- 1Precision Oncology Center, School of Medicine, Faculty of Medicine and Health Sciences, Universidad Mayor, Santiago, Chile
- 2Support Team for Oncological Research and Medicine (STORM), Santiago, Chile
- 3Department of Biological Sciences, Columbia University, New York, NY, United States
- 4Millennium Nucleus of Ion Channel-Associated Diseases (MiNICAD), Santiago, Chile
- 5Program of Cellular and Molecular Biology, Institute of Biomedical Sciences (ICBM), Faculty of Medicine, Universidad de Chile, Santiago, Chile
- 6Millennium Institute on Immunology and Immunotherapy, Faculty of Medicine, Universidad de Chile, Santiago, Chile
- 7Translational Medicine Laboratory, Fundación Arturo López Pérez Cancer Center, Santiago, Chile
- 8Department of Oral and Maxillofacial Medicine and Diagnostic Sciences, Case Western Reserve University School of Dental Medicine, Cleveland, OH, United States
Porphyromonas gingivalis (P. gingivalis) is a gram-negative oral pathogen associated with chronic periodontitis. Previous studies have linked poor oral health and periodontitis with oral cancer. Severe cases of periodontal disease can result in advanced periodontitis, leading to tissue degradation, tooth loss, and may also correlate with higher gastric cancer (GC) risk. In fact, tooth loss is associated with an elevated risk of cancer. However, the clinical evidence for this association remains inconclusive. Periodontitis is also characterized by chronic inflammation and upregulation of members of the Programmed Death 1/PD1 Ligand 1 (PD1/PDL1) axis that leads to an immunosuppressive state. Given that chronic inflammation and immunosuppression are conditions that facilitate cancer progression and carcinogenesis, we hypothesize that oral P. gingivalis and/or its virulence factors serve as a mechanistic link between oral health and gastric carcinogenesis/GC progression. We also discuss the potential impact of P. gingivalis’ virulence factors (gingipains, lipopolysaccharide (LPS), and fimbriae) on inflammation and the response to immune checkpoint inhibitors in GC which are part of the current standard of care for advanced stage patients.
1 Introduction
In recent decades, significant advances in sequencing technologies have expanded our knowledge on the microbiome, sparking researchers’ interest in the role of the human microbiome in homeostasis and disease. Consensus establishes that many diseases, ranging from Alzheimer’s to endocrine disorders, and cancer, are associated with microbial imbalances or dysbiosis (1).
The oral cavity is the entry portal to the gastrointestinal tract. Changes in the oral cavity’s bacterial diversity can have local and systemic consequences on health and disease (2, 3). Within the oral cavity, bacteria can be found in the saliva or as a part of biofilm-structured communities. Interestingly, previous studies have linked poor oral health and chronic periodontitis with oral cancer (4). Severe cases of periodontitis can result in tissue degradation and tooth loss, potentially correlating with an elevated risk of developing gastric cancer (GC). However, the existing evidence from case-control studies and five cohort studies regarding tooth loss as a potential marker remains inconclusive. Significant heterogeneity among studies and mixed results between case-control and cohort studies contribute to this association’s uncertainty (5).
2 Is Porphyromonas gingivalis the link between the oral microbiome and gastric cancer?
Perhaps the best example of an association between dysbiosis and cancer is Helicobacter pylori (H. pylori) and GC. This gram-negative bacterium colonizes the stomach and plays a central role in developing this pathology. H. pylori is widely recognized as an oncogenic factor and a driver of gastric carcinogenesis. In fact, the International Agency for Research on Cancer (IARC) classifies the H. pylori infection within the group I of human carcinogens (6). Within the gastric mucosa, H. pylori modulates acid secretion, affecting the gastric microbiome, leading to further dysbiosis, H. pylori overgrowth, and associated diseases. Current hypotheses suggest that recurrent and persistent H. pylori infections cause chronic inflammation, eventually leading to gastric carcinogenesis (7). In addition to H. pylori, new evidence suggests that other bacteria, including oral bacteria and their metabolites, may contribute to gastric carcinogenesis and GC progression (8, 9).
The oral microbiome is the most complex and dynamic arrangement of microbial communities within the human body. Changes in the oral microbiome can have profound consequences in an individual’s homeostasis. As pointed out earlier, periodontitis is a chronic inflammatory disease characterized by oral dysbiosis. Recent studies postulate that the transition from a healthy state into dysbiosis is driven by specific “keystone pathogens” that alter the host immune system, modifying the conditions of the microenvironment and disrupting the balance among bacterial communities.
Porphyromonas gingivalis (P. gingivalis or Pg) is a gram-negative, anaerobic, rod-shaped oral bacteria, and a keystone pathogen in chronic periodontitis (10). Despite its role in periodontitis, high levels of P. gingivalis have also been documented in healthy subjects without oral disease (11). Unlike other oral bacteria, P. gingivalis is an acid-resistant bacterium and studies demonstrate it is able to migrate from the oral cavity to the colon (12, 13). This suggests that P. gingivalis in the ingested saliva may easily reach the stomach. In addition to being swallowed, everyday activities such as brushing, flossing, chewing, and dental procedures cause transient P. gingivalis bacteremia (14, 15). Some of these activities can result in the translocation of the bacterium into other tissues, such as the liver, placenta, or even coronary arteries (16). Similar to other bacteria, P. gingivalis possess a variety of virulence factors, including gingipains (cysteine proteases), capsule, lipopolysaccharides (hereafter called Pg-LPS), and fimbriae. These factors modulate systemic inflammation, dysbiosis, tumorigenesis, and contribute to the evasion of the innate immune response. In one hand, gingipains are responsible for the development of periodontitis (16, 17), inducing cell migration and the release of other pro-inflammatory mediators (18). On the other hand, Pg-LPS is responsible for the maintenance of chronic inflammation by increasing the secretion of proinflammatory cytokines. Interestingly, a recent article reports that P. gingivalis and its gingipains may play a central role in other chronic, age-related pathologies, such as Alzheimer’s disease (19). Also, studies in rats demonstrate that topical applications of Pg-LPS lead to severe complications, including neuroinflammation and impaired learning and memory (20).
2.1 The contribution of P. gingivalis in carcinogenesis and cancer progression
Studies demonstrate that serum antibodies against P. gingivalis are associated with orodigestive cancer mortality (21) suggesting a role in cancer risk. Previous studies have reported the effects of P. gingivalis and its virulence factors upon some of the “hallmarks of cancer” (22). As summarized in Figure 1, P. gingivalis can mediate immune evasion and increase inflammation in cancer cells; it also stimulates proliferation and invasion/migration. Reports in gingival epithelial cells indicate that P. gingivalis also suppresses apoptosis.
