- 1Department of Pharmacy, West China Hospital, Sichuan University, Chengdu, China
- 2Department of Rehabilitation Medicine, West China Hospital, Sichuan University, Chengdu, China
- 3Key Laboratory of Rehabilitation Medicine in Sichuan Province, West China Hospital, Sichuan University, Chengdu, China
- 4Division of Nephrology, National Clinical Research Center for Geriatrics, Kidney Research Institute, West China Hospital of Sichuan University, Chengdu, China
Squamous cell carcinoma (SCC) is a prevalent malignancy affecting multiple organs in the human body, including the oral cavity, esophagus, cervix, and skin. Given its significant incidence and mortality rates, researchers are actively seeking effective diagnostic and therapeutic strategies. In recent years, exosomes and their molecular cargo, particularly circular RNA (circRNA), have emerged as promising areas of investigation in SCC research. Exosomes are small vesicles released into the extracellular environment by cells that contain biomolecules that reflect the physiological state of the cell of origin. CircRNAs, known for their unique covalently closed loop structure and stability, have garnered special attention in oncology and are closely associated with tumorigenesis, progression, metastasis, and drug resistance. Interestingly, exosomal circRNAs have been identified as ideal biomarkers for noninvasive cancer diagnosis and prognosis assessment. This article reviews the progress in research on exosomal circRNAs, focusing on their expression patterns, functions, and potential applications as biomarkers in SCC, aiming to provide new insights and strategies for the diagnosis and treatment of SCC.
1 Introduction
Squamous cell carcinoma (SCC) is an aggressive malignancy originating from the squamous epithelium that affects a wide array of organs, such as the oral cavity, esophagus, cervix, and skin (1–3). The incidence and mortality rates of SCC, a common cancer type, are significant concerns. Notably, SCC accounts for approximately 60% of all malignancies in the orofacial region and accounts for approximately 90% of all esophageal cancer cases (4). Furthermore, it constitutes the majority of cervical and skin cancer diagnoses (5–8). These statistics not only highlight the global prevalence of SCC but also underscore its severe public health implications. Unfortunately, the therapeutic landscape for SCC is fraught with challenges, as most patients are diagnosed at advanced stages, leading to poor treatment outcomes, high recurrence rates, and dismal prognoses. Therefore, the identification of effective diagnostic and prognostic biomarkers is crucial for enhancing patient survival and quality of life. In this context, exosomes and their cargo molecules have attracted significant scientific interest, particularly in the development and progression of SCC.
Exosomes are extracellular vesicles approximately 30–150 nm in diameter that are secreted into the extracellular milieu through exocytosis (9). Originating from the endosomal compartment, they fuse with the plasma membrane to be released from the cell (10). Exosomes are rich in biomolecules, including specific proteins, mRNAs, microRNAs, and notably, circular RNAs (circRNAs), which have recently attracted increased amounts of attention (11). The presence of these molecules not only reflects the biological state of the parent cell but also modulates the behavior of the recipient cells, facilitating intercellular communication and influencing disease pathogenesis (12). CircRNAs, a class of noncoding RNAs characterized by their covalently closed loop structure, are more stable than linear RNAs (13). CircRNAs generated within exosomes by back-splicing are strongly associated with the onset and progression of various diseases, particularly in oncology, where aberrant circRNA expression has been linked to cancer initiation, progression, metastasis, and chemoresistance (14–16). Given their rich circRNA content, exosomes are considered ideal biomarkers for noninvasive cancer diagnostics and prognosis.
In summary, exosomes and their encapsulated circRNAs play a pivotal role in SCC research due to their critical functions in disease biology and their vast potential in clinical applications. An in-depth investigation of the biological properties of exosomes and circRNAs, as well as their expression and function in SCC, promises to open new avenues for early diagnosis, therapeutic decision-making, and prognosis assessment in SCC. This paper reviews the current state of exosomal circRNA research, focusing on its expression patterns, functions, and potential as a biomarker in SCC, with the aim of providing fresh perspectives and strategies for the diagnosis and treatment of SCC.
2 Biological functions of exosomal circRNAs
Extracellular vesicles originating from endocytosis are nanoscale cell-derived vesicles with diameters ranging from approximately 30 to 150 nm. Most cell types are capable of producing extracellular vesicles, which circulate in bodily fluids such as blood, urine, saliva, and breast milk (17). The contents of extracellular vesicles consist of various growth factors, proteins, lipids, nucleic acids, lncRNAs, and circRNAs. Consequently, extracellular vesicles play critical biological roles in cell interactions, affecting multiple cellular activities in both healthy and disease states. Increasing evidence has demonstrated the role of extracellular vesicles in mediating intercellular communication, the tumor microenvironment, immune system function, development and differentiation, cell signaling, and viral replication (18).
Circular RNAs were initially discovered in RNA viruses and are widely present as a diverse class of endogenous noncoding RNAs (19). Initially, considered an aberrant splicing byproduct of RNA, circRNA forms a covalently closed continuous loop structure after backsplicing of exons, introns or both. This closed loop structure of circRNA prevents degradation by RNA exonucleases or RNases, making it more stable than linear RNA and potentially serving as a disease biomarker. Over 80% of circRNAs overlap with protein-coding regions, suggesting that circRNAs may play important roles in diseases or serve as novel biomarkers. CircRNAs function in various key ways, negatively regulating miRNA expression, acting as miRNA sponges, regulating splicing and transcription, posttranscriptionally modulating gene expression, and potentially influencing cell growth and invasion processes in various cancers, including gastric, colorectal, and esophageal cancers (17).
Numerous studies have demonstrated that circRNAs have significant impacts on cancer progression and treatment (Figure 1), exerting regulatory effects on the tumor microenvironment and serving as potential therapeutic targets and novel cancer biomarkers. Research has shown that circRNAs are highly enriched in extracellular vesicles and can exist stably within them, with a significantly increased abundance of circRNAs compared to those secreted by normal cells (12, 20). Several circRNA species with potential biological functions have been identified in extracellular vesicles, especially in human serum-derived extracellular vesicles containing more than 1,000 circRNAs, possibly originating from tumors (21, 22). Some circRNAs have been detected in serum, urine, and tumor-derived extracellular vesicles. Tumor-derived extracellular vesicle circRNAs may participate in processes such as cell growth, angiogenesis, and epithelial–mesenchymal transition (23).
