- 1Department of Radiation Oncology, Cancer Center, West China Hospital, Sichuan University, Chengdu, Sichuan, China
- 2Department of Thoracic Surgery and Institute of Thoracic Oncology, West China Hospital, Sichuan University, Chengdu, Sichuan, China
- 3Western China Collaborative Innovation Center for Early Diagnosis and Multidisciplinary Therapy of Lung Cancer, Chengdu, Sichuan, China
- 4Faculty of Dentistry, The University of Hong Kong, Hong Kong, Hong Kong SAR, China
Heat shock protein 70 (HSP70) is a highly conserved protein functioning as a “molecular chaperone”, which is integral to protein folding and maturation. In addition to its high expression within cells upon stressful challenges, HSP70 can be translocated to the cell membrane or released from cells in free form or within extracellular vesicles (EVs). Such trafficking of HSP70 is also present in cancer cells, as HSP70 is overexpressed in various types of patient samples across a range of common malignancies, signifying that extracellular HSP70 (eHSP70) can serve as a tumor biomarker. eHSP70 is involved in a broad range of cancer-related events, including cell proliferation and apoptosis, extracellular matrix (ECM) remodeling, epithelial-mesenchymal transition (EMT), angiogenesis, and immune response. eHSP70 can also induce cancer cell resistance to various treatments, such as chemotherapy, radiotherapy, and anti-programmed death-1 (PD-1) immunotherapy. Though the role of eHSP70 in tumors is contradictory, characterized by both pro-tumor and anti-tumor effects, eHSP70 serves as a promising target in cancer treatment. In this review, we comprehensively summarized the current knowledge about the role of eHSP70 in cancer progression and treatment resistance and discussed the feasibility of eHSP70 as a cancer biomarker and therapeutic target.
1 Introduction
Heat shock protein 70 (HSP70) is a crucial member of the heat shock protein family with a molecular weight of approximately 70 kD. This protein is highly conserved across different species, indicating its essential role in maintaining cellular homeostasis. The human HSP70 family has 13 homologs, among which mitochondrial HSP70 (HSPA9/GRP75/Mortalin) and endoplasmic reticulum HSP70 (HSPA5/GRP78/BiP) are the most extensively studied members (1). Despite differences in gene locus, amino acid residues, and subcellular localization, the HSP70 family has a common structure consisting of two major functional domains: a conserved nucleotide-binding domain (NBD) at N-terminal and a more variable substrate-binding domain (SBD) at C-terminal. The NBD is crucial for binding to and hydrolyzing ATP, while the SBD can bind to substrate proteins. Coupled with ATP hydrolysis, HSP70 interacts with substrate proteins, facilitating their correct folding, preventing aggregation, and refolding damaged proteins (2). Thus, HSP70 functions as a “molecular chaperone”.
Upon stressful challenges, HSP70 is highly induced and capable of directly inhibiting cellular apoptosis (3). However, in pathologic conditions like cancer, upregulated HSP70 induces disease progression and treatment resistance (4). Extensive studies have indicated that HSP70 is not only highly expressed in tumor cells but also can be released extracellularly. Notably, such a type of transport or extracellular expression is minimal in normal cells. Hence, extracellular HSP70 (eHSP70) endows with “cancer” characteristics. This character can be extended to two facets: first, it can be leveraged as a tumor biomarker for development; second, exploration of the correlation between eHSP70 and tumor progression can be undertaken to evaluate its feasibility as a molecular target for cancer therapy. Numerous researchers have robustly demonstrated the feasibility of these two approaches; however, there is no corresponding approved strategy for clinical application. In this review, we comprehensively summarized the current knowledge about the role of eHSP70 in cancer progression and treatment resistance and discussed and explored the feasibility of eHSP70 as a cancer biomarker and therapeutic target.
2 Translocation of eHSP70
HSP70 can be found on the cell membrane or be transported to the extracellular milieu, either associated with extracellular vesicles (EVs) or as free soluble protein (Figure 1). Among the existing reports and databases, the HSP70 family members identified for expression on the plasma membrane encompass HSPA1A, HSPA1B, HSPA1L, HSPA2, HSPA5, and HSPA8, while those secreted extracellularly in the form of exosomes include HSPA1A, HSPA1B, HSPA2, GRP78, HSPA6, HSPA8, mortalin, HSPA12A, and HSPA13 (5). The association of HSP70 with the cell membrane does not seem to be simply due to the lack of discernible membrane-binding motifs or other translocation signatures within HSP70. However, extensive studies have confirmed its insertion into lipid bilayers with high affinity towards negatively charged phospholipids, particularly phosphatidylserine (PS) (6–9). HSP70 spontaneously relocates from the cytosol into the plasma membrane after oligomerization and binding to PS (10). Lipid rafts are microdomains enriched in cholesterol, glycosphingolipids, and protein receptors. It has been discovered that HSP70 preferentially localizes in lipid rafts, with one potential mechanism being facilitated via non-covalent interactions with globotriaosylceramide (Gb3) (11, 12). Besides, palmitoyl-oleoyl phosphatidylglycerol (POPG) and sulfogalactosyl ceramide, which are also negatively charged, have been reported to mediate the insertion of HSP70 into membrane structures (8, 13).
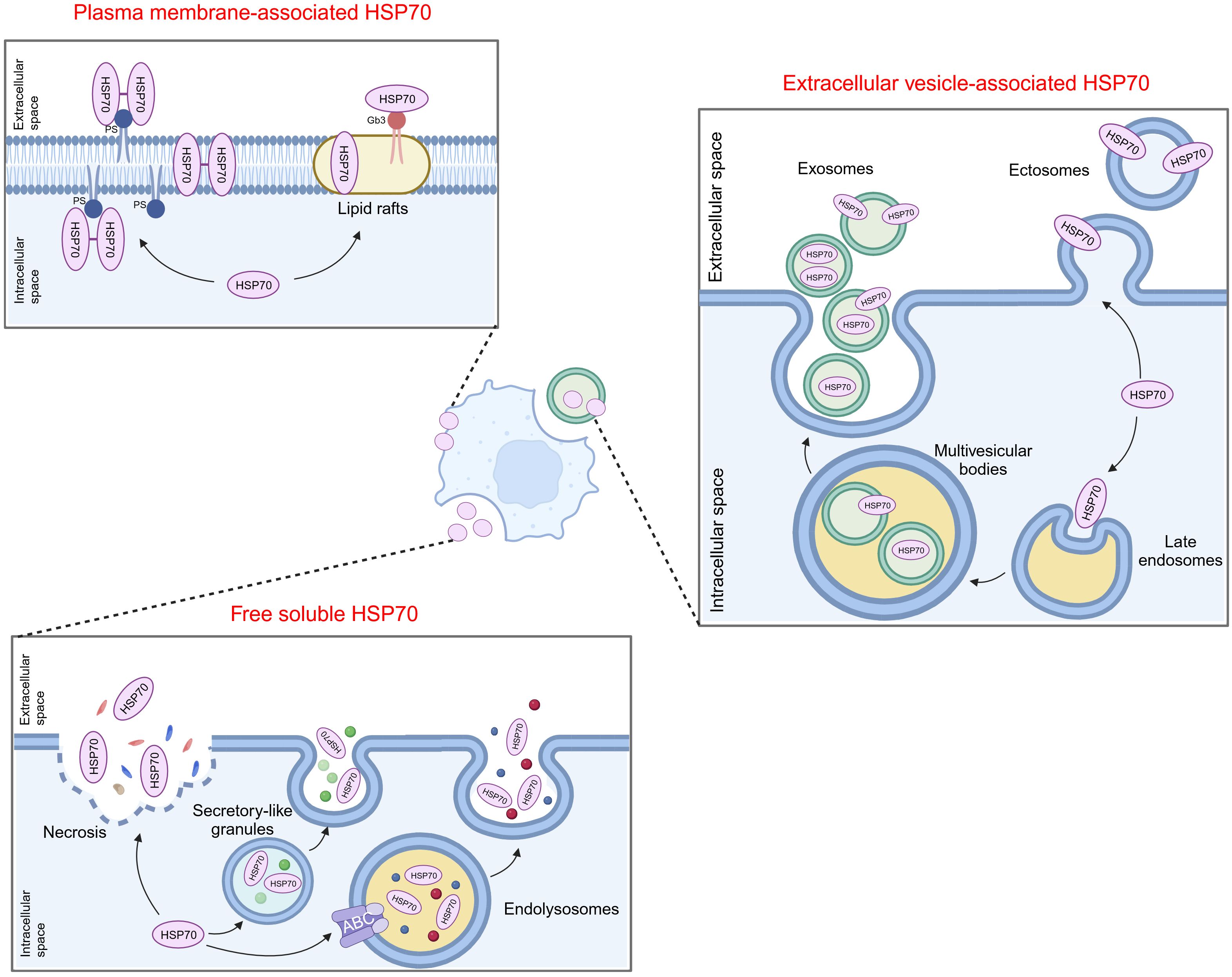
Figure 1 Translocation of extracellular HSP70. HSP70 can localize on the cell membrane or be transported to the extracellular milieu, either associated with extracellular vesicles or as free soluble protein. PS, phosphatidylserine; Gb3, globotriaosylceramide; ABC, ATP-binding cassette.
The secretion of HSP70 into the extracellular milieu also appears complex. Free soluble HSP70 was initially believed to be exclusively the result of passive release after cell death until several reports indicated that this release mainly depends on active mechanisms (14). One possible way is that HSP70 is exported via secretory-like granules (15). Additionally, Mambula and Calderwood found that HSP70 can infiltrate into endolysosomes via ATP-binding cassette (ABC) family transporter proteins and subsequently being released from cells after the fusion of endolysosomes with the plasma membrane (16). HSP70 can also be released into or onto EVs by either exocytosis of exosomes or by budding of the plasma membrane (ectosomes) (17–19). Several studies demonstrated that post-translational modifications may be involved in mediating the translocation of HSP70 into exosomes (20, 21). Moreover, oligomerized HSP70 was shown to be preferentially loaded into exosomes (22, 23).
It is noteworthy that these mechanisms are not exclusively activated in a single type of cell or under a uniform condition, and multiple eHSP70 transport modalities may concurrently exist in the same cell type. Moreover, there are many types of stimuli that induce HSP70 insertion into membrane structures or release into the extracellular space. It is evident that these translocations mostly occur in cancer cells. In response to stressful stimuli, such as hypoxia, immune response, and therapeutic stress, cancer cells may circumvent pressure by inducing the manifestation of eHSP70. Hence, advanced exploration of the extracellular transport of HSP70 and its triggering parameters can help obstruct the intrinsic protection of cancer cells at its origin.
3 The role of eHSP70 as a tumor biomarker
Previously, clinical studies indicated that HSP70 displays specific expression in numerous tumors across diverse types of samples, such as tumor tissues, peripheral blood, and urine, verifying its predictive role in tumor diagnosis, treatment, and prognosis (Table 1). Compared with healthy individuals or those suffering from benign conditions, elevated expression of circulating HSP70 is observed in the peripheral blood samples of cancer patients, and its expression is significantly amplified in the cell membranes within tumor tissues. This phenomenon spans a range of common malignancies; thus, eHSP70 can serve as a biomarker for cancer diagnosis (25, 26, 28, 29, 37, 40, 41, 43, 45, 49, 52–54, 58–63, 66, 68). Additionally, eHSP70 expression has been observed to positively correlate with the malignant behavior of tumors. Patients with advanced non-small cell lung cancer (NSCLC) exhibit elevated serum levels of HSP70 or GRP78, compared to the early-stage cases (34, 69). In breast cancer, individuals with increased serum levels of HSPA1A commonly display a higher histological grade and cell proliferation index (Ki67) (43). Given these findings, a decrease in eHSP70 levels often predict improved therapeutic responses. For instance, the concentrations of circulating HSP70 decrease as the tumor volume decreases after radiotherapy among patients with glioblastoma. Reduced expression of circulating HSP70 predicts prolonged overall survival (OS) in these patients (30, 64). It is worth noting that elevated eHSP70 levels do not necessarily imply a poorer outcome. Pfister et al. found that in patients with colon and gastric carcinomas, the expression of membrane-associated HSP70 correlates significantly with an improved OS (49). Moreover, a study also demonstrated that the concentration of circulating HSP70 significantly decreased in patients with lung cancer compared to healthy controls (26). These conclusions may originate from the differences in patient characteristics, or the paradoxical role of eHSP70 in tumor progression.
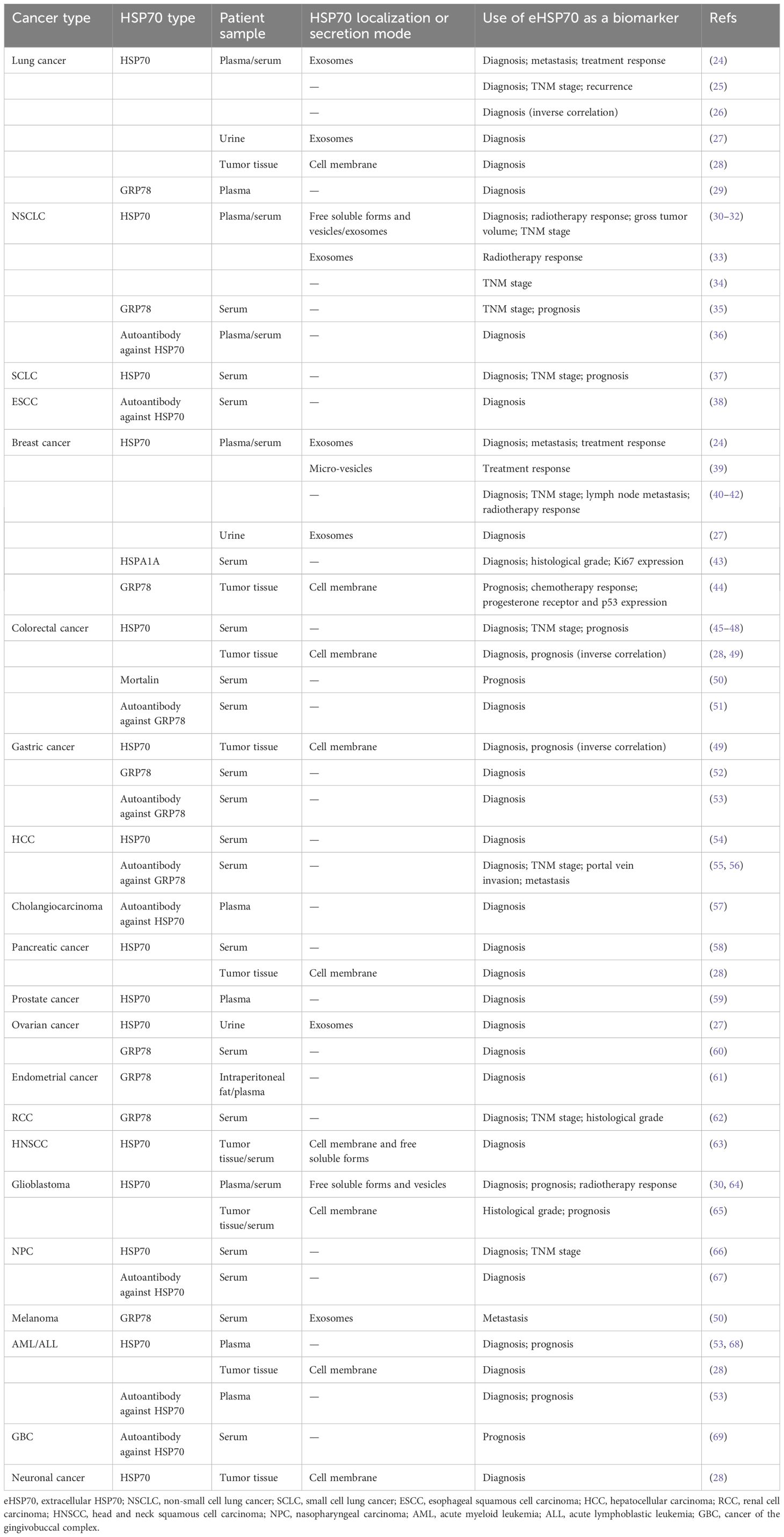
Table 1 Selection of studies assessing extracellular HSP70 as a potential cancer biomarker in patient samples.
