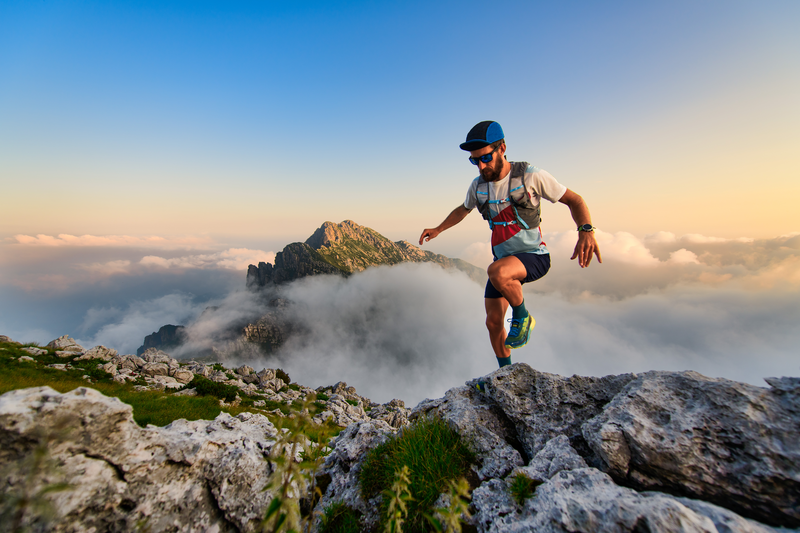
94% of researchers rate our articles as excellent or good
Learn more about the work of our research integrity team to safeguard the quality of each article we publish.
Find out more
REVIEW article
Front. Oncol. , 04 June 2024
Sec. Gynecological Oncology
Volume 14 - 2024 | https://doi.org/10.3389/fonc.2024.1387281
This article is part of the Research Topic Advances toward improved understanding and treatment of uncommon ovarian cancer types and subtypes View all 18 articles
Approximately 50% of tubo-ovarian high-grade serous carcinomas (HGSCs) have functional homologous recombination-mediated (HR) DNA repair, so-called HR-proficient tumors, which are often associated with primary platinum resistance (relapse within six months after completion of first-line therapy), minimal benefit from poly(ADP‐ribose) polymerase (PARP) inhibitors, and shorter survival. HR-proficient tumors comprise multiple molecular subtypes including cases with CCNE1 amplification, AKT2 amplification or CDK12 alteration, and are often characterized as “cold” tumors with fewer infiltrating lymphocytes and decreased expression of PD-1/PD-L1. Several new treatment approaches aim to manipulate these negative prognostic features and render HR-proficient tumors more susceptible to treatment. Alterations in multiple different molecules and pathways in the DNA damage response are driving new drug development to target HR-proficient cancer cells, such as inhibitors of the CDK or P13K/AKT pathways, as well as ATR inhibitors. Treatment combinations with chemotherapy or PARP inhibitors and agents targeting DNA replication stress have shown promising preclinical and clinical results. New approaches in immunotherapy are also being explored, including vaccines or antibody drug conjugates. Many approaches are still in the early stages of development and further clinical trials will determine their clinical relevance. There is a need to include HR-proficient tumors in ovarian cancer trials and to analyze them in a more targeted manner to provide further evidence for their specific therapy, as this will be crucial in improving the overall prognosis of HGSC and ovarian cancer in general.
Advanced tubo-ovarian high-grade serous carcinoma (HGSC) accounts for a majority of the disease burden and deaths from ovarian cancer (70–80%) due to its typical late presentation and high 5-year recurrence rate of 75% (1–3). Primary cytoreductive surgery followed by platinum- and taxane-based chemotherapy or neoadjuvant platinum- and taxane-based chemotherapy (NACT) followed by interval cytoreductive surgery is the standard treatment for HGSC (1–9). Most HGSC initially respond well to chemotherapy. However, the majority of patients will experience relapse with treatment resistant disease, particularly those without BRCA mutations and without homologous recombination deficiency (HRD) (10–13). Although there has been limited improvement in the 5-year survival rate of most patients over the past three decades (4, 8, 14–16), the introduction of poly(ADP-ribose) polymerase inhibitors (PARPis) as maintenance therapy in HGSC has had a profound impact leading to significant improvements in progression-free survival (PFS) and demonstrating a trend towards improved overall survival (OS), particularly in patients with BRCA1 or BRCA2 (BRCA) mutations and HRD (1, 3, 13, 17–29).
HRD refers to a loss of homologous recombination-mediated DNA repair (HRR), which is a pathway responsible for the high-fidelity repair of double-stranded DNA breaks that restores the original DNA sequence at the site of damage. HRD contributes to genomic instability and consequently intact HRR plays a role in preventing malignant transformation (30, 31). HRD is caused by inherited or somatic loss of function genetic alterations in well-known driver genes such as BRCA1 and BRCA2, but also by mutations or methylation of other HRR related genes and potentially other currently undefined mechanisms (32). Patients with HRD HGSC are more likely to benefit from a favorable chemotherapy response, maintenance treatment with PARPis and consequently a longer OS (1, 33–38). However, ~50% of HGSC are HR-proficient (HRP), an established poor prognostic marker associated with primary platinum and PARPi resistance and shorter survival times (36, 39, 40). Platinum-resistant ovarian cancer is defined as disease that relapses within six months of completing first-line treatment, and the probability of a response to platinum re-treatment is less than 10% (33, 41, 42). In fact, HGSC can also progress from HRD to become at least partially HRP by reversion of HR gene alterations through secondary genetic or epigenetic events (43–45). This acquired HR-proficiency is one of the most well described mechanisms of acquired treatment resistance and consequently a major clinical challenge.