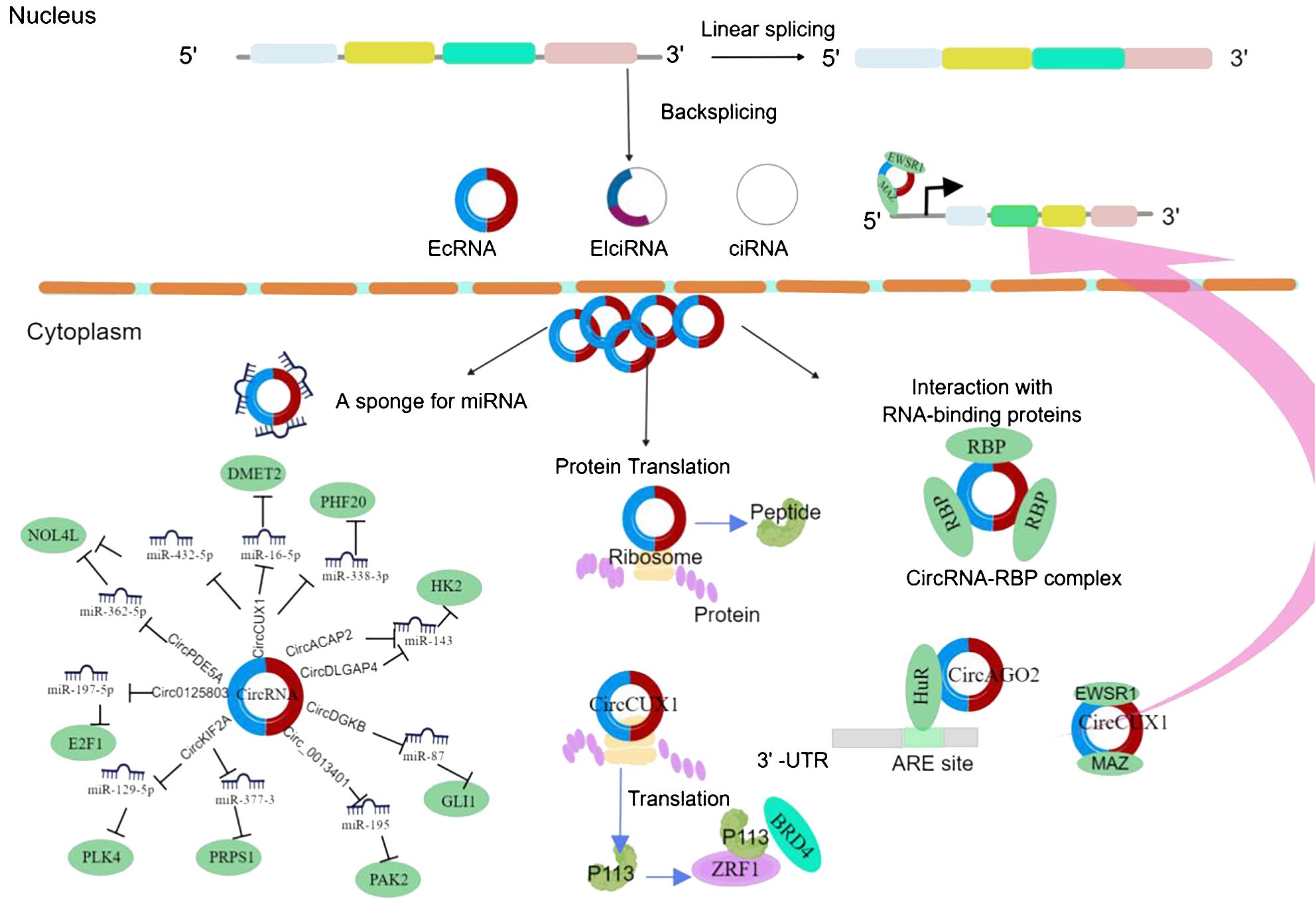
Figure 1 CircRNA biogenesis and mechanism in cancer cell progression. EcRNA, exonic circRNA; EIciRNA, exonic-intronic circRNA; CiRNA, intronic circRNA. EWSR1, EWS RNA-binding protein 1; MAZ, MYC-associated zinc finger protein; DMRT2, Doublesex and mab-3-related transcription factor 2; NOL4L, nucleolar protein 4-like; PHF20, PHD finger protein 20; BRD4, bromodomain protein 4; PLK4, polo-like kinase 4; PRPS1, phosphoribosyl pyrophosphate synthetase 1; GLI1, glioma-associated oncogene 1; AGO2, Argonaute 2; HK2, hexokinase 2; PAK2, p21-activated kinase 2; HuR, human antigen R.
Studies have shown that the abundance of circRNA in extracellular vesicles is twice that of the parent cells and six times that of linear RNA (20). The sorting of circRNA into extracellular vesicles is regulated by changes in related miRNA levels in parent cells and facilitates the transfer of biological activity to recipient cells, thereby participating in intercellular communication (20). The nanoscale size and lipid bilayer structure of extracellular vesicles can prolong the circulation time of circRNAs and enhance their biological activity. Therefore, extracellular vesicle circRNAs possess both the targeting properties of extracellular vesicle-like transfer and the inherent biological functions of circRNAs, offering more significant regulatory advantages (24). Multiple studies have shown that tumor-specific circRNAs can be selectively packaged, secreted, and transported via tumor-derived extracellular vesicles, participating in the regulation of the tumor microenvironment to promote or inhibit tumor cell growth and metastasis. Extracellular vesicle circRNAs exhibit greater diagnostic sensitivity and specificity than freely circulating circRNAs in body fluids and could serve as valuable biomarkers for clinical diagnosis and prognosis (25).
