- 1Department of Radiodiagnosis and Imaging, Postgraduate Institute of Medical Education and Research, Chandigarh, India
- 2Department of Radiology, Massachusetts General Hospital, Harvard Medical School, Boston, MA, United States
- 3Department of Medical Oncology, BALCO Medical Centre, Raipur, Chhattisgarh, India
Editorial on the Research Topic
Role of imaging in biliary tract cancer: diagnosis, staging, response prediction, and image-guided therapeutics
Biliary tract cancers (BTC) comprise a spectrum of cancers arising from the intrahepatic or extrahepatic biliary tree (cholangiocarcinoma, CCA) and gallbladder (gallbladder cancer, GBC) (1). There is marked geographical variation in the incidence of BTCs (1). The incidence of CCA and GBC is low in high-income countries (2–4). The incidence of CCA is 40 times higher in endemic regions of China and Thailand (5). GBC incidence is the highest in women in Southern Chile and Northern India (6).
Recent studies have shown that CCA arises from two types of stem cells that determine its radiological appearance and prognosis (7, 8). CCA is classified anatomically into intrahepatic CCA (iCCA) and extrahepatic CCA (perihilar and distal CCA) (9). Perihilar CCA is classified most commonly based on the longitudinal extent of the disease (Bismuth Corlette system). However, classification systems that consider vascular involvement, remnant liver volume, lymph node, and distant metastases (TNM, MSKCC, Deoliveira) allow better resectability assessment and prognostication (10).
The early diagnosis of BTC is challenging due to non-specific symptoms (11). Most patients have advanced unresectable disease at the time of diagnosis. The imaging appearance of iCCA depends on the potential cell of origin (12). Large and small duct types of ICCs have different morphological appearances (Figure 1). Perihilar CC presents most commonly as a periductal infiltrating lesion, which must be differentiated from benign strictures (13). GBC manifests as a mass-replacing the gallbladder, wall-thickening, combined form, or intraluminal polypoidal form (14). Each form poses a distinct challenge in differentiation from benign diseases (15). Ultrasound (US), computed tomography (CT), and magnetic resonance imaging (MRI) are the most common imaging tests employed for the detection of BTC. US-based risk stratification systems, e.g., gallbladder reporting and data system (GB-RADS), guide the utilization of further imaging tests in patients with gallbladder lesions (16, 17). Multiphasic contrast-enhanced CT allows accurate staging of BTC (18). MRI and magnetic resonance cholangiopancreatography (MRCP) are preferred to evaluate the longitudinal extent of perihilar CC, determination of local extent and intrahepatic metastases. A multiparametric MRI has been recently proposed to characterize gallbladder wall thickening (19). [18F]-2-fluoro-2-deoxy-d-glucose–positron emission tomography (FDG PET) is not recommended for primary diagnosis of BTC (20). FDG PET may allow accurate diagnosis of nodal metastases, distant metastases, and recurrence (21). Fibroblast-activated protein (FAP) is highly expressed in cancer-associated fibroblasts. Gallium 68 (68G)-FAP inhibitor (FAPI) is a molecular target of FAP. FAPI PET enables the detection of small primary or metastatic lesions with a strong desmoplastic reaction. 68G-FAPI PET has been reported to be superior to FDG-PET in detecting various primary tumors (22). In this Research Topic, Ouyang et al. reported a systematic review comparing 19F-FDG PET with 68G-FAPI-04 PET in primary digestive system malignancy. They reviewed 15 studies comprising 383 patients and reported higher pooled sensitivity (98% vs. 73%) and specificity of 68Ga-FAPI-04 PET (0.81 vs. 0.77) compared to FDG PET for gastric, liver, biliary tract, and pancreatic cancers.