HRD status in ovarian cancer is usually inferred by measurement of BRCA mutation status and/or the extent of cancer genome scarring associated with loss of HRR genes. Methodologies that assess HRD typically measure the extent of telomeric allelic imbalance, loss of heterozygosity, and large-scale transitions (31, 46). However, these scores are based on permanent genomic scars, thus failing to reflect the current HRD status in the case of HRR restoration (47). An alternative is a dynamic assessment of HR status using functional assays in ex-vivo cultures (46). Immunofluorescence microscopy can be used to measure the presence of RAD51 formed molecular complexes which accumulate at sites of double-stranded DNA breaks in HRP cells. By contrast, HRD cells are unable to form RAD51 formed molecular complexes and their absence thus provides a functional indication of a defect in the HR pathway (48, 49). However, such RAD51 assays are yet to be clinically validated. Additionally, resistance to PARPis may be driven by RAD51-independent mechanisms and consequently cannot be detected by RAD51 assays (50, 51). Current HRD tests vary in the number and type of mutational features assessed, and the optimal thresholds to classify samples as HRD or HRP are not yet well defined. Variation in assays should be considered when evaluating the overall value of such assays in providing prognostic and predictive information.
The heterogeneity of HGSC, including multiple molecular subtypes even within the HRP subgroup, poses a substantial challenge to proper prognostication and clinical management (3, 33, 36, 43, 52, 53). Treatment options for patients with platinum-resistant, non-HRD HGSC are scarce, and the goal of treatment is strongly focused on symptom control and palliation, delaying time to symptomatic progression and improving quality of life (33, 53–57). To date, apart from the antibody-drug conjugate (ADC) mirvetuximab (Elahere®), few treatments in addition to cytoreductive surgery and platinum- and taxane-based chemotherapy have shown a survival benefit in this poor prognosis group (3, 33, 42, 53–57).
Recent novel approaches to treat ovarian cancer has largely benefitted patients with HRD HGSC, with or without BRCA-alterations (1, 3, 13, 17–29, 58). Further progress in the treatment of HGSC requires approaches that benefit patients with HRP disease, who currently have limited treatment options other than surgery. Here we summarize recent clinical and molecular findings in HRP HGSC and provide an insight into ongoing trials of new potential treatment options.
Variation in outcomes between patients with HGSC is in part determined by the molecular characteristics of the tumor, with HR-status as one of the important determinants (Table 1). Patients with HRP tumors have an older median age at diagnosis compared to patients with HRD tumors (10, 11, 20, 36). A retrospective analysis of 352 patients showed that HRP tumors required a higher number of cycles of NACT to be considered for interval cytoreductive surgery compared to those with germline BRCA mutations and other defects conferring HRD, and less complete gross resection (R0) could be achieved (11). While complete resection in primary and interval cytoreductive surgery remains one of the strongest prognostic features in ovarian cancer (2, 3, 62, 63), the higher number of chemotherapy cycles and lower R0 rate also reflect an inherently resistant tumor (18, 22–24, 26, 33).
Extensive genomic and transcriptomic characterization has provided insight into HGSC with HRR pathway inactivation, most commonly caused by genetic or epigenetic alterations in the BRCA genes and alterations in other genes, including BRIP1, PALB2, RAD51C, or RAD51D, which encode proteins that are also involved in HR DNA repair (59). By contrast, the molecular drivers of HGSC that have no apparent defects in HR are less well defined (2).
HRP ovarian cancer cells are often characterized by genetic alterations in signaling pathways that contribute to cell cycle dysregulation, such as cyclin E1 (encoded by CCNE1) and cyclin dependent kinase (CDK) genes (44). Cyclin E1 is an important factor in the G1/S cell cycle transition through its activation of cyclin-dependent kinase 2 (CDK2), allowing the cell to enter the S-phase (64). Besides other cellular mechanisms, limiting the supply of cyclin E1 ensures that the cell remains in the G1 phase by keeping CDK2 inactive until mitogenic signals intervene (65). CCNE1 expression is dependent on E2F transcription factors that are bound to the retinoblastoma protein (Rb) in an inactivated state when cells are at rest. E2F is released through mitogenic stimuli such as c-MYC which increases the expression of D-type cyclins that in turn combine with CDK4 and CDK6 to phosphorylate and inactivate Rb (65). Furthermore, once activated, the cyclin E1/CDK2 complex is able to phosphorylate Rb and thus upregulate its own expression in the form of a positive feedback loop through the continued release of E2F, independent of mitogenic stimuli (65). Additionally, the cyclin E1/CDK2 complex is an essential component of the chromatin remodeling process required for DNA replication. Overexpression of cyclin E1 increases the speed at which cancer cells transition from G1 to the S phase (66). This can lead to replicative stress, whole genome duplication, and further promote the dysregulation of genes responsible for proliferation and cell survival, which are also associated with resistance to cytotoxic and targeted therapies (67, 68).
