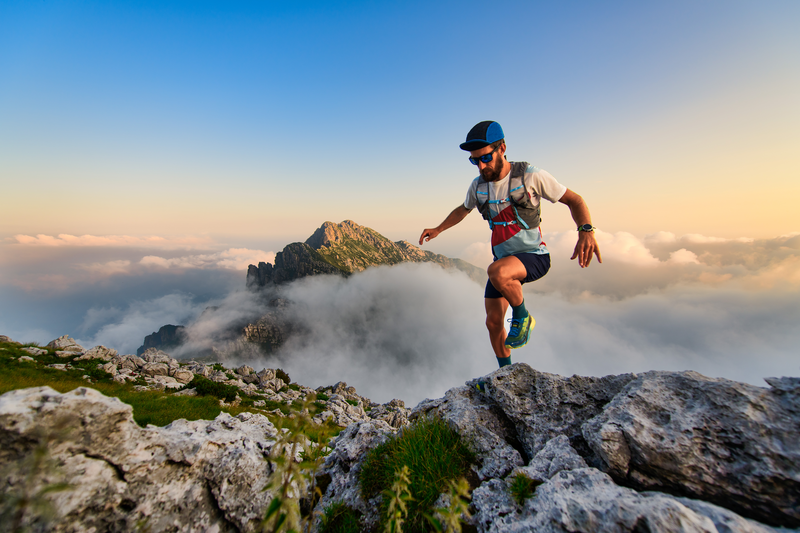
95% of researchers rate our articles as excellent or good
Learn more about the work of our research integrity team to safeguard the quality of each article we publish.
Find out more
ORIGINAL RESEARCH article
Front. Oncol. , 02 September 2024
Sec. Radiation Oncology
Volume 14 - 2024 | https://doi.org/10.3389/fonc.2024.1392741
Purpose: The body contour of patients with cervical cancer is prone to change between radiotherapy sessions. This study aimed to investigate the effect of body contour changes on the setup and dosimetric accuracy of radiotherapy.
Methods: 15 patients with cervical cancer after surgery were randomly selected for retrospective analysis. The body contours on the once-per-week cone-beam computed tomography (CBCT) were registered to the planning CT (pCT) for subsequent evaluation. A body contour conformity index (CIbody) was defined to quantify the variation of body changes. The body volume measured by CBCT was collected, and its relative difference in reference with the first CBCT was calculated and denoted by ΔVn. The relative setup errors, denoted by ΔSELR, ΔSEAP, ΔSESI, and ΔSEvec for left–right, anterior–posterior, superior–inferior, and vectorial shifts, respectively, were defined as the difference in measured setup errors between the reference and following CBCTs. The planned dose was calculated on the basis of virtual CT generated from CBCT and pCT by altering the CT body contour to fit the body on CBCT without deformable registration. The correlations between body contour changes and relative setup errors as well as dosimetric parameters were evaluated using Spearman’s correlation coefficient rs.
Results: CIbody was found to be negatively correlated with the superior–inferior and vectorial relative setup errors ΔSESI (rs = −0.448, p = 0.001) and ΔSEvec (rs = −0.387, p = 0.002), and no significant correlation was found between relative setup errors and ΔVn. Moreover, ΔVn was negatively correlated with ΔD2 (rs = −0.829, p < 0.001), ΔD98 (rs = −0.797, p < 0.001), and ΔTVPIV (rs = −0.819, p < 0.001). ΔD2, ΔD98, and ΔTVPIV were negatively correlated with ΔVn (p < 0.005). No correlation was found for other examined dosimetric parameters.
Conclusion: The body contour change of patients could be associated with the setup variability. The effect of body contour changes on dose distribution is minimal. The extent of body change could be used as a metric for radiation therapists to estimate the setup errors.
