- 1Department of Clinical and Molecular Pathology, Institute of Molecular and Translational Medicine, Faculty of Medicine and Dentistry, Palacký University and University Hospital, Olomouc, Czechia
- 2Department of Oncology, Faculty of Medicine and Dentistry, Palacký University and University Hospital, Olomouc, Czechia
- 3Department of Cytokinetics, Institute of Biophysics of the Czech Academy of Sciences, Brno, Czechia
Background: Prostate cancer is the second leading cause of male cancer-related deaths in Western countries, which is predominantly attributed to the metastatic castration-resistant stage of the disease (CRPC). There is an urgent need for better prognostic and predictive biomarkers, particularly for androgen receptor targeted agents and taxanes.
Methods: We have searched the PubMed database for original articles and meta-analyses providing information on blood-based markers for castration-resistant prostate cancer monitoring, risk group stratification and prediction of therapy response.
Results: The molecular markers are discussed along with the standard clinical parameters, such as prostate specific antigen, lactate dehydrogenase or C-reactive protein. Androgen receptor (AR) alterations are commonly associated with progression to CRPC. These include amplification of AR and its enhancer, point mutations and splice variants. Among DNA methylations, a novel 5-hydroxymethylcytosine activation marker of TOP2A and EZH2 has been identified for the aggressive disease. miR-375 is currently the most promising candidate among non-coding RNAs and sphingolipid analysis has recently emerged as a novel approach.
Conclusions: The promising biomarkers have the potential to improve the care of metastatic prostate cancer patients, however, they need further validation for routine implementation.
1 Introduction
Prostate cancer (PC) is the most common malignancy and the second leading cause of male cancer related deaths in developed countries (1). PC begins to grow as an asymptomatic localized cancer. However, PC is often diagnosed in the higher stage when it continues to grow through the prostate envelope and becomes advanced cancer, making treatment more challenging. While prostatectomy is an option for localized or locally advanced PC, androgen-deprivation therapy (ADT) is commonly employed for metastatic disease (Figure 1).
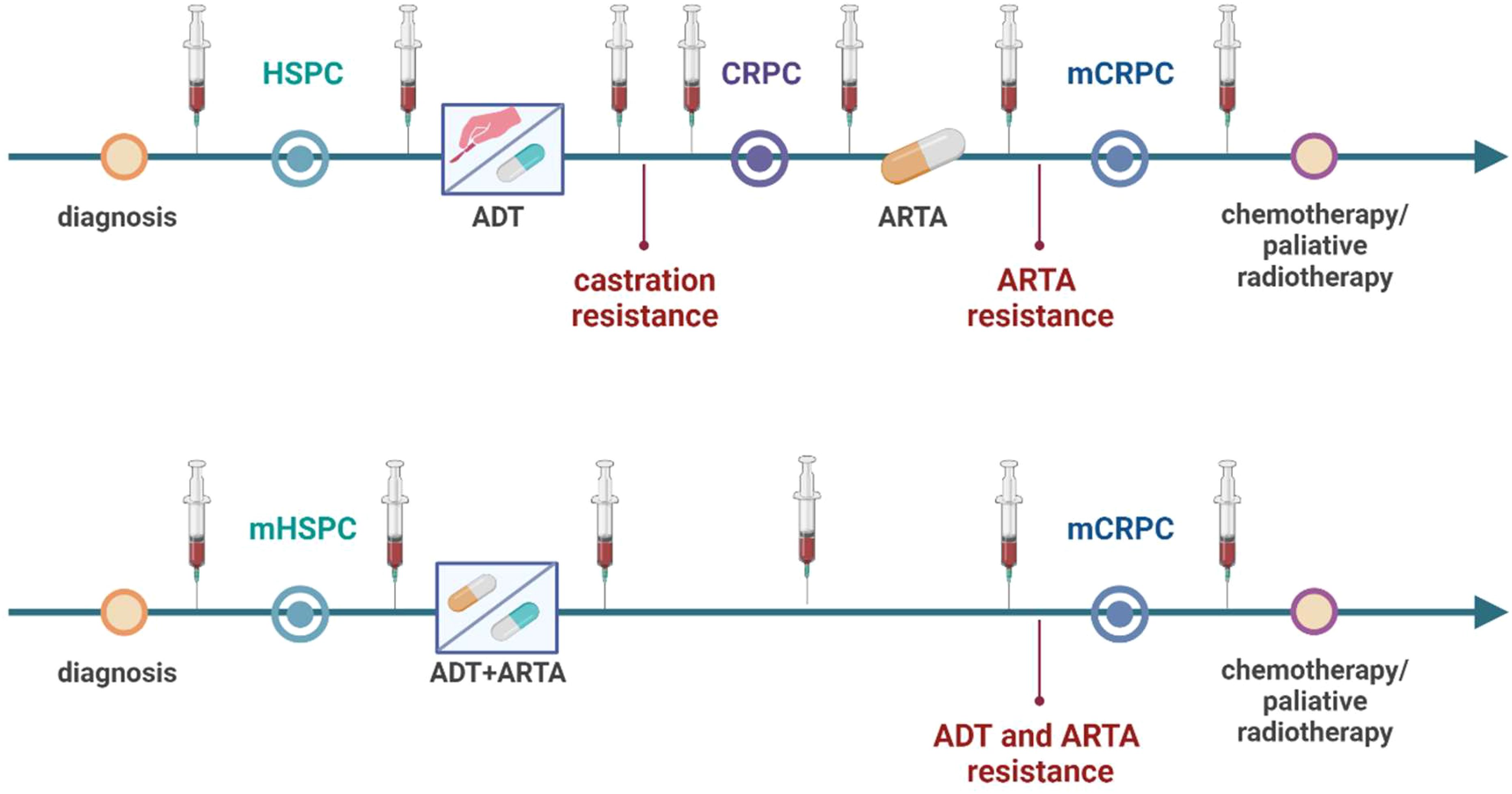
Figure 1. Scheme of liquid biopsy sampling in relation to different treatment schemes in hormone-sensitive prostate cancer (HSPC) and castration-resistant prostate cancer patients (CRPC). HSPC patients are first treated with androgen-deprivation therapy (ADT) and androgen-receptor targeted agents (ARTA) or androgen-receptor pathway inhibitors (ARPI) are used after castration resistance occurrence leading to ARTA resistance and metastatic CRPC (mCRPC). Now, based on novel treatment protocol metastatic HSPC patients treated with ADT and ARTA combination leading to double-resistance and mCRPC. Liquid biopsy sampling can be performed at different time points throughout the course of treatment for monitoring of progression and metastatic activity of the cancer. Created with BioRender.com.
PC cells are often androgen-dependent and need androgen stimuli for proliferation. Therefore, ADT aims to reduce androgen concentration and inhibit PC cell growth. Over time, castration-resistant PC (CRPC) can emerge, showing resistance to ADT. Some androgens can still be produced by the adrenal glands, fuelling PC growth, and AR-pathway inhibitors (ARPI) are used to neutralize this androgen effect by acting as androgen antagonists (e.g., enzalutamide) or further inhibit androgen production (e.g., abiraterone). ARPI are also known as AR-targeting agents (ARTA) (2).
The therapeutic armamentarium for treating metastatic CRPC (mCRPC) in clinical practice has evolved in recent decades. Nowadays, there are several classes of therapeutic options to manage the disease progression. Besides chemotherapeutic agents such as docetaxel and cabazitaxel, ARPI, innovative radioligand therapy represented by Lu-PSMA, and PARP inhibitors have extended the therapeutic scheme. Nevertheless, the specific biomarkers to choose the best therapeutic approach are still challenging to find because of the heterogeneity of CRPC (3, 4). Therefore, the liquid biopsy (LB) can offer a tremendous predictive tool for future personalized approaches, providing varied material for molecular examinations (Figure 2). Recent reviews were primarily dedicated to circulating tumor cells (5–8), and we have also focused on their potential in modeling metastatic PC (9). It’s worth noting that urine testing also holds great potential for examining PC patients; for more detailed information about LB based on urine examination, see the dedicated review (10). This article aims to present circulating cell-free markers with potential value in progression monitoring of CRPC from blood samples, including whole blood, plasma, or serum. Importantly, novel markers should be evaluated in the context of the established clinical protocols and currently available tools.
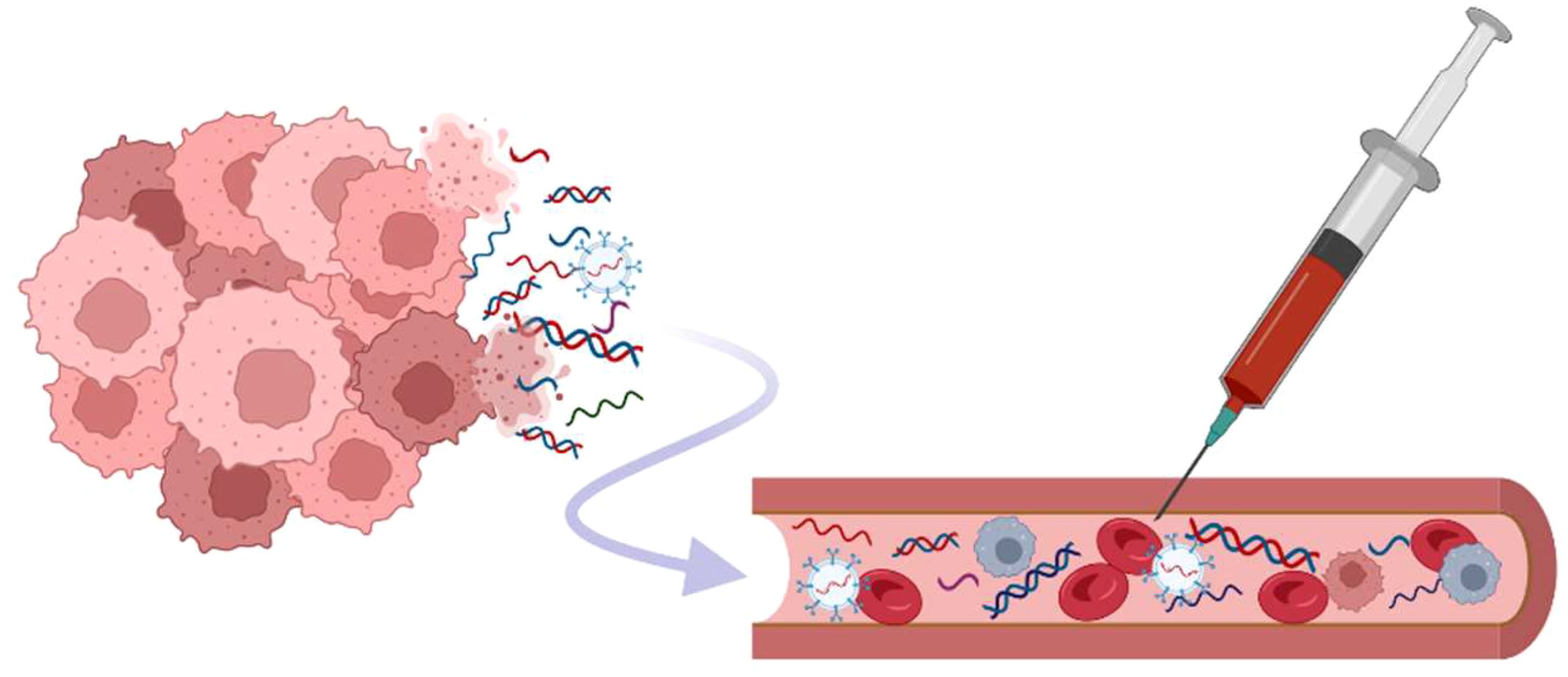
Figure 2. Different types of biomarkers are present in circulation. The cell-free DNAs, various types of RNAs, as well as nucleic acid captured in exosomes, are released from cancer cells in different pathological or normal biological processes (apoptosis, necrosis, tissue damage, …). There is a need for standardization of both the acquisition of samples and validation of methods used for the analysis to ensure the reproducibility of analysis and the accuracy of the results (measured in one workspace or across laboratories). Created with BioRender.com.