CCNE1 amplification is currently the best characterized driver of HGSC with HR-proficiency. It is important to note, however, that cyclin E1 protein overexpression itself has not been shown to be a predictive biomarker for chemotherapy resistance in epithelial ovarian cancer (EOC), so methods to detect amplification of a gene (e.g. whole-genome sequencing, fluorescence in situ hybridization, polymerase chain reaction, single nucleotide polymorphism arrays) are required to identify the CCNE1 amplified subgroup (69). Approximately 40% of HRP HGSC show an CCNE1 amplification, which has been shown to be an early event in their development (43, 64). HR pathway gene mutations and CCNE1 amplification have been shown to be mutually exclusive (44, 60, 65). This suggests that the pathogenesis of HGSC follows at least two distinct pathways, and that CCNE1-amplified tumors with cyclin E1 protein overexpression are more likely to be resistant to platinum-based chemotherapy and PARPi due to HR-proficiency (65).
AKT2 amplification is also a poor prognostic marker in EOC (34, 70, 71) and is associated with CCNE1 amplification (70). The co-amplification of the serine/threonine-protein kinase AKT2 and CCNE1 appears to be explained in part by their proximity on chromosome 19q. Pathway analysis indicates that CCNE1-amplified cell lines are dependent on multiple genes within the CDK and AKT pathways, suggesting a specific dependence of CCNE1-amplified tumors on AKT activity (70). Consequently, combined CDK2 and AKT inhibition may have synergistic anti-tumor activity against CCNE1-amplified tumors and hold promise for clinical development (70). It should be noted that although CDK4/6 inhibitors have been investigated in ovarian cancer (72), it is the CDK2 inhibitor which is likely to be effective (73–76).
CDK12-altered HGSC represent a unique subgroup that appear to be HR competent (36). Despite lacking the typical HRD genomic scarring, CDK12-altered tumors have a distinct tandem duplication signature and may be more susceptible to chemotherapy and PARPis than other HRP tumors (77). Aside from alterations in CCNE1, AKT2 and CDK12, the majority of HRP HGSC remain poorly defined, and integration of genomic, immune, proteomic and functional data is needed for their complete characterization (78–81).
Tumor-infiltrating lymphocytes (TILs) are an established prognostic factor in ovarian cancer, regardless of the extent of surgical cytoreduction and chemotherapy (82–84). The presence of CD8+ TILs in the tumor microenvironment is associated with slower tumor progression, prolonged survival and may be essential for immunotherapy response (84–86). HRD tumors have a significantly increased CD8+/CD4+ ratio of TILs and a higher number of peritumoral T cells (44). This is likely due to HRD cells accumulating a high number of somatic mutations, which is predicted to result in the expression of more tumor neoantigens that elicit an adaptive immune response and cytotoxic T cell infiltration. These cells are capable of killing cancer cells (84), and in addition to a more favorable response to chemotherapy, explains the improved survival of patients with BRCA-mutated ovarian cancer.
By contrast, HRP tumors are characterized by a non-inflamed or “cold” immune phenotype, with fewer CD3+ and CD8+ TILs as well as decreased expression of PD-1 and PD-L1 (87–89). HRP tumors generally have a lower tumor mutational burden due to having intact DNA repair, which, together with a low TIL density, would predict a poor response to immune checkpoint blockade (84). Therefore, HRP tumors may be poor candidates for targeted immunotherapy with PD-1 and PD-L1 inhibitors as recently shown (90–96). Recent approaches to immunotherapy for cold tumors have focused on restoring inflammation by reprogramming myeloid cells, stromal cells, and vascular epithelial cells (97). Additionally, PARPi, low-dose radiotherapy, epigenetic drugs and anti-angiogenesis therapy may enhance T cell infiltration, suggesting their use in combination with vaccines and redirected T-cells using chimeric antigen receptors or bispecific antibodies (84, 98). However, it should be noted that while T cell infiltration and the expression of PD-L1 and other immune checkpoint markers increases following chemotherapy, unlike primary disease, the extent of infiltration does not correlate with patient survival (99, 100).
Neoadjuvant chemotherapy with interval cytoreductive surgery is currently an alternative for patients with ovarian cancer who have a low chance of initial complete resection and chemosensitive histologic subtypes, or poor health status (1). However, there is a strong correlation between HR-status and response to platinum-based chemotherapy in HGSC; patients with HRP tumors have severely limited responses to chemotherapy, with reported median PFS ranging from 5.4 to 16.9 months (Table 1) (10–13). The chemoresistant nature of HRP tumors highlights the potential benefit of favoring the currently recommended option in HGSC (1) of primary debulking surgery followed by adjuvant platinum and taxane-based chemotherapy in these patients.
An ancillary data analysis of the VELIA/GOG-3005 trial focused on paclitaxel dosing schedule and BRCA mutation and HR-status (101). Dose-dense (weekly) paclitaxel was compared to a schedule of every three weeks showing an improved PFS with dose-dense paclitaxel in HRP but not in BRCA-mutation or HRD tumors. Previous clinical trials of shorter versus longer paclitaxel intervals in ovarian cancer did not evaluate HR status and therefore further studies are needed to confirm this finding (102, 103). Interestingly, it has been shown that paclitaxel suppresses CDK1 expression via decreased BRCA1 phosphorylation, thereby reducing HR activity in response to DNA damage and increasing sensitivity to PARPis (104), so this combination represents a potential new treatment strategy that needs to be further investigated in HRP HGSC.
