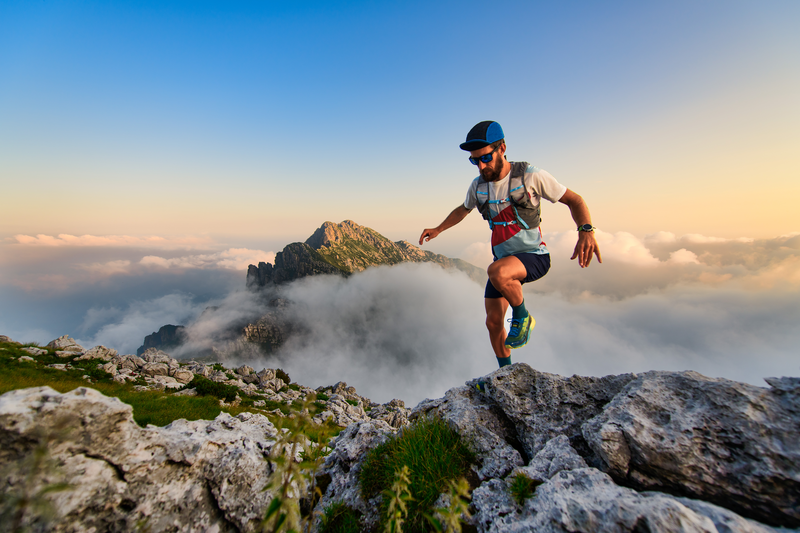
94% of researchers rate our articles as excellent or good
Learn more about the work of our research integrity team to safeguard the quality of each article we publish.
Find out more
CASE REPORT article
Front. Oncol. , 09 September 2024
Sec. Hematologic Malignancies
Volume 14 - 2024 | https://doi.org/10.3389/fonc.2024.1420666
This article is part of the Research Topic MDS: New Scientific and Clinical Developments View all 14 articles
Introduction: Inherited bone marrow failure (IBMF) syndromes are caused by mutations forming pathologic germline variants resulting in the production of defective hematopoietic stem cells (HSC) and in congenital failure in the production of one or more blood lineages. An acquisition of subsequent somatic mutations is determining further course of the disease. Nevertheless, a certain number of patients with IBMF may escape correct diagnosis in childhood, especially those with mild cytopenia and minimal clinical features without non-hematologic symptoms. These patients usually present in the third decade of life with unexplained cytopenia or myelodysplastic syndrome (MDS).
Methods and results: We report 2 patients with IBMF who were correctly diagnosed between 20 and 40 years of age when they were referred with progressive MDS with adverse prognostic factors that affected their outcome.
Discussion: IBMF syndromes should be excluded in all patients below 40 years of age with unexplained cytopenia. Early hematopoietic stem cell transplantation (HSCT) is the treatment of choice in these patients.
Inherited bone marrow failure (IBMF) syndromes are caused by mutations forming pathological germline variants. The result is a production of defective hematopoietic stem cells (HSCs) and stressed hematopoiesis (1). The pathogenesis of HSC progenitor cells failure depends on the type of the lesion caused by patient’s specific germline variant. The lesions may result in defective DNA cross-link mutation repair [Fanconi anemia (FA)], defective telomere maintenance [dyskeratosis congenita (DC)], ribosomopathies [Shwachman Diamond syndrome (SDS) and Diamond Blackfan anemia (DBA)], defect of terminal maturation (congenital neutropenia), defects of signaling receptors, cell growth regulation, and cell transcription (2). A germline genetic defect is responsible for an activation of DNA damage response. Less severe and potentially repairable damage activates cell senescence and cell growth arrest via p53-mediated pathway (3). An extensive DNA damage results in excessive p53-induced pathway. Both decreased stem cell fitness and stem cell depletion lead to cytopenia and marrow failure (2). Subsequent acquisition of somatic mutations is crucial for the development of an MDS clone. These somatic alterations may have an adaptive outcome resulting from the correction of germline phenotype or maladaptive outcome connected with an increased risk of neoplastic clonal evolution (2). The risk of development of myelodysplastic syndromes (MDSs) or myeloproliferative neoplasms (MNs) is from 40- to 7,700-fold higher than in normal population (4). IBMF is commonly diagnosed during childhood. However, some patients with discrete symptoms may escape an early diagnosis and present as young adults with unexplained cytopenia or MDS. Here, we report two patients with IBMF who were correctly diagnosed between 20 years and 40 years of age when they were referred to our department with the diagnosis of MDS.
