- 1State Key Laboratory of Biotherapy, West China Hospital, Sichuan University, Chengdu, Sichuan, China
- 2Cancer Center, Sichuan Provincial People’s Hospital, School of Medicine, University of Electronic Science and Technology of China, Chengdu, Sichuan, China
- 3Department of Thoracic Surgery, West China Hospital, Sichuan University, Chengdu, Sichuan, China
Introduction: Surfactant Protein C gene (SFTPC) is a marker gene of alveolar type 2 cells (AT2), which are the key structures of alveoli. Mutations or deletions in SFTPC cause idiopathic pulmonary fibrosis (IPF). Importantly, IPF is an independent risk factor for non-small cell lung cancer (NSCLC). It suggests that abnormal expression of SFTPC may be relevant to development of NSCLC. However, the function and mechanism of SFTPC in NSCLC are still poor understood until now.
Methods: The expression of SFTPC and the relationship between SFTPC and prognosis of NSCLC were analyzed in TCGA database and our collected clinical NSCLC tissues. Subsequently, the function and mechanism of SFTPC in NSCLC were explored by RNA-sequence, qRT-PCR, Western blot, Immunohistochemical, Wound-healing, Millicell, Transwell assays and mouse tumor xenograft model.
Results: SFTPC was dramatically downregulated in NSCLC tissues from TCGA database and 40 out of 46 collected clinical LUAD tissues compared with adjacent non-tumor tissues. Low expression of SFTPC was associated with poor prognosis of LUAD by TCGA database. Importantly, we confirmed that overexpression of SFTPC significantly inhibited Epithelial-to-Mesenchymal Transition (EMT) process of NSCLC cells by upregulating SOX7 and then inactivating WNT/β-catenin pathway in vitro and in vivo. Particularly, we discovered that low expression of SFTPC was associated with EMT process and low expression of SOX7 in NSCLC tissues.
Conclusion: Our study revealed a novel mechanism of SFTPC in NSCLC development. Meanwhile, it also might provide a new clue for exploring the molecular mechanism about NSCLC development in patients with IPF in the future.
1 Introduction
Lung cancer (LC) is the leading cause of cancer death globally (1). Non-small cell lung cancer (NSCLC), which mainly includes lung adenocarcinoma (LUAD) and lung squamous cell carcinoma (LUSC), is the main subtype of LC, accounting for about 85% of all subtypes (2). Unfortunately, about 70% of NSCLC patients were diagnosed at advanced stage, the 5-year overall survival (OS) rate was only 15% (3). Therefore, it is necessary to explore new molecular mechanisms of NSCLC development. The mammalian lung is a vital organ responsible for gas exchange. The alveoli, which account for 99% of lung’s surface area, are respiratory units of the lung (4). Alveolar epithelium consists of alveolar type 1 cells (AT1) and alveolar type 2 cells (AT2) (4). AT2 cells are the key structures of alveoli. Specially, AT2 cells also serve as alveolar stem cells, they are responsible for the alveolar epithelium repair and regeneration (5). Surfactant Protein C gene (SFTPC) is a marker gene of AT2 cells, which encodes surfactant protein C (SP-C) (6). It had been demonstrated SFTPC was only significantly expressed in mature AT2 cells eventually (7).
The previous studies demonstrated that mutations or deletions in SFTPC could cause idiopathic pulmonary fibrosis (IPF) (8). IPF is a progressive fibrotic interstitial lung disease associated with significant morbidity, high mortality and poor prognosis (9). Epithelial-to-Mesenchymal Transition (EMT) of AT2 cells in human IPF lesion had been observed in the earlier studies (10, 11). Particularly, Luis Rodriguez et al. (12) performed RNA-sequence (RNA-seq) on the SftpcC121G mice with spontaneous pulmonary fibrosis, and the Gene ontogeny (GO) analysis displayed that Sftpc mutation (SftpcC121G) was significantly associated with cell migration. Importantly, the migration abilities of cancer cells was closely related to EMT of cells (13). Therefore, the studies above suggested that abnormal expression of SFTPC might induce EMT process in AT2 cells.
Strikingly, the previous studies demonstrated that abnormal AT2 cells were the origin cells of NSCLC (14, 15). Recently, some investigators revealed that AT2-like cells appeared in human early-stage LUAD tissues by single-cell sequence, and the gene expression profiles of AT2-like cells were significantly different from those of AT2 cells (16, 17). Importantly, several epidemiological researches had indicated that IPF was also one of independent risk factors for NSCLC (18, 19). Meanwhile, the latest epidemiological research in Asia showed that the incidence of NSCLC was notably increased in IPF group than that in non-IPF group, and the hazard ratios of NSCLC in IPF group was 5.89 (19). To sum up, these results above suggested that AT2 cells marker gene SFTPC, which was aberrant in IPF, might also play an important role in development of NSCLC.
The previous researches about abnormal expression of SFTPC in lung disease were mainly focused on IPF. However, only a few studies found the deletions or mutations of SFTPC in clinical NSCLC tissues (20–22). Recently, Antonella F.M. Dost, et al. (16) discovered SFTPC was markedly decreased in AT2-like cells of human early-stage LUAD tissues compared with AT2 cells. Interestingly, the latest study found that compared with AT2-like cells of LUAD in situ, SFTPC and E-cadherin were significantly downregulated in AT2-like cells of invasive LUAD tissues by single cell sequence and RNA fluorescence in situ hybridization (RNA-FISH) (17). It implied that downregulation of SFTPC might be related to EMT process of NSCLC cells. Several studies demonstrated that EMT process enhanced the migration abilities of NSCLC cells, which was correlation with poor prognosis of NSCLC patients (13, 23). Recently, Baile Zuo et al. (24) also discovered that low expression of SFTPC was markedly associated with poor prognosis of NSCLC by multiple databases. However, the function and mechanism of SFTPC in EMT process of NSCLC cells are still poor understood until now.
Therefore, in our present study, the function and molecular mechanism of the AT2 cells marker gene SFTPC in EMT process of NSCLC cells were investigated in human NSCLC cell lines, tumor xenograft models and clinical NSCLC tissues.
2 Materials and methods
2.1 NSCLC patient samples
The approvals for patient samples study were obtained from the West China Hospital of Sichuan University Biomedical Research Ethics Committee and the Medical Ethics Committee, Sichuan Academy of Medical Sciences, Sichuan Provincial People’s Hospital (Chengdu, China). The study was performed in accordance with the Declaration of Helsinki. Written informed consent was obtained from all the patient. Clinical information of all NSCLC tissues was available in Supplementary Information (Supplementary Table S1).
2.2 Bioinformatics
All information of bioinformatics analysis was available in Supplementary Information.
2.3 Cell lines and cell cultures
Human NSCLC cell lines A549 and H1299 were purchased from the American Type Culture Collection (ATCC, Manassas, VA, USA). Full methods were available in Supplementary Information.
2.4 RNA-sequence
RNA-seq was conducted on A549-SFTPC and A549-Control cells. Full methods of RNA-seq analysis were available in Supplementary Information.
2.5 Quantitative real-time PCR
qRT-PCR procedure was performed as described in the previous study (25). To analyze the relative expression levels of genes, the 2-ΔΔCt method was performed on data of this experiment. All methods were available in Supplementary Information.
2.6 Western blot
Western blot procedure was performed as described in the previous study (25). All the primary antibodies were available in Supplementary Information.
2.7 Immunohistochemical staining
IHC staining was performed on the paraffin sections as described in the previous study (25). All methods and primary antibodies were available in Supplementary Information.
2.8 Wound-healing assays
6-well plastic dishes were inoculated with treated cells, then, Wound-healing was performed as described in the previous study (26). Full methods were available in the Supplementary Information.
2.9 Millcell and transwell assays
Cells were transplanted in Millicell or Transwell chambers (8μm pore size, Merck, Millipore, Switzerland), then cultured with serum-free media as described in our previous study (26). Full methods were available in Supplementary Information.
2.10 Tumor xenograft model
The animal study was approved by the West China Hospital of Sichuan University Animal Ethics Committee (Chengdu, China). The study was conducted in accordance with the local legislation and institutional requirements. Full methods were available in the Supplementary Information.
2.11 Statistical analysis
All data analyses were performed by using GraphPad Prism 9.5 software. Data were denoted as Mean ± SD. The analyses about clinical statistical were carried out using χ2 test. Unpaired two-tailed student t test was utilized to determine the statistical significance of two groups. (p values were shown in each figure, and were presented as “*” p< 0.05, “**” p<0.01, “***” p<0.005 and “****” p<0.001.
3 Results
3.1 The expression of SFTPC was significantly decreased in clinical NSCLC tissues and low expression of SFTPC was associated with poor prognosis of LUAD
To clarify the expression of SFTPC in clinical NSCLC samples, the expression of SFTPC was analyzed in TCGA database and 46 pairs of collected LUAD samples and their paired adjacent non-tumors. Firstly, the expression of SFTPC was notably downregulated in 515 LUAD cases compared with 59 adjacent non-tumors cases in TCGA database (Figure 1A). Then, qRT-PCR was conducted in 46 pairs of collected LUAD samples (Supplementary Table S1). The mRNA level of SFTPC was notably decreased in 40 out of 46 (40/46, 87.0%) LUAD samples compared with the matched adjacent non-tumors (Figures 1B, C). Meanwhile, compared with paired adjacent non-tumors, the protein level of proSP-C was observably decreased in tumors by IHC staining (Figure 1D) and Western blot assay (Figure 1E). The score criteria and outcomes for proSP-C of IHC staining were showed in Supplementary Table S2.