Cervical cancer is the fourth most common female cancer worldwide, with 60% of patients being diagnosed under the age of 50 years (1, 2). Radiotherapy for cervical cancer after radical surgery reduces the risk of local recurrence (3, 4). Treatment planning is typically performed on the basis of planning computed tomography (pCT) dataset of patients. During the course of radiation therapy, anatomy changes in patients are inevitable due to weight change, patient setup, and bladder and rectal fillings (2, 5–7). The effects of dietary control, drug effects, and toxic reactions to radiotherapy predispose to changes in body shape, especially in the abdomen. Consequently, the body contour is expected to deviate on each treatment fraction, as the setup tattoos could move with respect to the internal anatomy, which could lead to an increase in the setup error without daily imaging guidance (8–11). However, to the authors’ knowledge, the effect of body contour changes on the setup error is yet to be investigated.
Body contour changes could also cause variations in dose projection with deviated beam path and entry angle, which could be substantial for intensity-modulated radiotherapy characterized by high dose conformality and steep gradients (12). In particular, when using volumetric modulated arc therapy (VMAT) involving simultaneous modulation of the gantry speed, dose rate, and multi-leaf collimators, the effect of body contour changes on the dosimetric outcome is difficult to evaluate, and deviations between planned and delivered doses are complicated (13, 14). Replanning may be necessary with the body contour changes exceeding tolerance, and the examination of pre-treatment cone-beam CT (CBCT) is the primary tool for such judgements (15–18). The dose deviation could be quantitatively assessed by calculating the dose distribution on the basis of CBCT–CT registration (19–21). However, this method is not quite practical because the process is time-consuming and the imaging quality of CBCT could limit the calculation accuracy.
Several studies have investigated the effect of body contour change on the dose distribution and suggested various efficient methods for assessment. Weppler et al. proposed a body contour change threshold of 1.5 cm for replanning for head and neck cancer (22). Sun et al. proposed several rules of thumb for dose percentage change and isodose line shift caused by body contour change for prostate and head and neck cancer (12). However, few studies have examined this issue on the basis of patients’ body changes throughout the treatment course. Besides, for cervical cancer after surgery, where the patient anatomy is prone to change, the dose variation caused by body contour changes is worth investigating. In addition, a quantitative metric could be advantageous for reliable assessment.
Body contour change could significantly increase patient positioning variability. With proper imaging-guided positioning, the resulting dose distribution could generally remain within an acceptable range with the presence of body contour and size changes. However, the dosimetric accuracy without daily imaging guidance could be impaired with increasing body changes and corresponding setup errors. This study aimed to investigate the correlation of body contour change and the setup and dosimetric accuracy and provide an efficient metric for treatment accuracy.
A total of 15 patients with cervical cancer, who received VMAT radiotherapy after surgery in The First Hospital of Jilin University from 2019 to 2022, were randomly selected for this study. Table 1 summarizes the information of the patients. The height and weight of each patient was collected on the day of CT simulation to calculate the body mass index (BMI; the body mass divided by the square of the body height).
Patients were immobilized in supine position with thermoplastic masks (Klarity Medical & Equipment Co. Ltd., Guangzhou, China). The pCTs were acquired with 5 mm slice thickness using the Philips Brilliance Big Bore CT scanner (Philips Healthcare, Cleveland, OH) and then transmitted to the Eclipse treatment planning system (TPS) version 15.6 (Varian Medical Systems, Palo Alto, CA, USA).
According to the Radiation Therapy Oncology Group 0418 protocol (23), the clinical target volume (CTV), organs at risk (OARs; bladder, rectum, small bowel, and left and right femoral heads) and body were delineated on the pCT. The planning target volume (PTV) was obtained by adding an isotropic 5 mm margin to the CTV. The PTV was prescribed with a dose of 50 Gy in 25 fractions. Dual-arc VMAT plans using 6 MV photon beam were generated in the Eclipse TPS. The dose distribution was calculated using AcurosXB version 15.6 algorithm with a grid size of 2.5 mm. All plans were normalized to deliver 100% of the prescribed dose to 95% of the PTV. One TrueBeam linear accelerator (Varian Medical Systems, Palo Alto, CA, USA) was used to treat all patients.