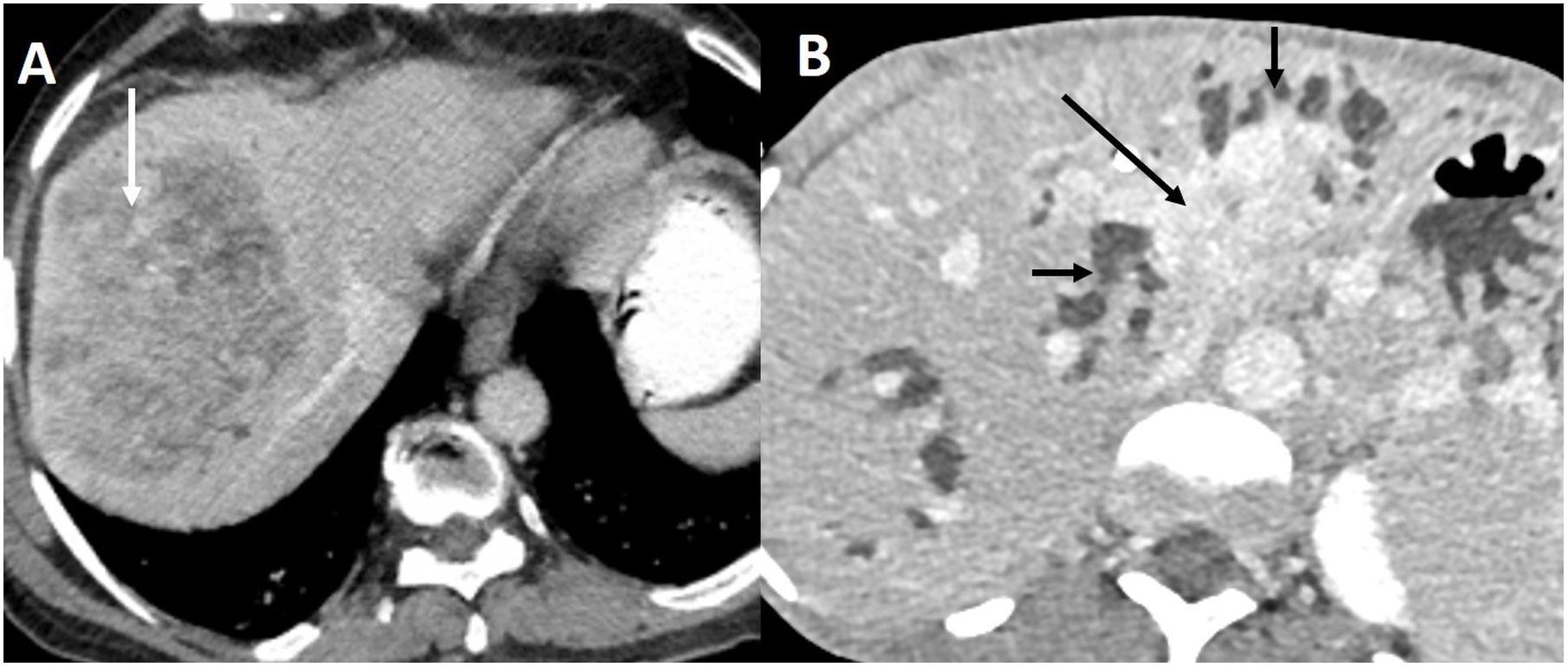
Figure 1 Imaging appearance of small and large duct intrahepatic cholangiocarcinoma. (A) Contrast-enhanced CT in the portal venous phase shows small duct ICC as a heterogeneously enhancing mass (arrow) in the periphery of the liver without bile duct dilatation. (B) Large duct ICC appears as an enhancing mass (arrow) infiltrating the left sided bile ducts causing biliary dilatation (short arrows) in the delayed phase CT.
Premalignant bile duct lesions include biliary intraepithelial neoplasm (BiN) and intraductal papillary neoplasm of the bile duct (IPNB) (23). Bile duct adenoma (BDA) is a rare benign bile duct lesion and is considered a controversial precursor to ICC (24). Pre-operative diagnosis of BDA is challenging. Huang et al. reported the imaging appearance of 32 pathologically proven BDAs. The most common site was the common bile duct (68.7%), and the most common morphology was focal eccentric mass (43.7%), followed by plaque-like masses (28.1%). BDAs with CC were associated with infiltrative masses. Dynamic contrast-enhanced MRI (18 lesions) showed moderate persistent enhancement in most lesions. The accurate diagnosis of BTC and differentiation from other bile duct lesions may necessitate advanced imaging techniques (25). Deng et al. reported a case where they used a single-operator peroral cholangioscopy system (SpyGlass) for diagnosing extrahepatic bile duct stricture seen at CT and MRI. Endoscopic retrograde cholangiopancreatography (ERCP) and cholangiogram revealed an indeterminate stricture. SpyGlass revealed an oval lesion with smooth, overlying mucosa. Extrahepatic biliary cystadenoma was confirmed at surgery. Zhu et al. compared the diagnostic accuracy of cytobrush, ERCP-guided biopsy, SpyGlass direct visual impression, and SpyGlass-guided biopsy (SpyBite) in the differentiation of benign and malignant biliary strictures in 1008 patients. The highest sensitivity was reported for SpyGlass (100%), followed by SpyBite (61.5%). However, the specificity of SpyGlass (55.6%) was significantly inferior to other methods (99-100%).