3 The role of exosomal circRNAs in the diagnosis and progression of squamous cell carcinoma
CircRNAs play crucial roles in exosomes and are closely associated with the development of squamous cell carcinoma (26). A study revealed that high expression of circ_0000199 in oral squamous cell carcinoma is linked to increased recurrence and mortality rates, with its overexpression promoting tumor cell growth while its silencing exhibiting inhibitory effects (27). Similarly, circ_0072387 inhibits the progression of oral squamous cell carcinoma by regulating the expression of microRNA-503–5p (miR-503–5p), suggesting its potential as a therapeutic target for this disease (28). Research by Tang et al. further revealed that the high expression of circFNDC3B in serum exosomes is directly associated with the occurrence and development of esophageal squamous cell carcinoma, possibly serving as an independent risk predictor for aiding in early diagnosis (29). CircfNDC3B not only affects tumor cells through exosomal transfer but also functions through the miR-490–5p/TXNRD1 axis, further driving the progression of esophageal squamous cell carcinoma (30). Transfection of exosomal circ-CYP24A1 can inhibit the malignant behavior of cutaneous squamous cell carcinoma, offering new possibilities for noninvasive therapeutic strategies and potentially serving as a diagnostic marker (31). In CC, the expression level of circRNA_0000285 in cancer tissue samples was significantly greater than that in corresponding normal tissue samples. After knocking out circRNA_0000285, the expression of downstream FUS was significantly downregulated, which indicates that circRNA_0000285 may enhance the proliferation of cervical squamous cell carcinoma cells by upregulating FUS and provides potential therapeutic targets for research on cervical cancer (32). Additionally, a significant reduction in the impact of circ_0005576 silencing on cervical squamous cell carcinoma cells can be achieved by inhibiting miR-153–3p, confirming that circ_0005576 promotes cancer progression through the miR-153–3p/KIF20A axis and that its overexpression enhances cell proliferation and migration through a sponge effect on miR-153–3p (33). In conclusion, exosomal circRNAs not only serve as biomarkers for squamous cell carcinoma but also reveal potential therapeutic targets through their dynamic regulation. Future research should further explore the specific functions of these circRNAs and their potential applications in cancer prevention and treatment.
4 The role of exosomal circRNAs in squamous cell carcinoma metastasis
The development and metastasis of tumors represent a complex and multifaceted process that involves the synergistic action of multiple oncogenes and pathways. Recent studies have highlighted the indispensable role of circRNAs in this process. For instance, Li et al. demonstrated the upregulation of circMYOF in laryngeal cancer, where its silencing inhibited tumor cell growth, metastasis, and glycolysis, thereby suppressing overall tumor progression (34). Notably, circMYOF is overexpressed in the serum exosomes of patients with laryngeal cancer and promotes cell migration by regulating the miR-145–5p/OTX1 axis, thereby accelerating the progression of laryngeal cancer. In the case of esophageal squamous cell carcinoma, lymph node metastasis represents a critical stage of progression. Studies have shown that the level of circ_0026611 in serum exosomes is significantly elevated in patients with lymph node metastasis, and its expression level can serve as a biomarker for assessing lymph node metastasis (35). Exosomal circ_0026611 derived from ESCC cells can inhibit NAA10-mediated PROX1 protein acetylation and ubiquitination to increase the expression level of the PROX1 protein (36). These findings suggest that circ_0026611 has potential value in the clinical monitoring and prognostic assessment of esophageal squamous cell carcinoma (35). Exosomal circRNAs not only directly enhance the invasiveness of tumor cells but also influence the invasive behavior of cancer cells by regulating the tumor microenvironment (37). Particularly in the malignant progression of lymph node metastasis, the preoperative detection of circRNAs in serum exosomes could provide valuable information for the early identification of lymph node metastasis. Therefore, the analysis of exosomal circRNAs is highly important for understanding the pathways involved in squamous cell carcinoma metastasis and optimizing personalized treatment strategies.
5 The role of exosomal circRNAs in the treatment of squamous cell carcinoma
The significance of exosomal circRNAs in cancer biology is increasingly evident, as their potential as therapeutic targets and biomarkers has attracted increased amounts of attention (38, 39). Studies have revealed that exosomal circGDI2 regulates the malignant behaviors of oral squamous cell carcinoma cells by targeting the miR-424–5p/SCAI axis. CircRNA GDP dissociation inhibitor 2 (circGDI2) can be transferred via exosomes, positioning it as a novel exosome-based biomarker and therapeutic agent for treating oral squamous cell carcinoma (40). Cancer stemness and immune evasion are closely linked and play pivotal roles in tumor development and resistance to immunotherapy. Elevated circFAT1 promotes the activation of STAT3, thereby mediating a positive correlation between cancer stemness and immune evasion (41). CircFAT1 knockdown (KD) enhances CD8+ cell infiltration into the tumor microenvironment and augments the efficacy of PD-1 blockade immunotherapy. CircFAT1 interacts with cytoplasmic STAT3 to prevent SHP1-induced dephosphorylation of STAT3, promoting its activation and inhibiting STAT1-mediated transcription, establishing circFAT1 as a crucial regulator of cancer stemness and antitumor immunity. Similarly, overexpression of circ_0060927 reduces the activity and promotes the apoptosis of skin squamous cell carcinoma cells while inhibiting epithelial–mesenchymal transition (EMT) processes within cells. The overexpression of circ_0060927 impedes the Wnt signaling pathway via miR-4438, thereby affecting proliferation, apoptosis, and EMT in skin squamous cell carcinoma cells. Recent studies have suggested that exosomes can regulate the immune system and serve as potential agents in immune therapy. Exosomal circRNAs are increasingly regarded as a novel subset of endogenous RNAs that regulate target genes either by modulating miRNA activity or by forming complexes with target proteins. Studies indicate that exosomal circRNAs can affect the drug resistance of tumor cells, and their significant role as mediators of chemotherapy resistance is gradually being elucidated (42).
6 The role of circRNAs in the regulation of tumor chemotherapy and radiotherapy resistance
In the management of locally advanced malignancies, radiotherapy is frequently employed, either as an adjunct to surgical interventions or synergistically with chemotherapeutic agents (43). A significant barrier to the efficacy of these therapies in patients with advanced cancer is the development of resistance to chemotherapy (44). One of the pivotal mechanisms underpinning this resistance involves ATP-binding cassette B1 (ABCB1), a protein associated with multidrug resistance that is overexpressed in resistant cell lines and facilitates the extracellular expulsion of intracellular chemotherapeutic agents (45, 46). Consequently, targeting and inhibiting the expression of ABCB1 is a promising strategy for mitigating drug resistance in tumors (47). A notable example of this mechanism is observed in oral squamous cell carcinoma (OSCC), where circ_0109291 has been shown to enhance resistance to cisplatin by modulating ABCB1 expression primarily through the adsorption of miR-188–3p. This interaction provides fundamental insight for strategies aimed at reducing the prevalence of resistance in OSCC (48).
