- Affiliated Hospital of Jiangsu University, Zhenjiang, Jiangsu, China
YB1 (Y box binding protein 1), a multifunctional protein capable of binding to DNA/RNA, is present in most cells and acts as a splicing factor. It is involved in numerous cellular processes such as transcription, translation, and DNA repair, significantly affecting cell proliferation, differentiation, and apoptosis. Abnormal expression of this protein is closely linked to the formation of various malignancies (osteosarcoma, nasopharyngeal carcinoma, breast cancer, etc.). This review examines the multifaceted functions of YB1 and its critical role in osteosarcoma progression, providing new perspectives for potential therapeutic strategies.
1 Introduction
Osteosarcoma originates from mesenchymal stem cells (MSCs) and is the most common primary malignant bone tumor, predominantly occurring in children and adolescents (1). It commonly affects areas of rapid growth such as the distal femur and the proximal humerus, possibly related to hormonal changes during puberty (2). Most cases of osteosarcoma invade surrounding tissues and metastasize, commonly to the lungs. With the introduction of chemotherapy, the 5-year survival rate has increased to about 70%, but the rate drops to 20%-30% for recurrent or lung-metastasized cases, highlighting the urgent need for novel therapeutic targets to enhance patient survival rates (3, 4).
RNA splicing, the process of removing non-coding introns from precursor RNA to produce mature mRNA, is a dynamic and complex process (5). Approximately 95% of gene splicing involves alternative splicing, where certain introns are selectively removed during the RNA splicing process. This mechanism allows a single gene to produce multiple mature mRNAs, thereby enabling the efficient realization of multiple functions by human genes (6, 7).
Alternative splicing plays a crucial role in the development of osteosarcoma, particularly through mechanisms that regulate the immune microenvironment. Research has identified several alternative splicing events associated with osteosarcoma, which may promote tumor progression by affecting the function of key immune cells, such as CD8+ T cells and dendritic cells. Additionally, RNA-binding proteins (RBPs) play a significant role in regulating these splicing events, suggesting that they may serve as potential therapeutic targets (8).
The splicing process is highly regulated, mediated by the spliceosome, a macromolecular complex composed of ribonucleoproteins (RNPs) and small nuclear RNA (snRNA), which recognizes intron 5’ GU and 3’ AG splice sites (9). However, in addition to regulation by splice sites and the spliceosome, the splicing process is also regulated by cis-regulatory elements and trans-acting factors, Cis-regulatory elements are specific RNA sequences located on precursor RNA, including exon splicing enhancers (ESE), intron splicing enhancers (ISE), and exon splicing silencers (ESS). Their primary function is to recruit trans-acting factors (splicing factors) to specific sites, thereby precisely regulating the activation or inhibition of splice sites by the spliceosome (10–12). With the deepening of research on the RNA splicing process, increasing evidence suggests that abnormal RNA splicing plays a crucial role in various diseases, especially cancer (13, 14). Here are some examples of how the splicing process affects osteosarcoma: Serine/Arginine-rich Splicing Factor 1 (SRSF1) is an important splicing factor that has been found to be closely associated with the progression of osteosarcoma when highly expressed. SRSF1 regulates the splicing of multiple genes, including HIF-1α and VEGF-A, which play crucial roles in angiogenesis, cell proliferation, and anti-apoptotic pathways. Additionally, SRSF1 may influence the function of tumor-infiltrating T cells by regulating the splicing of genes related to immune suppression, thereby helping tumor cells evade immune surveillance. Through these splicing events, SRSF1 enhances tumor cell invasiveness and drug resistance, further driving the progression of osteosarcoma (8, 15). Small extracellular vesicles (sEVs) derived from osteosarcoma cells influence the splicing factors by carrying and delivering specific miRNAs and proteins. These sEVs modulate the splicing of genes related to Notch2 and GATA3, further promoting tumor cell metastasis (16).
YB1, also known as DNA binding protein B (DbpB), often acts as a splicing factor in cellular regulation and plays a key role in a wide range of cellular functions. Its abnormal expression has a significant impact on tumor formation (17, 18). We found through the GEO database that YB1 is more abundant in osteosarcoma tissues compared to normal tissues (Figure 1). This article systematically introduces the molecular structure and functions of YB1 and its relationship with osteosarcoma, providing new targets for the treatment of this cancer.
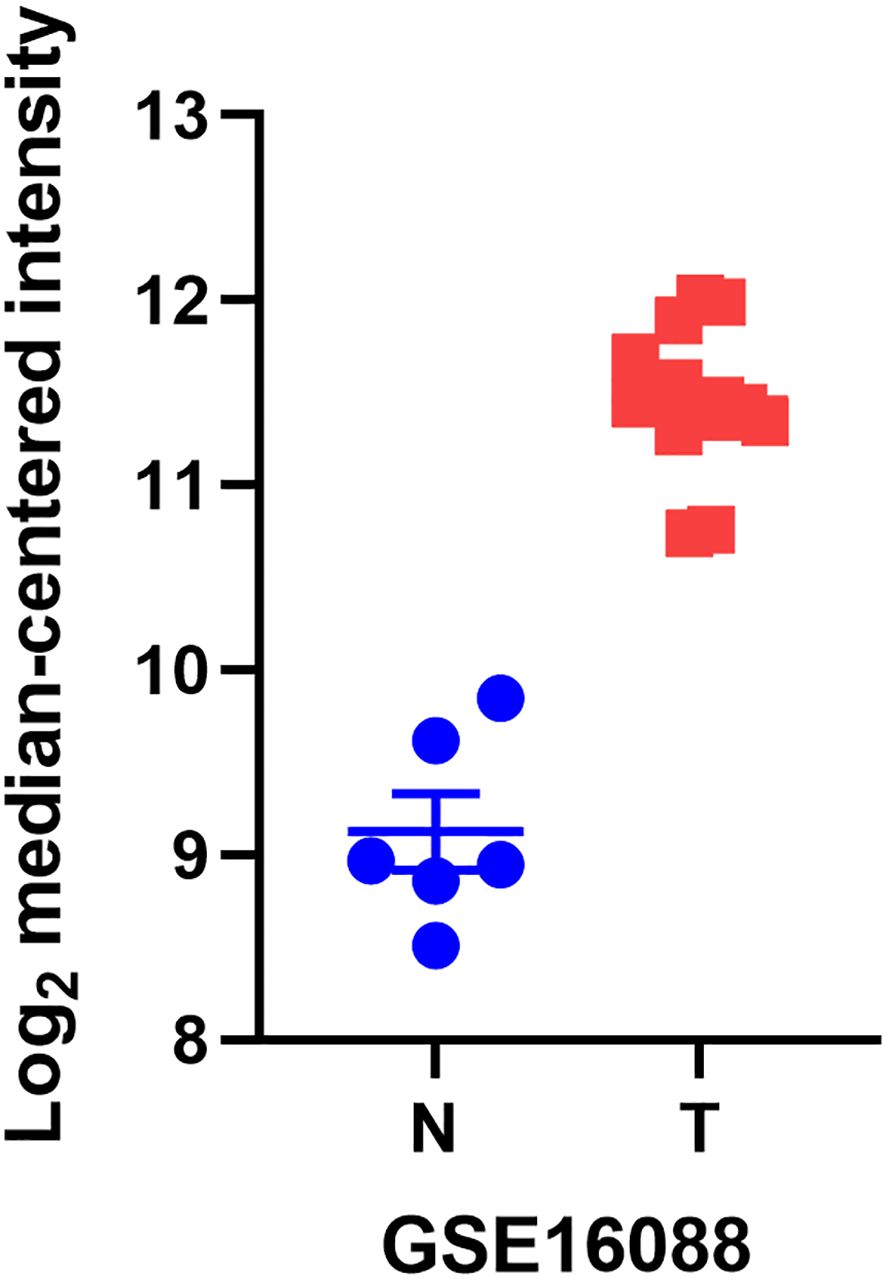
Figure 1. The differential mRNA expression of YB1 in normal kidney, liver, and lymph node tissues compared to osteosarcoma.