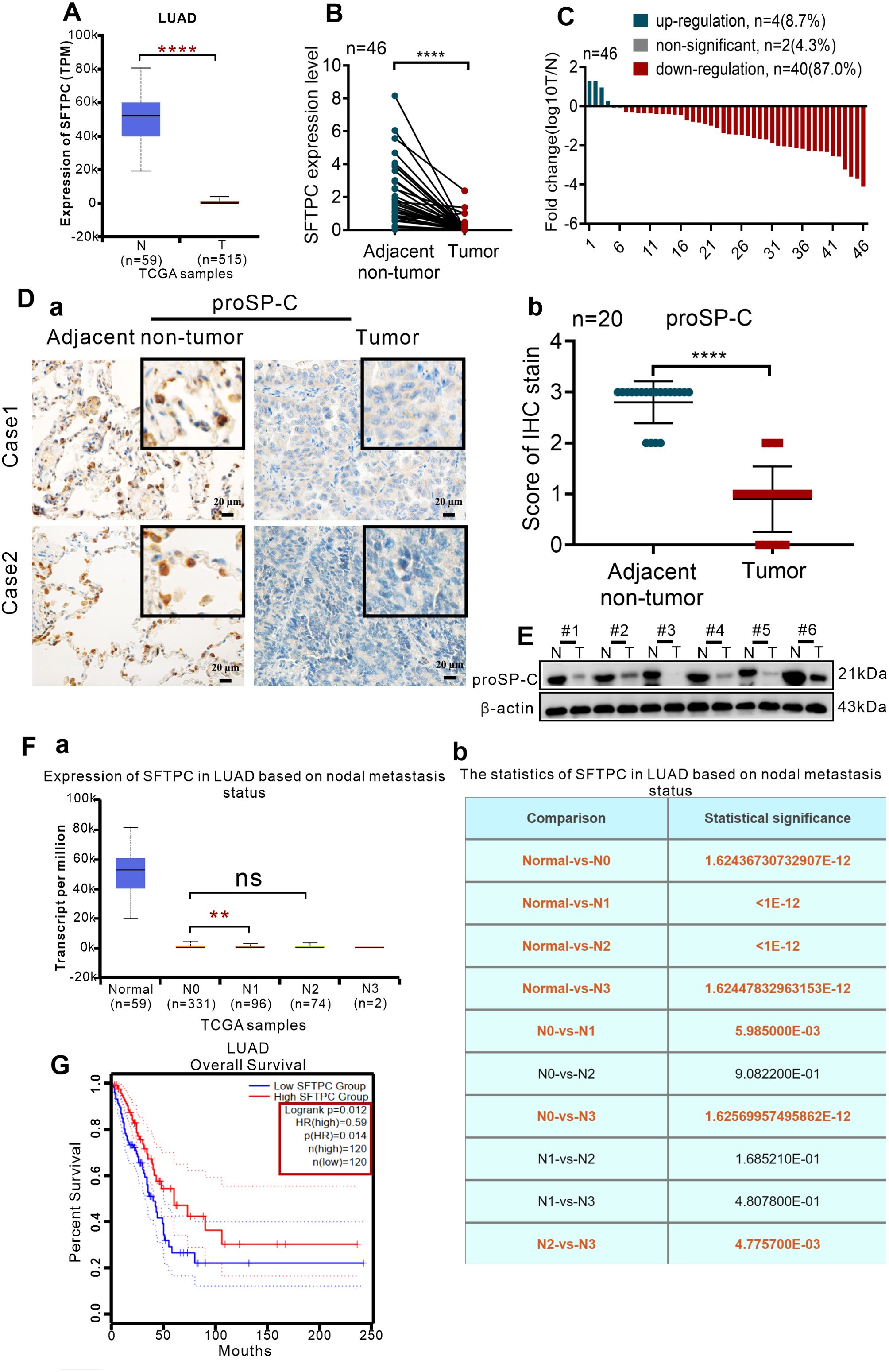
Figure 1. The expression of SFTPC was significantly decreased in clinical LUAD tissues and low expression of SFTPC was associated with poor prognosis of LUAD by a comprehensive analysis of TCGA database and collected clinical LUAD tissues. (A) The expression levels of SFTPC in clinical LUAD tissues and non-tumor tissues (The data were obtained from the TCGA database). T, tumor tissues. N, non-tumor tissues. (B) qRT-PCR analysis was carried out to assess the expression levels of SFTPC in collected clinical LUAD tissues and non-tumor tissues (n=46). β-actin was used as the internal control gene. Paired t-test. (C) The expression levels of SFTPC in collected clinical LUAD tissues were arranged by log10 T/N (n=46). T, tumor tissues. N, non-tumor tissues. (D) IHC staining was conducted on paraffin sections of paired clinical LUAD tissues by using primary antibody against proSP-C (n=20) (a). Staining without primary antibody was used as negative control. Scale bars, 20μm. The histogram was applied to quantify the results of IHC staining (b). The data were presented as the Mean ± SD, unpaired t-test. (E) Western blot assay was conducted on the protein of paired clinical LUAD tissues by using the primary antibody against proSP-C (n=6). (F) The relationship between the expression levels of SFTPC and lymph node metastasis of LUAD tissues (n=503) (a) the statistics were presented in (b) (The data were obtained from the TCGA database). (G) The relationship between the expression levels of SFTPC and the overall survival (OS) rate of LUAD patients (n=240). (The data were obtained from the TCGA database). *p<0.05, **p<0.01, ****p<0.001. ns, no significance.
Moreover, we further investigated the correlation between SFTPC and prognosis of NSCLCpatients in TCGA database and 45 pairs of collected LUAD tissues. Firstly, we found that lower SFTPC was notably correlation with lymph node metastasis of the 503 LUAD cases in TCGA database (Figure 1F, Supplementary Table S3). Meanwhile, survival analysis was performed in 240 cases of LUAD, and indicated that lower SFTPC was correlated with lower OS rate of LUAD patients (Figure 1G). Next, the correlation between SFTPC and age, gender, T stage, lymph node metastasis in 45 pairs of LUAD tissues was further analyzed, respectively. Unfortunately, the low expression of SFTPC had no obvious relationship with age, gender, T stage and lymph node metastasis in our collected 45 pairs of LUAD samples (Supplementary Table S4). All of the outcomes above indicated that the expression of SFTPC was markedly decreased in NSCLC, and TCGA database implied low expression of SFTPC was correlated with poor prognosis of LUAD.
3.2 Overexpression of SFTPC inhibited migration, invasion abilities and EMT process of NSCLC cells in vitro
Our results proved the expression of SFTPC were notably decreased in clinical NSCLC tissues compared with matched adjacent non-tumors. Analogously, other researchers also found the expression of SFTPC was remarkably decreased in NSCLCs compared with adjacent non-tumors (24, 27, 28). Therefore, the stable SFTPC-overexpressing A549 and H1299 cell lines (termed A549-SFTPC and H1299-SFTPC) and control cell lines (termed A549-Control and H1299-Control) were established successfully by lentivirus infection for exploring the functions of SFTPC in NSCLC.
The overexpression efficiencies of SFTPC were verified though qRT-PCR and Western blot (Supplementary Figures S1A–C). Previous studies demonstrated that overexpression of SFTPC repressed the proliferation capacity of NSCLC cells (24, 29). In present study, we focused on the other functions of SFTPC in NSCLC development. Thus, to determine whether overexpression of SFTPC inhibited migration, invasion abilities and EMT process of NSCLC cells, the Wound-healing, Millicell and Transwell assays were performed on A549-SFTPC, H1299-SFTPC and their control cells, respectively. We uncovered that overexpression of SFTPC obviously repressed the migration and invasion abilities of NSCLC cells (Figures 2A, B). And compared to control cells, a significant cobblestone-like shape change was observed in A549-SFTPC cells. However, the morphology of H1299-SFTPC cells was not changed (Figure 2C). Meanwhile, compared to control cells, CDH1(E-cadherin) was notably increased while CDH2(N-cadherin) and Snai2(Slug) were prominently decreased in A549-SFTPC and H1299-SFTPC cells though qRT-PCR and Western blot assays (Figures 2D, E). Taken together, all of these changes in cell movement, cell morphology and biomarkers proved that overexpression of SFTPC notably repressed migration, invasion abilities and EMT process of NSCLC cells.
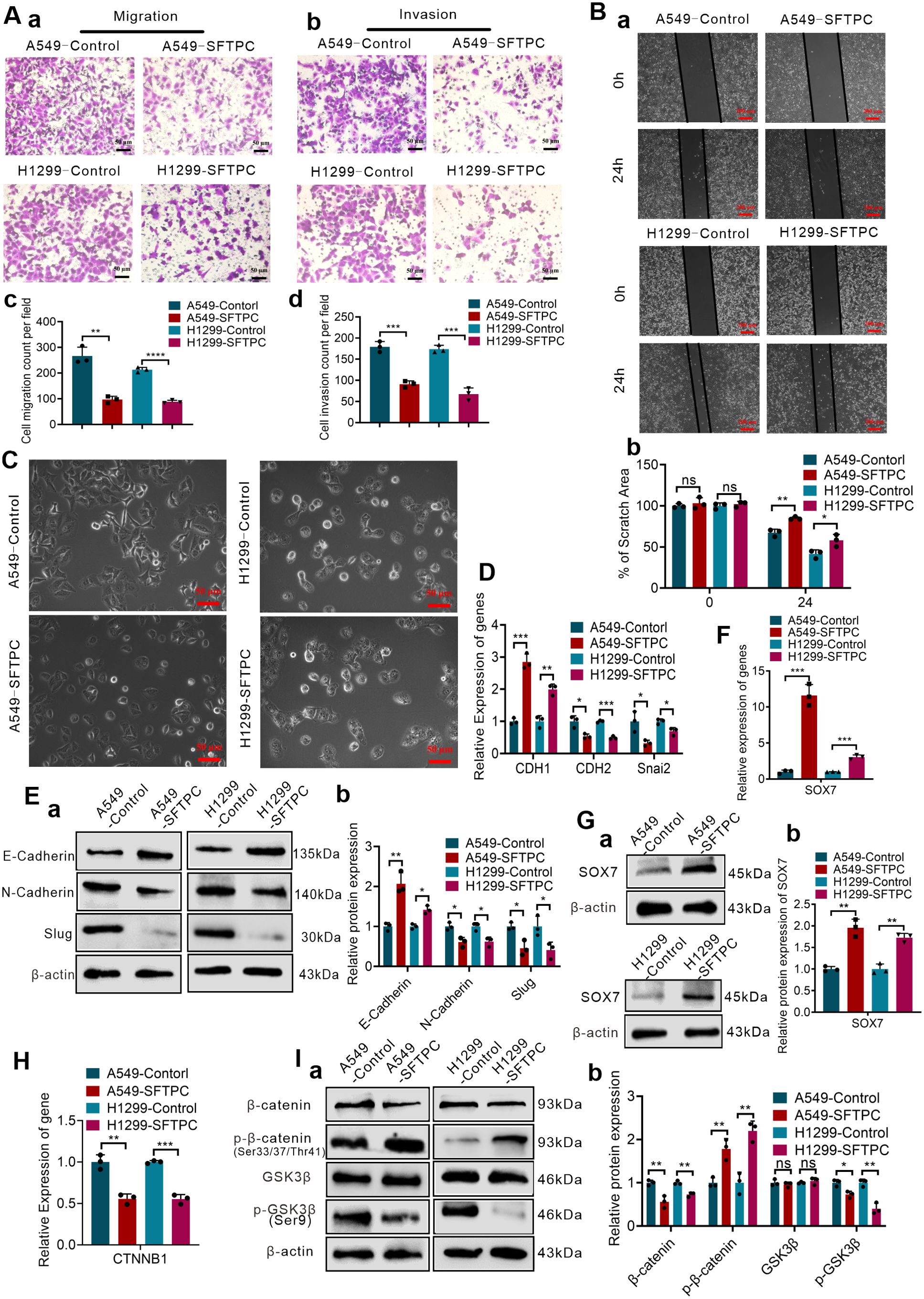
Figure 2. Overexpression of SFTPC repressed migration, invasion, EMT process, WNT/β-catenin pathway and upregulated SOX7 in NSCLC cells. (A) Millicell and Transwell assays were used to detect the vertical migration and invasion abilities of indicated cells (n=3) (n represented the number of fields for counting and statistics, experiments were conducted a minimum of three times independently). The images of vertical migrated cells or invaded cells were showed in (a, b). And the histograms were applied to quantify the vertical migration or invasion abilities of indicated cells (c, d). Scale bars, 50μm. (B) Wound-healing assay was used to detect lateral migration ability of indicated cells (n=3) (n represented the number of pictures for statistics, experiments were conducted a minimum of three times independently). The scratch areas of indicated cells were showed in (a), Scale bars, 200μm. And the histogram was applied to quantify the lateral migration ability of cells (b). (C) The morphology changes of indicated cells were showed in morphological images. Scale bar, 50μm. (D) qRT-RCR assay was used to detect the mRNA levels of EMT markers (CDH1(E-cadherin), CDH2(N-cadherin), Snai2(Slug)) in indicated cells, the histogram was applied to quantify the relative mRNA levels. (E) Western blot assay was used to detect the protein levels of EMT markers in indicated cells by using primary antibodies against E-cadherin, N-cadherin and Slug (a), and the histogram was applied to quantify the relative protein levels (b). (F) qRT-RCR assay was used to detect the mRNA levels of SOX7 in indicated cells, the histogram was applied to quantify the relative mRNA levels. (G) Western blot assay was used to detect the protein levels of SOX7 by using primary antibody against SOX7 in indicated cells (a), and the histogram was applied to quantify the relative protein levels (b). (H) qRT-RCR assay was used to detect the mRNA levels of CTNNB1(β-catenin) in indicated cells, the histogram was applied to quantify the relative mRNA levels. (I) Western blot assay was used to detect the protein levels of WNT/β-catenin pathway markers by using primary antibodies against GSK3β, p-GSK3β(Ser9), β-catenin, p-β-catenin(Ser33/Ser37/Thr41) in indicated cells (a), and the histogram was applied to quantify the relative protein levels (b). β-actin was used as the internal control gene. All data were presented as the Mean ± SD. Two groups were compared by un-paired t-test. *p<0.05, **p<0.01, “***”p<0.005 and “****”p<0.001. ns, no significance. All of these experiments were conducted a minimum of three times independently.