Furthermore, heat shock protein 27 (HSP27, also known as HSPB1) is a member of the small heat shock protein superfamily with Phospho-HSP27 (Ser15), Phospho-HSP27 (Ser78), and Phospho-HSP27 (Ser82) receptor sites (49). The function of HSP27 is regulated by posttranslational phosphorylation (50). In tongue squamous cell carcinoma (SCCT), HSP27 augments multidrug resistance by activating the NF-κB pathway (51). The overexpression of HSP27 is associated with cellular resistance to various chemotherapeutic agents, including cisplatin and staurosporin, in laryngeal squamous cell carcinoma (LSCC) through mechanisms involving the induction of cell cycle arrest and alterations in actin polymerization, which affect drug uptake (52). Interestingly, the circRNA circGNG7 has been shown to interfere with the phosphorylation of HSP27 at Ser78 and Ser82 in head and neck squamous cell carcinoma (HNSCC), thus impeding its phosphorylation within malignant signaling pathways and potentially reducing chemoresistance in HNSCC (53). In addition, circ-PKD2 in OSCC functions as a tumor suppressor by promoting the expression of autophagy-related 13 (Atg13) through the adsorption of miR-646, thereby enhancing sensitivity to cisplatin (54). Radiotherapy tolerability also serves as a critical prognostic indicator in patients with HNSCC. The upregulation of circCUX1 in radiation-resistant hypopharyngeal squamous cell carcinoma (HPSCC) is correlated with poorer survival outcomes (55). CircCUX1 interacts with caspase-1 mRNA, inhibiting its expression, which in turn modulates the inflammatory response of tumor cells to radiation, thereby fostering tolerance to radiation therapy.
7 Challenges and prospects
Exosomal circRNAs represent a cutting-edge area of research in oncology. These molecules are known to regulate key cancer cell processes, such as proliferation, invasion, migration, and metastasis, and hold potential as biomarkers for early cancer diagnosis. Increasing evidence has highlighted the significant role of exosomal circRNAs in the initiation and progression of squamous cell carcinoma (Table 1) (87). CircRNAs can modulate the expression of downstream oncogenic molecules through the circRNA-miRNA−mRNA axis, playing a crucial role in the progression of squamous cell carcinoma (17). The levels of specific exosomal circRNAs, which are correlated with tumor development in specific patients, have demonstrated potential as early diagnostic and prognostic biomarkers. These circRNAs circulate in bodily fluids via exosomes, enabling noninvasive detection. Additionally, exosomal circRNAs can influence the tumor microenvironment by facilitating communication between tumor cells and surrounding cells (such as immune and endothelial cells), regulating cancer cells’ ability to evade the immune system, and affecting angiogenesis (88). Exploring these mechanisms could provide insights for the development of new anticancer strategies. Drug resistance remains a critical factor in the failure of therapies for the treatment of squamous cell carcinoma. Some exosomal circRNAs have been shown to induce resistance in cancer cells to chemotherapy or radiotherapy by modulating intracellular signaling pathways (89). Therefore, the study of these circular RNAs not only aids in understanding the resistance mechanism but also may provide new approaches for overcoming this resistance.
While exosomal circRNAs hold great potential as cancer biomarkers, their detection and identification still face technological challenges, particularly in terms of sensitivity and specificity in low-abundance samples (90). Moreover, the overlap of circular structures and sequences with their linear mRNA counterparts makes accurate assessment of circular RNA expression and function challenging. The mechanisms by which exosomal circRNAs contribute to cancer development are extremely complex and involve not only gene regulation and protein expression and function but also potentially unknown pathways, necessitating extensive and in-depth studies from the molecular to the systems biology level. Furthermore, the mechanisms by which circRNAs are enriched during the formation of exosomes remain unclear. Although exosomal circRNAs show potential in cancer diagnosis and therapy, their translation from basic research to clinical application faces multiple challenges, including but not limited to the validation of biomarkers, confirmation of therapeutic targets, and assessment of the safety and efficacy of corresponding treatment strategies.
8 Conclusion
Exosomal circRNAs have emerged as promising biomarkers and therapeutic targets in the study of squamous cell carcinoma. These molecules are integral to the onset, progression, and therapeutic response of tumors, providing innovative avenues for cancer diagnosis, prognostic assessment, and the formulation of new therapeutic approaches. Nonetheless, significant challenges persist, encompassing technological limitations, lack of comprehensive insight into their biological mechanisms, and barriers to clinical implementation. Future research needs to address these issues to fully harness the potential of exosomal circRNAs in the treatment of squamous cell carcinoma.
Author contributions
RW: Writing – original draft. SW: Writing – review & editing. HJ: Writing – review & editing. YL: Supervision, Writing – review & editing. SY: Project administration, Writing – review & editing, Funding acquisition.
Funding
The author(s) declare financial support was received for the research, authorship, and/or publication of this article. This research was supported by 1•3•5 project for disciplines of excellence-Clinical Research Incubation Project, West China Hospital, Sichuan University (NO.2019HXFH067) and the Sciencetechnology Support Plan Projects in Sichuan Province (No2021YFS0162).
Conflict of interest
The authors declare that the research was conducted in the absence of any commercial or financial relationships that could be construed as a potential conflict of interest.
Publisher’s note
All claims expressed in this article are solely those of the authors and do not necessarily represent those of their affiliated organizations, or those of the publisher, the editors and the reviewers. Any product that may be evaluated in this article, or claim that may be made by its manufacturer, is not guaranteed or endorsed by the publisher.
References
1. Dreyfuss I, Kamath P, Frech F, Hernandez L, Nouri K. Squamous cell carcinoma: 2021 updated review of treatment. Dermatol Ther. (2022) 35:e15308. doi: 10.1111/dth.15308
2. Codipilly DC, Wang KK. Squamous cell carcinoma of the esophagus. Gastroenterol Clinics North America. (2022) 51:457–84. doi: 10.1016/j.gtc.2022.06.005
3. González-Guevara MB, Linares-Vieyra C, Castro-García ME, Muñiz-Lino MA, Abaroa-Chauvet C, Bello-Torrejón F. [Oral squamous cell carcinoma. Case report and review of literature]. Rev Med del Instituto Mexicano del Seguro Social. (2022) 60:85–90.
