- 1Instituto de Investigação e Inovação em Saúde (i3S), Universidade do Porto, Porto, Portugal
- 2Institute of Molecular Pathology and Immunology of the University of Porto (IPATIMUP), Porto, Portugal
- 3Instituto de Ciências Biomédicas Abel Salazar (ICBAS), Universidade do Porto, Porto, Portugal
- 4Department of Pathology, Unidade Local de Saúde (ULS) de São João, Porto, Portugal
- 5Faculty of Medicine of the University of Porto (FMUP), Porto, Portugal
- 6Porto Comprehensive Cancer Center (P.CCC), Porto, Portugal
Pancreatic cancer (PC) is the sixth leading cause of cancer-related deaths worldwide, primarily due to late-stage diagnosis and limited treatment options. While novel biomarkers and immunotherapies are promising, further research into specific molecular targets is needed. Glycans, which are carbohydrate structures mainly found on cell surfaces, play crucial roles in health and disease. The Thomsen-Friedenreich-related carbohydrate antigen Sialyl-Tn (STn), a truncated O-glycan structure, is selectively expressed in epithelial tumors, including PC. In this study, we performed a comprehensive analysis of STn expression patterns in normal, premalignant, and malignant pancreatic lesions. Additionally, we analyzed the association between STn expression and various clinicopathological features. STn expression was statistically associated with pathological diagnosis; it was absent in normal pancreatic tissue but prevalent in pancreatic carcinoma lesions, including pancreatic ductal adenocarcinoma (PDAC), pancreatic acinar cell carcinoma, and pancreatic adenosquamous carcinoma. Moreover, we found a significant association between STn expression and tumor stage, with higher STn levels observed in stage II tumors compared to stage I. However, STn expression did not correlate with patient survival or outcomes. Furthermore, STn expression was assessed in PDAC patient-derived xenograft (PDX) models, revealing consistent STn levels throughout engraftment and tumor growth cycles. This finding supports the PDX model as a valuable tool for testing new anti-STn therapeutic strategies for PC in clinical setting.
1 Introduction
Pancreatic cancer (PC) ranks as the sixth leading cause of cancer-related mortality worldwide, with 511,000 new cases and 467,000 deaths reported in 2022 (1). Over the last decades, the significance of PC as a public health concern has increased, while mortality rates for other common cancers, including gastric, colorectal, prostate, and breast cancers, have concurrently declined (1). Despite significant advances in cancer therapy, PC remains a challenging disease characterized by late-stage diagnosis and limited therapeutic options, resulting in a 5-year survival rate of only 12% (2). Currently, surgical resection followed by adjuvant chemotherapy is the sole potentially curative therapy available (3, 4). However, because PC is typically asymptomatic and there is a lack of biomarkers with sufficient specificity and sensitivity, most patients present with locally advanced or metastatic disease, rendering them ineligible for surgical intervention (3, 4). Emerging biomarkers and novel immunotherapeutic approaches, such as antibody-drug conjugates (ADCs) and Chimeric Antigen Receptor (CAR) T cells, hold promise for the future of PC treatment.
Glycans, carbohydrate structures attached to a variety of carrier molecules predominantly found on the surface of mammalian cells, play crucial cellular roles and have profound implications in both health and disease (5). Alterations in the glycan profiles of cancer cells have been extensively documented, often correlating with the aggressiveness and progression of cancer (6, 7). In the context of PC, glycan structures such as sialylated and fucosylated Lewis blood group antigens, including Sialyl Lewis a (SLea) and Sialyl Lewis x (SLex), and short O-glycans like the Tn and Sialyl-Tn (STn) antigens, are known to be upregulated and have been associated with increased invasion and metastasis (8–13). SLea, detectable by the CA-19.9 monoclonal antibody (mAb), is the only FDA-approved blood-based tumor biomarker for monitoring PC (14). However, the presence of SLea in benign conditions limits its diagnostic application, primarily confining it to a prognostic tool for managing late-stage disease (14). In contrast, STn (NeuAcα2–6-GalNAcα1-O-Ser/Thr), a truncated sialylated O-glycan structure, exhibits selective expression in epithelial tumors, including PC, while being notably absent in normal tissues (10–13, 15–21). Additionally, STn has been shown to play an important role in the malignant phenotype of cancer cells (12, 13, 22, 23). This distinctive profile positions STn as a promising candidate for both cancer biomarker and therapeutic target (24–42). Despite extensive research, accurately mapping and targeting of STn has been hindered by variations in the epitope binding features of available mAbs (39, 43, 44). Most studies on STn in PC have focused on its detection using HB-STn1 (clone 3F1) and TKH2 mAbs, with limited reports employing the B72.3 mAb (10, 11, 17, 45–48). The B72.3 mAb, generated by mice immunization with human breast carcinoma cells, was the first anti-STn mAb described and has been widely used in both tissue-based and serological assays, as well as in preclinical studies (49–53).In this work, we address this research gap by performing a comprehensive study on the expression patterns of STn in normal, premalignant, and malignant lesions of the pancreas using the B72.3 mAb. We further analyzed the association between STn expression and clinicopathological features of PC patients to understand its prognostic and therapeutic potential. Additionally, STn expression was assessed in pancreatic ductal adenocarcinoma (PDAC) patient-derived xenografts (PDXs), a potential tool for testing new anti-STn therapeutic approaches for PC.
2 Materials and methods
2.1 Clinical samples
Pancreatic tissue microarrays (TMAs) were obtained from US Biomax, Rockville, MD, USA. TMAs used in this study, BIC14011b and PA2082a, collectively comprised 14 normal pancreatic tissues, 15 premalignant lesions of the pancreas, and 78 pancreatic tumor cores, each case spotted in duplicate. Exclusion criteria included no available tumor tissue sample adequate for STn expression evaluation, or the absence of clinicopathological data. Patient’s specifications, including age, gender, pathological diagnosis, tumor stage, and the presence of lymph node metastasis were obtained from the US Biomax Inc. database (https://www.biomax.us/).
A retrospective cohort of PDAC (n=39) was obtained from the biobank of the Unidade Local de Saúde (ULS) de São João, Porto, Portugal. The collection and handling of these samples were performed in accordance with national regulatory laws governing the handling of biological specimens. This study was conducted in strict compliance with ethical guidelines and Helsinki declaration and approved by the Centro Hospitalar Universitário de São João (CHUSJ) and FMUP ethics committee (CES 253/22). Clinicopathological information was obtained from patients’ clinical records.