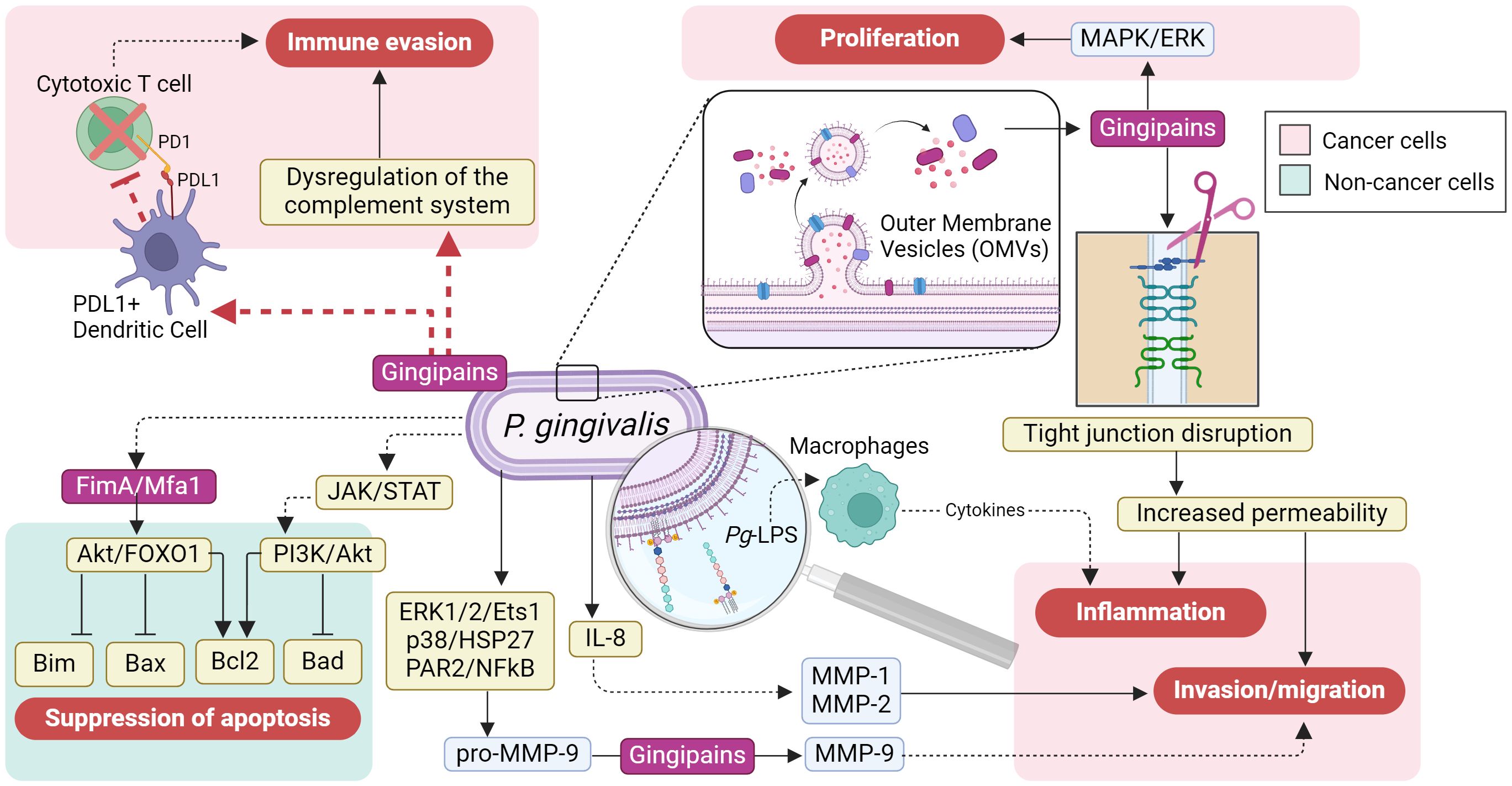
Figure 1 The cancer-promoting effects of Porphyromonas gingivalis on cancer cells. The cancer-promoting effects on gastric epithelial cells are mediated through its virulence factors, which include pathogen-associated molecular patterns (PAMPs) like lipopolysaccharides (LPS), gingipains, and fimbriae. These factors can directly trigger immune evasion, inflammation, invasion/migration, and proliferation by activating numerous molecular pathways in cancer cells (depicted in the light red boxes); these pathways are potentially influenced by the PD1/PDL1 axis (shown by the red dashed arrow). Additionally, P. gingivalis virulence factors may contribute to the suppression of apoptosis by activating signaling pathways, such as JAK/STAT, PI3K/AKT, and AKT/FOXO1, thereby promoting the progression of gastric cancer (shown in the light green box) (Figure created in BioRender.com).
As proteases, Pg-gingipains can degrade components of the tight junctions within the gingival and the gastrointestinal epithelia, disrupting their integrity (23, 24). A coordinated attack of Pg-gingipains and H. pylori toxins can severely damage the gastric epithelium, promoting an aggravated state of vulnerability with increased permeability to pathogens and virulence factors, leading to reduced functionality, chronic gastritis, and systemic inflammation (25–27). In GC patients, the degradation of tight junctions also facilitates the spread of cancer cells throughout the body, promoting metastasis (26, 28, 29).
Another mechanism by which Pg-gingipains can increase GC progression is by cleavage, phosphorylation, and degradation of the 27-kDa heat shock protein (HSP27), a chaperone that responds to stress and apoptosis (30–34). Then, HSP27 reduction impairs cells’ ability to respond to stress signals and their protection against damage, thereby contributing to GC progression.
Additionally, P. gingivalis can disrupt apoptosis by upregulating B-cell lymphoma proteins (Bcl2), a family of direct and indirect proapoptotic proteins, via virulence factors such as fimbriae, gingipains, and hemaphore-like proteins. This phenomenon has been observed in P. gingivalis-infected dendritic and CD3+ T cells as a mechanism to prolong the survival of infected cells in parallel to the host immunity evasion (35).
Similarly, P. gingivalis Mfa1 and FimA fimbriae also exhibit antiapoptotic activity by upregulating C-X-C chemokine receptor type 4 (CXCR4) and downregulating Forkhead Box O1/3 (FOXO1/3), which are associated with tumor progression (36). Moreover, Porphyromonas sp. can confer cancer cells with enhanced tumor invasion and metastatic capabilities by promoting matrix metalloproteinase 9 (MMP9), an extracellular matrix degrader, through activation of protease-activated receptor 2 (PAR2) and the ERK1/2-Ets1/3-p38 pathway (37, 38) (Figure 1).
Provided that the oral cavity is the entry portal to the gastrointestinal tract, P. gingivalis and its virulence factors have all been implicated in the carcinogenesis and progression of oral, esophageal, liver, colorectal, and pancreatic cancers. The fact that distant organs can be affected emphasizes that P. gingivalis has systemic tumorigenic and tumor enhancer effects (39–41). The damage mechanisms of P. gingivalis may include biological processes such as modifying and evading the innate immune response, promoting inflammation, and suppressing apoptosis (42, 43) (Figure 1).
2.2 How does Porphyromonas gingivalis evade the immunological surveillance?
P. gingivalis is a well-established microbial modulator that drives dysbiosis by activating Toll-like receptors (TLRs), resulting in a shift in the immune response in favor of the pathogens (44). In this context, Pg-LPS exhibits low endotoxin activity and structural variation, hence, the differential activation of TLR2 and TLR where Pg-LPS is predominantly recognized by TLR2 (45). In addition, another virulence factor of P. gingivalis, the fimbriae, stimulates cytokine expression by interacting with TLRs. The host cells recognizes the fimbriae as a potential threat to the immune system, and their detection via Pattern Recognition Receptors (PRRs) regulates the induction of immune costimulatory molecules that facilitate the initiation of T cell-mediated immunity. However, in persistent P. gingivalis infections, fimbriae can upregulate the expression of costimulatory molecules, thereby exacerbating inflammation mechanisms by activating the response mediated by CD4+ T cells (46).
