- 1Division of Anatomy, Department of Human Morphology and Embryology, Faculty of Medicine, Wroclaw Medical University, Wroclaw, Poland
- 2Fertility, Infertility and Perinatology Research Center, Ahvaz Jundishapur University of Medical Sciences, Ahvaz, Iran
- 3Clinical Research Development Unit, Imam Khomeini Hospital, Ahvaz Jundishapur University of Medical Sciences, Ahvaz, Iran
- 4Hematology and Oncology Research Center, Tabriz University of Medical Sciences, Tabriz, Iran
- 5Department of Obstetrics and Gynecology, School of Medicine, Ahvaz Jundishapur University of Medical Sciences, Ahvaz, Iran
- 6Department of Obstetrics and Gynecology, School of Medicine, Hamadan University of Medical Sciences, Hamadan, Iran
- 7Department of Veterinary Surgery, Institute of Veterinary Medicine, Nicolaus Copernicus University in Torun, Torun, Poland
- 8Physiology Graduate Program, North Carolina State University, Raleigh, NC, United States
- 9Division of Histology and Embryology, Department of Human Morphology and Embryology, Faculty of Medicine, Wroclaw Medical University, Wroclaw, Poland
- 10Department of Obstetrics and Gynecology, University Hospital and Masaryk University, Brno, Czechia
Endometrial cancer (EC) is one of the most common gynecologic cancers. In recent years, research has focused on the genetic characteristics of the tumors to detail their prognosis and tailor therapy. In the case of EC, genetic mutations have been shown to underlie their formation. It is very important to know the mechanisms of EC formation related to mutations induced by estrogen, among other things. Noncoding RNAs (ncRNAs), composed of nucleotide transcripts with very low protein-coding capacity, are proving to be important. Their expression patterns in many malignancies can inhibit tumor formation and progression. They also regulate protein coding at the epigenetic, transcriptional, and posttranscriptional levels. MicroRNAs (miRNAs), several varieties of which are associated with normal endometrium as well as its tumor, also play a particularly important role in gene expression. MiRNAs and long noncoding RNAs (lncRNAs) affect many pathways in EC tissues and play important roles in cancer development, invasion, and metastasis, as well as resistance to anticancer drugs through mechanisms such as suppression of apoptosis and progression of cancer stem cells. It is also worth noting that miRNAs are highly precise, sensitive, and robust, making them potential markers for diagnosing gynecologic cancers and their progression. Unfortunately, as the incidence of EC increases, treatment becomes challenging and is limited to invasive tools. The prospect of using microRNAs as potential candidates for diagnostic and therapeutic use in EC seems promising. Exosomes are extracellular vesicles that are released from many types of cells, including cancer cells. They contain proteins, DNA, and various types of RNA, such as miRNAs. The noncoding RNA components of exosomes vary widely, depending on the physiology of the tumor tissue and the cells from which they originate. Exosomes contain both DNA and RNA and have communication functions between cells. Exosomal miRNAs mediate communication between EC cells, tumor-associated fibroblasts (CAFs), and tumor-associated macrophages (TAMs) and play a key role in tumor cell proliferation and tumor microenvironment formation. Oncogenes carried by tumor exosomes induce malignant transformation of target cells. During the synthesis of exosomes, various factors, such as genetic and proteomic data are upregulated. Thus, they are considered an interesting therapeutic target for the diagnosis and prognosis of endometrial cancer by analyzing biomarkers contained in exosomes. Expression of miRNAs, particularly miR-15a-5p, was elevated in exosomes derived from the plasma of EC patients. This may suggest the important utility of this biomarker in the diagnosis of EC. In recent years, researchers have become interested in the topic of prognostic markers for EC, as there are still too few identified markers to support the limited treatment of endometrial cancer. Further research into the effects of ncRNAs and exosomes on EC may allow for cancer treatment breakthroughs.
1 Introduction
Endometrial cancer (EC) is one of the most common types of malignancies in women worldwide, and also the most common gynecologic cancer. According to Sung et al. (1), there were an estimated 420,000 new cases in 2021, including 97,000 deaths. In Europe, its incidence in 2021 was about 79 cases per 100,000 women (2). According to recent reports, 66,000 new cases and more than 13,000 deaths from endometrial cancer were detected in the USA in 2023, 83% of which were endometrial cancers (3). This type of cancer is divided, according to Bokhmnan’s dualistic theory, into two main clinicopathological and molecular types. Type I is endometrial adenocarcinoma, and type II includes nonendometrial subtypes such as serous, clear cell, or undifferentiated carcinoma (4). Despite early diagnosis in most patients, differences in the characteristics and histopathological features of the disease affect the prognosis as well as the recommended treatment. It also depends on the stage of the tumor (5). The most commonly used FIGO classification assigns cancer progression to four stages (6), shown in Figure 1. In recent years, research has focused on the genetic characterization of cancers to better determine their prognosis and personalize therapy. EC is one of the major cancers in which genetic mutations and epigenetic modifications have been found to underlie their development due to the increasing prevalence of the disease in the population (2). After a systematic review of EC prognostic markers, it was concluded that the lack of identification of specific markers is one of the main reasons for the absence of more clinical trials on EC (7). There is an urgent need to develop alternative biomarkers of EC in the blood to enable timely diagnosis for clinical use. Emerging blood biomarker tests are showing promise in cancer diagnosis, focusing on genes, proteins, and metabolites (8). Metabolic biomarkers, in particular, seem very promising due to a better picture of the genotype than gene and protein markers (9). However, current metabolite biomarkers in blood remain limited, and a panel of metabolic biomarkers needs to be constructed based on advanced analytical techniques (10). One promising marker may be phosphatase and tensin homolog deleted on chromosome ten (PTEN). It is a tumor growth suppressor that affects growth regulation and cell survival (11). Eritja et al. (12) conducted research on the mechanisms by which PTEN deficiency affects the development of endometrial cancer. Thus, it can be used as an immunohistochemical marker of EC. It has been suggested that cancer stem cells play an important role in the development of endometrial cancer because their presence is associated with resistance to chemotherapy. Various markers have been identified on the surface of these cells, such as CD40, CD44, or CD133, and their expression explains the increased carcinogenicity. However, research in this area is being further pursued, as there is a lack of strong evidence indicating their use as universal markers of EC stem cells (13).
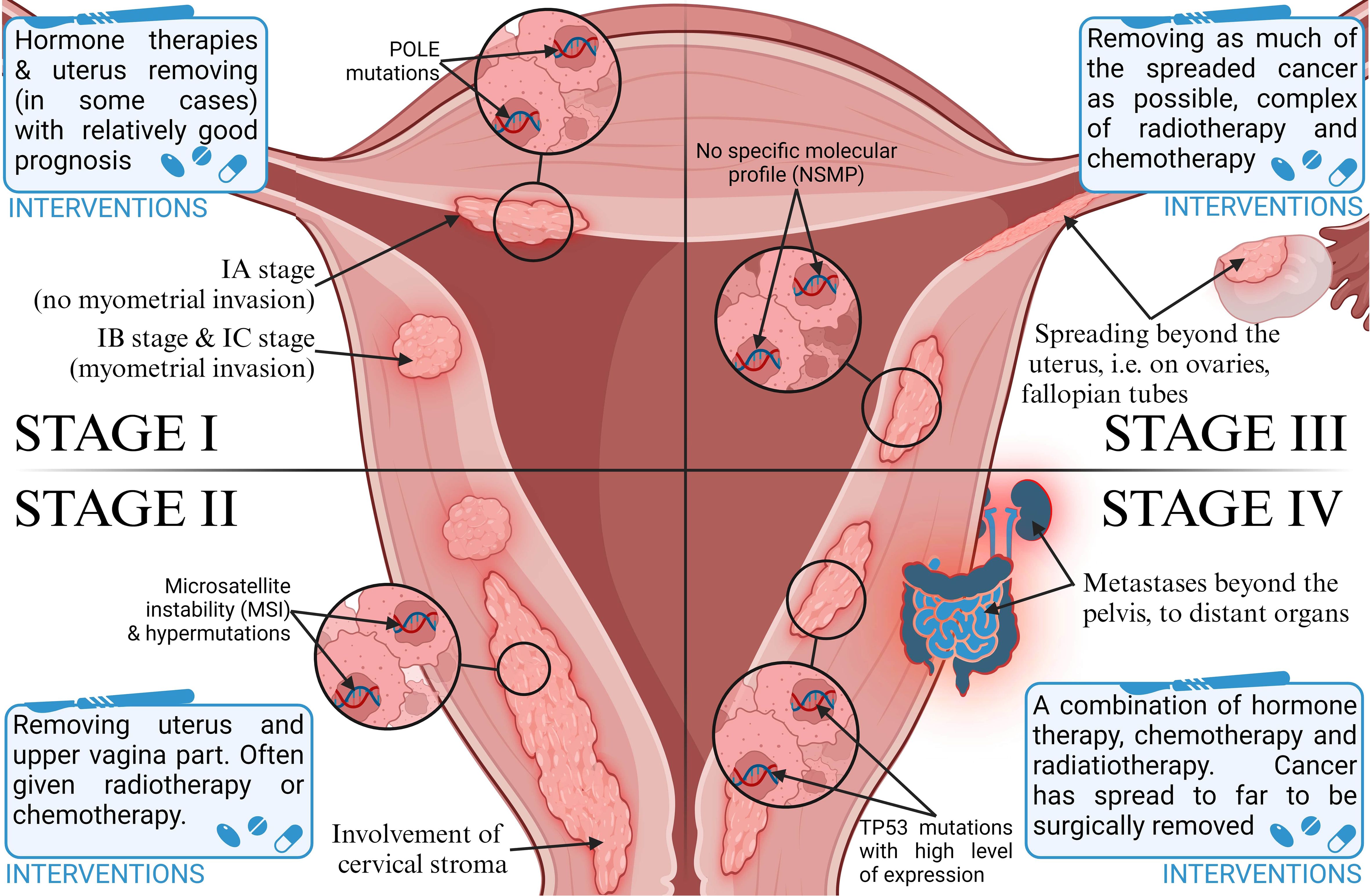
Figure 1. Classification of stages of endometrial cancer progression and therapeutic intervention strategies.
Noncoding RNAs (ncRNAs) consist of nucleotide transcripts with very little or no ability to code for proteins. Their expression patterns in many malignancies include changes that can promote or inhibit tumor formation and cancer progression. They may also regulate organic protein-coding phenomena at the epigenetic, transcriptional, posttranscriptional, and alternative levels (2). NcRNAs play a key role not only in cellular processes but also in the pathogenesis of human diseases. They also include long noncoding RNAs (lncRNAs), microRNAs (miRNAs), and circular RNAs (circRNAs). Dysregulation of the expression of various ncRNAs and the associated competing endogenous RNA network has been found to be involved in the genesis and development of various malignancies, including EC (14). Considerable interest has been aroused by the recently discovered group of lncRNAs, which have more than 200 nucleotides. Due to their significant presence in the human genome, as well as their tissue-specific expression patterns and importance in the context of physiological and pathological processes, their length allows them to form three-dimensional structures, presumably to determine specific interactions of lncRNAs with biomolecules such as transcription factors, histones, or other chromatin-modifying proteins (15). Another of the ncRNA representatives is miRNAs. This is a specialized class of noncoding RNAs with a small size of 20 to 25 nucleotides. They regulate gene expression as well as various biological processes, such as tumor progression or self-renewal (16). The development of endometrial receptivity in embryo implantation, or endometriosis, and EC as miRNA-related factors are important gene regulators in different parts of the human body. Key species differences in endometrial remodeling make it important to remember that discoveries made in mice do not automatically apply to humans. However, the sensitivity of the endometrium in humans is quite similar to mice. There are 29 miRNAs in humans and 15 miRNAs in mice. In particular, ncRNA molecules regulating Wnt signaling were revealed, as well as those in the let-7, miR-23, miR-200, and miR-183 groups. However, future studies require further investigation into the clinical significance of these miRNAs (17). Some tumor suppressors present in mice, such as phosphatase and tensin homologs, have been well studied in ECs, while another endoribonuclease responsible for miRNA genesis (DICER1) has not been investigated. These molecules were thought to play a key role in the development and progression of EC (18).
Exosomes are round, bilayered extracellular vesicles released from many cell types, both by normal and diseased cells. Notably, cancer cells release more exosomes compared to healthy cells (19). Tumor-derived exosomes contribute to proliferation, inflammation, drug resistance, metastasis, tumor formation, and immune response. They also contain bioactive compounds such as proteins, single-stranded DNA, and genomic DNA, as well as various types of RNAs, lncRNAs, and miRNAs. Exosomal miRNAs modulate the expression of oncogenes and activate suppressors in cancer cells. Their alterations also act as a critical modulator of carcinogenesis. Moreover, oncogenes carried by circulating tumor exosomes induce malignant transformation of target cells over long distances (20). By transporting proteins, lipids, and miRNAs, exosomes show great potential to play important roles in intercellular communication. They may have significant use as tumor markers. The delivery of exosomes and exosomal miRNA has been shown to inhibit tumor progression in mice, which may signify a promising research direction in the context of human cancer (21).
2 Endometrial cancer—epidemiology
EC, or cancer of the corpus uteri, is developed in the mucous membrane of the corpus uteri. Women aged 60 or older are affected, with mortality peak in 70 to 80 years. EC is the most common gynecological cancer in developed countries (22). In recent years, the incidence of endometrial cancer has been gradually increasing due to improved socioeconomic levels, accelerated aging of the population, and an increase in obesity and metabolic syndrome (1). According to the WHO, the worldwide incidence of endometrial cancer is 420,368 women, while the mortality rate is 97,723 women, which places it 15th among the most common cancers worldwide (23). There were circa 100,000 newly diagnosed cases in Europe every year, with an incidence of 13–20/100,000 and a low mortality rate of 2–2.7/100,000 women (24, 25). EC is the fourth (after breast, lung, and large intestine) cancer in women in Poland; it is diagnosed in circa 6,000 women, and each year more than 1,500 patients die. The morbidity and mortality ratio is continually rising, as shown in Figure 2 (26), which occurs for unknown reasons.
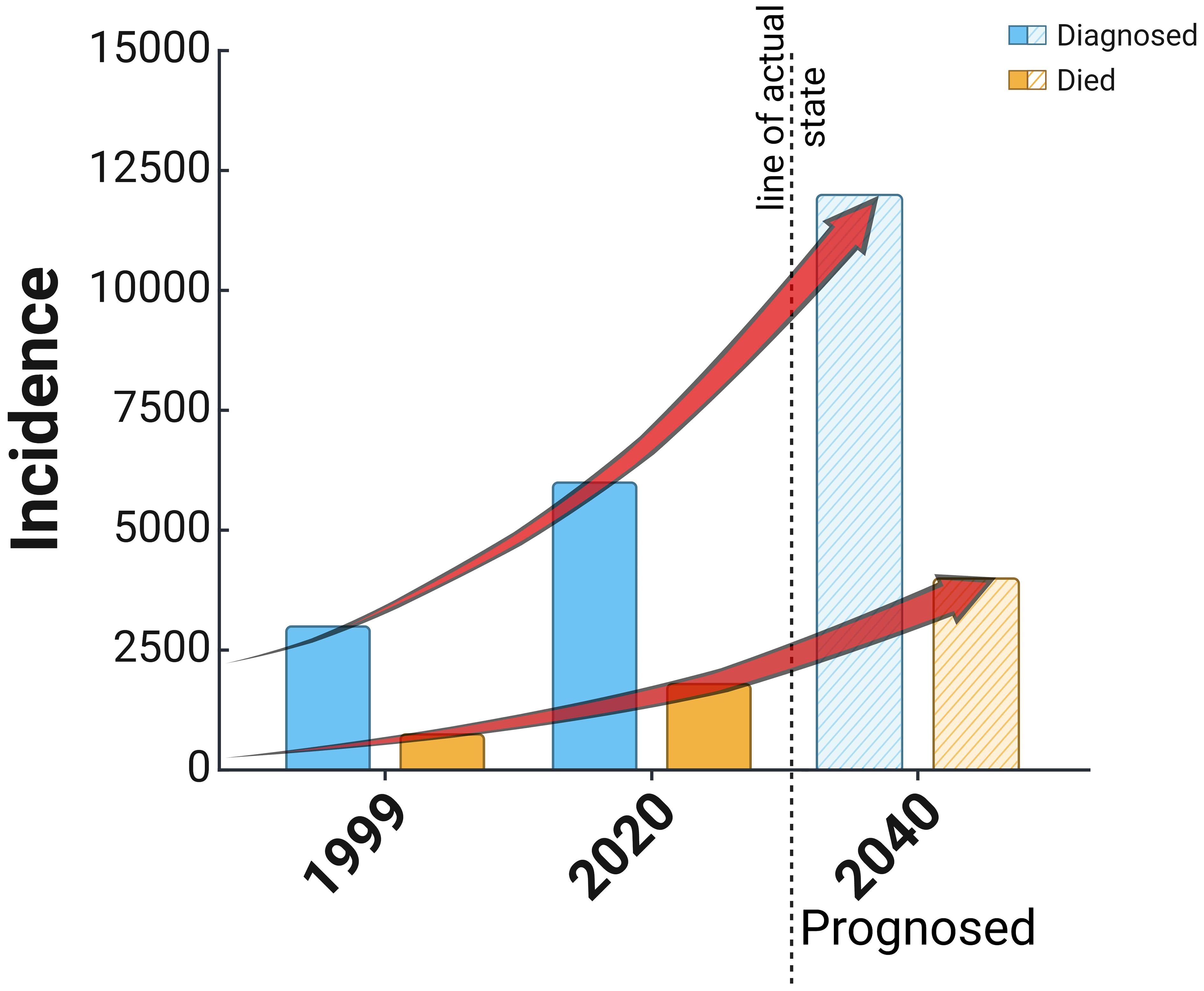
Figure 2. Reported cases of diagnosis and death in the cause of endometrial cancer in Poland since 1999 and a prognosis based on the actual trend of increasing cases of the disease.