2 Prognostic biomarkers for risk group stratification from a perspective of clinical practice
The current decision-making by the physicians is typically based on metastasis sites (nodes, bones, visceral), the progression pace, and the previous road of the treatment (3). Biomarkers extensively studied as a prognostic factor in patients with mCRPC are inflammatory response cells. The most valuable benefit of these markers is their easy accessibility in every clinical laboratory, cost-effectiveness, the possibility to evaluate them retrospectively, and their known prognostic correlation with many malignancies. Altered infiltration of the tumor microenvironment with various subgroups of lymphocytes is often associated with a prognosis and inflammation-promoted progression of the malignancy. The most used inflammation-related indicators are the neutrophil-to-lymphocyte ratio (NLR), platelet-to-lymphocyte ratio (PLR), and C-reactive protein (CRP).
In several meta-analyses, it was shown that higher NLR (cut-off value >3) correlates with poor overall survival (OS) in patients with advanced and metastatic PC treated with abiraterone or enzalutamide (11, 12). Recent reviews dedicated to cells of inflammation and their prognostic role in PC can be found elsewhere (13). Just for demonstration, Lolli et al. published a retrospective study with 230 patients diagnosed with mCRPC treated with abiraterone. The patients with NLR higher than three prior abiraterone had 14.7 months median OS, and those with NLR lower than 3 had 20.4 months median OS (14). Similar conclusions were also reported in a study by Koo and coworkers. They performed a retrospective analysis of 303 mCRPC patients treated with docetaxel before/after ARPI therapy. The NLR cut-off value of 2.5 was used for the stratification. The patients with NLR lower than 2.5 had better cancer-specific survival (CSS). Hence, this group (NLR<2.5) profited from the treatment sequence docetaxel-to-ARPI with better progression-free survival (PFS) and CSS than the group with the opposite treatment sequence (15).
PLR is another inflammation-related biomarker often reported with NLR as a systemic immune-inflammation index (SII). This index overall reflects the immune status connected with prognosis as the increased platelets can play an active role in the development of distant bone metastasis (16), while tumor-related neutrophils promote tumor growth and progression (17). On the other hand, lymphocytes have an anti-tumor role connected with apoptosis and proliferation suppression (17). Thus, the SII reflects the infiltration of the tumor microenvironment with immune cells that will determine further progression. The most used cut-off value for stratification is 535; patients with higher values have worse median OS and a more significant hazard ratio (18). The inflammatory response cells, therefore, can efficiently serve as effective biomarkers for prognosis prediction for patients with mCRPC.
CRP and procalcitonin (PCT), another non-specific inflammation-related indicator, have been associated with any inflammation within the body. They are usually increased in case of systemic infection or tissue damage but have also been described as reflecting the prognosis of cancer. In PC patients, the role of CRP has so far been inconclusive. Elevated preoperative CRP (CRP≥5mg/L) correlated with a postoperative pathological diagnosis of PC with aggressive patterns (19). Similarly, CRP levels before prostate biopsy were associated with increased Gleason score (20). Regarding prognosis, the higher levels of CRP were assigned as an independent predictor of poor OS in mCRPC (21). On the contrary, other studies claimed the absence of any correlation between CRP and PC (22, 23). PCT was helpful in terms of PC diagnostic accuracy since PCT was demonstrated to correlate with PC development (24, 25).
As an immune-related biomarker, CRP is often used in multivariable analysis to boost the significance of mCRPC stratification. The Glasgow Prognostic Score (GPS), used to determine cancer outcomes in general, is worth mentioning. The value of CRP and albumin from this prognostic score can even be explicitly refined for mCRPC diagnosis as was done in the work of Ando et al. in 2021. They set the cut-off value of CRP to 3 mg/L and albumin to 35 g/L to get to the high-sensitivity modified Glasgow prognostic score (26). These values were used for stratification of mCRPC patients treated with docetaxel; the clinical significance of this score can be seen in Table 1. A better result was reached when this score was combined with starting prostate-specific antigen (PSA) and testosterone levels (Table 1).
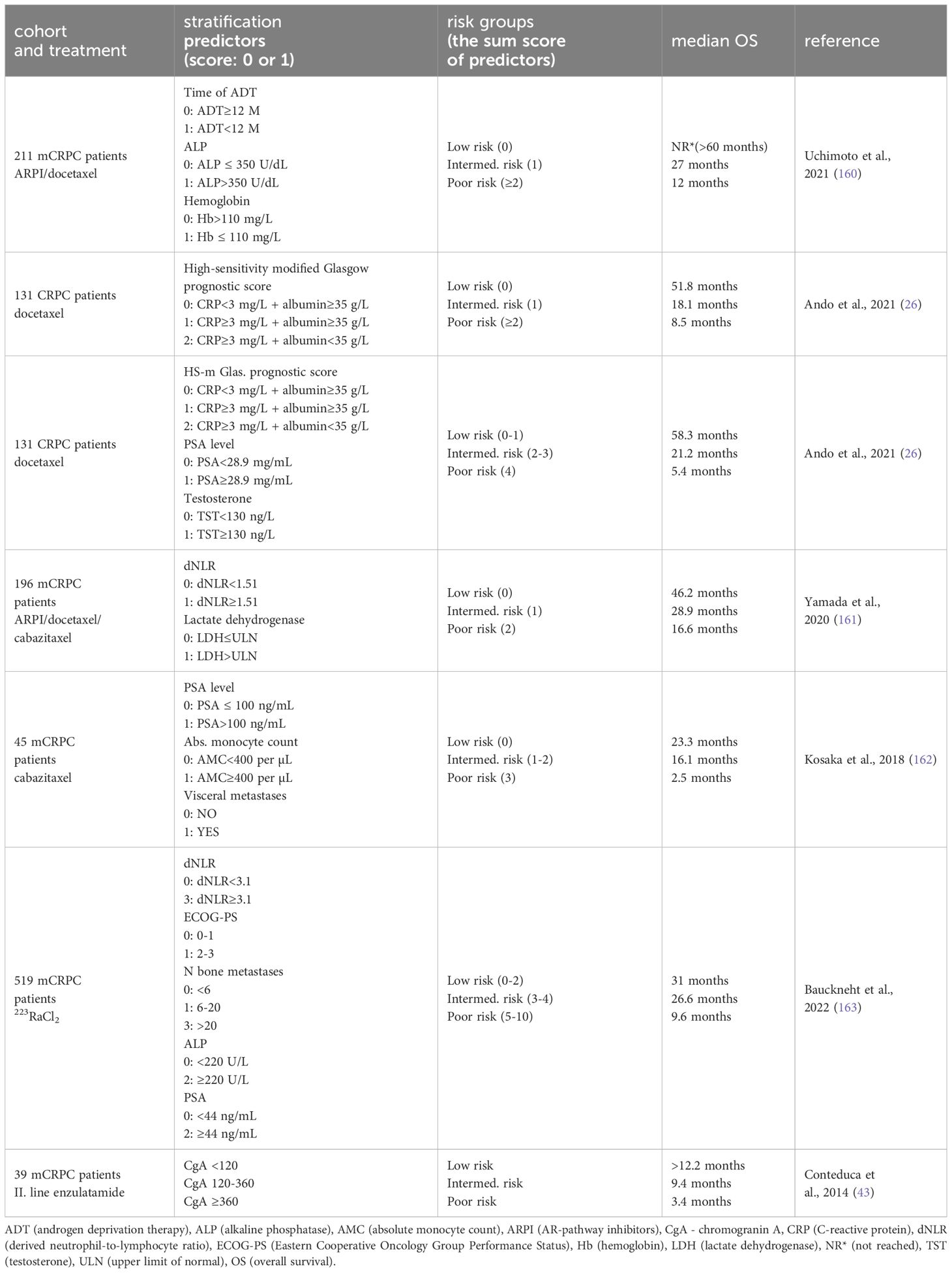
Table 1. Prognostic stratification of metastatic castration-resistant prostate cancer (mCRPC) patients based on clinical biomarkers and various cancer and health-related factors.
PSA is often used as a monitoring tool for the progression of the mCRPC disease. According to the Response Evaluation Criteria in Solid Tumors (RECIST) version 1.1, the progression of PC is defined as three consecutive elevated values of PSA resulting in two 50% rises over the nadir or the appearance of ≥2 bone lesions on bone scan or soft tissue lesions enlargement on computed tomography (CT). Nevertheless, the level of PSA and PSA kinetic are treatment-sensitive parameters. For example, non-rising PSA with metastatic radiographic progression is often observed in mCRPC patients treated with enzalutamide (27). In combination with the heterogeneity of the disease, the non-rising PSA can lead to delayed detection of the progressing disease. Similarly, initial PSA and mainly PSA response (PSAr; ≥50% decrease) as stratification factors must be used cautiously and only in cohorts with the same type of treatment. Still, the PSA kinetic (PSA NADIR, time to NADIR) during the initial ADT (during metastatic hormone-sensitive PC) can be helpful prognostic factors for patients with further PC progression to mCRPC. For example, in a study by Hamano and coworkers, the patients with PSA nadir lower than 0.64 ng/mL in less than seven months after the start of ADT had better OS after mCRPC diagnosis (28).
Several other biomarkers can also be found as factors in multivariable analysis, improving the prognostic model performance. Besides the previously mentioned biomarkers, such as PSA, PSA kinetic, and inflammatory response cells, the other biomarkers can be divided into several categories. Those are biomarkers and factors specifically related to PC, such as the level of testosterone, duration of ADT before mCRPC diagnosis, and localization of metastasis sides (nodes, bones, visceral). General cancer-related biomarkers are represented by alkaline phosphatase (ALP) – an indicator of bone metastatic tumor load (29), lactate dehydrogenase (LDH) – an increased biomarker of highly proliferating cancer cells connected with enhanced glycolysis (30), and presence of CTCs (31, 32). In the last category, other health-related features, such as hemoglobin level, albumin level, or performance status, can be found. All these factors have been repeatedly used to boost the stratification significance for patients diagnosed with mCRPC (33–35). Several combinations and their prognostic model performance are shown in Table 1. Nevertheless, despite the depth of the current understanding of multivariable prognostic indicators, none have been accepted as a tool in clinical practice.
Beyond the general biomarkers listed above, a particular category related to neuroendocrine phenotype related to poor prognosis must be highlighted. Typically, the histologic feature of de novo-diagnosed patients is prostate adenocarcinoma. However, in some cases, neuroendocrine differentiation of the primary adenocarcinoma histology can be detected during treatment (36). So far, two mechanisms have been suggested for the emergence of this very aggressive form of PC. The first is based on the fact that neuroendocrine cells can be sparsely distributed in the original adenocarcinoma cells. Since the malignant neuroendocrine cells are not sensitive to androgen inhibition, they can start to grow during hormonal therapy and cause resistance to treatment (clonal expansion) (37). The second mechanism, transdifferentiation of adenocarcinoma cells to neuroendocrine prostate cancer, is often observed after androgen deprivation and other stress stimuli (38, 39). Still, this highly complex process can also be related to some genomic alterations (PTEN, TP53, RB1, epigenetic events) (40, 41). Notably, the proliferation of neuroendocrine cells will be reflected in an increased expression of typical neuroendocrine biomarkers such as chromogranin A (CgA), enolase 2 or immunohistochemistry markers (42). Therefore, these biomarkers can be used as indicators of poor survival outcomes. Conteduca et al. (43) analyzed serum CgA levels and their dynamics during the course of therapy with enzalutamide. This retrospective study showed that mCRPC patients with CgA level above 360 ng/mL before enzalutamide treatment in the second line had much worse OS (3.4 months) than the patients with the initial CgA A level below 120 ng/mL (OS not reached for 12 months). Therefore, increased neuroendocrine biomarkers can, independently from PSA, predict a poor survival outcome. Suspicion of the development of an aggressive variant of prostate cancer with low PSA level can be also reflected in carcinoembryonic antigen (CEA) (44) or other clinical manifestations, including lytic bone metastases, bulky lymphadenopathy, exclusive visceral metastases along with short intervals of ADT response (45).
3 Circulating cell-free DNA and AR signaling
The circulating cell-free DNA (ccfDNA) molecules are predominantly random fragments with a length of about 180 base pairs formed during cell disintegration (46). Cancer cells contribute significantly to ccfDNA levels in the blood, leading to a higher abundance of cancer-derived ccfDNA. Healthy individuals typically have about 1-10 ng of ccfDNAs in one mL of blood (47, 48) and those levels are consistently elevated in cancer patients (49–51). Despite not being intact, ccfDNA molecules are still valuable for predictive and diagnostic purposes. Specific assays can examine different gene changes, such as point mutations, deletions, or amplifications (46). Furthermore, the amount of tumor-originated ccfDNA in plasma has been suggested as an independent prognostic biomarker for CRPC, in particular in combination with PSA evaluation (52–54). For more in-depth information on ccfDNA, dedicated reviews are available (48, 55–60).