Furthermore, gingipain degrades proinflammatory cytokines such as interleukin (IL)-1β, tumor necrosis factor (TNF)-α, interferon (IFN)-γ, IL-12, IL-8, IL-6, and their receptors, altering host defense mechanisms associated with inflammation. It also activates matrix metalloproteinases (MMPs), such as MMP-1, MMP-3, and MMP-9, conferring an aggressive, invasive, and prometastatic state to tumor cells (47, 48).
In summary, P. gingivalis may enhance tumor progression to an advanced stage from oral disease by translocating, enriching the tumor microenvironment with virulence factors, manipulating the immune response, maintaining chronic inflammation, and stimulating changes in the host’s immunological surveillance that ultimately serve to promote GC and facilitate the systemic progression of this disease.
3 Hypothesis: oral Porphyromonas gingivalis as a driver of gastric cancer progression
We hypothesize that oral P. gingivalis infection contributes to GC progression by increasing Pg-LPS, PAMPs, Pg-gingipains, inflammatory mediators, and immune checkpoint components, such as the PD1/PDL1 axis. Our hypothesis was based on the fact that most oral pathogens are unlikely to survive in an acidic stomach environment. Additionally, it has been observed that P. gingivalis, when present in oral plaque, is associated with a higher risk of precancerous gastric lesions (49). Furthermore, individuals with periodontal disease and high levels of colonization of periodontal pathogens are related to high levels of immunoglobulin G (IgG) anti-P. gingivalis in the serum of patients, indicating that this pathogen may also increase the mortality risk of cancer patients through other independent mechanisms associated with periodontitis (21).
A plausible explanation for this association could involve a “hit-and-run” mechanism in which transiently resident P. gingivalis is rapidly inactivated and destroyed by the acidic stomach environment, avoiding the installment of a local infection. However, bacteria can still release virulence factors such as Pg-LPS and gingipain-containing outer membrane vesicles (OMVs) locally and systemically (50) (Figure 1).
According to our hypothetical model, poor oral health generates a bacterial imbalance, or dysbiosis, that reduces the diversity of local bacteria (Figure 2). Thus, P. gingivalis becomes dominant in a process that can lead to severe chronic periodontitis and tissue destruction (51). Uncontrolled proliferation of P. gingivalis increases the production and delivery of virulence factors such as PAMPs, mainly Pg-LPS and gingipains via OMVs, impairing the host immune surveillance and further potentiating oral dysbiosis (52). At this stage, P. gingivalis could be translocated systemically to other organs. Previous studies have demonstrated the presence of P. gingivalis DNA in the cerebrospinal fluid of living subjects diagnosed with probable Alzheimer’s disease (19, 53). Subsequently, Pg-LPS and OMVs-containing gingipain reach the bloodstream and the lymphatic system via gingival vessels. Then, following our arguments to consolidate the hypothesis, Pg-LPS accesses the lymphatic ganglia and the gastric tumor microenvironment, where it binds to TLR4 receptors, increasing the expression of ligands and receptors of the immune checkpoint pathway (PD1/PDL1 axis). Activation of TLR4 receptors by Pg-LPS triggers the NF-κB pathway and production of pro-inflammatory cytokines in innate immune cells (54). Studies on peripheral T-cell lymphomas have shown that TLR4 overexpression is associated with higher PDL1 expression, translating into poorer prognosis (55).
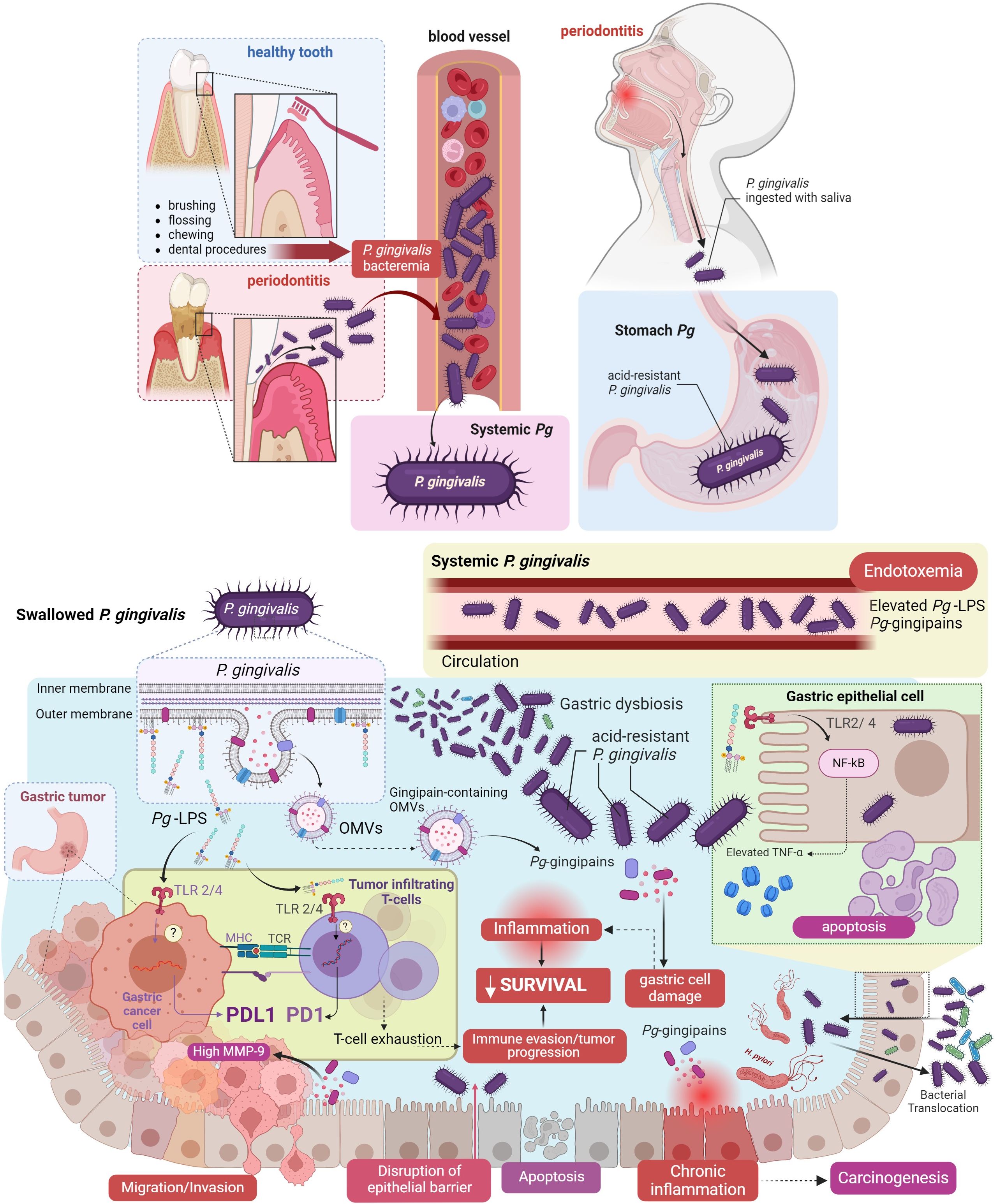
Figure 2 Hypothesis: Porphyromonas gingivalis and its pathogenic factors in oral health enhance immune evasion in gastric cancer. Oral infection with P. gingivalis can lead to bacterial dissemination through the bloodstream or via swallowing of saliva, reaching the stomach (depicted in the light blue box). P. gingivalis, utilizing its virulence factors, may induce gastric dysbiosis upon reaching the gastric epithelial cells, triggering inflammation, and reducing cell survival. These processes occur between tumor cells and the immune system and facilitate the infiltration of T-cells into the tumor, T-cell exhaustion, and activation of the PD1/PDL1 axis enhance immune evasion (illustrated in the yellow box). All these processes constitute a significant part of the mechanism proposed in our hypothesis. These cellular events progress from migration/invasion, disruption of the epithelial barrier, apoptosis, to chronic inflammation, ultimately culminating in carcinogenesis. (Figure created in BioRender.com).