2.2 Immunohistochemistry
Formalin-fixed paraffin-embedded (FFPE) tissue sections from PDAC cohort and tissue microarrays were used. The expression of the short O-glycan STn detected using the B72.3 mAb was analyzed by immunohistochemistry (IHC). For the tissue microarrays, an initial baking step for 1h at 60°C was performed to prevent tissue detachment. Deparaffinization and rehydration of tissue sections was done, followed by neutralization of endogenous peroxidase activity with 3% hydrogen peroxide. For blocking of non-specific background staining, the UltraVisionTM Protein Block (Thermo Fisher Scientific, Waltham, MA, USA) was used for 5 min at room temperature (RT). Tissue sections were incubated overnight (ON) at 4°C with the anti-STn mAb B72.3 diluted in 5% bovine serum albumin (BSA)/phosphate-buffered saline (PBS) (dilution 1:2), followed by incubation with goat anti-mouse biotin-labeled secondary antibody (Dako, Berlin, Germany) diluted in 5% BSA/PBS (dilution 1:200) for 30 min at RT. Tissue sections underwent chromogenic staining with 3,3′-diaminobenzidine (DAB, Sigma-Aldrich, St. Louis, MO, USA) supplemented with 0.01% hydrogen peroxide, and nuclear staining was performed with Mayers’ hematoxylin. Controls were included in every set of slides, with normal gastric mucosa tissues serving as negative controls and intestinal metaplasia tissues as positive controls. The IHC staining of each tissue core was blindly evaluated and scored by a board-certified pathologist with vast experience in gastrointestinal pathology, using a semi-quantitative methodology. Evaluation of STn expression involved both the percentage of stained cells and the localization of the staining within the tissue. For statistical analysis, STn positivity was defined as staining present in more than 5% of the cells. The staining patterns were classified as membranous, cytoplasmic, or a combination of both. Representative images illustrating STn staining pattern were selected by the specialist pathologist.
2.3 Patient-derived xenografts
Patient-derived xenografts from PDAC were obtained as previously described (54). Briefly, PDAC patient-derived tumor cells were orthotopically implanted in the pancreas of immunodeficient mice (Rag2−/−Il2rg−/−) (54). Tumor growth was monitored by ultrasound (MicroUltrasound Vevo 2100, RRID: SCR_015816). When tumor size reached 1500 mm3 or mice presented severe symptoms, mice were euthanized and tumors fixed in neutral buffered formalin, embedded in paraffin, and processed for IHC staining using the B72.3 mAb, as described above. This study was approved by the CHUSJ and FMUP ethics committee (2015-12-11).
2.4 Statistical analysis
Statistical analysis was performed using Statistical Package for the Social Sciences (SPSS, IBM Inc., New York, NY, USA) version 25. Categorical variables were described as absolute and relative frequencies, and continuous variables were designated using median values. Categorical variables were compared using the Chi-square test or Fisher exact test, as appropriate. Multivariate analysis was performed using Cox Regression. Differences were considered statistically significant if p<0.05.
3 Results
3.1 Patient’s clinicopathological characteristics
The retrospective cohort consisted of 146 individuals, including 84 males (57.5%) and 62 females (42.5%), with a median age of 57 years (range 21-81 years). The cohort comprised 14 individuals (9.6%) with normal pancreatic tissue, 15 (10.3%) with premalignant pancreatic lesions, and 117 (80.1%) diagnosed with PC. Among those with precursor lesions, 7 patients (4.8%) had chronic pancreatitis, 3 patients (2.1%) exhibited pancreatic intraepithelial neoplasia (PanIN) 1, 3 patients (2.1%) had PanIN 2, and 2 patients (1.4%) presented PanIN 3 lesions. This study encompassed patients with various subtypes of PC, with the majority having PDAC (112 patients, 76.7%). Additionally, 2 patients (1.4%) were diagnosed with pancreatic acinar cell carcinoma, and 1 patient each (0.7%) with pancreatic squamous cell carcinoma, adenosquamous carcinoma, and neuroendocrine carcinoma. Detailed clinicopathological parameters of the individuals included in this study are presented in Table 1.
3.2 Sialyl-Tn expression in pancreatic tissues
The expression of the STn glycan antigen was evaluated in the entire cohort, comprising normal, premalignant, and PC tissues, using the B72.3 mAb. STn was not detected in any of the 14 normal pancreatic tissues tested (Figure 1; Table 1). Among the 15 premalignant lesions, STn expression was observed in PanIN lesions (13.3%) (Table 1), with exclusive cytoplasmic staining (Figure 1). No STn expression was identified in chronic pancreatitis (Figure 1).
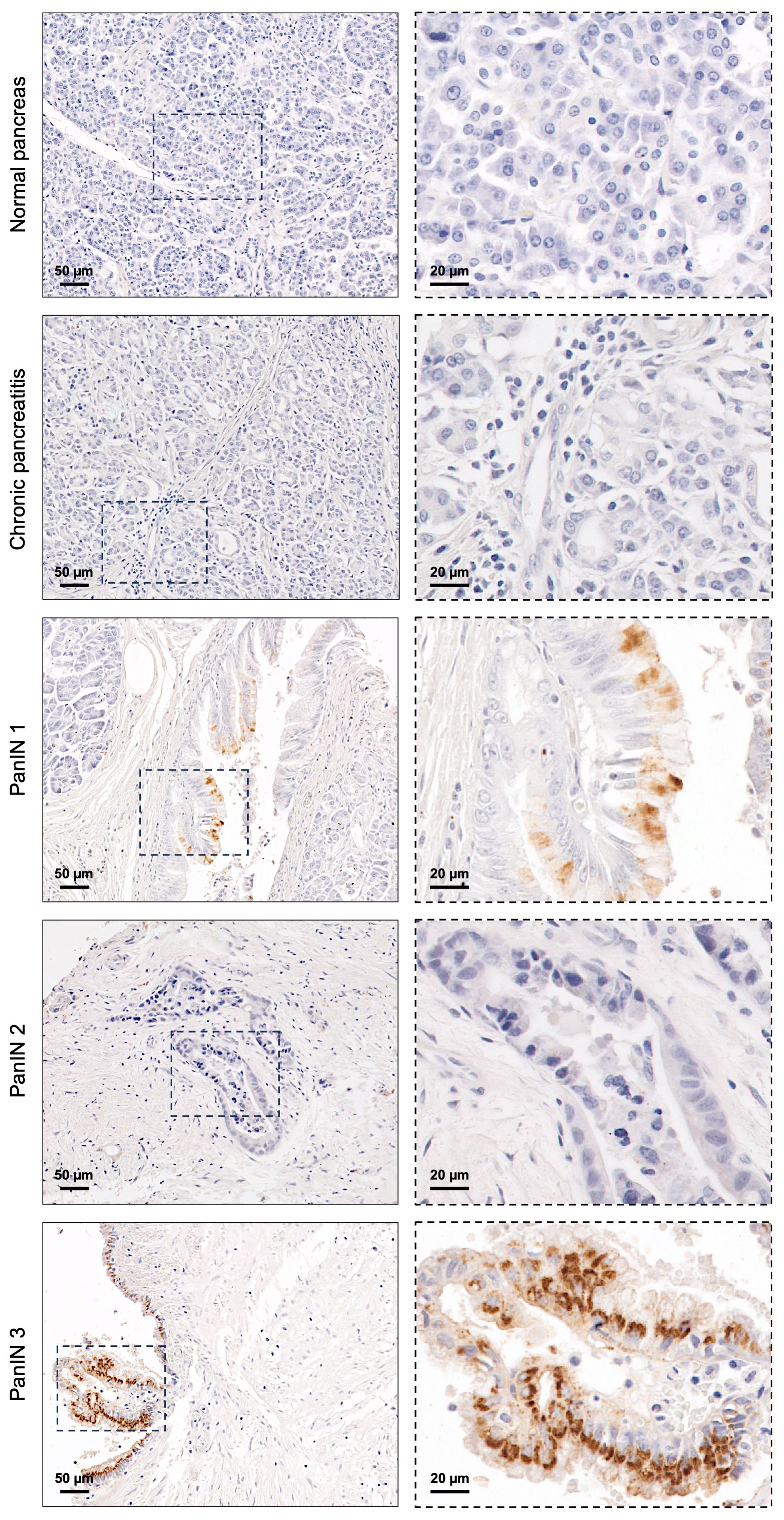
Figure 1. Sialyl-Tn expression in normal pancreatic tissue and premalignant lesions of the pancreas. Normal pancreatic tissue, chronic pancreatitis lesion, and pancreatic intraepithelial neoplasia (PanIN) 2 lesion without Sialyl-Tn (STn). PanIN 1 and PanIN 3 lesions with cytoplasmic STn expression.