The patient was followed at the regional department for sick children since the age of 4 years with mild cytopenia, growth retardation, repeated infections, and gut discomfort. The patient was consulted with Clinics for Pediatric Hematology, and SDS was hypothesized as one of the possible reasons of cytopenia. However, this suspicion was never confirmed, and a final diagnosis of MDS RCC (refractory childhood cytopenia) was established. At that time, his peripheral blood counts were as follows: Hb, 113 g/L; WBC, 2.66 × 109/L; NS, 35%; PLT, 70 × 109/L. Bone marrow was described as “slightly hypocellular without marked signs of dysplasia”. As an adult, the patient was followed at the regional hematology department. He suffered from recurrent pulmonary infections without substantial worsening of the cytopenia. He required the first red blood cell (RBC) transfusion at the age of 36. At that time, he was referred sent to the Institute of Hematology in Prague because of progressive cytopenia with dg. MDS RCMD (refractory cytopenia with multilineage dysplasia) and del(5q). The patient’s height was 146 cm with skeletal abnormalities of chest and spine (Figure 1). Peripheral blood counts and results of bone marrow investigation are summarized in Table 1. Investigation of SBDS gene revealed biallelic mutation within exon 2: c.183-184TA>CT. The diagnosis of MDS with excess of blasts II. (IB2) developed from SDS was established. The patient had multiple karyotype abnormalities and mutations of driver genes, including a biallelic mutation of TP53. His IPSS-R score was 8.5 (5).
Table 1. Peripheral blood and bone marrow findings in SDS patient (Hb, hemoglobin; RBC, red blood cells; RTC, reticulocytes; WBC, white blood cells; NS, neutrophil segments; PLT, platelets; NGS, next-generation sequencing).
The patient underwent combination chemotherapy (Daunorubicin 90 mg day 1–3 + Cytarabine 150 mg day 1–7). Bone marrow aspiration performed on day (D) 21 of induction chemotherapy revealed hypoplastic bone marrow with 11% of blasts, and the patient underwent immediately allogeneic peripheral blood stem cell transplantation (PBSCT) from HLA-matched unrelated donor using conditioning regimen Fludarabine + Melphalan + antithymocyte globulin (ATG). He was engrafted on D 23 after SCT in morphological remission, but within 3 months, he had mixed chimerism again despite repeated donor lymphocyte infusions. Nonetheless, he was relatively quite well with skin and liver chronic graft versus host disease (GVHD) grade II until D 485 after PBSCT when he relapsed. Best supportive care only was indicated because of his poor performance status. The patient died D 510 after PBSCT from sepsis and multiple organ failure.
At the age of 6 years, this patient underwent surgical removal of an abundant thumb on the right hand. Preoperative investigation of peripheral blood counts revealed macrocytosis (MCV=107 fl) and slightly decreased PLT count (91 × 109/L). The patient was asymptomatic and was infrequently followed by a regional pediatrician. At the age of 17, the patient was referred to Clinics for Pediatric Hematology with pancytopenia and macrocytosis (Hb= 105 g/L, MCV = 107 fl, WBC = 2.4 × 109/L, PLT = 71 × 109/L). A chromosome fragility assay showed an increased rate of chromosomal breaks [5% spontaneous, 8% with diepoxybutane (DEB)]. The patient was referred to the Institute of Hematology with suspected FA, but she canceled a planned appointment and disappeared for 2 years. She returned at the age of 20 with fatigue, dyspnea, and recurrent infections. Peripheral blood counts and results of bone marrow investigation are summarized in Table 2. Investigation of FANCA gene revealed biallelic mutation c.3788-3490 (del TcT).