Additionally, studies in mice suggest that TLR4 activation by systemic Pg-LPS can modulate the response to PD1 therapy during chronic viral infection (56). Therefore, following our line of argument, TLR4 activation by Pg-LPS increases PD1/PDL1 expression in T-cells and GC cells, leading to simultaneous T-cell exhaustion by PD1 and an increase in PDL1, which allows gastric tumor progression, culminating in a decrease in patient survival which evidences this fact (Figure 2).
Interestingly, studies combining colorectal cancer cell lines and mouse models have demonstrated that Pg-LPS promotes metastasis by increasing cell invasion and migration via NF-κB (57). Furthermore, regarding the association between PD1/PDL1 expression and cancer patients’ survival, a Japanese study showed that PDL1 expression was associated with worse overall survival in stage II/III GC patients (58). However, this association has been inconsistent among other malignancies, showing no association or improved patient survival in some cancers, and remain controversial (59–61).
4 Discussion
A dysbiotic oral microbiome may contribute to the development of both local (oral) and systemic diseases. This includes a wide range of illnesses from dental caries and periodontal disease (62, 63) to cardiovascular disease and cancer (64, 65). A genomic study analyzed the composition of the digestive tract microbiome and reported a 45% of overlap between oral and stool bacteria (66), suggesting the transfer of oral bacteria into the stomach is not uncommon. In fact, ingested saliva contains a great amount of oral bacteria. Although these are usually unable to colonize a healthy gut they have been reported in the intestine of individuals that suffer from colon cancer and inflammatory bowel disease, among others (67). This supports the idea of the oral cavity as a reservoir for potential gastrointestinal pathogens, which in turn can exacerbate gastrointestinal diseases.
However, microbiota components also induce immune evasion mechanisms to restore homeostasis in the host organism. Previous studies have demonstrated a correlation between various cancers, the presence of P. gingivalis, and its diverse virulence mechanisms. Additionally, these studies highlighted the activation of immune checkpoint mechanisms in the host.
While the prognostic significance of PDL1 in gastric carcinomas remains uncertain, certain studies have highlighted its relevance in clinical outcomes across the entire cohort via multivariate analysis. Specifically, PDL1(+) tumors were identified as an adverse prognostic factor in Epstein Barr Virus (EBV)-positive carcinomas but not MSI-high carcinomas. Also, CD8+ tumors have shown low tumor-infiltrating lymphocytes, and more advanced-stage tumors were associated with unfavorable clinical outcomes. Furthermore, the knockdown of PDL1 in gastric carcinoma cells demonstrated significant suppression of proliferation, invasion, and cell migration while increasing apoptosis. Notably, these effects were prominent in two EBV(+) cell lines but not consistently observed across all three EBV (–) cell lines (68).
An investigation on PDL1 levels in a prostate cancer cell line revealed that infection with P. gingivalis and its PAMPs positively influenced PDL1 expression. This positive regulation was facilitated through the nucleotide-binding oligomerization domain (NOD)1/NOD2 signaling pathway. Interestingly, no upregulation was observed following the treatment of cells with Pg-LPS. These findings suggest that chronic inflammatory conditions within the organ might play a role in tumor immune evasion by altering the tumor microenvironment (69).
In several studies conducted on different cancer types, such as PC, it has been observed that microbiota communities within tumors partially overlap with those found in the oral cavity. For instance, P. gingivalis has been found to aggregate significantly more in PC tissues than in adjacent normal tissues, thereby altering the tumor microenvironment and promoting pancreatic tumorigenesis in murine models. This altered microenvironment is characterized by a notable increase in neutrophil enrichment and a significant decrease in CD8+ cytotoxic T cells. In contrast, no significant changes were observed in CD4+ T cells and monocytes. These findings suggest that P. gingivalis may induce a neutrophil-dominated proinflammatory response in mice with PC, contributing to the suppression of the tumor immune environment (70).
Elevated levels of anti-P. gingivalis antibodies have been identified in patients with pancreatic cancer (PC) compared to healthy controls. Moreover, P. gingivalis and other microbiota members possess peptidyl arginine deaminase (PAD) enzymes. Together with mutations in the arginine of tumor protein p53 (TP53), these factors establish a close relationship between a microbiota component, virulence factors, and the progression of PC (71).
Current evidence supports the significant role of PDL1 in carcinogenesis, particularly in hepatocellular carcinoma (HCC), where the bidirectional regulation of PDL1 mediated by TP53/mechanistic Target of Rapamycin Complex 1 (mTORC1) has been elucidated. In HCC with non-mutated TP53, mTORC1 suppression leads to increased E2F1 transcription factor expression. This increase interrupts the cytoplasmic interaction between E2F transcription factor 1 (E2F1) and PDL1, facilitating the translocation of E2F1 into the nucleus, where it positively activates PDL1 transcription. Conversely, in HCC with mutated TP53, mTORC1 suppression promotes PDL1 protein degradation through autophagy. Additionally, it has been observed that tumor infiltration by CD8+ T cells is significantly reduced in this type of HCC. These findings highlight the pivotal role of TP53 in regulating PDL1 expression and modulating immune evasion in HCC (72).
Investigating the microbiota in low biomass environments like the stomach and lungs poses significant challenges for molecular studies, primarily due to the difficulty in detecting pathogenic agents. Nonetheless, these environments also display reduced microbial complexity, potentially limiting the number of causal agents and mitigating the risk of establishing spurious causal relationships (73, 74). Randomized control trials and prospective cohorts can confirm or refute the model that links GC, PD1/PDL1 axis, and periodontitis-related pathogens (75).
In the realm of human pathologies, the investigation into potential standard causal links between periodontitis and rheumatoid arthritis (RA) has revealed a connection between the ability of P. gingivalis to citrullinate peptides and the presence of autoantibodies against citrullinated peptides, which are particular and sensitive markers in the diagnosis of RA. Consequently, peptide citrullination has been implicated in triggering an autoimmune response, whereby the immune system perceives modified self-proteins and peptides as foreign. While citrullinated peptides may play a role in the pathogenesis of RA, the precise nature of their emergence and function remains unclear, and the potential contributions of the microbiota in the citrullination process are yet to be fully elucidated (76, 77).
Ultimately, prospective studies should evaluate oral health status at diagnosis and utilize next-generation sequencing (NGS) tools to fully examine the presence of specific oral bacteria and virulence factors. Enhanced methodologies for elucidating causal relationships and experimental disease models will contribute to a more comprehensive assessment of the role played by particular microbiome organisms and their virulence factors in GC onset, progression, and response to anticancer treatment (78), taking into account that the experimental design of such studies are the main cause of overstating or understating the importance of certain pathogens or microbiome profiles on cancer, as was detailed by a critical review (79).