In the 117 PC tissues analyzed, STn was detected in 72 lesions (61.5%) (Table 1). This group included rare PC subtypes, such as pancreatic acinar cell carcinoma, squamous cell carcinoma, adenosquamous cell carcinoma, and neuroendocrine carcinoma. Notably, one case of pancreatic acinar cell carcinoma exhibited 100% STn expression, with both membranous and cytoplasmic staining (Figure 2A). The pancreatic adenosquamous carcinoma case showed low STn expression, primarily localized to the cell membrane, while the pancreatic squamous cell carcinoma and neuroendocrine carcinoma cases completely lacked STn expression (Figure 2A). Among the total cases of PDAC (n=112), over half of the cases (62.5%) expressed STn (Table 1). Importantly, STn positivity was consistent in PDAC among the different cohorts studied (data not shown). Additionally, heterogeneity in STn expression was observed within PDAC, both within individual tumor lesions (Figure 2B) and among different tumors. Some PDAC cases exhibited moderate STn levels (Figure 2C), while others showed a very strong and uniform staining for STn (Figure 2D). In terms of STn staining patterns within PDAC lesions, some cases displayed exclusively cytoplasmic staining (Figure 2E), whereas others had STn presence both in the cytoplasm and on the cell membrane (Figure 2F).
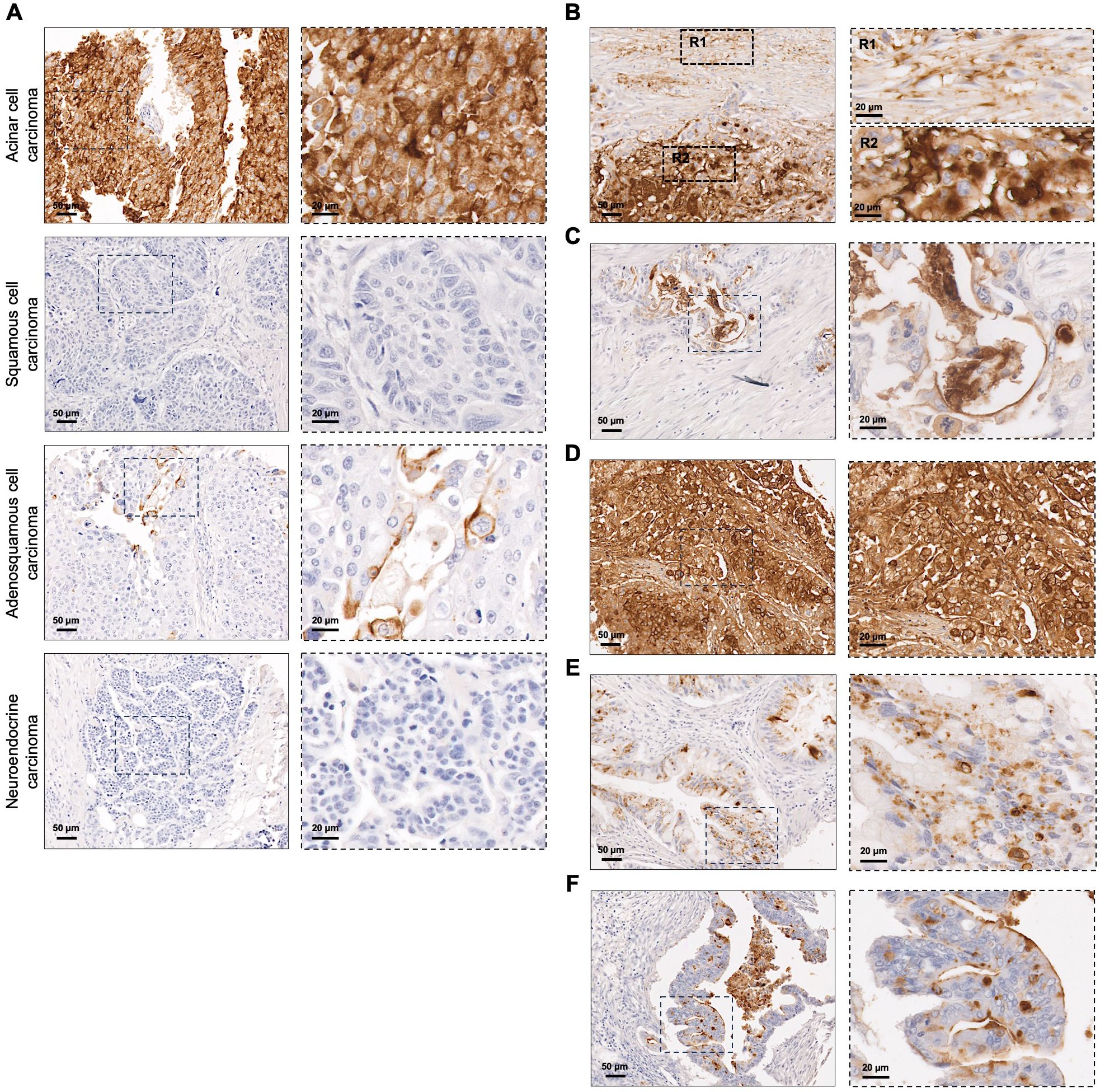
Figure 2. Sialyl-Tn expression in pancreatic cancer. (A) Screening of Sialyl-Tn (STn) within rare subtypes of pancreatic cancer (PC) revealing its presence in acinar and adenosquamous cell carcinomas, and absence in squamous cell and neuroendocrine carcinoma lesions. (B–F). STn mapping in pancreatic ductal adenocarcinoma (PDAC). (B) PDAC lesion with heterogeneous STn expression. Two regions (R1-2) were selected: R1- tumor with moderate STn expression; R2- tumor with high STn expression. (C) PDAC with moderate STn expression levels. (D) PDAC lesion with high and homogeneous STn expression. (E) PDAC with cytoplasmic STn expression. (F) PDAC lesion with both cytoplasmic and membranous STn staining.
The expression of STn was associated with clinicopathological parameters (Table 1). As expected, a significant association was found between STn expression and pathological diagnosis (p<0.001). STn was absent in normal pancreatic tissues, present in few premalignant pancreatic lesions (13.3%), and highly prevalent in pancreatic carcinoma (Table 1). Specifically, 61.5% of pancreatic carcinoma lesions tested positive for STn. There was no association between STn expression and gender or age. Among individuals with PC lesions (n=117), an association was observed between tumor stage and STn status (p=0.035), with higher STn expression noted in stage II tumors compared to stage I. However, no correlation was found between STn levels and the presence of lymph node metastasis (p=0.615).