Table 2. Peripheral blood and bone marrow findings in FA patient (Hb, hemoglobin; RBC, red blood cells; RTC, reticulocytes; WBC, white blood cells; NS, neutrophil segments; PLT, platelets; NGS, next generation sequencing).
The diagnosis of MDS with excess of blasts II. (IB2) progressing from FA was established. The patient had deleted MLL gene and RUNX1 gene mutation; her IPSS-R score was 5 (5). She underwent upfront allogeneic PBSCT from HLA-matched related donor with reduced conditioning (Fludarabine + Cyclophosphamide + ATG). Engraftment was achieved on D 35 after SCT. The patient did not have any complications after SCT except mild acute GVHD in gut. She is currently 8 years after SCT and doing well.
An acquisition of somatic mutations determines the subsequent course of the disease in IBMT patients. Correction of the germline defect can be mediated via gene reversion or back mutation, deletion of point mutation, or by second site mutation suppressing the effect of the original mutation (1, 6). Compensation of the germline defect indirectly by somatic alteration of other genes reduces impact of the germline defect, e.g., acquisition of i[7q] and del20q in SDS (7). On the other hand, somatic alteration directly in p53 gene or in a gene silencing p53 effectivity may allow increased cell senescence. For example, chromosome 1 gain with trisomy of MDM4 gene downregulates p53 signaling pathway (7). Clonal somatic changes may also increase cell vulnerability to acquire further genetic alterations, especially mutations of the so-called driver genes, which play an important role in the regulation of proliferation, differentiation, and cell apoptosis (8, 9). The most frequently acquired cytogenetic clones are 20q−, i[7q] in SDS, and 1q+, 3q+, and −7/7q− in FA. Complex cytogenetic aberrations, monosomy 7, and 3q+ represent high-risk changes. Most common somatic gene mutations are TP53, EIF6, RUNX1, UZAF1, BCOR, STAG2, ASXL1, NRAS/KRAS, and SETBP1 mutations. Biallelic TP53 alterations, RUNX1, SETB1, ASXL1, and U2AF1 gene mutations are now considered to be high-risk mutations. Progression towards MDS/AML has been described in 30%–40% patients aged 30–40 years with SDS, FA, or GATA-2 mutations (10). SCT is the only curative treatment, and 10 years survival is approximately 75% according to the EBMT study (11). In a retrospective study of transplanted patients below 40 years of age, IBMF preceded development of MDS in 4%–7% of patients with SDS (12) and in 10%–15% patients with aplastic anemia (10).
Shwachman–Diamond syndrome (SDS) is an inherited ribosomopathy characterized by bone marrow failure, exocrine pancreatic deficiency, and skeletal dysplasia (osteopenia, short flared ribs, delayed ossification, and low height). In 90% of patients with SDS, a biallelic mutation of the SBDS gene leads to impaired joining of 60S and 40S ribosome subunits. This results in impaired translational capacity and upregulation of TP53 activity and senescence and apoptosis of hematopoietic stem cells (HSCs). Subsequent recurrent mutations of the EIF6 gene may alleviate the blocking effect of eIF6 on subunits joining and correct to some extent the germline defect similarly as does the acquisition of i[7q] and del20q (13). On the other hand, patients with SDS have an increased risk of developing myeloid malignancies and clonal hematopoiesis caused by mutations bypassing or alleviating intrinsic fitness caused by SBDS mutation. TP53 mutations are generally missense, and TP53 inactivation is associated with increased leukemogenic potential (14). Isochromosome 7q and del20q are the most recurrent cytogenetic abnormalities, where i[7q] results in duplication of SBDS gene. However, an adverse prognosis is connected with monosomy 7 and del(7q) (10). In the French SDS Registry (15), the incidence of myeloid malignancy was 24.3% and 36% at the age of 20 years and 30 years, respectively. In patients who developed leukemic progression, the prognosis is poor, with an analysis of the North American Registry reporting median survival of 7.7 years and 1 year for MDS and AML, respectively (16). Allogeneic hematopoietic stem cell transplantation (HSCT) is the only potential curative therapy for IBMF. It has been shown that the results of transplantation performed prior to development of MDS were significantly better than those performed during leukemic progression (5-year overall survival of 70.7% vs. 28.8%, respectively) (13, 16). There was no difference in results between upfront HSCT and HSCT after cytoreductive chemotherapy. The experience with bridging therapy using hypomethylating agents (HMAs) before SCT is limited (13).