Nonetheless, quantifying the levels of HSP70 in patients’ blood or other body fluids revealed inconclusive results, partly due to the short half-life of HSP70 in blood. Consequently, several studies have quantified circulating autoantibodies against HSP70. Elevated levels of autoantibodies against HSP70 have been corroborated in different cancers, including NSCLC (36), esophageal squamous cell carcinoma (38), colorectal cancer (51), gastric cancer (70), hepatocellular carcinoma (HCC) (55, 56), cholangiocarcinoma (57), nasopharyngeal carcinoma (67), acute myeloid leukemia (AML)/acute lymphoblastic leukemia (ALL) (53), and cancer of gingivobuccal complex (69). In HCC, the presence of autoantibodies against GRP78 was associated with clinical stage, portal vein invasion, and metastasis (55). These findings prove the potential of autoantibodies against HSP70 as alternative cancer biomarkers.
Surprisingly, HSP70 also exists in the form of EVs in peripheral blood or urine samples of patients with lung cancer (24, 27), NSCLC (30–33), breast cancer (24, 27, 39), ovarian cancer (27), glioblastoma (30, 64), and melanoma (50), protecting it from the phospholipid bilayer membrane and making it more stable. This discovery has thus opened up novel avenues for validating eHSP70 as an effective biomarker for cancers. The levels of exosomal HSP70 in plasma/serum samples of patients with lung cancer, breast cancer, or melanoma are correlated with tumor metastasis (24, 50). Another study showed that among 35 patients with post-therapeutic regression two years after the initial diagnosis of non-metastatic breast cancer, escalating quantities of micro-vesicular HSP70 were observed in two patients during treatment. Conversely, patients who did not succumb to a relapse maintained unchanged levels of this protein, suggesting the potential role of micro-vesicular HSP70 in predicting therapeutic efficacy (39). Moreover, Chanteloup and colleagues found that circulating exosomal HSP70 levels, but not free soluble HSP70, reflect HSP70 levels in tumor biopsies. Exosomal levels of HSP70 were more sensitive tumor dissemination predictors compared with circulating tumor cells (24). Similar to purifying circulating tumor cells (CTCs), efficacious EV isolation from diverse biological fluids is technically challenging. There are several methods for EV isolation, and the most commonly used method is differential ultracentrifugation (UC). Other methods include density gradients (DG), immunoaffinity, precipitation, size-exclusion chromatography (SEC), ultrafiltration, and microfluidics (71). Though we will not elaborate in this comprehensive review, we believe that groundbreaking methods can validate HSP70-exosomes as a novel tumor biomarker.
4 The role of eHSP70 in cancer progression
While delving into the application of eHSP70 as a tumor biomarker, many researchers have attempted to validate the feasibility of eHSP70 as a therapeutic target for cancer. This notion originates from numerous studies indicating the significant role of eHSP70 in several aspects of cancer progression, including proliferation and apoptosis, extracellular matrix (ECM) remodeling, epithelial-mesenchymal transition (EMT), angiogenesis, and immune environment modulation.
4.1 Proliferation and apoptosis
eHSP70 regulates the proliferation and apoptosis of numerous tumors, including colon cancer, hepatocellular carcinoma, breast cancer, endometrial cancer, glioma, and others (Figure 2) (72–76). The toll-like receptor (TLR) family constitutes a class of type I transmembrane glycoproteins that can recognize damage-associated molecular patterns (DAMPs) and participate in innate immunity (77). As a ligand for TLR2 and TLR4, extracellular HSPA1A potently stimulates proliferation and inhibits apoptosis in hepatocellular carcinoma cells via TLR2 and TLR4 signaling pathway and activation of nuclear factor-κB (NF-κB) (73). Cripto is a glycoprotein anchored to the membrane via glycosylphosphatidylinositol (GPI) that plays a pivotal role in tumor progression (78). It was found that the interaction of Cripto and GRP78 at the surface of cell membranes is crucial for cancer cell proliferation, potentially mediated via the activation of mitogen-activated protein kinase (MAPK)-phosphoinositide 3 kinase (PI3K) pathways and suppression of transforming growth factor β (TGF-β)-dependent phosphorylation of small mother against decapentaplegic 2/3 (SMAD2/3) (79, 80). In prostate cancer cells, disrupting the attachment of membrane GRP78 and surfactant protein-D (SP-D), a constituent of the collectin family, may interfere with the pro-tumorigenic role of extracellular GRP78 (81). Notably, GRP78 is not only secreted extracellularly but also interacts with the membrane-localized GRP78 as a receptor. This facilitates the proliferation of colon cancer cells by activating the PI3K/protein kinase B (AKT) and Wnt/β-catenin signaling, thereby introducing an innovative paradigm for the role of autocrine GRP78 in cancer progression (72).
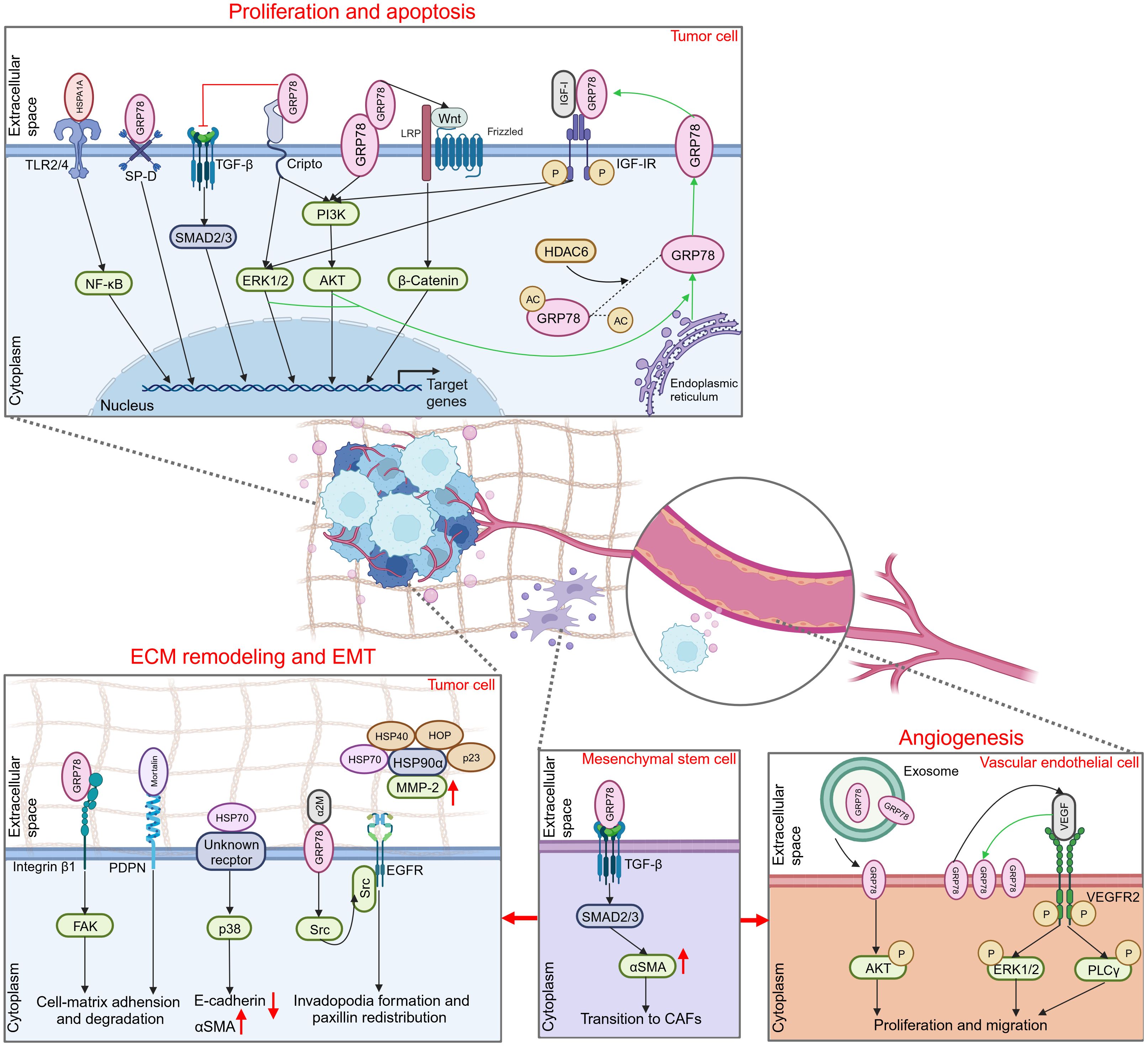
Figure 2 Promoting role of extracellular HSP70 in cancer progression. Through an autocrine way or tuning the behavior of different types of cells in the extracellular milieu, extracellular HSP70 is capable of enhancing cell proliferation, inhibiting cell apoptosis, and inducing ECM, EMT and angiogenesis to exert tumor-promoting function. TLR2/4, toll-like receptor 2/4; NF-κB, nuclear factor-κB; SP-D, surfactant protein-D; TGF-β, transforming growth factor β; SMAD2/3, small mother against decapentaplegic 2/3; ERK1/2, extracellular regulated protein kinases 1/2; PI3K, phosphoinositide 3-kinase; AKT, protein kinase B; LRP, lipoprotein receptor related protein; IGF-I, insulin-like growth factor I; IGF-IR, IGF-I receptor; HDAC6, histone deacetylase 6; ECM, extracellular matrix; EMT, epithelial-mesenchymal transition; FAK, focal adhesion kinase; PDPN, podoplanin; αSMA, α smooth muscle actin; α2M, α2-macroglobin; EGFR, epidermal growth factor receptor; MMP-2, matrix metalloproteinase-2; CAFs, cancer-associated fibroblasts; VEGF, vascular endothelial growth factor; VEGFR2, VEGF receptor 2; PLCγ, phospholipase C γ.
In addition, some studies have focused on the intracellular to extracellular transport of HSP70 to elucidate its primary proliferating effect on tumor cells. Kim and colleagues revealed that inhibition of histone deacetylase 6 (HDAC6), a cytoplasmically localized deacetylase, can potentiate GRP78 acetylation, thereby attenuating the translocation of GRP78 to the cell surface via the PI3K/AKT signaling pathway. This event substantially inhibits the proliferation of cholangiocarcinoma and promotes its apoptosis, suggesting the pivotal role of membrane GRP78 in the progression of cholangiocarcinoma (82). In hepatoma cells, insulin-like growth factor I (IGF-I) similarly initiates GRP78 expression from the endoplasmic reticulum to the plasma membrane via the PI3K and MAPK pathways. This subsequently stimulates the phosphorylation and activation of the IGF-I receptor (IGF-IR) via membrane GRP78 and promotes IGF-I-mediated cellular proliferation and migration (83).
4.2 ECM remodeling and EMT
ECM is a complex network composed of macromolecules synthesized and secreted by cells that furnish biochemically and structurally supportive functions to the cellular component. This dynamic structure constantly undergoes a remodeling process, playing a significant role in cancer invasion and migration. In certain circumstances, this dynamic structure provokes another hallmark of cancer, EMT (84, 85). Several studies have illustrated that HSP70 can exert its effects on ECM remodeling and EMT via expression on the cell surface, and corresponding extracellular client proteins or membrane surface receptors associated with it have been sequentially uncovered (Figure 2). Cell surface GRP78 was identified to interact with the ECM adhesion molecule β1-integrin and mediate cell-matrix adhesion and ECM degradation by modulating focal adhesion kinase (FAK), thereby promoting colorectal cancer cell migration and invasion (86). Podoplanin (PDPN), which plays an important role in cell adhesion, is associated with mortalin released by oral squamous cell carcinoma cells on their cell surface (87). This suggests that extracellular mortalin potentially promotes tumor growth and invasion through PDPN-mediated pathways. In liver cancer, eHSP70 plays a critical role in regulating the EMT process (88, 89). Despite its unidentified receptor, eHSP70 has been confirmed to induce E-cadherin degradation and α smooth muscle actin (αSMA) overexpression by activating the p38/MAPK signaling pathway (88).
Activated α2-macroglobin (α2M*) is a natural circulating ligand of cell surface GRP78 (90). In conjunction with α2M*, membrane-associated GRP78 in hepatocellular carcinoma (HCC) cells is capable of interacting directly with Src, a tyrosine kinase, stimulating its phosphorylation and further facilitating the interaction between Src and epidermal growth factor receptor (EGFR). This effect can elicit the invasion and migration of HCC by promoting invadopodia formation and paxillin redistribution (91). Furthermore, eHSP70 may exert its function by orchestrating cells within the TME or interacting with other molecular chaperones. Cancer-associated fibroblasts (CAFs), a type of tumor-associated stromal cells, provide a favorable environment for the progression of malignant tumors through multiple mechanisms, such as ECM remodeling and angiogenesis (92). Peng and co-workers identified that GRP78 secreted by tumor cells can induce the differentiation of bone marrow-derived mesenchymal stem cells (BMSCs) to CAFs by activating the TGF-β/Smad signaling pathway (93). Another study reported that synergizing with HOP, HSP40, and p23 potentiates the activation of HSP90α on matrix metalloproteinase-2 (MMP-2), thereby enhancing breast cancer cell invasion and migration (94).
4.3 Angiogenesis
Angiogenesis is pivotal for nutritional supply and tumor metabolism, particularly in hypoxic conditions. Hypoxia can stimulate tumor cells to secrete vascular endothelial growth factor A (VEGFA) that binds to VEGF receptor 2 (VEGFR2) on neighboring vascular endothelial cells, thereby inducing the motility of endothelial cells and remodeling of ECM (95). The association between HSP70 and tumor angiogenesis was initially proposed by Dong and colleagues. They discovered that the microvessel density (MVD) of endogenous mammary tumors was significantly decreased in GRP78 heterozygous mice, with no effect on the MVD of normal organs (96). Subsequently, they investigated the role of GRP78 in the TME, revealing that the vascular formation was suppressed during the early phase of wild-type mammary tumors in GRP78 heterozygous mice. Similarly, the growth and metastasis of the tumor were profoundly inhibited (97). This finding indicated that GRP78 can contribute to tumor progression by promoting angiogenesis. Although the exact mechanism has not been elaborated, researchers believe that the extracellular expression of GRP78 may be involved in VEGF-induced angiogenesis. Subsequent studies verified these findings (Figure 2). Iha et al. observed that exosomes secreted by gastric cancer cells overexpressing GRP78 increased the proliferation and migratory capacity of co-cultured vascular endothelial cells, likely by the augmentation of AKT phosphorylation (98). Another study revealed that GRP78 was notably overexpressed on the membrane of human umbilical vein endothelial cells (HUVECs) following VEGF treatment, and ablation of GRP78 significantly suppressed VEGF-induced phosphorylation of phospholipase Cγ (PLCγ) and extracellular regulated protein kinases 1/2 (ERK1/2) and inhibited endothelial cell proliferation (99). These findings suggest that GRP78 not only contributes to angiogenesis through the secretion of tumor cells but also via epithelial cells. In addition, elevated HSP70 level was detected in tumor tissue samples of certain patients and was positively correlated with VEGF expression and MVD, substantiating the proangiogenic role of eHSP70 in tumors (100, 101).
4.4 Immune response
The relationship between eHSP70 and immune response was first discovered to facilitate the release of pro-inflammatory cytokines from monocytes, such as interleukin (IL)-1β, IL-6, and tumor necrosis factor-α (TNF-α) (102–105). It engages monocytes via TLR2/4 in a CD14-dependent manner, stimulating the myeloid differentiation primary response gene 88 (MyD88)/interleukin-1 receptor-associated kinase (IRAK)/NF-κB signaling cascade to promote the production of pro-inflammatory cytokines (103). Other receptors, including CD40, CD36, and CD11b, were also found to mediate those effects, partly via the p38/MAPK pathway (104, 105). Subsequently, an increasing body of evidence indicated that eHSP70, contrary to its well-established oncogenic functions in other aspects of cancer biology, plays a paradoxical role in immune responses against cancer cells (Figure 3).
