: 10.3389/fonc.2022.846786
123. Zhang J, Dong D, Wei Q, Ren L. CXCL10 serves as a potential serum biomarker complementing SCC-Ag for diagnosing cervical squamous cell carcinoma. BMC Cancer. (2022) 22:1052. doi: 10.1186/s12885-022-10142-0
124. Pommier Y, Thomas A. New life of topoisomerase I inhibitors as antibody–drug conjugate warheads. Clin Cancer Res. (2023) 29:991–3. doi: 10.1158/1078-0432.Ccr-22-3640
125. Ho JSY, Mok BW-Y, Campisi L, Jordan T, Yildiz S, Parameswaran S, et al. TOP1 inhibition therapy protects against SARS-CoV-2-induced lethal inflammation. Cell. (2021) 184:2618–2632.e2617. doi: 10.1016/j.cell.2021.03.051
126. Sen T, Rodriguez BL, Chen L, Corte CMD, Morikawa N, Fujimoto J, et al. Targeting DNA damage response promotes antitumor immunity through STING-mediated T-cell activation in small cell lung cancer. Cancer Discovery. (2019) 9:646–61. doi: 10.1158/2159-8290.Cd-18-1020
127. Moody CA, Gusho E, Laimins LA. Human papillomaviruses sensitize cells to DNA damage induced apoptosis by targeting the innate immune sensor cGAS. PloS Pathog. (2022) 18:e1010725. doi: 10.1371/journal.ppat.1010725
128. Luo Y, Niu M, Liu Y, Zhang M, Deng Y, Mu D, et al. Oncoproteins E6 and E7 upregulate topoisomerase I to activate the cGAS-PD-L1 pathway in cervical cancer development. Front Pharmacol. (2024) 15:1450875. doi: 10.3389/fphar.2024.1450875
129. Wu J, Chen J, Zhang L, Masci PP, Zhao KN. Four major factors regulate phosphatidylinositol 3-kinase signaling pathway in cancers induced by infection of human papillomaviruses. Curr Med Chem. (2014) 21:3057–69. doi: 10.2174/0929867321666140414101528
130. Zhang L, Wu J, Ling MT, Zhao L, Zhao KN. The role of the PI3K/Akt/mTOR signalling pathway in human cancers induced by infection with human papillomaviruses. Mol Cancer. (2015) 14:87. doi: 10.1186/s12943-015-0361-x
131. Dong P, Xiong Y, Yu J, Chen L, Tao T, Yi S, et al. Control of PD-L1 expression by miR-140/142/340/383 and oncogenic activation of the OCT4-miR-18a pathway in cervical cancer. Oncogene. (2018) 37:5257–68. doi: 10.1038/s41388-018-0347-4
132. Accardi R, Rubino R, Scalise M, Gheit T, Shahzad N, Thomas M, et al. E6 and E7 from human papillomavirus type 16 cooperate to target the PDZ protein Na/H exchange regulatory factor 1. J Virol. (2011) 85:8208–16. doi: 10.1128/JVI.00114-11
133. Peng Z, Wang Q, Zhang Y, He J, Zheng J. EBP50 interacts with EGFR and regulates EGFR signaling to affect the prognosis of cervical cancer patients. Int J Oncol. (2016) 49:1737–45. doi: 10.3892/ijo.2016.3655
134. Pim D, Bergant M, Boon SS, Ganti K, Kranjec C, Massimi P, et al. Human papillomaviruses and the specificity of PDZ domain targeting. FEBS J. (2012) 279:3530–7. doi: 10.1111/j.1742-4658.2012.08709.x
135. Wang M, Qiao X, Cooper T, Pan W, Liu L, Hayball J, et al. HPV E7-mediated NCAPH ectopic expression regulates the carcinogenesis of cervical carcinoma via PI3K/AKT/SGK pathway. Cell Death Dis. (20201049) 11:1049. doi: 10.1038/s41419-020-03244-9
136. Trejo-Cerro O, Massimi P, Broniarczyk J, Myers M, Banks L. Repression of memo1, a novel target of human papillomavirus type 16 E7, increases cell proliferation in cervical cancer cells. J Virol. (2022) 96:e0122922. doi: 10.1128/jvi.01229-22
137. Ajiro M, Zheng Z-M, Imperiale MJ. E6^E7, a novel splice isoform protein of human papillomavirus 16, stabilizes viral E6 and E7 oncoproteins via HSP90 and GRP78. mBio. (2015) 6:e02068-14. doi: 10.1128/mBio.02068-14
138. Zeng J, He S-L, Li L-J, Wang C. Hsp90 up-regulates PD-L1 to promote HPV-positive cervical cancer via HER2/PI3K/AKT pathway. Mol Med. (2021) 27:130. doi: 10.1186/s10020-021-00384-2
139. Hu L, Wang Y, Chen Z, Fu L, Wang S, Zhang X, et al. Hsp90 inhibitor SNX-2112 enhances TRAIL-induced apoptosis of human cervical cancer cells via the ROS-mediated JNK-p53-autophagy-DR5 pathway. Oxid Med Cell Longev. (2019) 2019:9675450. doi: 10.1155/2019/9675450