Identifying novel molecular targets may improve survival in patients with locally advanced BTC (26). Actionable molecular aberrations have been reported in up to 40% of ICC (27). The most promising molecular targets in ICC are isocitrate dehydrogenase 1 (IDH1) and fibroblast growth factor receptor 2 (FGFR2) (28). Several studies have reported a favorable prognosis in patients with IDH1 and FGFR 2 alterations (29–32). Brandi et al. reviewed the literature on the role of IDH and FGFR molecular alterations as positive prognostic markers in CC. They highlighted the strengths and pitfalls of the available literature and concluded that better-designed trials are needed to provide conclusive evidence on the prognostic role of IDH and FGFR. Imaging studies may give a clue to the type of molecular aberration in ICC. Small bile duct ICCs are characterized by IDH and FGFR2 fusions (33). On the other hand, large duct ICC and extrahepatic CC show a high frequency of KRAS and TP53 gene mutations (33). Although there is relatively limited data on GBC, a precision medicine strategy is supported by some recent studies (34). Comprehensive genomic profiling of 760 GBC patients identified at least one actionable genetic aberration in 86.6% (35). The most frequent actionable gene alteration was CKDN2A, followed by ERBB2/HER-2. Anti-HER2-directed therapy may improve outcomes in unresectable GBC (35).
Imaging studies provide data for downstream tasks relevant to diagnosis, prognostication, or identification of gene mutations (36). Radiomics is one approach that extracts many quantitative features from radiological images (37). Radiomic data can also be used for tasks based on artificial intelligence (AI) (38). There are several studies on using radiomics and AI in BTC (39–46). Chen et al. extensively reviewed the current status of radiomics in ICC. They highlighted studies utilizing radiomics to predict lymph node metastases, microvascular invasion, early recurrence after surgery, prediction of survival, and differentiation of ICC from other liver tumors.
In conclusion, imaging plays a vital role in the detection and staging of BTC and also serves as a novel biomarker for response prediction. Imaging-based radiomics and AI can potentially impact outcomes in patients with BTC.
Author contributions
PG: Conceptualization, Writing – original draft, Writing – review & editing. AK: Conceptualization, Writing – review & editing. BS: Conceptualization, Writing – review & editing.
Conflict of interest
The authors declare that the research was conducted in the absence of any commercial or financial relationships that could be construed as a potential conflict of interest.
The author(s) declared that they were an editorial board member of Frontiers, at the time of submission. This had no impact on the peer review process and the final decision.
Publisher’s note
All claims expressed in this article are solely those of the authors and do not necessarily represent those of their affiliated organizations, or those of the publisher, the editors and the reviewers. Any product that may be evaluated in this article, or claim that may be made by its manufacturer, is not guaranteed or endorsed by the publisher.
References
1. Valle JW, Kelley RK, Nervi B, Oh DY, Zhu AX. Biliary tract cancer. Lancet. (2021) 397:428–44. doi: 10.1016/S0140-6736(21)00153-7
2. Patel T. Increasing incidence and mortality of primary intrahepatic cholangiocarcinoma in the United States. Hepatology. (2001) 33:1353–7. doi: 10.1053/jhep.2001.25087
3. Roa JC, García P, Kapoor VK, Maithel SK, Javle M, Koshiol J. Gallbladder cancer [published correction appears in Nat Rev Dis Primers. 2022 Nov 18;8(1):75]. Nat Rev Dis Primers. (2022) 8:69. doi: 10.1038/s41572-022-00398-y