Androgen receptor (AR) primarily drives PC proliferation and disease progression, and its signaling pathway is targeted by both ADT and ARPI. PC can develop various types of resistance, some of which are based on genetic or proteomic modifications of the AR (61, 62). The AR gene can be amplified (copy-number variation, CNV) or altered by point mutations primarily occurring in the ligand binding site (63–65). Approximately 60% of mCRPC samples exhibit aberrations in the AR gene (66), with nearly 50% of mCRPC cases showing AR gene amplification (67, 68). The plasma samples of CRPC and hormonal-sensitive patients were examined for AR CNV. However, CNV of AR was found exclusively in CRPC patients (69). AR gene amplification was associated with worse OS and/or PFS in both ADT and ARPI-treated patients (70–73). Later, CNV in different regions of the AR gene was found in 38% of mCRPC samples by Du et al. in 2020. They also suggested the assessment of CNV in the AR enhancer region and CNV in exon 8 of the AR gene as an excellent prognostic marker. The AR enhancer is a region 650 kb upstream of AR that contributes to the progression of mCRPC (69, 74, 75). The AR and its enhancer were also analyzed by targeted sequencing, which found alterations in 45% of metastatic patients (76). Importantly, this assay revealed amplification of AR/enhancer in 78% of patients with resistance to AR-directed therapy.
The AR gene point mutations are present in 15-20% of CRPC cases (68, 77). The missense AR mutations, including H875Y, T878A, and F876L, were detected in 18% of 62 CRPC patients treated with abiraterone (n=29), enzalutamide (n=19) or other agents (n=14) (73). The mutations H875Y and L702H were described in 11% of post-docetaxel, but in no chemotherapy-naïve, abiraterone-treated CRPC patients (71). Moreover, the presence of AR gene mutations H875Y and L702H was associated with worse OS in patients treated with abiraterone, or enzalutamide (71). The abiraterone-treated CRPC patients with AR aberration (mutations L702H or T878A or CNV of AR) had a higher risk of progression or primary resistance to abiraterone (70), and a significantly shorter PFS when AR aberrations (CNV of AR or mutations H875Y, L702H, and T878A) were analyzed (78). Interestingly, the presence of AR gene aberrations was not in correlation with the PFS of enzalutamide-treated CRPC patients in the same study (78). Importantly, whole genome sequencing of clinical samples showed a dramatically increased rate of AR binding sites which contributes to the upregulation of target genes and cancer progression (79). The AR alterations in relationship to PC progression and therapy are summarised in Table 2.
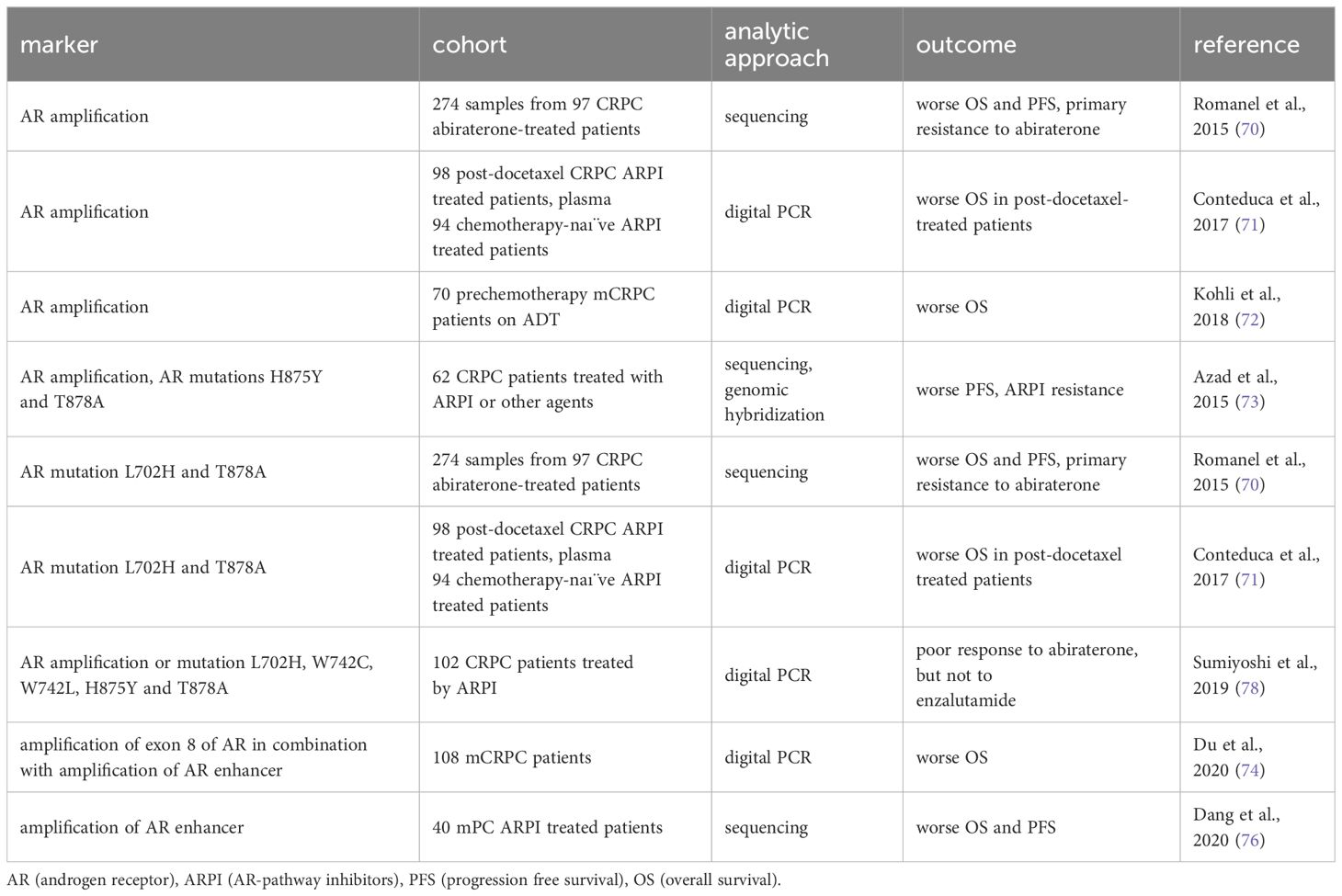
Table 2. The ccfDNA AR markers from plasma useful for prognostic stratification of CRPC (castration-resistant prostate cancer).
4 Methylation markers in CRPC
Apart from DNA alterations of the AR gene, the DNA methylation landscape of PC has gained interest as a potential marker for PC progression. Notably, ccfDNA extracted from CRPC patients’ plasma contains approximately 64% of tumor-specific DNA methylation patterns, making it suitable for investigating epigenetic changes in PC (80). In cases of PC progression, there is a notable increase in the levels of methylation in genes GSTP1, RASSF1A, APC, and RARB compared to patients without progression (81). The loss of GSTP1, RASSF1A, APC, and RARB expression due to methylation is observed even in the early stages of tumorigenesis as they serve as tumor suppressors involved in DNA repair, cell adhesion, cell cycle control, and signal transduction (82–84). Hypermethylation of GSTP1 and APC genes has also been elevated in the plasma of CRPC patients and was correlated with shorter OS (85). Additionally, the methylation of at least one gene among GSTP1, RASSF1a, APC, PTGS2, or MDR1 within plasma ccfDNA was associated with poor mCRPC patient outcomes (86). Besides the genes mentioned above, the hypermethylation of MDR1 may contribute to PC proliferation and drug resistance, while PTGS2 silencing was associated with an elevated chance of PC recurrence (87, 88).
Two other studies have identified methylation markers of poor OS. Methylation occurring in the promoter region of the cadherin 13 (CDH13) gene correlated with worse survival and elevated risk of death in serum samples from primary PC patients compared to age-matched controls (89). Notably, levels of methylated GSTP1 gene were detectable in 458 (81%) of mCRPC patients before undergoing docetaxel treatment, and these levels were associated with worse OS. Additionally, patients exhibiting methylated GSTP1 levels after two chemotherapy cycles experienced worsened OS and a shorter time to PSA progression (90). Moreover, a distinct methylation signature within the genes AKR1B1, LDAH, and KLF8 was identified as predictive of therapy failure in mCRPC patients’ plasma samples (91). The molecular consequences of silencing the genes mentioned above in PC prognosis and prediction of therapy response have yet to be clearly explained. Some clues may be found for KLF8 and LDAH. The KLF8 enhances the transcriptional activity of AR (92) and its methylation in therapy-resistant PC may indicate androgen-independent growth. Loss of LDAH, known as lipid droplet-associated hydrolase, has already been associated with prostate tumorigenesis (93). However, the molecular association between LDAH silencing, lipid metabolism, and driving cancer pathways has not been described yet.
The ccfDNA methylation of DOCK2, HAPLN3, and FBXO30 genes correlated with a shorter time to progression and CRPC occurrence and could be used for the identification of patients who could benefit from intensified treatment (94). While cytosine conversion to 5-methylcytosine commonly results in transcriptional repression, further conversion to 5-hydroxymethylcytosine (5hmC) is associated with transcriptional activation. Importantly, recent targeted sequencing of cell-free DNA has found 5hmC activation marker of proliferation TOP2A, and a marker of cell invasion and angiogenesis EZH2 in patients with an aggressive subtype of metastatic disease (95–97). TOP2A is an essential nuclear enzyme required to resolve topological stress associated with DNA replication (98). Its upregulation may induce rearrangements of genes that contribute to a more invasive phenotype. It has also been reported to enhance androgen receptor signaling by facilitating the transcription of androgen-responsive genes (99). EZH2, the catalytic subunit of the polycomb repressive complex 2, works in concert with histone deacetylases as epigenetic modifiers (100). Dual upregulation of TOP2A and EZH2 has also been associated with recurrence after prostatectomy as well as radiotherapy (101, 102).
The new generation sequencing analysis of ccfDNA methylome from CRPC plasma identified enrichment of AR binding sequences and hypomethylation of putative AR binding sites associated with the amplification of the AR gene and a more aggressive clinical course (103). Interestingly, the trimethylation of histone H3 lysine 27 (H3K27me3) has been implicated as an epigenetic marker potentially linked to prostate carcinogenesis (104). A significant decrease in H3K27me3 levels was found in the plasma of metastatic PC patients compared to those with localized or locally advanced PC (105). The ccfDNA methylation markers of PC progression are summarised in Table 3.
5 Messenger RNA of AR and its splice variants
PC cells can develop different splice variants of AR (ARv) during resistance development (106). There are more than 20 known ARv (107), and they mostly do not contain a ligand binding domain (LBS), which is replaced by a variant-specific short peptide (108). The ARv7 has received the most attention as it is the most abundant ARv in PC (109) and contributes to PC growth in a low-androgen environment (110, 111). The connection between ARv7 expression and the highly aggressive PC was investigated in many studies, mostly with the use of CTCs (76, 112–116).
The usefulness of ARv7 as a marker of CRPC progression was discussed in two meta-analyses in 2020 with the same results. The ARv7 significantly correlated with the worst outcome in mCRPC patients treated with hormonal therapy (the worst OS, PFS, and PSA-PFS) or chemotherapy (OS, and PFS) with the use of data from 13 studies (117) or data from 21 studies (118). These findings were further supported by the recent meta-analysis by Khan et al., where the presence of ARv7 significantly correlated with shorter OS, PFS, and PSA-PFS in the whole CRPC-patients’ group as well as in ARPI-treated CRPC group (with a hazard ratio of 4.34). Need to note the majority of the 37 studies analyzed ARv7 in CTCs, and only 7 used whole blood or exosomal RNA (119). For instance, Qu et al. correlated ARv7 and PSA ccfRNA levels in the plasma of 81 abiraterone- and 51 enzalutamide-treated CRPC patients (120). All patients with higher ARv7 transcript levels had a shorter time to treatment failure. On the other hand, when considering multiple factors together in a multivariate model, significant results were only observed in the enzalutamide-treated patients. In a subsequent study conducted by Del Re et al. in 2019, full-length AR and ARv7 were analyzed in plasma samples from 73 CRPC patients before ARPI treatment using digital droplet PCR. They were able to identify full-length AR in all samples and ARv7 in 22% of samples. Moreover, a high number of AR copies (≥ 900 copies/mL) in plasma correlated with shorter PFS and OS. ARv7 showed an even better predictive value (121). A study by Stupolyte and colleagues evaluated the second most common ARv in PC samples ARv1 (108), which was present in 17% of CRPC plasma samples. Both elevated AR levels and the presence of ARv1 were associated with shorter PFS and OS (122). The ccf mRNA markers of CRPC progression are summarised in Table 4.