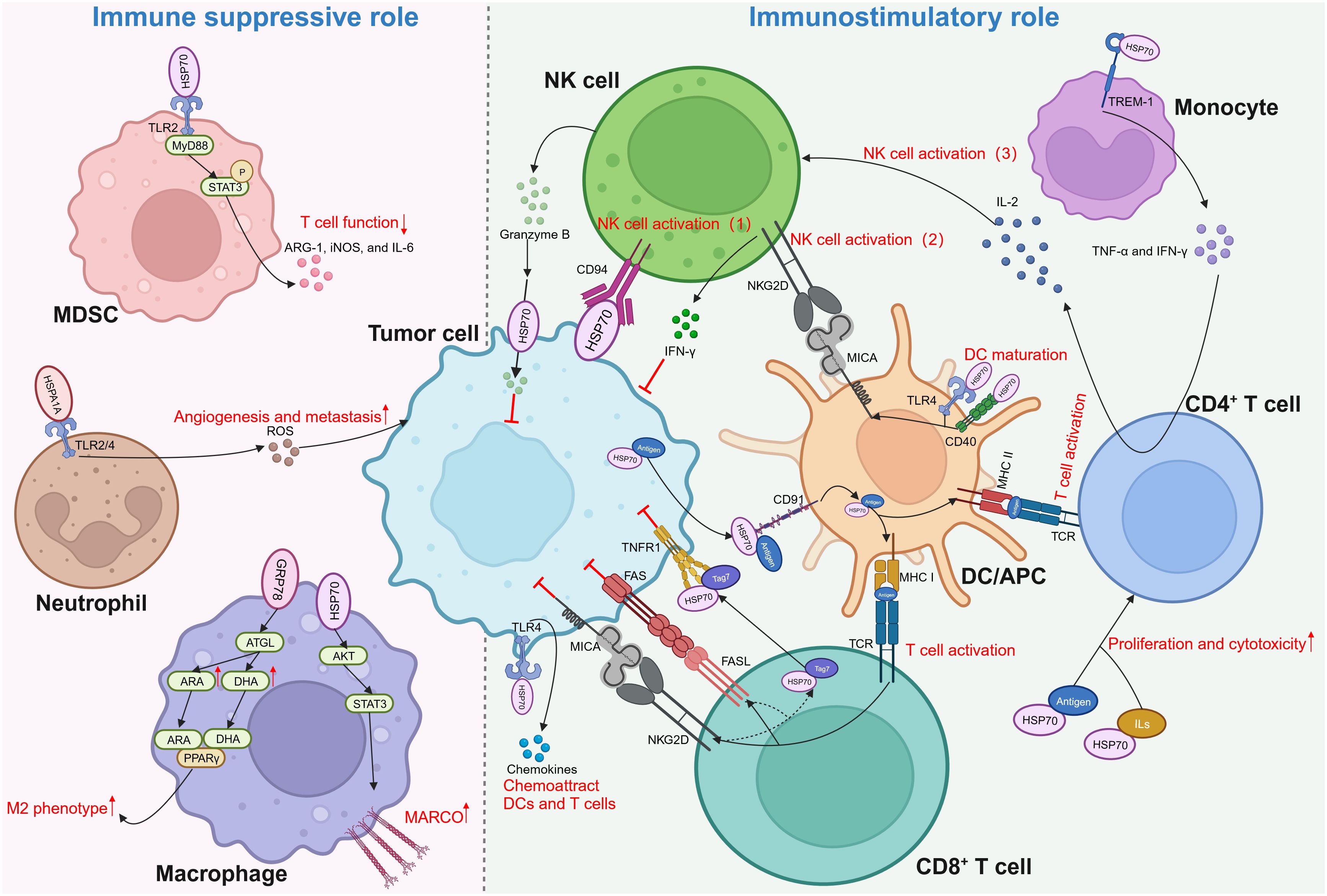
Figure 3 Dual role of extracellular HSP70 in immune response against cancers. Through activating different types of immune cells, extracellular HSP70 plays an immune suppressive role as well as immunostimulatory role. MDSC, myeloid-derived suppressor cells; TLR, toll-like receptor; MyD88, myeloid differentiation primary response gene 88; STAT3, p-signal transducer and activator of transcription 3; ARG-1, arginase-1; iNOS, inducible nitric oxide synthase; IL, interleukin; ROS, reactive oxygen species; ATGL, adipose triglyceride lipase; ARA, arachidonic acid; DHA, docosahexaenoic acid; PPARγ, peroxisome proliferator-activated receptor γ; AKT, protein kinase B; MARCO, macrophage receptor with collagenous structure; NK, natural killer; IFN-γ, interferon-γ; NKG2D, natural killer group 2 member D; TCR, T cell receptor; MHC, major histocompatibility complex; MICA, MHC class I chain-related protein A; TREM-1, triggering receptors expressed on myeloid cells-1; TNF-α, tumor necrosis factor-α; DC, dendritic cell; APC, antigen-presenting cell; TNFR1, tumor necrosis factor receptor 1; FAS, factor-associated suicide; FASL, FAS ligand.
4.4.1 Immune-suppressive function
Myeloid-derived suppressor cells (MDSCs) interact with HSP70 secreted in the form of exosomes by tumor cells, such as breast carcinoma, lung adenocarcinoma, ovarian carcinoma, and renal cell carcinoma cells (27, 106). In a TLR2/MyD88-dependent manner, exosomal HSP70 triggers p-signal transducer and activator of transcription 3 (STAT3) in MDSCs, thereby activating MDSCs and prompting the secretion of arginase-1 (ARG-1), inducible nitric oxide synthase (iNOS), and IL-6 (106). Reactive oxygen species (ROS) are important molecules that can enhance cancer progression, partly by stimulating the expression of VEGF and activating MMPs (107). Extracellular HSPA1A possesses the capacity to activate neutrophils and augment their generation of ROS by interacting with TLR2/4 on the surface of these innate immune cells (108). Macrophages demonstrate robust phagocytic proficiency in engulfing apoptotic cells. Specifically, during chemotherapy or radiation therapy, these cells are capable of phagocytosis and elimination of apoptotic tumor cells, demonstrating both anti-inflammatory and pro-tumor activities (109). A recent study revealed that HSP70 contained within EVs derived from colorectal cancer can enhance the phagocytosis ability of macrophages by upregulating macrophage receptors with collagenous structure (MARCO) via the AKT-STAT3 signaling pathways (110). Moreover, Tian et al. discovered that colorectal cancer cells can polarize macrophages into the M2 phenotype by regulating fatty acid metabolism in macrophages via secretory GRP78. By stabilizing adipose triglyceride lipase (ATGL), GRP78 can stimulate the synthesis of arachidonic acid (ARA) and docosahexaenoic acid (DHA) and enable them to interact with and activate peroxisome proliferator-activated receptor γ (PPARγ), resulting in the M2 polarization of macrophages (111).
4.4.2 Immunostimulatory function
Nevertheless, eHSP70 is believed to be more inclined towards exhibiting an anti-tumor role through its substantial effect on various aspects of innate and adaptive immunity. In 2001, Multhoff and colleagues delineated that HSP70 located on the membrane of tumor cells can activate natural killer (NK) cells to evoke an innate immune response (112). Subsequent studies unveiled that HSP70 can be secreted extracellularly in exosomes or free soluble forms by various tumors, such as melanoma, colorectal cancer, and pancreatic cancer, to activate NK cells (113, 114). NK cells can induce perforin-independent apoptosis in HSP70 membrane-positive tumor cells by releasing serine protease granzyme B (115). This might be mediated by the interaction between eHSP70 and type C lectin receptor CD94 on the surface of NK cells (116–118). Additionally, two other mechanisms indirectly regulate NK cell activation by eHSP70. eHSP70 was reported to induce the expression of major histocompatibility complex (MHC) class I chain-related protein A (MICA) on dendritic cells (DCs). It activates NK cells and promotes the production of interferon-γ (IFN-γ) by binding to natural killer group 2 member D (NKG2D) on NK cells (119, 120). eHSP70 can also interact with the triggering receptors expressed on myeloid cells-1 (TREM-1) receptor on monocytes, resulting in the secretion of TNF-α and IFN-γ, stimulating IL-2 production by CD4+T cells, and activating NK cells (121). Moreover, HSP70 released by tumor cells into the TME can limit the conversion of a considerable proportion of monocytes to the pro-tumor phenotype (122).
The role of eHSP70 in antigen presentation by antigen-presenting cells (APCs) and activation of T cells is noteworthy. eHSP70 can induce the maturation of DCs by acting as a ligand for CD40 or TLR4 (123, 124). Through the TLR4 pathway, eHSP70 can increase the production of chemokines from tumor cells to recruit DCs and T cells (124). Specifically, eHSP70 can promote the cross-presentation of major histocompatibility complex (MHC) class I molecules by APCs to differentiate between antigens, which results in the activation of CD8+ T cells (125, 126). Activated CD8+ T cells can further release a cytotoxic Tag7-HSP70 complex, which induces tumor cell lysis via the tumor necrosis factor receptor 1 (TNFR1) (127). Moreover, secretion of the Tag7-HSP70 complex can be facilitated by the factor-associated suicide ligand (FasL) and NKG2D receptors on the surface of CD8+ T cells (128). HSP70-peptide complexes can also enter into the MHC class II presentation pathway to augment antigen-specific CD4+ T cell responses. The proliferation and cytotoxicity of CD4+ T cells can be enhanced by the HSP70-antigen complexes or HSP70 plus IL-2 or IL-7/IL-12/IL-15 (129, 130). Several studies found that HSP70 can bind to CD91 on APCs to mediate both MHC class I and II presentations (131–134). Other scavenger receptors, such as lectin-like oxidized low-density lipoprotein receptor-1 (LOX-1), were also shown to be HSP70-binding elements expressed on APCs (135–137). Interestingly, studies on HSP70 structure indicated that SBD plays a pivotal role in transporting antigens for binding to MHC class I, while attachment of antigens to the N-terminal results in efficient presentation on MHC class II (138, 139).
eHSP70 plays a pivotal role in cancer immume response. However, its role in the secretion of pro-inflammatory cytokines and activation of DCs and T cells remains controversial. Some studies suggested that lipopolysaccharide (LPS) contamination, not HSP70 itself, mediates this process (140–143). Therefore, numerous researchers avoided LPS contamination during their experiments, revealing that HSP70 itself can evoke these effects (105, 129). Regardless, additional studies are warranted to illuminate this ambiguity.
5 The role of eHSP70 in cancer treatment resistance
Beyond its role in cancer progression, eHSP70 can contribute to the treatment resistance of tumors. GRP78 was found to be released by bortezomib-resistant solid tumor cells. By promoting the phosphorylation of ERK and AKT and inhibiting p53-mediated induction of BOK and NOXA in HUVECs, GRP78 can protect these cells against the anti-angiogenic effects of bortezomib (144). Extracellular GRP78 is also involved in the tamoxifen resistance by binding to its partner protein CD44v at the COOH-terminal proline-rich region (145). A recent study showed that tumor-associated macrophages (TAMs) play vital functions in mediating eHSP70-induced chemoresistance in breast cancer cells. Exposure to epirubicin (EPI) induces breast cancer cells to secret HSP70, which modulates the intracellular expression of TGF-β and directly or indirectly amplifies the pro-tumor effects of TAMs (146). Furthermore, transfer of eHSP70 via small EVs enhances adriamycin (ADR) resistance through reprogramming energy metabolism in recipient cells. eHSP70 may be linked to cell stemness in cisplatin-induced cellular senescence, given that HSP70 levels on the plasma membrane increase after treatment with cisplatin (147, 148).
In addition to chemoresistance, eHSP70 is involved in resistance to other treatments. Upon radiation exposure, the membrane HSP70 expression level was significantly elevated in tumor cells (149). A study revealed that reduced HSP70 expression in the plasma membrane, rather than in the cytosol, diminishes the expression of γH2AX, caspase 3/7, and Annexin V, and decreases the post-irradiation survival rate of tumor cells. This elucidates that eHSP70 has the potential capability to diminish the lethal effects of radiotherapy on tumor cells (150). Cell surface GRP78 regulates the radio-resistance of glioma stem cells (GSCs) by activating the downstream β-site APP-cleaving enzyme 2 (BACE2), which upregulates NF-κB and C/EBPβ pathways (151). Recently, two pioneering studies by Theivanthiran and colleagues unveiled the pivotal role of eHSP70 in tumor adaptive resistance to anti-programmed death-1 (PD-1) immunotherapy. Through the intrinsic NOD-like receptor protein 3 (NLRP3)-HSP70-TLR4 axis, tumor cells can induce the recruitment of MDSCs after treatment with anti-PD-1 antibody. Specifically, eHSP70 regulates this process in an autocrine manner. NLRP3 activation triggers tumor cells to secret HSP70, which, in turn, interacts with TLR4 on the membrane of tumor cells, leading to the release of Wnt5a and upregulation of the CXCR2 ligand, CXCL5 (152). Furthermore, downstream of the TLR4/Wnt5a signaling pathway, initiated by eHSP70 in lung epithelial cells, may promote the recruitment of MDSCs in distal lung tissues, establishing a premetastatic niche that supports disease progression in response to anti-PD-1 immunotherapy (153).
6 Targeting eHSP70 for cancer treatment
Recognizing the significant role of eHSP70 in cancer progression, numerous studies assessed the feasibility of using eHSP70 as a potential therapeutic approach for treating cancer. However, owing to the dual role of eHSP70 in tumors, research efforts can be divided into two directions: inhibiting the tumor-promoting role of HSP70 on the membrane surface of tumor cells (Table 2), and developing HSP70-based vaccines to stimulate the immune response against tumor cells (Table 3).
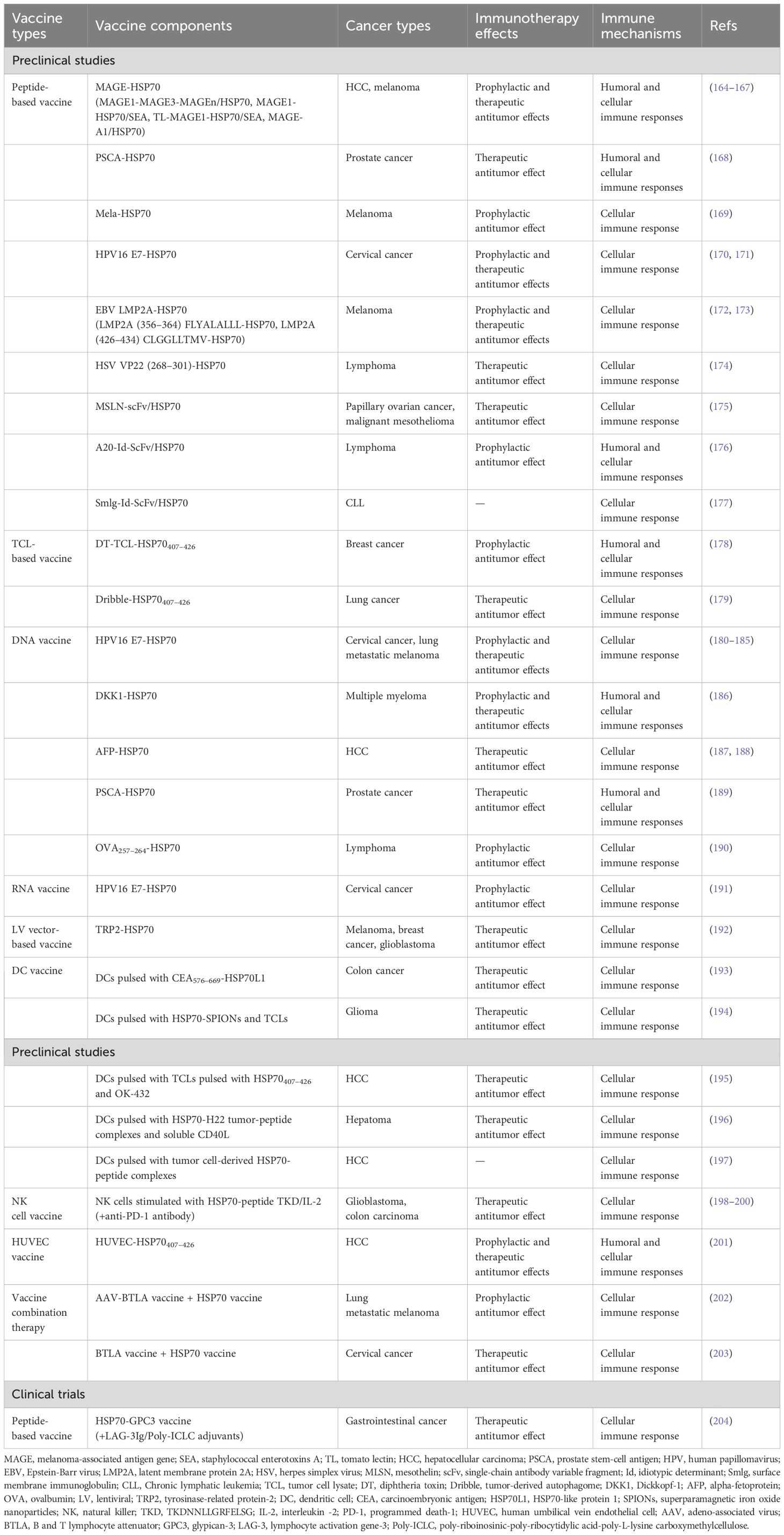
Table 3 Selection of preclinical studies and clinical trials assessing HSP70-based vaccines in cancer therapies.