4. Liu Y. Perioperative immunotherapy for esophageal squamous cell carcinoma: Now and future. World J Gastroenterol. (2023) 29:5020–37. doi: 10.3748/wjg.v29.i34.5020
5. Li J, Gu C, Zheng H, Geng X, Yang Z, Zhou L, et al. Ultrasonographic diagnosis in rare primary cervical cancer. Int J Gynecol Cancer: Off J Int Gynecol Cancer Soc. (2021) 31:1535–40. doi: 10.1136/ijgc-2021-002860
6. Winge MCG, Kellman LN, Guo K, Tang JY, Swetter SM, Aasi SZ, et al. Advances in cutaneous squamous cell carcinoma. Nat Rev Cancer. (2023) 23:430–49. doi: 10.1038/s41568-023-00583-5
7. CorChado-Cobos R, García-Sancha N, González-Sarmiento R, Pérez-Losada J, Cañueto J. Cutaneous squamous cell carcinoma: from biology to therapy. Int J Mol Sci. (2020) 21:2956–78. doi: 10.3390/ijms21082956
8. Heppt MV, Leiter U. Cutaneous squamous cell carcinoma: state of the art, perspectives and unmet needs. J der Deutschen Dermatologischen Gesellschaft = J German Soc Dermatol: JDDG. (2023) 21:421–4. doi: 10.1111/ddg.15052
9. Wang Y-K, Bao Y-R, Liang Y-X, Chen Y-J, Huang W-H, Xie M. Current progress and prospect of microfluidic-based exosome investigation. TrAC Trends Anal Chem. (2023) 168:117310. doi: 10.1016/j.trac.2023.117310
10. Wang C, Liu J, Yan Y, Tan Y. Role of exosomes in chronic liver disease development and their potential clinical applications. J Immunol Res. (2022) 2022:1695802. doi: 10.1155/2022/1695802
11. Yi Q, Yue J, Liu Y, Shi H, Sun W, Feng J, et al. Recent advances of exosomal circRNAs in cancer and their potential clinical applications. J Trans Med. (2023) 21:516. doi: 10.1186/s12967-023-04348-4
12. Han Z, Chen H, Guo Z, Shen J, Luo W, Xie F, et al. Circular RNAs and their role in exosomes. Front Oncol. (2022) 12:848341. doi: 10.3389/fonc.2022.848341
13. Loan Young T, Chang Wang K, James Varley A, Li B. Clinical delivery of circular RNA: Lessons learned from RNA drug development. Adv Drug Deliv Rev. (2023) 197:114826. doi: 10.1016/j.addr.2023.114826
14. Greene J, Baird AM, Brady L, Lim M, Gray SG, McDermott R, et al. Circular RNAs: biogenesis, function and role in human diseases. Front Mol Biosci. (2017) 4:38. doi: 10.3389/fmolb.2017.00038
15. Babin L, Andraos E, Fuchs S, Pyronnet S, Brunet E, Meggetto F. From circRNAs to fusion circRNAs in hematological Malignancies. JCI Insight. (2021) 6. doi: 10.1172/jci.insight.151513
16. Samavarchi Tehrani S, Esmaeili F, Shirzad M, Goodarzi G, Yousefi T, Maniati M, et al. The critical role of circular RNAs in drug resistance in gastrointestinal cancers. Med Oncol (Northwood London England). (2023) 40:116. doi: 10.1007/s12032-023-01980-4
17. Wang Y, Liu J, Ma J, Sun T, Zhou Q, Wang W, et al. Exosomal circRNAs: biogenesis, effect and application in human diseases. Mol Cancer. (2019) 18:116. doi: 10.1186/s12943-019-1041-z
18. Guo X, Tan W, Wang C. The emerging roles of exosomal circRNAs in diseases. Clin Trans Oncol: Off Publ Fed Spanish Oncol Societies Natl Cancer Institute Mexico. (2021) 23:1020–33. doi: 10.1007/s12094-020-02485-6
19. Zhou M, Xiao MS, Li Z, Huang C. New progresses of circular RNA biology: from nuclear export to degradation. RNA Biol. (2021) 18:1365–73. doi: 10.1080/15476286.2020.1853977
20. Li Y, Zheng Q, Bao C, Li S, Guo W, Zhao J, et al. Circular RNA is enriched and stable in exosomes: a promising biomarker for cancer diagnosis. Cell Res. (2015) 25:981–4. doi: 10.1038/cr.2015.82
21. Cui J, Wang J, Liu L, Zou C, Zhao Y, Xue Z, et al. Presence and prospects of exosomal circRNAs in cancer (Review). Int J Oncol. (2023) 62. doi: 10.3892/ijo
22. Tuo B, Chen Z, Dang Q, Chen C, Zhang H, Hu S, et al. Roles of exosomal circRNAs in tumour immunity and cancer progression. Cell Death Dis. (2022) 13:539. doi: 10.1038/s41419-022-04949-9
23. Dou Y, Cha DJ, Franklin JL, Higginbotham JN, Jeppesen DK, Weaver AM, et al. Circular RNAs are down-regulated in KRAS mutant colon cancer cells and can be transferred to exosomes. Sci Rep. (2016) 6:37982. doi: 10.1038/srep37982
24. Bai H, Lei K, Huang F, Jiang Z, Zhou X. Exo-circRNAs: a new paradigm for anticancer therapy. Mol Cancer. (2019) 18:56. doi: 10.1186/s12943-019-0986-2
25. Wang S, Dong Y, Gong A, Kong H, Gao J, Hao X, et al. Exosomal circRNAs as novel cancer biomarkers: Challenges and opportunities. Int J Biol Sci. (2021) 17:562–73. doi: 10.7150/ijbs.48782
26. Momen-Heravi F, Bala S. Emerging role of non-coding RNA in oral cancer. Cell Signal. (2018) 42:134–43. doi: 10.1016/j.cellsig.2017.10.009
27. Luo Y, Liu F, Guo J, Gui R. Upregulation of circ_0000199 in circulating exosomes is associated with survival outcome in OSCC. Sci Rep. (2020) 10:13739. doi: 10.1038/s41598-020-70747-y
28. Han L, Cheng J, Li A. hsa_circ_0072387 suppresses proliferation, metastasis, and glycolysis of oral squamous cell carcinoma cells by downregulating miR-503–5p. Cancer Biother Radiopharm. (2021) 36:84–94. doi: 10.1089/cbr.2019.3371
29. Tang B, Zhang Q, Liu K, Huang Y. Exosomal circRNA FNDC3B promotes the progression of esophageal squamous cell carcinoma by sponging miR-490–5p and regulating thioredoxin reductase 1 expression. Bioengineered. (2022) 13:13829–48. doi: 10.1080/21655979.2022.2084484