6 Non-coding ccfRNAs
The non-coding RNAs can be categorized based on their length into small non-coding RNAs (micro-RNAs, miRNAs) and long non-coding RNAs (lncRNAs). Plasma and serum samples can be utilized with comparable results for detecting miRNAs. Moreover, miRNAs demonstrate stability in the blood due to protection from endogenous RNAse activity (123). Several studies have provided insights into the possible role of miRNAs in the PC progression and development of CRPC (124–127).
The significance of miR-375 was demonstrated by several studies. The expression of miR-141 and miR-375 correlated with positive lymph node status and Gleason score in 71 samples of mCRPC (128). The upregulated miR-141, miR-375, and miR-378 were described in serum from CRPC patients, while miR-409-3p was significantly underexpressed compared to serum from low-risk localized patients (129).
Furthermore, the levels of miR-141 and miR-375 were associated with the docetaxel and ARPI treatment outcome in mCRPC patients. Levels of four miRNAs decreased after therapy started. However, levels of miR-141 and miR-375 elevated again at the time of radiological progression, showing potential to be markers of therapy failure and PC progression (130). Additionally, the miR-375 levels predicted which patients would develop metastases with 50% sensitivity, and 76% specificity (131) and were associated with progression-free survival in mCRPC patients treated with enzalutamide (132). The miR-375 can also be associated with non-cancerous diagnoses, e.g. diabetes (133–135). Further investigation is required to understand the impact of lifestyle, diet, and even circadian rhythms on cell-free molecules release into the bloodstream.
The patients with high expression of miR-7 and miR-221 in whole peripheral blood samples had shorter time to CRPC development. They had shorter OS than patients with low expression of these miRNAs. Therefore, the authors suggested the use of miR-7 for the prediction of CRPC occurrence (136). In the study by Lin et al., 2014, they analyzed 96 CRPC docetaxel-treated patients’ blood or plasma samples by a custom Taqman Array for 46 candidate miRNAs. While specific prognostic miRNAs were not identified, a combination of pre-docetaxel miR-200b levels, post-docetaxel miR-20a levels, pre-docetaxel hemoglobin levels, and visceral metastasis proved to be independent predictors of OS when used together.
In 2013, Watahiki et al. described 63 upregulated plasma miRNAs and four downregulated miRNAs in mCRPC compared to localized PC. They could distinguish between mCRPC and localized PC with higher specificity and sensitivity with the use of a specific combination of miRNAs rather than with the use of one miRNA. One set consisted of miR-141, miR-375 and miR-200c, the second included miR-151-3p, miR-423-3p, miR-126, miR-152 and miR-2, and the last miR-16 and miR-205. There were three most important molecules miR-141, miR-151-3p, and miR-16 from each group that increased the sensitivity of the PSA test and could be used for discrimination between localized PC and mCRPC (138). While these findings show promise, validation on a large cohort of patients is still required for routine use in personalized medicine. The prognostic miRNAs are summarized in Table 5.
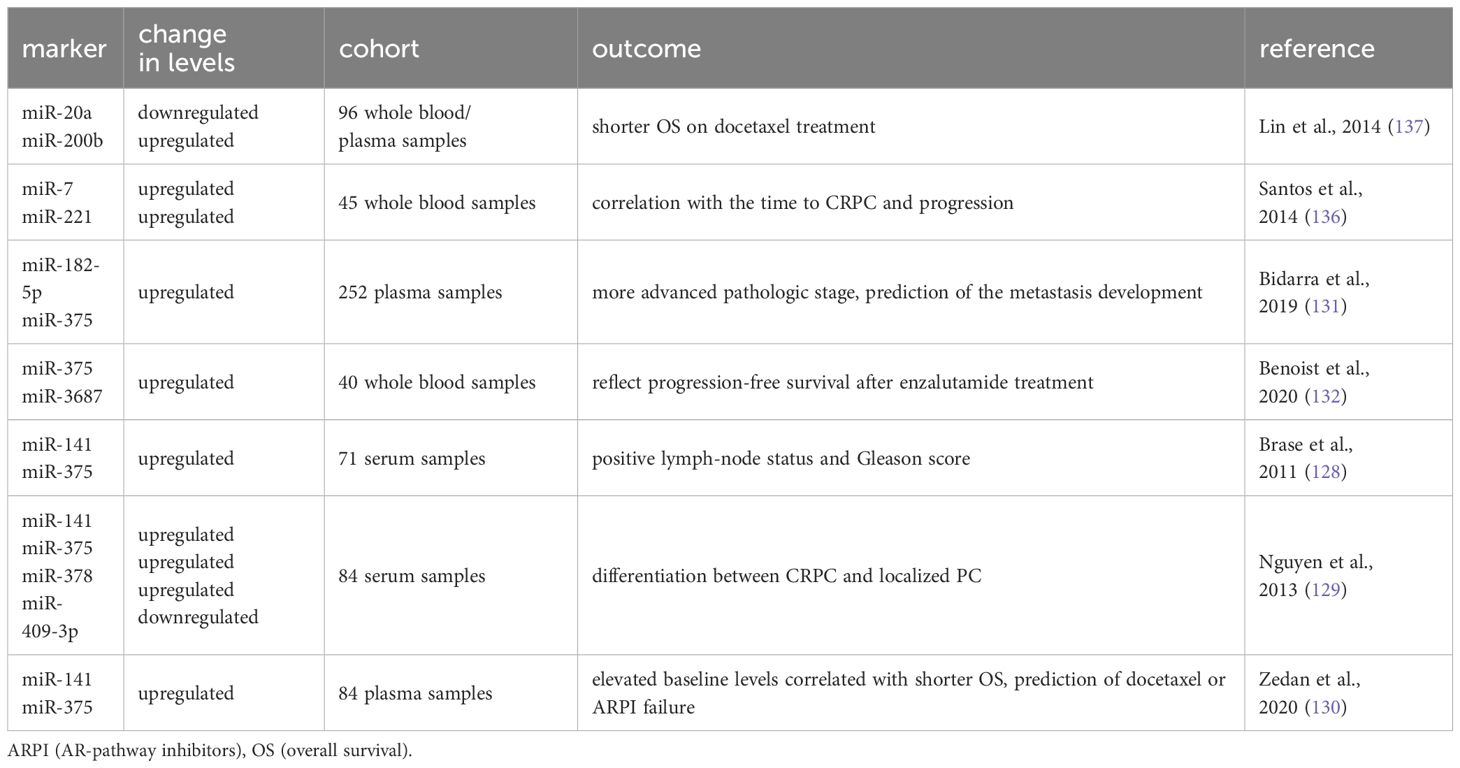
Table 5. The summary of miRNAs useful for prognostic stratification of CRPC patients analysed by quantitative polymerase chain reaction.
The role of lncRNAs in PC development and therapy resistance has been gradually unveiled (139–147). The prostate cancer antigen 3 (PCA3) is one of the most prostate-specific biomarkers routinely used in urine examination. Notably, PCA3 was also analyzed using a digital PCR approach in plasma LB samples from 201 PC patients. It was combined with other lncRNAs, S100A4 and MRC2, as a minimally invasive biomarker for screening and aggressiveness stratification of PC patients (148). Additionally, the lncRNA TUC338 was associated with shorter OS in a cohort of 52 PC patients (142). The H19 lncRNA encapsulated in EVs was significantly elevated in ARPI-resistant CRPC patients, however, the study was conducted on a small number of patients (only 6 ARPI-resistant CRPC patients) (149). In general, lncRNAs are frequently utilized as diagnostic biomarkers for PC when examining tissues (150). Despite the efforts of researchers in the field of lncRNAs, the implementation of circulating lncRNAs as LB markers for CRPC progression monitoring has yet to be realized.
7 Emerging role of circulating lipids in CRPC monitoring
Over the past decade, many studies have demonstrated the significance of lipid metabolism in PC development and progression (151, 152). We have shown that cholesterol metabolism is essential for the intratumoral production of androgens (153, 154), which is in line with clinical observation that medication with statins prolongs the time to progression in patients on ADT (153, 154). Furthermore, targeting sphingosine kinase, an enzyme involved in lipid metabolism, has emerged as a potential therapeutic approach to overcome enzalutamide resistance and improve patient treatment outcomes (155).
Several studies highlighted the usefulness of lipid detection in LB samples for prognostic purposes. A distinct plasma signature containing two sphingolipids (ceramide d18:1/24:1 and sphingomyelin d18:2/16:0) and glycerophospholipid phosphatidylcholine 16:0/16:0 was associated with poor prognosis and OS of mCRPC patients (156). The aberrations in circulating ceramide levels were associated with poor clinical outcomes in both localized and metastatic PC (157), prompting further studies to explore the combined effects of lipidomic and genetic aberrations on clinical outcomes in mCRPC. The elevated levels of the three-lipid signature and abnormalities in one or more genes (AR, TP53, RB1, or PI3K) were associated with worse prognoses in mCRPC patients (158). The same group has recently introduced a modified circulating lipid biomarker signature (PCPro), which contains ceramides (d18:1/18:0), (d18:1/24:0), and (d18:1/24:1), triglycerides, and total cholesterol. PCPro may be a clinically accessible panel of blood lipid markers capable of prospectively identifying men with mCRPC with a poor prognosis (159).
8 Conclusion
This article summarizes recent knowledge about liquid biopsy markers suitable for monitoring CRPC progression. Especially promising are AR alterations, miR-375, methylation markers, and emerging lipidomic analyses. These markers are discussed alongside standard clinical parameters, such as PSA, LDH, or CRP. Mutational and methylation analyses provide cancer-specific information from relatively stable ccfDNA; however, the methods may be laborious and expensive. Expression analysis may be hampered by mRNA degradation, but short miRNAs have repeatedly been shown to be reliable biomarkers. Lipidomics open new avenues in cancer monitoring thanks to fast mass spectrometry analysis. The standard clinical parameters should always serve as benchmarks in new biomarker studies.
Integrating genomic, transcriptomic, and epigenomic biomarkers in clinical practice can potentially revolutionize the management of CRPC. Among the various biomarkers studied, AR alterations, including gene amplifications, point mutations, and the AR enhancer region CNV, stand out as having significant prognostic and predictive value (70–78). Specifically, the AR enhancer CNV has been suggested as an excellent prognostic marker due to its strong association with progression in mCRPC and resistance to AR-directed therapy (76).
Additionally, ccfDNA methylation and ccfRNA markers, such as ARv7, have shown potential in providing prognostic information. When analyzed from non-invasive liquid biopsies, methylation markers could offer insights into tumor biology and patient prognosis (80, 81, 85, 86, 89–91, 94, 95, 103, 105). ARv7, a splice variant of the AR, has been linked to resistance to AR-targeted therapies, making it a promising predictive biomarker for therapy response (117–121). Early detection of treatment failure followed by the switch of therapy may significantly improve the patient´s prognosis.
Despite these advancements, it is crucial to standardize sample acquisition and validation methods across laboratories to ensure reproducibility and accuracy. Future research should focus on validating these biomarkers in large, independent cohorts and developing robust, clinically applicable assays. Adhering strictly to standardized sample collection procedures and employing robust methods are essential prerequisites for reproducing and facilitating the clinical use of LB markers. Their integration into routine clinical practice could improve prognostic stratification and personalized treatment strategies for patients with CRPC. After proper and multicentric validation, these promising biomarkers have great potential to enhance the care of metastatic prostate cancer patients.
Author contributions
EC: Conceptualization, Methodology, Resources, Visualization, Writing – original draft, Writing – review & editing. HŠ: Conceptualization, Writing – original draft. KH: Conceptualization, Writing – original draft. ZK: Visualization, Writing – review & editing. RH: Writing – review & editing. KS: Writing – review & editing. JB: Funding acquisition, Project administration, Resources, Supervision, Writing – review & editing.
Funding
The author(s) declare financial support was received for the research, authorship, and/or publication of this article. This work was supported by funds from the Czech Ministry of Health (grant NU20-03-00201 and DRO FNOl 00098892), by Palacky University (LF_2024_004 and LF_2024_010), by the European Regional Development Fund (BBMRI-CZ No. CZ.02.1.01/0.0/0.0/16_013/0001674), and by the project National Institute for Cancer Research (Programme EXCELES, ID Project No. LX22NPO5102) - Funded by the European Union - Next Generation EU. EC was supported by DSGC-2021-0143 (through the OP RDE project Reg. No. CZ.02.2.69/0.0/0.0/19_073/0016713).