There are several recognized EC risk factors, among them increased body mass index (BMI). For BMI 22–27.2, the increased incidence is +21%; for BMI 27.5–29.5, it is +43%; and for BMI more than 30, it is +273%. The remaining factors are age after 50–60, hypertension, hyperinsulinemia (or insulin resistance), and prolonged exposure to estrogen (often in infertility, nulliparity, polycystic ovarian syndrome, tamoxifen intake, early menarche, and late last menstruation). In very rare congenital syndromes such as Lynch or Cowden, the risk of EC is higher. The EC risk is lower in women having children, physical activity, a high-fat diet preventing obesity, contraceptive therapy (estrogens and androgens), intrauterine devices, and coffee (22, 25, 27).
There were initially two subtypes of endometrial cancer based on histopathology. The endometroid adenocarcinoma was classified as type I, and all other cancers (serous, clear cell, carcinosarcoma, and undifferentiated) were type II (4). The first type was related to good and the second type with poor prognosis. In the 1st type, there was an atypical endometrium hyperplasia, which is considered a precancerous state—in which 25% of cancer could develop. There were no precancerous states in the second type of cancer. Tumors are graded according to the International Federation of Gynecology and Obstetrics criteria as grades 1 (G1) and 2 (G2) as low grade and grade 3 (G3) as high grade (28). Several factors have been identified as recurrent risk: G3, at least 50% of myometrium invasion, histological pattern, lymphovascular space invasion, lymph node metastases, and tumors bigger than 2 cm (25).
Classification based on histopathology has been replaced by a system based on molecular phenotypes. More than 90% of ECs are sporadic; the rest are hereditary, most of them related to nonpolyposis colorectal cancer syndrome (HNPCC) or Lynch syndrome. The HNPCC patients have an increased EC developing risk of circa 10-fold, except for the possibility of colon or ovarian cancer. These are usually microsatellite-unstable tumors observed in younger women (29).
3 Current preclinical and clinical research on endometrial cancer
EC preclinical studies play a pivotal role in providing general information about the impact of the tested drug, dose, or therapy on a living organism. Several drugs have been produced for cancer research using cell lines, but some drug’s effectiveness in in vitro models is disproportionate to that observed in the human body. Commonly used immortalized cancer cell lines are cost-effective and convenient to work with; however, they are genetically unstable and less representative of the clinical phenotype observed in patients. Consequently, the EC preclinical studies are based on, e.g., animal models. The in vivo model makes a great opportunity for proper research, often based on invasive procedures. They allow research to be conducted in a way that would be impossible in humans but in accordance with ethical standards (30).
One of the examples of the anima model usage may be patient-derived xenograft (PDX) models. PDX are models of cancer where the tissue or cells from a patient’s tumor are implanted into an immunodeficient or humanized mouse. This strategy simulates human tumor biology allowing for natural cancer progression, and enables the most translational research model for evaluating efficacy. Furthermore, PDX models are applied to various cancers, such as endometrial, breast, colon pancreas, stomach, bladder, lung, cervix, and kidney (31). Imai et al. (32) investigated the presence or absence of the molecular properties of endometrial carcinomas in PDXs passaged up to eight times. It has been reported that established PDXs of endometrioid carcinomas maintained their histopathological characteristics; however, those of carcinosarcomas predominantly consisted of sarcomatous components in comparison to the parental tumors. Alterations in the proportion of cells with immunohistochemical staining for estrogen receptor, PTEN, paired box 2 (PAX2), and paired box 8 (PAX8) (32) are frequently observed. PTEN is the most frequently altered gene in human EC. Genomic analyses of ECs have revealed many recurrent genetic changes as well as the deregulation of signaling pathways. One of the biggest drawbacks of the current hybrid ex vivo/in vivo model of carcinogenesis is the lack of an immune response and tissue-specific physiological microenvironment. Most previous studies have focused on histological features but not on genomic, transcriptomic, and proteomic features regarding the similarity between induced mouse uterine tumors and human ECs (33). The molecular mechanism of pathogenesis is not yet sufficiently described. Hybrid ex vivo/in vivo modeling of EC may accelerate the understanding of EC (34).
Paired box (PAX) is a family of gene factors coding for tissue-specific transcription factors that play key roles in cell fate, early patterning, and organogenesis (35). Using this approach, Wang et al. (36) investigated the function of PAX2 on endometrial cancer both in vitro and in vivo. The results revealed that PAX2 remarkably enhanced proliferation and invasiveness. Moreover, PAX2 impacts the expression of cyclin-dependent kinase 1 (CDK1), which plays pivotal roles in the cell cycle pathway (35). PAX8 belongs to the paired-box gene family, which plays an important role in the organogenesis of different body organ systems. PAX8 plays a significant function in tumor metastasis, embryo development, central nervous system, angiogenesis, and immune regulation (37). Another example of using a mouse as a research model is the transforming growth factor beta (TGF-β). Protein signaling plays an important role in the development of endometrial cancer. PTEN depletion is one of the important factors in developing endometrial cancer in mice. Monsivais et al. (38) introduced and analyzed a mouse model with conditional inactivation of activin receptor-like kinase 5 (ALK5) in the uterus using progesterone receptor—the mice developed endometrial adenocarcinoma with metastases to the lungs. In this study, the authors postulated that TGF-β signaling through TGF-β receptor/ALK5 is responsible for tumor suppression and endometrial regeneration (38). Ruiz−Mitjana et al. (39) addressed the role of the extracellular matrix in the cellular responses to TGF-β. They showed that in the absence of an extracellular matrix, TGF-β-treated endometrial epithelial cells display features of epithelial-to-mesenchymal transition and also used mice housed in a barrier facility and pathogen-free procedures in all mouse rooms (37). The enhancer of zeste homolog 2 (EZH2) is also contributed and studied in endometrial cancer. In this study, mice harboring a uterine deletion of both Ezh2 and Pten showed reduced tumors in the early stage of cancer development. Unfortunately, the authors observed increased inflammatory reactions (40).
Furthermore, small-animal imaging in EC has increased over the last decade. Preclinical imaging enables in vivo observation of long-lasting therapeutic responses and monitoring of tumor growth and metastatic spread. Consequently, preclinical imaging provides imaging biomarkers for prediction and evaluation of treatment (41) Moreover, previous preclinical EC studies focused on caliper size measurements of less relevant subcutaneous models using cell lines (42). Pelvic magnetic resonance imaging (MRI) and whole-body positron emission tomography-computed tomography (PET-CT) play an essential role in primary diagnosis and in detecting recurrent EC disease in patients. Using this approach Espedal et al. (43) developed EC organoid-based orthotopic mouse xenograft models (O-PDX), in which they imitated tumor tissue, namely the histopathologic architecture and protein biomarker expression (43) Computed tomography (CT) is used to find lymph node metastases in EC patients. It has been reported that in preclinical studies, CT has been used to detect both local and advanced diseases. We applied the orthotopic EC xenograft using the estrogen-dependent Ishikawa cell line. In an estrogen-controlled orthotopic model of EC using contrast-enhanced CT (CE-CT), image-derived tumor volume was found to be positively correlated to tumor net weight at necroscopy. SPECT is a type of imaging test that uses a radioactive substance and a special camera to create 3D pictures. This test is also known as single-photon emission computerized tomography. While many imaging tests show what the internal organs look like, a SPECT scan can show how well the organs are working. In preclinical EC models, SPECT takes part in monitoring the treatment efficacy of two different strains of oncolytic viruses (Copenhagen and Wyeth vaccinia virus) in subcutaneous cell-line xenografts (44).
Another example is organoid-initiated precision cancer models (OPCMs). These models enable the functional studies of genetic alterations in tumorigenesis and create precision models for preclinical drug testing. The above model was used, and it was shown that the mutations in phosphatidylinositol-4,5-bisphosphate 3-kinase catalytic subunit alpha (Pik3ca) and phosphoinositide-3-kinase regulatory subunit 1 (Pik3r1) cooperate with PTEN loss to promote endometrial adenocarcinoma in mice. On the other hand, the Kras G12D mutation induces endometrial squamous cell carcinoma (45).
in addition to the mentioned technologies, the most used prognostic and diagnosis tools for cancer are molecular markers, such as proteins or gene mutations. There is a wide list of commonly used markers, the quantitative or qualitative identification of which gives a limited insight into the diagnosis and progression of the disease, but their importance still remains very significant. The importance of many of the factors involved in the pathogenesis and development of cancer is described in detail below.
3.1 Estrogen receptors
Also noteworthy in preclinical studies is the role of estrogen, which can affect the endometrium by interacting with estrogen receptors (ER) to induce endometrial proliferation during the proliferative phase and progesterone receptor (PR) synthesis, which prepares the endometrium for the secretory phase. Knockout studies in mice have shown that the expression of ER, including ERα, ERβ, and G-protein-coupled estrogen receptor (GPER) in the endometrium, is crucial for the normal function of the female reproductive system (46). The possible role of GPER in the regulation of reproductive function was further confirmed by Prossnitz et al. (47), demonstrating that GPER mRNA expression in the endometrial and mammary gland epithelium was regulated in an estrous cycle-dependent manner. In addition, gonadotropic hormones affected GPER protein expression in the granulosa and sheath cells of hamster ovaries. The above results may suggest that GPER may be involved in the regulation of pre-antral follicle development (48).
Abnormal ER expression can cause many diseases, such as endometriosis, endometrial hyperplasia (EH), and endometrial cancer (EC). ERα promotes endometrial cell proliferation and is strongly associated with an increased risk of EC, while ERβ has the opposite effect on ERα function. GPER is strongly expressed in abnormal EH, but its expression in patients with EC remains paradoxical (49). Successful treatment of endometrial-related diseases depends on understanding the physiological function of the ER (46).
3.2 Molecular markers
3.2.1 CD40
With innovative therapeutic methods and the integration of molecular analysis, the treatment of endometrial cancer is undergoing a profound transformation these days. In the past few decades, treatment methods have been limited to surgical resection, chemotherapy, radiation therapy, and hormonal therapy. However, in recent years, therapy based on specific biomarkers has begun to be practiced (50). To investigate tumorigenesis more broadly, it is also worth focusing on proteins that may have an important role in carcinogenesis. This will allow effective blocking of signals necessary for EC cell progression (51). CD40 is a protein that plays an important immunoregulatory role in tumor regression. It has a dual function, both antitumor and protumor. This is due to the expression of proinflammatory and anti-inflammatory cytokines, which is dependent on the strength of CD40 stimulation (52). Some of the pro- and anti-inflammatory mediators, such as vascular endothelial growth factor, help in angiogenesis by producing capillaries. However, they are also often channels for the metastasis of cancer cells (53). It is also included in the tumor necrosis factor (TNF) receptor family, which, when activated, can mediate tumor regression. However, despite the great potential of CD40 agonists, there are few studies on their therapeutic effects on cancers of the reproductive system (54). CD40 activation can significantly affect the tumor microenvironment, independent of innate immune sensors. CD40 knockout mice showed impaired stimulation of T cells to switch classes or form reproductive centers (55).
3.2.2 IL-11
IL-11 belongs to the IL-6 family of cytokines that promote Th17 differentiation in both mice and humans. Th17 cells are proinflammatory cells that secrete cytokines and provide resistance to extracellular pathogens, including defense against infections (56). However, IL-6 alone does not induce Th17 differentiation, unlike IL-11, which induces Th17 cell differentiation and expansion. In the context of signaling pathways in the tumor microenvironment, cytokines such as IL-6 and IL-11 can regulate proliferation, survival, differentiation, or cell death. They can also induce an antitumor response or induce cell transformation and malignancy (57). According to Wang et al. (51), YAP is a transducer protein involved in endometrial cancer progression through upregulation of IL-6 and IL-11. YAP is increased in endometrial cancer cell lines and tissues to a greater extent than in endometrial stromal cells or benign tissue and is involved in endometrial cancer progression with IL-6 and IL-11 (58).
3.3 Programmed death receptor
A programmed death receptor (PD-1) is a negative costimulatory molecule that exerts negative effects on T cells by inhibiting cytokine production and cell proliferation through decreased cytokine expression (59). PD-1 is a cell surface molecule consisting of 288 amino acids. It is a membrane protein in the superfamily of human immunoglobulins. Its role is to suppress the acquired and innate immune response. It is found in immune cells, including activated T cells. It is worth noting that tumor-specific T cells show high expression of PD-1 (60). PD-1 exhibits a dualistic nature, encompassing both beneficial and detrimental effects. It reduces ineffective or harmful immune responses and maintains immune tolerance. However, PD-1 activation may contribute to the development of malignant cells by impeding protective immune responses (50). This type of protein has two types of ligands: programmed cell death ligand 1 (PD-L1) and programmed cell death ligand 2 (PD-L2). They show different expression patterns, are located on chromosome 9p24.1, and encode the CD274 and PDCD1LG2 genes, respectively (61).
PD-L1 is usually detected in macrophages, activated T cells, NK cells, and some epithelial cells in the presence of inflammatory stimuli. In addition, tumor cells use PD-L1 expression to evade antitumor responses (62). The induction of protein kinase D isoform 2 (PKD2) by interferon-gamma (IFN-γ) plays an important role in the regulation of PD-L1. Inhibition of PKD2 activity suppresses PD-L1 expression, increasing the efficacy of the antitumor immune response (63). PD-L1 thus acts as a tumor growth promoter, engaging specific receptors and initiating signaling pathways that encourage cell proliferation and survival. This provides evidence that PD-L1’s involvement in cancer progression is significant. The PD-L1 pathway plays a role in facilitating tumor growth (64). The inclusion of PD-1/PD-L1 inhibitors in anticancer therapy seems promising. However, it is important to remember that the human immune system may create some roadblocks to its effectiveness, which may result in the opposite of the intended effect (65).
3.4 Chimeric antigen receptor for the T cells
Transfusion, which involves the infusion of lymphocytes to induce an antitumor response, also deserves special attention. This is a rapidly developing field, from the immuno-oncological form in preclinical studies to the chimeric antigen receptor for the T cells (CAR-T), which has found application in the treatment of leukemia and lymphoma, among others (66). CAR, or chimeric antigen receptor T-cell therapy, involves modifying a patient’s own T cells by attaching special receptors called chimeric antigen receptors (CAR) to them. CARs recognize and bind to specific antigens on the surface of tumor cells (67). Therapy with the abovementioned cells relies on the selection of a target antigen. This specific antigen must have higher expression against cancer cells and minimal expression in healthy tissues. This allows T cells to distinguish between healthy and diseased cells. In summary, this therapy aims to genetically program a patient’s T cells to target cancer cells in order to kill them (68). CAR-T-cell therapy has shown great promise for treating certain hematologic cancers. However, researchers continue to face obstacles related to the safety and efficacy of this type of therapy (69). In recent years, some miRNAs have also been isolated, including miR-34-5p, which are involved in the regulation of cancer cell proliferation, apoptosis, and metastasis. Novel CARmiR cells have been developed that can serve as a cell-based proof-of-concept therapeutic for in situ production and delivery of miRNAs with therapeutic applications. This was developed by cloning a CAR molecule with interleukin-13 (IL-13 E12Y), which was modified. It exhibits high affinity binding to tumor cells. When CAR is stimulated with cancer antigen, upregulation and export of miR-34a-5p exosomes is induced. Exosomes isolated from CARmiR cells have shown increased toxicity to cancer cells (70). MiRNA-34a is a tumor suppressor microRNA that is absent in many cancer stem cells (CSCs) and advanced cancers. It has been identified as a target of p53, and studies have shown that miR-34a is an essential mediator of p53 function and a potent tumor suppressor (71). MiR-34a inhibits tumor growth and tumorigenesis by inhibiting cell cycle and metastasis, among other things. Moreover, it induces tumor-suppressive processes such as apoptosis and cellular aging (72). The new CARmiR cells, due to their enhanced cytotoxic activity, are predicted, compared to CAR cells, to be an effective tool with high clinical potential. This may contribute to significant advances in CAR-T therapy for cancer treatment (70).
3.5 Cancer stem cell markers
CSCs also play an important role in cancer progression. Cancer stem cells share many similarities with healthy stem cells. When introduced into an animal model, they show the ability to self-renew, differentiate, and induce tumor formation. There is a specific group of markers that are often used to enrich and identify CSCs. These include CD133, CD90, and CD44, among others (73). Research shows that prominin glycoprotein 1 (CD133) is essential for metastasis and proliferation of cancer cells. The proliferative capacity of CD133+ cells is not clearly defined. However, it is speculated that they may have a reservoir function for producing more cells capable of metastasis. CD133 has also been found to have a strong effect on the growth and resistance of CSCs to chemotherapy (74). CD90, also known as Thy-1, belongs to the glycoproteins anchored by glycosylphosphatidylinositol (GPI). Studies show that it belongs to CSC markers in the context of lung cancer (75). A study by Cao et al. (76) shows that spheroid cells showed increased expression of several markers, including CD133 and CD90. Thus, they may be used as a promising treatment option for endometrial cancer. There are also ongoing studies on tumor heterotransplant cell lines to demonstrate the ability of CD44+ to induce tumor formation in NOD/SCID mice (77). Studies (36) also show that CD44 is a marker of CSCs and a regulator of tumor self-renewal, initiation, and metastasis. CD44 can therefore be used to isolate or enrich CSCs through fluorescence-activated sorting of patient cells, tumor tissue heterotransplantation, or cell culture.