3.3 Overexpression of SFTPC upregulated the expression of SOX7 and repressed WNT/β-catenin pathway in vitro
To gain insights into the mechanisms of stable SFTPC-overexpression inhibiting EMT process of NSCLC cells, RNA-seq was firstly performed in A549-SFTPC group and A549-Control group. A total of 953 differential expression genes (DEGs) were identified, and 331 genes were notably upregulated while 622 genes were dramatically downregulated in A549-SFTPC group compared with A549-Control group. (Supplementary Figure S2A). The distinct and consistent difference of the gene expression profile caused by stable SFTPC-overexpression had been shown in the hierarchical clustering heatmap (Supplementary Figure S2B). The GO analysis of biological process, cell components and molecular function all suggested that DEGs were associated with the characteristics of EMT process, such as cell adhesion, locomotion of cells, cell migration, extracellular matrix, and so on (Supplementary Figure S3A). The KEGG analysis and Reactome analysis also indicated that the DEGs were related to EMT process (Supplementary Figures S3B, C). The above results suggested that overexpression of SFTPC might inhibit EMT process of NSCLC cells.
To uncover the underlying genes of SFTPC, the DEGs got from RNA-seq and the two datasets “the differentially expressed genes in LUAD” and “the genes associated with SFTPC in LUAD” got from TCGA database were utilized to obtain the potential target genes of SFTPC (Supplementary Figure S3D-a). And in the 28 overlapping DEGs from Venn diagram, SOX7 was found to be upregulated in A549-SFTPC group (Supplementary Figure S3D-b). Previous researches reported that SOX7 was low expression in NSCLC tissues, which was related to lymph node metastasis and low OS rate of LUAD patients (30, 31). Meanwhile, it was reported that overexpression of SOX7 inhibited the migration and invasion abilities of NSCLC cells (32, 33). Importantly, our qRT-PCR and Western blot analysis proved the mRNA and protein levels of SOX7 were both remarkably upregulated in A549-SFTPC and H1299-SFTPC groups compared to their control groups (Figures 2F, G). Thus, we demonstrated overexpression of SFTPC significantly upregulated the expression of SOX7.
And it’s worth noting that the GSEA-GO, GSEA-KEGG and GSEA-Reactome analysis suggested that overexpression of SFTPC was negatively correlated with WNT/β-catenin pathway (Supplementary Figures S4A–C). β-catenin is the core of WNT/β-catenin pathway. Here, we found that overexpression of SFTPC inhibited the mRNA level of CTNNB1(β-catenin) by qRT-PCR (Figure 2H). Meanwhile, compared with A549-Control and H1299-Control groups, the protein level of GSK3β was not changed, but the levels of p-GSK3β(Ser9) and β-catenin were markedly downregulated while the level of p-β-catenin (Ser33/Ser37/Thr41) was observably upregulated in A549-SFTPC and H1299-SFTPC cells by Western blot assay (Figure 2I). Degradation of β-catenin by phosphorylation at Thr41, Ser37 and Ser33 residues via GSK3β could lead to inactivation of the WNT/β-catenin pathway, whereas inactivation of GSK3β by phosphorylation at Ser9 residue could lead to activation of the WNT/β-catenin pathway (34). Therefore, our results indicated overexpression of SFTPC inactivated WNT/β-catenin pathway observably.
Taken together, we proved that overexpression of SFTPC upregulated the expression of SOX7 and repressed WNT/β-catenin pathway. Interestingly, the previous studies displayed overexpression of SOX7 suppressed WNT/β-catenin pathway, which could be mediated by interaction between SOX7 protein and β-catenin (35, 36). Meanwhile, overactivation of WNT/β-catenin was the vital activator of EMT process (13). Thus, we further hypothesized that overexpression of SFTPC might inhibit EMT process of NSCLC cells by upregulating SOX7 and then repressing WNT/β-catenin pathway.
3.4 Overexpression of SFTPC inhibited migration, invasion abilities and EMT process of NSCLC cells via upregulating SOX7 in vitro
To explore whether overexpression of SFTPC inhibited migration, invasion abilities and EMT process via upregulating SOX7 in NSCLC cells, A549-SFTPC and H1299-SFTPC cells were treatment with si-SOX7-2, which was the most effective sequence of si-SOX7 (Supplementary Figures S5A–C). Then, the migratory and invasive capacities of A549-SFTPC-si-SOX7-2 and H1299-SFTPC-si-SOX7-2 cells were partially restored compared with A549-SFTPC-si-NC and H1299-SFTPC-si-NC cells (Figures 3A, B). Meanwhile, a typical spindle-like shape change was observed in A549-SFTPC-si-SOX7-2 cells compared to A549-SFTPC-si-NC cells, but the morphology of H1299-SFTPC-si-SOX7-2 cells was not changed compared to H1299-SFTPC-si-NC cells (Figure 3C). And CDH1(E-cadherin) was notably decreased while CDH2(N-cadherin) and Snai2(Slug) were markedly increased in A549-SFTPC-si-SOX7-2 and H1299-SFTPC-si-SOX7-2 cells compared with A549-SFTPC-si-NC and H1299-SFTPC-si-NC cells, respectively (Figures 3D, E). Collectively, we demonstrated that overexpression of SFTPC significantly repressed migration, invasion abilities and EMT process of NSCLC cells via upregulating SOX7.
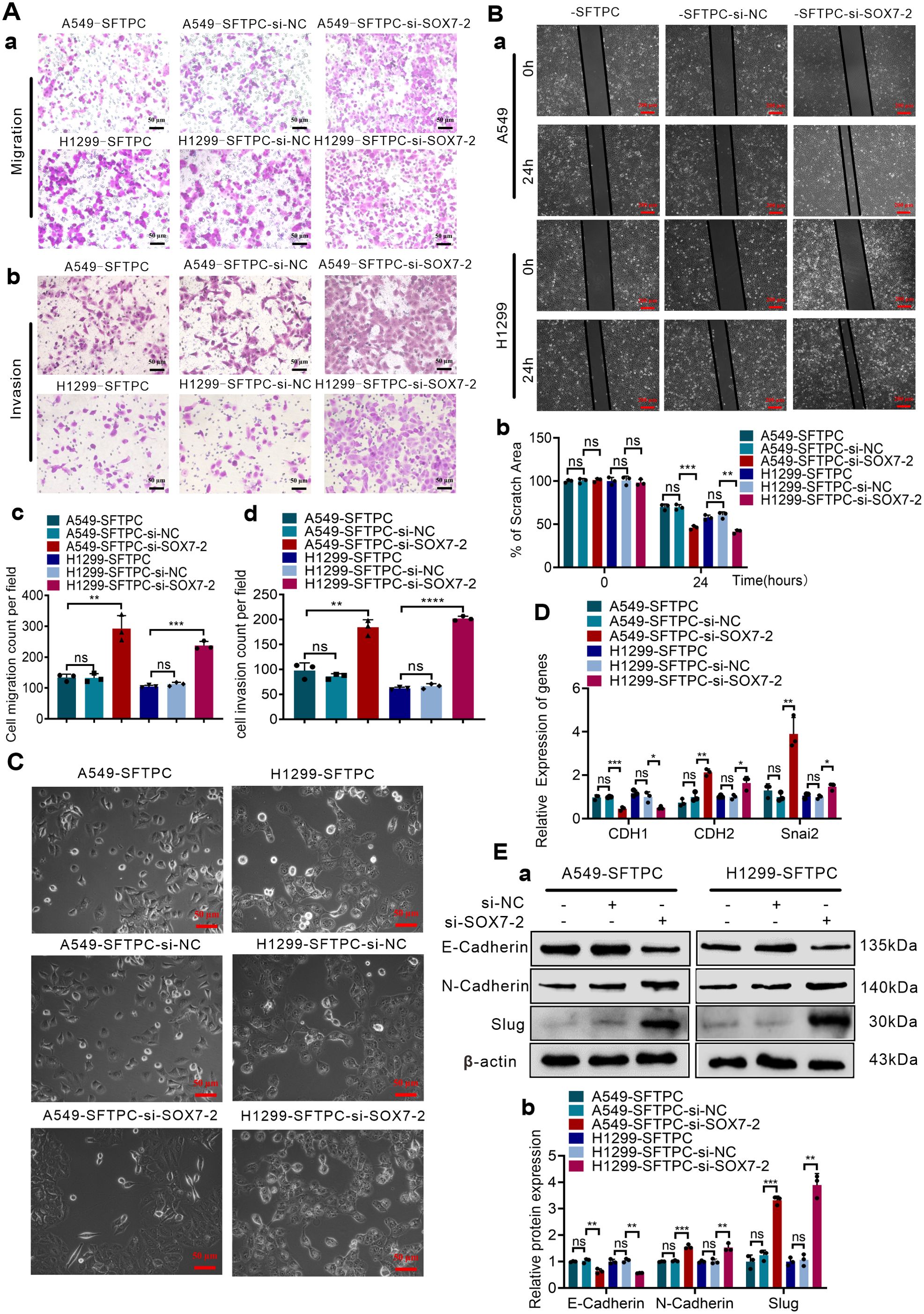
Figure 3. Overexpression of SFTPC inhibited migration, invasion abilities and EMT process of NSCLC cells via upregulation of SOX7. (A) Millcell and Transwell assay were used to detect the vertical migration and invasion abilities of the indicated cells (n=3) (n represented the number of fields for counting and statistics, experiments were conducted a minimum of three times independently). The images of vertical migrated and invaded cells were showed in (a, b). Scale bar, 50μm. The histograms were applied to quantify the vertical migration and invasion abilities of indicated cells (c, d). (B) Wound-healing assay was used to detect the lateral migration ability of the indicated cells (n=3) (n represented the number of pictures for statistics, experiments were conducted a minimum of three times independently). The scratch areas of indicated cells were showed in (a). Scale bar, 200μm. The histogram was applied to quantify the lateral migration ability of indicated cells (b). (C) The morphology changes of indicated cells were presented in morphological images. Scale bar, 50μm. (D) qRT-RCR assay was used to detect the mRNA levels of EMT markers [CDH1(E-cadherin), CDH2(N-cadherin), Snai2(Slug)]. The histogram was applied to quantify the relative mRNA levels. (E) Western blot assay was used to detect the protein levels of EMT markers by using primary antibodies against E-cadherin, N-cadherin and Slug (a), and the histogram was applied to quantify the relative protein levels (b). β-actin was used as the internal control gene. All the data were presented as the Mean ± SD, un-paired t-test. *p<0.05, **p<0.01, ***p<0.005, ****p<0.001. ns, no significance. All of these experiments were conducted a minimum of three times independently.