3.3 Patient outcomes
Patient outcomes were assessed in a subset of 39 individuals diagnosed with PDAC. The median follow-up time was 16.9 months (range 0.4-151.5 months). During this period, 28 patients (71.8%) died. The median overall survival (OS) was 16.4 months (range 0.4-147.1 months). As expected, patients without lymph node metastases had significantly better OS compared to those with lymph node involvement (p=0.037). No significant correlation was observed between OS and gender (p=0.605), age (p=0.676), or tumor stage (p=0.339). Additionally, in this exploratory cohort, no association was found between STn expression and patient outcome.
3.4 Sialyl-Tn expression validation in patient-derived xenografts
Sialyl-Tn expression was assessed in PDAC PDXs to investigate whether this short O-glycan is expressed after grafting patient tumor cells into a new microenvironment. Assessments were performed immediately after engraftment and tumor growth (passage 0), and after successive engraftments and tumor growth cycles (passage 1-4). Among the 12 PDXs tested, only 2 (16.7%), specifically PDX 8 and PDX 12, showed no STn expression (Supplementary Table 1). Importantly, the majority of PDXs maintained STn expression across passages, both in terms of the percentage of tumor area and the localization of the staining (Figure 3; Supplementary Table 1). As evidenced in Figure 3, STn-positive PDXs exhibited significant intra- and inter-tumoral heterogeneity in STn expression levels. Some PDXs showed moderate STn staining (Figures 3A, B), while others displayed very strong and uniform positive staining for STn (Figure 3C). The staining patterns also varied, with some cases showing exclusive cytoplasmic staining (Figure 3A), and others exhibiting both membranous and cytoplasmic staining (Figures 3B, C). One case demonstrated a significant decrease in STn expression with successive engraftment cycles, with an initial membranous localization shifting towards a more cytoplasmatic pattern (Figure 3D; Supplementary Table 1). Additionally, two large duct mucinous tumors that exhibited moderate STn expression in the first engraftment displayed increased STn levels in subsequent passages (Figures 3E, F; Supplementary Table 1).
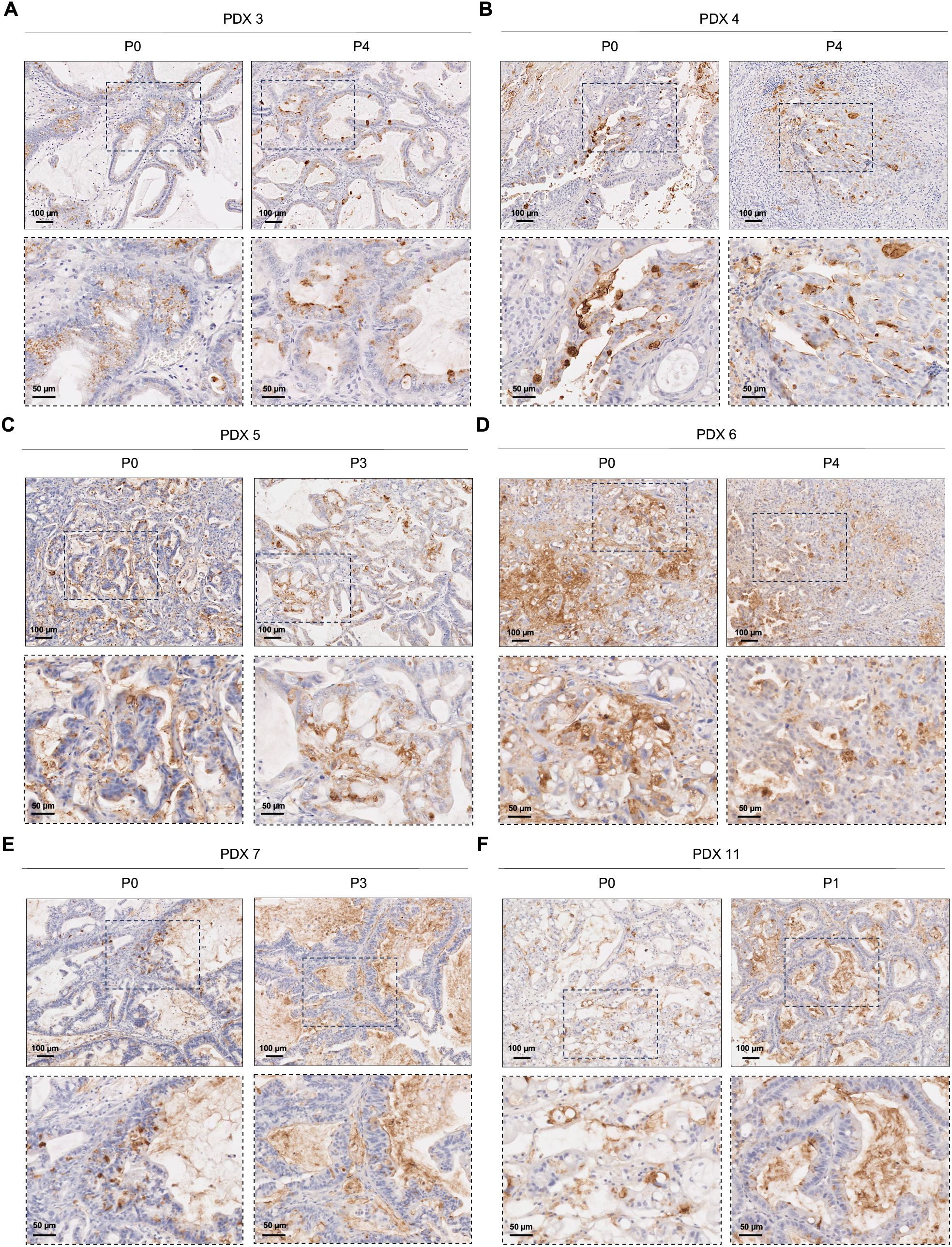
Figure 3. Sialyl-Tn expression in pancreatic ductal adenocarcinoma patient-derived xenografts. (A) Patient-derived xenografts (PDXs) with heterogeneous cytoplasmic Sialyl-Tn (STn) expression in both tested passages (P). (B) PDX with moderate STn expression within the membrane and the cytoplasm of cancer cells, both in P0 and P4. (C) PDX with high cytoplasmic and membranous STn expression, both in P0 and P3. (D) PDX with a decrease in STn expression with successive engraftment cycles. (E, F) PDXs with moderate STn expression in the initial engraftment (P0), with an increase in subsequent passages.