The problem in the case of our patient was an insufficient diagnostic workup in childhood and the insistence on diagnosis of MDS with 5q deletion, whereby no further follow-up karyotypes and NGS were performed. Del5q has rarely been described in SDS patients; however, no systematic functional analyses have confirmed a causal link between haplo-insufficiency of a single gene and development of clonal advantage as in 5q− syndrome (17). The presence of a high load of biallelic TP53 mutation contributes to a poor prognosis even in transplanted SDS patients as seen together with multiple karyotype abnormalities in our patient. Mutations of DNMT3A and ASXL1 driver genes have also been described in several SDS patients (6.7% of patients in the study by Kennedy et al.) (7).
Fanconi anemia (FA) is an IBMF with DNA instability caused by biallelic mutations in one of 22 FANC pathway genes that are involved in DNA damage repair and stress response (18). Activation of FANC pathway forms “FA core” multiprotein complex that activates D2/I heterodimer, which interacts with downstream proteins including BRCA2. The result is a replication-coupled excision of the damaged locus followed by homologous recombination. FA patients have usually normal blood counts at birth, and the first signs of bone marrow failure (macrocytic anemia and thrombocytopenia) occurs within the first decade of life (median age of onset is 7 years). Approximately 80% of FA patients will develop bone marrow failure by the age of 20. Skeletal malformations include abnormal thumbs, absent radii, short stature, skin hyperpigmentation, and abnormal facial features. Approximately 30% of FA patients do not have any of the classic physical findings, but the diepoxybutane (DEB) chromosome fragility assay showing increased chromosomal breaks could be diagnostic (19). One-half of the FA patients may develop gain of chromosome 1q driving enforced MDM4 oncogene expression that leads to repression of p53 response. p53 attenuation confers an advantage to the 1q+ clone that may expand, repopulate exhausted bone marrow, and form a base for acquisition of additional driver events: RUNX1 inactivation, 3q+/EVI1 mutation, 7q deletion, and finally, development of leukemic progression. Patients with FA have increased risk of hematologic malignancies, head and neck squamous cell carcinoma, gynecological cancers, and other solid tumors. The estimated incidence of AML in FA patients is 15%–20% in 40 years, and the cumulative incidence of MDS and solid tumors is 40% and 16% in 50 years, respectively (20). HSCT is also a potential curative treatment in FA patients, but the indication must be considered carefully. Combination chemotherapy and radiation including conditioning regimens are associated with excessive toxicity and an increased rate of solid tumors after HSCT. An optimal time for indication of HSCT is progressive cytopenia before development of adverse cytogenetic aberrations and gene mutations. The 3-year survival of FA patients transplanted following reduced conditioning from HLA-matched related donor is more than 90% (20). The problems in the history of the disease in our FA patient were insufficient diagnosis with the lack of NGS investigation and poor cooperation of the patient. Progressive cytopenia, increased percentage of blasts, and the presence of the RUNX1 gene mutation were adverse factors that forced us to indicate an immediate HSCT in the patient. On the other hand, the availability of HLA-matched related donor and only mild excess of bone marrow (BM) blasts enabled us to use reduced conditioning and avoid toxic cytoreductive treatment. These factors clearly played an important role in the outcome of transplantation.