4. Dutta U, Bush N, Kalsi D, Popli P, Kapoor VK. Epidemiology of gallbladder cancer in India. Chin Clin Oncol. (2019) 8:33. doi: 10.21037/cco.2019.08.03
5. Banales JM, Cardinale V, Carpino G, Marzioni M, Andersen JB, Invernizzi P, et al. Expert consensus document: Cholangiocarcinoma: current knowledge and future perspectives consensus statement from the European Network for the Study of Cholangiocarcinoma (ENS-CCA). Nat Rev Gastroenterol Hepatol. (2016) 13:261–80. doi: 10.1038/nrgastro.2016.51
6. Sung H, Ferlay J, Siegel RL, Laversanne M, Soerjomataram I, Jemal A, et al. Global cancer statistics 2020: GLOBOCAN estimates of incidence and mortality worldwide for 36 cancers in 185 countries. CA Cancer J Clin. (2021) 71:209–49. doi: 10.3322/caac.21660
7. Welzel TM, Graubard BI, El-Serag HB, Shaib YH, Hsing AW, Davila JA, et al. Risk factors for intrahepatic and extrahepatic cholangiocarcinoma in the United States: a population-based case-control study. Clin Gastroenterol Hepatol. (2007) 5:1221–8. doi: 10.1016/j.cgh.2007.05.020
8. Carpino G, Renzi A, Franchitto A, Cardinale V, Onori P, Reid L, et al. Stem/progenitor cell niches involved in hepatic and biliary regeneration. Stem Cells Int. (2016) 2016:3658013. doi: 10.1155/2016/3658013
9. Joo I, Lee JM, Yoon JH. Imaging diagnosis of intrahepatic and perihilar cholangiocarcinoma: recent advances and challenges. Radiology. (2018) 288:7–13. doi: 10.1148/radiol.2018171187
10. Deoliveira ML, Schulick RD, Nimura Y, Rosen C, Gores G, Neuhaus P, et al. New staging system and a registry for perihilar cholangiocarcinoma. Hepatology. (2011) 53:1363–71. doi: 10.1002/hep.24227
11. Matos C, Serrao E, Bali MA. Magnetic resonance imaging of biliary tumors. Magn Reson Imaging Clin N Am. (2010) 18:477–96. doi: 10.1016/j.mric.2010.08.004
12. Nakanuma Y, Sato Y, Harada K, Sasaki M, Xu J, Ikeda H. Pathological classification of intrahepatic cholangiocarcinoma based on a new concept. World J Hepatol. (2010) 2:419–27. doi: 10.4254/wjh.v2.i12.419
13. Katabathina VS, Dasyam AK, Dasyam N, Hosseinzadeh K. Adult bile duct strictures: role of MR imaging and MR cholangiopancreatography in characterization. RadioGraphics. (2014) 34:565–86. doi: 10.1148/rg.343125211
14. Chhabra M, Kalage D, Gupta P, Siddiqui R, Singh S, Yadav TD, et al. Proposal for a new morphological “combined type” of gallbladder cancer: description of radiopathological characteristics and comparison with other morphological types. Abdom Radiol (NY). (2024) 49:703–9. doi: 10.1007/s00261-023-04090-x
15. Gupta P, Marodia Y, Bansal A, Kalra N, Kumar-M P, Sharma V, et al. Imaging-based algorithmic approach to gallbladder wall thickening. World J Gastroenterol. (2020) 26:6163–81. doi: 10.3748/wjg.v26.i40.6163
16. Gupta P, Dutta U, Rana P, Singhal M, Gulati A, Kalra N, et al. Gallbladder reporting and data system (GB-RADS) for risk stratification of gallbladder wall thickening on ultrasonography: an international expert consensus. Abdom Radiol (NY). (2022) 47:554–65. doi: 10.1007/s00261-021-03360-w
17. Konstantinoff KS, Feister KF, Mellnick VM. RadioGraphics update: new follow-up and management recommendations for polypoid lesions of the gallbladder. Radiographics. (2023) 43:e220189. doi: 10.1148/rg.220189
18. Ramachandran A, Srivastava DN, Madhusudhan KS. Gallbladder cancer revisited: the evolving role of a radiologist. Br J Radiol. (2021) 94:20200726. doi: 10.1259/bjr.20200726
19. Kalage D, Gupta P, Gulati A, Yadav TD, Gupta V, Kaman L, et al. Multiparametric MR imaging with diffusion-weighted, intravoxel incoherent motion, diffusion tensor, and dynamic contrast-enhanced perfusion sequences to assess gallbladder wall thickening: a prospective study based on surgical histopathology. Eur Radiol. (2023) 33:4981–93. doi: 10.1007/s00330-023-09455-w