30. Sun K, Yao H, Zhang P, Sun Y, Ma J, Xia Q. Emerging landscape of circFNDC3B and its role in human Malignancies. Front Oncol. (2023) 13:1097956. doi: 10.3389/fonc.2023.1097956
31. Zhang Z, Guo H, Yang W, Li J. Exosomal circular RNA RNA-Seq profiling and the carcinogenic role of exosomal circ-CYP24A1 in cutaneous squamous cell carcinoma. Front Med. (2021) 8:675842. doi: 10.3389/fmed.2021.675842
32. Chen RX, Liu HL, Yang LL, Kang FH, Xin LP, Huang LR, et al. Circular RNA circRNA_0000285 promotes cervical cancer development by regulating FUS. Eur Rev Med Pharmacol Sci. (2019) 23:8771–8. doi: 10.26355/eurrev_201910_19271
33. Ma H, Tian T, Liu X, Xia M, Chen C, Mai L, et al. Upregulated circ_0005576 facilitates cervical cancer progression via the miR-153/KIF20A axis. Biomed Pharmacother = Biomed Pharmacother. (2019) 118:109311. doi: 10.1016/j.biopha.2019.109311
34. Li S, Zhang Y, He Z, Xu Q, Li C, Xu B. Knockdown of circMYOF inhibits cell growth, metastasis, and glycolysis through miR-145–5p/OTX1 regulatory axis in laryngeal squamous cell carcinoma. Funct Integr Genomics. (2022) 22:1–13. doi: 10.1007/s10142-022-00862-8
35. Liu S, Lin Z, Rao W, Zheng J, Xie Q, Lin Y, et al. Upregulated expression of serum exosomal hsa_circ_0026611 is associated with lymph node metastasis and poor prognosis of esophageal squamous cell carcinoma. J Cancer. (2021) 12:918–26. doi: 10.7150/jca.50548
36. Yao W, Jia X, Zhu L, Xu L, Zhang Q, Xia T, et al. Exosomal circ_0026611 contributes to lymphangiogenesis by reducing PROX1 acetylation and ubiquitination in human lymphatic endothelial cells (HLECs). Cell Mol Biol Lett. (2023) 28:13. doi: 10.1186/s11658-022-00410-z
37. Lu Q, Wang X, Zhu J, Fei X, Chen H, Li C. Hypoxic tumor-derived exosomal Circ0048117 facilitates M2 macrophage polarization acting as miR-140 sponge in esophageal squamous cell carcinoma. OncoTargets Ther. (2020) 13:11883–97. doi: 10.2147/OTT.S284192
38. Liu Q, Li S. Exosomal circRNAs: Novel biomarkers and therapeutic targets for urinary tumors. Cancer Lett. (2024) 588:216759. doi: 10.1016/j.canlet.2024.216759
39. Wang D, Li R, Jiang J, Qian H, Xu W. Exosomal circRNAs: Novel biomarkers and therapeutic targets for gastrointestinal tumors. Biomed Pharmacother = Biomed Pharmacother. (2023) 157:114053. doi: 10.1016/j.biopha.2022.114053
40. Zhang Y, Tang K, Chen L, Du M, Qu Z. Exosomal CircGDI2 suppresses oral squamous cell carcinoma progression through the regulation of MiR-424–5p/SCAI axis. Cancer Manage Res. (2020) 12:7501–14. doi: 10.2147/CMAR.S255687
41. Jia L, Wang Y, Wang CY. circFAT1 promotes cancer stemness and immune evasion by promoting STAT3 activation. Adv Sci (Weinheim Baden-Wurttemberg Germany). (2021) 8:2003376. doi: 10.1002/advs.202003376
42. Ye D, Gong M, Deng Y, Fang S, Cao Y, Xiang Y, et al. Roles and clinical application of exosomal circRNAs in the diagnosis and treatment of Malignant tumors. J Trans Med. (2022) 20:161. doi: 10.1186/s12967-022-03367-x
43. Marur S, Forastiere AA. Head and neck squamous cell carcinoma: update on epidemiology, diagnosis, and treatment. Mayo Clin Proc. (2016) 91:386–96. doi: 10.1016/j.mayocp.2015.12.017
44. Yang L, Xie HJ, Li YY, Wang X, Liu XX, Mai J. Molecular mechanisms of platinum−based chemotherapy resistance in ovarian cancer (Review). Oncol Rep. (2022) 47. doi: 10.3892/or
45. Sui H, Fan ZZ, Li Q. Signal transduction pathways and transcriptional mechanisms of ABCB1/Pgp-mediated multiple drug resistance in human cancer cells. J Int Med Res. (2012) 40:426–35. doi: 10.1177/147323001204000204
46. Barzegar S, Pirouzpanah S. Zinc finger proteins and ATP-binding cassette transporter-dependent multidrug resistance. Eur J Clin Invest. (2024) 54:e14120. doi: 10.1111/eci.14120
47. Wu CP, Li YC, Murakami M, Hsiao SH, Lee YC, Huang YH, et al. Furmonertinib, a third-generation EGFR tyrosine kinase inhibitor, overcomes multidrug resistance through inhibiting ABCB1 and ABCG2 in cancer cells. Int J Mol Sci. (2023) 24. doi: 10.3390/ijms241813972
48. Gao F, Han J, Wang Y, Jia L, Luo W, Zeng Y. Circ_0109291 promotes cisplatin resistance of oral squamous cell carcinoma by sponging miR-188–3p to increase ABCB1 expression. Cancer Biother Radiopharm. (2022) 37:233–45. doi: 10.1089/cbr.2020.3928
49. Choi SK, Kam H, Kim KY, Park SI, Lee YS. Targeting heat shock protein 27 in cancer: A druggable target for cancer treatment? Cancers. (2019) 11. doi: 10.20944/preprints201907.0081.v1
50. Benjamin IJ, McMillan DR. Stress (heat shock) proteins: molecular chaperones in cardiovascular biology and disease. Circ Res. (1998) 83:117–32. doi: 10.1161/01.RES.83.2.117
51. Zheng G, Zhang Z, Liu H, Xiong Y, Luo L, Jia X, et al. HSP27-mediated extracellular and intracellular signaling pathways synergistically confer chemoresistance in squamous cell carcinoma of tongue. Clin Cancer Res: an Off J Am Assoc Cancer Res. (2018) 24:1163–75. doi: 10.1158/1078-0432.CCR-17-2619
52. Lee JH, Sun D, Cho KJ, Kim MS, Hong MH, Kim IK, et al. Overexpression of human 27 kDa heat shock protein in laryngeal cancer cells confers chemoresistance associated with cell growth delay. J Cancer Res Clin Oncol. (2007) 133:37–46. doi: 10.1007/s00432-006-0143-3