140. Jonsson KL, Laustsen A, Krapp C, Skipper KA, Thavachelvam K, Hotter D, et al. IFI16 is required for DNA sensing in human macrophages by promoting production and function of cGAMP. Nat Commun. (2017) 8:14391. doi: 10.1038/ncomms14391
141. Alandijany T. Host intrinsic and innate intracellular immunity during herpes simplex virus type 1 (HSV-1) infection. Front Microbiol. (2019) 10:2611. doi: 10.3389/fmicb.2019.02611
142. Song Y, Wu X, Xu Y, Zhu J, Li J, Zou Z, et al. HPV E7 inhibits cell pyroptosis by promoting TRIM21-mediated degradation and ubiquitination of the IFI16 inflammasome. Int J Biol Sci. (2020) 16:2924–37. doi: 10.7150/ijbs.50074
143. Cai H, Yan L, Liu N, Xu M, Cai H. IFI16 promotes cervical cancer progression by upregulating PD-L1 in immunomicroenvironment through STING-TBK1-NF-kB pathway. BioMed Pharmacother. (2020) 123:109790. doi: 10.1016/j.biopha.2019.109790
144. Xiong H, Xi Y, Yuan Z, Wang B, Hu S, Fang C, et al. IFN-gamma activates the tumor cell-intrinsic STING pathway through the induction of DNA damage and cytosolic dsDNA formation. Oncoimmunology. (2022) 11:2044103. doi: 10.1080/2162402X.2022.2044103
145. Zhou C, Zhang Y, Yan R, Huang L, Mellor AL, Yang Y, et al. Exosome-derived miR-142-5p remodels lymphatic vessels and induces IDO to promote immune privilege in the tumour microenvironment. Cell Death Differ. (2021) 28:715–29. doi: 10.1038/s41418-020-00618-6
146. Ling J, Sun Q, Tian Q, Shi H, Yang H, Ren J. Human papillomavirus 16 E6/E7 contributes to immune escape and progression of cervical cancer by regulating miR-142–5p/PD-L1 axis. Arch Biochem Biophysics. (2022) 731:109449. doi: 10.1016/j.abb.2022.109449
147. Yu R, Wei Y, He C, Zhou P, Yang H, Deng C, et al. Integrative analyses of m6A regulators identify that METTL3 is associated with HPV status and immunosuppressive microenvironment in HPV-related cancers. Int J Biol Sci. (2022) 18:3874–87. doi: 10.7150/ijbs.70674
148. Ji H, Zhang JA, Liu H, Li K, Wang ZW, Zhu X. Comprehensive characterization of tumor microenvironment and m6A RNA methylation regulators and its effects on PD-L1 and immune infiltrates in cervical cancer. Front Immunol. (2022) 13:976107. doi: 10.3389/fimmu.2022.976107
149. Wang Q, Guo X, Li L, Gao Z, Su X, Ji M, et al. N6-methyladenosine METTL3 promotes cervical cancer tumorigenesis and Warburg effect through YTHDF1/HK2 modification. Cell Death Dis. (2020) 11:911. doi: 10.1038/s41419-020-03071-y
Keywords: cervical cancer, HPV oncoproteins, PD-L1/PD-1 pathway, regulators, immunotherapy
Citation: Li J, Ma Y, Wu Q, Ping P, Li J and Xu X (2024) The potential role of HPV oncoproteins in the PD-L1/PD-1 pathway in cervical cancer: new perspectives on cervical cancer immunotherapy. Front. Oncol. 14:1488730. doi: 10.3389/fonc.2024.1488730
Received: 30 August 2024; Accepted: 28 November 2024;
Published: 13 December 2024.
Edited by:
Robert Fruscio, University of Milano Bicocca, ItalyReviewed by:
Demirkan Gursel, Northwestern University, United StatesMing Yi, Zhejiang University, China
Copyright © 2024 Li, Ma, Wu, Ping, Li and Xu. This is an open-access article distributed under the terms of the Creative Commons Attribution License (CC BY). The use, distribution or reproduction in other forums is permitted, provided the original author(s) and the copyright owner(s) are credited and that the original publication in this journal is cited, in accordance with accepted academic practice. No use, distribution or reproduction is permitted which does not comply with these terms.
*Correspondence: Juan Li, MjM2NDA2MDI0QHFxLmNvbQ==; Xiaoying Xu, eGlhb3lpbmd4dTczQGFsaXl1bi5jb20=
†These authors share first authorship