20. Vogel A, Bridgewater J, Edeline J, Kelley RK, Klümpen HJ, et al. Biliary tract cancer: ESMO Clinical Practice Guideline for diagnosis, treatment and follow-up. Ann Oncol. (2023) 34:127–40. doi: 10.1016/j.annonc.2022.10.506
21. Lamarca A, Barriuso J, Chander A, McNamara MG, Hubner RA, ÓReilly D, et al. 18F-fluorodeoxyglucose positron emission tomography (18FDG-PET) for patients with biliary tract cancer: Systematic review and meta-analysis. J Hepatol. (2019) 71:115–29. doi: 10.1016/j.jhep.2019.01.038
22. Mori Y, Dendl K, Cardinale J, Kratochwil C, Giesel FL, Haberkorn U. FAPI PET: fibroblast activation protein inhibitor use in oncologic and nononcologic disease. Radiology. (2023) 306:e220749. doi: 10.1148/radiol.220749
23. Nakanuma Y, Sasaki M, Sato Y, Ren X, Ikeda H, Harada K. Multistep carcinogenesis of perihilar cholangiocarcinoma arising in the intrahepatic large bile ducts. World J Hepatol. (2009) 1:35–42. doi: 10.4254/wjh.v1.i1.35
24. Sasaki M, Sato Y, Nakanuma Y. Bile duct adenoma may be a precursor lesion of small duct type intrahepatic cholangiocarcinoma. Histopathology. (2021) 78:310–20. doi: 10.1111/his.14222
25. de Oliveira P, de Moura DTH, Ribeiro IB, Bazarbashi AN, Franzini TAP, Dos Santos MEL, et al. Efficacy of digital single-operator cholangioscopy in the visual interpretation of indeterminate biliary strictures: a systematic review and metaanalysis. Surg Endosc. (2020) 34:3321–9. doi: 10.1007/s00464-020-07583-8
26. Weinberg BA, Xiu J, Lindberg MR, Shields AF, Hwang JJ, Poorman K, et al. Molecular profiling of biliary cancers reveals distinct molecular alterations and potential therapeutic targets. J Gastrointest Oncol. (2019) 10:652–62. doi: 10.21037/jgo.2018.08.18
27. Mody K, Jain P, El-Refai SM, Azad NS, Zabransky DJ, Baretti M, et al. Clinical, genomic, and transcriptomic data profiling of biliary tract cancer reveals subtype-specific immune signatures. JCO Precis Oncol. (2022) 6:e2100510. doi: 10.1200/PO.21.00510
28. Churi CR, Shroff R, Wang Y, Rashid A, Kang HC, Weatherly J, et al. Mutation profiling in cholangiocarcinoma: prognostic and therapeutic implications. PloS One. (2014) 9:e115383. doi: 10.1371/journal.pone.0115383
29. Rizzato M, Brignola S, Munari G, Gatti M, Dadduzio V, Borga C, et al. Prognostic impact of FGFR2/3 alterations in patients with biliary tract cancers receiving systemic chemotherapy: the BITCOIN study. Eur J Cancer. (2022) 166:165–75. doi: 10.1016/j.ejca.2022.02.013
30. Maruki Y, Morizane C, Arai Y, Ikeda M, Ueno M, Ioka T, et al. Molecular detection and clinicopathological characteristics of advanced/recurrent biliary tract carcinomas harboring the FGFR2 rearrangements: a prospective observational study (PRELUDE study). J Gastroenterol. (2021) 56:250–60. doi: 10.1007/s00535-020-01735-2
31. Ma B, Meng H, Tian Y, Wang Y, Song T, Zhang T, et al. Distinct clinical and prognostic implication of IDH1/2 mutation and other most frequent mutations in large duct and small duct subtypes of intrahepatic cholangiocarcinoma. BMC Cancer. (2020) 20:318. doi: 10.1186/s12885-020-06804-6
32. Wang P, Dong Q, Zhang C, Kuan PF, Liu Y, Jeck WR, et al. Mutations in isocitrate dehydrogenase 1 and 2 occur frequently in intrahepatic cholangiocarcinomas and share hypermethylation targets with glioblastomas. Oncogene. (2013) 32:3091–100. doi: 10.1038/onc.2012.315
33. Banales JM, Marin JJG, Lamarca A, Rodrigues PM, Khan SA, Roberts LR, et al. Cholangiocarcinoma 2020: the next horizon in mechanisms and management. Nat Rev Gastroenterol Hepatol. (2020) 17:557–88. doi: 10.1038/s41575-020-0310-z
34. Verlingue L, Malka D, Allorant A, Massard C, Ferté C, Lacroix L, et al. Precision medicine for patients with advanced biliary tract cancers: an effective strategy within the prospective MOSCATO-01 trial. Eur J Cancer. (2017) 87:122–30. doi: 10.1016/j.ejca.2017.10.013