All patients underwent pre-treatment CBCT in the first fraction and at least once in each following week. The CBCT images were acquired using the clinical pelvis protocol (125 kV, 1080 mAs, half-fan mode, full trajectory, 88 slices with 2 mm slice thickness, 47 cm field of view) on a Varian on-board CBCT. If the obtained setup error exceeds or nears the clinical tolerance in the previous CBCT, CBCT would also be performed in the subsequent fraction, and the localization marks would be revised when applicable. In fractions with no CBCT taken, patient setup would be based on localization marks and reference couch positions. If the deviation of the couch position is beyond tolerance, patient repositioning and CBCT would be performed. A total of 77 CBCT images were collected (five CBCTs for thirteen patients and six CBCTs for two patients). CBCT–CT rigid registrations were performed on the basis of bony anatomy, and manual adjustments may be involved if necessary. The resulting treatment couch shift was applied automatically. The couch shift obtained in the first CBCT for each patient was defined as the reference setup error. The relative setup errors, denoted by ΔSELR, ΔSEAP, ΔSESI, and ΔSEvec for left–right, anterior–posterior, superior–inferior, and vectorial shifts, respectively, were defined as the difference between the reference and the following setup errors obtained in CBCTs to avoid potential errors from differences between simulation and treatment units as well as operation therapists. In addition, the interval days between CT and CBCTs were collected.
CBCT images were imported into TPS, and the body volume of each CBCT (Vbody) was extracted. As shown in Figure 1, each pair of CBCT and pCT datasets was rigidly registered. The body conformity index (CIbody) was defined as follows to quantify the degree of body contour change in each CBCT (n ≥ 2):
Figure 1. Demonstration of vCT generation for a patient. (A) pCT; (B) CBCT; (C) CBCT–pCT registration; (D) vCT. The CT values in the added area within the updated body contour of vCT are set as 0 HU.
where Vn∩1 is the overlapping body volume in CBCT1 and CBCTn, and V1 and Vn are the body volumes in CBCT1 and CBCTn, respectively.
The differences in body volumes between CBCT1 and the following CBCTn, denoted by ΔVn, were calculated as follows:
which was used as a surrogate of body size changes. The correlations between relative setup errors and ΔVn as well as CIbody were evaluated.
As the CBCT field of view is limited, only the overlapping body contour was assessed. The body contour in CBCT was copied into pCT, and the volume outside this contour was assigned with a CT number of −999 Hounsfield units (HU). The gap between the original and the new body contours in pCT were assigned with 0 HU for simplicity. Although in reality, this part could mostly be adipose tissue (mass density of ~0.9 g/cm3) with a typical CT number ranging from −190 to −30 HU (24), it could not substantially affect the dose distribution. The overlapping partin pCT and CBCT remained unchanged, including the PTV and OARs. Deformed registration of the patient anatomy was not used in this study to exclude confounding factors for the dosimetric variation caused by body contour changes. The resulting dataset was defined as the virtual CT (vCTn; n represents the order of CBCT). In order to eliminate the impact of the systematic deviation between CT and CBCT operations, the vCT1 was used as the reference image, which was later compared with each subsequent vCTn for the dosimetric evaluation.
The original treatment plan was copied onto each vCT, and the plan doses were recalculated with the same monitor units. The variations of dosimetric parameters with ΔVn and CIbody were evaluated, including CI, D2, D98, TVPIV, small bowel V40Gy, rectum V30Gy, bladder V45Gy, and femoral head V30Gy. The conformity index (CI) was calculated using Paddick’s formula as follows (25, 26):
where TV indicates the PTV volume, PIV indicates the prescription isodose volume, and TVPIV indicates the target volume within the PIV.