3.5 Overexpression of SFTPC inhibited EMT process of NSCLC cells through upregulation of SOX7 and then inactivation of WNT/β-catenin pathway in vitro
Furthermore, to verify whether overexpression of SFTPC inhibited EMT process of NSCLC cells through upregulation of SOX7 and then inactivation of WNT/β-catenin pathway, we firstly detected whether overexpression of SFTPC repressed WNT/β-catenin pathway via upregulating SOX7. Our results indicated the knockdown of SOX7 in A549-SFTPC and H1299-SFTPC observably increased mRNA level of CTNNB1(β-catenin) by qRT-PCR (Figure 4A). Meanwhile, GSK3β was not changed, but p-GSK3β(Ser9) and β-catenin were notably increased while p-β-catenin (Ser33/Ser37/Thr41) was observably decreased in A549-SFTPC-si-SOX7-2 and H1299-SFTPC-si-SOX7-2 compared with A549-SFTPC-si-NC and H1299-SFTPC-si-NC cells by Western blot assay (Figure 4B). Taken together, overexpression of SFTPC could upregulate SOX7, then inhibit the expression of CTNNB1(β-catenin), reduce p-GSK3β(Ser9) and increase p-β-catenin (Ser33/Ser37/Thr41), which could lead to the decrease of β-catenin. Moreover, to clarify whether SOX7 was the upstream factor of β-catenin, the inhibitor of WNT/β-catenin pathway was used in our experiment. IWR-1 had been proved to be a potent inhibitor of WNT/β-catenin pathway in a variety of cancer cells (37, 38).We found that the expression of SOX7 was not changed in A549-Control and H1299-Control cells after treatment with IWR-1 by qRT-PCR and Western blot assays (Supplementary Figures S6A, B). These outcomes indicated that overexpression of SFTPC inactivated WNT/β-catenin pathway though upregulating SOX7.
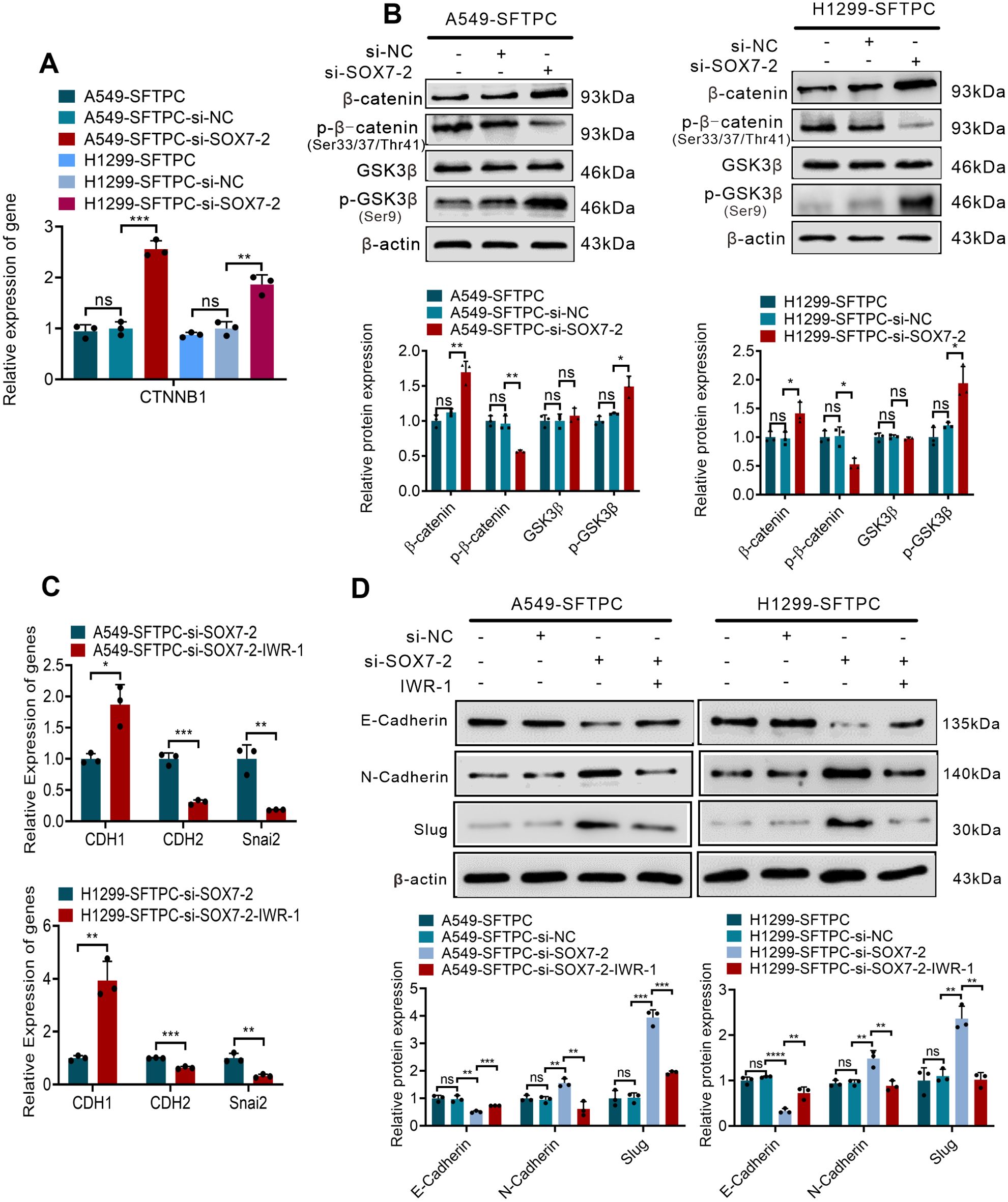
Figure 4. Overexpression of SFTPC inhibited EMT process through upregulation of SOX7 and then inactivation of WNT/β-catenin pathway in vitro. (A) qRT-PCR assay was used to detect the mRNA level of CTNNB1(β-catenin). The histogram was applied to quantify the relative mRNA level. (B) Western blot assay was used to detect the protein levels of WNT/β-catenin pathway markers by using primary antibodies against GSK3β, p-GSK3β(Ser9), β-catenin, p-β-catenin (Ser33/Ser37/Thr41) in indicated cells, and the histograms were applied to quantify the relative protein levels. (C) qRT-PCR assay was conducted on the indicated cells treated with IWR-1 (0.5μM) or without IWR-1 (n=3), the relative mRNA levels of EMT markers (CDH1(E-cadherin), CDH2(N-cadherin), Snai2(Slug) were detected. The histograms were applied to quantify the relative mRNA levels. (D) Western blot assay was conducted on the indicated cells treated with IWR-1 (0.5μM) or without IWR-1 by using primary antibodies against E-cadherin, N-cadherin and Slug (n=3), and the histograms were applied to quantify the relative protein levels. β-actin was used as the internal control gene. All the data were presented as the Mean ± SD, un-paired t-test. *p<0.05, **p<0.01, ***p<0.005, ****p<0.001. ns, no significance. All experiments were conducted a minimum of three times independently.
Then, based on the above results, IWR-1 was utilized in the cells where SOX7 had been knocked down. The A549-SFTPC-si-SOX7-2 and H1299-SFTPC-si-SOX7-2 cells were treated by IWR-1 (named A549-SFTPC-si-SOX7-2-IWR-1 and H1299-SFTPC-si-SOX7-2-IWR-1). And CDH1(E-cadherin) was prominently increased, while CDH2(N-cadherin) and Snai2(Slug) were notably decreased in A549-SFTPC-si-SOX7-2-IWR-1 and H1299-SFTPC-si-SOX7-2-IWR-1 cells compared with A549-SFTPC-si-SOX7-2 and H1299-SFTPC-si-SOX7-2 cells by qRT-PCR and Western blot assays (Figures 4C, D). Taken together, we illustrated that overexpression of SFTPC obviously inhibited EMT process of NSCLC cells by upregulating SOX7 and then repressing WNT/β-catenin pathway.
3.6 Overexpression of SFTPC inhibiting EMT process of NSCLC cells was associated with upregulation of SOX7 and inactivation of WNT/β-catenin pathway in vivo
To further confirm whether overexpression of SFTPC inhibiting EMT process was related to upregulation of SOX7 and inactivation of WNT/β-catenin pathway in vivo, the stable SFTPC-overexpression A549 cells tumor xenograft model and its control model were established via subcutaneous injection of A549-SFTPC and A549-Control cells according to our previous study (25). Experimentally, five days after cells were injected subcutaneously, the tumor formed, then, all tumors size was measured every three days. Compared to A549-Control tumors, the tumors volume was observably inhibited and the cell proliferation marker Ki-67 was notably decreased in A549-SFTPC tumors (Figure 5A). Importantly, compared to control tumors, E-cadherin was notably increased while N-cadherin and Slug were dramatically decreased in A549-SFTPC tumors by Western blot assay (Figure 5B). Moreover, the level of GSK3β was not changed, and the levels of proSP-C, SOX7 and p-β-catenin (Ser33/Ser37/Thr41) were markedly increased, while the levels of p-GSK3β(Ser9) and β-catenin were visibly decreased in A549-SFTPC tumors compared with A549-Control tumors by Western blot analysis (Figure 5C). All the outcomes in vivo were consistent with their results in vitro. It illustrated that overexpression of SFTPC inhibiting EMT process of NSCLC cells was relevant to upregulating SOX7 and repressing WNT/β-catenin pathway in tumor xenograft models.