Conflict of interest
The authors declare that the research was conducted in the absence of any commercial or financial relationships that could be construed as a potential conflict of interest.
The author(s) declared that they were an editorial board member of Frontiers, at the time of submission. This had no impact on the peer review process and the final decision.
Publisher’s note
All claims expressed in this article are solely those of the authors and do not necessarily represent those of their affiliated organizations, or those of the publisher, the editors and the reviewers. Any product that may be evaluated in this article, or claim that may be made by its manufacturer, is not guaranteed or endorsed by the publisher.
References
1. Siegel RL, Miller KD, Wagle NS, Jemal A. Cancer statistics, 2023. CA Cancer J Clin. (2023) 73:17–48. doi: 10.3322/caac.21763
2. Karantanos T, Corn PG, Thompson TC. Prostate cancer progression after androgen deprivation therapy: mechanisms of castrate resistance and novel therapeutic approaches. Oncogene. (2013) 32:5501–11. doi: 10.1038/onc.2013.206
3. Roviello G, Catalano M, Ottanelli C, Giorgione R, Rossi V, Gambale E, et al. Castration-resistant prostate cancer with bone metastases: toward the best therapeutic choice. Med Oncol. (2022) 39:145. doi: 10.1007/s12032-022-01739-3
4. Wang J, Ben-David R, Mehrazin R, Yang W, Tewari AK, Kyprianou N. Novel signatures of prostate cancer progression and therapeutic resistance. Expert Opin Ther Targets. (2023) 27:1195–206. doi: 10.1080/14728222.2023.2293757
5. Madueke I, Lee RJ, Miyamoto DT. Circulating tumor cells and circulating tumor DNA in urologic cancers. Urol Clin North Am. (2023) 50:109–14. doi: 10.1016/j.ucl.2022.09.010
6. Trujillo B, Wu A, Wetterskog D, Attard G. Blood-based liquid biopsies for prostate cancer: clinical opportunities and challenges. Br J Cancer. (2022) 127:1394–402. doi: 10.1038/s41416-022-01881-9
7. Tulpule V, Morrison GJ, Falcone M, Quinn DI, Goldkorn A. Integration of liquid biopsies in clinical management of metastatic prostate cancer. Curr Oncol Rep. (2022) 24:1287–98. doi: 10.1007/s11912-022-01278-0
8. Cieślikowski WA, Antczak A, Nowicki M, Zabel M, Budna-Tukan J. Clinical relevance of circulating tumor cells in prostate cancer management. Biomedicines. (2021) 9:1179. doi: 10.3390/biomedicines9091179
9. Kahounová Z, Pícková M, Drápela S, Bouchal J, Szczyrbová E, Navrátil J, et al. Circulating tumor cell-derived preclinical models: current status and future perspectives. Cell Death Dis. (2023) 14:530. doi: 10.1038/s41419-023-06059-6
10. Groen L, Schalken J. Liquid biopsy for prostate and bladder cancer: progress and pitfalls. Eur Urol Focus. (2022) 8:904–6. doi: 10.1016/j.euf.2022.08.013
11. Tang L, Li X, Wang B, Luo G, Gu L, Chen L, et al. Prognostic value of neutrophil-to-lymphocyte ratio in localized and advanced prostate cancer: A systematic review and meta-analysis. PloS One. (2016) 11:e0153981. doi: 10.1371/journal.pone.0153981
12. Guan Y, Xiong H, Feng Y, Liao G, Tong T, Pang J. Revealing the prognostic landscape of neutrophil-to-lymphocyte ratio and platelet-to-lymphocyte ratio in metastatic castration-resistant prostate cancer patients treated with abiraterone or enzalutamide: a meta-analysis. Prostate Cancer Prostatic Dis. (2020) 23:220–31. doi: 10.1038/s41391-020-0209-3
13. Zhou M, Liang J, Hui J, Xu J. Inflammation-related indicators have a potential to increase overall quality of the prostate cancer management: a narrative review. Transl Androl Urol. (2023) 12:809–22. doi: 10.21037/tau
14. Lolli C, Caffo O, Scarpi E, Aieta M, Conteduca V, Maines F, et al. Systemic immune-inflammation index predicts the clinical outcome in patients with mCRPC treated with abiraterone. Front Pharmacol. (2016) 13:7:376. doi: 10.3389/fphar.2016.00376
15. Koo KC, Lee JS, Ha JS, Han KS, Lee KS, Hah YS, et al. Optimal sequencing strategy using docetaxel and androgen receptor axis-targeted agents in patients with castration-resistant prostate cancer: utilization of neutrophil-to-lymphocyte ratio. World J Urol. (2019) 37:2375–84. doi: 10.1007/s00345-019-02658-1
16. Kerr BA, Harris KS, Shi L, Willey JS, Soto-Pantoja DR, Byzova TV. Platelet TSP-1 controls prostate cancer-induced osteoclast differentiation and bone marrow-derived cell mobilization through TGFβ-1. Am J Clin Exp Urol. (2021) 9:18–31.
17. Mantovani A, Allavena P, Sica A, Balkwill F. Cancer-related inflammation. Nature. (2008) 454:436–44. doi: 10.1038/nature07205
18. Qi W, Zhou Y, Liu Z, Wang J, Lv G, Zhong M, et al. Revealing the prognostic and clinicopathological significance of systemic immune-inflammation index in patients with diierent stage prostate cancer: A systematic review and meta-analysis. Front Med. (2022) 9:1–12. doi: 10.3389/fmed.2022.1052943
19. Sevcenco S, Mathieu R, Baltzer P, Klatte T, Fajkovic H, Seitz C, et al. The prognostic role of preoperative serum C-reactive protein in predicting the biochemical recurrence in patients treated with radical prostatectomy. Prostate Cancer Prostatic Dis. (2016) 19:163–7. doi: 10.1038/pcan.2015.60
20. Gómez-Gómez E, Carrasco-Valiente J, Campos-Hernández JP, Blanca-Pedregosa AM, Jiménez-Vacas JM, Ruiz-García J, et al. Clinical association of metabolic syndrome, C-reactive protein and testosterone levels with clinically significant prostate cancer. J Cell Mol Med. (2019) 23:934–42. doi: 10.1111/jcmm.13994
21. Xu L, Zhao Q, Huang S, Li S, Wang J, Li Q. Serum C-reactive protein acted as a prognostic biomarker for overall survival in metastatic prostate cancer patients. Tumor Biol. (2015) 36:669–73. doi: 10.1007/s13277-014-2670-x
22. Schnoeller TJ, Steinestel J, Steinestel K, Jentzmik F, Schrader AJ. Do preoperative serum C-reactive protein levels predict the definitive pathological stage in patients with clinically localized prostate cancer? Int Urol Nephrol. (2015) 47:765–70. doi: 10.1007/s11255-015-0952-x
23. Tulloch-Reid MK, McFarlane-Anderson N, Bennett FI, Aiken WD, Jackson MD. Effects of cholesterol, C-reactive protein, and interleukin-6 on prostate cancer risk in a population of African ancestry. Cancer Causes Control. (2017) 28:1313–21. doi: 10.1007/s10552-017-0945-4
24. Canat L, Atalay HA, Can O, Alkan İ, Ötünçtemur A. Serum procalcitonin levels in prostate cancer: A new biomarker? Urologia. (2018) 85:46–50. doi: 10.1177/0391560317752600
25. Ilktac A, Kalkan S, Caliskan S. C-reactive protein and procalcitonin levels in prostate cancer. Int J Clin Pract. (2021) 75:e13935. doi: 10.1111/ijcp.13935
26. Ando K, Sakamoto S, Saito S, Maimaiti M, Imamura Y, Sazuka T, et al. Prognostic value of high-sensitivity modified glasgow prognostic score in castration-resistant prostate cancer patients who received docetaxel. Cancers (Basel). (2021) 13:1–14. doi: 10.3390/cancers13040773
27. Bryce AH, Alumkal JJ, Armstrong A, Higano CS, Iversen P, Sternberg CN, et al. Radiographic progression with nonrising PSA in metastatic castration-resistant prostate cancer: Post hoc analysis of PREVAIL. Prostate Cancer Prostatic Dis. (2017) 20:221–7. doi: 10.1038/pcan.2016.71
28. Hamano I, Hatakeyama S, Narita S, Takahashi M, Sakurai T, Kawamura S, et al. Impact of nadir PSA level and time to nadir during initial androgen deprivation therapy on prognosis in patients with metastatic castration-resistant prostate cancer. World J Urol. (2019) 37:2365–73. doi: 10.1007/s00345-019-02664-3
29. Li D, Lv H, Hao X, Hu B, Song Y. Prognostic value of serum alkaline phosphatase in the survival of prostate cancer: Evidence from a meta-analysis. Cancer Manag Res. (2018) 10:3125–39. doi: 10.2147/CMAR
30. Forkasiewicz A, Dorociak M, Stach K, Szelachowski P, Tabola R, Augoff K. The usefulness of lactate dehydrogenase measurements in current oncological practice. Cell Mol Biol Lett. (2020) 9;25:35. doi: 10.1186/s11658-020-00228-7
31. Scher HI, Jia X, de Bono JS, Fleisher M, Pienta KJ, Raghavan D, et al. Circulating tumour cells as prognostic markers in progressive, castration-resistant prostate cancer: a reanalysis of IMMC38 trial data. Lancet Oncol. (2009) 10:233–9. doi: 10.1016/S1470-2045(08)70340-1
32. Galletti G, Portella L, Tagawa ST, Kirby BJ, Giannakakou P, Nanus DM. Circulating tumor cells in prostate cancer diagnosis and monitoring: an appraisal of clinical potential. Mol Diagn Ther. (2014) 18:389–402. doi: 10.1007/s40291-014-0101-8
33. Mitsui Y, Yamabe F, Hori S, Uetani M, Aoki H, Sakurabayashi K, et al. Combination of C-reactive protein/albumin ratio and time to castration resistance enhances prediction of prognosis for patients with metastatic castration-resistant prostate cancer. Front Oncol. (2023) 13:1162820. doi: 10.3389/fonc.2023.1162820
34. Hakozaki Y, Yamada Y, Takeshima Y, Taguchi S, Kawai T, Nakamura M, et al. Low hemoglobin and PSA kinetics are prognostic factors of overall survival in metastatic castration-resistant prostate cancer patients. Sci Rep. (2023) 13:2672. doi: 10.1038/s41598-023-29634-5
35. Wibmer AG, Morris MJ, Gonen M, Zheng J, Hricak H, Larson S, et al. Quantification of metastatic prostate cancer whole-body tumor burden with 18F-FDG PET parameters and associations with overall survival after first-line abiraterone or enzalutamide: A single-center retrospective cohort study. J Nucl Med. (2021) 62:1050–6. doi: 10.2967/jnumed.120.256602
36. Merkens L, Sailer V, Lessel D, Janzen E, Greimeier S, Kirfel J, et al. Aggressive variants of prostate cancer: underlying mechanisms of neuroendocrine transdifferentiation. J Exp Clin Cancer Res. (2022) 41:46. doi: 10.1186/s13046-022-02255-y
37. Huang YH, Zhang YQ, Huang JT. Neuroendocrine cells of prostate cancer: biologic functions and molecular mechanisms. Asian J Androl. (2019) 21:291–5. doi: 10.4103/aja.aja_128_18
38. Pernicová Z, Slabáková E, Fedr R, Šimečková Š, Jaroš J, Suchánková T, et al. The role of high cell density in the promotion of neuroendocrine transdifferentiation of prostate cancer cells. Mol Cancer. (2014) 13:113. doi: 10.1186/1476-4598-13-113
39. Pernicová Z, Slabáková E, Kharaishvili G, Bouchal J, Král M, Kunická Z, et al. Androgen depletion induces senescence in prostate cancer cells through down-regulation of Skp2. Neoplasia. (2011) 13:526–36. doi: 10.1593/neo.11182
40. Mu P, Zhang Z, Benelli M, Karthaus WR, Hoover E, Chen CC, et al. SOX2 promotes lineage plasticity and antiandrogen resistance in TP53- and RB1-deficient prostate cancer. Science. (2017) 355:84–8. doi: 10.1126/science.aah4307