Spearman’s rank correlation coefficient (rs) was used to evaluate possible correlations of CIbody and ΔVn with setup errors, treatment time, BMI, and dosimetric parameters. p < 0.05 was defined as statistically significant. |rs| ≥ 0.7 was considered a strong correlation, 0.7 > |rs| ≥ 0.5 was considered a moderate correlation, 0.5 > |rs| ≥ 0.3 was considered a week correlation, and |rs| < 0.3 was considered no correlation. All statistical analyses were performed using IBM SPSS Statistics 26.0 software (IBM Corporation, Armonk, NY).
Figure 2 demonstrates the correlation between relative setup errors and CIbody as well as ΔVn. ΔSESI (p = 0.001, rs = −0.448) and ΔSEvec (p = 0.002, rs = −0.387) were found to be negatively correlated with CIbody, and no correlation was found for ΔSELR nor ΔSEAP (p > 0.05). No significant correlation was found with ΔVn.
Figure 2. Correlation between relative setup errors (ΔSELR, ΔSEAP, ΔSESI, and ΔSEvec) and CIbody as well as ΔVn.
Figure 3 demonstrates that no significant correlation was found between the variation of CIbody and treatment time nor between the standard deviation of CIbody and BMI.
Figure 3. (A) Correlation between CIbody and Time (day); (B) correlation between standard deviation of CIbody and BMI.
Figure 4 shows that CIbody and ΔVn were significantly correlated with ΔD2, ΔD98, and ΔTVPIV (p < 0.005). Strong or near-strong correlations were found for ΔVn with these parameters, while weak correlations were found for CIbody. No correlations were found for the other examined dosimetric parameters, including CI, HI, and OAR dose-volume parameters of interest.
During the treatment of patients with cervical cancer, changes in body contour is expected in each treatment fraction. This study primarily focused on evaluating the effect of body contour changes on the setup errors and dosimetric accuracy in cervical cancer radiotherapy, providing parameters for prompt assessment of treatment accuracy.
CIbody and ΔVn were defined to measure the discrepancy in the patients’ body contour to quantitatively assess the body contour change. As mentioned above, the first CBCT of each patient was used as the reference dataset for the assessment of setup errors rather than the pCT. The aim of using the first CBCT as the reference is to exclude the potential errors brought by differences between CT simulations, treatment units and operation staff. Therefore, the relative setup errors, i.e., ΔSE, were calculated for subsequent analyses for the correlation between setup errors and CIbody as well as ΔVn.
As shown in Figure 2, the relative setup errors ΔSESI and ΔSEvec were negatively correlated with CIbody. Patni et al. measured higher SI setup variations than AP and LR variations for uterine and cervical cancer (17), in line with the present data. By comparison, no correlation was found with ΔVn, indicating that CIbody is a better metric to access patient setup variability. Patients with large setup variations might not have large body contour changes, but patients with out-of-tolerance CIbody would potentially possess a greater risk of larger setup variations. Since daily CBCT is time-consuming and may not be realistic in practice, CIbody could serve as an assistant metric for the consideration of CBCT schedules.
Regarding the dose distribution in this study, ΔVn was strongly or near strongly correlated with ΔD2, ΔD98, and ΔTVPIV, which are essentially the parameters susceptible to the beam attenuation variation caused by increased or decreased body size. However, the CI, HI, and all examined OAR dosimetric parameters showed no correlation with ΔVn, mainly because the dose calculation was performed on the basis of vCT generated with CBCT–pCT rigid registration with only body contour updated to isolate the effect of body contour changes. The internal changes in the patient anatomy would be a greater influence factor on the dosimetric distribution. Considering the patient anatomy change was not accounted for, the dosimetric change could solely depend on the body contour change, which is also the reason that no correlation between CIbody and most dosimetric parameters was found. Although CIbody was weakly correlated with ΔD2, ΔD98, and ΔTVPIV, it is hardly a meaningful correlation considering that CIbody could not reflect whether the body volume increases or decreases. Besides, the target region of patients with cervical cancer is relatively large, whereas the CBCT field of view is limited and hence could not fully cover the whole target area and OARs. Despite that the current results implied that the body contour changes may not cause substantial dosimeric variation, the patient setup variability could be increased for patients with steep changes in the body contour and body size. The increased risk of worsening dose distribution is not negligible, especially without daily CBCT.