2 Structure and expression of YB1
2.1 Molecular structure of YB1
YB-1 is a 42 kDa protein, primarily known as a specific DNA-binding protein and a member of the cold shock domain (CSD) protein superfamily. It was initially discovered for its ability to recognize and bind the Y box sequence 5′-CTGATTGGCCAA-3′ (an inverted CCAAT box) in the promoter of MHC class II genes. Subsequently, YB1 was found to be a major component of messenger ribonucleoprotein particles in the cytoplasm, interacting with mRNA (19, 20). The YBX1 gene, which encodes YB1, is located at 1p34 on chromosome 1 (21). It has three functional domains (Figure 2): a variable N-terminal domain rich in alanine and proline (A/P domain); a centrally located evolutionarily conserved CSD protein, which can bind nucleic acids specifically or non-specifically; and a hydrophilic C-terminal region (CTD), which can bind nucleic acids and many proteins (22, 23). The CTD regulates the distribution of YB1, containing atypical nuclear localization signals (NLS) and cytoplasmic retention signals (CRS). Under normal circumstances, CRS dominates over NLS, thus YB1 is mainly located in the cytoplasm (24, 25).
2.2 Expression of YB1
YB1 expression is dynamically regulated during developmental stages and in various tissue states. Its crucial role in embryonic development is underscored by the lethality observed in ybx1 gene knockout mouse embryos (26–28). As age increases, its expression declines, and its abnormal expression can serve as both a prognostic factor and a target for cancer therapy (24, 29, 30). For example, YBX1 is not expressed or maintained at very low levels in normal adult liver, but its expression increases again during fetal development or liver injury repair, as well as in liver cancer (31). Studies have shown that overexpression of YBX1 is associated with various tumor types’ resistance to therapy, cell proliferation, and prognosis, such as breast cancer, esophageal cancer, lung cancer, sarcomas, prostate cancer, glioblastoma, and non-Hodgkin lymphoma (32, 33). The expression of YB-1 is also regulated by other factors. At the transcriptional regulation level, certain transcription factors, DNA methylation, and histone modifications, as well as hypoxic environments, can affect its transcription. At the translational level, factors such as the structure of the mRNA and specific miRNAs can impact YB-1 translation. At the post-transcriptional level, the stability of YB-1 is influenced by various post-translational modifications, such as phosphorylation and ubiquitination. These modifications may affect the degradation and function of YB-1, thereby influencing its biological roles within the cell (34–37).
3 The role of YB1 in normal physiological functions
3.1 Regulation of bone marrow stromal cell (BMSC) activity
Alternative splicing (AS) as an important post-transcriptional regulatory pathway contributes to gene expression diversity and is related to the aging of BMSCs in humans and mice. The expression of splicing factor YB1 in BMSCs declines with cell aging. The loss of YB1 can lead to erroneous splicing of genes related to osteogenic differentiation and aging (such as Fn1, Nrp2, Sirt2, Sp7, and Spp1), thereby promoting the aging and bone loss of BMSCs, while stimulating overexpression of YB1 inhibits BMSC aging and promotes bone formation (38) (Figure 3).
3.2 DNA/RNA binding function
YB1 was initially identified as a transcription factor due to its ability to bind and enhance the activity of major histocompatibility complex (MHC) class II and epidermal growth factor receptor (EGFR) genes. In the cell nucleus, it acts as a transcription factor, while in the cytoplasm, it forms complexes with messenger ribonucleoproteins (mRNPs) and regulates mRNA stability (34). Studies have shown that YB1 acts as a transcriptional regulator involved in the transcriptional regulation of genes related to cell proliferation such as E2F1. Phosphorylated YB1 is an important regulator for RNPs, as unphosphorylated YB1 binds to translationally inactive mRNPs, inhibiting internal ribosome-dependent mRNA translation. However, once YB1 is phosphorylated at serine 102 (S102), its ability to bind mRNA is reduced, allowing mRNA translation and thereby regulating cell proliferation (39). For example, ribosomal S6 kinase 2 (RSK2) mediated phosphorylation of YB-1 at the Ser102 site promotes the formation of the YB-1/KLF5 transcription complex, which jointly regulates the expression of BLBC-specific genes such as keratin 16 (KRT16) and lymphocyte antigen 6 family member D (Ly6D), promoting cancer cell proliferation (40) (Figure 3).
3.3 Signal pathway regulation
YB1 promotes osteogenic differentiation of bone marrow MSCs by activating the PI3K/AKT pathway and can also lead to activation of the PI3K/AKT pathway by reducing the expression of the tumor suppressor gene PTEN (41, 42). Moreover, YB1 can control the mutation state of RAS/RAF, thereby regulating cell apoptosis and EGFR expression (43). Evidence suggests that the PI3K/AKT and RAS/RAF pathways are related to cancer formation, so YB1 is inextricably linked with cancer; YB1, through its role as a splicing factor, participates in regulating RNA splicing processes, thereby affecting the ERK signaling pathway; YB1 has been found to be a key factor in the persistence of JAK2 mutant tumors. Studies have shown that inactivation of YB1 increases cell sensitivity to JAK2 inhibitors, thereby promoting cell apoptosis. This finding reveals the important role of YBX1 in the survival and proliferation of JAK2 mutant cells, pointing out a potential therapeutic target, i.e., targeting YBX1 to enhance the efficacy of JAK2 inhibitors, providing a new strategy for treating JAK2 mutant-related diseases (6) (Figure 3).
3.4 Serving as an m5C binding protein
Methylation is the most common form of RNA modification, and 5-methylcytosine (m5C) is a post-transcriptional RNA modification involved in the progression of many cancers (44). However, YB-1 has been defined as an RNA m5C binding protein (45). Recent studies have shown that YB-1 preferentially binds m5C RNA through interactions with two tryptophan residues (Trp45 and Trp65) in the CSD, which is particularly important in the progression of diseases such as bladder cancer, as YB-1 overexpression is associated with tumor proliferation and metastasis. Specifically, YB-1 promotes cancer cell proliferation by stabilizing specific m5C-modified mRNAs, such as HDGF and KLF5 mRNA. Additionally, YB-1 also plays a role in stabilizing m5C-modified maternal mRNAs during early embryonic development in zebrafish and in the development of adult stem cells and the maintenance of germline stem cells in fruit flies (46, 47) (Figure 3).
3.5 Involvement in cellular stress responses
Of particular interest is YB-1’s role in the cellular stress response, especially in the formation of stress granules. These are non-membranous organelles that form in response to environmental stressors such as heat shock, oxidative stress, or viral infections. Stress granules consist predominantly of untranslated mRNAs and associated proteins, serving as protective storage sites for these molecules. YB-1 is implicated in both the assembly and regulatory functions of stress granules, which are vital for cell survival under stress conditions (48) (Figure 3).
These aspects are critical in understanding the multifaceted roles of YB-1 in cell biology. If these elements are not addressed in the review, it could lead to an incomplete understanding of YB-1’s functions and its significance in various cellular contexts.
4 The role of YB1 in cancers
4.1 Inhibition of cancer cell apoptosis
Studies have shown that low expression of YB1 promotes apoptosis of laryngeal squamous cell carcinoma (LSCC) cells and glioma stem cells, inhibiting cancer cell proliferation and metastasis (49, 50). YB1 can interact with insulin-like growth factor receptor 2 messenger RNA binding protein (IGF2BP) and stabilize N6-methyladenosine (m6A) modified RNA such as MYC and BCL2 mRNA, supporting the survival of myeloid leukemia cells, although YB1 does not appear to affect normal hematopoietic function (51). YB1 interacts with the potential oncogene LINC00857, preventing its degradation by the proteasome and increasing its nuclear translocation, and also regulates the protein expression of p-EGFR, p-AKT, and their downstream target genes, thus affecting cell apoptosis (52–54). YB-1 may also act as a transcriptional inhibitor of the cell death-related Fas receptor gene and can directly regulate the expression and activity of the apoptosis regulator p53 to inhibit cancer cell apoptosis (55). Cells deficient in YB1 seem to be more susceptible to TNF-induced apoptosis (56) (Figure 3).