4 Discussion
Pancreatic cancer remains a significant clinical burden worldwide, characterized by a poor survival rate (1, 2). This dismal outlook can be attributed to several factors, including the silent clinical progression of PC, the lack of early diagnostic tools, and the limited efficacy of currently available treatments (3, 4). Recent advances in immunotherapeutic approaches, such as ADCs and CAR T cell therapy, have shown promise in the cancer field (55, 56). Tumor-associated glycan antigens have emerged as potential molecular targets for immunotherapy due to their distinctive cell surface presentation and restricted expression patterns in malignant cells (57). In particular, the truncated O-glycan STn antigen stands out due to its high expression in epithelial-origin tumors, including PC, while being mostly absent in normal tissues (10–13, 15–21). This cancer-associated expression profile has positioned STn as a compelling therapeutic target for various therapeutic strategies, including radiolabeled antibodies, ADCs, and CAR T cells (Supplementary Table 2). Nonetheless, the challenge in accurately mapping and targeting STn arises from the variability in binding properties of available mAbs, which has impeded the approval of STn-targeted therapies. Current research on STn in PC has primarily used the HB-STn and TKH2 mAbs for detection (10, 17, 45–48, 58). However, there has been limited investigation into one of the most established anti-STn mAbs, B72.3, despite its extensive use in tissue-based, serologic, and preclinical assays (49, 50) (53, 59, 60).
In the present study, we performed a comprehensive mapping of STn expression in normal pancreatic tissues, as well as in premalignant and malignant pancreatic lesions, using the B72.3 mAb. Our results demonstrated a notable absence of STn in normal pancreatic tissues, consistent with previous studies (10, 17, 45, 46, 48, 59, 60). In premalignant pancreatic lesions, STn expression was observed in PanIN lesions but not in chronic pancreatitis. Previous studies have reported diverse findings regarding STn expression within premalignant lesions of the pancreas, ranging from absence to moderate expression (10, 47, 53, 59). The present study reinforces the need for further validation using a larger independent cohort to clarify the role of STn in pancreatic lesions and assess its potential as a biomarker for the early detection of PC.
In the context of PDAC, the most prevalent type of PC, accounting for more than 90% of all pancreatic malignancies, our study revealed the presence of STn in 61.5% of the cases. Our results align with previous studies reporting that STn expression in PDAC is highly variable (10, 17, 45–48, 53, 59, 60). This variability highlights the challenges involved in precisely detecting short O-glycans, potentially arising from different antibody specificities, each recognizing particular epitopes of the STn antigen, influencing detection outcomes across studies. Moreover, disparities in sample size, demographic characteristics of patients, and stages of disease progression within the studied populations may further contribute to the observed differences in STn levels. Heterogeneity in STn expression was observed not only among different tumors but also within individual tumor lesions. Intra-tumor heterogeneity suggests that subsets of tumor cells may express different STn levels. This is consistent with previous studies documenting the heterogenous nature of STn expression in PDAC (48, 59). Considering the poor clinical prognosis linked to STn-positive tumors and the oncogenic features induced by O-glycan truncation (11, 12, 22, 61, 62), these observations suggest that short O-glycans may play a role in a subpopulation of tumor cells, potentially contributing to cancer progression. Regarding the staining pattern of STn within PDAC, we observed the presence of this glycan both in the cytoplasm and on the membrane of cancer cells. The expression of STn on the surface of cancer cells is crucial for effective targeting, emphasizing its potential as a promising therapeutic target in PC.
Additionally, our study revealed the presence of STn in rare PC subtypes, such as pancreatic acinar cell carcinoma and adenosquamous carcinoma. Although STn detection in these rare pathological subtypes of PC suggests its potential as a biomarker and indicates possible avenues for exploring immunotherapeutic approaches, further validation in larger, more comprehensive cohorts is warranted to validate STn expression across rare PC subtypes and to assess its broader clinical relevance.
We further evaluated the potential association between STn expression and clinicopathological variables, revealing a correlation between STn status and pathological diagnosis, as expected. Notably, higher STn expression was observed in patients with stage II PDAC compared to those with stage I tumors. To our knowledge, this is the first evidence of an association between STn expression levels and PDAC staging. These findings suggest that STn may serve as a marker of tumor progression and could potentially hold prognostic implications in PDAC. However, our study did not reveal a correlation between STn status and OS in an exploratory cohort. Given the relatively small size of this exploratory cohort, future research involving larger cohorts with comprehensive follow-up data is warranted to assess STn’s potential as a prognostic marker for survival in PDAC patients.
To validate our findings and explore the potential of STn for therapeutic applications, STn expression was assessed within a preclinical PDX model of PDAC. Among the 12 PDXs evaluated, 10 exhibited positive STn expression (83.3%). Notably, most PDXs consistently sustained STn expression across passages, both in terms of the extend of stained tumor area and the subcellular staining location. This suggests that STn-expressing cancer cells persist following engraftment. However, some variations were observed: one case showed a decrease in STn with successive engraftment cycles, while two PDXs exhibited increasing STn expression in subsequent passages. The dynamic interplay with the tumor microenvironment may contribute to the different STn levels found in tumor cells, highlighting the importance of this truncated O-glycan structure in the cancer context (63, 64). Similar to PDAC patient tissues, variations in staining intensity and patterns were noted between different PDXs, confirming their representativeness of STn expression within the PDAC landscape. The consistent expression of STn in PDX models highlights their potential as valuable tools for preclinical studies and testing of STn-targeted therapies. To our knowledge, this study presents the first evidence of STn mapping in PDAC PDXs, highlighting their utility as preclinical models.
Overall, our study provides a comprehensive evaluation of STn expression using the B72.3 mAb across pancreatic tissues, from normal to premalignant and malignant lesions, and extending to a preclinical model. Despite the inherent challenges associated with mapping STn due to antibody specificity and variability, our findings offer valuable insights into its expression dynamics throughout different stages of pancreatic carcinogenesis. Moreover, our evaluation of STn in rare PC subtypes expands its potential as both a biomarker and therapeutic target for these specific PC subtypes. Additionally, our analysis of STn expression in PDAC PDX models underscores their reliability for preclinical studies and the development of STn-targeted therapies.
Data availability statement
The raw data supporting the conclusions of this article will be made available by the authors, without undue reservation.
Ethics statement
The studies involving humans were approved by CHUSJ and FMUP ethics committee (CES 253/22). The studies were conducted in accordance with the local legislation and institutional requirements. The participants provided their written informed consent to participate in this study. The animal study was approved by CHUSJ and FMUP ethics committee (2015-12-11). The study was conducted in accordance with the local legislation and institutional requirements. No potentially identifiable images or data are presented in this study.
Author contributions
RA: Conceptualization, Data curation, Investigation, Methodology, Writing – original draft, Writing – review & editing. JL: Formal analysis, Writing – review & editing. DL: Methodology, Writing – review & editing. JG: Formal analysis, Writing – review & editing. SAM: Conceptualization, Funding acquisition, Writing – review & editing. CAR: Conceptualization, Funding acquisition, Writing – review & editing, Supervision.
Funding
The author(s) declare financial support was received for the research, authorship, and/or publication of this article. This research was funded by National Funds through the Portuguese Foundation for Science and Technology (FCT): PTDC/MEC-ONC/0491/2021 to CAR and JG; 2022.02284.PTDC to SAM, and 2020.05483.BD PhD scholarship to RA.