6.1 Targeting cell surface HSP70
Misra and co-workers identified an antibody against the GRP78 COOH-terminal domain as a potential anti-cancer therapy, based on the results that it can promote tumor cell apoptosis by upregulating p53 expression and downregulating the activation of AKT, ERK1/2, and p38 MAPK (154, 155). Subsequently, several studies aimed to develop or evaluate monoclonal antibodies directed towards the COOH-terminal domain of GRP78. In pancreatic ductal adenocarcinoma (PDAC), the C38 monoclonal antibody was proven to curtail cell motility and invasion by inhibiting Rho-induced activation of Yes-associated protein (YAP) and tafazzin (TAZ). Using C38 can significantly enhance tumor cell radiosensitivity and increase the efficacy of radiation therapy (156). C107 monoclonal antibody was identified to have the ability of suppressing tumor growth as well. More crucially, its inhibitory effect appears superior to C38 in melanoma (157). Liu et al. reported another monoclonal antibody against GRP78 named MAb159, which exhibited notable anti-tumor effects in multiple tumor types, such as breast cancer, colon cancer, lung adenocarcinoma, small cell lung cancer (SCLC), prostate cancer, and leukemia. By blocking GRP78-induced activation of PI3K signaling, MAb159 can inhibit tumor growth and promote tumor metastasis in a mouse xenograft model (158).
In addition to monoclonal antibodies, some other drugs, compounds, or peptides were shown to disrupt the binding of HSP70 to its corresponding receptors or ligands on the cancer cell membrane, and remarkably inhibit its function. Treating with heparin derivatives can block autoantibodies binding to cell surface GRP78 and decrease tissue factor expression/activity, subsequently inhibiting tumor growth (159). By attenuating GRP78–p85α complex formation on the plasma membrane, 2’-fluoro-6,7-methylenedioxy-2-phenyl-4-quinolone (CHM-1) can reverse the inhibitory effect of apoptosis induced by the activation of PI3K/AKT signaling in nasopharyngeal carcinoma (NPC) cells (160). In colorectal cancer, the combination of a class III secretory peroxidase derived from foxtail millet bran (FMBP) with the NBD of cell surface GRP78 interferes with the downstream activation of STAT3, thus promoting the intracellular accumulation of ROS and cell grown inhibition (161). Isthmin (ISM) is a secretory 60-kDa protein that potently induces endothelial cell apoptosis. Two studies indicated that ISM or BC71 peptide derived from the ISM AMOP domain (adhesion-associated domain in MUC4 and other proteins) is capable of triggering the apoptosis of tumor cells and HUVEC by inducing mitochondrial dysfunction and activating caspase-8 and p53 signaling pathways, thus inhibiting tumor angiogenesis (162, 163). Furthermore, analyzing exosomes from various tumor samples indicated that A8 peptide aptamer can bind to the extracellular domain of membrane HSP70, effectively disrupting HSP70/TLR2 association and impairing the ability of tumor-derived exosomes to activate MDSCs. Given the fact that chemotherapy drugs, such as cisplatin and 5FU, augment the abundance of HSP70 exosomes, facilitating the activation of MDSCs and hindering the progression of anti-tumor immune response, a combination of A8 with cisplatin or 5FU can prevent MDSC activation (27).
Specifically, due to the high expression of HSP70 on the membrane of tumor cells, several studies have profiled eHSP70 as a molecular target for precision drug delivery. Chimeric antigen receptor T (CAR-T) cell immunotherapy is emerging as an effective cancer treatment; however, the application of CAR-T cell therapy in solid tumors remains limited. One possible reason is the absence of efficient tumor antigen targets (205). GRP78-directed CAR-T (GRP78-CAR-T) cells have shown strong anti-tumor efficacy in several solid tumors, such as pancreatic cancer, lung cancer, glioblastoma, and breast cancer, without obvious off-target effects or T cell infiltration in major organs (206–212). Moreover, several researchers have combined HSP70 binding peptide with other anti-cancer proteins or encased it within nanoparticles to precisely and effectively deliver chemotherapeutic drugs (213–220). For example, Farshbaf and co-workers loaded bortezomib with nanostructured lipid carriers (NLCs) modified with two proteolytically stable D-peptides, D8 and RI-VAP (Dual NLCs). Due to the high affinity of D8 for nicotine acetylcholine receptors on brain capillary endothelial cells and the high specificity of RI-VAP for binding to GRP78 on tumor cells, dual NLCs can pass the blood-brain tumor barrier with superior glioma-homing properties. They effectively deliver bortezomib and produce a potent anti-proliferative effect (221). The fusion of HSP70 tumor-penetrating peptide with nanoparticles can similarly be utilized to facilitate the delivery of novel hybrid iron oxide (Fe3O4), resulting in radiosensitization in triple-negative breast cancer cells (222). It is noteworthy that targeting cell surface HSP70 not only enhances drug delivery precision but also synergistically bolsters anti-tumor efficacy by suppressing HSP70 function. More studies, particularly clinical trials, are needed on the use of eHSP70 as a target for cancer treatment.
6.2 Developing HSP70-based vaccines
The development of HSP70-based vaccines is another key research topic. Although no relevant vaccination has been approved for clinical application, numerous studies have demonstrated robust anti-tumor effects of various types of vaccines that contain HSP70, effectively stimulating cellular or even humoral immune responses. Peptide-based vaccines are relatively easy to manufacture and usually composed of the HSP70 protein integrated with tumor-associated antigens (TAAs) or tumor-specific antigens (TSAs), such as melanoma-associated antigen gene (MAGE)-HSP70 (164–167), prostate stem-cell antigen (PSCA)-HSP70 (168), and Mela-HSP70 (169). Two studies produced a promising human papillomavirus 16 (HPV16) protein vaccine of E7-HSP70 that robustly induces E7-specific CD8+ T cell immune response and resulted in significant prophylactic and therapeutic effects against E7-expressing cervical cancer (170, 171). Other tumor-associated virus proteins, such as Epstein-Barr virus (EBV) latent membrane protein 2A (LMP2A) (172, 173) and herpes simplex virus (HSV) VP22 (174), were proved to generate effective cellular immune response after fusion with HSP70 protein in melanoma and lymphoma, respectively. Additionally, certain tumor markers were shown to possess limited immunogenicity but considerable specificity. Hence, linking the HSP70 protein to antibody fragments directed against them can dramatically enhance immunogenicity (175–177). Given these findings, a phase I study assessed the safety and efficacy of a novel vaccine comprising multi-human leukocyte antigen (HLA)-binding HSP70/glypican-3 (GPC3) peptides, a novel adjuvant combination of lymphocyte activation gene-3 (LAG-3) Ig, and poly-riboinosinic-poly-ribocytidylic acid-poly-L-lysine carboxymethylcellulose (Poly-ICLC) against metastatic gastrointestinal cancers. Seventeen patients received this vaccination therapy without dose-limiting toxicity, supporting the feasibility of this approach (204).
Compared to single TSA or TAA, tumor cell lysates (TCLs) encompass all self-tumor antigens and lack HLA-A2 restriction. Therefore, using them as vaccines can generate robust anti-tumor immune responses in a wide range of cancers (223). Fusion diphtheria toxin (DT) and two tandem repeats of HSP70407–426 with TCLs elicit strong humoral and cellular immune responses, leading to the growth inhibition of breast cancer (178). In addition, a tumor-derived autophagosome (Dribble) vaccine conjugated with HSP70407–426 significantly induced a higher expression of antigen-specific cytotoxic T lymphocytes (CTLs) (179). Genetic vaccines encompass DNA or RNA vaccines delivered by viruses or plasmids. Although no DNA vaccine has been commercialized globally to date, this particular strategy holds considerable promise. Studies indicated that the combination of HSP70 and HPV16 E7 (180–185, 191), Dickkopf-1 (DKK1) (186), alpha-fetoprotein (AFP) (187, 188), PSCA (189), or ovalbumin (OVA) (190) genes can induce potent immune responses against cancers. Lentiviral transfer of tyrosinase-related protein-2 (TRP2)-HSP70 gene is another efficacious and durable strategy in melanoma, breast cancer, and glioblastoma (192).
Several studies have prepared DC or NK cell vaccines stimulated with HSP70 to elicit an effective anti-tumor response by activating innate and adaptive immunity. Fusion protein CEA576–669-HSP70L1 can promote DC maturation and activate DC to produce cytokines, such as Il-12, IL-1β, and TNF-α, and chemokines, such as macrophage inflammatory protein-1α (MIP-1α) and MIP-1β. They subsequently elicit a potent CTL cytotoxicity against colon cancer (193). Immunization of glioma-bearing rats with DCs pulsed with superparamagnetic iron oxide nanoparticles (SPIONs) coated with HSP70 and tumor cell lysates (TCLs) resulted in a delayed tumor progression (194). In liver cancer, DCs pulsed with TCLs stimulated with HSP70407–426 and OK-432, HSP70-H22 tumor-peptide complexes and soluble CD40L, or tumor cell-derived HSP70-peptide complexes were all induced a cellular immune response (195–197). Furthermore, three studies demonstrated that NK cells stimulated with HSP70-peptide TKD/IL-2 may be a promising therapeutic approach for treating glioblastoma and colon carcinoma (198–200). A novel HUVEC-HSP70407–426 vaccine was reported to exert anti-angiogenesis effects by attenuating tumor-induced angiogenesis and reducing MVD of the intradermal tumor in mice (201). Other types of HSP70-based vaccines, like vaccine combination therapy, are also promising therapeutic approaches against cancer (202, 203). Interestingly, HSP70-based vaccines may induce acquired resistance of tumors to treatment. Geng et al. suggested that B7-H1 expressed by residual tumor cells may be the underlying mechanism. Blockade of B7-H1 by injection of a plasmid encoding the extracellular domain of PD-1 reversed this resistance and enhanced the therapeutic efficacy (224).
7 Conclusions
As a molecular chaperone, HSP70 is dynamically expressed and transported in response to stress stimuli, such as hypoxia, nutrient deficiency, immune stimulation, and therapeutic stress. Hence, the amount of HSP70 protein secreted by cancer cells is partly associated with the current state of the malignancy. This theory suggests that eHSP70 possesses the potential as a tumor biomarker. Analyzing clinical samples of cancer patients revealed that eHSP70 expression can be detected in tumor tissues or various body fluids, and variation in its expression level is associated with patients’ diagnosis, treatment response, and prognosis. Various types of cancers may show specific patterns of eHSP70 upregulation and downregulation that may distinguish them from healthy individuals and from patients with other non-cancerous conditions. Changes in eHSP70 expression may even discriminate one type of cancer from another. Moreover, the monitoring of therapeutic efficacy and potential recurrence can be facilitated by analyzing the expression levels of eHSP70 across several time points specific to each patient. Thus, it is not difficult to integrate artificial intelligence (AI) tools. For instance, quantitative proteomics and machine learning statistics can be integrated to construct a comprehensive cancer prediction network that depends on eHSP70. Nevertheless, the major challenge is how to detect eHSP70 expression real-timely, accurately, and sensitively. Aside from cell membrane eHSP70, the discernible forms of eHSP70 presently comprise the free soluble form, exosomal form, and autoantibodies against HSP70. Each one of these forms possesses advantages and disadvantages. The simplified detection method of free soluble HSP70 is advantageous; however, the short half-life of this form makes it challenging to precisely quantify its expression in a real-time manner. Exosomal HSP70 is more stable, but the current isolation technology for exosomes remains immature, and autoantibodies against HSP70 lack sufficient specificity and sensitivity. Therefore, overcoming these technological challenges can greatly facilitate the clinical use of eHSP70 as a tumor biomarker.
eHSP70 regulates the biological behavior of tumor cells. Not only can eHSP70 activate several signaling pathways in tumor cells in an autocrine manner but it can also tune the behavior of endothelial cells, immune cells, and other cells by interacting with extracellular components and membrane receptors. The diverse function of eHSP70 forms a complex network in the TME. On the one hand, it provides a favorable environment for tumor progression by promoting ECM, EMT, angiogenesis, and treatment resistance. On the other hand, it can act as a tumor-associated antigen to stimulate the activation and maturation of various immune cells, thereby provoking the immune response against tumor cells. Although some studies suggest its immunosuppressive role, most studies still support its immunostimulatory function. Given these findings, studies directly or indirectly targeted HSP70 on the membrane surface of tumor cells or developed HSP70-based vaccines. However, none of those therapeutic strategies have been approved for marketing. The inadequate safety and stability of HSP70-based tumor therapeutic strategies are challenging. On one hand, eHSP70 has different isoforms and is pivotal for preserving routine cellular activity. On the other hand, targeting distinct tumor-associated cells, such as cancer cells or immunocytes in the TME can potentially trigger different responses. Hence, understanding the structure of eHSP70, especially the SBD, and designing drugs based on this may be an effective way to solve the low specificity of eHSP70-targeting drugs. Furthermore, precisely targeting cell types that express eHSP70, such as precisely targeting HUVECs to inhibit angiogenesis promoted by eHSP70 or developing HSP70-pulsed DC vaccines, would make HSP70-based cancer treatment mores stable without affecting normal cell homeostasis or inducing unpredictable responses. Uncoupling the pro- and anti-tumorigenic effects of extracellular targets and membrane receptors of eHSP70 and selectively inhibiting their pathological activity can allow us to develop and assess HSP70-based anti-cancer therapies with greater clinical efficacies.
Author contributions
BH: Writing – original draft, Investigation, Funding acquisition, Conceptualization. GL: Writing – original draft, Software, Conceptualization. KZ: Writing – review & editing, Supervision. GZ: Writing – review & editing, Validation, Supervision.
Funding
The author(s) declare financial support was received for the research, authorship, and/or publication of this article. This work was supported by the Science and Technology Department of Sichuan Province (grant number 2023NSFSC0713).
Acknowledgments
The authors would like to express their gratitude to EditSprings (https://www.editsprings.cn) for the expert linguistic services provided.
Conflict of interest
The authors declare that the research was conducted in the absence of any commercial or financial relationships that could be construed as a potential conflict of interest.
Publisher’s note
All claims expressed in this article are solely those of the authors and do not necessarily represent those of their affiliated organizations, or those of the publisher, the editors and the reviewers. Any product that may be evaluated in this article, or claim that may be made by its manufacturer, is not guaranteed or endorsed by the publisher.