4.2 Promotion of cancer angiogenesis
In mouse models, YB1 in endothelial cells can upregulate the motility of endothelial cells and the secretion of angiogenic factors in extracellular vesicles (including exosomes), thus playing a crucial role in endothelial cell-induced tumor formation and angiogenesis and YB-1 is transferred to human umbilical vein endothelial cells (HUVECs) via gastric cancer cell-derived exosomes, directly promoting cancer angiogenesis by upregulating key angiogenic factors (57). YB1 can interact with linc00665 and activate the YB1-ANGPT4/ANGPTL3/VEGF axis to induce tumor-related angiogenesis in LUAD (58);YB1 can also bind to the GC boxes in the promoters of Klf4, SM22α, p21, and cyclin D1, promoting VSMC differentiation. Since VSMCs are major components of the vascular wall and play a crucial role in vascular formation, remodeling, and angiogenesis, they further promote cancer angiogenesis (59, 60). Using antisense oligonucleotides targeting YB1 (YB1-ASOA) can specifically inhibit YB-1 protein expression in tumor vascular endothelial cells, thereby downregulating the Bcl-xL/VEGFR2 and Bcl-xL/Tie signaling axes, inhibiting these two key angiogenic regulatory factors, reducing angiogenesis, and thereby inhibiting tumor growth, which also indirectly illustrates YB1’s role in promoting cancer vascular growth (61) (Figure 3).
4.3 Induction of tumor drug resistance
YB1 acts as a transcriptional activator, promoting the expression of multiple genes associated with drug resistance. This includes genes such as MDR1, MVP/LRP, MYC, CD44, BCL2, PCNA, TOP2A, p53, CD49f, ABCB1, and androgen receptor (AR). This upregulation contributes to the ability of cancer cells to resist various anti-cancer treatments. The expression of YB1 is usually associated with resistance to platinum drugs (such as cisplatin) and also promotes the malignant progression of cancer cells and acquisition of drug resistance by regulating genes involved in cell proliferation and the cell cycle, such as EGFR and HER2 (62–66); targeting the AKT/mTOR/p70S6k or MEK/ERK/p90RSK signaling pathways to inhibit downstream YB1 activity can inhibit tumor drug resistance (67) (Figure 3).
5 The role of YB1 in osteosarcoma
5.1 YB1 regulation of VEGF promotes osteosarcoma progression
Vascular endothelial growth factor (VEGF) is highly expressed in osteosarcoma and can act as a paracrine molecule in the tumor microenvironment to promote tumor angiogenesis, as well as an autocrine molecule to promote tumor proliferation and metastasis, thus promoting the occurrence and development of osteosarcoma and being related to the prognosis of osteosarcoma. Among these, VEGF165 and its homologous isoform VEGF165b, although identical in length, have completely opposite effects; the former promotes cancer while the latter inhibits it. As a splicing factor, YB1 modulates the expression of VEGF165 and VEGF165b, leading to increased VEGF165 levels and decreased VEGF165b expression. This alteration in VEGF isoform balance promotes osteosarcoma cell formation and metastasis (68, 69).
5.2 YB1 interaction with RNA affects osteosarcoma progression
Circular RNA (circRNA) can regulate gene expression in many malignancies by capturing microRNAs (miRNAs). Studies have shown that circ0001658 and YB1 are highly expressed in osteosarcoma and promote the proliferation and metastasis of osteosarcoma, while miR-382 is expressed very little, inhibiting the progression of osteosarcoma. The promotion of osteosarcoma development by circ0001658 is related to its regulation of miR-382-5p/YB1 expression. Circ0001658 negatively regulates miR-382-5p while positively regulating YB1 expression, thereby promoting the occurrence and development of osteosarcoma. In contrast, miR-382 targets and inhibits YB1 expression, exerting its inhibitory effect on osteosarcoma progression (70, 71).
5.3 The critical role of YB-1 in activating HIF-1α during osteosarcoma progression
Hypoxia or low oxygen is a common feature of solid tumors, including sarcomas (72). To cope with hypoxic conditions, tumors initiate transcription and other processes to adapt to this environment. Hypoxia promotes cancer progression and metastasis, among which the key molecule is tumor hypoxia-inducible factor 1 (HIF-1). Under hypoxic conditions, the expression of HIF-1 increases, and it participates in carcinogenesis and tumor growth by regulating genes involved in angiogenesis, glycolytic metabolism, and other biological mechanisms (73). Cancer cells switch their metabolism from oxidative phosphorylation to aerobic glycolysis under hypoxic conditions, producing large amounts of lactate, a process known as the Warburg effect, which is directly related to HIF-1. Enhanced glycolysis not only meets the ATP demand for rapid tumor growth but also provides sufficient metabolic intermediates for rapid tumor proliferation (74, 75). El-Naggar and colleagues found that YB-1 is a key regulator of hypoxia-inducible factor 1α (HIF1α) expression in sarcoma cells. YB-1 binds to the IRES sequence in the 5′ UTR region of HIF1-α mRNA, activating HIF-1α at the translational level, thereby enhancing the invasion and metastatic capabilities of sarcoma cells, highlighting the importance of the translationally regulated YB-1-HIF1α axis in sarcoma metastasis (37, 76–78).
5.4 YB1 promotes osteosarcoma cell proliferation
YB1 can promote the transcription of cell cycle proteins (D1, A, B1), enhancing cancer cell proliferation. In vitro and in vivo experiments using specific siRNAs to inhibit YB1 expression have also inhibited the expression of cell cycle proteins D1 and A, key regulatory proteins for the G1/S phase transition of the cell cycle, thereby inhibiting the proliferation of osteosarcoma cells (79–81). Studies have shown that YB1 not only affects the G1/S phase transition of the cell cycle but also affects the G2/M phase transition. Silencing YB1 leads to G2/M phase cell cycle arrest (82).
6 The role of YB1 in other tumors
In non-small cell lung cancer, the lncRNA HOXC-AS3 directly interacts with YB1 and inhibits YB1 ubiquitination, thereby reducing YB1 degradation and increasing YB1 levels. HOXC-AS3 and YB1 also promote lung cancer proliferation and metastasis by increasing the amount of HOXC8 (83). In nasopharyngeal cancer, Circlpo7 interacts with cytoplasmic YB1, promoting the phosphorylation of YB1 at S102, further promoting YB1’s nuclear translocation, and activating FGFR1, TNC, and NTRK1 transcription, thereby promoting nasopharyngeal cancer metastasis and resistance to cisplatin (84). YB1 can bind to the promoter region of cytidine nucleotide triphosphatase (CTPS1) in triple-negative breast cancer (estrogen receptor-deficient, progesterone receptor-deficient, human epidermal growth factor 2-deficient) cells and activate CTPS1 transcription, increasing CTPS1 expression, and thereby promoting breast cancer proliferation and metastasis (85). The long non-coding RNA (lncRNA) AWPPH is highly expressed in liver cancer and indicates a poor prognosis. At the same time, the interaction between lncRNA AWPPH and YB1 promotes liver cancer cell proliferation and metastasis (86). The lncRNA lnc-SOX9-4 can increase YB1 levels, thereby enhancing the proliferation and metastasis capabilities of colorectal cancer (CRC) cells (87). YB1 is also significantly related to many other tumors, which are not all listed here.