Acknowledgments
The authors acknowledge the support of the i3S Histology and Electron Microscopy Scientific Platform. The authors acknowledge the Department of Pathology of the ULS for providing the clinical samples. The authors acknowledge the projects: "Cancer Research on Therapy Resistance: From Basic Mechanisms to Novel Targets" - NORTE-01-0145-FEDER000051 and “P.CCC: Centro Compreensivo de Cancro do Porto” - NORTE-01-0145-FEDER-072678, supported by Norte Portugal Regional Operational Programme (NORTE 2020), under the PORTUGAL 2020 Partnership Agreement, through the European Regional Development Fund (ERDF).
Conflict of interest
SAM holds patents in the area of exosomes biology.
The remaining authors declare that the research was conducted in the absence of any commercial or financial relationships that could be construed as a potential conflict of interest.
Publisher’s note
All claims expressed in this article are solely those of the authors and do not necessarily represent those of their affiliated organizations, or those of the publisher, the editors and the reviewers. Any product that may be evaluated in this article, or claim that may be made by its manufacturer, is not guaranteed or endorsed by the publisher.
Supplementary material
The Supplementary Material for this article can be found online at: https://www.frontiersin.org/articles/10.3389/fonc.2024.1466255/full#supplementary-material
References
1. Bray F, Laversanne M, Sung H, Ferlay J, Siegel RL, Soerjomataram I, et al. Global cancer statistics 2022: GLOBOCAN estimates of incidence and mortality worldwide for 36 cancers in 185 countries. CA: A Cancer J Clin. (2024) 74:229–63. doi: 10.3322/caac.21834
2. Siegel RL, Miller KD, Wagle NS, Jemal A. Cancer statistics, 2023. CA: A Cancer J Clin. (2023) 73:17–48. doi: 10.3322/caac.21763
3. Halbrook CJ, Lyssiotis CA, Pasca di Magliano M, Maitra A. Pancreatic cancer: Advances and challenges. Cell. (2023) 186:1729–54. doi: 10.1016/j.cell.2023.02.014
4. Hosein AN, Dougan SK, Aguirre AJ, Maitra A. Translational advances in pancreatic ductal adenocarcinoma therapy. Nat Cancer. (2022) 3:272–86. doi: 10.1038/s43018-022-00349-2
5. Pinho SS, Reis CA. Glycosylation in cancer: mechanisms and clinical implications. Nat Rev Cancer. (2015) 15:540–55. doi: 10.1038/nrc3982
6. Campos D, Freitas D, Gomes J, Magalhães A, Steentoft C, Gomes C, et al. Probing the O-glycoproteome of gastric cancer cell lines for biomarker discovery. Mol Cell Proteomics. (2015) 14:1616–29. doi: 10.1074/mcp.M114.046862
7. Gomes C, Almeida A, Barreira A, Calheiros J, Pinto F, Abrantes R, et al. Carcinoembryonic antigen carrying SLe(X) as a new biomarker of more aggressive gastric carcinomas. Theranostics. (2019) 9:7431–46. doi: 10.7150/thno.33858
8. Balmaña M, Sarrats A, Llop E, Barrabés S, Saldova R, Ferri MJ, et al. Identification of potential pancreatic cancer serum markers: Increased sialyl-Lewis X on ceruloplasmin. Clin Chim Acta. (2015) 442:56–62. doi: 10.1016/j.cca.2015.01.007
9. Takahashi S, Oda T, Hasebe T, Sasaki S, Kinoshita T, Konishi M, et al. Overexpression of sialyl Lewis x antigen is associated with formation of extratumoral venous invasion and predicts postoperative development of massive hepatic metastasis in cases with pancreatic ductal adenocarcinoma. Pathobiology. (2001) 69:127–35. doi: 10.1159/000048767
10. Remmers N, Anderson JM, Linde EM, DiMaio DJ, Lazenby AJ, Wandall HH, et al. Aberrant expression of mucin core proteins and o-linked glycans associated with progression of pancreatic cancer. Clin Cancer Res. (2013) 19:1981–93. doi: 10.1158/1078-0432.CCR-12-2662
11. Thomas D, Sagar S, Caffrey T, Grandgenett PM, Radhakrishnan P. Truncated O-glycans promote epithelial-to-mesenchymal transition and stemness properties of pancreatic cancer cells. J Cell Mol Med. (2019) 23:6885–96. doi: 10.1111/jcmm.14572
12. Hofmann BT, Schlüter L, Lange P, Mercanoglu B, Ewald F, Fölster A, et al. COSMC knockdown mediated aberrant O-glycosylation promotes oncogenic properties in pancreatic cancer. Mol Cancer. (2015) 14:109. doi: 10.1186/s12943-015-0386-1
13. Radhakrishnan P, Dabelsteen S, Madsen FB, Francavilla C, Kopp KL, Steentoft C, et al. Immature truncated O-glycophenotype of cancer directly induces oncogenic features. Proc Natl Acad Sci U.S.A. (2014) 111:E4066–4075. doi: 10.1073/pnas.1406619111
14. Luo G, Jin K, Deng S, Cheng H, Fan Z, Gong Y, et al. Roles of CA19-9 in pancreatic cancer: Biomarker, predictor and promoter. Biochim Biophys Acta (BBA) - Rev Cancer. (2021) 1875:188409. doi: 10.1016/j.bbcan.2020.188409
15. Marcos NT, Pinho S, Grandela C, Cruz A, Samyn-Petit B, Harduin-Lepers A, et al. Role of the human ST6GalNAc-I and ST6GalNAc-II in the synthesis of the cancer-associated sialyl-Tn antigen. Cancer Res. (2004) 64:7050–7. doi: 10.1158/0008-5472.CAN-04-1921
16. Marcos NT, Bennett EP, Gomes J, Magalhaes A, Gomes C, David L, et al. ST6GalNAc-I controls expression of sialyl-Tn antigen in gastrointestinal tissues. Front Biosci (Elite Ed). (2011) 3:1443–55. doi: 10.2741/e345
17. Itzkowitz S, Kjeldsen T, Friera A, Hakomori S, Yang US, Kim YS. Expression of Tn, sialosyl Tn, and T antigens in human pancreas. Gastroenterology. (1991) 100:1691–700. doi: 10.1016/0016-5085(91)90671-7
18. Itzkowitz SH, Yuan M, Montgomery CK, Kjeldsen T, Takahashi HK, Bigbee WL, et al. Expression of Tn, sialosyl-Tn, and T antigens in human colon cancer. Cancer Res. (1989) 49:197–204.
19. Van Elssen CH, Frings PW, Bot FJ, Van de Vijver KK, Huls MB, Meek B, et al. Expression of aberrantly glycosylated Mucin-1 in ovarian cancer. Histopathology. (2010) 57:597–606. doi: 10.1111/j.1365-2559.2010.03667.x
20. Myers RB, Meredith RF, Schlom J, LoBuglio AF, Bueschen AJ, Wheeler RH, et al. Tumor associated glycoprotein-72 is highly expressed in prostatic adenocarcinomas. J Urol. (1994) 152:243–6. doi: 10.1016/S0022-5347(17)32870-7
21. Kjeldsen T, Clausen H, Hirohashi S, Ogawa T, Iijima H, Hakomori S. Preparation and characterization of monoclonal antibodies directed to the tumor-associated O-linked sialosyl-2—6 alpha-N-acetylgalactosaminyl (sialosyl-Tn) epitope. Cancer Res. (1988) 48:2214–20.