4.1 An experimental approach to our hypothesis: microbiota, metabolites, and evasion of the host immune response
The experimental approach to our hypothesis encompasses three key axes. First, elucidating the relationship between P. gingivalis, periodontal disease, and the progression of digestive tract tumors, particularly GC, would require a large-scale prospective multicenter study employing transcriptome RNA sequencing to characterize the metabolically active microbiota. This analysis should encompass bacteria in the gastrointestinal tract and within tumors (80). The second aspect involves identifying metabolites produced by the bacterial community (metabolomics) to obtain a comprehensive overview of metabolites, including amino acids, carbohydrates, carbohydrate conjugates, fatty acids, glycerophospholipids, nucleosides, and nucleotides. This analysis and its findings would pave the way for the third aspect of our hypothesis, which aims to pinpoint key metabolites, such as adenosine, a classic regulator of metabolic and immunological checkpoints, that participate in the tumor’s evasion of the host immune system.
Finally, studies have reported increased nucleoside concentrations in GC patients with peritoneal recurrence compared with those without peritoneal recurrence. Unraveling this intricate landscape would enable us to identify the metabolites produced by P. gingivalis that manipulate the host’s immune system. Consequently, this phenomenon may inadvertently facilitate cancer cell progression towards tumorigenesis as a secondary effect (80, 81).
Data availability statement
The original contributions presented in the study are included in the article/supplementary material. Further inquiries can be directed to the corresponding author.
Author contributions
MM-M: Conceptualization, Writing – original draft, Writing – review & editing. MP: Conceptualization, Writing – original draft, Writing – review & editing, Visualization. LG: Writing – original draft, Writing – review & editing. MC: Writing – original draft, Writing – review & editing. FV-E: Writing – original draft, Writing – review & editing. PM: Writing – original draft, Writing – review & editing. AP: Writing – original draft, Writing – review & editing. BG-B: Writing – original draft, Writing – review & editing. TM: Writing – original draft, Writing – review & editing. JG: Writing – original draft, Writing – review & editing. MG: Writing – original draft, Writing – review & editing. IR: Writing – original draft, Writing – review & editing, Conceptualization, Funding acquisition, Supervision, Visualization.
Funding
The author(s) declare financial support was received for the research, authorship, and/or publication of this article. This work was partly supported by ANID 11220563 to INR, 1221499 to MG, and 1221415; ICN 2021_045.
Acknowledgments
Authors wish to thank our families for their support, tenderness, warmness, and love, making this work possible.
Conflict of interest
MG is a principal investigator in clinical trials from MSD, BMS, Novartis, Roche, Astellas, Deciphera, PPD, IQVIA, Bayer, Principia, Covance, Daiichi-Sankyo, Basilea, PRA-Exelisis, Syneos, Zimeworks. MG participates in the Scientific Advisory Board for Bayer, Novartis, MSD, BMS, Pfizer, Macrogenic, Merck. MG has participated in Teaching/Speaker Bureau Activities for Novartis, Pfizer, Bayer, BMS,MSD, GBT Biotoscana, Lilly. MM-M and IR are board members of Fundacion GIST Chile, a non-profit patient advocacy organization for patients with gastrointestinal cancers.
The remaining authors declare that the research was conducted in the absence of any commercial or financial relationships that could be construed as a potential conflict of interest.
Publisher’s note
All claims expressed in this article are solely those of the authors and do not necessarily represent those of their affiliated organizations, or those of the publisher, the editors and the reviewers. Any product that may be evaluated in this article, or claim that may be made by its manufacturer, is not guaranteed or endorsed by the publisher.
Abbreviations
Bcl2, B-cell lymphoma two proteins; CXCR4, C-X-C chemokine receptor type 4; E2F1, E2F transcription factor 1; ERK, Extracellular signal-regulated kinase; FOXO1/3, Forkhead box O1/3; GC, Gastric Cancer; H. pylori, Helicobacter pylori; HSP27, 27-kDa heat shock protein; IARC, International Agency for Research on Cancer; ICI, Immune checkpoint inhibitor; IgG, Immunoglobulin G; IL, Interleukin; JAK, Janus kinase; JAM1, Junctional adhesion molecule 1; LPS, Lipopolysaccharide; MAPK, Mitogen-activated protein kinase; MHC, Major histocompatibility complex; MMP9, Matrix metalloproteinase 9; NF-κβ, Nuclear Factor-kB; NGS, Next-generation sequencing; NOD, nucleotide-binding oligomerization domain; OMV, Outer membrane vesicle; OS, Overall survival; PAR2, Protease-activated receptor 2; PC, Pancreatic cancer; PD1, Programmed Death 1; PDL1, PD1 Ligand 1; P. gingivalis, Porphyromonas gingivalis; PI3K, Phosphoinositide 3-kinase; PRRs, Pattern recognition receptors; RA, rheumatoid arthritis; STAT, Signal transducer and activation of transcription proteins; TER, DNA-replication terminus site-binding protein; TIM-3, T-cell immunoglobulin and mucin-domain containing-3; TLR4, Toll Like Receptor 4; TNF, Tumor necrosis factor; TP53, Tumor protein p53.
References
1. Thomas S, Izard J, Walsh E, Batich K, Chongsathidkiet P, Clarke G, et al. The host microbiome regulates and maintains human health: A primer and perspective for non-microbiologists. Cancer Res. (2017) 77:1783–812. doi: 10.1158/0008-5472.CAN-16-2929
2. Kozak M, Pawlik A. The role of the oral microbiome in the development of diseases. Int J Mol Sci. (2023) 24:5231. doi: 10.3390/ijms24065231
3. Tan X, Wang Y, Gong T. The interplay between oral microbiota, gut microbiota and systematic diseases. J Oral Microbiol. (2023) 15:2213112. doi: 10.1080/20002297.2023.2213112
4. Zhang L, Liu Y, Zheng HJ, Zhang CP. The oral microbiota may have influence on oral cancer. Front Cell Infect Microbiol. (2019) 9:476. doi: 10.3389/fcimb.2019.00476
5. Yin XH, Wang YD, Luo H, Zhao K, Huang GL, Luo SY, et al. Association between tooth loss and gastric cancer: A meta-analysis of observational studies. PloS One. (2016) 11:e0149653. doi: 10.1371/journal.pone.0149653