7 Research on YB1 as a target for treating osteosarcoma
How to target and inhibit YB1 to treat osteosarcoma is a current challenge. Studies have shown that miR-382 can target and inhibit YB-1 expression at the 3′UTR, thereby inhibiting the proliferation, invasion, and epithelial-mesenchymal transition of osteosarcoma cells. Simultaneously, the combination of miR-382 with chemotherapeutic drugs such as doxorubicin can inhibit osteosarcoma growth and recurrence and reduce the proportion of cancer stem cells (CSC), making miR-382 a potential target for osteosarcoma treatment (71). Class I HDACs, such as MS-275, can enhance the ROS levels in osteosarcoma, while inhibiting YB1-mediated antioxidant regulator NRF2 expression, thereby indirectly increasing ROS levels in tumor cells. This drug can also enhance the acetylation of lysine 81 in the YB1 RNA-binding domain, thereby losing YB1-mediated translation activation, ultimately blocking the translation and synthesis of NRF2, G3BP1, and HIF1α in sarcoma cells mediated by MS-275, thereby inhibiting the progression of osteosarcoma (88). Somasekharan et al. demonstrated that YB-1 interacts with G3BP1 mRNA transcripts in an osteosarcoma model, which plays a significant role in the biology of osteosarcoma, particularly in tumor progression and metastasis. This interaction highlights how YB-1 may modulate the expression of G3BP1, influencing the invasive capabilities and overall aggressiveness of osteosarcoma cells (89). Niraparib (P1), as a PARP-1 inhibitor, has been approved for use in advanced ovarian cancer treatment. Its main mechanism is to interact with key residues in the Quercetin pocket of YB-1, such as F85 and K118, especially through π-π stacking and van der Waals (vdW) interactions, to specifically disrupt the interaction of YB-1 with mRNA, showing high selectivity for YB-1 (90).
8 Research on YB1 as a target for treating tumors
Strategies to inhibit YB1 and its downstream pathways are currently at the forefront of osteosarcoma research. YB1 is a protein characterized by its intrinsically disordered nature, which means it lacks a stable, fixed three-dimensional structure. This intrinsic disorder poses challenges for traditional structural biology approaches, which typically rely on stable protein conformations. The flexibility conferred by this disorder allows YB-1 to engage in diverse interactions with various RNA and protein partners, playing crucial roles in multiple cellular processes. Here are some examples for reference (89) (Figure 4).
8.1 Inhibition of YB1-related pathways
Studies have shown that the PI3K/mTOR inhibitor BEZ235 can inhibit YB1 expression and enhance the toxicity of radiation to cells. The combined treatment of BEZ235 and radiotherapy has shown strong anti-tumor effects in mouse models (91).
8.2 Gene interference technology inhibits YB1
The circRNA circNEIL3 (hsa_circ_0001460) and Nfix circRNA (circNfix) have shown strong anti-cancer effects in in vitro and in vivo models. CircNEIL3 directly acts on YB1, promoting Nedd4L-mediated proteasome elevation to enzymatically degrade oncogenic protein YB1, thereby reducing YB1 levels and exerting an inhibitory effect on cancer metastasis (92, 93). The lncRNA LINC00472 interacts with YB1, inhibiting the epithelial-to-mesenchymal transition of epithelial cells and affecting the mechanical properties of cancer cells, thereby inhibiting the invasion and metastasis capabilities of lung cancer cells (94). MiR-216a can directly bind to the 3′ untranslated region of the YB-1 gene to inhibit YB-1 expression, thereby inhibiting the invasion and metastasis of pancreatic cancer (95). Linc01612 promotes the ubiquitination and proteasome pathway degradation of YBX1, thereby reducing YB1 protein stability, reducing YB1 protein levels, but not affecting its RNA expression levels, exerting its inhibitory effect in hepatocellular carcinoma (36). SiRNA knockdown of Ybx1 can reduce Ybx1 mRNA levels by about 80%. Disrupting YB1’s ability to bind to related mRNA can also inhibit tumor development (96).
It is also possible to design DNA/RNA sequences that specifically bind to YB1 mRNA and attach corresponding specific carriers to enhance the enzymatic cleavage of YB1 mRNA, thereby inhibiting YB1 (97).
8.3 Inhibition of YB1 phosphorylation at relevant sites
RBM10 forms a ternary complex with YB1 and PPM1B to inhibit YB1 phosphorylation, or natural products such as lacquer yellow, TAS0612/everolimus inhibit AKT/mTORC1-mediated phosphorylation of YB1 at ser102, thereby inhibiting tumor drug resistance and further development (63, 98–100). However, not all phosphorylation sites of YB1 promote tumor formation. For example, when YB-1 protein is phosphorylated by Akt kinase at serine 209 (S209), its nuclear translocation activity is inhibited. This phosphorylation prevents the nuclear import of YB-1 protein, which is otherwise facilitated by phosphorylation at serine 102 (S102) (35).
8.4 Direct physical interaction of SU056 inhibits YB-1 function and protein levels
SU056, a small molecule drug, directly physically interacts with YB-1, significantly reducing YB-1 protein levels and affecting various proteins and pathways related to YB-1 functions, including apoptosis, RNA degradation, and cell cycle regulation, effectively inhibiting YB-1 (101, 102).
8.5 Inhibition of YB1 binding to mRNA
TET2 is a DNA dioxygenase, which acts on m5C in mRNA through its DNA dioxygenase activity, oxidizing it to form 5-hydroxymethylcytosine (5hmC). TET2’s oxidation weakens YBX1’s binding affinity to mRNA because YBX1’s recognition ability for 5hmC-modified mRNA is weaker than that for 5mC, thus indirectly inhibiting YBX1’s function by weakening its binding to target mRNA, thereby reducing mRNA stability and inhibiting tumor development. Similarly, α-ketoglutarate-dependent dioxygenase ABH1 (ALKBH1) has a similar effect (103, 104).
8.6 Traditional Chinese medicine (TCM)
Yifei Sanjie (YFSJ), a well-applied traditional Chinese medicine formula, can inhibit YB1 expression, thereby inhibiting lung tumor progression (105).
In summary, significant progress has been made in the research on targeting YB-1 for the treatment of osteosarcoma. Gene interference techniques, natural products, and small molecule drugs each demonstrate distinct advantages and potential (Table 1). In the future, the combination of multiple strategies and combination therapies may provide more effective approaches for the clinical treatment of osteosarcoma.
9 Outlook
In summary, the splicing factor YB1 is highly expressed in osteosarcoma cells and promotes the proliferation, metastasis, angiogenesis, and inhibition of apoptosis of osteosarcoma cells through its influence on downstream pathways. Researching YB1 helps further study the mechanisms of osteosarcoma and other tumors’ occurrence and development, thereby better treating osteosarcoma. However, there are still many issues to be resolved regarding YB1: (1) The downstream pathways of YB1 are very complex, and how YB1 affects the occurrence and development of tumors in different tumors still requires further study. (2) How to target and inhibit YB1 is a current challenge, and few drugs can precisely target and inhibit YB1. (3) Whether inhibiting YB1 will produce irreversible effects on cells other than tumor cells is unknown. (4) The mechanism of tumor onset is complex, and single-target treatment cannot effectively treat osteosarcoma. It is very urgent to seek other targets in the mechanism of osteosarcoma onset. This review offers insights into potential combinatorial therapeutic approaches targeting YB1 alongside other molecular targets, paving the way for more effective osteosarcoma treatments.
Author contributions
FW: Data curation, Visualization, Writing – original draft, Writing – review & editing. DL: Funding acquisition, Supervision, Writing – review & editing.
Funding
The author(s) declare that no financial support was received for the research, authorship, and/or publication of this article.
Conflict of interest
The authors declare that the research was conducted in the absence of any commercial or financial relationships that could be construed as a potential conflict of interest.