22. Freitas D, Campos D, Gomes J, Pinto F, Macedo JA, Matos R, et al. O-glycans truncation modulates gastric cancer cell signaling and transcription leading to a more aggressive phenotype. EBioMedicine. (2019) 40:349–62. doi: 10.1016/j.ebiom.2019.01.017
23. Pinho S, Marcos NT, Ferreira B, Carvalho AS, Oliveira MJ, Santos-Silva F, et al. Biological significance of cancer-associated sialyl-Tn antigen: modulation of Malignant phenotype in gastric carcinoma cells. Cancer Lett. (2007) 249:157–70. doi: 10.1016/j.canlet.2006.08.010
24. Meredith RF, Bueschen AJ, Khazaeli MB, Plott WE, Grizzle WE, Wheeler RH, et al. Treatment of metastatic prostate carcinoma with radiolabeled antibody CC49. J Nucl Med. (1994) 35:1017.
25. Minnix M, Li L, Yazaki P, Chea J, Poku E, Colcher D, et al. Improved targeting of an anti-TAG-72 antibody drug conjugate for the treatment of ovarian cancer. Cancer Med. (2020) 9:4756–67. doi: 10.1002/cam4.3078
26. Al-Alem L, Prendergast JM, Clark J, Zarrella B, Zarrella DT, Hill SJ, et al. Sialyl-Tn serves as a potential therapeutic target for ovarian cancer. J Ovarian Res. (2024) 17:71. doi: 10.1186/s13048-024-01397-1
27. Shu R, Evtimov VJ, Hammett MV, Nguyen NN, Zhuang J, Hudson PJ, et al. Engineered CAR-T cells targeting TAG-72 and CD47 in ovarian cancer. Mol Ther Oncolyt. (2021) 20:325–41. doi: 10.1016/j.omto.2021.01.002
28. Loureiro LR, Feldmann A, Bergmann R, Koristka S, Berndt N, Arndt C, et al. Development of a novel target module redirecting UniCAR T cells to Sialyl Tn-expressing tumor cells. Blood Cancer J. (2018) 8:81. doi: 10.1038/s41408-018-0113-4
29. Miles D, Roché H, Martin M, Perren TJ, Cameron DA, Glaspy J, et al. Phase III multicenter clinical trial of the sialyl-TN (STn)-keyhole limpet hemocyanin (KLH) vaccine for metastatic breast cancer. Oncologist. (2011) 16:1092–100. doi: 10.1634/theoncologist.2010-0307
30. Holmberg LA, Oparin DV, Gooley T, Lilleby K, Bensinger W, Reddish MA, et al. Clinical outcome of breast and ovarian cancer patients treated with high-dose chemotherapy, autologous stem cell rescue and THERATOPE STn-KLH cancer vaccine. Bone Marrow Transplant. (2000) 25:1233–41. doi: 10.1038/sj.bmt.1702430
31. Gilewski TA, Ragupathi G, Dickler M, Powell S, Bhuta S, Panageas K, et al. Immunization of High-Risk Breast Cancer Patients with Clustered sTn-KLH Conjugate plus the Immunologic Adjuvant QS-21. Clin Cancer Res. (2007) 13:2977–85. doi: 10.1158/1078-0432.CCR-06-2189
32. Meredith RF, Khazaeli MB, Plott WE, Saleh MN, Liu T, Allen LF, et al. Phase I trial of iodine-131-chimeric B72. 3 (human IgG4) metastatic colorectal cancer. J Nucl Med. (1992) 33:23–9.
33. Doerr RJ, Abdel-Nabi H, Krag D, Mitchell E. Radiolabeled antibody imaging in the management of colorectal cancer. Results multicenter Clin study. Ann Surg. (1991) 214:118–24. doi: 10.1097/00000658-199108000-00005
34. Rosenblum MG, Verschraegen CF, Murray JL, Kudelka AP, Gano J, Cheung L, et al. Phase I study of 90Y-labeled B72.3 intraperitoneal administration in patients with ovarian cancer: effect of dose and EDTA coadministration on pharmacokinetics and toxicity. Clin Cancer Res. (1999) 5:953–61.
35. Murray JL, Macey DJ, Kasi LP, Rieger P, Cunningham J, Bhadkamkar V, et al. Phase II radioimmunotherapy trial with 131I-CC49 in colorectal cancer. Cancer. (1994) 73:1057–66. doi: 10.1002/(ISSN)1097-0142
36. Meredith RF, Alvarez RD, Partridge EE, Khazaeli MB, Lin C-Y, Macey DJ, et al. Intraperitoneal radioimmunochemotherapy of ovarian cancer: A phase I study. Cancer Biotherapy Radiopharmaceut. (2001) 16:305–15. doi: 10.1089/108497801753131381
37. Alvarez RD, Partridge EE, Khazaeli MB, Plott G, Austin M, Kilgore L, et al. Intraperitoneal radioimmunotherapy of ovarian cancer with 177Lu-CC49: a phase I/II study. Gynecol Oncol. (1997) 65:94–101. doi: 10.1006/gyno.1996.4577
38. Eavarone DA, Al-Alem L, Lugovskoy A, Prendergast JM, Nazer RI, Stein JN, et al. Humanized anti-Sialyl-Tn antibodies for the treatment of ovarian carcinoma. PloS One. (2018) 13:e0201314. doi: 10.1371/journal.pone.0201314
39. Prendergast JM, Galvao da Silva AP, Eavarone DA, Ghaderi D, Zhang M, Brady D, et al. Novel anti-Sialyl-Tn monoclonal antibodies and antibody-drug conjugates demonstrate tumor specificity and anti-tumor activity. MAbs. (2017) 9:615–27. doi: 10.1080/19420862.2017.1290752
40. McGuinness RP, Ge Y, Patel SD, Kashmiri SV, Lee HS, Hand PH, et al. Anti-tumor activity of human T cells expressing the CC49-zeta chimeric immune receptor. Hum Gene Ther. (1999) 10:165–73. doi: 10.1089/10430349950018968
41. Hege KM, Bergsland EK, Fisher GA, Nemunaitis JJ, Warren RS, McArthur JG, et al. Safety, tumor trafficking and immunogenicity of chimeric antigen receptor (CAR)-T cells specific for TAG-72 in colorectal cancer. J Immunother Cancer. (2017) 5:22. doi: 10.1186/s40425-017-0222-9
42. Murad JP, Kozlowska AK, Lee HJ, Ramamurthy M, Chang WC, Yazaki P, et al. Effective targeting of TAG72(+) peritoneal ovarian tumors via regional delivery of CAR-engineered T cells. Front Immunol. (2018) 9:2268. doi: 10.3389/fimmu.2018.02268
43. Reddish MA, Jackson L, Koganty RR, Qiu D, Hong W, Longenecker BM. Specificities of anti-sialyl-Tn and anti-Tn monoclonal antibodies generated using novel clustered synthetic glycopeptide epitopes. Glycoconj J. (1997) 14:549–60. doi: 10.1023/a:1018576224062
44. Loureiro LR, Sousa DP, Ferreira D, Chai W, Lima L, Pereira C, et al. Novel monoclonal antibody L2A5 specifically targeting sialyl-Tn and short glycans terminated by alpha-2-6 sialic acids. Sci Rep. (2018) 8:12196. doi: 10.1038/s41598-018-30421-w