The effect of changes in body contour, body size, or body weight on the dose distribution have been investigated in a number of studies (27–31). Miguel et al. (27) studied the effect of body change of patients with prostate cancer on the planned dose and found that the difference between planned and actual doses could be more than 5% when the difference between the anterior and lateral contour of patients exceeded 1.7 cm. Sasaki et al. (28) investigated the dosimetric effect of body contour changes in patients with cervical cancer and suggested that re-planning is rarely necessary with the body contour manually reduced by 1 cm in the front, whereas the variation became significant with the body contour reduced by 1 cm in all directions except the back, implying that dose distribution is affected by body size. The simulated variations of body contour and body size differed from the variations based on CBCTs in this study, but the results are in agreement. D’Souza et al. (29) evaluated the dosimetric effects due to changes in external body surface during the treatment course for prostate and head and neck cancers and proposed a 3.7%–5.2% change in the plan maximum dose per centimeter change in path length to isocenter. The body contours in the above two studies were either reduced or increased globally, which is rarely the case in clinical practice. In the present study, the body contour changes were extracted from patient CBCTs. The variation of body contour is complicated, and it may include simultaneous expansion and shrinkage. Therefore, the dosimetric effect was not considerably substantial as in previous studies.
Although the results in the present study suggested that the conformity of body contour throughout the treatment course may not significantly affect the dose distribution as long as the body size did not change drastically, it may not be the case without pre-treatment CBCT. The setup error variability could rise with increased discrepancy in the body contour, which could lead to a potentially greater difference in the planned and delivered doses. Based on the setup and dosimetric effect of body contour changes, radiation therapists should pay further attention to the change in body contour through pre-treatment CBCT in clinical practice. The extent of body contour changes defined by CIbody and ΔVn could be used as reference parameters for radiation therapists to provide feedback to physicians on whether replanning is required. Actionable cutoff values for the metrics would be practical for this method to be applied in practice. Considering that the clinical protocol varies among treatment centers, the adopted cutoff could be decided individually.
One of the limitations in this study is that the BMI of each patient was only recorded prior to treatment but not in each fraction. The initial BMI is analyzed with the standard deviation of CIbody for the predictability of body changes. The present result indicated that the initial BMI could not serve as a predictor of body contour changes during the treatment course. BMI or body weight in each treatment fraction could be a potential indicator for body contour changes. Another limitation is that, as mentioned above, the current dosimetric evaluation was performed on the basis of vCT with only body contour changed within CBCTs. The patient anatomy was kept unchanged to isolate the effect of body contour changes. Deformable registration of patient anatomy in the vCT could potentially provide different dosimetric results (19–21), which is not within the scope of this study.
CIbody and ΔVn were proposed to quantitatively assess the extent of body contour changes. CIbody showed moderate correlation with setup errors in the SI direction, and ΔVn was strongly correlated with dosimetric changes including ΔD2, ΔD98, and ΔTVPIV. Considering that the dosimetric accuracy without daily CBCT could be impaired with increasing body changes and corresponding setup errors, CIbody and ΔVn could be used as an indicator for clinical radiation therapists to provide feedback to physicists for assessments of CBCT scheduling and replanning in clinical treatment.
The original contributions presented in the study are included in the article/supplementary material. Further inquiries can be directed to the corresponding author.
The studies involving humans were approved by The Ethics Committee of the First Hospital of Jilin University. The studies were conducted in accordance with the local legislation and institutional requirements. Written informed consent for participation was not required from the participants or the participants’ legal guardians/next of kin in accordance with the national legislation and institutional requirements.