Publisher’s note
All claims expressed in this article are solely those of the authors and do not necessarily represent those of their affiliated organizations, or those of the publisher, the editors and the reviewers. Any product that may be evaluated in this article, or claim that may be made by its manufacturer, is not guaranteed or endorsed by the publisher.
References
1. Chen J, Liu G, Wu Y, Ma J, Wu H, Xie Z, et al. CircMYO10 promotes osteosarcoma progression by regulating miR-370-3p/RUVBL1 axis to enhance the transcriptional activity of beta-catenin/LEF1 complex via effects on chromatin remodeling. Mol Cancer. (2019) 18:150. doi: 10.1186/s12943-019-1076-1
2. Zeng L, Liu L, Ni WJ, Xie F, Leng XM. Circular RNAs in osteosarcoma: An update of recent studies (Review). Int J Oncol. (2023) 63 (5). doi: 10.3892/ijo.2023.5571
3. Bian J, Liu Y, Zhao X, Meng C, Zhang Y, Duan Y, et al. Research progress in the mechanism and treatment of osteosarcoma. Chin Med J (Engl). (2023) 136:2412–20. doi: 10.1097/CM9.0000000000002800
4. Yang C, Tian Y, Zhao F, Chen Z, Su P, Li Y, et al. Bone microenvironment and osteosarcoma metastasis. Int J Mol Sci. (2020) 21(19). doi: 10.3390/ijms21196985
5. Stanley RF, Abdel-Wahab O. Dysregulation and therapeutic targeting of RNA splicing in cancer. Nat Cancer. (2022) 3:536–46. doi: 10.1038/s43018-022-00384-z
6. Jayavelu AK, Schnoder TM, Perner F, Herzog C, Meiler A, Krishnamoorthy G, et al. Splicing factor YBX1 mediates persistence of JAK2-mutated neoplasms. Nature. (2020) 588:157–63. doi: 10.1038/s41586-020-2968-3
7. Zhao S. Alternative splicing, RNA-seq and drug discovery. Drug Discovery Today. (2019) 24:1258–67. doi: 10.1016/j.drudis.2019.03.030
8. Dai Z, Sun Y, Maihemuti M, Jiang R. Genome-wide identification of alternative splicing and splicing regulated in immune infiltration in osteosarcoma patients. Front Genet. (2023) 14:1051192. doi: 10.3389/fgene.2023.1051192
9. Bessa C, Matos P, Jordan P, Gonçalves V. Alternative splicing: expanding the landscape of cancer biomarkers and therapeutics. Int J Mol Sci. (2020) 21(23). doi: 10.3390/ijms21239032
10. Bonner EA, Lee SC. Therapeutic targeting of RNA splicing in cancer. Genes (Basel). (2023) 14(7). doi: 10.3390/genes14071378
11. Bradley RK, Anczukow O. RNA splicing dysregulation and the hallmarks of cancer. Nat Rev Cancer. (2023) 23:135–55. doi: 10.1038/s41568-022-00541-7
12. Liu M, Zhang S, Zhou H, Hu X, Li J, Fu B, et al. The interplay between non-coding RNAs and alternative splicing: from regulatory mechanism to therapeutic implications in cancer. Theranostics. (2023) 13:2616–31. doi: 10.7150/thno.83920
13. Qi F, Li Y, Yang X, Wu YP, Lin LJ, Liu XM. Significance of alternative splicing in cancer cells. Chin Med J (Engl). (2020) 133:221–8. doi: 10.1097/CM9.0000000000000542
14. Zhang Y, Qian J, Gu C, Yang Y. Alternative splicing and cancer: a systematic review. Signal Transduct Target Ther. (2021) 6:78. doi: 10.1038/s41392-021-00486-7
15. Li S, Huang X, Zheng S, Zhang W, Liu F, Cao Q. High expression of SRSF1 facilitates osteosarcoma progression and unveils its potential mechanisms. BMC Cancer. (2024) 24:580. doi: 10.1186/s12885-024-12346-y
16. Wang P, Yang L, Dong J, Liu W, Xie F, Lu Y, et al. The sEVs miR-487a/Notch2/GATA3 axis promotes osteosarcoma lung metastasis by inducing macrophage polarization toward the M2-subtype. Cancer Cell Int. (2024) 24:301. doi: 10.1186/s12935-024-03488-x
17. Hartley AV, Wang B, Mundade R, Jiang G, Sun M, Wei H, et al. PRMT5-mediated methylation of YBX1 regulates NF-kappaB activity in colorectal cancer. Sci Rep. (2020) 10:15934. doi: 10.1038/s41598-020-72942-3
18. Lan P, Li M, Wang Y, Wang J, Li L, Zhang S, et al. Y-box protein-1 modulates circSPECC1 to promote glioma tumorigenesis via miR-615-5p/HIP1/AKT axis. Acta Biochim Biophys Sin (Shanghai). (2023) 55:1902–12. doi: 10.3724/abbs.2023230
19. Jung YM, Yu KL, Park SH, Lee SD, Kim MJ, You JC. Investigation of function and regulation of the YB-1 cellular factor in HIV replication. BMB Rep. (2018) 51:290–5. doi: 10.5483/BMBRep.2018.51.6.231
20. Yang XJ, Zhu H, Mu SR, Wei WJ, Yuan X, Wang M, et al. Crystal structure of a Y-box binding protein 1 (YB-1)-RNA complex reveals key features and residues interacting with RNA. J Biol Chem. (2019) 294:10998–1010. doi: 10.1074/jbc.RA119.007545
21. Bergmann S, Royer-Pokora B, Fietze E, Jürchott K, Hildebrandt B, Trost D, et al. YB-1 provokes breast cancer through the induction of chromosomal instability that emerges from mitotic failure and centrosome amplification. Cancer Res. (2005) 65:4078–87. doi: 10.1158/0008-5472.CAN-04-4056
22. Gao W, Chen L, Lin L, Yang M, Li T, Wei H, et al. SIAH1 reverses chemoresistance in epithelial ovarian cancer via ubiquitination of YBX-1. Oncogenesis. (2022) 11:13. doi: 10.1038/s41389-022-00387-6
23. Matsumoto K, Kose S, Kuwahara I, Yoshimura M, Imamoto N, Yoshida M. Y-box protein-associated acidic protein (YBAP1/C1QBP) affects the localization and cytoplasmic functions of YB-1. Sci Rep. (2018) 8:6198. doi: 10.1038/s41598-018-24401-3
24. Kosnopfel C, Sinnberg T, Schittek B. Y-box binding protein 1–a prognostic marker and target in tumour therapy. Eur J Cell Biol. (2014) 93:61–70. doi: 10.1016/j.ejcb.2013.11.007
25. Kretov DA, Mordovkina DA, Eliseeva IA, Lyabin DN, Polyakov DN, Joshi V, et al. Inhibition of transcription induces phosphorylation of YB-1 at ser102 and its accumulation in the nucleus. Cells. (2019) 9(1). doi: 10.3390/cells9010104
26. Lindquist JA, Mertens PR. Cold shock proteins: from cellular mechanisms to pathophysiology and disease. Cell Commun Signal. (2018) 16:63. doi: 10.1186/s12964-018-0274-6
27. Meyer N, Schumacher A, Coenen U, Woidacki K, Schmidt H, Lindquist JA, et al. Y-box binding protein 1 expression in trophoblast cells promotes fetal and placental development. Cells. (2020) 9(9). doi: 10.3390/cells9091942