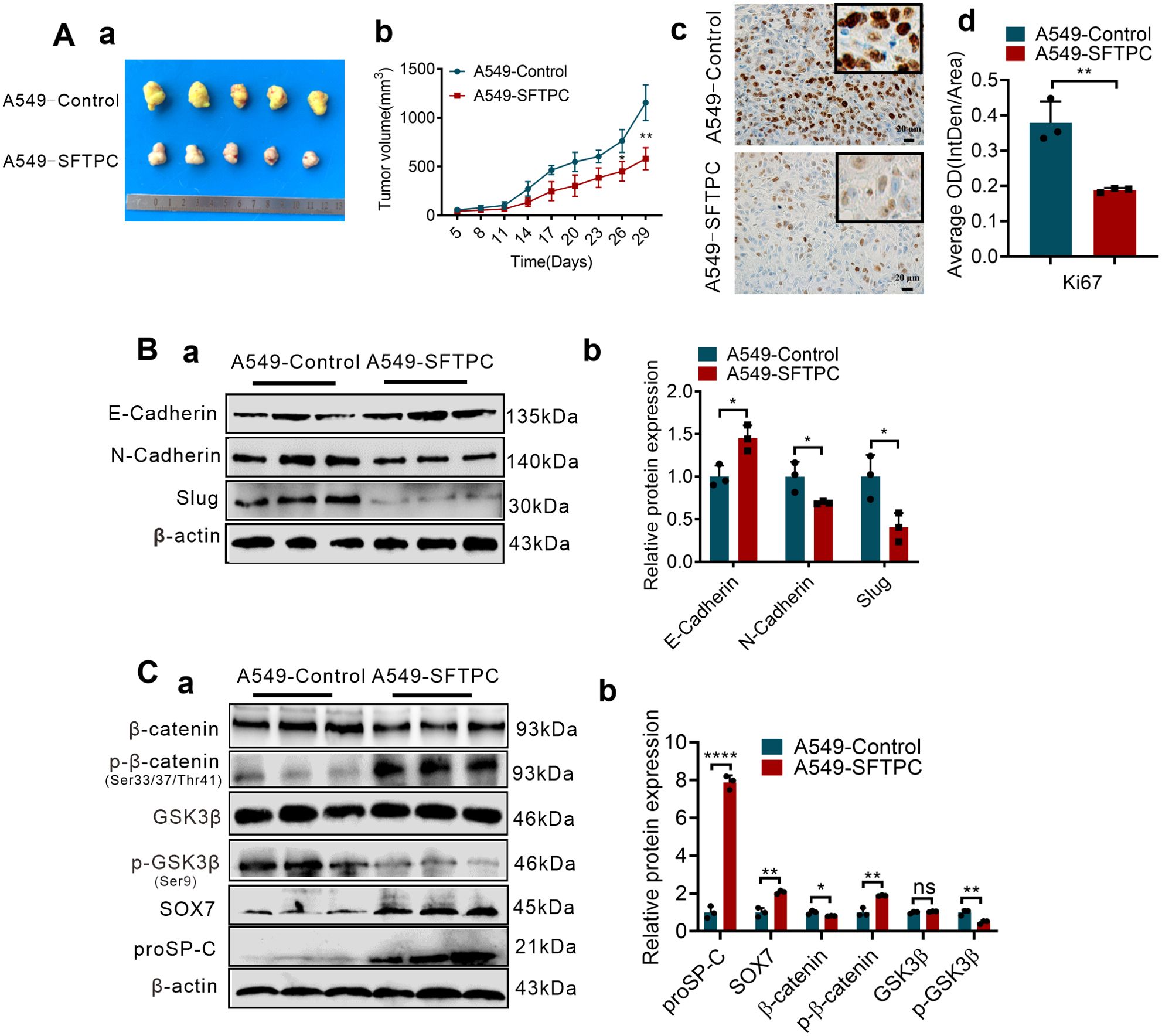
Figure 5. Overexpression of SFTPC inhibiting EMT process of NSCLC cells was associated with upregulation of SOX7 and inactivation of WNT/β-catenin pathway in vivo. (A) Establishment of stable SFTPC-overexpression A549 and control tumor xenograft models. BALB/c nude mice (4-week-old male, n=5/group) were subcutaneously injected with A549-SFTPC cells or A549-Control cells, respectively. The mice were sacrificed after 29 days, the retrieved tumor samples were showed in (a). The statistical graph of changes in tumor volume were showed in (b). IHC staining was conducted on paraffin sections of retrieved tumor samples by using primary antibody against the proliferation marker Ki-67 (n=3) (c), staining without primary antibody was used as negative control. Image J 180 was used to measure the average optical density. The histogram was applied to quantify the results of IHC staining (d). Scale bars, 20μm. (B) Western blot assay was conducted on the protein of retrieved tumor samples (n=3) by using the primary antibodies against E-cadherin, N-cadherin and Slug (a), and the histogram was applied to quantify the relative protein levels (b). (C) Western blot assay was conducted on the protein of the retrieved tumor samples (n=3) by using the primary antibodies against SOX7, proSP-C, GSK3β, p-GSK3β(Ser9), β-catenin and p-β-catenin (Ser33/Ser37/Thr41) (a), the histogram was applied to quantify the relative protein levels (b). β-actin was used as the internal control gene. All data were presented as the Mean ± SD, un-paired t-test. *p<0.05, **p<0.01, ****p<0.001. All experiments were conducted a minimum of three times independently.
3.7 Low expression of SFTPC was correlated with EMT process and low expression of SOX7 in collected clinical LUAD tissues
To further clarity the correlation of SFTPC and EMT phenotype in clinical NSCLC tissues, the protein levels of EMT markers in 40 cases of clinical LUAD tissues with low expression of SFTPC were detected by IHC staining. According to our previous study (25), the EMT phenotype of tumors was distinguished though the protein levels of EMT markers. Therefore, the EMT phenotype were classified based on the defined criteria in present study (Figure 6A). In 40 cases of clinical LUAD tissues, the ratio of mesenchymal (n=21, 52.5%) and EMT (n=7, 17.5%) phenotypes were significantly higher than the ratio of epithelial (n=2, 5.0%) phenotype (Figure 6B, Supplementary Table S5). It demonstrated that low expression of SFTPC was correlation with EMT process of clinical NSCLC samples.
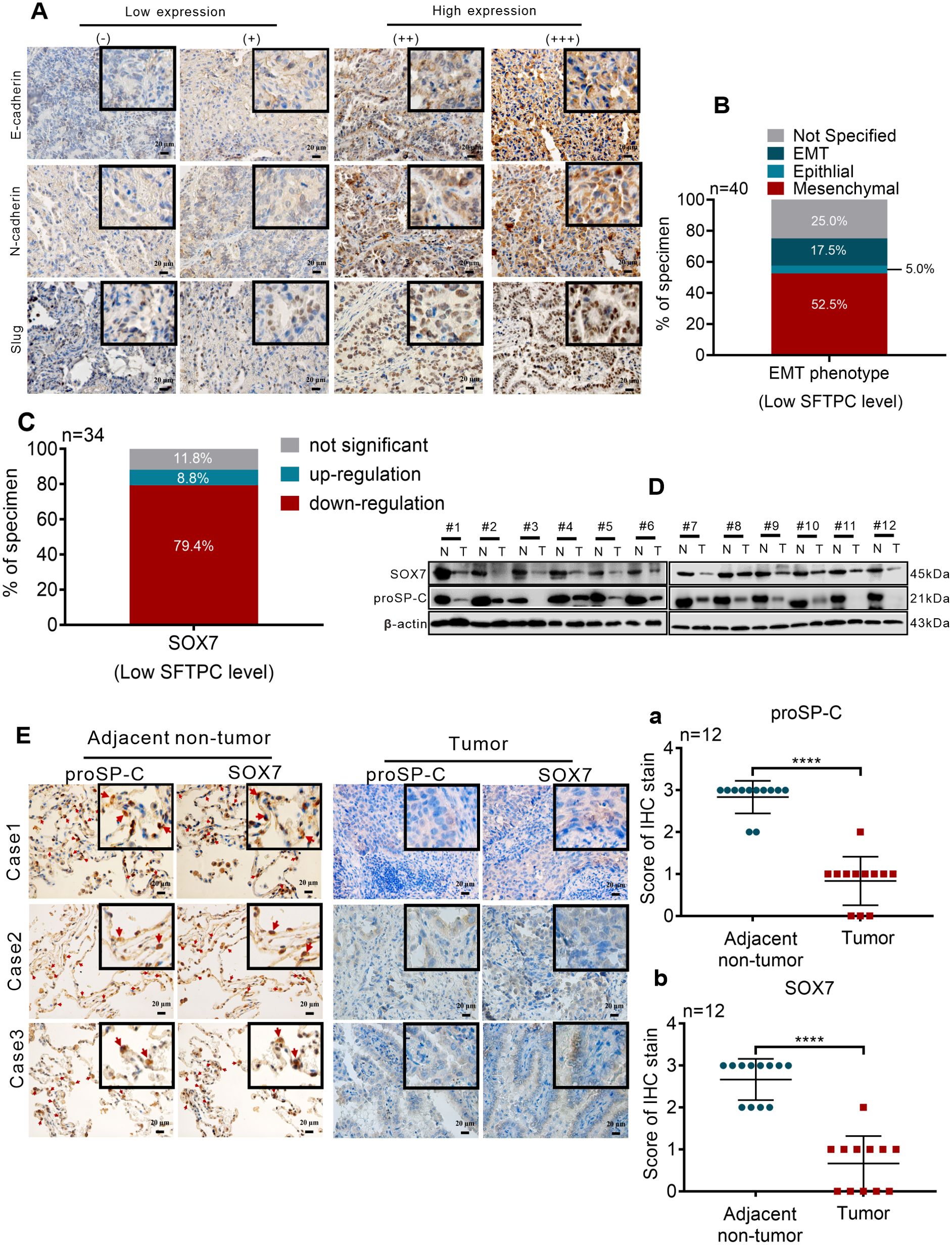
Figure 6. Low expression of SFTPC was correlated with the EMT process and low expression of SOX7 in clinical LUAD tissues (A) IHC staining was conducted on paraffin sections of collected clinical LUAD tumor tissues with low mRNA level of SFTPC (n=40) by using primary antibodies against E-Cadherin, N-Cadherin and Slug. Staining without primary antibody was used as negative control. The IHC staining yielded grades of E-Cadherin, N-Cadherin and Slug as follows, (-) and (+) represented low expression of EMT markers. (++) and (+++) represented high expression of EMT markers. Scale bar, 20μm. (B) The relationship between low expression of SFTPC and EMT phenotype status in LUAD tissues. (C) The mRNA levels of SOX7 in the collected clinical LUAD tumor tissues with low mRNA levels of SFTPC (n=34) were detected by qRT-PCR assay. (D) Western blot assay was conducted on the protein of paired clinical LUAD tumor tissues with both low mRNA levels of SFTPC and SOX7 (n=12) by using the primary antibodies against SOX7 and proSP-C. (E) IHC staining was conducted on the paraffin sections of the collected paired clinical LUAD tumor tissues with both low mRNA levels of SFTPC and SOX7 (n=12) by using primary antibodies against proSP-C and SOX7. Staining without primary antibody was used as negative control. And the site of SOX7 in alveolar (indicated by red arrow, adjacent non-tumor groups, right) was detected by IHC staining (n=12). The histograms were applied to quantify the experimental results of IHC staining (a, b). n, numbers of clinical tissues. Scale bar, 20μm. All data were presented as the Mean ± SD, un-paired t-test. ****p<0.001.