7. Malfertheiner P, Camargo MC, El-Omar E, Liou JM, Peek R, Schulz C, et al. Helicobacter pylori infection. Nat Rev Dis Primers. (2023) 9:1–24. doi: 10.1038/s41572-023-00431-8
8. Coker OO, Dai Z, Nie Y, Zhao G, Cao L, Nakatsu G, et al. Mucosal microbiome dysbiosis in gastric carcinogenesis. Gut. (2018) 67:1024–32. doi: 10.1136/gutjnl-2017-314281
9. Lo CH, Kwon S, Wang L, Polychronidis G, Knudsen MD, Zhong R, et al. Periodontal disease, tooth loss, and risk of oesophageal and gastric adenocarcinoma: a prospective study. Gut. (2021) 70:620–1. doi: 10.1136/gutjnl-2020-321949
10. Coussens LM, Werb Z. Inflammation and cancer. Nature. (2002) 420:860–7. doi: 10.1038/nature01322
11. Sato K, Takahashi N, Kato T, Matsuda Y, Yokoji M, Yamada M, et al. Aggravation of collagen-induced arthritis by orally administered Porphyromonas gingivalis through modulation of the gut microbiota and gut immune system. Sci Rep. (2017) 7:6955. doi: 10.1038/s41598-017-07196-7
12. Walker MY, Pratap S, Southerland JH, Farmer-Dixon CM, Lakshmyya K, Gangula PR. Role of oral and gut microbiome in nitric oxide-mediated colon motility. Nitric Oxide Biol Chem. (2018) 73:81–8. doi: 10.1016/j.niox.2017.06.003
13. Waghmare AS, Vhanmane PB, Savitha B, Chawla RL, Bagde HS. Bacteremia following scaling and root planing: A clinico-microbiological study. J Indian Soc Periodontol. (2013) 17:725–30. doi: 10.4103/0972-124X.124480
14. Ambrosio N, Marín MJ, Laguna E, Herrera D, Sanz M, Figuero E. Detection and quantification of Porphyromonas gingivalis and Aggregatibacter actinomycetemcomitans in bacteremia induced by interdental brushing in periodontally healthy and periodontitis patients. Arch Oral Biol. (2019) 98:213–9. doi: 10.1016/j.archoralbio.2018.11.025
15. Forner L, Larsen T, Kilian M, Holmstrup P. Incidence of bacteremia after chewing, tooth brushing and scaling in individuals with periodontal inflammation. J Clin Periodontol. (2006) 33:401–7. doi: 10.1111/j.1600-051X.2006.00924.x
16. O’Brien-Simpson NM, Veith PD, Dashper SG, Reynolds EC. Porphyromonas gingivalis gingipains: the molecular teeth of a microbial vampire. Curr Protein Pept Science. (2003) 4:409–26. doi: 10.2174/1389203033487009
17. Imamura T. The role of gingipains in the pathogenesis of periodontal disease. J Periodontology. (2003) 74:111–8. doi: 10.1902/jop.2003.74.1.111
18. Liu Y, Wu Z, Nakanishi Y, Ni J, Hayashi Y, Takayama F, et al. Infection of microglia with Porphyromonas gingivalis promotes cell migration and an inflammatory response through the gingipain-mediated activation of protease-activated receptor-2 in mice. Sci Rep. (2017) 7:11759. doi: 10.1038/s41598-017-12173-1
19. Dominy SS, Lynch C, Ermini F, Benedyk M, Marczyk A, Konradi A, et al. Porphyromonas gingivalis in Alzheimer’s disease brains: Evidence for disease causation and treatment with small-molecule inhibitors. Sci Adv. (2019) 5:eaau3333. doi: 10.1126/sciadv.aau3333
20. Hu Y, Li H, Zhang J, Zhang X, Xia X, Qiu C, et al. Periodontitis induced by P. gingivalis-LPS is associated with neuroinflammation and learning and memory impairment in sprague-dawley rats. Front Neurosci. (2020) 14:658. doi: 10.3389/fnins.2020.00658
21. Ahn J, Segers S, Hayes RB. Periodontal disease, Porphyromonas gingivalis serum antibody levels and orodigestive cancer mortality. Carcinogenesis. (2012) 33:1055–8. doi: 10.1093/carcin/bgs112
22. Hanahan D, Weinberg RA. The hallmarks of cancer. Cell. (2000) 100:57–70. doi: 10.1016/S0092-8674(00)81683-9
23. Leech AO, Cruz RGB, Hill ADK, Hopkins AM. Paradigms lost—an emerging role for over-expression of tight junction adhesion proteins in cancer pathogenesis. Ann Trans Med. (2015) 3:184–4. doi: 10.3978/j.issn.2305-5839.2015.08.01
24. Takeuchi H, Amano A. Invasion of gingival epithelial cells by porphyromonas gingivalis. Methods Mol Biol. (2021) 2210:215–24. doi: 10.1007/978-1-0716-0939-2_21
25. Bhat AA, Uppada S, Achkar IW, Hashem S, Yadav SK, Shanmugakonar M, et al. Tight junction proteins and signaling pathways in cancer and inflammation: A functional crosstalk. Front Physiol. (2018) 9:1942. doi: 10.3389/fphys.2018.01942
26. Martin TA, Jiang WG. Loss of tight junction barrier function and its role in cancer metastasis. Biochim Biophys Acta (BBA) - Biomembranes. (2009) 1788:872–91. doi: 10.1016/j.bbamem.2008.11.005
27. Rendón-Huerta EP, García-García CA, Estrada LFM, Rendón-Huerta EP, García-García CA, Estrada LFM. Effect of Helicobacter pylori on Tight Junctions in Gastric Epithelia. In: Helicobacter pylori - From First Isolation to 2021 (2021) London, United Kingdom: IntechOpen. Available at: https://www.intechopen.com/chapters/75788.
28. Martin TA, Mason MD, Jiang WG. Tight junctions in cancer metastasis. Front Biosci (Landmark Ed). (2011) 16:898–936. doi: 10.2741/3726
29. Nehme Z, Roehlen N, Dhawan P, Baumert TF. Tight junction protein signaling and cancer biology. Cells. (2023) 12:243. doi: 10.3390/cells12020243
30. Kapranos N, Kominea A, Konstantinopoulos P, Savva S, Artelaris S, Vandoros G, et al. Expression of the 27-kDa heat shock protein (HSP27) in gastric carcinomas and adjacent normal, metaplastic, and dysplastic gastric mucosa, and its prognostic significance. J Cancer Res Clin Oncol. (2002) 128:426–32. doi: 10.1007/s00432-002-0357-y
31. Katsogiannou M, Andrieu C, Rocchi P. Heat shock protein 27 phosphorylation state is associated with cancer progression. Front Genet. (2014) 5:346. doi: 10.3389/fgene.2014.00346
32. Choi SK, Kam H, Kim KY, Park SI, Lee YS. Targeting heat shock protein 27 in cancer: A druggable target for cancer treatment? Cancers (Basel). (2019) 11:1195. doi: 10.3390/cancers11081195
33. Liu T, Liu D, Kong X, Dong M. Clinicopathological significance of heat shock protein (HSP) 27 expression in gastric cancer: A updated meta-analysis. Evid Based Complement Alternat Med. (2020) 2020:7018562. doi: 10.1155/2020/7018562
34. Drexler R, Wagner KC, Küchler M, Feyerabend B, Kleine M, Oldhafer KJ. Significance of unphosphorylated and phosphorylated heat shock protein 27 as a prognostic biomarker in pancreatic ductal adenocarcinoma. J Cancer Res Clin Oncol. (2020) 146:1125–37. doi: 10.1007/s00432-020-03175-0