HGSC typically involves extensive peritoneal spread and therefore intraperitoneal chemotherapy and hyperthermic intraperitoneal chemotherapy (HIPEC) have been evaluated in multiple clinical trials. The goal of intraperitoneal chemotherapy is to increase local exposure to the chemotherapeutic agent, and in the case of HIPEC, heated chemotherapy has an additional cytotoxic effect and increases sensitivity to platinum compounds by inducing a transient state of HRD (105). Koole et al. analyzed the effect of HIPEC among patients with ovarian cancer previously enrolled in the phase III OVHIPEC1 trial (105) stratified by BRCA-like (HRD) versus non BRCA-like (HRP) (106) or BRCA mutation status. Although patients with HRD/BRCA-wildtype showed a strong benefit in terms of recurrence-free survival (RFS) and a promising trend in OS from HIPEC, this was non-significant in HRP/BRCA-wildtype patients and absent in patients with pathogenic BRCA mutations, both in terms of RFS and OS (58). It appears that HRP tumors remain resistant to chemotherapy despite hyperthermia. However, there is a lack of long-term survival data for HIPEC, and thus the benefit of this treatment modality remains unclear. The importance of tumor HR status in predicting response and survival following HIPEC may be addressed in ongoing studies (107).
Maintenance PARPi therapy after first-line treatment and in the platinum sensitive recurrent setting have become standard treatment options in patients with BRCA-mutated and HRD EOC (1, 3). PARP is an enzyme that helps repair DNA damage and PARP inhibition causes an accumulation of single- and double-stranded DNA breaks (108). HRD cells are unable to effectively repair the DNA damage, resulting in an accumulation of chromosomal aberrations and cell death (109). As a maintenance therapy PARPi have led to improved PFS and shown a promising trend towards improved OS in EOC, particularly in patients with BRCA mutant and/or HRD tumors (17, 18, 20, 21, 23, 24). While the greatest benefit is seen in HRD cancers, an exploratory analysis of the Phase III PRIMA trial showed improvements in PFS with niraparib versus placebo as first-line maintenance monotherapy, regardless of BRCA and HR-status (20). Patients with BRCA-wildtype/HRP tumors treated with niraparib who responded to first-line chemotherapy had a median PFS of 8.1 months versus 5.4 months for placebo, with an estimated probability of survival at 24 months of 81% in the niraparib group versus 59% in the placebo group. Therefore, niraparib is clinically approved for use in patients with HRP HGSC, with beneficial effects and a manageable tolerability profile (110, 111).
An exploratory analysis of the VELIA/GOG-3005 trial (27) showed that some patients with HRP ovarian cancer and also poor chemosensitivity may have gained a transient, but non-significant benefit from the addition of the PARPi veliparib to carboplatin-paclitaxel (median PFS 14.7 vs median 6.7 months, HR 0.62, 95% CI 0.37–1.05) (112). The authors of the study hypothesized that veliparib may have induced a chemosensitizing effect on HRP tumors (112, 113). In addition, the Phase III ATHENA-MONO trial demonstrated improved PFS with rucaparib monotherapy compared to placebo in first-line maintenance in patients with newly diagnosed EOC without evidence of HRD (12.1 vs 9.1 months, HR 0.65, 95% CI 0.45–0.95) (13). As a result of such findings, the ESGO-ESMO-ESP consensus guidelines state that niraparib or rucaparib maintenance therapy may be used for patients with HRP HGSC if they have had a complete or partial response to first line chemotherapy or no evidence of disease (1).
Vascular endothelial growth factor (VEGF) promotes increased vascularity and angiogenesis in response to hypoxic conditions and is a key promoter of tumor growth (114). The anti-angiogenic VEGF monoclonal antibody bevacizumab was the first targeted agent to be approved for use in stage III and IV EOC, showing an improved PFS when used in combination with chemotherapy and as maintenance therapy in the first-line setting, however without OS benefit (115, 116). According to the ESGO-ESMO-ESP consensus guidelines, patients with HRP HGSC may receive platinum-based chemotherapy with bevacizumab followed by bevacizumab maintenance as an alternative to the option of maintenance with rucaparib or niraparib (1). Among other mechanisms of action, bevacizumab exposure may trigger HRD by inducing a hypoxic cellular state that can downregulate HR-related genes such as BRCA1/2 and RAD51 (117). In addition, the relative benefit of bevacizumab in EOC has been shown to increase as the disease becomes more platinum resistant (118). A retrospective analysis of 124 patients with platinum-sensitive recurrent ovarian cancer showed extended PFS with bevacizumab in patients with cyclin E1 overexpression (median 16.3 vs 7.1 months, P=0.010) (118).