28. Uchiumi T, Fotovati A, Sasaguri T, Shibahara K, Shimada T, Fukuda T, et al. YB-1 is important for an early stage embryonic development: neural tube formation and cell proliferation. J Biol Chem. (2006) 281:40440–9. doi: 10.1074/jbc.M605948200
29. Guens GP. YB-1 protein in breast cancer (Scientific and personal meetings with professor ovchinnikov). Biochem (Mosc). (2022) 87:S86–47. doi: 10.1134/S0006297922140073
30. Yang HW, Kim HD, Kim TS, Kim J. Senescent cells differentially translate senescence-related mRNAs via ribosome heterogeneity. J Gerontol A Biol Sci Med Sci. (2019) 74:1015–24. doi: 10.1093/gerona/gly228
31. Chao HM, Huang HX, Chang PH, Tseng KC, Miyajima A, Chern E. Y-box binding protein-1 promotes hepatocellular carcinoma-initiating cell progression and tumorigenesis via Wnt/beta-catenin pathway. Oncotarget. (2017) 8:2604–16. doi: 10.18632/oncotarget.13733
32. Miao X, Wu Y, Wang Y, Zhu X, Yin H, He Y, et al. Y-box-binding protein-1 (YB-1) promotes cell proliferation, adhesion and drug resistance in diffuse large B-cell lymphoma. Exp Cell Res. (2016) 346:157–66. doi: 10.1016/j.yexcr.2016.07.003
33. Wu W, Xu J, Gao D, Xie Z, Chen W, Li W, et al. TOPK promotes the growth of esophageal cancer in vitro and in vivo by enhancing YB1/eEF1A1 signal pathway. Cell Death Dis. (2023) 14:364. doi: 10.1038/s41419-023-05883-0
34. Wang Y, Su J, Wang Y, Fu D, Ideozu J E, Geng H, et al. The interaction of YBX1 with G3BP1 promotes renal cell carcinoma cell metastasis via YBX1/G3BP1-SPP1- NF-kappaB signaling axis. J Exp Clin Cancer Res. (2019) 38:386. doi: 10.1186/s13046-019-1347-0
35. Sogorina EM, Kim ER, Sorokin AV, Lyabin DN, Ovchinnikov LP, Mordovkina DA, et al. YB-1 phosphorylation at serine 209 inhibits its nuclear translocation. Int J Mol Sci. (2021) 23(1). doi: 10.3390/ijms23010428
36. Liu P, Zhong Q, Song Y, Guo D, Ma D, Chen B, et al. Long noncoding RNA Linc01612 represses hepatocellular carcinoma progression by regulating miR-494/ATF3/p53 axis and promoting ubiquitination of YBX1. Int J Biol Sci. (2022) 18:2932–48. doi: 10.7150/ijbs.69514
37. El-Naggar AM, Veinotte CJ, Cheng H, Grunewald TG, Negri GL, Somasekharan SP, et al. Translational activation of HIF1alpha by YB-1 promotes sarcoma metastasis. Cancer Cell. (2015) 27:682–97. doi: 10.1016/j.ccell.2015.04.003
38. Xiao Y, Cai GP, Feng X, Li Y J, Guo W H, Guo Q, et al. Splicing factor YBX1 regulates bone marrow stromal cell fate during aging. EMBO J. (2023) 42:e111762. doi: 10.15252/embj.2022111762
39. Alkrekshi A, Wang W, Rana PS, Markovic V, Sossey-Alaoui K. A comprehensive review of the functions of YB-1 in cancer stemness, metastasis and drug resistance. Cell Signal. (2021) 85:110073. doi: 10.1016/j.cellsig.2021.110073
40. Jiang D, Qiu T, Peng J, Li S, Tala, Ren W, et al. YB-1 is a positive regulator of KLF5 transcription factor in basal-like breast cancer. Cell Death Differ. (2022) 29:1283–95. doi: 10.1038/s41418-021-00920-x
41. Chen J, Liu Z, Zhang H, Yang Y, Zeng H, Zhong R, et al. YBX1 promotes MSC osteogenic differentiation by activating the PI3K/AKT pathway. Curr Stem Cell Res Ther. (2023) 18:513–21. doi: 10.2174/1574888X17666220805143833
42. Delicato A, Montuori E, Angrisano T, Pollice A, Calabro V. YB-1 oncoprotein controls PI3K/akt pathway by reducing pten protein level. Genes (Basel). (2021) 12(10). doi: 10.3390/genes12101551
43. Nagasu S, Sudo T, Kinugasa T, Yomoda T, Fujiyoshi K, Shigaki T, et al. Y−box−binding protein 1 inhibits apoptosis and upregulates EGFR in colon cancer. Oncol Rep. (2019) 41:2889–96. doi: 10.3892/or.2019.7038
44. Liu K, Xu P, Lv J, Ge H, Yan Z, Huang S, et al. Peritoneal high-fat environment promotes peritoneal metastasis of gastric cancer cells through activation of NSUN2-mediated ORAI2 m5C modification. Oncogene. (2023) 42:1980–93. doi: 10.1038/s41388-023-02707-5
45. Chen YS, Yang WL, Zhao YL, Yang YG. Dynamic transcriptomic m(5) C and its regulatory role in RNA processing. Wiley Interdiscip Rev RNA. (2021) 12:e1639. doi: 10.1002/wrna.v12.4
46. Wang Y, Wei J, Feng L, Li O, Huang L, Zhou S, et al. Aberrant m5C hypermethylation mediates intrinsic resistance to gefitinib through NSUN2/YBX1/QSOX1 axis in EGFR-mutant non-small-cell lung cancer. Mol Cancer. (2023) 22:81. doi: 10.1186/s12943-023-01780-4
47. Yin Q, Zheng M, Luo Q, Jiang D, Zhang H, Chen C. YB-1 as an oncoprotein: functions, regulation, post-translational modifications, and targeted therapy. Cells. (2022) 11(7). doi: 10.3390/cells11071217
48. Guarino AM, Troiano A, Pizzo E, Bosso A, Vivo M, Pinto G, et al. Oxidative stress causes enhanced secretion of YB-1 protein that restrains proliferation of receiving cells. Genes (Basel). (2018) 9(10). doi: 10.3390/genes9100513
49. Li X, Chen G, Liu B, Tao Z, Wu Y, Zhang K, et al. PLK1 inhibition promotes apoptosis and DNA damage in glioma stem cells by regulating the nuclear translocation of YBX1. Cell Death Discovery. (2023) 9:68. doi: 10.1038/s41420-023-01302-7
50. Zhao J, Zhang P, Wang X. YBX1 promotes tumor progression via the PI3K/AKT signaling pathway in laryngeal squamous cell carcinoma. Transl Cancer Res. (2021) 10:4859–69. doi: 10.21037/tcr-21-2087
51. Feng M, Xie X, Han G, Zhang T, Li Y, Li Y, et al. YBX1 is required for maintaining myeloid leukemia cell survival by regulating BCL2 stability in an m6A-dependent manner. Blood. (2021) 138:71–85. doi: 10.1182/blood.2020009676
52. Liang C, Ma Y, Yong L, Yang C, Wang P, Liu X, et al. Y-box binding protein-1 promotes tumorigenesis and progression via the epidermal growth factor receptor/AKT pathway in spinal chordoma. Cancer Sci. (2019) 110:166–79. doi: 10.1111/cas.2019.110.issue-1