35. Meghil MM, Tawfik OK, Elashiry M, Rajendran M, Arce RM, Fulton DJ, et al. Disruption of Immune Homeostasis in Human Dendritic Cells via Regulation of Autophagy and Apoptosis by Porphyromonas gingivalis. Front Immunol. (2019) 10:2286. doi: 10.3389/fimmu.2019.02286
36. Hasegawa Y, Nagano K. Porphyromonas gingivalis FimA and Mfa1 fimbriae: Current insights on localization, function, biogenesis, and genotype. Jpn Dent Sci Rev. (2021) 57:190–200. doi: 10.1016/j.jdsr.2021.09.003
37. Inaba H, Sugita H, Kuboniwa M, Iwai S, Hamada M, Noda T, et al. Porphyromonas gingivalis promotes invasion of oral squamous cell carcinoma through induction of proMMP9 and its activation. Cell Microbiol. (2014) 16:131–45. doi: 10.1111/cmi.2014.16.issue-1
38. Inaba H, Yoshida S, Nomura R, Kato Y, Asai F, Nakano K, et al. Porphyromonas gulae lipopolysaccharide elicits inflammatory responses through toll-like receptor 2 and 4 in human gingivalis epithelial cells. Cell Microbiol. (2020) 22:e13254. doi: 10.1111/cmi.13254
39. Liu XB, Gao ZY, Sun CT, Wen H, Gao B, Li SB, et al. The potential role of P.gingivalis in gastrointestinal cancer: a mini review. Infect Agents Cancer. (2019) 14:23. doi: 10.1186/s13027-019-0239-4
40. Hajishengallis G, Tapping RI, Harokopakis E, Nishiyama S, Ratti P, Schifferle RE, et al. Differential interactions of fimbriae and lipopolysaccharide from Porphyromonas gingivalis with the Toll-like receptor 2-centred pattern recognition apparatus. Cell Microbiol. (2006) 8:1557–70. doi: 10.1111/j.1462-5822.2006.00730.x
41. Olsen I, Yilmaz Ö. Possible role of Porphyromonas gingivalis in orodigestive cancers. J Oral Microbiol. (2019) 11:1563410. doi: 10.1080/20002297.2018.1563410
42. Sobocki BK, Basset CA, Bruhn-Olszewska B, Olszewski P, Szot O, Kaźmierczak-Siedlecka K, et al. Molecular mechanisms leading from periodontal disease to cancer. Int J Mol Sci. (2022) 23:970. doi: 10.3390/ijms23020970
43. Shahoumi LA, Saleh MHA, Meghil MM. Virulence factors of the periodontal pathogens: tools to evade the host immune response and promote carcinogenesis. Microorganisms. (2023) 11:115. doi: 10.3390/microorganisms11010115
44. Hajishengallis G, Diaz PI. Porphyromonas gingivalis: immune subversion activities and role in periodontal dysbiosis. Curr Oral Health Rep. (2020) 7:12–21. doi: 10.1007/s40496-020-00249-3
45. Zhang D, Chen L, Li S, Gu Z, Yan J. Lipopolysaccharide (LPS) of Porphyromonas gingivalis induces IL-1beta, TNF-alpha and IL-6 production by THP-1 cells in a way different from that of Escherichia coli LPS. Innate Immun. (2008) 14:99–107. doi: 10.1177/1753425907088244
46. Hajishengallis G, Sojar H, Genco RJ, DeNardin E. Intracellular signaling and cytokine induction upon interactions of Porphyromonas gingivalis fimbriae with pattern-recognition receptors. Immunol Invest. (2004) 33:157–72. doi: 10.1081/imm-120030917
47. Chopra A, Shiheido-Watanabe Y, Eberhard J. Editorial: Porphyromonas gingivalis: molecular mechanisms of invasion, immune evasion, and dysbiosis. Front Cell Infect Microbiol. (2023) 13:1289103. doi: 10.3389/fcimb.2023.1289103
48. Chow YC, Yam HC, Gunasekaran B, Lai WY, Wo WY, Agarwal T, et al. Implications of Porphyromonas gingivalis peptidyl arginine deiminase and gingipain R in human health and diseases. Front Cell Infect Microbiol. (2022) 12:987683. doi: 10.3389/fcimb.2022.987683
49. Salazar CR, Sun J, Li Y, Francois F, Corby P, Perez-Perez G, et al. Association between selected oral pathogens and gastric precancerous lesions. PloS One. (2013) 8:e51604. doi: 10.1371/journal.pone.0051604
50. Rajilic-Stojanovic M, Figueiredo C, Smet A, Hansen R, Kupcinskas J, Rokkas T, et al. Systematic review: gastric microbiota in health and disease. Aliment Pharmacol Ther. (2020) 51:582–602. doi: 10.1111/apt.15650
51. How KY, Song KP, Chan KG. Porphyromonas gingivalis: An Overview of Periodontopathic Pathogen below the Gum Line. Front Microbiol. (2016) 7:53. doi: 10.3389/fmicb.2016.00053
52. Zenobia C, Hajishengallis G. Porphyromonas gingivalis virulence factors involved in subversion of leukocytes and microbial dysbiosis. Virulence. (2015) 6:236–43. doi: 10.1080/21505594.2014.999567
53. Zhang C, Cleveland K, Schnoll-Sussman F, McClure B, Bigg M, Thakkar P, et al. Identification of low abundance microbiome in clinical samples using whole genome sequencing. Genome Biol. (2015) 16:265. doi: 10.1186/s13059-015-0821-z
54. Maeshima N, Fernandez RC. Recognition of lipid A variants by the TLR4-MD-2 receptor complex. Front Cell Infect Microbiol. (2013) 3:3. doi: 10.3389/fcimb.2013.00003
55. Zhao S, Sun M, Meng H, Ji H, Liu Y, Zhang M, et al. TLR4 expression correlated with PD-L1 expression indicates a poor prognosis in patients with peripheral T-cell lymphomas. Cancer Manag Res. (2019) 11:4743–56. doi: 10.2147/CMAR
56. Wang Y, Chung YR, Eitzinger S, Palacio N, Gregory S, Bhattacharyya M, et al. TLR4 signaling improves PD-1 blockade therapy during chronic viral infection. PloS Pathog. (2019) 15:e1007583. doi: 10.1371/journal.ppat.1007583
57. Wu X, Qian S, Zhang J, Feng J, Luo K, Sun L, et al. Lipopolysaccharide promotes metastasis via acceleration of glycolysis by the nuclear factor-κB/snail/hexokinase3 signaling axis in colorectal cancer. Cancer Metab. (2021) 9:23. doi: 10.1186/s40170-021-00260-x
58. Tamura T, Ohira M, Tanaka H, Muguruma K, Toyokawa T, Kubo N, et al. Programmed death-1 ligand-1 (PDL1) expression is associated with the prognosis of patients with stage II/III gastric cancer. Anticancer Res. (2015) 35:5369–76. doi: 10.1186/s40170-021-00260-x
59. Tu L, Guan R, Yang H, Zhou Y, Hong W, Ma L, et al. Assessment of the expression of the immune checkpoint molecules PD-1, CTLA4, TIM-3 and LAG-3 across different cancers in relation to treatment response, tumor-infiltrating immune cells and survival. Int J Cancer. (2020) 147:423–39. doi: 10.1002/ijc.32785
60. Sorensen SF, Zhou W, Dolled-Filhart M, Georgsen JB, Wang Z, Emancipator K, et al. PD-L1 expression and survival among patients with advanced non-small cell lung cancer treated with chemotherapy. Transl Oncol. (2016) 9:64–9. doi: 10.1016/j.tranon.2016.01.003