Tumor VEGF secretion has been shown to be at least partially responsible for the development and maintenance of ascites, and the AURELIA trial demonstrated that the addition of bevacizumab to chemotherapy improved ascites control. This beneficial effect is certainly relevant for the HRP group as they are more frequently associated with suboptimal debulking, earlier recurrence and ascites (54). Furthermore, the combination of niraparib and bevacizumab evaluated in the pre-specified subgroup analysis of the AVANOVA trial showed a significant improvement in PFS compared to niraparib alone in the HRP population (HR 0.40, 95% CI 0.19–0.85) (119). The Phase III GOG-218 trial also showed prolonged PFS in patients with no HRR gene mutations who received bevacizumab in addition to standard chemotherapy with carboplatin and paclitaxel (HR 0.71, 95% CI 0.60–0.85, P = 0.0001). This benefit was not observed in patients with HRR gene mutations (HR 0.95; 95% CI 0.71–1.26) (120). Therefore, the ESMO guidelines recommend that the decision on bevacizumab versus niraparib maintenance in the HRP population should be based on the patient’s disease and clinical characteristics, the toxicity profile of the two drug classes, the availability of each drug, and national guidelines (3, 121). The ongoing Phase I/II MITO 25 trial (NCT03462212) may provide clearer evidence about potential therapy options by comparing whether the carboplatin-paclitaxel-bevacizumab-rucaparib or carboplatin-paclitaxel-rucaparib arms improve PFS compared to standard carboplatin-paclitaxel-bevacizumab in patients with HRP HGSC.
The inhibition of VEGF receptor-3 (VEGFR3) has been shown to decrease BRCA1 and BRCA2 expression in ovarian cancer cells and resulted in increased chemosensitivity (122). The randomized Phase II trial (NCT01116648) showed that the combination of olaparib plus cediranib, a VEGF receptor 1/2/3 inhibitor, significantly improved PFS in relapsed platinum-sensitive EOC compared to olaparib alone (median 17.7 months vs 9 months, P=0.005), with the greatest benefit in BRCA-wildtype patients (HR 0.32, P=0.008) (123). These results suggest that there may be greater synergism between the two agents in HRP tumors, with the response to olaparib in HRP tumors being enhanced by diminished HRR due to VEGFR3 inhibition. However, experimental in vivo efficacy data showed that the combination exhibited broad anti-tumor activity independent of HRR and that the combination effect was largely driven by influencing independent mechanisms affecting tumor cells and the tumor microenvironment (124). Clinically, the combination of cediranib and olaparib also showed some activity in the CONVERTO trial, a single-arm Phase IIb study of the two compounds in heavily pretreated, platinum-resistant, non-germline BRCA-mutated patients. However, the target objective response rate (ORR) of 20% was not reached (15.6%) and the overall benefit was unclear (OS 13.2 months, 95% CI 9.4–16.4; PFS 5.1 months, 95% CI 3.5–5.5) given it was a single arm study in a disease setting where most patients are expected to progress or die within 12 months (125). A Phase III trial [NCT02446600] in patients with relapsed platinum-sensitive ovarian cancer found that neither the combination of olaparib and cediranib nor olaparib monotherapy improved PFS compared to standard chemotherapy (126). An ongoing Phase II/III trial (NCT02502266) is evaluating cediranib plus olaparib compared to their monotherapies and standard chemotherapy. It remains to be determined if there is a clinical benefit of VEGF receptor inhibitors in treating EOC, particularly in chemoresistant HRP tumors. Future research efforts must focus on identifying other predictive biomarkers for anti-angiogenic therapy, as not all observed responses can be explained by BRCA mutation or HR-status.
There have been significant advances in the surgical management of HGSC with improved PFS and OS due to intensification of surgical efforts (62, 127, 128). A multicenter, open-label, randomized, controlled Phase III trial SOC-1 (NCT01611766) demonstrated in 357 patients with platinum-sensitive relapsed ovarian cancer that secondary cytoreductive surgery (SCS) followed by chemotherapy was associated with significantly longer PFS than with chemotherapy alone (median 17.4 vs 11.9 months, HR 0.58, 95% CI 0.45–0.74, P<0.0001) (129). Furthermore, the DESKTOP III trial (NCT01166737) analyzed 407 patients with platinum-sensitive recurrent ovarian cancer and showed that SCS followed by chemotherapy leads to a longer OS than chemotherapy alone (median 53.7 vs 46.0 months, HR for death 0.75, 95% CI 0.59–0.96, P=0.02). Patients with a complete resection had the most favorable outcome (130). In addition to these two positive studies, in the GOG-0213 trial, which also included patients with platinum-sensitive, recurrent ovarian cancer, SCS followed by chemotherapy did not result in a longer OS than chemotherapy alone (131). There are some differences between the trials that may explain the inconsistent results, such as the additional use of bevacizumab in the DESKTOP III trial (NCT01166737) or the process of selecting patients and centers (130). Therefore, it is important that patients are appropriately counseled about the option of SCS.
The role of surgery in patients with platinum-resistant disease has received increasing attention (132). In fact, patients with HRP tumors may benefit from SCS, similar to patients with low-grade serous ovarian cancer (133). To our knowledge, only three retrospective studies have been published analyzing the role of SCS in patients with platinum-resistant recurrent ovarian cancer. Both Petrillo et al. and Musella et al. showed a prolonged OS after recurrence when SCS was combined with chemotherapy instead of chemotherapy alone (median 32 months vs 8 months, P=0.002 and 67 months vs 24 months, P=0.035) (134, 135). However, when evaluating these two studies, it is important to consider that they were carried out before the PARPi era and therefore their conclusions must be put into perspective with current treatment options. A recent multicenter retrospective series by Tuninetti et al. in 50 heavily pretreated platinum-resistant ovarian cancer patients showed a statistically significant longer OS in the group of patients who received complete cytoreduction after SCS compared to the very low survival of patients with residual disease (meidan 33 months vs 5 months, HR 4.21, 95% CI 2.07–8.60, P=0.001) (136). These retrospective studies did not include stratification by BRCA mutation or HR-status, and any discussion of the extent of surgical clearance should also consider how residual disease may be a marker of biology that drives outcome. However, in a recent multicenter retrospective study investigating platinum sensitive recurrent ovarian cancer, SCS was shown to be effective in BRCA-wildtype patients, with an improvement in post-recurrence survival (PRS) when complete resection was performed (5-year PRS of 54% vs 42%, P=0.048), whereas in BRCA-mutated patients, prognosis appears to be related to molecular tumor characteristics rather than tumor resectability (137). A current prospective randomized controlled trial (NCT05633199) is now comparing SCS in platinum-resistant recurrent ovarian cancer and is expected to provide further information on whether and to what extent SCS can be used in the “platinum-resistant” HRP HGSC subgroup. Another advantage of SCS is to opportunistically obtain more comprehensive information on the pathological and molecular characteristics of HRP HGSC and how this may affect tumor evolution and clinical outcome (127). SCS in HGSC warrants further investigation in prospective trials, with particular attention paid to patient BRCA and HR-status.