YL: Conceptualization, Data curation, Methodology, Writing – original draft, Writing – review & editing. WS: Data curation, Formal analysis, Methodology, Software, Writing – original draft, Writing – review & editing. SL: Data curation, Writing – review & editing. WX: Data curation, Writing – review & editing. XY: Formal analysis, Investigation, Writing – review & editing. LW: Formal analysis, Investigation, Writing – review & editing. CG: Formal analysis, Writing – review & editing. KC: Data curation, Formal analysis, Writing – review & editing. YS: Data curation, Writing – review & editing. HW: Conceptualization, Funding acquisition, Supervision, Writing – original draft, Writing – review & editing.
The author(s) declare financial support was received for the research, authorship, and/or publication of this article. This work was supported by the Jilin Scientific and Technological Development Program (No. YDZJ202201ZYTS685).
The authors declare that the research was conducted in the absence of any commercial or financial relationships that could be construed as a potential conflict of interest.
All claims expressed in this article are solely those of the authors and do not necessarily represent those of their affiliated organizations, or those of the publisher, the editors and the reviewers. Any product that may be evaluated in this article, or claim that may be made by its manufacturer, is not guaranteed or endorsed by the publisher.
1. Bray F, Ferlay J, Soerjomataram I, Siegel RL, Torre LA, Jemal A. Global cancer statistics 2018: GLOBOCAN estimates of incidence and mortality worldwide for 36 cancers in 185 countries. CA Cancer J Clin. (2018) 68:394–424. doi: 10.3322/caac.21492
2. Jadon R, Pembroke CA, Hanna CL, Palaniappan N, Evans M, Cleves AE, et al. A systematic review of organ motion and image-guided strategies in external beam radiotherapy for cervical cancer. Clin Oncol. (2014) 26:185–96. doi: 10.1016/j.clon.2013.11.031
3. Asano H, Todo Y, Watari H. Adjuvant chemotherapy for early-stage cervical cancer. Chin J Cancer Res. (2016) 28:228–34. doi: 10.21147/j.issn.1000-9604.2016.02.12
4. Salani R, Khanna N, Frimer M, Bristow RE, Chen LM. An update on post-treatment surveillance and diagnosis of recurrence in women with gynecologic Malignancies: Society of Gynecologic Oncology (SGO) recommendations. Gynecol Oncol. (2017) 146:3–10. doi: 10.1016/j.ygyno.2017.03.022
5. Mahantshetty U, Naga P, Nachankar A, Ghadi Y, Dheera A, Scaria L, et al. Set-up errors, organ motion, tumour regression and its implications on internal target volume-planning target volume during cervical cancer radiotherapy: results from a prospective study. Clin Oncol (R Coll Radiol). (2022) 34:189–97. doi: 10.1016/j.clon.2021.10.010
6. Chan P, Dinniwell R, Haider MA, Cho YB, Jaffray D, Lockwood G, et al. Inter- and intrafractional tumor and organ movement in patients with cervical cancer undergoing radiotherapy: a cinematic-MRI point-of-interest study. Int J Radiat Oncol Biol Phys. (2008) 70:1507–15. doi: 10.1016/j.ijrobp.2007.08.055
7. Ahmad R, Hoogeman MS, Bondar M, Dhawtal V, Quint S, De Pree I, et al. Increasing treatment accuracy for cervical cancer patients using correlations between bladder-filling change and cervix-uterus displacements: proof of principle. Radiother Oncol. (2011) 98:340–6. doi: 10.1016/j.radonc.2010.11.010
8. Johansen J, Bertelsen A, Hansen CR, Westberg J, Hansen O, Brink C. Set-up errors in patients undergoing image guided radiation treatment. Relationship to body mass index and weight loss. Acta Oncol. (2008) 47:1454–8. doi: 10.1080/02841860802256517