41. Ku SY, Rosario S, Wang Y, Mu P, Seshadri M, Goodrich ZW, et al. Rb1 and Trp53 cooperate to suppress prostate cancer lineage plasticity, metastasis, and antiandrogen resistance. Science. (2017) 355:78–83. doi: 10.1126/science.aah4199
42. Sreekumar A, Saini S. Role of transcription factors and chromatin modifiers in driving lineage reprogramming in treatment-induced neuroendocrine prostate cancer. Front Cell Dev Biol. (2023) 11:1075707. doi: 10.3389/fcell.2023.1075707
43. Conteduca V, Burgio SL, Menna C, Carretta E, Rossi L, Bianchi E, et al. Chromogranin A is a potential prognostic marker in prostate cancer patients treated with enzalutamide. Prostate. (2014) 74:1691–6. doi: 10.1002/pros.v74.16
44. Bray AW, Duan R, Malalur P, Drusbosky LM, Gourdin TS, Hill EG, et al. Elevated serum CEA is associated with liver metastasis and distinctive circulating tumor DNA alterations in patients with castration-resistant prostate cancer. Prostate. (2022) 82:1264–72. doi: 10.1002/pros.24400
45. Aparicio AM, Harzstark AL, Corn PG, Wen S, Araujo JC, Tu SM, et al. Platinum-based chemotherapy for variant castrate-resistant prostate cancer. Clin Cancer Res. (2013) 19:3621–30. doi: 10.1158/1078-0432.CCR-12-3791
46. Bardelli A, Pantel K. Liquid biopsies, what we do not know (Yet). Cancer Cell Cell Press;. (2017) 31:172–9. doi: 10.1016/j.ccell.2017.01.002
47. Mouliere F, Rosenfeld N. Circulating tumor-derived DNA is shorter than somatic DNA in plasma. Proc Natl Acad Sci U S A. (2015) 112:3178–9. doi: 10.1073/pnas.1501321112
48. Wan JCM, Massie C, Garcia-Corbacho J, Mouliere F, Brenton JD, Caldas C, et al. Liquid biopsies come of age: Towards implementation of circulating tumour DNA. Nat Rev Cancer. (2017) 17:223–38. doi: 10.1038/nrc.2017.7
49. Diehl F, Schmidt K, Choti MA, Romans K, Goodman S, Li M, et al. Circulating mutant DNA to assess tumor dynamics. Nat Med. (2008) 14:985–90. doi: 10.1038/nm.1789
50. Dawson SJ, Tsui DWY, Murtaza M, Biggs H, Rueda OM, Chin SF, et al. Analysis of circulating tumor DNA to monitor metastatic breast cancer. N Engl J Med. (2013) 368:1199–209. doi: 10.1056/NEJMoa1213261
51. Zainfeld D, Goldkorn A. Liquid biopsy in prostate cancer: circulating tumor cells and beyond. Cancer Treat Res. (2018) 175:87–104. doi: 10.1007/978-3-319-93339-9_4
52. Torquato S, Pallavajjala A, Goldstein A, Toro PV, Silberstein JL, Lee J, et al. Genetic alterations detected in cell-free DNA are associated with enzalutamide and abiraterone resistance in castration-resistant prostate cancer. JCO Precis Oncol. (2019) 3:PO.18.00227. doi: 10.1200/PO.18.00227
53. Liu H, Gao Y, Vafaei S, Gu X, Zhong X. The prognostic value of plasma cell-free DNA concentration in the prostate cancer: A systematic review and meta-analysis. Front Oncol. (2021) 11:599602. doi: 10.3389/fonc.2021.599602
54. Reichert ZR, Morgan TM, Li G, Castellanos E, Snow T, Dall’Olio FG, et al. Prognostic value of plasma circulating tumor DNA fraction across four common cancer types: a real-world outcomes study. Ann Oncol. (2023) 34:111–20. doi: 10.1016/j.annonc.2022.09.163
55. Aghamir SMK, Rahimnia A, Zadeh SST, Roudgari H. Circulating tumor cells and DNAs in prostate tumors. In: Liquid biopsy in urogenital cancers and its clinical utility. Amsterdam, Netherlands: Elsevier (2022). p. 67–99.
56. Husain H, Pavlick DC, Fendler BJ, Madison RW, Decker B, Gjoerup O, et al. Tumor fraction correlates with detection of actionable variants across > 23,000 circulating tumor DNA samples. JCO Precis Oncol. (2022) 6:2200261. doi: 10.1200/PO.22.00261
57. Patel KM, Tsui DWY. The translational potential of circulating tumour DNA in oncology. Clin Biochem. (2015) 48:957–61. doi: 10.1016/j.clinbiochem.2015.04.005
58. Bettegowda C, Sausen M, Leary RJ, Kinde I, Wang Y, Agrawal N, et al. Detection of circulating tumor DNA in early- and late-stage human Malignancies. Sci Transl Med. (2014) 6:224ra24. doi: 10.1093/neuonc/nou206.24
59. Alix-Panabières C, Pantel K. Clinical applications of circulating tumor cells and circulating tumor DNA as liquid biopsy. Cancer Discovery. (2016) 6:479–91. doi: 10.1158/2159-8290.CD-15-1483
60. Diaz LA, Bardelli A. Liquid biopsies: Genotyping circulating tumor DNA. J Clin Oncol. (2014) 32:579–86. doi: 10.1200/JCO.2012.45.2011
61. Mostaghel EA, Plymate SR, Montgomery B. Molecular pathways: Targeting resistance in the androgen receptor for therapeutic benefit. Clin Cancer Res. (2014) 20:791–8. doi: 10.1158/1078-0432.CCR-12-3601
62. Tan ME, Li J, Xu HE, Melcher K, Yong EL. Androgen receptor: Structure, role in prostate cancer and drug discovery. Acta Pharmacol Sin. (2015) 36:3–23. doi: 10.1038/aps.2014.18
63. Shiota M, Akamatsu S, Tsukahara S, Nagakawa S, Matsumoto T, Eto M. Androgen receptor mutations for precision medicine in prostate cancer. Endocr Relat Cancer. (2022) 29:R143–155. doi: 10.1530/ERC-22-0140
64. Watson PA, Arora VK, Sawyers CL. Emerging mechanisms of resistance to androgen receptor inhibitors in prostate cancer. Nat Rev Cancer. (2015) 15:701–11. doi: 10.1038/nrc4016
65. Kwan EM, Wyatt AW. Androgen receptor genomic alterations and treatment resistance in metastatic prostate cancer. Prostate. (2022) 82:S25–36. doi: 10.1002/pros.24356
66. Henzler C, Li Y, Yang R, McBride T, Ho Y, Sprenger C, et al. Truncation and constitutive activation of the androgen receptor by diverse genomic rearrangements in prostate cancer. Nat Commun. (2016) 7:13668. doi: 10.1038/ncomms13668
67. Armenia J, Wankowicz SAM, Liu D, Gao J, Kundra R, Reznik E, et al. The long tail of oncogenic drivers in prostate cancer. Nat Genet. (2018) 50:645–51. doi: 10.1038/s41588-018-0078-z
68. Robinson D, van Allen EM, Wu YM, Schultz N, Lonigro RJ, Mosquera JM, et al. Integrative clinical genomics of advanced prostate cancer. Cell. (2015) 161:1215–28. doi: 10.1016/j.cell.2015.05.001
69. Quigley DA, Dang HX, Zhao SG, Lloyd P, Aggarwal R, Alumkal JJ, et al. Genomic hallmarks and structural variation in metastatic prostate cancer. Cell. (2018) 174:758–69. doi: 10.1016/j.cell.2018.06.039
70. Romanel A, Tandefelt DG, Conteduca V, Jayaram A, Casiraghi N, Wetterskog D, et al. Plasma AR and abiraterone-resistant prostate cancer. Sci Transl Med. (2015) 7:312re10. doi: 10.1126/scitranslmed.aac9511
71. Conteduca V, Wetterskog D, Sharabiani MTA, Grande E, Fernandez-Perez MP, Jayaram A, et al. Androgen receptor gene status in plasma DNA associates with worse outcome on enzalutamide or abiraterone for castration-resistant prostate cancer: A multi-institution correlative biomarker study. Ann Oncol. (2017) 28:1508–16. doi: 10.1093/annonc/mdx155
72. Kohli M, Li J, Du M, Hillman DW, Dehm SM, Tan W, et al. Prognostic association of plasma cell-free DNA-based androgen receptor amplification and circulating tumor cells in pre-chemotherapy metastatic castration-resistant prostate cancer patients. Prostate Cancer Prostatic Dis. (2018) 21:411–8. doi: 10.1038/s41391-018-0043-z
73. Azad AA, Volik SV, Wyatt AW, Haegert A, Le Bihan S, Bell RH, et al. Androgen receptor gene aberrations in circulating cell-free DNA: Biomarkers of therapeutic resistance in castration-resistant prostate cancer. Clin Cancer Res. (2015) 21:2315–24. doi: 10.1158/1078-0432.CCR-14-2666
74. Du M, Huang CC, Tan W, Kohli M, Wang L. Multiplex digital pcr to detect amplifications of specific androgen receptor loci in cell-free DNA for prognosis of metastatic castration-resistant prostate cancer. Cancers. (2020) 12:1–13. doi: 10.3390/cancers12082139
75. Takeda DY, Spisák S, Seo JH, Bell C, O’Connor E, Korthauer K, et al. A somatically acquired enhancer of the androgen receptor is a noncoding driver in advanced prostate cancer. Cell. (2018) 174:422–32. doi: 10.1016/j.cell.2018.05.037
76. Dang HX, Chauhan PS, Ellis H, Feng W, Harris PK, Smith G, et al. Cell-free DNA alterations in the AR enhancer and locus predict resistance to AR-directed therapy in patients with metastatic prostate cancer. JCO Precis Oncol. (2020) 4:680–713. doi: 10.1200/PO.20.00047
77. Beltran H, Yelensky R, Frampton GM, Park K, Downing SR, MacDonald TY, et al. Targeted next-generation sequencing of advanced prostate cancer identifies potential therapeutic targets and disease heterogeneity. Eur Urol. (2013) 63:920–6. doi: 10.1016/j.eururo.2012.08.053
78. Sumiyoshi T, Mizuno K, Yamasaki T, Miyazaki Y, Makino Y, Okasho K, et al. Clinical utility of androgen receptor gene aberrations in circulating cell-free DNA as a biomarker for treatment of castration-resistant prostate cancer. Sci Rep. (2019) 9:4030. doi: 10.1038/s41598-019-40719-y
79. Morova T, McNeill DR, Lallous N, Gönen M, Dalal K, Wilson DM, et al. Androgen receptor-binding sites are highly mutated in prostate cancer. Nat Commun. (2020) 11:832. doi: 10.1038/s41467-020-14644-y
80. Silva R, Moran B, Russell NM, Fahey C, Vlajnic T, Manecksha RP, et al. Evaluating liquid biopsies for methylomic profiling of prostate cancer. Epigenetics. (2020) 15:715–27. doi: 10.1080/15592294.2020.1712876
81. Rouprêt M, Hupertan V, Catto JWF, Yates DR, Rehman I, Proctor LM, et al. Promoter hypermethylation in circulating blood cells identifies prostate cancer progression. Int J Cancer. (2008) 122:952–6. doi: 10.1002/ijc.23196
82. Martignano F, Gurioli G, Salvi S, Calistri D, Costantini M, Gunelli R, et al. GSTP1 methylation and protein expression in prostate cancer: diagnostic implications. Dis Markers. (2016) 2016:4358292. doi: 10.1155/2016/4358292
83. Dubois F, Bergot E, Zalcman G, Levallet G. RASSF1A, puppeteer of cellular homeostasis, fights tumorigenesis, and metastasis-an updated review. Cell Death Dis. (2019) 10:928. doi: 10.1038/s41419-019-2169-x
84. Shin HJ, Hua JT, Li H. Recent advances in understanding DNA methylation of prostate cancer. Front Oncol. (2023) 13:1182727. doi: 10.3389/fonc.2023.1182727
85. Hendriks RJ, Dijkstra S, Smit FP, Vandersmissen J, Van de Voorde H, Mulders PFA, et al. Epigenetic markers in circulating cell-free DNA as prognostic markers for survival of castration-resistant prostate cancer patients. Prostate. (2018) 78:336–42. doi: 10.1002/pros.23477
86. Okegawa T, Nutahara K, Higashihara E. Association of circulating tumor cells with tumor-related methylated DNA in patients with hormone-refractory prostate cancer. Int J Urol. (2010) 17:466–75. doi: 10.1111/j.1442-2042.2010.02502.x