References
1. Radons J. The human hsp70 family of chaperones: where do we stand? Cell Stress chaperones. (2016) 21:379–404. doi: 10.1007/s12192-016-0676-6
2. Saibil H. Chaperone machines for protein folding, unfolding and disaggregation. Nat Rev Mol Cell Biol. (2013) 14:630–42. doi: 10.1038/nrm3658
3. Rosenzweig R, Nillegoda NB, Mayer MP, Bukau B. The hsp70 chaperone network. Nat Rev Mol Cell Biol. (2019) 20:665–80. doi: 10.1038/s41580-019-0133-3
4. Albakova Z, Armeev GA, Kanevskiy LM, Kovalenko EI, Sapozhnikov AM. Hsp70 multi-functionality in cancer. Cells. (2020) 9:587. doi: 10.3390/cells9030587
5. Vostakolaei MA, Hatami-Baroogh L, Babaei G, Molavi O, Kordi S, Abdolalizadeh J. Hsp70 in cancer: A double agent in the battle between survival and death. J Cell Physiol. (2021) 236:3420–44. doi: 10.1002/jcp.30132
6. Arispe N, Doh M, Simakova O, Kurganov B, De Maio A. Hsc70 and hsp70 interact with phosphatidylserine on the surface of pc12 cells resulting in a decrease of viability. FASEB J. (2004) 18:1636–45. doi: 10.1096/fj.04-2088com
7. Schilling D, Gehrmann M, Steinem C, De Maio A, Pockley AG, Abend M, et al. Binding of heat shock protein 70 to extracellular phosphatidylserine promotes killing of normoxic and hypoxic tumor cells. FASEB J. (2009) 23:2467–77. doi: 10.1096/fj.08-125229
8. Armijo G, Okerblom J, Cauvi DM, Lopez V, Schlamadinger DE, Kim J, et al. Interaction of heat shock protein 70 with membranes depends on the lipid environment. Cell Stress chaperones. (2014) 19:877–86. doi: 10.1007/s12192-014-0511-x
9. Bilog AD, Smulders L, Oliverio R, Labanieh C, Zapanta J, Stahelin RV, et al. Membrane localization of hspa1a, a stress inducible 70-kda heat-shock protein, depends on its interaction with intracellular phosphatidylserine. Biomolecules. (2019) 9:152. doi: 10.3390/biom9040152
10. Vega VL, Rodríguez-Silva M, Frey T, Gehrmann M, Diaz JC, Steinem C, et al. Hsp70 Translocates into the Plasma Membrane after Stress and Is Released into the Extracellular Environment in a Membrane-Associated Form That Activates Macrophages. J Immunol. (2008) 180:4299–307. doi: 10.4049/jimmunol.180.6.4299
11. Broquet AH, Thomas G, Masliah J, Trugnan G, Bachelet M. Expression of the molecular chaperone hsp70 in detergent-resistant microdomains correlates with its membrane delivery and release. J Biol Chem. (2003) 278:21601–6. doi: 10.1074/jbc.M302326200
12. Gehrmann M, Liebisch G, Schmitz G, Anderson R, Steinem C, De Maio A, et al. Tumor-specific hsp70 plasma membrane localization is enabled by the glycosphingolipid gb3. PloS One. (2008) 3:e1925. doi: 10.1371/journal.pone.0001925
13. Mamelak D, Mylvaganam M, Whetstone H, Hartmann E, Lennarz W, Wyrick PB, et al. Hsp70s contain a specific sulfogalactolipid binding site. Differential aglycone influence on sulfogalactosyl ceramide binding by recombinant prokaryotic and eukaryotic hsp70 family members. Biochemistry. (2001) 40:3572–82. doi: 10.1021/bi001643u
14. Mambula SS, Stevenson MA, Ogawa K, Calderwood SK. Mechanisms for hsp70 secretion: crossing membranes without a leader. Methods. (2007) 43:168–75. doi: 10.1016/j.ymeth.2007.06.009
15. Evdonin AL, Martynova MG, Bystrova OA, Guzhova IV, Margulis BA, Medvedeva ND. The release of hsp70 from A431 carcinoma cells is mediated by secretory-like granules. Eur J Cell Biol. (2006) 85:443–55. doi: 10.1016/j.ejcb.2006.02.008
16. Mambula SS, Calderwood SK. Heat shock protein 70 is secreted from tumor cells by a nonclassical pathway involving lysosomal endosomes. J Immunol. (2006) 177:7849–57. doi: 10.4049/jimmunol.177.11.7849
17. Pilzer D, Gasser O, Moskovich O, Schifferli JA, Fishelson Z. Emission of membrane vesicles: roles in complement resistance, immunity and cancer. Springer Semin Immunopathol. (2005) 27:375–87. doi: 10.1007/s00281-005-0004-1
18. Kahroba H, Hejazi MS, Samadi N. Exosomes: from carcinogenesis and metastasis to diagnosis and treatment of gastric cancer. Cell Mol Life Sci CMLS. (2019) 76:1747–58. doi: 10.1007/s00018-019-03035-2
19. Lancaster GI, Febbraio MA. Exosome-dependent trafficking of hsp70: A novel secretory pathway for cellular stress proteins. J Biol Chem. (2005) 280:23349–55. doi: 10.1074/jbc.M502017200
20. Li Z, Zhuang M, Zhang L, Zheng X, Yang P, Li Z. Acetylation modification regulates grp78 secretion in colon cancer cells. Sci Rep. (2016) 6:30406. doi: 10.1038/srep30406
21. Yang Y, Fiskus W, Yong B, Atadja P, Takahashi Y, Pandita TK, et al. Acetylated hsp70 and kap1-mediated vps34 sumoylation is required for autophagosome creation in autophagy. Proc Natl Acad Sci U.S.A. (2013) 110:6841–6. doi: 10.1073/pnas.1217692110
22. Fang Y, Wu N, Gan X, Yan W, Morrell JC, Gould SJ. Higher-order oligomerization targets plasma membrane proteins and hiv gag to exosomes. PloS Biol. (2007) 5:e158. doi: 10.1371/journal.pbio.0050158
23. Nimmervoll B, Chtcheglova LA, Juhasz K, Cremades N, Aprile FA, Sonnleitner A, et al. Cell surface localised hsp70 is a cancer specific regulator of clathrin-independent endocytosis. FEBS Lett. (2015) 589:2747–53. doi: 10.1016/j.febslet.2015.07.037
24. Chanteloup G, Cordonnier M, Isambert N, Bertaut A, Hervieu A, Hennequin A, et al. Monitoring hsp70 exosomes in cancer patients' Follow up: A clinical prospective pilot study. J Extracell Vesicles. (2020) 9:1766192. doi: 10.1080/20013078.2020.1766192
25. Safi S, Messner L, Kliebisch M, Eggert L, Ceylangil C, Lennartz P, et al. Circulating hsp70 levels and the immunophenotype of peripheral blood lymphocytes as potential biomarkers for advanced lung cancer and therapy failure after surgery. Biomolecules. (2023) 13:874. doi: 10.3390/biom13050874
26. Tang T, Yang C, Brown HE, Huang J. Circulating heat shock protein 70 is a novel biomarker for early diagnosis of lung cancer. Dis Markers. (2018) 2018:6184162. doi: 10.1155/2018/6184162
27. Gobbo J, Marcion G, Cordonnier M, Dias AMM, Pernet N, Hammann A, et al. Restoring anticancer immune response by targeting tumor-derived exosomes with a hsp70 peptide aptamer. J Natl Cancer Inst. (2016) 108. doi: 10.1093/jnci/djv330
28. Hantschel M, Pfister K, Jordan A, Scholz R, Andreesen R, Schmitz G, et al. Hsp70 plasma membrane expression on primary tumor biopsy material and bone marrow of leukemic patients. Cell Stress chaperones. (2000) 5:438–42. doi: 10.1379/1466-1268(2000)005<0438:hpmeop>2.0.co;2
29. Huang F, Li X, Zhao N, Duan L, Chen Y. Circulating grp78 acts as a biomarker in the early diagnosis of lung cancer. Int J Clin Exp Pathol. (2018) 11:5223–31.
30. Werner C, Stangl S, Salvermoser L, Schwab M, Shevtsov M, Xanthopoulos A, et al. Hsp70 in liquid biopsies-a tumor-specific biomarker for detection and response monitoring in cancer. Cancers. (2021) 13:3706. doi: 10.3390/cancers13153706
31. Gunther S, Ostheimer C, Stangl S, Specht HM, Mozes P, Jesinghaus M, et al. Correlation of hsp70 serum levels with gross tumor volume and composition of lymphocyte subpopulations in patients with squamous cell and adeno non-small cell lung cancer. Front Immunol. (2015) 6:556. doi: 10.3389/fimmu.2015.00556
32. Seier S, Bashiri Dezfouli A, Lennartz P, Pockley AG, Klein H, Multhoff G. Elevated levels of circulating hsp70 and an increased prevalence of cd94+/cd69+ Nk cells is predictive for advanced stage non-small cell lung cancer. Cancers. (2022) 14:5701. doi: 10.3390/cancers14225701
33. Ostheimer C, Gunther S, Bache M, Vordermark D, Multhoff G. Dynamics of heat shock protein 70 serum levels as a predictor of clinical response in non-small-cell lung cancer and correlation with the hypoxia-related marker osteopontin. Front Immunol. (2017) 8:1305. doi: 10.3389/fimmu.2017.01305
34. Zimmermann M, Nickl S, Lambers C, Hacker S, Mitterbauer A, Hoetzenecker K, et al. Discrimination of clinical stages in non-small cell lung cancer patients by serum hsp27 and hsp70: A multi-institutional case-control study. Clin Chim Acta. (2012) 413:1115–20. doi: 10.1016/j.cca.2012.03.008
35. Ma X, Guo W, Yang S, Zhu X, Xiang J, Li H. Serum grp78 as a tumor marker and its prognostic significance in non-small cell lung cancers: A retrospective study. Dis Markers. (2015) 2015:814670. doi: 10.1155/2015/814670
36. Zhong L, Peng X, Hidalgo GE, Doherty DE, Stromberg AJ, Hirschowitz EA. Antibodies to hsp70 and hsp90 in serum in non-small cell lung cancer patients. Cancer Detect Prev. (2003) 27:285–90. doi: 10.1016/s0361-090x(03)00097-7
37. Balázs M, Zsolt H, László G, Gabriella G, Lilla T, Gyula O, et al. Serum heat shock protein 70, as a potential biomarker for small cell lung cancer. Pathol Oncol Res. (2017) 23:377–83. doi: 10.1007/s12253-016-0118-x
38. Fujita Y, Nakanishi T, Miyamoto Y, Hiramatsu M, Mabuchi H, Miyamoto A, et al. Proteomics-based identification of autoantibody against heat shock protein 70 as a diagnostic marker in esophageal squamous cell carcinoma. Cancer Lett. (2008) 263:280–90. doi: 10.1016/j.canlet.2008.01.013
39. Xanthopoulos A, Samt AK, Guder C, Taylor N, Roberts E, Herf H, et al. Hsp70-a universal biomarker for predicting therapeutic failure in human female cancers and a target for ctc isolation in advanced cancers. Biomedicines. (2023) 11:2276. doi: 10.3390/biomedicines11082276
40. Fahim HH, Mohamed G, Safwat G, Abo-Bakr A, Ibraheem MH, Al-Mofty S, et al. Hsp70 as a diagnostic and prognostic marker in Egyptian women with breast cancer. Clin Breast Cancer. (2021) 21:e177–e88. doi: 10.1016/j.clbc.2020.11.005
41. Gunaldi M, Afsar CU, Okuturlar Y, Gedikbasi A, Kocoglu H, Kural A, et al. Elevated serum levels of heat shock protein 70 are associated with breast cancer. Tohoku J Exp Med. (2015) 236:97–102. doi: 10.1620/tjem.236.97
42. Rothammer A, Sage EK, Werner C, Combs SE, Multhoff G. Increased heat shock protein 70 (Hsp70) serum levels and low nk cell counts after radiotherapy - potential markers for predicting breast cancer recurrence? Radiat Oncol. (2019) 14:78. doi: 10.1186/s13014-019-1286-0
43. de Freitas GB, Penteado L, Miranda MM, Filassi JR, Baracat EC, Linhares IM. The circulating 70 kda heat shock protein (Hspa1a) level is a potential biomarker for breast carcinoma and its progression. Sci Rep. (2022) 12:13012. doi: 10.1038/s41598-022-17414-6
44. Yerushalmi R, Raiter A, Nalbandyan K, Hardy B. Cell surface grp78: A potential marker of good prognosis and response to chemotherapy in breast cancer. Oncol Lett. (2015) 10:2149–55. doi: 10.3892/ol.2015.3579
45. Gunaldi M, Kocoglu H, Okuturlar Y, Gedikbasi A, Karabulut M, Alis H, et al. Heat shock protein 70 is a useful marker for predicting colorectal cancer. J buon. (2015) 20:1464–70.
46. Gráf L, Barabás L, Madaras B, Garam N, Maláti É, Horváth L, et al. High serum hsp70 level predicts poor survival in colorectal cancer: results obtained in an independent validation cohort. Cancer Biomarkers section A Dis Markers. (2018) 23:539–47. doi: 10.3233/cbm-181683
47. Jubran R, Kocsis J, Garam N, Maláti É, Gombos T, Barabás L, et al. Circulating mitochondrial stress 70 protein/mortalin and cytosolic hsp70 in blood: risk indicators in colorectal cancer. Int J Cancer. (2017) 141:2329–35. doi: 10.1002/ijc.30918
48. Kocsis J, Madaras B, Tóth EK, Füst G, Prohászka Z. Serum level of soluble 70-kd heat shock protein is associated with high mortality in patients with colorectal cancer without distant metastasis. Cell Stress chaperones. (2010) 15:143–51. doi: 10.1007/s12192-009-0128-7
49. Pfister K, Radons J, Busch R, Tidball JG, Pfeifer M, Freitag L, et al. Patient survival by hsp70 membrane phenotype: association with different routes of metastasis. Cancer. (2007) 110:926–35. doi: 10.1002/cncr.22864
50. Guan M, Chen X, Ma Y, Tang L, Guan L, Ren X, et al. Mda-9 and grp78 as potential diagnostic biomarkers for early detection of melanoma metastasis. Tumour Biol. (2015) 36:2973–82. doi: 10.1007/s13277-014-2930-9
51. Raiter A, Vilkin A, Gingold R, Levi Z, Halpern M, Niv Y, et al. The presence of anti-grp78 antibodies in the serum of patients with colorectal carcinoma: A potential biomarker for early cancer detection. Int J Biol Markers. (2014) 29:e431–5. doi: 10.5301/jbm.5000086
52. Qin J, Yang Q, Ye H, Wang K, Zhang M, Zhu J, et al. Using serological proteome analysis to identify and evaluate anti-grp78 autoantibody as biomarker in the detection of gastric cancer. J Oncol. (2020) 2020:9430737. doi: 10.1155/2020/9430737
53. Piszcz J, Bolkun Ł, Cichocka E, Galar M, Hołownia A, Kłoczko J. Prognostic relevance of hsp70 antigen and antibody measurement in patients with acute myeloid leukemia of intermediate and unfavorable cytogenetic risk. Pol Arch Med Wewn. (2014) 124:165–72. doi: 10.20452/pamw.2184
54. Gehrmann M, Cervello M, Montalto G, Cappello F, Gulino A, Knape C, et al. Heat shock protein 70 serum levels differ significantly in patients with chronic hepatitis, liver cirrhosis, and hepatocellular carcinoma. Front Immunol. (2014) 5:307. doi: 10.3389/fimmu.2014.00307
55. Ying X, Han SX, He CC, Zhou CY, Dong YP, Cai MJ, et al. Autoantibodies against glucose-regulated protein 78 as serological biomarkers in metastatic and recurrent hepatocellular carcinoma. Oncotarget. (2017) 8:24828–39. doi: 10.18632/oncotarget.15192
56. Shao Q, Ren P, Li Y, Peng B, Dai L, Lei N, et al. Autoantibodies against glucose-regulated protein 78 as serological diagnostic biomarkers in hepatocellular carcinoma. Int J Oncol. (2012) 41:1061–7. doi: 10.3892/ijo.2012.1515
57. Rucksaken R, Pairojkul C, Pinlaor P, Khuntikeo N, Roytrakul S, Selmi C, et al. Plasma autoantibodies against heat shock protein 70, enolase 1 and ribonuclease/angiogenin inhibitor 1 as potential biomarkers for cholangiocarcinoma. PloS One. (2014) 9:e103259. doi: 10.1371/journal.pone.0103259
58. Dutta SK, Girotra M, Singla M, Dutta A, Otis Stephen F, Nair PP, et al. Serum hsp70: A novel biomarker for early detection of pancreatic cancer. Pancreas. (2012) 41:530–4. doi: 10.1097/MPA.0b013e3182374ace
59. Abe M, Manola JB, Oh WK, Parslow DL, George DJ, Austin CL, et al. Plasma levels of heat shock protein 70 in patients with prostate cancer: A potential biomarker for prostate cancer. Clin Prostate Cancer. (2004) 3:49–53. doi: 10.3816/cgc.2004.n.013