35. Abdel-Wahab R, Yap TA, Madison R, Pant S, Cooke M, Wang K, et al. Genomic profiling reveals high frequency of DNA repair genetic aberrations in gallbladder cancer. Sci Rep. (2020) 10:22087. doi: 10.1038/s41598-020-77939-6
36. van Timmeren JE, Cester D, Tanadini-Lang S, Alkadhi H, Baessler B. Radiomics in medical imaging-”how-to” guide and critical reflection. Insights Imaging. (2020) 11:91. doi: 10.1186/s13244-020-00887-2
37. Mayerhoefer ME, Materka A, Langs G, Häggström I, Szczypiński P, Gibbs P, et al. Introduction to radiomics. J Nucl Med. (2020) 61:488–95. doi: 10.2967/jnumed.118.222893
38. Koçak B, Durmaz EŞ, Ateş E, Kılıçkesmez Ö. Radiomics with artificial intelligence: a practical guide for beginners. Diagn Interv Radiol. (2019) 25:485–95. doi: 10.5152/dir.2019.19321
39. Ji GW, Zhang YD, Zhang H, Zhu FP, Wang K, Xia YX, et al. Biliary tract cancer at CT: A radiomics-based model to predict lymph node metastasis and survival outcomes. Radiology. (2019) 290:90–8. doi: 10.1148/radiol.2018181408
40. Tang Y, Zhang T, Zhou X, Zhao Y, Xu H, Liu Y, et al. The preoperative prognostic value of the radiomics nomogram based on CT combined with machine learning in patients with intrahepatic cholangiocarcinoma. World J Surg Oncol. (2021) 19:45. doi: 10.1186/s12957-021-02162-0
41. Liu Z, Luo C, Chen X, Feng Y, Feng J, Zhang R, et al. Non-invasive prediction of perineural invasion in intrahepatic cholangiocarcinoma by clinicoradiological features and computed tomography radiomics based on interpretable machine learning: a multicenter cohort study. Int J Surg. (2024) 110:1039–51. doi: 10.1097/JS9.0000000000000881
42. Bo Z, Chen B, Yang Y, Yao F, Mao Y, Yao J, et al. Machine learning radiomics to predict the early recurrence of intrahepatic cholangiocarcinoma after curative resection: A multicentre cohort study. Eur J Nucl Med Mol Imaging. (2023) 50:2501–13. doi: 10.1007/s00259-023-06184-6
43. Song Y, Zhou G, Zhou Y, Xu Y, Zhang J, Zhang K, et al. Artificial intelligence CT radiomics to predict early recurrence of intrahepatic cholangiocarcinoma: a multicenter study. Hepatol Int. (2023). doi: 10.1007/s12072-023-10487-z
44. Jolissaint JS, Wang T, Soares KC, Chou JF, Gönen M, Pak LM, et al. Machine learning radiomics can predict early liver recurrence after resection of intrahepatic cholangiocarcinoma. HPB (Oxford). (2022) 24:1341–50. doi: 10.1016/j.hpb.2022.02.004
45. Gupta P, Basu S, Rana P, Dutta U, Soundararajan R, Kalage D, et al. Deep-learning enabled ultrasound based detection of gallbladder cancer in northern India: a prospective diagnostic study. Lancet Reg Health South East Asia. (2023). doi: 10.1016/j.lansea.2023.100279
Keywords: staging, biliary tract cancer (BTC), gallbladder cancer (GBC), computed tomography, radiomics, endoscopy, ERCP (cholangiopancreatography, endoscopic retrograde)
Citation: Gupta P, Kambadakone A and Sirohi B (2024) Editorial: Role of imaging in biliary tract cancer: diagnosis, staging, response prediction and image-guided therapeutics. Front. Oncol. 14:1387531. doi: 10.3389/fonc.2024.1387531
Received: 17 February 2024; Accepted: 07 March 2024;
Published: 19 March 2024.
Edited and Reviewed by:
Zaver Bhujwalla, Johns Hopkins Medicine, United StatesCopyright © 2024 Gupta, Kambadakone and Sirohi. This is an open-access article distributed under the terms of the Creative Commons Attribution License (CC BY). The use, distribution or reproduction in other forums is permitted, provided the original author(s) and the copyright owner(s) are credited and that the original publication in this journal is cited, in accordance with accepted academic practice. No use, distribution or reproduction is permitted which does not comply with these terms.
*Correspondence: Pankaj Gupta, UGFua2FqZ3VwdGE5NTlAZ21haWwuY29t