87. Yegnasubramanian S, Kowalski J, Gonzalgo ML, Zahurak M, Piantadosi S, Walsh PC, et al. Hypermethylation of CpG islands in primary and metastatic human prostate cancer. Cancer Res. (2004) 64:1975–86. doi: 10.1158/0008-5472.CAN-03-3972
88. Enokida H, Shiina H, Igawa M, Ogishima T, Kawakami T, Bassett WW, et al. CpG hypermethylation of MDR1 gene contributes to the pathogenesis and progression of human prostate cancer. Cancer Res. (2004) 64:5956–62. doi: 10.1158/0008-5472.CAN-04-0081
89. Wang L, Lin YL, Li B, Wang YZ, Li WP, Ma JG. Aberrant promoter methylation of the cadherin 13 gene in serum and its relationship with clinicopathological features of prostate cancer. J Int Med Res. (2014) 42:1085–92. doi: 10.1177/0300060514540631
90. Mahon KL, Qu W, Lin HM, Spielman C, Cain D, Jacobs C, et al. Serum free methylated glutathione S-transferase 1 DNA levels, survival, and response to docetaxel in metastatic, castration-resistant prostate cancer: post hoc analyses of data from a phase 3 trial. Eur Urol. (2019) 76:306–12. doi: 10.1016/j.eururo.2018.11.001
91. Dillinger T, Sheibani-Tezerji R, Pulverer W, Stelzer I, Hassler MR, Scheibelreiter J, et al. Identification of tumor tissue-derived DNA methylation biomarkers for the detection and therapy response evaluation of metastatic castration resistant prostate cancer in liquid biopsies. Mol Cancer. (2022) 21:7. doi: 10.1186/s12943-021-01445-0
92. He HJ, Gu XF, Xu WH, Yang DJ, Wang XM, Su Y. Krüppel-like factor 8 is a novel androgen receptor co-activator in human prostate cancer. Acta Pharmacol Sin. (2013) 34:282–8. doi: 10.1038/aps.2012.130
93. Currall BB, Chen M, Sallari RC, Cotter M, Wong KE, Robertson NG, et al. Loss of LDAH associated with prostate cancer and hearing loss. Hum Mol Genet. (2018) 27:4194–203. doi: 10.1093/hmg/ddy310
94. Bjerre MT, Nørgaard M, Larsen OH, Jensen SØ, Strand SH, Østergren P, et al. Epigenetic analysis of circulating tumor DNA in localized and metastatic prostate cancer: evaluation of clinical biomarker potential. Cells. (2020) 9:1362. doi: 10.3390/cells9061362
95. Sjostrom M, Zhao SG, Levy S, Zhang M, Ning Y, Shrestha R, et al. The 5-hydroxymethylcytosine landscape of prostate cancer. Cancer Res. (2022) 82:3888–902. doi: 10.1158/0008-5472.CAN-22-1123
96. Xin L. EZH2 accompanies prostate cancer progression. Nat Cell Biol. (2021) 23:934–6. doi: 10.1038/s41556-021-00744-4
97. de Resende MF, Vieira S, Chinen LT, Chiappelli F, da Fonseca FP, Guimarães GC, et al. Prognostication of prostate cancer based on TOP2A protein and gene assessment: TOP2A in prostate cancer. J Transl Med. (2013) 11:36. doi: 10.1186/1479-5876-11-36
98. Pommier Y, Nussenzweig A, Takeda S, Austin C. Human topoisomerases and their roles in genome stability and organization. Nat Rev Mol Cell Biol. (2022) 23:407–27. doi: 10.1038/s41580-022-00452-3
99. Schaefer-Klein JL, Murphy SJ, Johnson SH, Vasmatzis G, Kovtun IV. Topoisomerase 2 alpha cooperates with androgen receptor to contribute to prostate cancer progression. PloS One. (2015) 10:e0142327. doi: 10.1371/journal.pone.0142327
100. van der Vlag J, Otte AP. Transcriptional repression mediated by the human polycomb-group protein EED involves histone deacetylation. Nat Genet. (1999) 23:474–8. doi: 10.1038/70602
101. Labbé DP, Sweeney CJ, Brown M, Galbo P, Rosario S, Wadosky KM, et al. TOP2A and EZH2 provide early detection of an aggressive prostate cancer subgroup. Clin Cancer Res. (2017) 23:7072–83. doi: 10.1158/1078-0432.CCR-17-0413
102. Hansen AF, Høiem TS, Selnaes KM, Bofin AM, Størkersen Ø, Bertilsson H, et al. Prediction of recurrence from metabolites and expression of TOP2A and EZH2 in prostate cancer patients treated with radiotherapy. NMR Biomed. (2023) 36:e4694. doi: 10.1002/nbm.4694
103. Wu A, Cremaschi P, Wetterskog D, Conteduca V, Franceschini GM, Kleftogiannis D, et al. Genome-wide plasma DNA methylation features of metastatic prostate cancer. J Clin Invest. (2020) 130:1991–2000. doi: 10.1172/JCI130887
104. Ke XS, Qu Y, Rostad K, Li WC, Lin B, Halvorsen OJ, et al. Genome-wide profiling of histone h3 lysine 4 and lysine 27 trimethylation reveals an epigenetic signature in prostate carcinogenesis. PloS One. (2009) 4:e4687. doi: 10.1371/journal.pone.0004687
105. Deligezer U, Yaman F, Darendeliler E, Dizdar Y, Holdenrieder S, Kovancilar M, et al. Post-treatment circulating plasma BMP6 mRNA and H3K27 methylation levels discriminate metastatic prostate cancer from localized disease. Clin Chim Acta. (2010) 411:1452–6. doi: 10.1016/j.cca.2010.05.040
106. Ehsani M, David FO, Baniahmad A. Androgen receptor-dependent mechanisms mediating drug resistance in prostate cancer. Cancers (Basel). (2021) 13:1534. doi: 10.3390/cancers13071534
107. Paliouras M, Alvarado C, Trifiro M. Redefining androgen receptor function: clinical implications in understanding prostate cancer progression and therapeutic resistance. Prostate Cancer - Leading-edge Diagn Procedures Treatments. InTech. (2016) 93–103. doi: 10.5772/64392
108. Lu C, Luo J. Decoding the androgen receptor splice variants. Transl Androl Urol. (2013) 2:178–86. doi: 10.3978/j.issn.2223-4683.2013.09.08
109. Scher HI, Graf RP, Schreiber NA, McLaughlin B, Lu D, Louw J, et al. Nuclear-specific AR-V7 protein localization is necessary to guide treatment selection in metastatic castration-resistant prostate cancer. Eur Urol. (2017) 71:874–82. doi: 10.1016/j.eururo.2016.11.024
110. Hu R, Dunn TA, Wei S, Isharwal S, Veltri RW, Humphreys E, et al. Ligand-independent androgen receptor variants derived from splicing of cryptic exons signify hormone-refractory prostate cancer. Cancer Res. (2009) 69:16–22. doi: 10.1158/0008-5472.CAN-08-2764
111. Guo Z, Yang X, Sun F, Jiang R, Linn DE, Chen H, et al. A novel androgen receptor splice variant is up-regulated during prostate cancer progression and promotes androgen depletion-resistant growth. Cancer Res. (2009) 69:2305–13. doi: 10.1158/0008-5472.CAN-08-3795
112. Tagawa ST, Antonarakis ES, Gjyrezi A, Galletti G, Kim S, Worroll D, et al. Expression of AR-V7 and ARV 567Es in circulating tumor cells correlates with outcomes to taxane therapy in men with metastatic prostate cancer treated in taxynergy. Clin Cancer Res. (2019) 25:1880–8. doi: 10.1158/1078-0432.CCR-18-0320
113. Erb HHH, Sparwasser P, Diehl T, Hemmerlein-Thomas M, Tsaur I, Jüngel E, et al. AR-V7 protein expression in circulating tumour cells is not predictive of treatment response in mCRPC. Urol Int. (2020) 104:253–62. doi: 10.1159/000504416
114. Ma Y, Luk A, Young FP, Lynch D, Chua W, Balakrishnar B, et al. Droplet digital PCR based androgen receptor variant 7 (AR-V7) detection from prostate cancer patient blood biopsies. Int J Mol Sci. (2016) 17:1264. doi: 10.3390/ijms17081264
115. Antonarakis ES, Lu C, Luber B, Wang H, Chen Y, Zhu Y, et al. Clinical significance of androgen receptor splice variant-7 mRNA detection in circulating tumor cells of men with metastatic castration-resistant prostate cancer treated with first-and second-line abiraterone and enzalutamide. J Clin Oncol. (2017) 35:2149–56. doi: 10.1200/JCO.2016.70.1961
116. Antonarakis ES, Lu C, Wang H, Luber B, Nakazawa M, Roeser JC, et al. AR-V7 and resistance to enzalutamide and abiraterone in prostate cancer. N Engl J Med. (2014) 371:1028–38. doi: 10.1056/NEJMoa1315815
117. Zhou J, Liu R. The association between androgen receptor splice variant 7 status and prognosis of metastatic castration-resistant prostate cancer: A systematic review and meta-analysis. Andrologia. (2020) 52:00:e13642. doi: 10.1111/and.13642
118. Wang J, Zhang Y, Wei C, Gao X, Yuan P, Gan J, et al. Prognostic value of androgen receptor splice variant 7 in the treatment of metastatic castration-resistant prostate cancer: A systematic review and meta-analysis. Front Oncol. (2020) 10:562504. doi: 10.3389/fonc.2020.562504
119. Khan T, Khan T, Becker TM, Scott KF, Descallar J, de Souza P, et al. Prognostic and predictive value of liquid biopsy-derived androgen receptor variant 7 (AR-V7) in prostate cancer: A systematic review and meta-analysis. Front Oncol. (2022) 12:868031. doi: 10.3389/fonc.2022.868031
120. Qu F, Xie W, Nakabayashi M, Zhang H, Jeong SH, Wang X, et al. Association of AR-V7 and prostate-specific antigen RNA levels in blood with efficacy of abiraterone acetate and enzalutamide treatment in men with prostate cancer. Clin Cancer Res. (2017) 23:726–34. doi: 10.1158/1078-0432.CCR-16-1070
121. Del Re M, Crucitta S, Sbrana A, Rofi E, Paolieri F, Gianfilippo G, et al. Androgen receptor (AR) splice variant 7 and full-length AR expression is associated with clinical outcome: a translational study in patients with castrate-resistant prostate cancer. BJU Int. (2019) 124:693–700. doi: 10.1111/bju.14792
122. Stuopelyte K, Sabaliauskaite R, Bakavicius A, Haflidadóttir BS, Visakorpi T, Väänänen RM, et al. Analysis of AR-FL and AR-V1 in whole blood of patients with castration resistant prostate cancer as a tool for predicting response to abiraterone acetate. J Urol. (2020) 204:71–8. doi: 10.1097/JU.0000000000000803
123. Mitchell PS, Parkin RK, Kroh EM, Fritz BR, Wyman SK, Pogosova-Agadjanyan EL, et al. Circulating microRNAs as stable blood-based markers for cancer detection. Proc Natl Acad Sci USA. (2008) 105:10513–8. doi: 10.1073/pnas.0804549105
124. Cava C, Bertoli G, Colaprico A, Bontempi G, Mauri G, Castiglioni I. In-silico integration approach to identify a key miRNA regulating a gene network in aggressive prostate cancer. Int J Mol Sci. (2018) 19:910. doi: 10.3390/ijms19030910
125. Zhang W, Zang J, Jing X, Sun Z, Yan W, Yang D, et al. Identification of candidate miRNA biomarkers from miRNA regulatory network with application to prostate cancer. J Transl Med. (2014) 12:66. doi: 10.1186/1479-5876-12-66
126. Slabáková E, Kahounová Z, Procházková J, Souček K. Regulation of neuroendocrine-like differentiation in prostate cancer by non-coding rnas. Noncoding RNA. (2021) 7:75. doi: 10.3390/ncrna7040075
127. Gujrati H, Ha S, Wang BD. Deregulated microRNAs involved in prostate cancer aggressiveness and treatment resistance mechanisms. Cancers (Basel). (2023) 15:3140. doi: 10.3390/cancers15123140
128. Brase JC, Johannes M, Schlomm T, Haese A, Steuber T, Beissbarth T, et al. Circulating miRNAs are correlated with tumor progression in prostate cancer. Int J Cancer. (2011) 128:608–16. doi: 10.1002/ijc.25376