60. Paris EA, Bahr JM, Abramowicz JS, Basu S, Barua A. Glucose-regulated protein 78 is a potential serum and imaging marker for early detection of ovarian cancer. Cancers. (2023) 15:1140. doi: 10.3390/cancers15041140
61. Ciortea R, Berceanu C, Măluţan AM, Mocan R, Iuhas C, Bucuri CE, et al. Intraperitoneal fat through grp78: A risk factor for endometrial cancer. Anal Cell Pathol (Amst). (2016) 2016:3496538. doi: 10.1155/2016/3496538
62. Kumar M, Garg H, Gupta N, Sharma A, Kaushal S, Kumar R, et al. Glucose- regulated protein 78 (Grp78) in renal cell carcinoma: A novel biomarker for predicting tumor behavior. Heliyon. (2021) 7:e07300. doi: 10.1016/j.heliyon.2021.e07300
63. Gehrmann M, Specht HM, Bayer C, Brandstetter M, Chizzali B, Duma M, et al. Hsp70–a biomarker for tumor detection and monitoring of outcome of radiation therapy in patients with squamous cell carcinoma of the head and neck. Radiat Oncol. (2014) 9:131. doi: 10.1186/1748-717x-9-131
64. Lennartz P, Thölke D, Bashiri Dezfouli A, Pilz M, Lobinger D, Messner V, et al. Biomarkers in adult-type diffuse gliomas: elevated levels of circulating vesicular heat shock protein 70 serve as a biomarker in grade 4 glioblastoma and increase nk cell frequencies in grade 3 glioma. Biomedicines. (2023) 11:3235. doi: 10.3390/biomedicines11123235
65. Lobinger D, Gempt J, Sievert W, Barz M, Schmitt S, Nguyen HT, et al. Potential role of hsp70 and activated nk cells for prediction of prognosis in glioblastoma patients. Front Mol Biosci. (2021) 8:669366. doi: 10.3389/fmolb.2021.669366
66. Liao Q, Zhao L, Chen X, Deng Y, Ding Y. Serum proteome analysis for profiling protein markers associated with carcinogenesis and lymph node metastasis in nasopharyngeal carcinoma. Clin Exp Metastasis. (2008) 25:465–76. doi: 10.1007/s10585-008-9152-8
67. Tong YQ, Zhang ZJ, Liu B, Huang J, Liu H, Liu Y, et al. Autoantibodies as potential biomarkers for nasopharyngeal carcinoma. Proteomics. (2008) 8:3185–93. doi: 10.1002/pmic.200700651
68. Yeh CH, Tseng R, Hannah A, Estrov Z, Estey E, Kantarjian H, et al. Clinical correlation of circulating heat shock protein 70 in acute leukemia. Leuk Res. (2010) 34:605–9. doi: 10.1016/j.leukres.2009.09.014
69. Pranay A, Shukla S, Kannan S, Malgundkar SA, Govekar RB, Patil A, et al. Prognostic utility of autoantibodies to Α-enolase and hsp70 for cancer of the gingivo-buccal complex using immunoproteomics. Proteomics Clin Appl. (2013) 7:392–402. doi: 10.1002/prca.201200081
70. Tsunemi S, Nakanishi T, Fujita Y, Bouras G, Miyamoto Y, Miyamoto A, et al. Proteomics-based identification of a tumor-associated antigen and its corresponding autoantibody in gastric cancer. Oncol Rep. (2010) 23:949–56. doi: 10.3892/or_00000719
71. Ramirez MI, Amorim MG, Gadelha C, Milic I, Welsh JA, Freitas VM, et al. Technical challenges of working with extracellular vesicles. Nanoscale. (2018) 10:881–906. doi: 10.1039/C7NR08360B
72. Fu R, Yang P, Wu HL, Li ZW, Li ZY. Grp78 secreted by colon cancer cells facilitates cell proliferation via pi3k/akt signaling. Asian Pac J Cancer Prev. (2014) 15:7245–9. doi: 10.7314/apjcp.2014.15.17.7245
73. Wu FH, Yuan Y, Li D, Liao SJ, Yan B, Wei JJ, et al. Extracellular hspa1a promotes the growth of hepatocarcinoma by augmenting tumor cell proliferation and apoptosis-resistance. Cancer Lett. (2012) 317:157–64. doi: 10.1016/j.canlet.2011.11.020
74. Nigro A, Mauro L, Giordano F, Panza S, Iannacone R, Liuzzi GM, et al. Recombinant arabidopsis hsp70 sustains cell survival and metastatic potential of breast cancer cells. Mol Cancer Ther. (2016) 15:1063–73. doi: 10.1158/1535-7163.Mct-15-0830
75. Kang BR, Yang SH, Chung BR, Kim W, Kim Y. Cell surface grp78 as a biomarker and target for suppressing glioma cells. Sci Rep. (2016) 6:34922. doi: 10.1038/srep34922
76. Calì G, Insabato L, Conza D, Bifulco G, Parrillo L, Mirra P, et al. Grp78 mediates cell growth and invasiveness in endometrial cancer. J Cell Physiol. (2014) 229:1417–26. doi: 10.1002/jcp.24578
77. Takeda K, Kaisho T, Akira S. Toll-like receptors. Annu Rev Immunol. (2003) 21:335–76. doi: 10.1146/annurev.immunol.21.120601.141126
78. Adewumi O, Aflatoonian B, Ahrlund-Richter L, Amit M, Andrews PW, Beighton G, et al. Characterization of human embryonic stem cell lines by the international stem cell initiative. Nat Biotechnol. (2007) 25:803–16. doi: 10.1038/nbt1318
79. Kelber JA, Panopoulos AD, Shani G, Booker EC, Belmonte JC, Vale WW, et al. Blockade of cripto binding to cell surface grp78 inhibits oncogenic cripto signaling via mapk/pi3k and smad2/3 pathways. Oncogene. (2009) 28:2324–36. doi: 10.1038/onc.2009.97
80. Shani G, Fischer WH, Justice NJ, Kelber JA, Vale W, Gray PC. Grp78 and cripto form a complex at the cell surface and collaborate to inhibit transforming growth factor beta signaling and enhance cell growth. Mol Cell Biol. (2008) 28:666–77. doi: 10.1128/mcb.01716-07
81. Thakur G, Sathe G, Kundu I, Biswas B, Gautam P, Alkahtani S, et al. Membrane interactome of a recombinant fragment of human surfactant protein D reveals grp78 as a novel binding partner in pc3, a metastatic prostate cancer cell line. Front Immunol. (2020) 11:600660. doi: 10.3389/fimmu.2020.600660
82. Kim C, Lee S, Kim D, Lee DS, Lee E, Yoo C, et al. Blockade of grp78 translocation to the cell surface by hdac6 inhibition suppresses proliferation of cholangiocarcinoma cells. Anticancer Res. (2022) 42:471–82. doi: 10.21873/anticanres.15505
83. Yin Y, Chen C, Chen J, Zhan R, Zhang Q, Xu X, et al. Cell surface grp78 facilitates hepatoma cells proliferation and migration by activating igf-ir. Cell Signal. (2017) 35:154–62. doi: 10.1016/j.cellsig.2017.04.003
84. Lester BR, McCarthy JB. Tumor cell adhesion to the extracellular matrix and signal transduction mechanisms implicated in tumor cell motility, invasion and metastasis. Cancer Metastasis Rev. (1992) 11:31–44. doi: 10.1007/bf00047601
85. Park J, Kim DH, Shah SR, Kim HN, Kshitiz, Kim P, et al. Switch-like enhancement of epithelial-mesenchymal transition by yap through feedback regulation of wt1 and rho-family gtpases. Nat Commun. (2019) 10:2797. doi: 10.1038/s41467-019-10729-5
86. Li Z, Zhang L, Zhao Y, Li H, Xiao H, Fu R, et al. Cell-surface grp78 facilitates colorectal cancer cell migration and invasion. Int J Biochem Cell Biol. (2013) 45:987–94. doi: 10.1016/j.biocel.2013.02.002
87. Tsuneki M, Maruyama S, Yamazaki M, Xu B, Essa A, Abé T, et al. Extracellular heat shock protein A9 is a novel interaction partner of podoplanin in oral squamous cell carcinoma cells. Biochem Biophys Res Commun. (2013) 434:124–30. doi: 10.1016/j.bbrc.2013.03.057
88. Li H, Li Y, Liu D, Sun H, Su D, Yang F, et al. Extracellular hsp70/hsp70-pcs promote epithelial-mesenchymal transition of hepatocarcinoma cells. PloS One. (2013) 8:e84759. doi: 10.1371/journal.pone.0084759
89. Zhang XX, Li HD, Zhao S, Zhao L, Song HJ, Wang G, et al. The cell surface grp78 facilitates the invasion of hepatocellular carcinoma cells. BioMed Res Int. (2013) 2013:917296. doi: 10.1155/2013/917296
90. Misra UK, Pizzo SV. Receptor-recognized Α2-macroglobulin binds to cell surface-associated grp78 and activates mtorc1 and mtorc2 signaling in prostate cancer cells. PloS One. (2012) 7:e51735. doi: 10.1371/journal.pone.0051735
91. Zhao S, Li H, Wang Q, Su C, Wang G, Song H, et al. The role of C-src in the invasion and metastasis of hepatocellular carcinoma cells induced by association of cell surface grp78 with activated Α2m. BMC Cancer. (2015) 15:389. doi: 10.1186/s12885-015-1401-z
92. Sahai E, Astsaturov I, Cukierman E, DeNardo DG, Egeblad M, Evans RM, et al. A framework for advancing our understanding of cancer-associated fibroblasts. Nat Rev Cancer. (2020) 20:174–86. doi: 10.1038/s41568-019-0238-1
93. Peng Y, Li Z, Li Z. Grp78 secreted by tumor cells stimulates differentiation of bone marrow mesenchymal stem cells to cancer-associated fibroblasts. Biochem Biophys Res Commun. (2013) 440:558–63. doi: 10.1016/j.bbrc.2013.09.108
94. Sims JD, McCready J, Jay DG. Extracellular heat shock protein (Hsp)70 and hsp90α Assist in matrix metalloproteinase-2 activation and breast cancer cell migration and invasion. PloS One. (2011) 6:e18848. doi: 10.1371/journal.pone.0018848
95. De Palma M, Biziato D, Petrova TV. Microenvironmental regulation of tumour angiogenesis. Nat Rev Cancer. (2017) 17:457–74. doi: 10.1038/nrc.2017.51
96. Dong D, Ni M, Li J, Xiong S, Ye W, Virrey JJ, et al. Critical role of the stress chaperone grp78/bip in tumor proliferation, survival, and tumor angiogenesis in transgene-induced mammary tumor development. Cancer Res. (2008) 68:498–505. doi: 10.1158/0008-5472.Can-07-2950
97. Dong D, Stapleton C, Luo B, Xiong S, Ye W, Zhang Y, et al. A critical role for grp78/bip in the tumor microenvironment for neovascularization during tumor growth and metastasis. Cancer Res. (2011) 71:2848–57. doi: 10.1158/0008-5472.Can-10-3151
98. Iha K, Sato A, Tsai HY, Sonoda H, Watabe S, Yoshimura T, et al. Gastric cancer cell-derived exosomal grp78 enhances angiogenesis upon stimulation of vascular endothelial cells. Curr Issues Mol Biol. (2022) 44:6145–57. doi: 10.3390/cimb44120419
99. Katanasaka Y, Ishii T, Asai T, Naitou H, Maeda N, Koizumi F, et al. Cancer antineovascular therapy with liposome drug delivery systems targeted to bip/grp78. Int J Cancer. (2010) 127:2685–98. doi: 10.1002/ijc.25276
100. Al-Keilani M, Alqudah MA, Almomani B, Alrjoub MM, Shhabat BA, Alzoubi K. Grp78 is overexpressed in non-small cell lung cancer tissues and is associated with high vegf expression in squamous cell carcinoma: A pilot study. Curr Cancer Drug Targets. (2023) 23:805–16. doi: 10.2174/1568009623666230418111020
101. Zhai LL, Xie Q, Zhou CH, Huang DW, Tang ZG, Ju TF. Overexpressed hspa2 correlates with tumor angiogenesis and unfavorable prognosis in pancreatic carcinoma. Pancreatology. (2017) 17:457–63. doi: 10.1016/j.pan.2017.04.007
102. Asea A, Kraeft SK, Kurt-Jones EA, Stevenson MA, Chen LB, Finberg RW, et al. Hsp70 stimulates cytokine production through a cd14-dependant pathway, demonstrating its dual role as a chaperone and cytokine. Nat Med. (2000) 6:435–42. doi: 10.1038/74697
103. Asea A, Rehli M, Kabingu E, Boch JA, Bare O, Auron PE, et al. Novel signal transduction pathway utilized by extracellular hsp70: role of toll-like receptor (Tlr) 2 and tlr4. J Biol Chem. (2002) 277:15028–34. doi: 10.1074/jbc.M200497200
104. Becker T, Hartl FU, Wieland F. Cd40, an extracellular receptor for binding and uptake of hsp70-peptide complexes. J Cell Biol. (2002) 158:1277–85. doi: 10.1083/jcb.200208083
105. Ogbodo E, Michelangeli F, Williams JHH. Exogenous heat shock proteins hspa1a and hspb1 regulate tnf-Α, il-1β and il-10 secretion from monocytic cells. FEBS Open Bio. (2023) 13:1922–40. doi: 10.1002/2211-5463.13695
106. Diao J, Yang X, Song X, Chen S, He Y, Wang Q, et al. Exosomal hsp70 mediates immunosuppressive activity of the myeloid-derived suppressor cells via phosphorylation of stat3. Med Oncol. (2015) 32:453. doi: 10.1007/s12032-014-0453-2
107. Aggarwal V, Tuli HS, Varol A, Thakral F, Yerer MB, Sak K, et al. Role of reactive oxygen species in cancer progression: molecular mechanisms and recent advancements. Biomolecules. (2019) 9:735. doi: 10.3390/biom9110735
108. Klink M, Nowak M, Kielbik M, Bednarska K, Blus E, Szpakowski M, et al. The interaction of hspa1a with tlr2 and tlr4 in the response of neutrophils induced by ovarian cancer cells in vitro. Cell Stress chaperones. (2012) 17:661–74. doi: 10.1007/s12192-012-0338-2
109. Tajbakhsh A, Gheibi Hayat SM, Movahedpour A, Savardashtaki A, Loveless R, Barreto GE, et al. The complex roles of efferocytosis in cancer development, metastasis, and treatment. Biomedicine pharmacotherapy = Biomedecine pharmacotherapie. (2021) 140:111776. doi: 10.1016/j.biopha.2021.111776
110. Sun Y, Xiao W, Yu Y, Jiang Y, Xiao Z, Huang D, et al. Colorectal cancer-derived extracellular vesicles containing hsp70 enhance macrophage phagocytosis by up-regulating marco expression. Exp Cell Res. (2023) 426:113565. doi: 10.1016/j.yexcr.2023.113565
111. Tian J, Zhang L, La X, Fan X, Li A, Wu C, et al. Tumor-secreted grp78 induces M2 polarization of macrophages by promoting lipid catabolism. Cell Signal. (2023) 108:110719. doi: 10.1016/j.cellsig.2023.110719
112. Multhoff G, Pfister K, Gehrmann M, Hantschel M, Gross C, Hafner M, et al. A 14-mer hsp70 peptide stimulates natural killer (Nk) cell activity. Cell Stress chaperones. (2001) 6:337–44. doi: 10.1379/1466-1268(2001)006<0337:Amhpsn>2.0.Co;2
113. Gastpar R, Gehrmann M, Bausero MA, Asea A, Gross C, Schroeder JA, et al. Heat shock protein 70 surface-positive tumor exosomes stimulate migratory and cytolytic activity of natural killer cells. Cancer Res. (2005) 65:5238–47. doi: 10.1158/0008-5472.Can-04-3804
114. Komarova EY, Suezov RV, Nikotina AD, Aksenov ND, Garaeva LA, Shtam TA, et al. Hsp70-containing extracellular vesicles are capable of activating of adaptive immunity in models of mouse melanoma and colon carcinoma. Sci Rep. (2021) 11:21314. doi: 10.1038/s41598-021-00734-4
115. Gross C, Koelch W, DeMaio A, Arispe N, Multhoff G. Cell surface-bound heat shock protein 70 (Hsp70) mediates perforin-independent apoptosis by specific binding and uptake of granzyme B. J Biol Chem. (2003) 278:41173–81. doi: 10.1074/jbc.M302644200
116. Multhoff G, Botzler C, Jennen L, Schmidt J, Ellwart J, Issels R. Heat shock protein 72 on tumor cells: A recognition structure for natural killer cells. J Immunol. (1997) 158:4341–50. doi: 10.4049/jimmunol.158.9.4341
117. Gross C, Hansch D, Gastpar R, Multhoff G. Interaction of heat shock protein 70 peptide with nk cells involves the nk receptor cd94. Biol Chem. (2003) 384:267–79. doi: 10.1515/bc.2003.030
118. Specht HM, Ahrens N, Blankenstein C, Duell T, Fietkau R, Gaipl US, et al. Heat shock protein 70 (Hsp70) peptide activated natural killer (Nk) cells for the treatment of patients with non-small cell lung cancer (Nsclc) after radiochemotherapy (Rctx) - from preclinical studies to a clinical phase ii trial. Front Immunol. (2015) 6:162. doi: 10.3389/fimmu.2015.00162
119. Qiao Y, Liu B, Li Z. Activation of nk cells by extracellular heat shock protein 70 through induction of nkg2d ligands on dendritic cells. Cancer Immun. (2008) 8:12.