53. Ju H, Hu Z, Wei D, Huang J, Zhang X, Rui M, et al. A novel intronic circular RNA, circGNG7, inhibits head and neck squamous cell carcinoma progression by blocking the phosphorylation of heat shock protein 27 at Ser78 and Ser82. Cancer Commun (London England). (2021) 41:1152–72. doi: 10.1002/cac2.12213
54. Gao L, Zhang Q, Li S, Zheng J, Ren W, Zhi K. Circ-PKD2 promotes Atg13-mediated autophagy by inhibiting miR-646 to increase the sensitivity of cisplatin in oral squamous cell carcinomas. Cell Death Dis. (2022) 13:192. doi: 10.1038/s41419-021-04497-8
55. Wu P, Fang X, Liu Y, Tang Y, Wang W, Li X, et al. N6-methyladenosine modification of circCUX1 confers radioresistance of hypopharyngeal squamous cell carcinoma through caspase1 pathway. Cell Death Dis. (2021) 12:298. doi: 10.1038/s41419-021-03558-2
56. Su W, Wang Y, Wang F, Sun S, Li M, Shen Y, et al. Hsa_circ_0005379 regulates Malignant behavior of oral squamous cell carcinoma through the EGFR pathway. BMC Cancer. (2019) 19:400. doi: 10.1186/s12885-019-5593-5
57. Zhong S, Feng J. CircPrimer 2.0: a software for annotating circRNAs and predicting translation potential of circRNAs. BMC Bioinf. (2022) 23:215. doi: 10.1186/s12859-022-04705-y
58. Zhong S, Wang J, Zhang Q, Xu H, Feng J. CircPrimer: a software for annotating circRNAs and determining the specificity of circRNA primers. BMC Bioinf. (2018) 19:292. doi: 10.1186/s12859-018-2304-1
59. Zhang PF, Gao C, Huang XY, Lu JC, Guo XJ, Shi GM, et al. Cancer cell-derived exosomal circUHRF1 induces natural killer cell exhaustion and may cause resistance to anti-PD1 therapy in hepatocellular carcinoma. Mol Cancer. (2020) 19:110. doi: 10.1186/s12943-020-01222-5
60. Zhao W, Cui Y, Liu L, Qi X, Liu J, Ma S, et al. Splicing factor derived circular RNA circUHRF1 accelerates oral squamous cell carcinoma tumorigenesis via feedback loop. Cell Death Differ. (2020) 27:919–33. doi: 10.1038/s41418-019-0423-5
61. Dou Z, Gao L, Ren W, Zhang H, Wang X, Li S, et al. CiRS-7 functions as a ceRNA of RAF-1/PIK3CD to promote metastatic progression of oral squamous cell carcinoma via MAPK/AKT signaling pathways. Exp Cell Res. (2020) 396:112290. doi: 10.1016/j.yexcr.2020.112290
62. Chen G, Li Y, He Y, Zeng B, Yi C, Wang C, et al. Upregulation of circular RNA circATRNL1 to sensitize oral squamous cell carcinoma to irradiation. Mol Ther Nucleic Acids. (2020) 19:961–73. doi: 10.1016/j.omtn.2019.12.031
63. Li K, Fan X, Yan Z, Zhan J, Cao F, Jiang Y. Circ_0000745 strengthens the expression of CCND1 by functioning as miR-488 sponge and interacting with HuR binding protein to facilitate the development of oral squamous cell carcinoma. Cancer Cell Int. (2021) 21:271. doi: 10.1186/s12935-021-01884-1
64. Su W, Sun S, Wang F, Shen Y, Yang H. Retraction Note: Circular RNA hsa_circ_0055538 regulates the Malignant biological behavior of oral squamous cell carcinoma through the p53/Bcl-2/caspase signaling pathway. J Trans Med. (2023) 21:312. doi: 10.1186/s12967-023-04147-x
65. Zheng X, Du F, Gong X, Xu P. Circ_0005320 promotes oral squamous cell carcinoma tumorigenesis by sponging microRNA-486–3p and microRNA-637. Bioengineered. (2022) 13:440–54. doi: 10.1080/21655979.2021.2009317
66. Wang J, Jiang C, Li N, Wang F, Xu Y, Shen Z, et al. The circEPSTI1/mir-942–5p/LTBP2 axis regulates the progression of OSCC in the background of OSF via EMT and the PI3K/Akt/mTOR pathway. Cell Death Dis. (2020) 11:682. doi: 10.1038/s41419-020-02851-w
67. Chen B, Wei W, Huang X, Xie X, Kong Y, Dai D, et al. circEPSTI1 as a prognostic marker and mediator of triple-negative breast cancer progression. Theranostics. (2018) 8:4003–15. doi: 10.7150/thno.24106
68. Peng QS, Cheng YN, Zhang WB, Fan H, Mao QH, Xu P. circRNA_0000140 suppresses oral squamous cell carcinoma growth and metastasis by targeting miR-31 to inhibit Hippo signaling pathway. Cell Death Dis. (2020) 11:112. doi: 10.1038/s41419-020-2273-y
69. He Y, Huang H, Jin L, Zhang F, Zeng M, Wei L, et al. CircZNF609 enhances hepatocellular carcinoma cell proliferation, metastasis, and stemness by activating the Hedgehog pathway through the regulation of miR-15a-5p/15b-5p and GLI2 expressions. Cell Death Dis. (2020) 11:358. doi: 10.1038/s41419-020-2441-0
70. Yin X, Wang J, Shan C, Jia Q, Bian Y, Zhang H. Circular RNA ZNF609 promotes laryngeal squamous cell carcinoma progression by upregulating epidermal growth factor receptor via sponging microRNA-134–5p. Bioengineered. (2022) 13:6929–41. doi: 10.1080/21655979.2022.2034703
71. Zhang J, Hu H, Zhao Y, Zhao Y. CDR1as is overexpressed in laryngeal squamous cell carcinoma to promote the tumour’s progression via miR-7 signals. Cell Prolif. (2018) 51:e12521. doi: 10.1111/cpr.12521
72. Mao W, Wang K, Xu B, Zhang H, Sun S, Hu Q, et al. Correction to: ciRS-7 is a prognostic biomarker and potential gene therapy target for renal cell carcinoma. Mol Cancer. (2021) 20:155. doi: 10.1186/s12943-021-01459-8