Immunotherapy for HGSC has fallen short of expectations, with immune checkpoint inhibitors so far showing limited benefit in ovarian cancer (138–142). However, there are new, potentially promising approaches, including ADCs that deliver a toxic ‘payload’ of chemotherapy directly to cancer cells via a linker attached to an antibody that binds to a specific surface antigen expressed on cancer cells (143). Mirvetuximab is a first-in-class ADC targeting folate receptor α (FRα), a cell surface protein that is commonly overexpressed on ovarian cancer (80–100%) and minimally expressed on normal tissue (144–146). This ADC incorporates the maytansinoid DM4 payload, a potent tubulin-targeting antimitotic agent, and is the first novel agent to demonstrate an OS benefit when used as a single agent compared to chemotherapy alone in platinum-resistant ovarian cancer, as shown in the MIRASOL Phase III clinical trial (NCT04209855) (144). Patients with platinum-resistant, FRα-positive ovarian cancer treated with mirvetuximab (n=227) experienced an OS of 16.46 months (95% CI, 4.46–24.57) vs 12.75 months (95% CI, 10.91–14.36) for the chemotherapy arm (HR 0.67, 95% CI 0.50–0.89, P=0.005) and showed fewer Grade 3 or higher adverse events with mirvetuximab than with chemotherapy (41.7% vs 54.1%).
Another promising immunotherapy approach is Gemogenovatucel-T (Vigil, formerly known as FANG®), the first immunotherapy to demonstrate specific efficacy in the frontline maintenance setting for the HRP population. Vigil is a vaccine composed of autologous tumor cells derived from malignant tissue removed during cytoreductive surgery (147) (Figure 1). Tumor cells are transfected with a plasmid containing GM-CSF and bi-shRNA to reduce furin activity, which subsequently downregulates the expression of the immunosuppressive proteins TGF-β1 and TGF-β2 (transforming growth factor ). This is important because the expression of furin and the resulting immunosuppressive TGF-β isoforms are increased in ovarian tumors compared to normal ovarian tissue (148). Long-term safety of Vigil and evidence of patient benefit have been demonstrated in multiple solid tumors, including advanced ovarian cancer (149, 150). The ongoing Phase IIb VITAL trial (NCT02346747) evaluated the efficacy of Vigil in patients with stage III/IV ovarian cancer. RFS was 11.5 months for patients treated with Vigil versus 8.4 months for patients treated with placebo (HR 0.69, 90% CI 0.44–1.07, P=0.078) with an acceptable toxicity profile (151). Although the primary endpoint of RFS was not met, a small subgroup analysis (n=45) showed that RFS and OS was significantly improved with Vigil compared to placebo in HRP patients (HR 0.38 and 0.34, 90% CI 0.2–0.75 and 0.14–0.83, P=0.007 and P=0.019), while no difference was seen in patients with BRCA-mutated disease (151, 152). Vigil increases the expression of cancer-associated neoantigens by upregulating MHC-II and processing by dendritic cells, which enhances the afferent immune response, the initial phase of immune activation characterized by antigen presentation and recognition, resulting in a systemic anti-tumor immune response including CD3+/CD8+ T cell circulation (152). T cells showed to preferentially recognize clonal neoantigens over subclonal neoantigens to target the tumor in lung adenocarcinoma and melanoma (153). HRP tumors are associated with higher clonal neoantigen expression compared to HRD tumors, which therefore contain higher proportions of subclonal neoantigen subpopulations, which may explain why Vigil is more effective on HRP tumors (152). A Phase III trial is planned to validate the efficacy of Vigil compared to bevacizumab and niraparib in the HRP ovarian cancer population (152). It has been suggested that the increased expression of clonal tumor neoantigens and reduced tumor suppressive effect of TGF-β may synergistically enhance the activity of checkpoint inhibitor treatment (84, 154, 155). A prospective, randomized Phase I trial of Vigil plus the immune checkpoint inhibitor atezolizumab in patients with recurrent ovarian cancer explored this approach and showed that the combination was safe, supporting further investigation of this combination, particularly in BRCA-wildtype patients (155).