4 Signaling pathways involved in induction, progression, and metastasis of endometrial cancer
4.1 Janus kinase/signal transducer and activator of transcription 3
The signal transducer and activator of transcription 3 (STAT3) is the most carcinogenic prominent protein in the whole signal transducer and activator of transcription (STAT) protein family. It is engaged in activating and controlling a wide variety of cancer hallmarks, such as proliferation, cell stemness, metastasis, angiogenesis, immunosuppression, inflammation, and reprogramming metabolism (78). Another STAT3 role is cell cycle regulated, especially G1- to S-phase transition and G2/M-phase checkpoint via cyclins p21 and p27 (79). Therefore, STAT3 has become a promising marker and potential therapeutic target in various types of cancers, such as lung, prostate, pancreatic, breast, and endometrial cancer (80–84). The canonical pathway of STAT3 activation is induced by the binding of an extracellular ligand, such as cytokines and growth factors, with a receptor complex composed of the cell membrane receptor, Janus kinase (JAK), and STAT3, as shown in Figure 3. Ligand–receptor interaction provides STAT3 phosphorylation and separation from the complex via JAK signaling (85). The most well-known traditional activator of the JAK/STAT3 pathway is interleukin-6 (IL-6), indicated as the most significant ligand with carcinogenic potential, which also contributed to endometrial cancer development (86). Elevated levels of IL-6 are strictly related to obesity and oxidative stress, especially in EC, and are associated with a poorer prognosis (87). IL-6, as a multifunctional factor, promotes endometrial cancer, not only in relation to JAK/STAT3. Regulatory activity of IL-6 is universally reported in many tissue cancers, negatively influencing drug chemoresistance and enhancing cell proliferation (88, 89). Other signaling pathways that are regulated with IL-6 in EC are cGAS-STING, nuclear factor kappa B (NF-κB), HIF1α, VEGF, mTOR, and above all, STAT3-related pathways (51, 90, 91). Hyperactivation of STAT3, which is especially linked with tumors, can occur through the following mechanisms: extended activity of receptors for pro-oncogenic ligands; excessive cytokines or growth factor stimulation; enhanced activity of cytoplasmic nonreceptor tyrosine kinases, like JAKs or Abelson kinases, directly or indirectly related to STAT3 activity. The fourth mechanism is a loss of negative JAK/STAT3 regulation (92). In physiological conditions, the negative pathway control has proceeded with protein inhibitors of activated STAT (PIAS) and suppressors of cytokine signaling (SOCS) protein families. The SOCS are shielding STAT- and JAK-binding sites, preventing the activation of subsequent stages of the activation cascade, while the PIAS are blocking active STAT dimers from binding to DNA (93). The universality and ubiquity of STAT3 make it difficult to determine at what stage of cancer development it plays the greatest role. In the early stages of cancer, the STAT3 pathways participate in several tissues, which are linked with cancer growth upregulating cell stemness and proliferation (94, 95). In cases of endometrial cancer, STAT3 inhibition reduces primary cell viability, proliferation, and invasion, impairing not only tumor development but also metastasis (11, 96, 97). Migration and invasion properties of endometrial cancer cells are provided via upregulating, among others, cyclooxygenase-2 (COX2) and matrix metalloproteinase 2 (MMP2) (86, 98). Activated STAT3 directly binds to a lncRNA promoter, promoting its expression and having the ability to form STAT3/lncRNA/miRNA-positive feedback loops (10). Among others, STAT3 promotes CASC9 expression, essential lncRNA in glioma cells, which upregulates the expression of STAT3 via sponging miR-519d, generating a positive feedback loop of STAT3/CASC9/miR-519d (99). LncRNA is involved, among others, in initiating a prometastatic endometrial cancer phenotype; nuclear paraspeckle assembly transcript 1 (NEAT1)-mediated miR-361 sponging activates the STAT3 axis. Modulating the Stat3 axis also engages the myocyte enhancer factor-2 (MEF2), Rho-associated protein kinase 1 (ROCK 1), Wnt family member 7A (WNT7A), and Karyopherin subunit alpha 4 (KPNA4), thus suppressing only one of the factors will inhibit the prometastatic cascade (100).
In endometrial diseases, the JAK/STAT3 pathway is especially triggered with leptin, a protein hormone related to obesity and predominantly expressed by adipocytes (101). The deregulated JAK/STAT3 pathway is a common factor in endometrial cancer and diabetes. Diabetes constitutes a significant risk factor for endometrial cancer induction and development; conversely, a diabetes prevention diet may decrease the occurrence and development of endometrial cancer (102). The symptoms of diabetes may be modulated by regulating insulin and insulin growth factor (IGF) levels, which activate the JAK/STAT3 pathway (18). That effect may be reduced by using antidiabetic drugs, like metformin, which inhibit IGF activity (103), but there is a poor chance of inhibiting or blocking the development of cancer using metformin (104). The effects of metformin may only reduce the upregulating effects of high glucose conditions on JAK/STAT3 levels (105).
4.2 Transforming growth factor-β
TGF-β is a wide family of secreted cytokines, demonstrating conservativeness in the whole animal kingdom and appearing in the early days of multicellular evolution. It fulfills universal signaling functions that provide tissue- or cell-specific control of differentiation, proliferation, and metabolism across the full spectrum of embryonic and adult development (106). Through the developmental processes, TGF-β pathways are responsible for the regulation of cell proliferation and apoptosis, especially in terms of endometrial epithelial cells (107). The canonical TGF-β uptake proceeds with recipient cells’ receptor kinases, mediating phosphorylation of the SMAD protein family, activating the cascade, and manipulating the expression of target genes, as shown in Figure 3 (108, 109). The signaling pathway is important in in tumor development and metastasis of gynecological cancers, but it also plays a dual role, activating or suppressing carcinogenesis depending on the tissue (110). One of the family members, bone morphogenetic protein (BMP), has a tumor-promoting effect on ovarian and endometrial cancer, whereas it fulfills a protective role on uterine cervical cancer (111). In endometrial cancer, enhanced activity of the TGF-β/Smad pathway is correlated with tumor progression, metastasis, and recurrence (112), and its implication in the malignant phenotype of endometrial tumors also promotes cell stemness (113, 114). TGF-β enhances the malignancy of endometrial cancer by disturbing the epithelial permeability barrier, cell migration, and mitochondrial metabolism (115). The effect of endometrial cancer development is additionally increased by the immunosuppressive effect of TGF-β, disrupting the functionality of natural killer cells and T cells (116, 117). The regulation of the TGF-β/Smad pathway occurs variously and is modulated according to the expression of other proteins, especially in cancers with inactive TGF-β expression. The activity of the pathway is counter-proportionally correlated with cancer-related proteins, like flotillin 2 (FLOT2) or early growth response 2 (EGR2) (118, 119). Targeting the miR-650/SMAD7 axis via lncRNA MCTP1-AS1 suppresses cell proliferation, migration, invasion, and epithelial–mesenchymal transition in endometrial cancer (120). The result of stopping that axis is downregulation of the TGF-β activity, demonstrating therapeutic potential. In other research, inhibiting lncRNA AFAP1-AS1 negatively regulated the TGF-β/Smad axis, influencing proliferation, migration, and apoptosis via miR-424-5p. The AFAP1-AS1 effect was demonstrated on an endometriosis model, but potentially inhibiting this signaling pathway may also support the inhibition of endometrial cancer development. Due to endometrial cancer related to metabolic syndrome, the pro-oncogenic effect of TGF-β/Smad is enhanced by lysophosphatidylcholine acyltransferases (LPCATs), a regulator of intracellular lipid metabolism, which promotes cell stemness and metastasis (121). The opposite effect can be achieved by using metformin, a well-known antidiabetic agent, which has a neoplastic effect by silencing the activity of the pathway (39). Protein kinase C α (PKCα) activity affects the TGF-β receptor 1 (TGF-βR1), which positively influences the TGF-β ability to induce SMAD phosphorylation and cell cycle arrest (122). The process is dependent on extracellular signal-regulated kinase 2 (ERK2) and runt-related transcription factor 2 (Runx2), and the knockdown of either of the genes immediately suppresses tumorigenesis (123).
4.3 Mammalian target of rapamycin
The mammalian target of rapamycin (mTOR) plays a central role in endometrial behavior and fertility, being involved in estrogen signal transduction. Dysregulation of the mTOR pathway is critical in the induction and progression of endometrial diseases, including cancer (124). Additionally, in various tumors, mTOR signaling interacts and loops with another axis, like the WNT/β-catenin pathway, which may activate mTOR signaling, while mTOR signaling may suppress the WNT/β-catenin pathway (125). Both of the above are the key regulators of endometrial cancer progression (126). The interaction of the pathways may be useful for indicating tumor grading. Identification of WNT in the cell membrane may exclude the third grade of neoplasms, whereas the presence of cytoplasmic WNT and nuclear mTOR may indicate the third grade of neoplasms (127). The canonical pathway of mTOR activation is preceded by phosphoinositide 3-kinase (PI3K) and protein kinase B (AKT or PKB) activity, as shown in Figure 3, the standard signaling pathway in tumor induction and progression, promoting cell metabolism, proliferation, migration, and angiogenesis (128). Pro-oncogenic properties of the pathway can be suppressed at many levels, such as blocking PI3K with p53, substituting AKT with the MLLT11-TRIL molecule complex, or inhibiting mTOR with AMPK upregulation (129–131). Suppressing activity of the AKT/mTOR pathway with some of the factors effectively inhibits the tumor tissue activity (132, 133), but endometrial cancer therapies engaging only a single inhibiting factor are insufficiently effective as monotherapy (134); thus, using some factors enhancing the suppressing mTOR inhibitors effect significantly intensifies reducing endometrial cancer cell viability, colony formation ability, and induced apoptosis (135). The most frequently followed genetic alterations in endometrial cancer are aberrations of PTEN, which is a negative PI3K/AKT/mTOR regulator. The mutation or deletion of the gene leads to disturbed activation of the PI3K/AKT/mTOR pathway, which occurs in 60%–80% of endometrial cancer cases (136).
5 Dysregulated noncoding RNA profile in endometrial cancer
Genome biology has evolved beyond the traditional comprehension that DNA is merely transcribed in order to be translated into protein molecules. The majority of the RNA transcripts do not code for any proteins. Instead, they govern dozens of intracellular and intercellular processes in normal or diseased settings (137). To address some functional models for these ncRNAs, lncRNAs depict multiple roles in the modulation of chromatin structure, gene silencing, mitochondrial homeostasis, and protein recruitment via interaction with other RNAs. It appears to be rational to consider networks and pathways in ncRNAs’ biology and function to achieve a plenary viewpoint (138, 139). Through influencing the gene expression profile, diverse types of ncRNAs modify the cell phenotype. Malignancy is a major landscape in which ncRNA dysregulation is a significant determinant (140). miRNAs, circRNAs, PIWI-interacting RNAs (piRNAs), and lncRNAs play divergent roles in cancer development, invasion, and metastasis, as well as in cancer drug resilience via different mechanisms such as apoptosis suppression and CSC progression (140, 141). According to the literature, ncRNAs may be practical therapeutic objects and handy clinical markers in various cancer types (142, 143). Previous studies have also revealed the dysregulated patterns of ncRNAs in EC. Noncoding RNAs especially miRNAs and lncRNAs, affect many pathways in EC tissues, leading to EC cell viability or mortality. For example, lncRNA GAS5 overexpression causes apoptosis in EC cells, and its expression is reduced in EC tissues. GAS5 targets the miR-222-3p/p27 axis, resulting in the suppression of EC progression secondary to elevated glucose levels (144, 145). In addition, by investigating EC cell lines KLE and HEC-1-B, (146) demonstrated that miR-25-3p binds and inhibits BTG2 gene mRNA, causing EC proliferation and motility. They also reported that lncRNA SNHG5 acts as competing endogenous RNA (ceRNA) to sponge miR-25-3p and attenuates EC progression (147). Also, the differential levels of circRNAs have been investigated in EC. (148) demonstrated that hsa-circ-000579 is upregulated in EC tissue samples and is a positive regulator of EC. According to the fact that miR-298, which targets the oncogene CTNND1, is downregulated in EC cells, this circRNA was introduced as miR-298 sponge by utilizing related assays. Thus, hsa-circ-000579/miR-298/CTNND1 axis functioning is related to unfavorable outcomes (149). In a recent study, Lai et al. (150) discovered that the lncRNA BMPR1B-AS1 is highly expressed in EC tissues compared to its normal counterparts. They also reported that this lncRNA causes aggressive behaviors in EC cells through mechanisms like cell cycle activation and promoting mesenchymal features. BMPR1B-AS1 targeted and negatively regulated miR-7-2-3p in EC cell lines, leading to DCLK1 upregulation. Due to the fact that DCLK1 promotes EC via the PI3K/Akt/NF-κB pathway, BMPR1B-AS1/miR-7-2-3p/DCLK1 axis was propounded as a machinery for EC promotion (150). CCP110 is a conserved protein essential for centrosome function, and its upregulation is associated with invasive behavior in tumor cells. In an attempt to reveal the regulation of this protein in EC cells, (151) found that CCP110 is overexpressed in EC cells, and they reported that miR−129−2−3p inhibits the translation of CCP110 mRNA as well. Moreover, their research revealed that lncRNA XIST is highly expressed in EC cells and acts as ceRNA to sponge miR−129−2−3p. Therefore, the XIST/miR−129−2−3p/CCP110 axis was suggested to be involved in EC advancement (151). Overexpressed lncRNA HEIH has been discovered to be a factor for EC cells’ resistance to paclitaxel via MAPK signaling enhancement, which interferes with HEIH function, leading to vulnerability of the EC cells exposed to paclitaxel treatment (152). LncRNA SNHG4 is also expressed at higher degrees in EC cells and is correlated with cancer cell proliferation and migration. It is believed that this effect is established proportionally through upregulation of epithelial-to-mesenchymal transition (EMT) markers and transcription factor SP-1 (153).
6 Exosomes and tumor biology: emphasis on noncoding RNA cargos
Tumor structure is known to be an organized system of diverse cellular and other biological components. The innumerable processes in a highly dynamic environment make it essential for the tumor tissue to be under continuous rearrangement. The main factor is the effective communication apparatus of the tumor cells with other cells and tumor microenvironment (TME) cells (154). Exosomes have been described for a couple of decades to act as interesting tools of paracrine crosstalk in TME. These cup-shaped membranous vesicles shed outside the cells have an average diameter of approximately 100 nm and are capable of carrying multiple molecular cargos, including proteins, lipid products, DNA, and coding and noncoding RNAs (155). Exosomes could either deliver the components to the neighboring cells or may be transmitted by the bloodstream to exert their effects remotely. On most occasions, the net consequence of exosomal signal transduction is to facilitate tumor progression and invasion. Many exosomal elements take part in oncogene expansion, tumor suppressor gene regulation, angiogenesis, metastasis, and drug resistance (156). Exosomes also play a role in the modulation of cancer immunity by mediating the expansion or repression of tumor-residing immune cells (157). Exosomes secreted by the cancer cells were found to carry the checkpoint protein PD-L1 and suppress the anticancer immunity exerted by cytotoxic T cells. Also, the PD-L1-containing exosomes may inhibit the maturation of dendritic cells, leading to diminished T-cell immunity (158, 159). Exosomes are considerable factors that pave the way for tumor metastasis. Their components may induce EMT via several mechanisms, like upregulation of mesenchymal markers or EMT-related signaling pathways (e.g., N-cadherin and STAT3, respectively) (159, 160). Aiming to facilitate tumor invasion, exosomes also mediate the extracellular matrix (ECM) remodeling by degradation and/or synthesis of the ECM network (161). Interestingly, tumor-derived exosomes are remarkable factors in the determination of target metastasis organs (162).
Exosomes are capable of carrying various ncRNAs to establish cancer development by promoting multiple aspects of tumor biology. Exosomal ncRNAs (also called ncRNA-exosomes) are known as key determinants of tumor fate and could be chased or manipulated to achieve clinical advantages (163). RNA products remain viable and conserve their potential in the exosomes while they travel outside the cell, where the major amount of miRNAs in circulation are packed in the exosomes (164). Noncoding RNA components of the exosomes are highly divergent according to the physiology of the tumor tissue and the cells from which they originate. All the ncRNAs’ subtypes along with other ncRNAs, are found in tumor-derived exosomes, influencing the condition of the recipient cells (165). Li and Tang (166) reported that exosomes containing miR-221-3p derived from M2 macrophages in ovarian cancer could enhance the cell cycle by facilitating G1/S transition as a result of CDKN1B downregulation. Also, by targeting PIK3R1, miR-221-3p augments resistance to adriamycin when delivered by exosomes to breast cancer cells (167). HOTAIR is a relatively well-known lncRNA that is dysregulated in diverse cancer types, including lung cancer. Exosomal HOTAIR derived from nonsmall cell lung cancer (NSCLC) cells enhanced cancer cell growth, migration, and invasion by acting as ceRNA for miR-203 (168). Recently, Xie et al. (169) concluded that delivery of exosomal circRNA vacuole membrane protein 1 (circVMP1) to cisplatin-sensitive NSCLC cells targets and sponges miR-524-5p and upregulates METTL3 and SOX2. The outcome was enhanced tumor resistance to cisplatin both in vitro and in vivo (169). It is also revealed that exosome-derived lnc01559 mediates gastric cancer (GC) stemness and metastasis, and its suppression leads to tumor inhibition. In addition, in a study by Xie et al. (169) in GC, exosomal circSHKBP1 inhibited miR-582-3p function to upregulate HUR and VEGF signaling the consequence of which was tumor progression (170). Another investigation designed by Yang et al. (171) identified the tumor-promoting role of exosomal hsa-circ-0085361 (circTRPS1) in bladder cancer. The study demonstrated that circTRPS1 delivered by exosomes is able to sponge and neutralize miR-141-3p, which in turn enhances glutamine metabolism via GLS1 overexpression. GLS1 upregulation could also induce CD8+ T-cell exhaustion and bladder cancer cell proliferation (171). Noncoding RNAs transferred in exosomes intensify tumor chemoresistance as well. For instance, exosomal lncRNA PART1 amplifies esophageal squamous cell carcinoma (ESCC) cells’ resistance to gefitinib. Regulation of miR-129/Bcl-2 pathway has been introduced as the underlying mechanism. Furthermore, PKM2 is reported to be upregulated in oxaliplatin-resistant colorectal cancer cells. Transportation of hsa-circ-0005963 via exosomes from resistant cells to sensitive counterparts and miR-122 neutralization were found to be the culprit machinery (148). The role of exosomal ncRNAs is also identified in the crosstalk between tumor cells and TME-residing macrophages and fibroblasts. Tumor-associated macrophages (TAMs) can switch phenotypes between M1 (proinflammatory) and M2 (anti-inflammatory) states in a reversible and dynamic manner. M2 macrophages secrete growth factors and inhibit antitumor immunity. Hepatocellular carcinoma (HCC) tissues are highly enriched with exosomal lncRNA TUC339, which induces M2 polarization in TAMs, decreased cytokine production and compromised phagocytosis. LncRNA TUC339 takes part in cytokine and chemokine receptor signaling pathways. Moreover, increased exosomal has-circ-0048117 secondary to hypoxia in ESCC tissue results in M2 polarization mediated by upregulating TLR-4 and miR-140 sponging. While in colon cancer, tumor-derived exosomal miR-21 causes M1 polarization by TLR-7 signal transduction, producing a suitable premetastatic niche in the liver (172, 173). Exosomes containing miR-501-3p, which originate from M2 TAMs, facilitate pancreatic cancer progression, invasion, and tube formation by targeting the TGFBR3 gene and TGF-β pathway promotion (174). Intriguingly, cancer-associated fibroblasts (CAFs) are also modulated by exosomal ncRNAs. Metastatic HCC cells produce exosomes carrying miR-1247-3p in order to convert the normal tissue fibroblasts into CAFs by regulating B4GALT3 and β1-integrin-NF-κB activation in the pulmonary metastatic niche (175). All the mentioned example studies indicate that ncRNA-containing exosomes influence cancer behavior in multiple divergent ways, and profound apprehension of their aspects is crucial to acquiring a broader viewpoint toward the complexity of tumor setup.