129. Nguyen HCN, Xie W, Yang M, Hsieh CL, Drouin S, Lee GSM, et al. Expression differences of circulating microRNAs in metastatic castration resistant prostate cancer and low-risk, localized prostate cancer. Prostate. (2013) 73:346–54. doi: 10.1002/pros.22572
130. Zedan AH, Osther PJS, Assenholt J, Madsen JS, Hansen TF. Circulating miR-141 and miR-375 are associated with treatment outcome in metastatic castration resistant prostate cancer. Sci Rep. (2020) 10:227. doi: 10.1038/s41598-019-57101-7
131. Bidarra D, Constâncio V, Barros-Silva D, Ramalho-Carvalho J, Moreira-Barbosa C, Antunes L, et al. Circulating microRNAs as biomarkers for prostate cancer detection and metastasis development prediction. Front Oncol. (2019) 9:900. doi: 10.3389/fonc.2019.00900
132. Benoist GE, van Oort IM, Boerrigter E, Verhaegh GW, van Hooij O, Groen L, et al. Prognostic value of novel liquid biomarkers in patients with metastatic castration-resistant prostate cancer treated with enzalutamide: A prospective observational study. Clin Chem. (2020) 66:842–51. doi: 10.1093/clinchem/hvaa095
133. Al-Muhtaresh HA, Al-Kafaji G. Evaluation of Two-Diabetes Related microRNAs Suitability as Earlier Blood Biomarkers for Detecting Prediabetes and type 2 Diabetes Mellitus. J Clin Med. (2018) 7:12. doi: 10.3390/jcm7020012
134. Dandare A, Khan MJ, Naeem A, Liaquat A. Clinical relevance of circulating non-coding RNAs in metabolic diseases: Emphasis on obesity, diabetes, cardiovascular diseases and metabolic syndrome. Genes Dis. (2022) 10:2393–413. doi: 10.1016/j.gendis.2022.05.022
135. Heianza Y, Krohn K, Yaskolka Meir A, Wang X, Ziesche S, Ceglarek U, et al. Changes in circulating miR-375-3p and improvements in visceral and hepatic fat contents in response to lifestyle interventions: the CENTRAL trial. Diabetes Care. (2022) 45:1911–3. doi: 10.2337/dc21-2517
136. Santos JI, Teixeira AL, Dias F, Maurício J, Lobo F, Morais A, et al. Influence of peripheral whole-blood microRNA-7 and microRNA-221 high expression levels on the acquisition of castration-resistant prostate cancer: Evidences from in vitro and in vivo studies. Tumor Biol. (2014) 35:7105–13. doi: 10.1007/s13277-014-1918-9
137. Lin HM, Castillo L, Mahon KL, Chiam K, Lee BY, Nguyen Q, et al. Circulating microRNAs are associated with docetaxel chemotherapy outcome in castration-resistant prostate cancer. Br J Cancer. (2014) 110:2462–71. doi: 10.1038/bjc.2014.181
138. Watahiki Y, Macfarlane RJ, Gleave ME, Crea F, Wang Y, Helgason CD, et al. Plasma miRNAs as biomarkers to identify patients with castration-resistant metastatic prostate cancer. Int J Mol Sci. (2013) 14:7757–70. doi: 10.3390/ijms14047757
139. Groen L, Yurevych V, Ramu H, Chen J, Steenge L, Boer S, et al. The androgen regulated lncRNA NAALADL2-AS2 promotes tumor cell survival in prostate cancer. Noncoding RNA. (2022) 8:81. doi: 10.3390/ncrna8060081
140. Zhang B, Zhang M, Shen C, Liu G, Zhang F, Hou J, et al. LncRNA PCBP1-AS1-mediated AR/AR-V7 deubiquitination enhances prostate cancer enzalutamide resistance. Cell Death Dis. (2021) 12:856. doi: 10.1038/s41419-021-04144-2
141. Zhang G, Luo Y. An immune-related lncrna signature to predict the biochemical recurrence and immune landscape in prostate cancer. Int J Gen Med. (2021) 14:9031–49. doi: 10.2147/IJGM.S336757
142. Li G, Zhang Y, Mao J, Hu P, Chen Q, Ding W, et al. LncRNA TUC338 is overexpressed in prostate carcinoma and downregulates miR-466. Gene. (2019) 707:224–30. doi: 10.1016/j.gene.2019.05.026
143. Chandra Gupta S, Nandan Tripathi Y. Potential of long non-coding RNAs in cancer patients: From biomarkers to therapeutic targets. Int J Cancer. (2017) 140:955–67. doi: 10.1002/ijc.30546
144. Anil P, Ghosh Dastidar S, Banerjee S. Unravelling the role of long non-coding RNAs in prostate carcinoma. Adv Cancer Biol - Metastasis. (2022) 6:100067. doi: 10.1016/j.adcanc.2022.100067
145. Huang H, Tang Y, Ye X, Chen W, Xie H, Chen S. The influence of lncRNAs on the prognosis of prostate cancer based on TCGA database. Transl Androl Urol. (2021) 10:1302–13. doi: 10.21037/tau
146. An C, Wang I, Li X, Xia R, Deng F. Long non-coding RNA in prostate cancer. Am J Clin Exp Urol. (2022) 10:170–9.
147. Song S, Zhu Y, Zhang X, Chen S, Liu S. Prognostic values of long noncoding RNA in bone metastasis of prostate cancer: A systematic review and meta-analysis. Front Oncol. (2023) 13:1085464. doi: 10.3389/fonc.2023.1085464
148. Alvarez-Cubero MJ, Arance E, de Santiago E, Sanchez P, Sepúlveda MR, Marrero R, et al. Follow-up biomarkers in the evolution of prostate cancer, levels of S100A4 as a detector in plasma. Int J Mol Sci. (2023) 24:547. doi: 10.3390/ijms24010547
149. Kato T, Kawakami K, Mizutani K, Ando T, Sakai Y, Sakurai K, et al. H19 in serum extracellular vesicles reflects resistance to AR axis-targeted therapy among CRPC patients. Cancer Genomics Proteomics. (2023) 20:456–68. doi: 10.21873/cgp.20397
150. Misawa A, Takayama KI, Inoue S. Long non-coding RNAs and prostate cancer. Cancer Sci. (2017) 108:2107–14. doi: 10.1111/cas.13352
151. Scaglia N, Frontini-López YR, Zadra G. Prostate cancer progression: as a matter of fats. Front Oncol. (2021) 11:719865. doi: 10.3389/fonc.2021.719865
152. Beier AMK, Puhr M, Stope MB, Thomas C, Erb HHH. Metabolic changes during prostate cancer development and progression. J Cancer Res Clin Oncol. (2023) 149:2259–70. doi: 10.1007/s00432-022-04371-w
153. Harshman LC, Wang X, Nakabayashi M, Xie W, Valenca L, Werner L, et al. Statin use at the time of initiation of androgen deprivation therapy and time to progression in patients with hormone-sensitive prostate cancer. JAMA Oncol. (2015) 1:495–504. doi: 10.1001/jamaoncol.2015.0829
154. Neuwirt H, Bouchal J, Kharaishvili G, Ploner C, Jöhrer K, Pitterl F, et al. Cancer-associated fibroblasts promote prostate tumor growth and progression through upregulation of cholesterol and steroid biosynthesis. Cell Communication Signaling. (2020) 18:11. doi: 10.1186/s12964-019-0505-5
155. Lin HM, Mak B, Yeung N, Huynh K, Meikle TG, Mellett NA, et al. Overcoming enzalutamide resistance in metastatic prostate cancer by targeting sphingosine kinase. EBioMedicine. (2021) 72:103625. doi: 10.1016/j.ebiom.2021.103625
156. Lin HM, Mahon KL, Weir JM, Mundra PA, Spielman C, Briscoe K, et al. A distinct plasma lipid signature associated with poor prognosis in castration-resistant prostate cancer. Int J Cancer. (2017) 141:2112–20. doi: 10.1002/ijc.30903
157. Lin HM, Huynh K, Kohli M, Tan W, Azad AA, Yeung N, et al. Aberrations in circulating ceramide levels are associated with poor clinical outcomes across localised and metastatic prostate cancer. Prostate Cancer Prostatic Dis. (2021) 24:860–70. doi: 10.1038/s41391-021-00338-z
158. Mak B, Lin HM, Kwan EM, Fettke H, Tran B, Davis ID, et al. Combined impact of lipidomic and genetic aberrations on clinical outcomes in metastatic castration-resistant prostate cancer. BMC Med. (2022) 20:112. doi: 10.1186/s12916-022-02298-0
159. Scheinberg T, Lin HM, Fitzpatrick M, Azad AA, Bonnitcha P, Davies A, et al. PCPro: a clinically accessible, circulating lipid biomarker signature for poor-prognosis metastatic prostate cancer. Prostate Cancer Prostatic Dis. (2023) 27:136–43. doi: 10.1038/s41391-023-00666-2
160. Uchimoto T, Komura K, Fukuokaya W, Kimura T, Takahashi K, Fujiwara Y, et al. Risk stratification for the prediction of overall survival could assist treatment decision-making at diagnosis of castration-resistant prostate cancer: a multicentre collaborative study in Japan. BJU Int. (2021) 127:212–21. doi: 10.1111/bju.15187
161. Yamada Y, Sakamoto S, Rii J, Yamamoto S, Kamada S, Imamura Y, et al. Prognostic value of an inflammatory index for patients with metastatic castration-resistant prostate cancer. Prostate. (2020) 80:559–69. doi: 10.1002/pros.23969
162. Kosaka T, Hongo H, Mizuno R, Oya M. Risk stratification of castration-resistant prostate cancer patients treated with cabazitaxel. Mol Clin Oncol. (2018) 9:683–8. doi: 10.3892/mco.2018.1724
163. Bauckneht M, Rebuzzi SE, Signori A, Frantellizzi V, Murianni V, Lodi Rizzini E, et al. The prognostic power of inflammatory indices and clinical factors in metastatic castration-resistant prostate cancer patients treated with radium-223 (BIO-Ra study). Eur J Nucl Med Mol Imaging. (2022) 49:1063–74. doi: 10.1007/s00259-021-05550-6
164. Del Re M, Biasco E, Crucitta S, Derosa L, Rofi E, Orlandini C, et al. The detection of androgen receptor splice variant 7 in plasma-derived exosomal RNA strongly predicts resistance to hormonal therapy in metastatic prostate cancer patients. Eur Urol. (2017) 71:680–7. doi: 10.1016/j.eururo.2016.08.012
165. Seitz AK, Thoene S, Bietenbeck A, Nawroth R, Tauber R, Thalgott M, et al. AR-V7 in peripheral whole blood of patients with castration-resistant prostate cancer: association with treatment-specific outcome under abiraterone and enzalutamide. Eur Urol. (2017) 72:828–34. doi: 10.1016/j.eururo.2017.07.024
166. Todenhöfer T, Azad A, Stewart C, Gao J, Eigl BJ, Gleave ME, et al. AR-V7 transcripts in whole blood RNA of patients with metastatic castration resistant prostate cancer correlate with response to abiraterone acetate. J Urol. (2017) 197:135–42. doi: 10.1016/j.juro.2016.06.094
Keywords: castration-resistant prostate cancer, liquid biopsy, biomarker, progression monitoring, therapy response
Citation: Chrenková E, Študentová H, Holá K, Kahounová Z, Hendrychová R, Souček K and Bouchal J (2024) Castration-resistant prostate cancer monitoring by cell-free circulating biomarkers. Front. Oncol. 14:1394292. doi: 10.3389/fonc.2024.1394292
Received: 01 March 2024; Accepted: 23 August 2024;
Published: 10 September 2024.
Edited by:
Pierre-Jean Lamy, Groupe Inovie, FranceCopyright © 2024 Chrenková, Študentová, Holá, Kahounová, Hendrychová, Souček and Bouchal. This is an open-access article distributed under the terms of the Creative Commons Attribution License (CC BY). The use, distribution or reproduction in other forums is permitted, provided the original author(s) and the copyright owner(s) are credited and that the original publication in this journal is cited, in accordance with accepted academic practice. No use, distribution or reproduction is permitted which does not comply with these terms.
*Correspondence: Jan Bouchal, jan.bouchal@upol.cz