120. Elsner L, Flügge PF, Lozano J, Muppala V, Eiz-Vesper B, Demiroglu SY, et al. The endogenous danger signals hsp70 and mica cooperate in the activation of cytotoxic effector functions of nk cells. J Cell Mol Med. (2010) 14:992–1002. doi: 10.1111/j.1582-4934.2008.00677.x
121. Sharapova TN, Romanova EA, Ivanova OK, Yashin DV, Sashchenko LP. Hsp70 interacts with the trem-1 receptor expressed on monocytes and thereby stimulates generation of cytotoxic lymphocytes active against mhc-negative tumor cells. Int J Mol Sci. (2021) 22:6889. doi: 10.3390/ijms22136889
122. Komarova EY, Marchenko LV, Zhakhov AV, Nikotina AD, Aksenov ND, Suezov RV, et al. Extracellular hsp70 reduces the pro-tumor capacity of monocytes/macrophages co-cultivated with cancer cells. Int J Mol Sci. (2019) 21:59. doi: 10.3390/ijms21010059
123. Wang Y, Whittall T, McGowan E, Younson J, Kelly C, Bergmeier LA, et al. Identification of stimulating and inhibitory epitopes within the heat shock protein 70 molecule that modulate cytokine production and maturation of dendritic cells. J Immunol. (2005) 174:3306–16. doi: 10.4049/jimmunol.174.6.3306
124. Chen T, Guo J, Han C, Yang M, Cao X. Heat shock protein 70, released from heat-stressed tumor cells, initiates antitumor immunity by inducing tumor cell chemokine production and activating dendritic cells via tlr4 pathway. J Immunol. (2009) 182:1449–59. doi: 10.4049/jimmunol.182.3.1449
125. Bendz H, Ruhland SC, Pandya MJ, Hainzl O, Riegelsberger S, Braüchle C, et al. Human Heat Shock Protein 70 Enhances Tumor Antigen Presentation through Complex Formation and Intracellular Antigen Delivery without Innate Immune Signaling. J Biol Chem. (2007) 282:31688–702. doi: 10.1074/jbc.M704129200
126. Noessner E, Gastpar R, Milani V, Brandl A, Hutzler PJ, Kuppner MC, et al. Tumor-derived heat shock protein 70 peptide complexes are cross-presented by human dendritic cells. J Immunol. (2002) 169:5424–32. doi: 10.4049/jimmunol.169.10.5424
127. Yashin DV, Ivanova OK, Soshnikova NV, Sheludchenkov AA, Romanova EA, Dukhanina EA, et al. Tag7 (Pglyrp1) in complex with hsp70 induces alternative cytotoxic processes in tumor cells via tnfr1 receptor. J Biol Chem. (2015) 290:21724–31. doi: 10.1074/jbc.M115.639732
128. Sashchenko LP, Romanova EA, Ivanova OK, Sharapova TN, Yashin DV. Fasl and the nkg2d receptor are required for the secretion of the tag7/pgrp-S-hsp70 complex by the cytotoxic cd8(+) lymphocytes. IUBMB Life. (2017) 69:30–6. doi: 10.1002/iub.1587
129. Figueiredo C, Wittmann M, Wang D, Dressel R, Seltsam A, Blasczyk R, et al. Heat shock protein 70 (Hsp70) induces cytotoxicity of T-helper cells. Blood. (2009) 113:3008–16. doi: 10.1182/blood-2008-06-162727
130. Haug M, Dannecker L, Schepp CP, Kwok WW, Wernet D, Buckner JH, et al. The heat shock protein hsp70 enhances antigen-specific proliferation of human cd4+ Memory T cells. Eur J Immunol. (2005) 35:3163–72. doi: 10.1002/eji.200535050
131. Tobian AA, Canaday DH, Boom WH, Harding CV. Bacterial heat shock proteins promote cd91-dependent class I mhc cross-presentation of chaperoned peptide to cd8+ T cells by cytosolic mechanisms in dendritic cells versus vacuolar mechanisms in macrophages. J Immunol. (2004) 172:5277–86. doi: 10.4049/jimmunol.172.9.5277
132. Fischer N, Haug M, Kwok WW, Kalbacher H, Wernet D, Dannecker GE, et al. Involvement of cd91 and scavenger receptors in hsp70-facilitated activation of human antigen-specific cd4+ Memory T cells. Eur J Immunol. (2010) 40:986–97. doi: 10.1002/eji.200939738
133. Li Z, Menoret A, Srivastava P. Roles of heat-shock proteins in antigen presentation and cross-presentation. Curr Opin Immunol. (2002) 14:45–51. doi: 10.1016/s0952-7915(01)00297-7
134. Martin CA, Carsons SE, Kowalewski R, Bernstein D, Valentino M, Santiago-Schwarz F. Aberrant extracellular and dendritic cell (Dc) surface expression of heat shock protein (Hsp)70 in the rheumatoid joint: possible mechanisms of hsp/dc-mediated cross-priming. J Immunol. (2003) 171:5736–42. doi: 10.4049/jimmunol.171.11.5736
135. Delneste Y, Magistrelli G, Gauchat J, Haeuw J, Aubry J, Nakamura K, et al. Involvement of lox-1 in dendritic cell-mediated antigen cross-presentation. Immunity. (2002) 17:353–62. doi: 10.1016/s1074-7613(02)00388-6
136. Thériault JR, Adachi H, Calderwood SK. Role of scavenger receptors in the binding and internalization of heat shock protein 70. J Immunol. (2006) 177:8604–11. doi: 10.4049/jimmunol.177.12.8604
137. Thériault JR, Mambula SS, Sawamura T, Stevenson MA, Calderwood SK. Extracellular hsp70 binding to surface receptors present on antigen presenting cells and endothelial/epithelial cells. FEBS Lett. (2005) 579:1951–60. doi: 10.1016/j.febslet.2005.02.046
138. Takemoto S, Nishikawa M, Guan X, Ohno Y, Yata T, Takakura Y. Enhanced generation of cytotoxic T lymphocytes by heat shock protein 70 fusion proteins harboring both cd8(+) T cell and cd4(+) T cell epitopes. Mol Pharm. (2010) 7:1715–23. doi: 10.1021/mp1001069
139. Rohrer KM, Haug M, Schwörer D, Kalbacher H, Holzer U. Mutations in the substrate binding site of human heat-shock protein 70 indicate specific interaction with hla-dr outside the peptide binding groove. Immunology. (2014) 142:237–47. doi: 10.1111/imm.12249
140. Bausinger H, Lipsker D, Ziylan U, Manié S, Briand JP, Cazenave JP, et al. Endotoxin-free heat-shock protein 70 fails to induce apc activation. Eur J Immunol. (2002) 32:3708–13. doi: 10.1002/1521-4141(200212)32:12<3708::Aid-immu3708>3.0.Co;2-c
141. Triantafilou K, Triantafilou M, Ladha S, Mackie A, Dedrick RL, Fernandez N, et al. Fluorescence recovery after photobleaching reveals that lps rapidly transfers from cd14 to hsp70 and hsp90 on the cell membrane. J Cell Sci. (2001) 114:2535–45. doi: 10.1242/jcs.114.13.2535
142. Gao B, Tsan MF. Endotoxin contamination in recombinant human heat shock protein 70 (Hsp70) preparation is responsible for the induction of tumor necrosis factor alpha release by murine macrophages. J Biol Chem. (2003) 278:174–9. doi: 10.1074/jbc.M208742200
143. Ye Z, Gan YH. Flagellin contamination of recombinant heat shock protein 70 is responsible for its activity on T cells. J Biol Chem. (2007) 282:4479–84. doi: 10.1074/jbc.M606802200
144. Kern J, Untergasser G, Zenzmaier C, Sarg B, Gastl G, Gunsilius E, et al. Grp-78 secreted by tumor cells blocks the antiangiogenic activity of bortezomib. Blood. (2009) 114:3960–7. doi: 10.1182/blood-2009-03-209668
145. Tseng CC, Zhang P, Lee AS. The cooh-terminal proline-rich region of grp78 is a key regulator of its cell surface expression and viability of tamoxifen-resistant breast cancer cells. Neoplasia. (2019) 21:837–48. doi: 10.1016/j.neo.2019.05.008
146. Yamaguchi-Tanaka M, Takagi K, Miki Y, Sato A, Iwabuchi E, Miyashita M, et al. The pro-tumorigenic role of chemotherapy-induced extracellular hsp70 from breast cancer cells via intratumoral macrophages. Cancers. (2023) 15:1903. doi: 10.3390/cancers15061903
147. Hu W, Xu Z, Zhu S, Sun W, Wang X, Tan C, et al. Small extracellular vesicle-mediated hsp70 intercellular delivery enhances breast cancer adriamycin resistance. Free Radic Biol Med. (2021) 164:85–95. doi: 10.1016/j.freeradbiomed.2020.12.436
148. Sriratanasak N, Chunhacha P, Ei ZZ, Chanvorachote P. Cisplatin induces senescent lung cancer cell-mediated stemness induction via grp78/akt-dependent mechanism. Biomedicines. (2022) 10:2703. doi: 10.3390/biomedicines10112703
149. Gehrmann M, Marienhagen J, Eichholtz-Wirth H, Fritz E, Ellwart J, Jäättelä M, et al. Dual function of membrane-bound heat shock protein 70 (Hsp70), bag-4, and hsp40: protection against radiation-induced effects and target structure for natural killer cells. Cell Death Differ. (2005) 12:38–51. doi: 10.1038/sj.cdd.4401510
150. Murakami N, Kühnel A, Schmid TE, Ilicic K, Stangl S, Braun IS, et al. Role of membrane hsp70 in radiation sensitivity of tumor cells. Radiat Oncol. (2015) 10:149. doi: 10.1186/s13014-015-0461-1
151. Chen Z, Wang H, Zhang Z, Xu J, Qi Y, Xue H, et al. Cell surface grp78 regulates bace2 via lysosome-dependent manner to maintain mesenchymal phenotype of glioma stem cells. J Exp Clin Cancer Res. (2021) 40:20. doi: 10.1186/s13046-020-01807-4
152. Theivanthiran B, Evans KS, DeVito NC, Plebanek M, Sturdivant M, Wachsmuth LP, et al. A tumor-intrinsic pd-L1/nlrp3 inflammasome signaling pathway drives resistance to anti-pd-1 immunotherapy. J Clin Invest. (2020) 130:2570–86. doi: 10.1172/jci133055
153. Theivanthiran B, Yarla N, Haykal T, Nguyen YV, Cao L, Ferreira M, et al. Tumor-intrinsic nlrp3-hsp70-tlr4 axis drives premetastatic niche development and hyperprogression during anti-pd-1 immunotherapy. Sci Transl Med. (2022) 14:eabq7019. doi: 10.1126/scitranslmed.abq7019
154. Misra UK, Mowery Y, Kaczowka S, Pizzo SV. Ligation of Cancer Cell Surface Grp78 with Antibodies Directed against Its Cooh-Terminal Domain up-Regulates P53 Activity and Promotes Apoptosis. Mol Cancer Ther. (2009) 8:1350–62. doi: 10.1158/1535-7163.Mct-08-0990
155. Misra UK, Pizzo SV. Ligation of cell surface grp78 with antibody directed against the cooh-terminal domain of grp78 suppresses ras/mapk and pi 3-kinase/akt signaling while promoting caspase activation in human prostate cancer cells. Cancer Biol Ther. (2010) 9:142–52. doi: 10.4161/cbt.9.2.10422
156. Gopal U, Mowery Y, Young K, Pizzo SV. Targeting cell surface grp78 enhances pancreatic cancer radiosensitivity through yap/taz protein signaling. J Biol Chem. (2019) 294:13939–52. doi: 10.1074/jbc.RA119.009091
157. de Ridder GG, Ray R, Pizzo SV. A murine monoclonal antibody directed against the carboxyl-terminal domain of grp78 suppresses melanoma growth in mice. Melanoma Res. (2012) 22:225–35. doi: 10.1097/CMR.0b013e32835312fd
158. Liu R, Li X, Gao W, Zhou Y, Wey S, Mitra SK, et al. Monoclonal antibody against cell surface grp78 as a novel agent in suppressing pi3k/akt signaling, tumor growth, and metastasis. Clin Cancer Res an Off J Am Assoc Cancer Res. (2013) 19:6802–11. doi: 10.1158/1078-0432.Ccr-13-1106
159. Al-Hashimi AA, Lebeau P, Majeed F, Polena E, Lhotak Š, Collins CAF, et al. Autoantibodies against the cell surface-associated chaperone grp78 stimulate tumor growth via tissue factor. J Biol Chem. (2017) 292:21180–92. doi: 10.1074/jbc.M117.799908
160. Lin ML, Chen SS, Ng SH. Chm-1 suppresses formation of cell surface-associated grp78-P85α Complexes, inhibiting pi3k-akt signaling and inducing apoptosis of human nasopharyngeal carcinoma cells. Anticancer Res. (2015) 35:5359–68.
161. Shan S, Niu J, Yin R, Shi J, Zhang L, Wu C, et al. Peroxidase from foxtail millet bran exerts anti-colorectal cancer activity via targeting cell-surface grp78 to inactivate stat3 pathway. Acta Pharm Sin B. (2022) 12:1254–70. doi: 10.1016/j.apsb.2021.10.004
162. Chen M, Zhang Y, Yu VC, Chong YS, Yoshioka T, Ge R. Isthmin targets cell-surface grp78 and triggers apoptosis via induction of mitochondrial dysfunction. Cell Death Differ. (2014) 21:797–810. doi: 10.1038/cdd.2014.3
163. Kao C, Chandna R, Ghode A, Dsouza C, Chen M, Larsson A, et al. Proapoptotic cyclic peptide bc71 targets cell-surface grp78 and functions as an anticancer therapeutic in mice. EBioMedicine. (2018) 33:22–32. doi: 10.1016/j.ebiom.2018.06.004
164. Zhang X, Huang Y, Li X, Wang Y, Yuan Y, Li M. Preparation of a new combination nanoemulsion-encapsulated mage1-mage3-magen/hsp70 vaccine and study of its immunotherapeutic effect. Pathol Res Pract. (2020) 216:152954. doi: 10.1016/j.prp.2020.152954
165. Long P, Zhang Q, Xue M, Cao G, Li C, Chen W, et al. Tomato lectin-modified nanoemulsion-encapsulated mage1-hsp70/sea complex protein vaccine: targeting intestinal M cells following peroral administration. Biomedicine pharmacotherapy = Biomedecine pharmacotherapie. (2019) 115:108886. doi: 10.1016/j.biopha.2019.108886
166. Ge W, Li Y, Li ZS, Zhang SH, Sun YJ, Hu PZ, et al. The antitumor immune responses induced by nanoemulsion-encapsulated mage1-hsp70/sea complex protein vaccine following peroral administration route. Cancer Immunol Immunother. (2009) 58:201–8. doi: 10.1007/s00262-008-0539-9
167. Jiang J, Xie D, Zhang W, Xiao G, Wen J. Fusion of hsp70 to mage-A1 enhances the potency of vaccine-specific immune responses. J Transl Med. (2013) 11:300. doi: 10.1186/1479-5876-11-300
168. Dong L, Zhang X, Ren J, Wu S, Yu T, Hou L, et al. Human prostate stem cell antigen and hsp70 fusion protein vaccine inhibits prostate stem cell antigen-expressing tumor growth in mice. Cancer Biother Radiopharm. (2013) 28:391–7. doi: 10.1089/cbr.2012.1357
169. Zhang H, Huang W. Fusion proteins of hsp70 with tumor-associated antigen acting as a potent tumor vaccine and the C-terminal peptide-binding domain of hsp70 being essential in inducing antigen-independent anti-tumor response in vivo. Cell Stress chaperones. (2006) 11:216–26. doi: 10.1379/CSC-191R.1
170. Zong J, Wang C, Liu B, Liu M, Cao Y, Sun X, et al. Human hsp70 and hpv16 oe7 fusion protein vaccine induces an effective antitumor efficacy. Oncol Rep. (2013) 30:407–12. doi: 10.3892/or.2013.2445
171. Li YL, Liu J, Liu JN, Zhang J. Immunization of protein hpv16 E7 in fusion with mouse hsp70 inhibits the growth of tc-1 cells in tumor bearing mice. Vaccine. (2011) 29:5959–62. doi: 10.1016/j.vaccine.2011.06.046
172. Liu G, Yao K, Wang B, Zhou F, Chen Y, Li L, et al. Reconstituted complexes of mycobacterial hsp70 and ebv lmp2a-derived peptides elicit peptide-specific cytotoxic T lymphocyte responses and anti-tumor immunity. Vaccine. (2011) 29:7414–23. doi: 10.1016/j.vaccine.2011.07.063
173. Liu G, Yao K, Wang B, Chen Y, Zhou F, Guo Y, et al. Immunotherapy of epstein-barr virus associated Malignancies using mycobacterial hsp70 and lmp2a356-364 epitope fusion protein. Cell Mol Immunol. (2009) 6:423–31. doi: 10.1038/cmi.2009.54
174. Nishikawa M, Otsuki T, Ota A, Guan X, Takemoto S, Takahashi Y, et al. Induction of tumor-specific immune response by gene transfer of hsp70-cell-penetrating peptide fusion protein to tumors in mice. Mol Ther. (2010) 18:421–8. doi: 10.1038/mt.2009.203
175. Yuan J, Kashiwagi S, Reeves P, Nezivar J, Yang Y, Arrifin NH, et al. A novel mycobacterial hsp70-containing fusion protein targeting mesothelin augments antitumor immunity and prolongs survival in murine models of ovarian cancer and mesothelioma. J Hematol Oncol. (2014) 7:15. doi: 10.1186/1756-8722-7-15
176. Karyampudi L, Ghosh SK. Mycobacterial hsp70 as an adjuvant in the design of an idiotype vaccine against a murine lymphoma. Cell Immunol. (2008) 254:74–80. doi: 10.1016/j.cellimm.2008.07.003
177. Ye Q, Wang ZH, Qin SK. [Preparation of heat shock protein 70 (Hsp70) and idiotypic determinant single-chain antibody (Id-scfv) in a patient with B-cell chronic lymphatic leukemia (B-cll) and antitumor effect of peptide complex hsp70-id]. Ai Zheng. (2008) 27:133–8.