9. Lin LL, Hertan L, Rengan R, Teo BK. Effect of body mass index on magnitude of setup errors in patients treated with adjuvant radiotherapy for endometrial cancer with daily image guidance. Int J Radiat Oncol Biol Phys. (2012) 83:670–5. doi: 10.1016/j.ijrobp.2011.07.026
10. Tsujii K, Ueda Y, Isono M, Miyazaki M, Teshima T, Koizumi M. Dosimetric impact of rotational setup errors in volumetric modulated arc therapy for postoperative cervical cancer. J Radiat Res. (2021) 62:688–98. doi: 10.1093/jrr/rrab044
11. Singh A, Singh S, Mani KR, Das KJM, Kumar S. Comparing setup errors using portal imaging in patients with gynecologic cancers by two methods of alignment. J Med Imaging Radiat Sci. (2020) 51:394–403. doi: 10.1016/j.jmir.2020.04.001
12. Sun L, Kirkby C, Smith W. Dosimetric effect of body contour changes for prostate and head and neck volumetric modulated arc therapy plans. J Appl Clin Med Phys. (2019) 20:115–24. doi: 10.1002/acm2.12571
13. Lee J, Park JM, Wu HG, Kim JH, Ye SJ. The effect of body contouring on the dose distribution delivered with volumetric-modulated arc therapy technique. J Appl Clin Med Phys. (2015) 16:365–75. doi: 10.1120/jacmp.v16i6.5810
14. Chow JC, Jiang R. Comparison of dosimetric variation between prostate IMRT and VMAT due to patient’s weight loss: Patient and phantomstudy. Rep Pract Oncol Radiother. (2013) 18:272–8. doi: 10.1016/j.rpor.2013.05.003
15. Brown E, Owen R, Harden F, Mengersen K, Oestreich K, Houghton W, et al. Predicting the need for adaptive radiotherapy in head and neck cancer. Radiother Oncol. (2015) 116:57–63. doi: 10.1016/j.radonc.2015.06.025
16. Yao L, Zhu L, Wang J, Liu L, Zhou S, Jiang S, et al. Positioning accuracy during VMAT of gynecologic Malignancies and the resulting dosimetric impact by a 6-degree-of-freedom couch in combination with daily kilovoltage cone beam computed tomography. Radiat Oncol. (2015) 10:104. doi: 10.1186/s13014-015-0412-x
17. Patni N, Burela N, Pasricha R, Goyal J, Soni TP, Kumar TS, et al. Assessment of three-dimensional setup errors in image-guided pelvic radiotherapy for uterine and cervical cancer using kilovoltage cone-beam computed tomography and its effect on planning target volume margins. J Cancer Res Ther. (2017) 13:131–6. doi: 10.4103/0973-1482.199451
18. Stauch Z, Zoller W, Tedrick K, Walston S, Christ D, Hunzeker A, et al. An evaluation of adaptive planning by assessing the dosimetric impact of weight loss throughout the course of radiotherapy in bilateral treatment of head and neck cancer patients. Med Dosim. (2020) 45:52–9. doi: 10.1016/j.meddos.2019.05.003
19. Fischer AM, Hague T, Hoskin PJ. CBCT-based deformable dose accumulation of external beam radiotherapy in cervical cancer. Acta Oncol. (2023) 62:923–31. doi: 10.1080/0284186X.2023.2238543
20. van Heerden LE, Visser J, Koedooder K, Rasch CR, Pieters BR, Bel A. Role of deformable image registration for delivered dose accumulation of adaptive external beam radiation therapy and brachytherapy in cervical cancer. J Contemp Brachytherapy. (2018) 10:542–50. doi: 10.5114/jcb.2018.79840
21. Salehi M, Vafaei Sadr A, Mahdavi SR, Arabi H, Shiri I, Reiazi R. Deep learning-based non-rigid image registration for high-dose rate brachytherapy in inter-fraction cervical cancer. J Digit Imaging. (2023) 36:574–87. doi: 10.1007/s10278-022-00732-6
22. Weppler S, Quon H, Banerjee R, Schinkel C, Smith W. Framework for the quantitative assessment of adaptive radiation therapy protocols. J Appl Clin Med Phys. (2018) 19:26–34. doi: 10.1002/acm2.12437