53. Su W, Wang L, Zhao H, Hu S, Zhou Y, Guo C, et al. LINC00857 interacting with YBX1 to regulate apoptosis and autophagy via MET and phosphor-AMPKa signaling. Mol Ther Nucleic Acids. (2020) 22:1164–75. doi: 10.1016/j.omtn.2020.10.025
54. Xu WF, Ma YC, Ma HS, Shi L, Mu H, Ou WB, et al. Co-targeting CK2alpha and YBX1 suppresses tumor progression by coordinated inhibition of the PI3K/AKT signaling pathway. Cell Cycle. (2019) 18:3472–90. doi: 10.1080/15384101.2019.1689474
55. Wang H, Sun R, Gu M, Li S, Zhang B, Chi Z, et al. shRNA-mediated silencing of Y-box binding protein-1 (YB-1) suppresses growth of neuroblastoma cell SH-SY5Y in vitro and in vivo. PloS One. (2015) 10:e0127224. doi: 10.1371/journal.pone.0127224
56. Shah A, Plaza-Sirvent C, Weinert S, Buchbinder JH, Lavrik IN, Mertens PR, et al. YB-1 mediates TNF-induced pro-survival signaling by regulating NF-kappaB activation. Cancers (Basel). (2020) 12(8). doi: 10.3390/cancers12082188
57. Xue X, Huang J, Yu K, Chen X, He Y, Qi D, et al. YB-1 transferred by gastric cancer exosomes promotes angiogenesis via enhancing the expression of angiogenic factors in vascular endothelial cells. BMC Cancer. (2020) 20:996. doi: 10.1186/s12885-020-07509-6
58. Cong Z, Diao Y, Li X, Jiang Z, Xu Y, Zhou H, et al. Long non-coding RNA linc00665 interacts with YB-1 and promotes angiogenesis in lung adenocarcinoma. Biochem Biophys Res Commun. (2020) 527:545–52. doi: 10.1016/j.bbrc.2020.04.108
59. Cao X, Zhu N, Li L, Zhang Y, Chen Y, Zhang J, et al. Y-box binding protein 1 regulates ox-LDL mediated inflammatory responses and lipid uptake in macrophages. Free Radic Biol Med. (2019) 141:10–20. doi: 10.1016/j.freeradbiomed.2019.05.032
60. Shi JH, Cui NP, Wang S, Zhao MZ, Wang B, Wang YN, et al. Overexpression of YB1 C-terminal domain inhibits proliferation, angiogenesis and tumorigenicity in a SK-BR-3 breast cancer xenograft mouse model. FEBS Open Bio. (2016) 6:33–42. doi: 10.1002/2211-5463.12004
61. Setoguchi K, Cui L, Hachisuka N, Obchoei S, Shinkai K, Hyodo F, et al. Antisense oligonucleotides targeting Y-box binding protein-1 inhibit tumor angiogenesis by downregulating bcl-xL-VEGFR2/-tie axes. Mol Ther Nucleic Acids. (2017) 9:170–81. doi: 10.1016/j.omtn.2017.09.004
62. Inoue I, Matsumoto K, Yu Y, Bay BH. Surmounting chemoresistance by targeting the Y-box binding protein-1. Anat Rec (Hoboken). (2012) 295:215–22. doi: 10.1002/ar.v295.2
63. Kuwano M, Shibata T, Watari K, Ono M. Oncogenic Y-box binding protein-1 as an effective therapeutic target in drug-resistant cancer. Cancer Sci. (2019) 110:1536–43. doi: 10.1111/cas.2019.110.issue-5
64. Maurya PK, Mishra A, Yadav BS, Singh S, Kumar P, Chaudhary A, et al. Role of Y Box Protein-1 in cancer: As potential biomarker and novel therapeutic target. J Cancer. (2017) 8:1900–7. doi: 10.7150/jca.17689
65. Taylor L, Wade PK, Johnson JEC, Aldighieri M, Morlando S, Di Leva G, et al. Drug resistance in medulloblastoma is driven by YB-1, ABCB1 and a seven-gene drug signature. Cancers (Basel). (2023) 15(4). doi: 10.3390/cancers15041086
66. Tong H, Zhao K, Zhang J, Zhu J, Xiao J. YB-1 modulates the drug resistance of glioma cells by activation of MDM2/p53 pathway. Drug Des Devel Ther. (2019) 13:317–26. doi: 10.2147/DDDT.S185514
67. Dong H, Jian Y, Yu T, Wang M, Zhang W, Peng Z. YB-1 expression is associated with lymph node metastasis and drug resistance to adriamycin in breast cancer. Dis Markers. (2023) 2023:4667089. doi: 10.1155/2023/4667089
68. Quan B, Li Z, Yang H, Li S, Yan X, Wang Y. The splicing factor YBX1 promotes the progression of osteosarcoma by upregulating VEGF(165) and downregulating VEGF(165b). Heliyon. (2023) 9:e18706. doi: 10.1016/j.heliyon.2023.e18706
69. Siveen KS, Prabhu K, Krishnankutty R, Kuttikrishnan S, Tsakou M, Alali F Q, et al. Vascular endothelial growth factor (VEGF) signaling in tumour vascularization: potential and challenges. Curr Vasc Pharmacol. (2017) 15:339–51. doi: 10.2174/1570161115666170105124038
70. Wang L, Wang P, Su X, Zhao B. Circ_0001658 promotes the proliferation and metastasis of osteosarcoma cells via regulating miR-382-5p/YB-1 axis. Cell Biochem Funct. (2020) 38:77–86. doi: 10.1002/cbf.v38.1
71. Xu M, Jin H, Xu CX, Sun B, Song ZG, Bi WZ, et al. miR-382 inhibits osteosarcoma metastasis and relapse by targeting Y box-binding protein 1. Mol Ther. (2015) 23:89–98. doi: 10.1038/mt.2014.197
72. Toth RK, Warfel NA. Strange bedfellows: nuclear factor, erythroid 2-like 2 (Nrf2) and hypoxia-inducible factor 1 (HIF-1) in tumor hypoxia. Antioxidants (Basel). (2017) 6(2). doi: 10.3390/antiox6020027
73. Pezzuto A, Carico E. Role of HIF-1 in cancer progression: novel insights. A review. Curr Mol Med. (2018) 18:343–51. doi: 10.2174/1566524018666181109121849
74. Elzakra N, Kim Y. HIF-1alpha metabolic pathways in human cancer. Adv Exp Med Biol. (2021) 1280:243–60. doi: 10.1007/978-3-030-51652-9_17
75. Xu L, Li H, Wu L, Huang S. YBX1 promotes tumor growth by elevating glycolysis in human bladder cancer. Oncotarget. (2017) 8:65946–56. doi: 10.18632/oncotarget.19583
76. Hou P, Chen F, Yong H, Lin T, Li J, Pan Y, et al. PTBP3 contributes to colorectal cancer growth and metastasis via translational activation of HIF-1alpha. J Exp Clin Cancer Res. (2019) 38:301. doi: 10.1186/s13046-019-1312-y
77. Ivanova IG, Park CV, Yemm AI, Kenneth NS. PERK/eIF2alpha signaling inhibits HIF-induced gene expression during the unfolded protein response via YB1-dependent regulation of HIF1alpha translation. Nucleic Acids Res. (2018) 46:3878–90. doi: 10.1093/nar/gky127
78. Smith MR, Costa G. RNA-binding proteins and translation control in angiogenesis. FEBS J. (2022) 289:7788–809. doi: 10.1111/febs.v289.24
79. Cybulski M, Jarosz B, Nowakowski A, Jeleniewicz W, Kutarska E, Bednarek W, et al. Cyclin A correlates with YB1, progression and resistance to chemotherapy in human epithelial ovarian cancer. Anticancer Res. (2015) 35:1715–21.