7 ncRNA-exosomes and endometrial cancer: biology and applicable opportunities
ncRNAs, which are transferred by exosomes, regulate and foster the cancer hallmarks in gynecologic cancers, too. Investigations and multi-aspect study designs have been directed to elucidate the roles and tasks of ncRNA-exosomes in gynecologic solid cancers. However, the available data about these biological particles in EC pathogenesis is more limited (176). Similar to other cancers, EC tumor tissue cells communicate and affect the phenotypic features of each other by means of exosomes, which could be detected in the patients’ sera. Exosomes appear to be of great importance in the context of endometrial cancer. In a recent clinical plus in silico proteomic study by Sommella et al. (177), they suggested candidate exosomal proteins able to discriminate the patients from healthy controls with an acceptable sensitivity (178). Exosomal signal transduction participates in EC growth and proliferation, invasion, metastasis, and angiogenesis through diverse methods (179). Song et al. (180) proposed a model in which exosomes may promote EC growth and angiogenesis. They depicted that plasma exosomes from EC patients bear the capacity to induce tumor proliferation and human umbilical vein endothelial cell angiogenesis. The suggested mediator was exosomal lectin galactoside-binding soluble 3 binding protein (LGALS3BP), which caused EC growth and angiogenesis via the PI3K/AKT/VEGFA machinery pathway. Interestingly, patients with high tissue LGALS3BP had a limited survival period (180). The tumor physiology of EC is also regulated by the means of ncRNA-exosomes, and EC cells, TAMs, and CAFs exchange signals by these nanosized messenger vehicles (181). Zheng et al. (182) have reported that exosomal miR-93 is increased in amount in the EC patients’ bloodstream. More recently, Zhang et al. (183) revealed that this miRNA can target the ZBTB7A gene and promote EC. Therefore, exosomal miR-93 transduction could be considered an attempt by the tumor to stay viable and migrate to remote sites. It is worth indicating that miR-93 is sponged by lncRNA SNHG14, and SNHG14/miR-93/ZBTB7A is a functional tumor-repressor axis in the EC cells (182, 184). Like other tumor types, CAFs have positive effects on the development of EC. It is concluded by Li et al. (147) that in EC tissues, exosomes derived from CAFs bear fewer amounts of miR-148b than exosomes from normal fibroblasts. The authors also reported that miR-148b targets and downregulates the oncogene DNMT1. Thus, the loss of exosomal miR-148b is a tumor-promoting phenomenon in EC. By exogenously transfecting CAFs with miR-148b, this RNA molecule was delivered by CAF-derived exosomes to the EC cells and inhibited EMT and tumor invasion, bringing a potential approach in the field of EC treatment (185). miRNA-499a-5p depicts a lower expression level in EC tissues compared to the neighboring normal endometrial cells. By embedding it into the exosomes obtained from EC mesenchymal stem cells, Jing et al. (186) were able to repress EC proliferation and angiogenesis both in laboratory and animal settings. They also demonstrated that Vav guanine nucleotide exchange factor 3 (VAV3) gene neutralization by miR-499a-5p is the underlying mechanism (186). We previously stated that ncRNA-exosomes have the ability to alter the characteristics of TAMs in the TME. An in vitro investigation by Xiao et al. (187) showed that under hypoxic conditions, EC cells increase their exosome production, and the expression profile of exosome-associated miRNAs differs as well. There was a significant increase in the exosomal miR-21, and when transferred into the THP-1 monocyte cells, these cells depicted M2-like polarization, as confirmed by IL-10 and CD206 overexpression. The abundance of M2 macrophages in EC samples is correlated with tumor aggressiveness and poor prognosis (187). Moreover, Wang et al. (188) indicated that TAMs enhance EMT and inhibit apoptosis in EC cells, partly by means of exosome transduction. They revealed that miR-192-5p is expressed lowly in TAM-derived exosomes. The oncogene axis IRAK1/NF-κB was reported as the target for the miRNA. Therefore, overexpressed miR-192-5p in exosomes secreted by TAMs would be a promising tool to be absorbed by EC cells to exert anticancer activity (188). Aiming to determine the differentially expressed core genes, the related pathways, and their influence on the prognosis of EC patients, Shi et al. (189) designed an in silico study. Their results included a couple of genes from different pathways; among them was FOXL2, which depicted reduced expression in EC tissues associated with reduced patient survival. In addition to verification of the obtained data in EC cell lines, they also investigated exosomal ncRNAs secreted from the tumor cells. By knowing the fact that miR-133a targets FOXL2 gene transcript, they found increased levels of miR-133a in the exosomes derived from the EC cells, which could be absorbed by normal endometrial cells. These results suggest a potential regulatory process in the EC tumorigenesis mediated by miRNA-containing exosomes (189). It is conventionally believed that tumor infiltration with effector CD8+ T cells results in antitumor cytotoxicity and tumor degradation. Novel studies indicate that there are additional mechanisms for the tumor-repressing role of the CD8+ T cells like exosome transduction. The study by Zhou et al. (190) depicted that, as a consequence of estrogen signaling, miR-765 is downregulated in EC tissues compared to the normal endometrium. They also inferred that the PLP2 gene, which promotes EMT and EC cell migration via Notch signaling, is regulated by this miRNA. The outstanding finding of this research was the elevated levels of miR-765 in exosomes produced by CD45RO−CD8+ T cells. This evidence indicates the activity of CD8+ T cells in EC via the formation of exosomes enriched with miR-765 to restrict the oncogenic impact of estrogen (191). Recent evidence also supports the potential of exosomal miR-26a-5p released by EC tumor cells and uptaken by lymphatic endothelial cells to induce lymphatic vessel generation and promote lymphatic spread of EC via LEF1/c-Myc/VEGFA axis (192). One of the remarkable risk factors for EC tumorigenesis is the concomitant underlying polycystic ovarian syndrome (PCOS). In order to explore the probable role of exosomal ncRNAs in this association, Che et al. found that EC cells demonstrate high levels of migration and invasion when exposed to exosomes isolated from the sera of PCOS patients. MicroRNA-27a-5p was the most notably upregulated miRNA in those exosomes. Target-prediction databases, confirmed by further luciferase assay, brought SMAD4 as the target for miR-27a-5p. Thus, circulating exosome-derived miR-27a-5p facilitates EC progression via downregulation of SMAD signaling in PCOS patients (193). Recently, Pan et al. (194) intended to bring an approach based on ncRNA-exosomes to target EC cells. They purified and collected exosomes containing miR-503-3p secreted from human umbilical cord blood mesenchymal stem cells. They also revealed that miR-503-3p targets mesoderm-specific transcript (MEST) gene, which is a potential cancer promoter. After transfecting with exosomal miR-503-3p, the growth and biologic features of the EC cells were suppressed proposing a beneficial opportunity in the EC treatment (194).
Also, lncRNAs could be the cargo for exosomes to transduce signals in the TME. As discussed previously, CAFs bear notable tumor-promoting characteristics in the EC by releasing ncRNA-exosomes. LncRNA NEAT1 is upregulated in EC tissues, and its abundance is correlated with higher tumor proliferation capacity. Fan et al. (168) found that NEAT1 is overexpressed in CAFs derived from EC tissues compared to normal endometrial fibroblasts, and this discrepancy was also spotted in exosomes derived from those cells. By transferring exosomal NEAT1 to EC cells, an acceleration in tumor growth was observed. Moreover, the authors concluded that the tumor progression is due to the influence of NEAT1 on the miR-26a/b-5p/STAT3 axis by sponging the miRNA, which increases YKL-40 expression (195). Since a couple of years ago, lncRNA DLEU1 has been introduced as a mediator in EC cell viability and invasion. Recent research by Jia et al. (196) confirmed the upregulation and cancer-boosting role of this lncRNA in the EC tissues. They additionally stated that DLEU1 is transmitted by the exosomes to the adjacent EC cells and increases their capacity to proliferate and migrate. The underlying mechanism for this phenomenon is that DLEU1 targets and sponges miR-381-3p, which is an identified regulator of the E2F3 oncogene (196). LncRNA NONHAT076754 functions to facilitate metastasis and invasion in cancer. Qiu and Hua, (197) revealed that NONHAT076754 is highly detected in ectopic endometrial stroma relative to the normal eutopic endometrium in endometriosis patients. In addition, this lncRNA could be shuttled via exosomes from the ectopic cells and uptaken by the normal cells, resulting in an upregulation of invasion capacity in the recipients. The underlying mechanism was the regulation of the tight junction protein ZO-1, E-cadherin, and N-cadherin (197). Identification of exosomal circRNAs in cancers has shed more light on the mechanisms by which tumor biology is regulated. This concept, as well as other ncRNA-exosomes, may determine the clinical features or predict the EC patients’ prognosis. The profile of circulating exosomal circRNAs in EC patients and healthy controls is diverse. The study by Xu et al. (198) demonstrated 209 increased and 66 decreased exosomal circRNAs in the sera of EC patients. The major molecular pathways in which the dysregulated circRNAs took part were actin cytoskeleton regulation, ECM–receptor interaction, and focal adhesion pathways. However, increased fold changes for only two circRNAs, hsa-circ-0002577 and hsa-circ-0109046, were calculated to be greater than 2 (198). An interesting investigation by Gu et al. (199) depicted a model for the role of exosomal circRNAs as a communication tool for cellular members of the TME. According to their report, TAMs obtained from human EC tissues bear M2-like features, and TAM-derived exosomes absorbed by EC cells reduced radiosensitivity of the EC cells in vitro. Circular RNA microarray analysis indicated that hsa-circ-0001610 was the most abundant circRNA in the exosomes from TAMs. They also concluded that hsa-circ-0001610 was the mediator for the radioresistance of the EC cells via functioning as ceRNA for miR-139-5p. In silico and in vitro analyses suggested cyclin B1, a vital facilitator of radioresistance in multiple cancers, as the target for miR-139-5p. The mentioned data were confirmed in a xenograft tumor model study on nude mice. Thus, TAM-derived exosomal hsa-circ-0001610 contributes to radioresistance in EC by regulating the miR-139-5p/cyclin B1 axis (199). Eventually, as a novel approach in the field of ncRNA-exosome discovery in EC, Qian et al. (200) studied the expression profile of transfer RNA-derived small RNAs (tsRNAs) in tissue samples and serum specimens. They concluded that the expression of tRF-20-S998LO9D was significantly inhibited in both the tissue and serum exosomes of the patients. tRF-20-S998LO9D overexpression led to EC cell apoptosis as well as decreased proliferation, invasion, and migration capacity. However, the field of exosomal tsRNAs requires additional precise investigations (200).
Taken together, recent studies considering the prospects of ncRNA-exosomes in EC have brought encouraging results so far. Some studies have designated the ncRNAs transmitted by the exosomes as a part of the tumor physiology, while others have disclosed the possible applications of ncRNA delivery via exosomes with therapeutic intentions to alter the EC biology. Table 1 summarizes the prominent details of the aforementioned studies about exosomal ncRNA transportation in EC.
8 The role of exosomes in diagnosis, prognosis, and anticancer therapy
Exosomes belong to phospholipid bilayer extracellular vesicles, and they are released by all types of cells, including cancer cells. Exosomes contain DNA and RNA, lipids, and proteins and also perform communication functions between cells (202). As a result, exosomes have been used in the diagnosis and prognosis of endometrial cancer through the analysis of biomarkers, showed at Table 2 constituting the content of exosomes. Moreover, the above diagnostics are specific and noninvasive (234). The first biomarker may be ncRNA, which includes miR-15a-5p (227). miR-15a-5p expression was consistently upregulated in plasma-derived exosomes from EC patients. Moreover, patients with a large tumor had higher exosomal miR-15a5p expression compared with a small tumor, which may indicate the significant usefulness of this biomarker in EC diagnostics (190). It is also worth mentioning that miR204-5p, miR-423-3p, and miR-20b-5p, miR-143-3p, and miR-195-5p expression were increased in the serum of EC patients. miR204-5p could suppress cancer by suppressing EMT, and self-renewing cancer stem cells. miR-423-3p may participate in the development and progression of EC, namely inhibiting cisplatin-induced apoptosis. miR-20b-5p could modulate vascular endothelial growth factor A (VEGFA) transcription by targeting hypoxia-inducible 4 factor 1-alpha (HIF1α), may act as a tumor suppressor by hindering MMP-2 expression, leading to cell cycle arrest, and also has a regulatory function in oxygen balance (195). Another biomarker, miR-143-3p, can inhibit cell proliferation and EC metastasis through mitogen-activated protein kinase (MAPK1) (235). miR-195-5p-bound signaling pathway target genes involved in oncogenic mechanisms, such as cell proliferation and apoptosis (234). miR-205 has increased expression in the EC compared to normal endometrial tissues; mutations in miR-205 were found in EC for 25%–83% of cases. miR-205 participates in regulating the expression of PTEN, which is the most common mutated tumor suppressor gene. PTEN takes part in inhibitory function by proliferation and promoting apoptosis, deletion, or mutation leading to carcinogenesis. miR-205 upregulation blocked PTEN translation and activated the AKT pathway; constitutive activation of AKT contributes to tumor progression. It should also be noted that miR-205 acts as an oncogene and inhibits cellular apoptosis in the EC by targeting the PTEN/AKT pathway. Also, miR-205 plays a significant role in the invasion and immigration of endometrial cancer. This mechanism is based on the targeting of miR-205 to the AKT pathway by inhibition of E-cadherin expression, promotion of Snail expression, and downregulation of glycogen synthesizing kinase 3β (236). Circular RNAs (circRNAs) also belong to noncoding RNA and may be a useful diagnostic and prognostic tool; specifically, they are hsa_circ_0109046 and hsa_circ_0002577. Increased expression of circRNAs was demonstrated in EC. circRNAs are related to, e.g., neoplastic migration, the ECM–receptor interaction pathway, the regulation of actin cytoskeleton pathway, and the focal adhesion pathway (198). It is also worth emphasizing that increased expression of proteins such as metalloproteinase 9 (MMP9) and pyruvate kinase (KPYM) has been found in EC patients and showed 94% sensitivity and 87% specificity for EC (237). The NEAT1 (a long noncoding RNA) participates in invasion, chemoresistance in EC cells, and remodeling tumor microenvironment by induced miR-361 suppression, which activates STAT3. Higher levels of NEAT1 may be positively correlated with advanced tumor stage and lymph node metastasis. Another important biomarker is WNT7A, coding protein Wnt-7a. The expression of WNT7A was higher in EC tissue than in normal endometrial tissue. Furthermore, increased WNT7A expression was associated with a high tumor grade, increased depth of myometrial invasion, lymph node metastasis, and vascular invasion (100). LGALS3BP may be a biomarker with diagnostic and prognostic value that causes EC growth and angiogenesis by the PI3K/AKT/VEGFA machinery pathway (180).
Exosomes have been used not only in diagnosis and prognosis but also as therapeutic tools in the treatment of EC due to their properties that play a key role in the communication between the tumor cells and the tumor microenvironment EC, and they can also be collected from various body fluids such as uterine fluid and blood. As a result, they provide a noninvasive method for diagnosing and monitoring endometrial cancer. Exosomes contain a cargo that may constitute tumor suppression by adding tumor-suppressive miRNAs or proteins to inhibit their growth and metastasis cells of EC (238). Engineered exosomes with cargo tumor-suppressor miRNAs may become a useful therapeutic tool in the fight against EC cancer progression. miR-499a-5p inhibits EC proliferation by targeting VAV3 and suppresses EC growth, metastasis, angiogenesis, or metastasis (186). It should also be noted that human umbilical cord mesenchymal stem cell (hUCMSC)-derived exosomes may be a valuable tool for target-based therapies, namely engineered exosomes that, with tumor-suppressor miRNAs, can be delivered by hUCMSCs to EC cells and finally inhibit their proliferation (239) targeted EC cells overexpressing miR-302a exosomes and disrupted their migration proliferation and by suppressing cyclin D1 levels and inactivating the AKT signaling pathway (240). Additionally, overexpression of miR-503-3p in hUCMSC-derived exosomes inhibited tumor growth and suppressed the expression of MEST, which is involved in EC by cell proliferation, differentiation, and apoptosis (181) Another application example is exogenously transfected miR148b cancer-associated fibroblasts (CAFs)-derived exosomes, which suppress EC cell invasion and metastasis by targeting DNMT1 gene. DNA (cytosine-5)-methyltransferase 1 (Dnmt1) in humans is encoded by the DNMT1 gene. Dnmt1 takes part in DNA methylation. Aberrant methylation patterns are associated with certain human tumors, including EC (185). The direct transfer of CAF-secreted exosomal miR-320a to EC cells inhibited their invasion, proliferation, and migration by targeting the HIF1α–VEGFA axis. MiR-320a binds directly and regulates the mRNA of HIF1α. HIF1α is known to target the vascular endothelial VEGFA (184). MSC-derived exosomes loaded with miR-499a-5p could suppress tumor growth migration, invasion, or angiogenesis of EC cells by targeting VAV3, which is a guanine nucleotide exchange factor (GEF) that regulates the activity of Rho/Rac family GTPases. The main role is the regulation of cell differentiation, motility, and proliferation (186).