Figure 1 Immune profile of HRP vs. HRD tumors and effect of Vigil. HRP tumors show reduced immunophenotypic markers compared to HRD tumors. Gemogenovatucel-T (Vigil) is a vaccine composed of autologous tumor cells transfected with a plasmid containing GM-CSF and bi-shRNA resulting in a systemic anti-tumor immune response including CD8+ T cell circulation. HRP tumors have a higher proportion of clonal neoantigen expression, which explains the better effect of Vigil on HRP tumors compared to HRD. HRP, Homologous recombination proficient; HRD, Homologous recombination deficient; TILs, Tumor-infiltrating lymphocytes; Bi-shRNA, Bifunctional short hairpin RNA; GM-CSF, Granulocyte/Macrophage Colony Stimulating Factor; TGF, Transforming growth factor; APC, Antigen presenting cell.
Adoptive cell therapy is another emerging personalized form of immunotherapy in which patients are treated with their ex vivo expanded natural TILs, genetically engineered T lymphocytes (CAR T cells) or T-cell receptor (TCR)-engineered T cells, which could offer a potential therapeutic option for patients with cold tumors. To date, CAR T cells that have been tested in clinical trials for HGSC have not yet demonstrated clear benefit (84, 156). While this technology is promising, further development is required to investigate the full potential of T cell engineering and other novel immunotherapy approaches to address the problem of immunologically cold tumors (84).
Rational drug combinations are a potential strategy to prevent or delay the development of resistance and offer the opportunity to improve the therapeutic window by potentially reducing the required drug doses, resulting in fewer side effects (70). Several strategies to selectively disrupt HRR in cancer cells with drugs have been investigated both preclinically and in clinical trials in HGSC or EOC in general, including HRP tumors, and have provided the rationale for new potential therapeutic approaches (Figure 2, Table 2). Here we review the most promising approaches for HRP tumors that have been or are being investigated in ovarian cancer, including targeting the CDK, P13K/AKT or CHK pathways.
Figure 2 Potential combination therapies to induce homologous recombination deficiency (HRD). VEGFR3i, Vascular endothelial growth factor receptor 3 inhibitor; EGFRi, Epidermal growth factor receptor inhibitor; CDKi, Cyclin-Dependent Kinases inhibitor; WEE1i, Weel-like kinase 1 inhibitor; PI3K, Phosphatidylinositol-3-Kinase and AKT, Serine/threonine protein kinases; ATRi, Ataxia teleangiectasia Rad3-related inhibitor; HDACi, Histone deacetylase inhibitor; HSP90i, Heat shock protein 90 inhibitor; BETi, Brodomain and extraterminal protein family inhibitor.
Approximately 40% of HGSC with HR-proficiency have an amplification of CCNE1 (64). Cyclins are typically regulatory proteins that modulate the activity of CDKs (65). The CDK pathway offers attractive targets for the treatment of CCNE1-amplified tumors due to its role as the kinase partner of cyclin E1 in the activated cyclin E1/CDK complex (65, 163) (Figure 3). Cyclin E1 is primarily regulated by CDK2 in CCNE1-amplified tumors, which are selectively dependent on CDK2 activity (73). Combination therapy with the multi-CDK inhibitor dinaciclib (targets CDK1/2/5/9) has shown positive preclinical responses in CCNE1-amplified HGSC (164–166), and there is currently an active but not recruiting Phase I trial [NCT01434316] evaluating dinaciclib in combination with the PARPi veliparib in advanced solid tumors. However, a disadvantage of broad-spectrum CDK inhibitors is their high toxicity (167). Recently, more selective CDK2 inhibitors have been investigated (74–76), including promising preclinical results using INX-315, a novel, potent and highly selective CDK2 inhibitor. INX-315 treatment resulted in tumor growth inhibition of CCNE1-amplified tumors by promoting retinoblastoma protein hypophosphorylation, inducing cell cycle arrest and delaying the onset of CDK4/6 inhibitor resistance in breast cancer (74). In addition, a recent first-in-human Phase I/IIa study (NCT04553133) of a novel and potent selective CDK2i (PF-07104091) found that it was well tolerated and showed antitumor activity in heavily pretreated metastatic breast cancer patients who had progressed on prior CDK4/6 inhibitors (75). Further development of selective CDK2 inhibitors in Phase I/II clinical trials are ongoing and may be of major importance for HRP HGSC.
Figure 3 CDK/cyclin E1 complex including targets for therapy. Activation of CDK2 by cyclin E1, allowing the cell to enter the S phase. Overexpression of cyclin E1 increases the rate at which cancer cells transition from G1 to S phase, leading to replicative stress and genomic instability. The WEE1 kinase is involved in regulating cell cycle progression by inhibiting CDK1 and CDK2 and WEE1 inhibition leads to cell cycle acceleration, with early mitotic entry and consequent mitotic catastrophe leading to irreparable DNA damage. CDK1 phosphorylates BRCA1 and CDK1 inhibition impairs the ability of cells to carry out functional DNA repair through homologous recombination. PKMYT1 encodes the protein kinase Myt1, a negative regulator of CDK1.