73. Wu T, Wang G, Zeng X, Sun Z, Li S, Wang W, et al. Hsa_circ_0006232 promotes laryngeal squamous cell cancer progression through FUS-mediated EZH2 stabilization. Cell Cycle (Georgetown Tex). (2021) 20:1799–811. doi: 10.1080/15384101.2021.1959973
74. Li J, Huang C, Zou Y, Yu J, Gui Y. Circular RNA MYLK promotes tumour growth and metastasis via modulating miR-513a-5p/VEGFC signalling in renal cell carcinoma. J Cell Mol Med. (2020) 24:6609–21. doi: 10.1111/jcmm.15308
75. Duan X, Shen N, Chen J, Wang J, Zhu Q, Zhai Z. Circular RNA MYLK serves as an oncogene to promote cancer progression via microRNA-195/cyclin D1 axis in laryngeal squamous cell carcinoma. Biosci Rep. (2019) 39. doi: 10.1042/BSR20190227
76. Zang Y, Li J, Wan B, Tai Y. circRNA circ-CCND1 promotes the proliferation of laryngeal squamous cell carcinoma through elevating CCND1 expression via interacting with HuR and miR-646. J Cell Mol Med. (2020) 24:2423–33. doi: 10.1111/jcmm.14925
77. Bai Y, Hou J, Wang X, Geng L, Jia X, Xiang L, et al. Circ_0000218 plays a carcinogenic role in laryngeal cancer through regulating microRNA-139–3p/Smad3 axis. Pathol Res Pract. (2020) 216:153103. doi: 10.1016/j.prp.2020.153103
78. Gao W, Guo H, Niu M, Zheng X, Zhang Y, Xue X, et al. circPARD3 drives Malignant progression and chemoresistance of laryngeal squamous cell carcinoma by inhibiting autophagy through the PRKCI-Akt-mTOR pathway. Mol Cancer. (2020) 19:166. doi: 10.1186/s12943-020-01279-2
79. Wu Y, Zhang Y, Zheng X, Dai F, Lu Y, Dai L, et al. Correction: Circular RNA circCORO1C promotes laryngeal squamous cell carcinoma progression by modulating the let-7c-5p/PBX3 axis. Mol Cancer. (2023) 22:109. doi: 10.1186/s12943-023-01819-6
80. Wu Y, Zhang Y, Niu M, Shi Y, Liu H, Yang D, et al. Whole-transcriptome analysis of CD133+CD144+ Cancer stem cells derived from human laryngeal squamous cell carcinoma cells. Cell Physiol Biochem: Int J Exp Cell Physiol Biochem Pharmacol. (2018) 47:1696–710. doi: 10.1159/000490992
81. Zhao X, Zhong Q, Cheng X, Wang S, Wu R, Leng X, et al. miR-449c-5p availability is antagonized by circ-NOTCH1 for MYC-induced NOTCH1 upregulation as well as tumor metastasis and stemness in gastric cancer. J Cell Biochem. (2020) 121:4052–63. doi: 10.1002/jcb.29575
82. Chen Z, Xu W, Zhang D, Chu J, Shen S, Ma Y, et al. circCAMSAP1 promotes osteosarcoma progression and metastasis by sponging miR-145–5p and regulating FLI1 expression. Mol Ther Nucleic Acids. (2021) 23:1120–35. doi: 10.1016/j.omtn.2020.12.013
83. Wang Y, Yan Q, Mo Y, Liu Y, Wang Y, Zhang S, et al. Splicing factor derived circular RNA circCAMSAP1 accelerates nasopharyngeal carcinoma tumorigenesis via a SERPINH1/c-Myc positive feedback loop. Mol Cancer. (2022) 21:62. doi: 10.1186/s12943-022-01502-2
84. Liu Q, Shuai M, Xia Y. Knockdown of EBV-encoded circRNA circRPMS1 suppresses nasopharyngeal carcinoma cell proliferation and metastasis through sponging multiple miRNAs. Cancer Manage Res. (2019) 11:8023–31. doi: 10.2147/CMAR
85. Hong X, Liu N, Liang Y, He Q, Yang X, Lei Y, et al. Circular RNA CRIM1 functions as a ceRNA to promote nasopharyngeal carcinoma metastasis and docetaxel chemoresistance through upregulating FOXQ1. Mol Cancer. (2020) 19:33. doi: 10.1186/s12943-020-01149-x
86. Ge J, Wang J, Xiong F, Jiang X, Zhu K, Wang Y, et al. Epstein-Barr virus-encoded circular RNA circBART2.2 promotes immune escape of nasopharyngeal carcinoma by regulating PD-L1. Cancer Res. (2021) 81:5074–88. doi: 10.1158/0008-5472.CAN-20-4321
87. Li Y, Feng W, Kong M, Liu R, Wu A, Shen L, et al. Exosomal circRNAs: A new star in cancer. Life Sci. (2021) 269:119039. doi: 10.1016/j.lfs.2021.119039
88. Zhang F, Jiang J, Qian H, Yan Y, Xu W. Exosomal circRNA: emerging insights into cancer progression and clinical application potential. J Hematol Oncol. (2023) 16:67. doi: 10.1186/s13045-023-01452-2
89. Guo X, Gao C, Yang DH, Li S. Exosomal circular RNAs: A chief culprit in cancer chemotherapy resistance. Drug Resist Updates: Rev Commentaries Antimicrob Anticancer Chemother. (2023) 67:100937. doi: 10.1016/j.drup.2023.100937
Keywords: squamous cell carcinoma, exosomes, circRNA, biomarkers, noninvasive diagnosis
Citation: Wang R, Wang S, Jiang H, Lan Y and Yu S (2024) Prospects for the clinical application of exosomal circular RNA in squamous cell carcinoma. Front. Oncol. 14:1430684. doi: 10.3389/fonc.2024.1430684
Received: 10 May 2024; Accepted: 31 May 2024;
Published: 12 June 2024.
Edited by:
Madhabananda Kar, All India Institute of Medical Sciences Bhubaneswar, IndiaReviewed by:
Kulbhushan Thakur, University of Delhi, IndiaCopyright © 2024 Wang, Wang, Jiang, Lan and Yu. This is an open-access article distributed under the terms of the Creative Commons Attribution License (CC BY). The use, distribution or reproduction in other forums is permitted, provided the original author(s) and the copyright owner(s) are credited and that the original publication in this journal is cited, in accordance with accepted academic practice. No use, distribution or reproduction is permitted which does not comply with these terms.
*Correspondence: Yingmei Lan, NTM1ODMwNjJAcXEuY29t; Shaobin Yu, eXVzaGFvYmluaHhAd2Noc2N1LmVkdS5jbg==