IBMF syndromes are characterized by congenital failure in the production of one or more blood lineages. The clinical manifestations of the IBMF may vary according to the type and number of blood cell lines involved. In some IBMF syndromes, systemic non-hematologic manifestations, including congenital malformations and impaired development, may be present. Despite these features, a certain number of patients with IBMF may escape correct diagnosis, especially those with mild cytopenia and minimal clinical features without non-hematologic symptoms. The need for regular follow-up of these patients is not usually stressed sufficiently. IBMF syndromes represent a qualitatively impaired functional state and a basis for somatic alterations. This process can promote clonal hematopoiesis by improving the competitive fitness of specific hematopoietic stem cell clones. All children with unexplained anemia or cytopenia should by investigated for the presence of IBMF. Similarly, all adolescents with suspected MDS should be tested for underlying IBMF. All patients with IBMF should undergo serial assessment of peripheral blood and bone marrow including cytogenetics and NGS. Progressive cytopenia, development of MDS and increased percentage of bone marrow blasts, abnormal karyotype (3q+, −7,/7q, complex karyotype), RUNX1, and biallelic TP53 mutations are factors that identify risk patients and support the early indication of HSCT in IBMF (1, 6).
The original contributions presented in the study are included in the article/supplementary material. Further inquiries can be directed to the corresponding author.
Written informed consent was obtained from the individual(s) for the publication of any potentially identifiable images or data included in this article.
JC: Writing – original draft, Writing – review & editing.
The author(s) declare that no financial support was received for the research, authorship, and/or publication of this article.
The author thanks colleagues from the Department of Clinical Hematology and Department of Molecular Genetics and Cytogenetics of EuroBloodNet Center at the Institute of Hematology and Blood Transfusion in Prague and physicians from Clinics for Hematology and Oncology for children in Prague for their help with collecting the data.
The author declares that the research was conducted in the absence of any commercial or financial relationships that could be construed as a potential conflict of interest.
All claims expressed in this article are solely those of the authors and do not necessarily represent those of their affiliated organizations, or those of the publisher, the editors and the reviewers. Any product that may be evaluated in this article, or claim that may be made by its manufacturer, is not guaranteed or endorsed by the publisher.
1. Chojilsuren HB, Park Y, Jung M. Mechanisms of of somatic transformation in inherited bone marrow failure syndromes. Am.Soc.Hematol Educ.Program; Hematol. (2021), 390–8. doi: 10.1182/hematology.2021000271
2. Elgethany MT, Patnaik MM, Khoury JD. Myelodysplastic neoplasms evolving from inherited bone marrow syndromes / germline predisposition syndromes: Back under the microscope. Leukemia Res. (2024) 134:1–7. doi: 10.1016/j.leukres.2024.107441
3. Capuozzo M, Santarsola M, Bocchetti M, Perri F, Cascella M, Granata V, et al. p53 from fundamental biology to clinical applications in cancer. Biology. (2022) 11:1325. doi: 10.3390/biology11091325
4. Cada M, Segbefia CI, Klassen R, Fernandez CV, Yanofsky RA, Wu J, et al. The impact of category, cytopathology and cytogenetics on development and progression of Malignant myeloid transformation in inherited bone marrow failure syndromes. Haematologica. (2015) 100:632–42. doi: 10.3324/haematol.2014.117457
5. Greenberg PL, Tuechler H, Schanz J, Sanz G, Garcia-Manero G, Sole F, et al. Revised international prognostic scoring system for myelodysplastic syndromes. Blood. (2012) 120:2454–65. doi: 10.1182/blood-2012-03-420489
6. Tsai FD, Lindsley RC. Clonal hematopoiesis in the inherited bone marrow failure syndromes. Blood. (2020) 136:1615–22. doi: 10.1182/blood.2019000990
7. Kennedy AN, Myers KC, Bowman J, Gibson DJ, Camerda ND, Furutani E, et al. Distinct genetic pathways define pre-malignant versus compensatory clonal hematopoiesis in Shwachman-Diamond syndrome. Nat.Commun. (2021) 12:1334–49. doi: 10.1038/s41467-021-21588-4