9 Future perspectives
There is still not enough research on specific markers for EC, despite the growing interest in treating endometrial cancer. Some markers, such as CA-125 or HEP-4, are not specific only to EC. They can give false-positive results, especially in the presence of pelvic inflammation or other gynecological cancers or endometriosis. Another limitation is the absence of elevated marker levels in the early stages of the disease. This makes early diagnosis impossible. Unfortunately, marker levels can also change in response to treatment, which also makes it difficult to interpret the results.
EC shows quite significant genetic variability, which also contributes to problems in finding universal markers. Another challenge is the variability of this type of cancer and the problem of diagnosis. Due to the use of mostly invasive methods of EC treatment, it is worthwhile to look into noninvasive technologies. Magnetic resonance imaging with molecular contrasts can aid in the early detection and assessment of tumor extent. Despite the limitations of markers, research in this direction continues. The investigation of genetic and epigenetic markers shows great specificity and sensitivity. Metabolic markers also seem very promising; this is related to their frequent variability in the very early stages of the disease. This would favorably affect the problems of late diagnosis of this type of cancer, increasing the chances for patients.
CAR-T research, which is developing quite rapidly in the context of endometrial cancer, also seems promising. A key factor in this therapy is the identification of specific antigens for endometrial cells that are not present in healthy cells. Increasing the safety of the therapy by using molecular “breakers” also seems promising. This minimizes the risk of cytokine release syndrome as well as other toxicities. Another limitation may be the costs associated with conducting such studies, as well as their length and complexity. It is also worth mentioning tumor-infiltrating lymphocytes, these are lymphocytes isolated directly from the tumor, multiplied and activated in vitro, and then reintroduced into the patient’s body. This is a type of adoptive cell therapy that supports the patient’s immune system in the fight against cancer. This therapy is promising due to its low toxicity. However, there are still limitations, mainly related to isolation time, cell multiplication, and cost. Unfortunately, the effectiveness of this therapy is mainly dependent on the number of isolated lymphocytes.
Researchers suggest a potential role for p53 as an antigen in the acquired immune response and as a key monitor of the innate immune system. The use of antibodies against p53 is expected to be therapeutically effective against cancers carrying the same p53 mutant. In recent years, more and more promising approaches to p53-based therapy have emerged, which may suggest its efficacy. The molecular classification of EC, consisting of ultra-mutated POLE, mismatch repair deficiency, p53 deficiency, and subgroups with nonspecific molecular profile (NSMP), has strong and independent prognostic value, including potential response to chemotherapy.
Exosomes and miRNAs also show great and crucial potential. The advantage of using exosomes may be primarily the lack of invasive monitoring methods, as they can be extracted from body fluids. They can also carry small RNAs that target genes responsible for cancer progression. They may also find use in carrying anticancer drugs directly to EC cells without adversely affecting healthy cells. However, there are also limitations to using this therapy. It is necessary to invent new isolation techniques that are more precise. In recent years, interest in the use of exosomes and miRNAs in endometrial cancer has been growing and may prove to be a breakthrough in the treatment of this problematic type of cancer.
The fact that there is no up-to-date classification of endometrial cancer worldwide is also a very serious limitation. There is very little information newer than 2022. It would be tremendously helpful to create a newer classification, which would certainly update the data.
Author contributions
JN: Writing – original draft. SW: Conceptualization, Writing – review & editing. DD: Writing – original draft. KD: Visualization, Writing – original draft. MF: Writing – review & editing. MZ: Visualization, Writing – original draft. MA: Writing – review & editing. FP: Writing – original draft. AB: Software, Visualization, Writing – original draft. MK: Supervision, Writing – review & editing. PM: Project administration, Supervision, Writing – review & editing. PD: Supervision, Writing – review & editing. BK: Conceptualization, Project administration, Writing – review & editing.
Funding
The author(s) declare that no financial support was received for the research, authorship, and/or publication of this article.
Conflict of interest
The authors declare that the research was conducted in the absence of any commercial or financial relationships that could be construed as a potential conflict of interest.
Publisher’s note
All claims expressed in this article are solely those of the authors and do not necessarily represent those of their affiliated organizations, or those of the publisher, the editors and the reviewers. Any product that may be evaluated in this article, or claim that may be made by its manufacturer, is not guaranteed or endorsed by the publisher.
References
1. Sung H, Ferlay J, Siegel RL, Laversanne M, Soerjomataram I, Jemal A, et al. Global cancer statistics 2020: GLOBOCAN estimates of incidence and mortality worldwide for 36 cancers in 185 countries. CA Cancer J Clin. (2021) 71:209–49. doi: 10.3322/caac.21660
2. Piergentili R, Zaami S, Cavaliere AF, Signore F, Scambia G, Mattei A, et al. Non-coding RNAs as prognostic markers for endometrial cancer. Int J Mol Sci. (2021) 22:3151. doi: 10.3390/ijms22063151
3. Siegel RL, Miller KD, Wagle NS, Jemal A. Cancer statistic. CA Cancer J Clin. (2023) 73:17–48. doi: 10.3322/caac.21763
4. Bokhman JV. Two pathogenetic types of endometrial carcinoma. Gynecologic Oncol. (1983) 15:10–7. doi: 10.1016/0090-8258(83)90111-7
5. Colombo N, Creutzberg C, Amant F, Bosse T, González-Martín A, Ledermann J, et al. ESMO-ESGO-ESTRO consensus conference on endometrial cancer: diagnosis, treatment and follow-up. Ann Oncology: Off J Eur Soc Med Oncol. (2016) 27:16–41. doi: 10.1093/annonc/mdv484
6. Berek JS, Matias-Guiu X, Creutzberg C, Fotopoulou C, Gaffney D, Kehoe S, et al. FIGO staging of endometrial cancer: 2023. Int J Gynaecology Obstetrics: Off Organ Int Fed Gynaecology Obstetrics. (2023) 162:383–945. doi: 10.1002/ijgo.14923
7. Coll-de la Rubia E, Martinez-Garcia E, Dittmar G, Gil-Moreno A, Cabrera S, Colas E. Prognostic biomarkers in endometrial cancer: A systematic review and meta-analysis. J Clin Med. (2020) 9:1900. doi: 10.3390/jcm9061900
8. Yao Y, Wang X, Guan J, Xie C, Zhang H, Yang J, et al. Metabolomic differentiation of benign vs Malignant pulmonary nodules with high specificity via high-resolution mass spectrometry analysis of patient sera. Nat Commun. (2023) 14:2339. doi: 10.1038/s41467-023-37875-1
9. Buergel T, Steinfeldt J, Ruyoga G, Pietzner M, Bizzarri D, Vojinovic D, et al. Metabolomic profiles predict individual multidisease outcomes. Nat Med. (2022) 28:2309–20. doi: 10.1038/s41591-022-01980-3
10. Liu S, Li W, Liang L, Zhou Y, Li Y. The regulatory relationship between transcription factor STAT3 and noncoding RNA. Cell Mol Biol Lett. (2024) 29:4. doi: 10.1186/s11658-023-00521-1
11. Chen G, Huang AC, Zhang W, Zhang G, Wu M, Xu W, et al. Exosomal PD-L1 contributes to immunosuppression and is associated with anti-PD-1 response. Nature. (2018) 560:382–86. doi: 10.1038/s41586-018-0392-8
12. Eritja N, Navaridas R, Ruiz-Mitjana A, Vidal-Sabanés M, Egea J, Encinas M, et al. Endometrial PTEN deficiency leads to SMAD2/3 nuclear translocation. Cancers (Basel). (2021) 13:4990. doi: 10.3390/cancers13194990
13. Frąszczak K, Barczyński B. Characteristics of cancer stem cells and their potential role in endometrial cancer. Cancers (Basel). (2024) 16:1083. doi: 10.3390/cancers16061083
14. Vallone C, Rigon G, Gulia C, Baffa A, Votino R, Morosetti G, et al. Non-coding RNAs and endometrial cancer. Genes. (2018) 9:187. doi: 10.3390/genes9040187
15. Ling H, Vincent K, Pichler M, Fodde R, Berindan-Neagoe I, Slack FJ, et al. Junk DNA and the long non-coding RNA twist in cancer genetics. Oncogene. (2015) 34:5003–11. doi: 10.1038/onc.2014.456
16. Muhammad N, Bhattacharya S, Steele R, Ray RB. Anti-miR-203 suppresses ER-positive breast cancer growth and stemness by targeting SOCS3. Oncotarget. (2016) 7 (36):58595–605 doi: 10.18632/oncotarget.11193
17. Shekibi M, Heng S, Nie G. MicroRNAs in the regulation of endometrial receptivity for embryo implantation. Int J Mol Sci. (2022) 23:6210. doi: 10.3390/ijms23116210
18. Wang X, Wendel JRH, Emerson RE, Broaddus RR, Creighton CJ, Rusch DB, et al. “Pten and dicer1 loss in the mouse uterus causes poorly differentiated endometrial adenocarcinoma. Oncogene. (2020) 39:6286–995. doi: 10.1038/s41388-020-01434-5
19. Zhang C, Ji Q, Yang Y, Li Qi, Wang Z. Exosome: function and role in cancer metastasis and drug resistance. Technol Cancer Res Treat. (2018) 17:1533033818763450. doi: 10.1177/1533033818763450
20. Park Y, Lee K, Kim SW, Lee MW, Kim B, Lee SG. Effects of induced exosomes from endometrial cancer cells on tumor activity in the presence of aurea helianthus extract. Molecules (Basel Switzerland). (2021) 26:2207. doi: 10.3390/molecules26082207
21. Krylova SV, Feng D. The machinery of exosomes: biogenesis, release, and uptake. Int J Mol Sci. (2023) 24:1337. doi: 10.3390/ijms24021337
22. Crosbie EJ, Kitson SJ, McAlpine JN, Mukhopadhyay A, Powell ME, Singh N. Endometrial cancer. Lancet (London England). (2022) 399:1412–285. doi: 10.1016/S0140-6736(22)00323-3
23. Ferlay J, Ervik M, Lam F, Laversanne M, Colombet M, Mery L, et al. Global Cancer Observatory: Cancer Today. Lyon, France: International Agency for Research on Cancer (2024).
24. Ferlay J, Colombet M, Soerjomataram I, Mathers C, Parkin DM, Piñeros M, et al. Estimating the global cancer incidence and mortality in 2018: GLOBOCAN sources and methods. Int J Cancer. (2019) 144:1941–53. doi: 10.1002/ijc.31937
25. Oaknin A, Bosse TJ, Creutzberg CL, Giornelli G, Harter P, Joly F, et al. Endometrial cancer: ESMO clinical practice guideline for diagnosis, treatment and follow-up. Ann Oncology: Off J Eur Soc Med Oncol. (2022) 33:860–775. doi: 10.1016/j.annonc.2022.05.009
26. Wojciechowska U, Barańska K, Miklewska M, Didkowska JA. Cancer incidence and mortality in Poland in 2020. Nowotwory J Oncol. (2023) 73:129–45. doi: 10.5603/NJO.2023.0026
27. Arthur RS, Kabat GC, Kim MY, Wild RA, Shadyab AH, Wactawski-Wende J, et al. Metabolic syndrome and risk of endometrial cancer in postmenopausal women: A prospective study. Cancer Causes Control: CCC. (2019) 30:355–63. doi: 10.1007/s10552-019-01139-5
28. Soslow RA, Tornos C, Park KJ, Malpica A, Matias-Guiu X, Oliva E, et al. Endometrial carcinoma diagnosis: use of FIGO grading and genomic subcategories in clinical practice: recommendations of the international society of gynecological pathologists. Int J Gynecological Pathology: Off J Int Soc Gynecological Pathologists. (2019) 38 Suppl 1:S64–74. doi: 10.1097/PGP.0000000000000518
29. Domchek SM, Robson ME. “Update on genetic testing in gynecologic cancer. J Clin Oncol. (2019) 37:2501–95. doi: 10.1200/JCO.19.00363
30. Mukherjee P, Roy S, Ghosh D, Nandi SK. Role of animal models in biomedical research: a review. Lab Anim Res. (2022) 38. doi: 10.1186/s42826-022-00128-1
31. Cho SY. Patient-derived xenografts as compatible models for precision oncology. Lab Anim Res. (2020) 36:14. doi: 10.1186/s42826-020-00045-1
32. Imai T, Yoshida H, Machida Y, Kuramochi M, Ichikawa H, Kubo T, et al. Alteration in molecular properties during establishment and passaging of endometrial carcinoma patient-derived xenografts. Sci Rep. (2023) 13(1):8511. doi: 10.1038/s41598-023-35703-6
33. Kato S, Fushimi K, Yabuki Y, Maru Y, Hasegawa S, Matsuura T, et al. Precision modeling of gall bladder cancer patients in mice based on orthotopic implantation of organoid-derived tumor buds. Oncogenesis. (2021) 10:1–13. doi: 10.1038/s41389-021-00322-1
34. Maru Y, Hippo Y. Two-way development of the genetic model for endometrial tumorigenesis in mice: current and future perspectives. Front Genet. (2021) 12:798628. doi: 10.3389/fgene.2021.798628
35. Wang J, Jia N, Lyv T, Wang C, Tao X, Wong KK, et al. Paired box 2 promotes progression of endometrial cancer via regulating cell cycle pathway. J Cancer. (2018) 9:3743–54. doi: 10.7150/jca.22418
36. Wang L, Zuo X, Xie K, Wei D. The role of CD44 and cancer stem cells. Methods Mol Biol. (2018) 1692:31–42. doi: 10.1007/978-1-4939-7401-6_3
37. Khizer K, Padda J, Khedr A, Tasnim F, Al-Ewaidat OA, Patel V, et al. Paired-box gene 8 (PAX8) and its association with epithelial carcinomas. Cureus. (2021) 13(8):e17208. doi: 10.7759/cureus.17208
38. Monsivais D, Peng J, Kang Y, Matzuk MM. Activin-like kinase 5 (ALK5) inactivation in the mouse uterus results in metastatic endometrial carcinoma. Proc Natl Acad Sci United States America. (2019) 116:3883–92. doi: 10.1073/pnas.1806838116
39. Ruiz-Mitjana A, Vidal-Sabanés M, Navaridas Raúl, Perramon-Güell A, Yeramian A, Nicholson-Sabaté N, et al. Metformin exhibits antineoplastic effects on pten-deficient endometrial cancer by interfering with TGF-β and P38/ERK MAPK signalling. Biomedicine Pharmacotherapy = Biomedecine Pharmacotherapie. (2023) 168:115817. doi: 10.1016/j.biopha.2023.115817
40. Fang X, Ni N, Wang X, Tian Y, Ivanov I, Rijnkels M, et al. “EZH2 and endometrial cancer development: insights from a mouse model. Cells. (2022) 11:9095. doi: 10.3390/cells11050909
41. Franklin MR, Platero S, Saini KS, Curigliano G, Anderson S. Immuno-oncology trends: Preclinical models, biomarkers, and clinical development. J ImmunoTherapy Cancer. (2022) 10(1):e003231. doi: 10.1136/jitc-2021-003231
42. Nyen T, Moiola CP, Colas E, Annibali D, Amant F. Modeling endometrial cancer: Past, present, and future. Int J Mol Sci. (2018) 19(8):2348. doi: 10.3390/ijms19082348
43. Espedal H, Berg HF, Fonnes T, Fasmer KE, Krakstad C, Haldorsen IS. Feasibility and utility of MRI and dynamic 18F-FDG-PET in an orthotopic organoid-based patient-derived mouse model of endometrial cancer. J Trans Med. (2021) 19(1):406. doi: 10.1186/s12967-021-03086-9
44. Espedal H, Fonnes T, Fasmer KE, Krakstad C, Haldorsen IS. Imaging of preclinical endometrial cancer models for monitoring tumor progression and response to targeted therapy. Cancers. (2019) 11(12):1885. doi: 10.3390/cancers11121885
45. Chen J, Dai S, Zhao L, Peng Y, Sun C, Peng H, et al. A new type of endometrial cancer models in mice revealing the functional roles of genetic drivers and exploring their susceptibilities. Advanced Science. (2023) 10(24):e2300383. doi: 10.1002/advs.202300383
46. Yu K, Huang Z-Y, Xu X-L, Li J, Fu X-W, Deng S-L. Estrogen receptor function: impact on the human endometrium. Front Endocrinol. (2022) 13:827724. doi: 10.3389/fendo.2022.827724
47. Prossnitz ER, Arterburn JB, Smith HO, Oprea TI, Sklar LA, Hathaway HJ. Estrog en signaling through the transmembrane G protein-coupled receptor GPR30. Annu Rev Physiol. (2008) 70:165–90. doi: 10.1146/annurev.physiol.70.113006.100518
48. Wang C, Prossnitz ER, Roy SK. Expression of G protein-coupled receptor 30 in the hamster ovary: differential regulation by gonadotropins and steroid hormones. Endocrinology. (2007) 148:4853–64. doi: 10.1210/en.2007-0727
49. Otto C, Fuchs I, Kauselmann G, Kern H, Zevnik B, Andreasen P, et al. GPR30 does not mediate estrogenic responses in reproductive organs in mice. Biol Reprod. (2009) 80:34–41. doi: 10.1095/biolreprod.108.071175
50. Mamat Yusof MN, Chew KT, Kampan NC, Shafiee MN. Expression of PD-1 and PD-L1 in endometrial cancer: molecular and clinical significance. Int J Mol Sci. (2023) 24:15233. doi: 10.3390/ijms242015233
51. Wang J, Song T, Zhou S, Kong X. YAP promotes the Malignancy of endometrial cancer cells via regulation of IL-6 and IL-11. Mol Med. (2019) 25:32. doi: 10.1186/s10020-019-0103-4
52. Khan TH, Srivastava N, Srivastava A, Sareen A, Mathur RK, Chande AG, et al. SHP-1 plays a crucial role in CD40 signaling reciprocity. J Immunol. (2014) 193:3644–53. doi: 10.4049/jimmunol.1400620
53. Murugaiyan G, Martin S, Saha B. CD40-induced countercurrent conduits for tumor escape or elimination? Trends Immunol. (2007) 28:467–73. doi: 10.1016/j.it.2007.08.010
54. Dumas G, Dufresne M, Asselin É, Girouard J, Carrier C, Reyes-Moreno C. CD40 pathway activation reveals dual function for macrophages in human endometrial cancer cell survival and invasion. Cancer Immunol Immunother. (2013) 62:273–83. doi: 10.1007/s00262-012-1333-2
55. Zhou Y, Richmond A, Yan C. Harnessing the potential of CD40 agonism in cancer therapy. Cytokine Growth Factor Rev. (2024) 75:40–56. doi: 10.1016/j.cytogfr.2023.11.002
56. Zhang X, Kiapour N, Kapoor S, Khan T, Thamilarasan M, Tao Y, et al. IL-11 induces encephalitogenic th17 cells in multiple sclerosis and experimental autoimmune encephalomyelitis. J Immunol. (2019) 203:1142–50. doi: 10.4049/jimmunol.1900311
57. Seyedsadr M, Wang Y, Elzoheiry M, Shree Gopal S, Jang S, Duran G, et al. IL-11 induces NLRP3 inflammasome activation in monocytes and inflammatory cell migration to the central nervous system. Proc Natl Acad Sci U S A. (2023) 120:e2221007120. doi: 10.1073/pnas.2221007120
58. Ray I, Meira LB, Michael A, Ellis PE. Adipocytokines and disease progression in endometrial cancer: a systematic review. Cancer Metastasis Rev. (2022) 41:211–42. doi: 10.1007/s10555-021-10002-6
59. Tarique M, Naz H, Suhail M, Turan A, Saini C, Muhammad N, et al. Differential expression of programmed death 1 (PD-1) on various immune cells and its role in human leprosy. Front Immunol. (2023) 14:1138145. doi: 10.3389/fimmu.2023.1138145
60. Simon S, Labarriere N. PD-1 expression on tumor-specific T cells: Friend or foe for immunotherapy? Oncoimmunology. (2017) 7:e1364828. doi: 10.1080/2162402X.2017.1364828
61. Shen X, Zhang L, Li J, Li Y, Wang Y, Xu ZX. Recent findings in the regulation of programmed death ligand 1 expression. Front Immunol. (2019) 10:1337. doi: 10.3389/fimmu.2019.01337