Then, to further investigate the relationship between SFTPC and SOX7 in NSCLC samples, the expression changes of SOX7 in 34 cases of clinical LUAD tissues with low expression of SFTPC were detected by qRT-PCR assay. Compared to paired adjacent non-tumor tissues, the mRNA levels of SOX7 were notably decreased in 27 out of 34 LUAD tissues (Figure 6C, Supplementary Table S6). Then, 12 pairs of LUAD were further selected from the 27 pairs of LUAD with both low mRNA levels of SFTPC and SOX7 randomly. The protein levels of proSP-C and SOX7 were both downregulated in the 12 pairs of LUAD by IHC staining and Western blot assays (Figures 6D, E). The score criteria and outcomes for proSP-C and SOX7 of IHC staining were showed in Supplementary Table S2. Moreover, we discovered that SOX7 and proSP-C protein were colocalized in AT2 cells in adjacent non-tumor tissues by IHC staining (Figure 6E). The outcomes above indicated that there was a positive relationship between SFTPC and SOX7 in LUAD tissues. In total, we proved that low expression of SFTPC was correlated with the EMT process and low expression of SOX7 in clinical LUAD tissues.
4 Discussion
The functions and mechanisms of AT2 cells marker gene SFTPC in NSCLC development are still poor understood until now. In our present study, we demonstrated that overexpression of SFTPC repressed EMT process of NSCLC cells via upregulating SOX7 and then inactivating WNT/β-catenin pathway for the first time.
Firstly, we discovered that the expression of SFTPC was extremely decreased in 515 LUAD cases compared with 59 adjacent non-tumors in TCGA database. Subsequently, we further clarified that the expression of SFTPC was observably decreased in 40 out of 46 collected LUAD samples compared with matched adjacent non-tumors. Analogously, some researchers also discovered that the expression of SFTPC was notably downregulated in NSCLC samples compared with the adjacent non-tumors (24, 27, 28). Consistent with the results of others, our current research further proved that the expression of SFTPC was remarkably decreased in NSCLCs compared with the adjacent non-tumors.
Subsequently, we further found that low expression of SFTPC in NSCLC tissues was related to lymph node metastasis of LUAD, and was also related to low OS rate of LUAD patients in TCGA database. Unfortunately, in the 45 cases of collected LUAD samples, the expression level of SFTPC had no significant difference between the LUAD samples with lymph node metastasis and without lymph node metastasis. It might be limited by the number of LUAD samples, and it will be further analyzed in more LUAD samples in the future. It is worth mentioning that Li Wang et al. (39) uncovered that the expression of SFTPC was progressively downregulated during the malignant progression of LUAD, and it was completely absent in LUAD tissues with brain metastases. Similarly, the survival analysis conducted by multiple researchers across multiple databases suggested that low expression of SFTPC was observably correlated with the decreased OS rate and progression-free survival (FP) rate of NSCLC patients (24, 28, 40, 41). Taken together, our results and others all indicated that low expression of SFTPC was associated with poor prognosis of NSCLC.
Next, we found that overexpression of SFTPC significantly repressed the migration and invasion abilities of NSCLC cells. Importantly, we further proved that overexpression of SFTPC dramatically repressed EMT process of NSCLC cells. Previously, Bin Li et al. (29) and Baile Zuo et al. (24) only found that overexpression of SFTPC inhibited the proliferation ability of NSCLC cells. In comparison, our study further enriched the functions of SFTPC in the malignant progression of NSCLC. Especially, we found low expression of SFTPC was correlation with EMT process in clinical NSCLC tissues. Of note, EMT process of NSCLC cells promoted metastasis of tumors (13, 23), and low expression of E-cadherin was markedly relevant to poor prognosis of LUAD (23). Therefore, our results also implied that SFTPC might be a potential prognostic marker gene for NSCLC. Then, we discovered that overexpression of SFTPC could prominently upregulate the expression of SOX7 in NSCLC cells through RNA-seq, qRT-PCR and Western blot assays. SOX7 protein belongs to SOX family, which are transcription factors (42). It was demonstrated that SOX7 was a tumor suppressor in lung cancer (31, 33). Previous studies discovered that the downregulation of SOX7 in lung tumor tissues could be caused by hypermethylation in its promoter (43). What’s more, the expression of SOX7 could also be downregulated or upregulated by miRNA, lncRNA or GATA4 (36). Here, our study found that overexpression of SFTPC upregulated the expression of SOX7. Our results further enriched the upstream regulatory network of SOX7. Of course, we didn’t clarify the underlying molecular mechanism about overexpression of SFTPC upregulating SOX7, and it would be an important part in our subsequent study.
Strikingly, several studies illustrated that low expression of SOX7 in LUAD tissues was related to lymph node metastasis and low OS rate of LUAD patients (30, 31). And Lichun Han et al. (33) proved overexpression of SOX7 inhibited the malignant progression of NSCLC. Here, we found that knockdown of SOX7 enhanced the migratory and invasive abilities of A549-SFTPC and H1299-SFTPC cells. Meanwhile, a typical spindle-like shape change had been observed in A549-SFTPC-si-SOX7-2 cells. And knockdown of SOX7 significantly downregulated E-cadherin while notably upregulated N-cadherin and Snai2 in A549-SFTPC and H1299-SFTPC cells. Collectively, our results above confirmed that overexpression of SFTPC repressed EMT process of NSCLC cells via upregulating SOX7, thereby suppressed their migration and invasion abilities.
Furthermore, our RNA-seq result suggested that overexpression of SFTPC was negatively associated with WNT/β-catenin pathway, then we demonstrated that overexpression of SFTPC prominently inactivated WNT/β-catenin pathway by qRT-PCR and Western blot assays. It is worth mentioning that Lizheng Guo et al. (35) uncovered that SOX7 protein interacted with β-catenin directly, which mediated the degradation of active β-catenin in the APC-independent mechanism. Here, we found knockdown of SOX7 in A549-SFTPC and H1299-SFTPC cells observably increased the mRNA level of CTNNB1(β-catenin), the protein levels of p-GSK3β(Ser9) and β-catenin, but decreased the level of p-β-catenin (Ser33/Ser37/Thr41) compared with control cells. Taken together, we demonstrated that overexpression of SFTPC inactivated WNT/β-catenin pathway via upregulation of SOX7. However, the mechanism about overexpression of SFTPC upregulating SOX7 inhibited the expression of CTNNB1(β-catenin) didn’t been further explored in our present study. It will be clarified thorough further research in the future. Interestingly, Yumeng Zhang et al. (44) found that overexpression of SOX7 could directly activate SLIT2 in human breast cancer MDA-MB-231 cells. Meanwhile, we found that SLIT2 was upregulated in A549-SFTPC group Supplementary Figure S3D-b). Importantly, Dinesh K. Ahirwar et al. (45) found that overexpression of SLIT2 inhibiting small cell lung cancer growth was associated with the inactivation of the GSK3β/β-catenin signaling pathway in tumor cells and tumor-associated macrophages. In our present study, we didn’t clarify the mechanism about overexpression of SFTPC upregulating SOX7 inhibited the protein level of p-GSK3β(Ser9) in NSCLC cells. Therefore, based on our and others’ research, we can hypothesize that overexpression of SFTPC upregulating SOX7 might directly activate SLIT2, and then inhibit the protein level of p-GSK3β(Ser9) in NSCLC cells. And this hypothetical will be an essential part in our subsequent research.
WNT/β-catenin pathway, which promoted the formation of airway and alveoli, played critical role in pulmonary development (34). The hyperactivation of this pathway was thought to be a vital factor of EMT process (13). We discovered that E-cadherin was notably increased while N-cadherin and Snai2 were markedly decreased in A549-SFTPC-si-SOX7-2 and H1299-SFTPC-si-SOX7-2 cells after treatment with IWR-1, the potent inhibitor of WNT/β-catenin pathway. The outcomes above confirmed that overexpression of SFTPC repressed EMT process of NSCLC cells via upregulation of SOX7 and then inactivation of WNT/β-catenin pathway.
Particularly, overactivation of WNT/β-catenin pathway was found in AT2 cells when alveoli were severely injured (5), and overactivation of this pathway was tightly linked with malignant progression of lung cancer (34). Importantly, abnormal AT2 cells were one of the origin cells of NSCLC (17). However, it was difficult to explore the molecular mechanisms about how NSCLC cells could derive from malignant transformation of the AT2 cells directly, since AT2 cells would rapidly differentiate into AT1 cells in 2D culture in vitro (46). Here, we found overexpression of SFTPC repressed EMT process of NSCLC cells via upregulating SOX7 and then inactivating WNT/β-catenin pathway. It might provide a new idea for further investigating the mechanisms about the malignant transformation of AT2 cells into NSCLC cells in the future. Importantly, Hsin-Jung Li et al. (47) discovered that the inactivation of WNT/β-catenin pathway inhibited EMT process and metastasis of NSCLC recently. Moreover, some inhibitors of WNT/β-catenin pathway were investigated in a series of NSCLC clinical trials (48). Therefore, our results suggested that SFTPC might also be a novel target for clinical NSCLC treatment.
In addition, several studies found that mutations or deletions in SFTPC caused IPF (8, 12, 49). Strikingly, Liudi Yao et al. (50) discovered that abnormal gene in AT2 cells induced EMT progress of AT2 cells, then promoted IPF development. The epidemiological researches reported IPF was also an independent risk factor for lung cancer (19). Importantly, major lung tumors (70%~82%) of the patients with IPF were located near fibrotic lesions by pathological studies (51, 52). However, the mechanisms about lung tumor development in patients with IPF are still poorly understood. Interestingly, Takayuki Honda et al. (53) found SFTPC mutation in patients with both IPF and NSCLC (IPF-NSCLC) by Whole-exome analysis, and revealed that SFTPC mutation could notably decrease the expression of SFTPC. Here, we proved the expression of SFTPC was extremely decreased in clinical NSCLC samples, and we clarified that overexpression of SFTPC repressed EMT process of NSCLC cells via upregulating SOX7 and then inactivating WNT/β-catenin pathway. Collectively, our study suggested that the abnormal expression of SFTPC might induce EMT process of AT2 cells, then promote the development of IPF and NSCLC.
In conclusion, our results revealed a new molecular mechanism of the AT2 cells marker gene SFTPC in development of NSCLC. Moreover, our research might also provide a novel clue for exploring the molecular mechanism about NSCLC development in patients with IPF in the future (Figure 7).