80. Fujiwara-Okada Y, Matsumoto Y, Fukushi J, Setsu N, Matsuura S, Kamura S, et al. Y-box binding protein-1 regulates cell proliferation and is associated with clinical outcomes of osteosarcoma. Br J Cancer. (2013) 108:836–47. doi: 10.1038/bjc.2012.579
81. Prabhu L, Hartley AV, Martin M, Warsame F, Sun E, Lu T. Role of post-translational modification of the Y box binding protein 1 in human cancers. Genes Dis. (2015) 2:240–6. doi: 10.1016/j.gendis.2015.05.001
82. Kotake Y, Arikawa N, Tahara K, Maru H, Naemura M. Y-box binding protein 1 is involved in regulating the G(2)/M phase of the cell cycle. Anticancer Res. (2017) 37:1603–8. doi: 10.21873/anticanres.11490
83. Su H, Fan G, Huang J, Qiu X. LncRNA HOXC-AS3 promotes non-small-cell lung cancer growth and metastasis through upregulation of YBX1. Cell Death Dis. (2022) 13:307. doi: 10.1038/s41419-022-04723-x
84. Hong X, Li Q, Li J, Chen K, He Q, Zhao Y, et al. CircIPO7 promotes nasopharyngeal carcinoma metastasis and cisplatin chemoresistance by facilitating YBX1 nuclear localization. Clin Cancer Res. (2022) 28:4521–35. doi: 10.1158/1078-0432.CCR-22-0991
85. Lin Y, Zhang J, Li Y, Guo W, Chen L, Chen M, et al. CTPS1 promotes Malignant progression of triple-negative breast cancer with transcriptional activation by YBX1. J Transl Med. (2022) 20:17. doi: 10.1186/s12967-021-03206-5
86. Wang M, Dai M, Wang D, Tang T, Xiong F, Xiang B, et al. The long noncoding RNA AATBC promotes breast cancer migration and invasion by interacting with YBX1 and activating the YAP1/Hippo signaling pathway. Cancer Lett. (2021) 512:60–72. doi: 10.1016/j.canlet.2021.04.025
87. Zhao Y, Yang S, Nie H, Zhang D, Wang T, Sun Q, et al. Promotion of colorectal cancer progression by immune-related lnc-SOX9-4 via suppression of YBX1 poly-ubiquitination and degradation. Cell Signal. (2023) 111:110854. doi: 10.1016/j.cellsig.2023.110854
88. El-Naggar AM, Somasekharan SP, Wang Y, Cheng H, Negri GL, Pan M, et al. Class I HDAC inhibitors enhance YB-1 acetylation and oxidative stress to block sarcoma metastasis. EMBO Rep. (2019) 20:e48375. doi: 10.15252/embr.201948375
89. Somasekharan SP, El-Naggar A, Leprivier G, Cheng H, Hajee S, Grunewald TG, et al. YB-1 regulates stress granule formation and tumor progression by translationally activating G3BP1. J Cell Biol. (2015) 208:913–29. doi: 10.1083/jcb.201411047
90. El Hage K, Babault N, Maciejak O, Desforges B, Craveur P, Steiner E, et al. Targeting RNA:protein interactions with an integrative approach leads to the identification of potent YBX1 inhibitors. Elife. (2023) 12:e80387. doi: 10.7554/eLife.80387
91. Kim A, Shim S, Kim YH, Kim MJ, Park S, Myung JK. Inhibition of Y box binding protein 1 suppresses cell growth and motility in colorectal cancer. Mol Cancer Ther. (2020) 19:479–89. doi: 10.1158/1535-7163.MCT-19-0265
92. Chen S, Li K, Guo J, Chen HN, Ming Y, Jin Y, et al. circNEIL3 inhibits tumor metastasis through recruiting the E3 ubiquitin ligase Nedd4L to degrade YBX1. Proc Natl Acad Sci USA. (2023) 120:e2215132120. doi: 10.1073/pnas.2215132120
93. Huang S, Li X, Zheng H, Si X, Li B, Wei G, et al. Loss of super-enhancer-regulated circRNA nfix induces cardiac regeneration after myocardial infarction in adult mice. Circulation. (2019) 139:2857–76. doi: 10.1161/CIRCULATIONAHA.118.038361
94. Deng X, Xiong W, Jiang X, Zhang S, Li Z, Zhou Y, et al. LncRNA LINC00472 regulates cell stiffness and inhibits the migration and invasion of lung adenocarcinoma by binding to YBX1. Cell Death Dis. (2020) 11:945. doi: 10.1038/s41419-020-03147-9
95. Lu J, Li X, Wang F, Guo Y, Huang Y, Zhu H, et al. YB-1 expression promotes pancreatic cancer metastasis that is inhibited by microRNA-216a. Exp Cell Res. (2017) 359:319–26. doi: 10.1016/j.yexcr.2017.07.039
96. Varma E, Burghaus J, Schwarzl T, Sekaran T, Gupta P, Gorska AA, et al. Translational control of Ybx1 expression regulates cardiac function in response to pressure overload in vivo. Basic Res Cardiol. (2023) 118:25. doi: 10.1007/s00395-023-00996-1
97. Izumi H, Nagao S, Mochizuki S, Fujiwara N, Sakurai K, Morimoto Y. Optimal sequence of antisense DNA to silence YB-1 in lung cancer by use of a novel polysaccharide drug delivery system. Int J Oncol. (2016) 48:2472–8. doi: 10.3892/ijo.2016.3451
98. Khan MI, Adhami VM, Lall RK, Sechi M, Joshi DC, Haidar OM, et al. YB-1 expression promotes epithelial-to-mesenchymal transition in prostate cancer that is inhibited by a small molecule fisetin. Oncotarget. (2014) 5:2462–74. doi: 10.18632/oncotarget.1790
99. Li Y, Wei D, Chen Z, Chen Y, Deng Y, Li M, et al. RBM10 regulates the tumorigenic potential of human cancer cells by modulating PPM1B and YBX1 activities. Exp Cell Res. (2024) 435:113932. doi: 10.1016/j.yexcr.2024.113932
100. Shibata T, Watari K, Kawahara A, Sudo T, Hattori S, Murakami Y, et al. Targeting phosphorylation of Y-box-binding protein YBX1 by TAS0612 and everolimus in overcoming antiestrogen resistance. Mol Cancer Ther. (2020) 19:882–94. doi: 10.1158/1535-7163.MCT-19-0690
101. Tailor D, Resendez A, Garcia-Marques FJ, Pandrala M, Going CC, Bermudez A, et al. Y box binding protein 1 inhibition as a targeted therapy for ovarian cancer. Cell Chem Biol. (2021) 28:1206–20.e6. doi: 10.1016/j.chembiol.2021.02.014
102. Dheeraj A, Garcia Marques FJ, Tailor D, Bermudez A, Resendez A, Pandrala M, et al. Inhibition of protein translational machinery in triple-negative breast cancer as a promising therapeutic strategy. Cell Rep Med. (2024) 5:101552. doi: 10.1016/j.xcrm.2024.101552
103. Chen SJ, Zhang J, Zhou T, Rao SS, Li Q, Xiao LY, et al. Epigenetically upregulated NSUN2 confers ferroptosis resistance in endometrial cancer via m(5)C modification of SLC7A11 mRNA. Redox Biol. (2024) 69:102975. doi: 10.1016/j.redox.2023.102975
104. He J, Lin M, Zhang X, Zhang R, Tian T, Zhou Y, et al. TET2 is required to suppress mTORC1 signaling through urea cycle with therapeutic potential. Cell Discovery. (2023) 9:84. doi: 10.1038/s41421-023-00567-7
Keywords: osteosarcoma, splicing factor, YB1, treatment of osteosarcoma, YBX1 phosphorylation
Citation: Wu F and Li D (2024) YB1 and its role in osteosarcoma: a review. Front. Oncol. 14:1452661. doi: 10.3389/fonc.2024.1452661
Received: 03 July 2024; Accepted: 07 October 2024;
Published: 21 October 2024.
Edited by:
Tamer Saad Kaoud, The University of Texas at Austin, United StatesReviewed by:
Michael M. Lizardo, British Columbia Cancer Agency, CanadaAmal El-Naggar, University of British Columbia, Canada
Copyright © 2024 Wu and Li. This is an open-access article distributed under the terms of the Creative Commons Attribution License (CC BY). The use, distribution or reproduction in other forums is permitted, provided the original author(s) and the copyright owner(s) are credited and that the original publication in this journal is cited, in accordance with accepted academic practice. No use, distribution or reproduction is permitted which does not comply with these terms.
*Correspondence: Dapeng Li, lidapeng706@163.com