62. Han Y, Liu D, Li L. PD-1/PD-L1 pathway: current researches in cancer. Am J Cancer Res. (2020) 10:727–42.
63. Bellucci R, Martin A, Bommarito D, Wang K, Hansen SH, Freeman GJ, et al. Interferon-γ-induced activation of JAK1 and JAK2 suppresses tumor cell susceptibility to NK cells through upregulation of PD-L1 expression. Oncoimmunology. (2015) 4:e1008824. doi: 10.1080/2162402X.2015.1008824
64. Nunes-Xavier CE, Angulo JC, Pulido R, López JI. A critical insight into the clinical translation of PD-1/PD-L1 blockade therapy in clear cell renal cell carcinoma. Curr Urol Rep. (2019) 20:1. doi: 10.1007/s11934-019-0866-8
65. Ren D, Hua Y, Yu B, Ye X, He Z, Li C, et al. Predictive biomarkers and mechanisms underlying resistance to PD1/PD-L1 blockade cancer immunotherapy. Mol Cancer. (2020) 19:19. doi: 10.1186/s12943-020-1144-6
66. June CH, O'Connor RS, Kawalekar OU, Ghassemi S, Milone MC. CAR T cell immunotherapy for human cancer. Science. (2018) 359:1361–5. doi: 10.1126/science.aar6711
67. Sadelain M, Brentjens R, Riviere I. 2013. The basic principles of chimeric antigen receptor (CAR) design.”. Cancer Disc. (2013) 3 (4):388–98 doi: 10.1158/2159-8290.CD-12-0548
68. Khan TH, Muhammad N, Tarique M, Usmani D, Sarode A.Y, Naz H. The role of cancer-specific target antigens in CAR T cell therapy in hematological Malignancies. Curr Tissue Microenviron Rep. (2024) 5(2):1–7. doi: 10.1007/s43152-024-00055-4
69. Rafiq S, Hackett CS, Brentjens RJ. Engineering strategies to overcome the current roadblocks in CAR T cell therapy. Nat Rev Clin Oncol. (2020) 17:147–67. doi: 10.1038/s41571-019-0297-y
70. Yeware A, Helton A, Dong Y, Dong C, Pritchard J, Mineishi S, et al. Novel fourth generation-like CARmiR cells release therapeutic miRNA via exosomes and enhance glioblastoma cell killing activity. Biochem Eng J. (2023) 199:109068. doi: 10.1016/j.bej.2023.109068
71. Wang L, Bu P, Ai Y, Srinivasan T, Chen HJ, Xiang K, et al. A long non-coding RNA targets microRNA miR-34a to regulate colon cancer stem cell asymmetric division. Elife. (2016) 5:e14620. doi: 10.7554/eLife.14620
72. Li WJ, Wang Y, Liu R, Kasinski AL, Shen H, Slack FJ, et al. MicroRNA-34a: potent tumor suppressor, cancer stem cell inhibitor, and potential anticancer therapeutic. Front Cell Dev Biol. (2021) 9:640587. doi: 10.3389/fcell.2021.640587
73. Papaccio F, Paino F, Regad T, Papaccio G, Desiderio V, Tirino V. Concise review: cancer cells, cancer stem cells, and mesenchymal stem cells: influence in cancer development. Stem Cells Transl Med. (2017) 6:2115–25. doi: 10.1002/sctm.17-0138
74. Adcock IM, Caramori G, Barnes PJ. Chronic obstructive pulmonary disease and lung cancer: new molecular insights. Respiration. (2011) 81:265–84. doi: 10.1159/000324601
75. Yan X, Luo H, Zhou X, Zhu B, Wang Y, Bian X. "Identification of CD90 as a marker for lung cancer stem cells in A549 and H446 cell lines". Oncol Rep. (2013) 30(6):2733–40. doi: 10.3892/or.2013.2784
76. Cao M, Liu Z, You D, Pan Y, Zhang Q. TMT-based quantitative proteomic analysis of spheroid cells of endometrial cancer possessing cancer stem cell properties. Stem Cell Res Ther. (2023) 14:119. doi: 10.1186/s13287-023-03348-x
77. Das S, Khan TH, Sarkar D. Comprehensive review on the effect of stem cells in cancer progression. Curr Tissue Microenviron Rep. (2024) 5:39–59. doi: 10.1007/s43152-024-00053-6
78. Hu Y, Dong Z, Liu K. Unraveling the complexity of STAT3 in cancer: molecular understanding and drug discovery. J Exp Clin Cancer research : CR. (2024) 43:23. doi: 10.1186/s13046-024-02949-5
79. Sun J, Du Y, Song Q, Nan J, Guan P, Guo J, et al. E2F is required for STAT3-mediated upregulation of cyclin B1 and cdc2 expressions and contributes to G2-M phase transition. Acta Biochim Et Biophys Sin. (2019) 51:313–22. doi: 10.1093/abbs/gmy174
80. Shen L, Zhang C, Cui K, Liang X, Zhu G. Low-density lipoprotein contributes to endometrial carcinoma cell proliferation, migration, and invasion by activating the JAK-STAT signaling pathway. Analytical Cell Pathol (Amsterdam). (2023) 2023:4015167. doi: 10.1155/2023/4015167
81. Wang J, He X, Jia Z, Yan A, Xiao K, Liu S, et al. Shenqi fuzheng injection restores the sensitivity to gefitinib in non-small cell lung cancer by inhibiting the IL-22/STAT3/AKT pathway. Pharm Biol. (2024) 62:33–41. doi: 10.1080/13880209.2023.2292266
82. Wang L, Lyu C, Stadlbauer B, Buchner A, Nößner E, Pohla H. Berbamine targets cancer stem cells and reverses cabazitaxel resistance via inhibiting IGF2BP1 and P-STAT3 in prostate cancer. Prostate. (2024) 84:131–47. doi: 10.1002/pros.24632
83. Luo Xu, Ye Z, Xu C, Chen H, Dai S, Chen W, et al. Corosolic acid enhances oxidative stress-induced apoptosis and senescence in pancreatic cancer cells by inhibiting the JAK2/STAT3 pathway. Mol Biol Rep. (2024) 51:176. doi: 10.1007/s11033-023-09105-w
84. Solaimuthu B, Khatib A, Tanna M, Karmi A, Hayashi A, Rmaileh AA, et al. The exostosin glycosyltransferase 1/STAT3 axis is a driver of breast cancer aggressiveness. Proc Natl Acad Sci United States America. (2024) 121:e2316733121. doi: 10.1073/pnas.2316733121
85. Garg M, Shanmugam MK, Bhardwaj V, Goel A, Gupta R, Sharma A, et al. “The pleiotropic role of transcription factor STAT3 in oncogenesis and its targeting through natural products for cancer prevention and therapy. Medicinal Res Reviews grudzień. (2020). doi: 10.1002/med.21761
86. Che Q, Xiao X, Liu M, Lu Y, Dong X, Liu S. IL-6 promotes endometrial cancer cells invasion and migration through signal transducers and activators of transcription 3 signaling pathway. Pathol Res Pract. (2019) 215:152392. doi: 10.1016/j.prp.2019.03.020
87. Richenberg G, Francis A, Owen CN, Gray V, Robinson T, Gabriel AA, et al. The tumor multi-omic landscape of endometrial cancers developed on a germline genetic background of adiposity. medRxiv [Preprint]. (2023) 10:2023.10.09.23296765. doi: 10.1101/2023.10.09.23296765
88. Mahon KL, Sutherland SI, Lin HM, Stockler MR, Gurney H, Mallesara G, et al. Clinical validation of circulating GDF15/MIC-1 as a marker of response to docetaxel and survival in men with metastatic castration-resistant prostate cancer. Prostate. (2024) 84:747–55. doi: 10.1002/pros.24691
89. Krishnamoorthy K, Natarajan SR, Veeraraghavan VP, Jayaraman S. Blueberry extract and its bioactive compounds mitigate oxidative stress and suppress human lung cancer cell (A549) growth by modulating the expression of P53/EGFR/STAT3/IL6-mediated signaling molecules. Cell Biochem Funct. (2024) 42:e4027. doi: 10.1002/cbf.4027
90. Xue Z, Li X, Zhang Y, Cao C, Zhou Q. IL6 induces mtDNA leakage to affect the immune escape of endometrial carcinoma via cGAS-STING. J Immunol Res. (2022) 2022:3815853. doi: 10.1155/2022/3815853
91. Olivera M, Živković E, Subotički T, Diklić M, Đikić D, Vukotić M, et al. Inflammation mediated angiogenesis in chronic lymphocytic leukemia. Ann Hematol. (2024) 103:2865–75. doi: 10.1007/s00277-024-05781-1
92. Tolomeo M, Cascio A. The multifaced role of STAT3 in cancer and its implication for anticancer therapy. Int J Mol Sci. (2021) 22:603. doi: 10.3390/ijms22020603
93. Wu M, Song D, Li H, Yang Y, Ma X, Deng Sa, et al. Negative regulators of STAT3 signaling pathway in cancers. Cancer Manage Res. (2019) 11:4957–69. doi: 10.2147/CMAR.S206175
94. Li W, Lee M-R, Kim T, Kim YW, Cho M-Y. Activated STAT3 may participate in tumor progression through increasing CD133/survivin expression in early stage of colon cancer. Biochem Biophys Res Commun. (2018) 497:354–61. doi: 10.1016/j.bbrc.2018.02.084
95. Luna GCH, Imasa MS, Juat N, Hernandez KV, Sayo TM, Cristal-Luna G, et al. Expression landscapes in non-small cell lung cancer shaped by the thyroid transcription factor 1. Lung Cancer (Amsterdam Netherlands). (2023) 176:121–31. doi: 10.1016/j.lungcan.2022.12.015
96. Chu Y, Wang Y, Peng W, Xu L, Liu M, Li J, et al. STAT3 activation by IL-6 from adipose-derived stem cells promotes endometrial carcinoma proliferation and metastasis. Biochem Biophys Res Commun. (2018) 500:626–31. doi: 10.1016/j.bbrc.2018.04.121
97. Chen J, Huang S, Li H, Li Y, Zeng H, Hu J, et al. STAT3 inhibitor BBI608 reduces patient-specific primary cell viability of cervical and endometrial cancer at a clinical-relevant concentration. Clin Trans Oncology: Off Publ Fed Spanish Oncol Societies Natl Cancer Institute Mexico. (2023) 25:662–72. doi: 10.1007/s12094-022-02970-0
98. Gao J, Tian J, Lv Y, Shi F, Kong F, Shi H, et al. Leptin induces functional activation of cyclooxygenase-2 through JAK2/STAT3, MAPK/ERK, and PI3K/AKT pathways in human endometrial cancer cells. Cancer Sci. (2009) 100:389–95. doi: 10.1111/j.1349-7006.2008.01053.x
99. Liu H, Li C, Yang J, Sun Y, Zhang S, Yang J, et al. Long noncoding RNA CASC9/miR-519d/STAT3 positive feedback loop facilitate the glioma tumourigenesis. J Cell Mol Med. (2018) 22:6338–44. doi: 10.1111/jcmm.13932
100. Dong P, Xiong Y, Yue J, Hanley SJB, Kobayashi N, Todo Y, et al. Exploring lncRNA-mediated regulatory networks in endometrial cancer cells and the tumor microenvironment: advances and challenges. Cancers. (2019b) 11:234. doi: 10.3390/cancers11020234
101. Ahn J-H, Choi YS, Choi J-H. Leptin promotes human endometriotic cell migration and invasion by up-regulating MMP-2 through the JAK2/STAT3 signaling pathway. Mol Hum Reprod. (2015) 21:792–802. doi: 10.1093/molehr/gav039
102. Esposito G, Bravi F, Serraino D, Parazzini F, Crispo A, Augustin LSA, et al. “Diabetes risk reduction diet and endometrial cancer risk. Nutrients. (2021) 13:26305. doi: 10.3390/nu13082630
103. Stenckova M, Liu Y, Nekulova M, Holcakova J, Pokorna Z, Nenutil R, et al. ΔNp63 is regulated by insulin/IGF-1 signaling in normal basal/progenitor mammary cells and in luminal-type breast cancer cells. Neoplasma. (2023) 70:621–32. doi: 10.4149/neo_2023_230719N376
104. Franchi M, Asciutto R, Nicotra F, Merlino L, Vecchia CLa, Corrao G, et al. Metformin, other antidiabetic drugs, and endometrial cancer risk: A nested case-control study within italian healthcare utilization databases. Eur J Cancer Prev. (2017) 26:225–31. doi: 10.1097/CEJ.0000000000000235
105. Wallbillich JJ, Josyula S, Saini U, Zingarelli RA, Dorayappan KDP, Riley MK, et al. “High glucose-mediated STAT3 activation in endometrial cancer is inhibited by metformin: therapeutic implications for endometrial cancer. PloS One. (2017) 12:e01703185. doi: 10.1371/journal.pone.0170318
106. Tzavlaki K, Moustakas A. TGF-β Signaling. Biomolecules. (2020) 10:487. doi: 10.3390/biom10030487
107. Eritja N, Felip I, Dosil MA, Vigezzi L, Mirantes C, Yeramian A, et al. A Smad3-PTEN regulatory loop controls proliferation and apoptotic responses to TGF-β in mouse endometrium. Cell Death Differentiation. (2017) 24:1443–58. doi: 10.1038/cdd.2017.73
108. Pisklova M, Osmak G, Favorova O. Regulation of SMAD signaling pathway by miRNAs associated with myocardial fibrosis: in silico analysis of target gene networks. Biochem Biokhimiia. (2022) 87:832–38. doi: 10.1134/S0006297922080144
109. Chaudhary R, Goodman LS, Wang S, Asimakopoulos A, Weiskirchen R, Dooley S, et al. “Cholesterol modulates type I/II TGF-β Receptor complexes and alters the balance between smad and akt signaling in hepatocytes. Commun Biol. (2024) 7:85. doi: 10.1038/s42003-023-05654-9
110. Liu J-L, He J-P, Zhu C, Cheng H-Z. Endometrial carcinoma may favor partial, but not complete, loss of the TGF-β signaling pathway. Proc Natl Acad Sci United States America. (2019) 116:9164–65. doi: 10.1073/pnas.1903646116
111. Fukuda T, Suzuki E, Fukuda R. Bone morphogenetic protein signaling is a possible therapeutic target in gynecologic cancer. Cancer Sci. (2022) 114:722–29. doi: 10.1111/cas.15682
112. Safavi P, Moghadam KB, Haghighi Z, Ferns GA, Rahmani F. “Interplay between lncRNA/miRNA and TGF-β Signaling in the tumorigenesis of gynecological cancer. Curr Pharm Design luty. (2024) 30(5):352–61. doi: 10.2174/0113816128284380240123071409
113. Li Y, Liu Q, McGrail DJ, Dai H, Li K, Lin S-Y. CHD4 mutations promote endometrial cancer stemness by activating TGF-beta signaling. Am J Cancer Res. (2018) 8:903–14.