61. Park JH, Luchini C, Nottegar A, Tizaoui K, Koyanagi A, Ogino S, et al. Effect of CD274 (PD-L1) overexpression on survival outcomes in 10 specific cancers: a systematic review and meta-analysis. J Clin Pathol. (2023) 76:450–6. doi: 10.1136/jcp-2023-208848
62. Costalonga M, Herzberg MC. The oral microbiome and the immunobiology of periodontal disease and caries. Immunol Lett. (2014) 162:22–38. doi: 10.1016/j.imlet.2014.08.017
63. Di Stefano M, Polizzi A, Santonocito S, Romano A, Lombardi T, Isola G. Impact of oral microbiome in periodontal health and periodontitis: A critical review on prevention and treatment. Int J Mol Sci. (2022) 23:5142. doi: 10.3390/ijms23095142
64. Irfan M, Delgado RZR, Frias-Lopez J. The oral microbiome and cancer. Front Immunol. (2020) 11:591088. doi: 10.3389/fimmu.2020.591088
65. Tonelli A, Lumngwena EN, Ntusi NAB. The oral microbiome in the pathophysiology of cardiovascular disease. Nat Rev Cardiol. (2023) 20:386–403. doi: 10.1038/s41569-022-00825-3
66. Segata N, Haake SK, Mannon P, Lemon KP, Waldron L, Gevers D, et al. Composition of the adult digestive tract bacterial microbiome based on seven mouth surfaces, tonsils, throat and stool samples. Genome Biol. (2012) 13:R42. doi: 10.1186/gb-2012-13-6-r42
67. Atarashi K, Suda W, Luo C, Kawaguchi T, Motoo I, Narushima S, et al. Ectopic colonization of oral bacteria in the intestine drives TH1 cell induction and inflammation. Science. (2017) 358:359–65. doi: 10.1126/science.aan4526
68. Choi E, Chang MS, Byeon SJ, Jin H, Jung KC, Kim H, et al. Prognostic perspectives of PD-L1 combined with tumor-infiltrating lymphocytes, Epstein-Barr virus, and microsatellite instability in gastric carcinomas. Diagn Pathol. (2020) 15:69. doi: 10.1186/s13000-020-00979-z
69. Groeger S, Wu F, Wagenlehner F, Dansranjav T, Ruf S, Denter F, et al. PD-L1 up-regulation in prostate cancer cells by porphyromonas gingivalis. Front Cell Infect Microbiol. (2022) 12:935806. doi: 10.3389/fcimb.2022.935806
70. Tan Q, Ma X, Yang B, Liu Y, Xie Y, Wang X, et al. Periodontitis pathogen Porphyromonas gingivalis promotes pancreatic tumorigenesis via neutrophil elastase from tumor-associated neutrophils. Gut Microbes. (2022) 14:2073785. doi: 10.1080/19490976.2022.2073785
71. Öğrendik M. Oral bacteria in pancreatic cancer: mutagenesis of the p53 tumour suppressor gene. Int J Clin Exp Pathol. (2015) 8:11835–6. doi: 10.1080/19490976.2022.2073785
72. Yu J, Ling S, Hong J, Zhang L, Zhou W, Yin L, et al. TP53/mTORC1-mediated bidirectional regulation of PD-L1 modulates immune evasion in hepatocellular carcinoma. J Immunother Cancer. (2023) 11:e007479. doi: 10.1136/jitc-2023-007479
73. Fischbach MA. Microbiome: focus on causation and mechanism. Cell. (2018) 174:785–90. doi: 10.1016/j.cell.2018.07.038
74. Corander J, Hanage WP, Pensar J. Causal discovery for the microbiome. Lancet Microbe. (2022) 3:e881–7. doi: 10.1016/S2666-5247(22)00186-0
75. Zaura E, Pappalardo VY, Buijs MJ, Volgenant CMC, Brandt BW. Optimizing the quality of clinical studies on oral microbiome: A practical guide for planning, performing, and reporting. Periodontology 2000. (2021) 85:210–36. doi: 10.1111/prd.12359
76. Rosenstein ED, Greenwald RA, Kushner LJ, Weissmann G. Hypothesis: the humoral immune response to oral bacteria provides a stimulus for the development of rheumatoid arthritis. Inflammation. (2004) 28:311–8. doi: 10.1007/s10753-004-6641-z
77. Wegner N, Wait R, Sroka A, Eick S, Nguyen KA, Lundberg K, et al. Peptidylarginine deiminase from Porphyromonas gingivalis citrullinates human fibrinogen and α-enolase: implications for autoimmunity in rheumatoid arthritis. Arthritis Rheumatol. (2010) 62:2662–72. doi: 10.1002/art.27552
78. Everett C, Li C, Wilkinson JE, Nguyen LH, McIver LJ, Ivey K, et al. Overview of the Microbiome Among Nurses study (Micro-N) as an example of prospective characterization of the microbiome within cohort studies. Nat Protoc. (2021) 16:2724–31. doi: 10.1038/s41596-021-00519-z
79. Teles FRF, Alawi F, Castilho RM, Wang Y. Association or causation? Exploring the oral microbiome and cancer links. J Dent Res. (2020) 99:1411–24. doi: 10.1177/0022034520945242
80. Younginger BS, Mayba O, Reeder J, Nagarkar DR, Modrusan Z, Albert ML, et al. Enrichment of oral-derived bacteria in inflamed colorectal tumors and distinct associations of Fusobacterium in the mesenchymal subtype. Cell Rep Med. (2023) 4:100920. doi: 10.1016/j.xcrm.2023.100920
Keywords: P. gingivalis, microbiome, PAMPS, gastric cancer, PD1/PDL1 axis
Citation: Muñoz-Medel M, Pinto MP, Goralsky L, Cáceres M, Villarroel-Espíndola F, Manque P, Pinto A, Garcia-Bloj B, de Mayo T, Godoy JA, Garrido M and Retamal IN (2024) Porphyromonas gingivalis, a bridge between oral health and immune evasion in gastric cancer. Front. Oncol. 14:1403089. doi: 10.3389/fonc.2024.1403089
Received: 18 March 2024; Accepted: 02 May 2024;
Published: 14 May 2024.
Edited by:
Xiaomin Cai, University of Miami, United StatesReviewed by:
Bo Jiang, Atila Biosystems, United StatesJiakun Lu, University of California, Los Angeles, United States
Xin Xu, Bristol Myers Squibb, United States
Copyright © 2024 Muñoz-Medel, Pinto, Goralsky, Cáceres, Villarroel-Espíndola, Manque, Pinto, Garcia-Bloj, de Mayo, Godoy, Garrido and Retamal. This is an open-access article distributed under the terms of the Creative Commons Attribution License (CC BY). The use, distribution or reproduction in other forums is permitted, provided the original author(s) and the copyright owner(s) are credited and that the original publication in this journal is cited, in accordance with accepted academic practice. No use, distribution or reproduction is permitted which does not comply with these terms.
*Correspondence: Ignacio N. Retamal, aWduYWNpby5yZXRhbWFsQHVtYXlvci5jbA==
†ORCID: Matías Muñoz-Medel, orcid.org/0000-0002-1262-8052
Mauricio P. Pinto, orcid.org/0000-0003-2484-8033
Lauren Goralsky, orcid.org/0000-0002-2420-1312
Mónica Cáceres, orcid.org/0000-0002-0456-0721
Franz Villarroel-Espíndola, orcid.org/0000-0003-0080-2444
Patricio Manque, orcid.org/0000-0002-6606-0896
Andrés Pinto, orcid.org/0000-0003-2101-8886
Benjamin Garcia-Bloj, orcid.org/0000-0001-6634-025X
Juan A. Godoy, orcid.org/0000-0001-9920-6698
Marcelo Garrido, orcid.org/0000-0001-8905-8986
Ignacio N. Retamal, orcid.org/0000-0003-4633-2556