8. Papaemmanuil E, Gerstung M, Malcovati L, Tauro S, Gunden G, Van Loo P, et al. Clinical and biological implications of driver mutations in myelodysplastic syndromes. Blood. (2013) 122:3616–27. doi: 10.1182/blood-2013-08-518886
9. Malcovati L, Galli A, Travaglino E, Rizzo C, Motteni E, Elena C, et al. Clinical significance of somatic mutation in unexplained blood cytopenia. Blood. (2017) 129:3371–8. doi: 10.1182/blood-2017-01-763425
10. Park M. Overview of inherited bone marrow failure syndromes. Blood Res. (2022) 57:S49–54. doi: 10.5045/br.2022.2022012
11. Bonfim C. Special pre- and posttransplant considerations in inherited bone marrow failure and hematopoietic Malignancy predisposition syndromes. Hematol Am Soc Hematol Educ Program: Hematol. (2020), 107–14. doi: 10.1182/hematology.2020000095
12. Lindsley RC, Saber W, Mar BG, Redd R, Wang T, Haagenson MD, et al. Prognostic Mutations in Myelodysplastic syndromes after Stem-Cell Transplantation. N Engl J Med. (2017) 376:536–47. doi: 10.1056/NEJMoa1611604
13. Reilly CR, Shimamura A. Predisposition to myeloid Malignancies in Shwachman-Diamond syndrome: biological insights and clinical advances. Blood. (2023) 141:1513–23. doi: 10.1182/blood.2022017739
14. Donadieu J, Delhommeau F. TP53 mutations: the dawn of Shwachman clones. Blood. (2018) 131:376–7. doi: 10.1182/blood-2017-11-815431
15. Donadieu J, Fenneteau O, Beaupain B, Beaufils S, Bellanger F, Mahlaoui N, et al. Classification of and risk factors for hematologic complications in a French national cohort of 102 patients with Shwachman-Diamond syndrome. Haematologica. (2012) 97:1312–9. doi: 10.3324/haematol.2011.057489
16. Myers KC, Furutani E, Weller E, Siegele B, Galvin A, Arsenault V, et al. Clinical features and outcomes of patients with Shwachman-Diamond syndrome and myelodysplastic syndrome or acute myeloid leukemia: a multicentre, restrospective, cohort study. Lancet Haematol. (2020) 7:e238–46. doi: 10.1016/S2352-3026(19)30206-6
17. Burwick N, Shimamura A, Johnson ML. Non-Diamond blackfan anemia disorders of ribosome function : shwachman diamond syndrome and 5q-Syndrome. Semin Hematol. (2011) 48:136–43. doi: 10.1053/j.seminhematol.2011.01.002
18. Ceccaldi R, Parmar K, Mouly E, Delord M, Kim JM, Regairaz M, et al. Bone marrow failure in Fanconi anemia is triggered by an exacerbated p53/p21 DNA damage response that impairs hematopoietic stem and progenitor. Cell Stem Cell. (2012) 11:36–49. doi: 10.1016/j.stem.2012.05.013
19. Shimamura A. Aplastic anemia and clonal evolution: germ line and somatic genetics. Am Soc Hematol Educ Program; Hematol. (2016), 74–82. doi: 10.1182/asheducation-2016.1.74
Keywords: inherited bone marrow failure, cytopenia, diagnosis, treatment, myelodysplastic syndromes, transplantation
Citation: Cermak J (2024) Case report: Development of clonal hematologic disorders from inherited bone marrow failure. Front. Oncol. 14:1420666. doi: 10.3389/fonc.2024.1420666
Received: 20 April 2024; Accepted: 08 July 2024;
Published: 09 September 2024.
Edited by:
Argiris Symeonidis, University of Patras, GreeceReviewed by:
Jose Manuel Vagace Valero, University Hospital of Badajoz, SpainCopyright © 2024 Cermak. This is an open-access article distributed under the terms of the Creative Commons Attribution License (CC BY). The use, distribution or reproduction in other forums is permitted, provided the original author(s) and the copyright owner(s) are credited and that the original publication in this journal is cited, in accordance with accepted academic practice. No use, distribution or reproduction is permitted which does not comply with these terms.
*Correspondence: Jaroslav Cermak, Y2VybWFrQHVoa3QuY3o=
Disclaimer: All claims expressed in this article are solely those of the authors and do not necessarily represent those of their affiliated organizations, or those of the publisher, the editors and the reviewers. Any product that may be evaluated in this article or claim that may be made by its manufacturer is not guaranteed or endorsed by the publisher.
Research integrity at Frontiers
Learn more about the work of our research integrity team to safeguard the quality of each article we publish.