114. Wang P, Zhang T, Jiang N, Wang K, Feng L, Liu T, et al. PDIA6, which is regulated by TRPM2-AS/miR-424-5p axis, promotes endometrial cancer progression via TGF-beta pathway. Cell Death Dis. (2023) 14:1–12. doi: 10.1038/s41419-023-06297-8
115. Konno T, Kohno T, Kikuchi S, Kura A, Saito K, Okada T, et al. The interplay between the epithelial permeability barrier, cell migration and mitochondrial metabolism of growth factors and their inhibitors in a human endometrial carcinoma cell line. Tissue Barriers. (2024) 0:2304443. doi: 10.1080/21688370.2024.2304443
116. Formenti SC, Lee P, Adams S, Goldberg JD, Li X, Xie MW, et al. Focal irradiation and systemic TGFβ Blockade in metastatic breast cancer. Clin Cancer Res. (2018) 24:2493–504. doi: 10.1158/1078-0432.CCR-17-3322
117. Liu J, Tian T, Liu X, Cui Z. BCHE as a prognostic biomarker in endometrial cancer and its correlation with immunity. J Immunol Res. (2022) 2022:6051092. doi: 10.1155/2022/6051092
118. Xu H, Yin Y, Li Y, Shi N, Xie W, Luo W, et al. FLOT2 promotes nasopharyngeal carcinoma progression through suppression of TGF-β pathway via facilitating CD109 expression. iScience. (2023) 27:108580. doi: 10.1016/j.isci.2023.108580
119. Jin X, Xu H, Hu Q, Yin Y, Qin M, Xia Z. Early growth response 2, a novel target of pelvic organ prolapse, is highly expressed in anterior vaginal wall tissues with pelvic organ prolapse. Histochem Cell Biol. (2024) 161:195–205. doi: 10.1007/s00418-023-02240-2
120. Gao Q, Huang Q, Li F, Luo F. LncRNA MCTP1-AS1 regulates EMT process in endometrial cancer by targeting the miR-650/SMAD7 axis. OncoTargets Ther. (2021) 14:751–61. doi: 10.2147/OTT.S240010
121. Zhao T, Sun R, Ma X, Wei L, Hou Y, Song K, et al. Overexpression of LPCAT1 enhances endometrial cancer stemness and metastasis by changing lipid components and activating TGF-β/Smad2/3 signaling pathway. Acta Biochim Biophys Sin. (2022) 54:904–16. doi: 10.3724/abbs.2022076
122. Frank D, Moussi CJ, Ulferts S, Lorenzen L, Schwan C, Grosse R. Vesicle-associated actin assembly by formins promotes TGFβ-induced ANGPTL4 trafficking, secretion and cell invasion. Advanced Sci (Weinheim Baden-Wurttemberg Germany). (2023) 10:e2204896. doi: 10.1002/advs.202204896
123. Li X, Kaur N, Albahrani M, Karpf AR, Black AR, Black JD. Crosstalk between protein kinase C α and transforming growth factor β Signaling mediated by runx2 in intestinal epithelial cells. J Biol Chem. (2023) 299:1030175. doi: 10.1016/j.jbc.2023.103017
124. Driva TS, Schatz C, Sobočan M, Haybaeck J. “The Role of mTOR and eIF Signaling in Benign Endometrial Diseases. Int J Mol Sci. (2022) 23:34165. doi: 10.3390/ijms23073416
125. Zeng H, Lu Bo, Zamponi R, Yang Z, Wetzel K, Loureiro J, et al. mTORC1 signaling suppresses wnt/β-catenin signaling through DVL-dependent regulation of wnt receptor FZD level. Proc Natl Acad Sci United States America. (2018) 115:E10362–69. doi: 10.1073/pnas.1808575115
126. Fatima I, Barman S, Rai R, Thiel KW, Chandra V. “Targeting wnt signaling in endometrial cancer. Cancers. (2021) 13:23515. doi: 10.3390/cancers13102351
127. Pietrus M, Pitynski K, Waligora M, Milian-Ciesielska K, Ludwin A, Socha MW, et al. “Association of membranous WNT-1 and nuclear mTOR with endometrial cancer grade. Int J Mol Sci. (2023) 24:83425. doi: 10.3390/ijms24098342
128. Xu X, Kong X, Liu T, Zhou L, Wu J, Fu J, et al. Metastasis-Associated Protein 1, Modulated by miR-30c, Promotes Endometrial Cancer Progression through AKT/mTOR/4E-BP1 Pathway. Gynecologic Oncol. (2019) 154:207–17. doi: 10.1016/j.ygyno.2019.04.005
129. Jin Li, Feng X, Luo D. Adiponectin regulates the Malignant biological behavior of endometrial cancer cells via AMPK/mTOR signal pathway. Trop J Pharm Res. (2021) 20:933–38. doi: 10.4314/tjpr.v20i5.7
130. Alizadeh A, Goldeberg D. The role of key oncogenes in reprogramming of glycolysis in cancer cells; an overview. (2022). doi: 10.13140/RG.2.2.31466.59840/1
131. Liao J, Chen H, Qi M, Wang J, Wang M. MLLT11-TRIL complex promotes the progression of endometrial cancer through PI3K/AKT/mTOR signaling pathway. Cancer Biol Ther. (2022) 23:211–24. doi: 10.1080/15384047.2022.2046450
132. Deng B, Kong W, Suo H, Shen X, Newton MA, Burkett WC, et al. Oleic acid exhibits anti-proliferative and anti-invasive activities via the PTEN/AKT/mTOR pathway in endometrial cancer. Cancers. (2023) 15:5407. doi: 10.3390/cancers15225407
133. Yan Y, Wang S, Zhang Z, Tang M, Zhao AZ, Li Z, et al. FKBP38 suppresses endometrial cancer cell proliferation and metastasis by inhibiting the mTOR pathway. Arch Biochem Biophysics. (2024) 752:109891. doi: 10.1016/j.abb.2024.109891
134. Mitamura T, Dong P, Ihira K, Kudo M, Watari H. Molecular-targeted therapies and precision medicine for endometrial cancer. Japanese J Clin Oncol. (2019) 49:108–20. doi: 10.1093/jjco/hyy159
135. Yang X, Liu Z, Tang W, Pratap UP, Collier AB, Altwegg KA, et al. PELP1 inhibition by SMIP34 reduces endometrial cancer progression via attenuation of ribosomal biogenesis. Mol Oncol. (2023). doi: 10.1002/1878-0261.13539
136. McConechy MK, Ding J, Cheang MCu, Wiegand K, Senz J, Tone A, et al. “Use of mutation profiles to refine the classification of endometrial carcinomas. J Pathol. (2012) 228:20–305. doi: 10.1002/path.4056
137. Bhatti GK, Khullar N, Sidhu IS, Navik US, Reddy AP, Reddy PH. Emerging role of non-coding RNA in health and disease. Metab Brain Dis. (2021) 36:1119–34. doi: 10.1007/s11011-021-00739-y
138. Statello L, Guo C-J, Chen L-L, Huarte M. Gene regulation by long non-coding RNAs and its biological functions. Nat Rev Mol Cell Biol. (2021) 22:96–118. doi: 10.1038/s41580-020-00315-9
139. Panni S, Lovering RC, Porras P, Orchard S. Non-coding RNA regulatory networks. Biochim Et Biophys Acta Gene Regul Mech. (2020) 18636:194417. doi: 10.1016/j.bbagrm.2019.194417
140. Yan H, Bu P. Non-coding RNA in cancer. Essays Biochem. (2021) 65:625–39. doi: 10.1042/EBC20200032
141. Zhou X, Ao X, Jia Z, Li Y, Kuang S, Du C, et al. Non-coding RNA in cancer drug resistance: underlying mechanisms and clinical applications. Front Oncol. (2022) 12:951864. doi: 10.3389/fonc.2022.951864
142. Chen B, Dragomir MP, Yang C, Li Q, Horst D, Calin GA. Targeting non-coding RNAs to overcome cancer therapy resistance. Signal Transduction Targeted Ther. (2022) 7:1–205. doi: 10.1038/s41392-022-00975-3
143. Toden S, Zumwalt TJ, Goel A. Non-coding RNAs and potential therapeutic targeting in cancer. Biochim Biophys Acta Rev Cancer. (2021) 1875:188491. doi: 10.1016/j.bbcan.2020.188491
144. Zhu Y, Shen Y, Chen R, Li H, Wu Y, Zhang F, et al. KCNQ1OT1 lncRNA affects the proliferation, apoptosis, and chemoresistance of small cell lung cancer cells via the JAK2/STAT3 axis. Ann Trans Med. (2021) 9:891. doi: 10.21037/atm-21-1761
145. Dey P, Buragohain T, Das M, Banerjee S. Exploring the role of non-coding RNA mediated regulation of signaling pathways in endometrial cancer. Adv Cancer Biol - Metastasis. (2023) 9:100111. doi: 10.1016/j.adcanc.2023.100111
146. Li X, Jia L, Ouyang J, An Y, Luo G, Song R, et al. A PRISMA-compliant systematic review and meta-analysis of integrated chinese and western medicine in treating hepatitis C. Medicine. (2020) 99:e21825. doi: 10.1097/MD.0000000000021825
147. Li S, Shan Y, Li X, Zhang C, Wei S, Dai S, et al. lncRNA SNHG5 modulates endometrial cancer progression via the miR-25-3p/BTG2 axis. J Oncol. (2019) 2019:7024675. doi: 10.1155/2019/7024675
148. Liu Q-W, He Y, Xu WW. Molecular functions and therapeutic applications of exosomal noncoding RNAs in cancer. Exp Mol Med. (2022) 54:216–25. doi: 10.1038/s12276-022-00744-w
149. Liu Y, Yuan H, He T. Downregulated circular RNA hsa_circ_0005797 inhibits endometrial cancer by modulating microRNA-298/catenin delta 1 signaling. Bioengineered. (2022) 13:4634–45. doi: 10.1080/21655979.2021.2013113
150. Lai T, Qiu H, Si L, Zhen Yu, Chu D, Guo R. Long noncoding RNA BMPR1B-AS1 facilitates endometrial cancer cell proliferation and metastasis by sponging miR-7-2-3p to modulate the DCLK1/akt/NF-κB pathway. Cell Cycle (Georgetown Tex.). (2022) 21:1599–618. doi: 10.1080/15384101.2022.2060003
151. Chen S, Liang Y, Shen Y, Wang X. lncRNA XIST/miR-129-2-3p axis targets CCP110 to regulate the proliferation, invasion and migration of endometrial cancer cells. Exp Ther Med. (2023) 25:159. doi: 10.3892/etm.2023.11858
152. Guo J-L, Tang T, Li J-H, Yang Y-H, Zhang L, Quan Yi. LncRNA HEIH enhances paclitaxel-tolerance of endometrial cancer cells via activation of MAPK signaling pathway. Pathol Oncol Research: POR. (2020) 26:1757–66. doi: 10.1007/s12253-019-00718-w
153. Kim LK, Park S-A, Nam EJi, Kim YT, Heo T-H, Kim HJ. LncRNA SNHG4 modulates EMT signal and antitumor effects in endometrial cancer through transcription factor SP-1. Biomedicines. (2023) 11:1018. doi: 10.3390/biomedicines11041018
154. Kok VC, Yu C-C. Cancer-derived exosomes: their role in cancer biology and biomarker development. Int J Nanomedicine. (2020) 15:8019–36. doi: 10.2147/IJN.S272378
155. Han Q-F, Li W-J, Hu K-S, Gao J, Zhai W-L, Yang J-H, et al. Exosome biogenesis: machinery, regulation, and therapeutic implications in cancer. Mol Cancer. (2022) 21:207. doi: 10.1186/s12943-022-01671-0
156. Rajagopal C, Harikumar KB. The origin and functions of exosomes in cancer. Front Oncol. (2018) 8:66. doi: 10.3389/fonc.2018.00066
157. Zhou L, Wang W, Wang F, Yang S, Hu J, Lu B, et al. Plasma-derived exosomal miR-15a-5p as a promising diagnostic biomarker for early detection of endometrial carcinoma. Mol Cancer. (2021) 20:57. doi: 10.1186/s12943-021-01352-4
158. Chen CY, Chen J, He L, Stiles BL. PTEN: tumor suppressor and metabolic regulator. Front Endocrinol (Lausanne). (2018) 9:338. doi: 10.3389/fendo.2018.00338
159. Liu J, Ren L, Li S, Li W, Zheng X, Yang Y, et al. The biology, function, and applications of exosomes in cancer. Acta Pharm Sinica B. (2021) 11:2783–97. doi: 10.1016/j.apsb.2021.01.001
160. Zhang X, Sai B, Wang F, Wang L, Wang Y, Zheng L, et al. Hypoxic BMSC-Derived Exosomal miRNAs Promote Metastasis of Lung Cancer Cells via STAT3-Induced EMT. Mol Cancer. (2019) 18:40. doi: 10.1186/s12943-019-0959-5
161. Wu Q, Zhou L, Lv D, Zhu X, Tang H. Exosome-mediated communication in the tumor microenvironment contributes to hepatocellular carcinoma development and progression. J Hematol Oncol. (2019) 12:53. doi: 10.1186/s13045-019-0739-0
162. Steinbichler TB, Dudás József, Riechelmann H, Skvortsova I-I. The role of exosomes in cancer metastasis. Semin Cancer Biol. (2017) 44:170–81. doi: 10.1016/j.semcancer.2017.02.006
163. Bryja A, Zadka Łukasz, Farzaneh M, Zehtabi M, Ghasemian M, Dyszkiewicz-Konwińska M, et al. Small extracellular vesicles - A host for advanced bioengineering and «Trojan horse» of non-coding RNAs. Life Sci. (2023) 332:122126. doi: 10.1016/j.lfs.2023.122126
164. Shurtleff MJ, Temoche-Diaz MM, Karfilis KV, Ri S, Schekman R. Y-Box Protein 1 Is Required to Sort microRNAs into Exosomes in Cells and in a Cell-Free Reaction. eLife. (2016) 5:e19276. doi: 10.7554/eLife.19276
165. Zhang Yu, Wei Y-J, Zhang Y-F, Liu H-W, Zhang Y-F. Emerging functions and clinical applications of exosomal ncRNAs in ovarian cancer. Front Oncol. (2021) 11:765458. doi: 10.3389/fonc.2021.765458
166. Li X, Tang M. Exosomes Released from M2 Macrophages Transfer miR-221-3p Contributed to EOC Progression through Targeting CDKN1B. Cancer Med. (2020) 9:5976–88. doi: 10.1002/cam4.3252
167. Pan X, Hong X, Lai J, Cheng Lu, Cheng Y, Yao M, et al. Exosomal microRNA-221-3p confers adriamycin resistance in breast cancer cells by targeting PIK3R1. Front Oncol. (2020) 10:441. doi: 10.3389/fonc.2020.00441
168. Fan J-T, Zhou Z-Y, Luo Y-L, Luo Q, Chen S-B, Zhao J-C, et al. Exosomal lncRNA NEAT1 from Cancer-Associated Fibroblasts Facilitates Endometrial Cancer Progression via miR-26a/b-5p-Mediated STAT3/YKL-40 Signaling Pathway. Neoplasia (New York N.Y.). (2021) 23:692–703. doi: 10.1016/j.neo.2021.05.004
169. Xie H, Yao J, Wang Y, Ni B. Exosome-Transmitted circVMP1 Facilitates the Progression and Cisplatin Resistance of Non-Small Cell Lung Cancer by Targeting miR-524-5p-METTL3/SOX2 Axis. Drug Delivery. (2022) 29:1257–71. doi: 10.1080/10717544.2022.2057617
170. Tang X-H, Guo T, Gao X-Y, Wu X-L, Xing X-F, Ji J-F, et al. Exosome-derived noncoding RNAs in gastric cancer: functions and clinical applications. Mol Cancer. (2021) 20:99. doi: 10.1186/s12943-021-01396-6
171. Yang C, Wu S, Mou Z, Zhou Q, Dai X, Ou Y, et al. Exosome-derived circTRPS1 promotes Malignant phenotype and CD8+ T cell exhaustion in bladder cancer microenvironments. Mol Therapy: J Am Soc Gene Ther. (2022) 30:1054–70. doi: 10.1016/j.ymthe.2022.01.022
172. Lu Q, Wang X, Zhu Ji, Fei X, Chen H, Li C. Hypoxic tumor-derived exosomal circ0048117 facilitates M2 macrophage polarization acting as miR-140 sponge in esophageal squamous cell carcinoma. OncoTargets Ther. (2020) 13:1883–97. doi: 10.2147/OTT.S284192
173. Xu Z, Chen Yi, Ma L, Chen Y, Liu J, Guo Y, et al. Role of exosomal non-coding RNAs from tumor cells and tumor-associated macrophages in the tumor microenvironment. Mol Therapy: J Am Soc Gene Ther. (2022) 30:3133–54. doi: 10.1016/j.ymthe.2022.01.046
174. Yin Zi, Ma T, Huang B, Lin L, Zhou Yu, Yan J, et al. Macrophage-Derived Exosomal microRNA-501-3p Promotes Progression of Pancreatic Ductal Adenocarcinoma through the TGFBR3-Mediated TGF-β Signaling Pathway. J Exp Clin Cancer Research: CR. (2019) 38:310. doi: 10.1186/s13046-019-1313-x
175. Fang T, Lv H, Lv G, Li T, Wang C, Han Q, et al. Tumor-derived exosomal miR-1247-3p induces cancer-associated fibroblast activation to foster lung metastasis of liver cancer. Nat Commun. (2018) 9:191. doi: 10.1038/s41467-017-02583-0
176. Hashemipour M, Boroumand H, Mollazadeh S, Tajiknia V, Nourollahzadeh Z, Borj MR, et al. “Exosomal microRNAs and exosomal long non-coding RNAs in gynecologic cancers. Gynecologic Oncol. (2021) 161:314–275. doi: 10.1016/j.ygyno.2021.02.004
177. Sommella E, Capaci V, Aloisio M, Salviati E, Campiglia P, Molinario G, et al. A label-free proteomic approach for the identification of biomarkers in the exosome of endometrial cancer serum. Cancers. (2022) 14:6262. doi: 10.3390/cancers14246262
178. Maida Y, Takakura M, Nishiuchi T, Yoshimoto T, Kyo S. Exosomal transfer of functional small RNAs mediates cancer-stroma communication in human endometrium. Cancer Med. (2016) 5:304–14. doi: 10.1002/cam4.545
179. Esfandyari S, Elkafas H, Chugh RM, Park H-S, Navarro A, Al-Hendy A. Exosomes as biomarkers for female reproductive diseases diagnosis and therapy. Int J Mol Sci. (2021) 22:2165. doi: 10.3390/ijms22042165