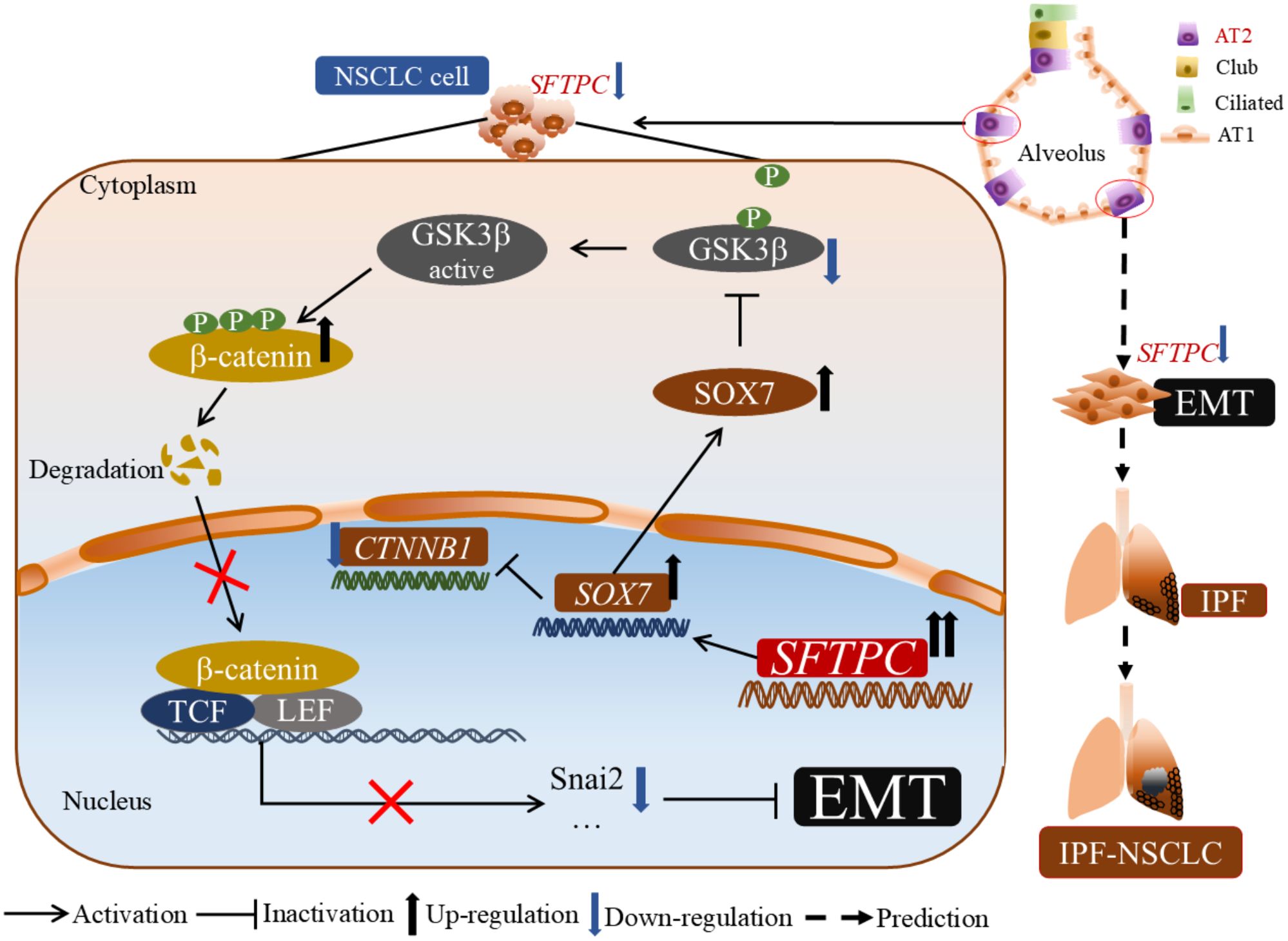
Figure 7. The molecular model of overexpression of SFTPC inhibiting the EMT process of NSCLC cells. Mechanistically, overexpression of SFTPC upregulated the expression of SOX7, then inhibited the mRNA level of CTNNB1(β-catenin) and decreased the protein level of p-GSK3β(Ser9), whereas increased the protein level of p-β-catenin (Ser33/Ser37/Thr41), and further decreased the protein level of β-catenin, and inhibited the EMT process of NSCLC cells eventually. In addition, we further speculated that the abnormal expression of AT2 cells marker gene SFTPC might induce EMT process in AT2 cells, then promote the development of IPF-NSCLC. (This figure was drawn by power point with Science Slides suite).
Data availability statement
The RNA-seq datasets presented in this study can be found in online repositories. The names of the repository/repositories and accession number(s) can be found below: http://www.ncbi.nlm.nih.gov/bioproject/1157334, BioProject ID: PRJNA1157334. Further inquiries can be directed to the corresponding authors.
Ethics statement
The approvals for patient samples study were obtained from the West China Hospital of Sichuan University Biomedical Research Ethics Committee and the Medical Ethics Committee, Sichuan Academy of Medical Sciences, Sichuan Provincial People's Hospital (Chengdu, China). This study was performed in accordance with the Declaration of Helsinki. Written informed consent was obtained from all the patients. The animal study was approved by the West China Hospital of Sichuan University Animal Ethics Committee (Chengdu, China). These studies were conducted in accordance with the local legislation and institutional requirements.
Author contributions
QZ: Conceptualization, Writing – review & editing, Data curation, Formal analysis, Investigation, Methodology, Validation, Writing – original draft. NA: Formal analysis, Methodology, Writing – review & editing, Funding acquisition, Resources, Supervision. YL: Formal analysis, Methodology, Investigation, Writing – review & editing. YZ: Formal analysis, Investigation, Methodology, Writing – review & editing. WP: Formal analysis, Investigation, Methodology, Writing – review & editing. PG: Formal analysis, Investigation, Methodology, Writing – review & editing. JZ: Formal analysis, Investigation, Methodology, Writing – review & editing. QP: Resources, Supervision, Writing – review & editing. WZ: Resources, Supervision, Conceptualization, Funding acquisition, Project administration, Writing – review & editing.
Funding
The author(s) declare financial support was received for the research, authorship, and/or publication of this article. This research was partly supported by Key Research and Development Program of Sichuan Province (No. 2022YFS0004) and by Applied Basic Research Program of Sichuan Province (No. 2022NSFSC0742).
Acknowledgments
We would like to thank Li Zhou, Di Wang, Yan Liang, Lingqiao Tang (Core Facilities of West China Hospital, Sichuan University) for helping with the IHC analysis.
Conflict of interest
The authors declare that the research was conducted in the absence of any commercial or financial relationships that could be construed as a potential conflict of interest.
Publisher’s note
All claims expressed in this article are solely those of the authors and do not necessarily represent those of their affiliated organizations, or those of the publisher, the editors and the reviewers. Any product that may be evaluated in this article, or claim that may be made by its manufacturer, is not guaranteed or endorsed by the publisher.
Supplementary material
The Supplementary Material for this article can be found online at: https://www.frontiersin.org/articles/10.3389/fonc.2024.1448379/full#supplementary-material
References
1. Bray F, Laversanne M, Sung H, Ferlay J, Siegel RL, Soerjomataram I, et al. Global cancer statistics 2022: GLOBOCAN estimates of incidence and mortality worldwide for 36 cancers in 185 countries. CA Cancer J Clin. (2024) 74(3):229–63. doi: 10.3322/caac.21834
2. Herbst RS, Morgensztern D, Boshoff C. The biology and management of non-small cell lung cancer. Nature. (2018) 553:446–54. doi: 10.1038/nature25183
3. Zhang H, Jiang H, Zhu L, Li J, Ma S. Cancer-associated fibroblasts in non-small cell lung cancer: Recent advances and future perspectives. Cancer Lett. (2021) 514:38–47. doi: 10.1016/j.canlet.2021.05.009
4. Guillot L, Nathan N, Tabary O, Thouvenin G, Le Rouzic P, Corvol H, et al. Alveolar epithelial cells: master regulators of lung homeostasis. Int J Biochem Cell Biol. (2013) 45:2568–73. doi: 10.1016/j.biocel.2013.08.009
5. Nabhan AN, Brownfield DG, Harbury PB, Krasnow MA, Desai TJ. Single-cell Wnt signaling niches maintain stemness of alveolar type 2 cells. Sci (New York NY). (2018) 359:1118–23. doi: 10.1126/science.aam6603
6. Beers MF, Mulugeta S. Surfactant protein C biosynthesis and its emerging role in conformational lung disease. Annu Rev Physiol. (2005) 67:663–96. doi: 10.1146/annurev.physiol.67.040403.101937
7. Treutlein B, Brownfield DG, Wu AR, Neff NF, Mantalas GL, Espinoza FH, et al. Reconstructing lineage hierarchies of the distal lung epithelium using single-cell RNA-seq. Nature. (2014) 509:371–5. doi: 10.1038/nature13173
8. Katzen J, Beers MF. Contributions of alveolar epithelial cell quality control to pulmonary fibrosis. J Clin Invest. (2020) 130:5088–99. doi: 10.1172/JCI139519
9. Selman M, Pardo A. The leading role of epithelial cells in the pathogenesis of idiopathic pulmonary fibrosis. Cell Signal. (2020) 66:109482. doi: 10.1016/j.cellsig.2019.109482
10. Kim KK, Kugler MC, Wolters PJ, Robillard L, Galvez MG, Brumwell AN, et al. Alveolar epithelial cell mesenchymal transition develops in vivo during pulmonary fibrosis and is regulated by the extracellular matrix. Proc Natl Acad Sci USA. (2006) 103:13180–5. doi: 10.1073/pnas.0605669103
11. Marmai C, Sutherland RE, Kim KK, Dolganov GM, Fang X, Kim SS, et al. Alveolar epithelial cells express mesenchymal proteins in patients with idiopathic pulmonary fibrosis. Am J Physiol Lung Cell Mol Physiol. (2011) 301:L71–8. doi: 10.1152/ajplung.00212.2010
12. Rodriguez L, Tomer Y, Carson P, Dimopoulos T, Zhao M, Chavez K, et al. Chronic expression of a clinical SFTPC mutation causes murine lung fibrosis with idiopathic pulmonary fibrosis features. Am J Respir Cell Mol Biol. (2023) 68:358–65. doi: 10.1165/rcmb.2022-0203MA
13. Dongre A, Weinberg RA. New insights into the mechanisms of epithelial-mesenchymal transition and implications for cancer. Nat Rev Mol Cell Biol. (2019) 20:69–84. doi: 10.1038/s41580-018-0080-4
14. Ferone G, Lee MC, Sage J, Berns A. Cells of origin of lung cancers: lessons from mouse studies. Genes Dev. (2020) 34:1017–32. doi: 10.1101/gad.338228.120
15. Juul NH, Yoon JK, Martinez MC, Rishi N, Kazadaeva YI, Morri M, et al. KRAS(G12D) drives lepidic adenocarcinoma through stem-cell reprogramming. Nature. (2023) 619:860–7. doi: 10.1038/s41586-023-06324-w
16. Dost AFM, Moye AL, Vedaie M, Tran LM, Fung E, Heinze D, et al. Organoids model transcriptional hallmarks of oncogenic KRAS activation in lung epithelial progenitor cells. Cell Stem Cell. (2020) 27:663–78.e8. doi: 10.1016/j.stem.2020.07.022
17. Wang Z, Li Z, Zhou K, Wang C, Jiang L, Zhang L, et al. Deciphering cell lineage specification of human lung adenocarcinoma with single-cell RNA sequencing. Nat Commun. (2021) 12:6500. doi: 10.1038/s41467-021-26770-2
18. Hubbard R, Venn A, Lewis S, Britton J. Lung cancer and cryptogenic fibrosing alveolitis. A population-based cohort study. Am J Respir Crit Care Med. (2000) 161:5–8. doi: 10.1164/ajrccm.161.1.9906062
19. Lee HY, Lee J, Lee CH, Han K, Choi SM. Risk of cancer incidence in patients with idiopathic pulmonary fibrosis: A nationwide cohort study. Respirol (Carlton Vic). (2021) 26:180–7. doi: 10.1111/resp.13911
20. Jiang F, Yin Z, Caraway NP, Li R, Katz RL. Genomic profiles in stage I primary non small cell lung cancer using comparative genomic hybridization analysis of cDNA microarrays. Neoplasia (New York NY). (2004) 6:623–35. doi: 10.1593/neo.04142
21. Imielinski M, Guo G, Meyerson M. Insertions and deletions target lineage-defining genes in human cancers. Cell. (2017) 168:460–72.e14. doi: 10.1016/j.cell.2016.12.025
22. Darawshy F, Rmeileh AA, Kuint R, Berkman N. Possible association between SP-C mutations and lung cancer: Two case reports and review of literature. Cancer Treat Res Commun. (2021) 29:100461. doi: 10.1016/j.ctarc.2021.100461