178. Wang ZY, Xing Y, Liu B, Lu L, Huang X, Ge CY, et al. Protective antitumor immunity induced by tumor cell lysates conjugated with diphtheria toxin and adjuvant epitope in mouse breast tumor models. Chin J Cancer. (2012) 31:295–305. doi: 10.5732/cjc.011.10384
179. Li J, Xing Y, Zhou Z, Yao W, Cao R, Li T, et al. Microbial hsp70 peptide epitope 407-426 as adjuvant in tumor-derived autophagosome vaccine therapy of mouse lung cancer. Tumour Biol. (2016) 37:15097–105. doi: 10.1007/s13277-016-5309-2
180. Soleimanjahi H, Razavinikoo H, Fotouhi F, Ardebili A. Antitumor response to a codon-optimized hpv-16 E7/hsp70 fusion antigen DNA vaccine. Iran J Immunol. (2017) 14:180–91.
181. Zong J, Wang C, Wang Q, Peng Q, Xu Y, Xie X, et al. Hsp70 and modified hpv 16 E7 fusion gene without the addition of a signal peptide gene sequence as a candidate therapeutic tumor vaccine. Oncol Rep. (2013) 30:3020–6. doi: 10.3892/or.2013.2742
182. Farzanehpour M, Soleimanjahi H, Hassan ZM, Amanzadeh A, Ghaemi A, Fazeli M. Hsp70 modified response against hpv based tumor. Eur Rev Med Pharmacol Sci. (2013) 17:228–34.
183. Zong J, Peng Q, Wang Q, Zhang T, Fan D, Xu X. Human hsp70 and modified hpv16 E7 fusion DNA vaccine induces enhanced specific cd8+ T cell responses and anti-tumor effects. Oncol Rep. (2009) 22:953–61. doi: 10.3892/or_00000522
184. Li H, Ou X, Xiong J. Modified hpv16 E7/hsp70 DNA vaccine with high safety and enhanced cellular immunity represses murine lung metastatic tumors with downregulated expression of mhc class I molecules. Gynecol Oncol. (2007) 104:564–71. doi: 10.1016/j.ygyno.2006.09.027
185. Hauser H, Chen SY. Augmentation of DNA vaccine potency through secretory heat shock protein-mediated antigen targeting. Methods. (2003) 31:225–31. doi: 10.1016/s1046-2023(03)00136-1
186. Liu TT, Wu Y, Niu T. Human dkk1 and human hsp70 fusion DNA vaccine induces an effective anti-tumor efficacy in murine multiple myeloma. Oncotarget. (2018) 9:178–91. doi: 10.18632/oncotarget.23352
187. Lan YH, Li YG, Liang ZW, Chen M, Peng ML, Tang L, et al. A DNA vaccine against chimeric afp enhanced by hsp70 suppresses growth of hepatocellular carcinoma. Cancer Immunol Immunother. (2007) 56:1009–16. doi: 10.1007/s00262-006-0254-3
188. Lan YH, Li YG, Chen M, Tang L, Ren H. [Immunotherapy with a chimeric afp and hsp70 gene DNA vaccine targeting on a murine hepatocellular carcinoma]. Zhonghua Gan Zang Bing Za Zhi. (2006) 14:510–3.
189. Zhang X, Yu C, Zhao J, Fu L, Yi S, Liu S, et al. Vaccination with a DNA vaccine based on human psca and hsp70 adjuvant enhances the antigen-specific cd8+ T-cell response and inhibits the psca+ Tumors growth in mice. J Gene Med. (2007) 9:715–26. doi: 10.1002/jgm.1067
190. Yamaoka A, Guan X, Takemoto S, Nishikawa M, Takakura Y. Development of a novel hsp70-based DNA vaccine as a multifunctional antigen delivery system. J Controlled release Off J Controlled Release Soc. (2010) 142:411–5. doi: 10.1016/j.jconrel.2009.11.005
191. Chen CH, Wang TL, Hung CF, Yang Y, Young RA, Pardoll DM, et al. Enhancement of DNA vaccine potency by linkage of antigen gene to an hsp70 gene. Cancer Res. (2000) 60:1035–42.
192. Kim JH, Majumder N, Lin H, Watkins S, Falo LD Jr., You Z. Induction of therapeutic antitumor immunity by in vivo administration of a lentiviral vaccine. Hum Gene Ther. (2005) 16:1255–66. doi: 10.1089/hum.2005.16.1255
193. Wu Y, Wan T, Zhou X, Wang B, Yang F, Li N, et al. Hsp70-like protein 1 fusion protein enhances induction of carcinoembryonic antigen-specific cd8+ Ctl response by dendritic cell vaccine. Cancer Res. (2005) 65:4947–54. doi: 10.1158/0008-5472.Can-04-3912
194. Shevtsov MA, Nikolaev BP, Yakovleva LY, Parr MA, Marchenko YY, Eliseev I, et al. 70-kda heat shock protein coated magnetic nanocarriers as a nanovaccine for induction of anti-tumor immune response in experimental glioma. J Controlled release Off J Controlled Release Soc. (2015) 220:329–40. doi: 10.1016/j.jconrel.2015.10.051
195. Ge C, Xing Y, Wang Q, Xiao W, Lu Y, Hu X, et al. Improved efficacy of therapeutic vaccination with dendritic cells pulsed with tumor cell lysate against hepatocellular carcinoma by introduction of 2 tandem repeats of microbial hsp70 peptide epitope 407-426 and ok-432. Int Immunopharmacol. (2011) 11:2200–7. doi: 10.1016/j.intimp.2011.10.001
196. Gao J, Luo SM, Peng ML, Deng T. Enhanced immunity against hepatoma induced by dendritic cells pulsed with hsp70-H22 peptide complexes and cd40l. J Cancer Res Clin Oncol. (2012) 138:917–26. doi: 10.1007/s00432-012-1166-6
197. Wang XH, Qin Y, Hu MH, Xie Y. Dendritic cells pulsed with hsp70-peptide complexes derived from human hepatocellular carcinoma induce specific anti-tumor immune responses. World J Gastroenterol. (2005) 11:5614–20. doi: 10.3748/wjg.v11.i36.5614
198. Shevtsov M, Pitkin E, Ischenko A, Stangl S, Khachatryan W, Galibin O, et al. Ex vivo hsp70-activated nk cells in combination with pd-1 inhibition significantly increase overall survival in preclinical models of glioblastoma and lung cancer. Front Immunol. (2019) 10:454. doi: 10.3389/fimmu.2019.00454
199. Sharifzad F, Mardpour S, Mardpour S, Fakharian E, Taghikhani A, Sharifzad A, et al. Hsp70/il-2 treated nk cells effectively cross the blood brain barrier and target tumor cells in a rat model of induced glioblastoma multiforme (Gbm). Int J Mol Sci. (2020) 21:2263. doi: 10.3390/ijms21072263
200. Moser C, Schmidbauer C, Gürtler U, Gross C, Gehrmann M, Thonigs G, et al. Inhibition of tumor growth in mice with severe combined immunodeficiency is mediated by heat shock protein 70 (Hsp70)-peptide-activated, cd94 positive natural killer cells. Cell Stress chaperones. (2002) 7:365–73. doi: 10.1379/1466-1268(2002)007<0365:Iotgim>2.0.Co;2
201. Xu M, Zhou L, Zhang Y, Xie Z, Zhang J, Guo L, et al. A fixed human umbilical vein endothelial cell vaccine with 2 tandem repeats of microbial hsp70 peptide epitope 407-426 as adjuvant for therapy of hepatoma in mice. J Immunother. (2015) 38:276–84. doi: 10.1097/cji.0000000000000091
202. Han L, Wang W, Lu J, Kong F, Ma G, Zhu Y, et al. Aav-sbtla facilitates hsp70 vaccine-triggered prophylactic antitumor immunity against a murine melanoma pulmonary metastasis model in vivo. Cancer Lett. (2014) 354:398–406. doi: 10.1016/j.canlet.2014.08.006
203. Han L, Wang W, Fang Y, Feng Z, Liao S, Li W, et al. Soluble B and T lymphocyte attenuator possesses antitumor effects and facilitates heat shock protein 70 vaccine-triggered antitumor immunity against a murine tc-1 cervical cancer model in vivo. J Immunol. (2009) 183:7842–50. doi: 10.4049/jimmunol.0804379
204. Nakajima M, Hazama S, Tamada K, Udaka K, Kouki Y, Uematsu T, et al. A phase I study of multi-hla-binding peptides derived from heat shock protein 70/glypican-3 and a novel combination adjuvant of hlag-3ig and poly-iclc for patients with metastatic gastrointestinal cancers: ynp01 trial. Cancer Immunol Immunother. (2020) 69:1651–62. doi: 10.1007/s00262-020-02518-7
205. Jo Y, Ali LA, Shim JA, Lee BH, Hong C. Innovative car-T cell therapy for solid tumor; current duel between car-T spear and tumor shield. Cancers. (2020) 12:2087. doi: 10.3390/cancers12082087
206. Yuan Y, Fan J, Liang D, Wang S, Luo X, Zhu Y, et al. Cell surface grp78-directed car-T cells are effective at treating human pancreatic cancer in preclinical models. Trans Oncol. (2024) 39:101803. doi: 10.1016/j.tranon.2023.101803
207. Wang S, Wei W, Yuan Y, Guo J, Liang D, Zhao X. Cell-surface grp78-targeted chimeric antigen receptor T cells eliminate lung cancer tumor xenografts. Int J Mol Sci. (2024) 25:564. doi: 10.3390/ijms25010564
208. Wang S, Wei W, Yuan Y, Sun B, Yang D, Liu N, et al. Chimeric antigen receptor T cells targeting cell surface grp78 efficiently kill glioblastoma and cancer stem cells. J Transl Med. (2023) 21:493. doi: 10.1186/s12967-023-04330-0
209. Ibanez J, Hebbar N, Thanekar U, Yi Z, Houke H, Ward M, et al. Grp78-Car T cell Effector Function against Solid and Brain Tumors Is Controlled by Grp78 Expression on T cells. Cell Rep Med. (2023) 4:101297. doi: 10.1016/j.xcrm.2023.101297
210. Yu W, Zhang H, Yuan Y, Tang J, Chen X, Liu T, et al. Chimeric antigen receptor T cells targeting cell surface grp78 to eradicate acute myeloid leukemia. Front Cell Dev Biol. (2022) 10:928140. doi: 10.3389/fcell.2022.928140
211. Hebbar N, Epperly R, Vaidya A, Thanekar U, Moore SE, Umeda M, et al. Car T cells redirected to cell surface grp78 display robust anti-acute myeloid leukemia activity and do not target hematopoietic progenitor cells. Nat Commun. (2022) 13:587. doi: 10.1038/s41467-022-28243-6
212. Bashiri Dezfouli A, Yazdi M, Benmebarek MR, Schwab M, Michaelides S, Miccichè A, et al. Car T cells targeting membrane-bound hsp70 on tumor cells mimic hsp70-primed nk cells. Front Immunol. (2022) 13:883694. doi: 10.3389/fimmu.2022.883694
213. Li Z, Zhao C, Li Z, Zhao Y, Shan S, Shi T, et al. Reconstructed mung bean trypsin inhibitor targeting cell surface grp78 induces apoptosis and inhibits tumor growth in colorectal cancer. Int J Biochem Cell Biol. (2014) 47:68–75. doi: 10.1016/j.biocel.2013.11.022
214. Miao YR, Eckhardt BL, Cao Y, Pasqualini R, Argani P, Arap W, et al. Inhibition of established micrometastases by targeted drug delivery via cell surface-associated grp78. Clin Cancer Res an Off J Am Assoc Cancer Res. (2013) 19:2107–16. doi: 10.1158/1078-0432.Ccr-12-2991
215. Larson N, Ray A, Malugin A, Pike DB, Ghandehari H. Hpma copolymer-aminohexylgeldanamycin conjugates targeting cell surface expressed grp78 in prostate cancer. Pharm Res. (2010) 27:2683–93. doi: 10.1007/s11095-010-0267-7
216. Xie R, Wang Y, Tong F, Yang W, Lei T, Du Y, et al. Hsp70-targeting and size-tunable nanoparticles combine with pd-1 checkpoint blockade to treat glioma. Small. (2023) 19:e2300570. doi: 10.1002/smll.202300570
217. Shin J, Kim B, Lager TW, Mejia F, Guldner I, Conner C, et al. A nanotherapeutic approach to selectively eliminate metastatic breast cancer cells by targeting cell surface grp78. Nanoscale. (2023) 15:13322–34. doi: 10.1039/D3NR00800B
218. Wang J, Bhargava P, Yu Y, Sari AN, Zhang H, Ishii N, et al. Novel caffeic acid phenethyl ester-mortalin antibody nanoparticles offer enhanced selective cytotoxicity to cancer cells. Cancers. (2020) 12:2370. doi: 10.3390/cancers12092370
219. Zhao L, Li H, Shi Y, Wang G, Liu L, Su C, et al. Nanoparticles inhibit cancer cell invasion and enhance antitumor efficiency by targeted drug delivery via cell surface-related grp78. Int J Nanomedicine. (2015) 10:245–56. doi: 10.2147/ijn.S74868
220. Delie F, Petignat P, Cohen M. Grp78-targeted nanotherapy against castrate-resistant prostate cancer cells expressing membrane grp78. Target Oncol. (2013) 8:225–30. doi: 10.1007/s11523-012-0234-9
221. Farshbaf M, Mojarad-Jabali S, Hemmati S, Khosroushahi AY, Motasadizadeh H, Zarebkohan A, et al. Enhanced bbb and bbtb penetration and improved anti-glioma behavior of bortezomib through dual-targeting nanostructured lipid carriers. J Controlled release Off J Controlled Release Soc. (2022) 345:371–84. doi: 10.1016/j.jconrel.2022.03.019
222. Wu Z, Stangl S, Hernandez-Schnelzer A, Wang F, Hasanzadeh Kafshgari M, Bashiri Dezfouli A, et al. Functionalized hybrid iron oxide-gold nanoparticles targeting membrane hsp70 radiosensitize triple-negative breast cancer cells by ros-mediated apoptosis. Cancers. (2023) 15:1167. doi: 10.3390/cancers15041167
223. González FE, Gleisner A, Falcón-Beas F, Osorio F, López MN, Salazar-Onfray F. Tumor cell lysates as immunogenic sources for cancer vaccine design. Hum Vaccin Immunother. (2014) 10:3261–9. doi: 10.4161/21645515.2014.982996
Keywords: extracellular HSP70, cancer, biomarker, treatment, vaccine
Citation: Hu B, Liu G, Zhao K and Zhang G (2024) Diversity of extracellular HSP70 in cancer: advancing from a molecular biomarker to a novel therapeutic target. Front. Oncol. 14:1388999. doi: 10.3389/fonc.2024.1388999
Received: 20 February 2024; Accepted: 25 March 2024;
Published: 05 April 2024.
Edited by:
Khosrow Kashfi, City University of New York, United StatesReviewed by:
Zahra Alimardan, Arak University of Medical Sciences, IranMau-Shin Chi, Shin Kong Wu Ho-Su Memorial Hospital, Taiwan
Copyright © 2024 Hu, Liu, Zhao and Zhang. This is an open-access article distributed under the terms of the Creative Commons Attribution License (CC BY). The use, distribution or reproduction in other forums is permitted, provided the original author(s) and the copyright owner(s) are credited and that the original publication in this journal is cited, in accordance with accepted academic practice. No use, distribution or reproduction is permitted which does not comply with these terms.
*Correspondence: Kejia Zhao, a2VqaWEuemhhb0B3Y2hzY3UuZWR1LmNu; Gao Zhang, Z3poYW5nNkBtZS5jb20=
†These authors have contributed equally to this work and share first authorship