180. Song Y, Wang M, Tong H, Tan Y, Hu X, Wang K, et al. Plasma exosomes from endometrial cancer patients contain LGALS3BP to promote endometrial cancer progression. Oncogene. (2021) 40:633–46. doi: 10.1038/s41388-020-01555-x
181. Sykaras AG, Christofidis K, Politi E, Theocharis S. Exosomes on endometrial cancer: A biomarkers treasure trove? Cancers. (2022) 14:17335. doi: 10.3390/cancers14071733
182. Zheng W, Yang J, Wang Y, Liu X. Exosomal miRNA-93 and miRNA-205 expression in endometrial cancer. J King Saud Univ - Sci. (2020) 32:1111–15. doi: 10.1016/j.jksus.2019.10.006
183. Zhang K, Cai Y, Zhou Qi, Sun H, Wei J. Long non-coding RNA SNHG14 impedes viability, migration and invasion of endometrial carcinoma cells through modulating miR-93-5p/ZBTB7A axis. Cancer Manage Res. (2020) 12:9515–25. doi: 10.2147/CMAR.S257419
184. Zhang Na, Wang Y, Liu H, Shen W. Extracellular vesicle encapsulated microRNA-320a inhibits endometrial cancer by suppression of the HIF1α/VEGFA axis. Exp Cell Res. (2020) 394:112113. doi: 10.1016/j.yexcr.2020.112113
185. Li B-L, Lu W, Qu J-J, Ye L, Du G-Q, Wan X-P. Loss of exosomal miR-148b from cancer-associated fibroblasts promotes endometrial cancer cell invasion and cancer metastasis. J Cell Physiol. (2019) 234:2943–53. doi: 10.1002/jcp.27111
186. Jing L, Hua Xu, Yuanna Du, Rukun Z, Junjun M. Exosomal miR-499a-5p Inhibits Endometrial Cancer Growth and Metastasis via Targeting VAV3. Cancer Manage Res. (2020) 12:13541–52. doi: 10.2147/CMAR.S283747
187. Xiao Li, He Y, Peng F, Yang J, Yuan C. Endometrial Cancer Cells Promote M2-Like Macrophage Polarization by Delivering Exosomal miRNA-21 under Hypoxia Condition. J Immunol Res. (2020) 2020:9731049. doi: 10.1155/2020/9731049
188. Wang Y, Ma H, Li Y, Su R. MiR-192-5p-modified tumor-associated macrophages-derived exosome suppressed endometrial cancer progression through targeting IRAK1/NF-κB signaling. Reprod Sci (Thousand Oaks Calif.). (2022) 29:436–47. doi: 10.1007/s43032-021-00789-8
189. Shi S, Tan Q, Feng F, Huang H, Liang J, Cao D, et al. Identification of core genes in the progression of endometrial cancer and cancer cell-derived exosomes by an integrative analysis. Sci Rep. (2020) 10:9862. doi: 10.1038/s41598-020-66872-3
190. Zhou W-J, Zhang J, Xie F, Wu J-N, Ye J-F, Wang J, et al. CD45RO-CD8+ T cell-derived exosomes restrict estrogen-driven endometrial cancer development via the ERβ/miR-765/PLP2/notch axis. Theranostics. (2021) 11:5330–45. doi: 10.7150/thno.58337
191. Zhou Y, Zhang Y, Gong H, Luo S, Cui Y. The role of exosomes and their applications in cancer. Int J Mol Sci. (2021) 22:12204. doi: 10.3390/ijms222212204
192. Wang J, Gong X, Yang L, Li L, Gao X, Ni T, et al. Loss of exosomal miR-26a-5p contributes to endometrial cancer lymphangiogenesis and lymphatic metastasis. Clin Trans Med. (2022) 12:e846. doi: 10.1002/ctm2.846
193. Che X, Jian F, Chen C, Liu C, Liu G, Feng W. PCOS serum-derived exosomal miR-27a-5p stimulates endometrial cancer cells migration and invasion. J Mol Endocrinol. (2020) 64:1–12. doi: 10.1530/JME-19-0159
194. Pan Y, Wang X, Li Y, Yan P, Zhang H. Human Umbilical Cord Blood Mesenchymal Stem Cells-Derived Exosomal microRNA-503-3p Inhibits Progression of Human Endometrial Cancer Cells through Downregulating MEST. Cancer Gene Ther. (2022) 29:1130–39. doi: 10.1038/s41417-021-00416-3
195. Fan X, Zou X, Liu C, Cheng W, Zhang S, Geng X, et al. MicroRNA expression profile in serum reveals novel diagnostic biomarkers for endometrial cancer. Bioscience Rep. (2021) 41:BSR20210111. doi: 10.1042/BSR20210111
196. Jia J, Guo S, Zhang D, Tian X, Xie X. Exosomal-lncRNA DLEU1 accelerates the proliferation, migration, and invasion of endometrial carcinoma cells by regulating microRNA-E2F3. OncoTargets Ther. (2020) 13:8651–63. doi: 10.2147/OTT.S262661
197. Qiu J, Hua K. Exosomal long noncoding RNA-NONHAT076754 faciliates endometriosis invasion and predicts endometriosis recurrence. J Minimally Invasive Gynecology. (2019) 26:S12. doi: 10.1016/j.jmig.2019.09.044
198. Xu H, Gong Z, Shen Y, Fang Y, Zhong S. Circular RNA expression in extracellular vesicles isolated from serum of patients with endometrial cancer. Epigenomics. (2018) 10:187–97. doi: 10.2217/epi-2017-0109
199. Gu X, Shi Y, Dong M, Jiang Li, Yang J, Liu Z. Exosomal transfer of tumor-associated macrophage-derived hsa_circ_0001610 reduces radiosensitivity in endometrial cancer. Cell Death Dis. (2021) 12:818. doi: 10.1038/s41419-021-04087-8
200. Qian T, Yu X, Xu A, Li H, Chen W, Zhong S. tRF-20-S998LO9D inhibits endometrial carcinoma by upregulating SESN2. Epigenomics. (2022) 14:1563–77. doi: 10.2217/epi-2022-0349
201. Liu Y, Bailey JT, Abu-Laban M, Li S, Chen C, Glick AB, et al. Photocontrolled miR-148b nanoparticles cause apoptosis, inflammation and regression of ras induced epidermal squamous cell carcinomas in mice. Biomaterials. (2020) 256:120212. doi: 10.1016/j.biomaterials.2020.120212
202. Doyle LM, Wang MZ. “Overview of extracellular vesicles, their origin, composition, purpose, and methods for exosome isolation and analysis. Cells. (2019) 8:7275. doi: 10.3390/cells8070727
203. Chen S, Lu H, Jiang S, Li M, Weng H, Zhu J, et al. An analysis of clinical characteristics and prognosis of endometrioid ovarian cancer based on the SEER database and two centers in China. BMC Cancer. (2023) 23(1):608.
204. Das S, Saha R, Das C, Deb M, Kamilya G. Prognostic Role of Human Epididymis Protein4 (HE4) in Endometrial Lesions: Study in a Tertiary Care Centre. Indian J Surg Oncol. (2023) 14(2):428–33.
205. Barr CE, Sergeant JC, Agnew HJ, Bolton J, McVey RJ, Crosbie EJ. Serum HE4 predicts progestin treatment response in endometrial cancer and atypical hyperplasia: A prognostic study. BJOG. (2023) 130(8):941–8.
206. Yue XN, He XY, Wu JJ, Fan W, Zhang HJ, Wang CW. Endometrioid adenocarcinoma: combined multiparametric MRI and tumour marker HE4 to evaluate tumour grade and lymphovascular space invasion. Clin Radiol. (2023) 78(8):e574–81.
207. Zhai ZY, Li H, Li LW, Shen ZH, Zhang XB, Wang ZQ, et al. [Prognosis analysis of radical or modified radical hysterectomy and simple hysterectomy in patients with stage II endometrial cancer]. Zhonghua Fu Chan Ke Za Zhi. (2023) 58(6):442–50.
208. Cheng C, Jenkins N, Aikman N, ElSahwi K. Secondary cytoreductive surgery in recurrent clear cell carcinoma of the endometrium: A case report. Int J Surg Case Rep. (2023) 108:108412.
209. Sezgin B, Camuzcuoğlu A, Camuzcuoğlu H. Laparoscopic Resection of An Extragastrointestinal Stromal Tumor in the Presacral Area. J Minim Invasive Gynecol. (2019) 26(5):812–3.
210. Gök F, Ekin S, Karaman E, Erten R, Yıldız D, Bakır A. Total Sialic Acid, Antioxidant Enzyme Activities, Trace Elements, and Vitamin Status Before and After Surgery in Women with Uterine Myoma and Endometrial Cancer. Reprod Sci. (2023) 30(9):2743–57.
211. Thavaneswaran S, Kansara M, Lin F, Espinoza D, Grady JP, Lee CK, et al. A signal-seeking Phase 2 study of olaparib and durvalumab in advanced solid cancers with homologous recombination repair gene alterations. Br J Cancer. (2023) 129(3):475–85.
212. Huang H, Cai X, Lin J, Wu Q, Zhang K, Lin Y, et al. A novel five-gene metabolism-related risk signature for predicting prognosis and immune infiltration in endometrial cancer: A TCGA data mining. Comput Biol Med. (2023) 155:106632.
213. Zhao J, Jiao W, Sui X, Zou J, Wang J, Lin Z. Construction of a prognostic model of luteolin for endometrial carcinoma. Am J Transl Res. (2023) 15(3):2122–39.
214. Moroney MR, Davies KD, Wilberger AC, Sheeder J, Post MD, Berning AA, et al. Molecular markers in recurrent stage I, grade 1 endometrioid endometrial cancers. Gynecol Oncol. (2019) 153(3):517–20.
215. Raffone A, Travaglino A, Saccone G, Viggiani M, Giampaolino P, Insabato L, et al. PTEN expression in endometrial hyperplasia and risk of cancer: a systematic review and meta-analysis. Arch Gynecol Obstet. (2019) 299(6):1511–24.
216. Hu TWY, Li L, Yang E, Nie D, Li ZY. Molecular expression characteristics confirm the malignancy concealed by morphological alterations in endometrial cancer after fertility-preserving treatment. Arch Gynecol Obstet. (2019) 299(6):1673–82.
217. Bamberger AM, Briese J, Götze J, Erdmann I, Schulte HM, Wagener C, et al. Stimulation of CEACAM1 expression by 12-O-tetradecanoylphorbol-13-acetate (TPA) and calcium ionophore A23187 in endometrial carcinoma cells. Carcinogenesis. (2006) 27(3):483–90.
218. Li X, Cheng Y, Dong Y, Shen B, Yang X, Wang J, et al. An elevated preoperative serum calcium level is a significant predictor for positive peritoneal cytology in endometrial carcinoma. Chin J Cancer Res. (2019) 31(6):965–73.
219. Ge L, Liu G, Hu K, Huang K, Zhang M, Zhou J, et al. A New Risk Index Combining d-Dimer, Fibrinogen, HE4, and CA199 Differentiates Suspecting Endometrial Cancer From Patients With Abnormal Vaginal Bleeding or Discharge. Technol Cancer Res Treat. (2020) 19:1533033819901117.
220. Song N, Zhang Y, Kong F, Yang H, Ma X. HOXA-AS2 promotes type I endometrial carcinoma via miRNA-302c-3p-mediated regulation of ZFX. Cancer Cell Int. (2020) 20:359.
221. Fan JT, Zhou ZY, Luo YL, Luo Q, Chen SB, Zhao JC, et al. Exosomal lncRNA NEAT1 from cancer-associated fibroblasts facilitates endometrial cancer progression via miR-26a/b-5p-mediated STAT3/YKL-40 signaling pathway. Neoplasia. (2021) 23(7):692–703.
222. Unuvar S, Melekoglu R, Turkmen NB, Yilmaz E, Yasar S, Yuce H. Comparison of preoperative serum neopterin, periostin, indoleamine 2,3-dioxygenase, YKL-40, and tenascin-c levels with current tumor markers for early-stage endometrial cancer. Int J Gynaecol Obstet. (2021) 155(3):417–24.
223. Omer B, Genc S, Takmaz O, Dirican A, Kusku-Kiraz Z, Berkman S, et al. The diagnostic role of human epididymis protein 4 and serum amyloid-A in early-stage endometrial cancer patients. Tumour Biol. (2013) 34(5):2645–50.
224. Ren X, Liang J, Zhang Y, Jiang N, Xu Y, Qiu M, et al. Single-cell transcriptomic analysis highlights origin and pathological process of human endometrioid endometrial carcinoma. Nat Commun. (2022) 13(1):6300.
225. Jiang J, Zhu J, Qiu P, Ni J, Zhu W, Wang X. HNRNPA2B1-mediated m6A modification of FOXM1 promotes drug resistance and inhibits ferroptosis in endometrial cancer via regulation of LCN2. Funct Integr Genomics. (2023) 24(1):3.
226. Su S, Yin L. Application of Pelvic Magnetic Resonance Imaging Scan Combined with Serum Pyruvate Kinase Isozyme M2, Neutrophil Gelatinase-Associated Lipocalin, and Soluble Leptin Receptor Detection in Diagnosing Endometrial Carcinoma. Contrast Media Mol Imaging. (2022) 2022:7197505.
227. Dong P, Xiong Y, Yue J, Xu D, Ihira K, Konno Y, et al. Long noncoding RNA NEAT1 drives aggressive endometrial cancer progression via miR-361-regulated networks involving STAT3 and tumor microenvironment-related genes. J Exp Clin Cancer Research : CR. (2019a) 38:295. doi: 10.1186/s13046-019-1306-9
228. Jimeno A, Moore KN, Gordon M, Chugh R, Diamond JR, Aljumaily R, et al. A first-in-human phase 1a study of the bispecific anti-DLL4/anti-VEGF antibody navicixizumab (OMP-305B83) in patients with previously treated solid tumors. Invest New Drugs. (2019) 37(3):461–72.
229. Guan L, Zhang A. Amniotic epithelial cells reverse abnormal vascular structure and function in endometrial carcinoma. Int J Clin Exp Pathol. (2019) 12(7):2405–24.
230. Hassani M, Mahdevar M, Peymani M. Exploring the role of interleukin 11 in cancer progression, patient survival, and therapeutic insights. Mol Biol Rep. (2024) 51(1):461.
231. Lam C, Sarasohn D, Weigelt B, Zamarin D. Tumor lenvatinib addiction and withdrawal rebound response in patients with advanced endometrial cancer. Gynecol Oncol Rep. (2023) 49:101258.
232. Ducceschi M, Polignano M, Bini M, Lopez S, Conca E, Tamborini E, et al. The Revolution of Immunotherapy in Gynecological Cancers: The Lazarus Effect in Endometrial Cancer. J Clin Med. (2023) 12(17):5540.
233. Goodman AM, Sokol ES, Frampton GM, Lippman SM, Kurzrock R. Microsatellite-Stable Tumors with High Mutational Burden Benefit from Immunotherapy. Cancer Immunol Res. (2019) 7(10):1570–3.
234. Wang K-H, Ding D-C. The role and applications of exosomes in gynecological cancer: A review. Cell Transplant. (2023) 32:9636897231195240. doi: 10.1177/09636897231195240
235. Chang L, Zhang D, Shi H, Bian Y, Guo R. MiR-143 inhibits endometrial cancer cell proliferation and metastasis by targeting MAPK1. Oncotarget. (2017) 8:84384–95. doi: 10.18632/oncotarget.v8i48
236. Bogaczyk A, Zawlik I, Zuzak T, Kluz M, Potocka N, Kluz T. The role of miRNAs in the development, proliferation, and progression of endometrial cancer. Int J Mol Sci. (2023) 24:114895. doi: 10.3390/ijms241411489
237. Li X, Zha Li, Li Bo, Sun R, Liu J, Zeng iH. Clinical significance of MMP-9 overexpression in endometrial cancer: A PRISMA-compliant meta-analysis. Front Oncol. (2022) 12:925424. doi: 10.3389/fonc.2022.925424
238. Maqsood Q, Sumrin A, Saleem Y, Wajid A, Mahnoor M. Exosomes in cancer: diagnostic and therapeutic applications. Clin Med Insights: Oncol. (2024) 18. doi: 10.1177/11795549231215966
239. Li S, Shan Y, Li X, Zhang C, Wei S, Dai S, et al. lncRNA SNHG5 Modulates Endometrial Cancer Progression via the miR-25-3p/BTG2 Axis. J Oncol. (2019) 2019:7024675. doi: 10.1155/2019/7024675
Keywords: gynecological cancer, microRNA, endometrium, carcinogenesis, tumor microenvironment, anticancer therapy
Citation: Niebora J, Woźniak S, Domagała D, Data K, Farzaneh M, Zehtabi M, Dari MAG, Pour FK, Bryja A, Kulus M, Mozdziak P, Dzięgiel P and Kempisty B (2024) The role of ncRNAs and exosomes in the development and progression of endometrial cancer. Front. Oncol. 14:1418005. doi: 10.3389/fonc.2024.1418005
Received: 15 April 2024; Accepted: 26 June 2024;
Published: 12 August 2024.
Edited by:
Anjali Geethadevi, Medical College of Wisconsin, United StatesReviewed by:
Muzaffer Bhat, Albert Einstein College of Medicine, United StatesLavannya Sabharwal, Medical College of Wisconsin, United States
Naoshad Muhammad, Washington University in St. Louis, United States
Sudhir Kumar, Emory University, United States
Copyright © 2024 Niebora, Woźniak, Domagała, Data, Farzaneh, Zehtabi, Dari, Pour, Bryja, Kulus, Mozdziak, Dzięgiel and Kempisty. This is an open-access article distributed under the terms of the Creative Commons Attribution License (CC BY). The use, distribution or reproduction in other forums is permitted, provided the original author(s) and the copyright owner(s) are credited and that the original publication in this journal is cited, in accordance with accepted academic practice. No use, distribution or reproduction is permitted which does not comply with these terms.
*Correspondence: Bartosz Kempisty, YmFydG9zei5rZW1waXN0eUB1bXcuZWR1LnBs