23. Sowa T, Menju T, Sonobe M, Nakanishi T, Shikuma K, Imamura N, et al. Association between epithelial-mesenchymal transition and cancer stemness and their effect on the prognosis of lung adenocarcinoma. Cancer Med. (2015) 4:1853–62. doi: 10.1002/cam4.556
24. Zuo B, Wang L, Li X, Li X, Wang J, Xiong Y, et al. Abnormal low expression of SFTPC promotes the proliferation of lung adenocarcinoma by enhancing PI3K/AKT/mTOR signaling transduction. Aging (Albany NY). (2023) 15:12451–75. doi: 10.18632/aging.v15i21
25. Yuan Y, Liao H, Pu Q, Ke X, Hu X, Ma Y, et al. miR-410 induces both epithelial-mesenchymal transition and radioresistance through activation of the PI3K/mTOR pathway in non-small cell lung cancer. Signal Transduct Target Ther. (2020) 5:85. doi: 10.1038/s41392-020-0182-2
26. Zhu Y, Pu Q, Zhang Q, Liu Y, Ma Y, Yuan Y, et al. Selenium-binding protein 1 inhibits Malignant progression and induces apoptosis via distinct mechanisms in non-small cell lung cancer. Cancer Med. (2023) 12:17149–70. doi: 10.1002/cam4.6309
27. Bhattacharjee A, Richards WG, Staunton J, Li C, Monti S, Vasa P, et al. Classification of human lung carcinomas by mRNA expression profiling reveals distinct adenocarcinoma subclasses. Proc Natl Acad Sci U S A. (2001) 98:13790–5. doi: 10.1073/pnas.191502998
28. Ren L, Wen X, Liu M, Xiao Y, Leng P, Luo H, et al. Comprehensive analysis of the molecular characteristics and prognosis value of AT II-associated genes in non-small cell lung cancer. Comput Math Methods Med. (2022) 2022:3106688. doi: 10.1155/2022/3106688
29. Li B, Meng YQ, Li Z, Yin C, Lin JP, Zhu DJ, et al. MiR-629-3p-induced downregulation of SFTPC promotes cell proliferation and predicts poor survival in lung adenocarcinoma. Artif Cells Nanomed Biotechnol. (2019) 47:3286–96. doi: 10.1080/21691401.2019.1648283
30. Li B, Ge Z, Song S, Zhang S, Yan H, Huang B, et al. Decreased expression of SOX7 is correlated with poor prognosis in lung adenocarcinoma patients. Pathol Oncol Res. (2012) 18:1039–45. doi: 10.1007/s12253-012-9542-8
31. Sun QY, Ding LW, Johnson K, Zhou S, Tyner JW, Yang H, et al. SOX7 regulates MAPK/ERK-BIM mediated apoptosis in cancer cells. Oncogene. (2019) 38:6196–210. doi: 10.1038/s41388-019-0865-8
32. Yan L, Ma J, Zhu Y, Zan J, Wang Z, Ling L, et al. miR-24-3p promotes cell migration and proliferation in lung cancer by targeting SOX7. J Cell Biochem. (2018) 119:3989–98. doi: 10.1002/jcb.26553
33. Han L, Wang W, Ding W, Zhang L. MiR-9 is involved in TGF-β1-induced lung cancer cell invasion and adhesion by targeting SOX7. J Cell Mol Med. (2017) 21:2000–8. doi: 10.1111/jcmm.13120
34. Liu J, Xiao Q, Xiao J, Niu C, Li Y, Zhang X, et al. Wnt/β-catenin signalling: function, biological mechanisms, and therapeutic opportunities. Signal Transduct Target Ther. (2022) 7:3. doi: 10.1038/s41392-021-00762-6
35. Guo L, Zhong D, Lau S, Liu X, Dong XY, Sun X, et al. Sox7 Is an independent checkpoint for beta-catenin function in prostate and colon epithelial cells. Mol Cancer Res. (2008) 6:1421–30. doi: 10.1158/1541-7786.MCR-07-2175
36. Hu W, Han Y, Yang W, Xu B, Zhang W, Jin Z, et al. Novel role of sex-determining region Y-box 7 (SOX7) in tumor biology and cardiovascular developmental biology. Semin Cancer Biol. (2020) 67:49–56. doi: 10.1016/j.semcancer.2019.08.032
37. Cheng C, Huang Z, Zhou R, An H, Cao G, Ye J, et al. Numb negatively regulates the epithelial-to-mesenchymal transition in colorectal cancer through the Wnt signaling pathway. Am J Physiol Gastrointest Liver Physiol. (2020) 318:G841–g53. doi: 10.1152/ajpgi.00178.2019
38. Guo BJ, Ruan Y, Wang YJ, Xiao CL, Zhong ZP, Cheng BB, et al. Jiedu Recipe, a compound Chinese herbal medicine, inhibits cancer stemness in hepatocellular carcinoma via Wnt/β-catenin pathway under hypoxia. J Integr Med. (2023) 21:474–86. doi: 10.1016/j.joim.2023.06.008
39. Wang L, Liu W, Liu K, Wang L, Yin X, Bo L, et al. The dynamic dysregulated network identifies stage-specific markers during lung adenocarcinoma Malignant progression and metastasis. Mol Ther Nucleic Acids. (2022) 30:633–47. doi: 10.1016/j.omtn.2022.11.019
40. Ni KW, Sun GZ. The identification of key biomarkers in patients with lung adenocarcinoma based on bioinformatics. Math Biosci eng: MBE. (2019) 16:7671–87. doi: 10.3934/mbe.2019384
41. Moreno-Rubio J, Ponce S, Álvarez R, Olmedo ME, Falagan S, Mielgo X, et al. Clinical-pathological and molecular characterization of long-term survivors with advanced non-small cell lung cancer. Cancer Biol Med. (2020) 17:444–57. doi: 10.20892/j.issn.2095-3941.2019.0363
42. Grimm D, Bauer J, Wise P, Krüger M, Simonsen U, Wehland M, et al. The role of SOX family members in solid tumours and metastasis. Semin Cancer Biol. (2020) 67:122–53. doi: 10.1016/j.semcancer.2019.03.004
43. Hayano T, Garg M, Yin D, Sudo M, Kawamata N, Shi S, et al. SOX7 is down-regulated in lung cancer. J Exp Clin Cancer Res. (2013) 32:17. doi: 10.1186/1756-9966-32-17
44. Zhang Y, Stovall DB, Wan M, Zhang Q, Chou JW, Li D, et al. SOX7 target genes and their contribution to its tumor suppressive function. Int J Mol Sci. (2018) 19:1451. doi: 10.3390/ijms19051451
45. Ahirwar DK, Peng B, Charan M, Misri S, Mishra S, Kaul K, et al. Slit2/Robo1 signaling inhibits small-cell lung cancer by targeting β-catenin signaling in tumor cells and macrophages. Mol Oncol. (2023) 17:839–56. doi: 10.1002/1878-0261.13289
46. Salahudeen AA, Choi SS, Rustagi A, Zhu J, van Unen V, de la OS, et al. Progenitor identification and SARS-CoV-2 infection in human distal lung organoids. Nature. (2020) 588:670–5. doi: 10.1038/s41586-020-3014-1
47. Li HJ, Ke FY, Lin CC, Lu MY, Kuo YH, Wang YP, et al. ENO1 promotes lung cancer metastasis via HGFR and WNT signaling-driven epithelial-to-mesenchymal transition. Cancer Res. (2021) 81:4094–109. doi: 10.1158/0008-5472.CAN-20-3543
48. Yu F, Yu C, Li F, Zuo Y, Wang Y, Yao L, et al. Wnt/β-catenin signaling in cancers and targeted therapies. Signal Transduct Target Ther. (2021) 6:307. doi: 10.1038/s41392-021-00701-5
49. Katzen J, Wagner BD, Venosa A, Kopp M, Tomer Y, Russo SJ, et al. An SFTPC BRICHOS mutant links epithelial ER stress and spontaneous lung fibrosis. JCI Insight. (2019) 4:e126125. doi: 10.1172/jci.insight.126125
50. Yao L, Conforti F, Hill C, Bell J, Drawater L, Li J, et al. Paracrine signalling during ZEB1-mediated epithelial-mesenchymal transition augments local myofibroblast differentiation in lung fibrosis. Cell Death different. (2019) 26:943–57. doi: 10.1038/s41418-018-0175-7
51. Lee T, Park JY, Lee HY, Cho YJ, Yoon HI, Lee JH, et al. Lung cancer in patients with idiopathic pulmonary fibrosis: clinical characteristics and impact on survival. Respir Med. (2014) 108:1549–55. doi: 10.1016/j.rmed.2014.07.020
52. Khan KA, Kennedy MP, Moore E, Crush L, Prendeville S, Maher MM, et al. Radiological characteristics, histological features and clinical outcomes of lung cancer patients with coexistent idiopathic pulmonary fibrosis. Lung. (2015) 193:71–7. doi: 10.1007/s00408-014-9664-8
Keywords: SFTPC, NSCLC, EMT, SOX7, Wnt/β-Catenin pathway
Citation: Zhang Q, An N, Liu Y, Zhu Y, Pan W, Gu P, Zhao J, Pu Q and Zhu W (2024) Alveolar type 2 cells marker gene SFTPC inhibits epithelial-to-mesenchymal transition by upregulating SOX7 and suppressing WNT/β-catenin pathway in non-small cell lung cancer. Front. Oncol. 14:1448379. doi: 10.3389/fonc.2024.1448379
Received: 13 June 2024; Accepted: 22 August 2024;
Published: 13 September 2024.
Edited by:
Emanuela Grassilli, Università Link Campus, ItalyReviewed by:
Christine Fillmore Brainson, University of Kentucky, United StatesLuca Mologni, University of Milano-Bicocca, Italy
Copyright © 2024 Zhang, An, Liu, Zhu, Pan, Gu, Zhao, Pu and Zhu. This is an open-access article distributed under the terms of the Creative Commons Attribution License (CC BY). The use, distribution or reproduction in other forums is permitted, provided the original author(s) and the copyright owner(s) are credited and that the original publication in this journal is cited, in accordance with accepted academic practice. No use, distribution or reproduction is permitted which does not comply with these terms.
*Correspondence: Wen Zhu, zhuwen@scu.edu.cn; Qiang Pu, puqiang100@163.com
†These authors have contributed equally to this work and share first authorship