23. Jhingran A, Winter K, Portelance L, Miller B, Salehpour M, Gaur R, et al. A phase II study of intensity modulated radiation therapy to the pelvis for postoperative patients with endometrial carcinoma: radiation therapy oncology group trial 0418. Int J Radiat Oncol Biol Phys. (2012) 84:e23–8. doi: 10.1016/j.ijrobp.2012.02.044
24. Cote JA, Nazare JA, Nadeau M, Leboeuf M, Blackburn L, Despres JP, et al. Computed tomography-measured adipose tissue attenuation and area both predict adipocyte size and cardiometabolic risk in women. Adipocyte. (2016) 5:35–42. doi: 10.1080/21623945.2015.1106057
25. Paddick I. A simple scoring ratio to index the conformity of radiosurgical treatment plans. Tech note J Neurosurg. (2000) 93 Suppl 3:219–22. doi: 10.3171/jns.2000.93.supplement
26. ICRU. ICRU report 91: prescribing, recording, and reporting of stereotactic treatments with small photon beams. J Int Comm Radiat Units Meas. (2014) 14:1–145. doi: 10.1093/jicru_ndx010
27. Miguel D, de la Llana V, Martinez D, Del Castillo A, Alonso D, De Frutos J, et al. Dosimetric variations for high-risk prostate cancer by VMAT plans due to patient’s weight changes. J Radiother Pract. (2019) 18:336–42. doi: 10.1017/S1460396919000177
28. Sasaki M, Ikushima H. Impact of changes in body contours on radiation therapy dose distribution after uterine cervical cancer surgery. Jpn J Radiol. (2020) 38:1099–107. doi: 10.1007/s11604-020-01011-6
29. D’Souza H, Weatherburn H, Dwivedi A, Ganesh T. Volumetric arc therapy treatment plan dosimetry correction method to account patient weight loss during a course of radiation therapy. J Med Phys. (2020) 45:1–6. doi: 10.4103/jmp.JMP_86_19
30. Jou J, Coulter E, Roberts T, Binder P, Saenz C, McHale M, et al. Assessment of malnutrition by unintentional weight loss and its implications on oncologic outcomes in patient with locally advanced cervical cancer receiving primary chemoradiation. Gynecol Oncol. (2021) 160:721–8. doi: 10.1016/j.ygyno.2020.12.009
Keywords: cervical cancer, volumetric modulated arc therapy, body contour change, delivery accuracy, setup accuracy
Citation: Li Y, Sun W, Liu S, Xia W, Yang X, Wang L, Ge C, Chen K, Shi Y and Wang H (2024) Effect of body contour changes on the setup and dosimetric accuracy of radiotherapy after cervical cancer surgery. Front. Oncol. 14:1392741. doi: 10.3389/fonc.2024.1392741
Received: 28 February 2024; Accepted: 16 August 2024;
Published: 02 September 2024.
Edited by:
Timothy James Kinsella, Brown University, United StatesReviewed by:
Francesco Ricchetti, Sacro Cuore Don Calabria Hospital (IRCCS), ItalyCopyright © 2024 Li, Sun, Liu, Xia, Yang, Wang, Ge, Chen, Shi and Wang. This is an open-access article distributed under the terms of the Creative Commons Attribution License (CC BY). The use, distribution or reproduction in other forums is permitted, provided the original author(s) and the copyright owner(s) are credited and that the original publication in this journal is cited, in accordance with accepted academic practice. No use, distribution or reproduction is permitted which does not comply with these terms.
*Correspondence: Huidong Wang, d2FuZ19oZDIwMTBAamx1LmVkdS5jbg==
Disclaimer: All claims expressed in this article are solely those of the authors and do not necessarily represent those of their affiliated organizations, or those of the publisher, the editors and the reviewers. Any product that may be evaluated in this article or claim that may be made by its manufacturer is not guaranteed or endorsed by the publisher.
Research integrity at Frontiers
Learn more about the work of our research integrity team to safeguard the quality of each article we publish.