45. Yonezawa S, Tachikawa T, Shin S, Sato E. Sialosyl-Tn antigen. Its distribution in normal human tissues and expression in adenocarcinomas. Am J Clin Pathol. (1992) 98:167–74. doi: 10.1093/ajcp/98.2.167
46. Osako M, Yonezawa S, Siddiki B, Huang J, Ho JJ, Kim YS, et al. Immunohistochemical study of mucin carbohydrates and core proteins in human pancreatic tumors. Cancer. (1993) 71:2191–9. doi: 10.1002/(ISSN)1097-0142
47. Kim GE, Bae HI, Park HU, Kuan SF, Crawley SC, Ho JJ, et al. Aberrant expression of MUC5AC and MUC6 gastric mucins and sialyl Tn antigen in intraepithelial neoplasms of the pancreas. Gastroenterology. (2002) 123:1052–60. doi: 10.1053/gast.2002.36018
48. Rømer TB, Aasted MKM, Dabelsteen S, Groen A, Schnabel J, Tan E, et al. Mapping of truncated O-glycans in cancers of epithelial and non-epithelial origin. Br J Cancer. (2021) 125:1239–50. doi: 10.1038/s41416-021-01530-7
49. Hombach A, Heuser C, Sircar R, Tillmann T, Diehl V, Kruis W, et al. T cell targeting of TAG72+ tumor cells by a chimeric receptor with antibody-like specificity for a carbohydrate epitope. Gastroenterology. (1997) 113:1163–70. doi: 10.1053/gast.1997.v113.pm9322511
50. Diniz F, Lamas S, Osório H, Aguiar P, Freitas D, Gärtner F, et al. Nanoparticles targeting Sialyl-Tn for efficient tyrosine kinase inhibitor delivery in gastric cancer. Acta Biomaterialia. (2023) 170:142–54. doi: 10.1016/j.actbio.2023.08.014
51. Colcher D, Hand PH, Nuti M, Schlom J. A spectrum of monoclonal antibodies reactive with human mammary tumor cells. Proc Natl Acad Sci U.S.A. (1981) 78:3199–203. doi: 10.1073/pnas.78.5.3199
52. Dombek GE, Ore AS, Cheng J, Matsumoto Y, Glickman JN, Fleishman A, et al. Immunohistochemical analysis of Tn antigen expression in colorectal adenocarcinoma and precursor lesions. BMC Cancer. (2022) 22:1281. doi: 10.1186/s12885-022-10376-y
53. Ching CK, Holmes SW, Holmes GK, Long RG. Comparison of two sialosyl-Tn binding monoclonal antibodies (MLS102 and B72.3) in detecting pancreatic cancer. Gut. (1993) 34:1722–5. doi: 10.1136/gut.34.12.1722
54. Ruivo CF, Bastos N, Adem B, Batista I, Duraes C, Melo CA, et al. Extracellular Vesicles from Pancreatic Cancer Stem Cells Lead an Intratumor Communication Network (EVNet) to fuel tumour progression. Gut. (2022) 71:2043. doi: 10.1136/gutjnl-2021-324994
55. Cappell KM, Kochenderfer JN. Long-term outcomes following CAR T cell therapy: what we know so far. Nat Rev Clin Oncol. (2023) 20:359–71. doi: 10.1038/s41571-023-00754-1
56. Senior M. Cancer-targeting antibody–drug conjugates drive dealmaking frenzy. Nat Biotechnol. (2024) 42:362–6. doi: 10.1038/s41587-024-02168-5
57. Mereiter S, Balmaña M, Campos D, Gomes J, Reis CA. Glycosylation in the era of cancer-targeted therapy: where are we heading? Cancer Cell. (2019) 36:6–16. doi: 10.1016/j.ccell.2019.06.006
58. Motoo Y, Kawakami H, Watanabe H, Satomura Y, Ohta H, Okai T, et al. Serum sialyl-Tn antigen levels in patients with digestive cancers. Oncology. (1991) 48:321–6. doi: 10.1159/000226951
59. Lyubsky S, Madariaga J, Lozowski M, Mishriki Y, Schuss A, Chao S, et al. A tumor-associated antigen in carcinoma of the pancreas defined by monoclonal antibody B72. 3. Am J Clin Pathol. (1988) 89:160–7. doi: 10.1093/ajcp/89.2.160
60. Takiyama Y, Tempero MA, Takasaki H, Onda M, Tsuchiya R, Büchler M, et al. Reactivity of CO17-1A and B72.3 in benign and Malignant pancreatic diseases. Hum Pathol. (1989) 20:832–8. doi: 10.1016/0046-8177(89)90093-2
61. Julien S, Lagadec C, Krzewinski-Recchi MA, Courtand G, Le Bourhis X, Delannoy P. Stable expression of sialyl-Tn antigen in T47-D cells induces a decrease of cell adhesion and an increase of cell migration. Breast Cancer Res Treat. (2005) 90:77–84. doi: 10.1007/s10549-004-3137-3
62. Julien S, Adriaenssens E, Ottenberg K, Furlan A, Courtand G, Vercoutter-Edouart AS, et al. ST6GalNAc I expression in MDA-MB-231 breast cancer cells greatly modifies their O-glycosylation pattern and enhances their tumourigenicity. Glycobiology. (2006) 16:54–64. doi: 10.1093/glycob/cwj033
63. Carrascal MA, Severino PF, Guadalupe Cabral M, Silva M, Ferreira JA, Calais F, et al. Sialyl Tn-expressing bladder cancer cells induce a tolerogenic phenotype in innate and adaptive immune cells. Mol Oncol. (2014) 8:753–65. doi: 10.1016/j.molonc.2014.02.008
Keywords: Sialyl-Tn, glycosylation, pancreatic cancer, targeted therapy, truncated O-glycans
Citation: Abrantes R, Lopes J, Lopes D, Gomes J, Melo SA and Reis CA (2024) Sialyl-Tn glycan epitope as a target for pancreatic cancer therapies. Front. Oncol. 14:1466255. doi: 10.3389/fonc.2024.1466255
Received: 17 July 2024; Accepted: 19 August 2024;
Published: 13 September 2024.
Edited by:
Matthew S. Macauley, University of Alberta, CanadaReviewed by:
Mukulika Bose, University of Texas MD Anderson Cancer Center, United StatesPaula Alexandra Quintela Videira, Universidade NOVA de Lisboa, Portugal
Copyright © 2024 Abrantes, Lopes, Lopes, Gomes, Melo and Reis. This is an open-access article distributed under the terms of the Creative Commons Attribution License (CC BY). The use, distribution or reproduction in other forums is permitted, provided the original author(s) and the copyright owner(s) are credited and that the original publication in this journal is cited, in accordance with accepted academic practice. No use, distribution or reproduction is permitted which does not comply with these terms.
*Correspondence: Celso A. Reis, Y2Vsc29yQGkzcy51cC5wdA==