Another strategy is to target Weel-like kinase (WEE1), which is highly upregulated in HGSC (108). Its inhibition causes activation of CDK1 and CDK2, resulting in cell cycle acceleration with an early mitotic entry and mitotic catastrophe leading to irreparable DNA damage (121). The multicenter Phase II IGNITE trial [ACTRN12619001185156P] is a non-comparative trial evaluating the WEE1-inhibitor adavosertib in two cohorts of platinum resistant recurrent HGSC (cyclin E1 overexpressed/CCNE1 amplified and cyclin E1 overexpressed/CCNE1 non-amplified) and demonstrated an ORR of 53% and a clinical benefit of 61% in an interim analysis of 32 patients in the cyclin E1 overexpressed/CCNE1 non-amplified cohort (168). CDK1 is a key cell-cycle regulator and phosphorylates BRCA1, which is required for DNA damage-induced checkpoint control through the formation of BRCA1-containing foci (169); consequently, inhibition of CDK1 impairs the ability of cells to functionally repair DNA by HRR (165). Therefore, depletion or inhibition of CDK1 creates a state of “BRCAness” in transformed cells (170). Results from preclinical studies in other cancer modalities support the effect of WEE1 inhibition on HR, and thus the assumption that WEE1 inhibitors, in combination with a DNA damaging agent, specifically render HRP cell lines more susceptible to treatment (171, 172).
An ongoing Phase II trial (NCT03579316) in recurrent PARPi-resistant EOC (including 98% HGSC) is evaluating the efficacy of the WEE1 inhibitor adavosertib with or without olaparib. The combination showed to have a greater clinical benefit rate than adavosertib alone (89% vs 63%), but the ORR was similar between the two arms (160). Interestingly, exploratory analyses showed a larger benefit of the combination in the BRCA-wildtype subgroup compared to the BRCA-mutated subgroup (39% vs 19% ORR). Translational analyses are underway to further explore potential predictive biomarkers (160). However, adavosertib use requires consideration of single agent toxicity as well as interactions when used as a drug combination. For example, the use of adavosertib in combination with carboplatin showed an increased incidence of bone marrow suppression, diarrhea, vomiting and fatigue (168, 173). Additionally, adavosertib is metabolized via the enzyme cytochrome P450 3A4 (CYP3A4), which means that patients receiving any co-medications that are strong CYP3A4 inhibitors (for example, antibacterials such as clarithromycin and erythromycin, anticancer agents such as tamoxifen and irinotecan, anti-HIV agents such as ritonavir and delavirdine, or antihypertensives such as dihydro-dralazine and verapamil) (174) would be excluded from clinical trials.
Another promising therapeutic target of the CDK pathway specifically for CCNE1-amplified HGSC is PKMYT1 (68). PKMYT1 is a kinase encoding the pro-protein kinase Myt1, a negative regulator of CDK1, and was identified in a genetic screen of cellular dependencies in CCNE1 amplified HGSC (68). Inhibition of PKMYT1 results in activation of CDK1, causing unscheduled mitotic entry and genome instability. In contrast, the WEE1inhibitor showed no selectivity towards CCNE1-amplified cell lines (175, 176). Ongoing first-in-human clinical trials are evaluating the PKMYT1 inhibitor lunresertib (RP-6306) as monotherapy or in combination with the ataxia-telangiectasia Rad3-related (ATR) inhibitor RP-3500 (NCT04855656) and in combination with gemcitabine (NCT05147272) in advanced solid tumors.
Phosphatidylinositol 3-kinase (PI3K) activity is stimulated by a wide range of oncogenes and growth factor receptors (177) and the activity of the PI3K pathway is important to the development of drug resistance in a variety of cancer types and treatment settings (178). Inhibition of the PI3K pathway also results in PI3K-mediated downregulation of BRCA, accompanied by extracellular signal-regulated kinase (ERK) phosphorylation and subsequent abrogation of HRR (179). Preclinical work in ovarian cancer patient-derived xenograft models has shown that the PI3K inhibitor alpelisib (BYL719) inhibits HRR and consequently sensitizes ovarian cancer models with de novo or acquired HR-proficiency to olaparib (180). A Phase I study in 28 patients with EOC (75% HGSC) provided preliminary clinical evidence of the efficacy of the combination of olaparib and alpelisib. An ORR of 33% was seen in patients with BRCA-wildtype platinum-resistant EOC compared to an ORR of 3–10% with olaparib or other PARPi monotherapy in the same setting, and with acceptable toxicity (121, 181). Importantly, objective responses to this combination of agents occurred regardless of HR status, as measured by targeted DNA sequencing (181). Further evidence will be provided by the ongoing Phase III EPIK-O/ENGOT-OV61 trial (NCT04729387), which is evaluating the efficacy and safety of alpelisib/olaparib compared to single-agent cytotoxic chemotherapy in patients with platinum-resistant or refractory BRCA-wildtype HGSC (161).
The AKT serine/threonine protein kinases (AKT1, AKT2, AKT3) are key downstream mediators of PI3K signaling (182, 183) and in particular, AKT2 has emerged as a poor prognostic marker and potential target in EOC (34, 70, 71). Drugs targeting AKT have shown activity in breast, endometrial, and ovarian cancer and are currently being investigated in Phase I/II/III trials (183, 184). An active Phase Ib/II trial (NCT02208375) is evaluating the combination of olaparib and the AKT inhibitor capivasertib (AZD5363) in a heavily pretreated cohort of 159 patients, with encouraging clinical activity regardless of the presence of a BRCA mutation and despite platinum resistance (183). Further studies are needed to explore the potential of AKT and PI3K inhibitors in combination with PARPi or as monotherapy in HRP HGSC and ovarian cancer in general.
ATR has a major role in the CHK1 (checkpoint kinase 1) pathway of DNA repair and is a regulator of several proteins in the HRR pathway, including activation of BRCA1, PALB2 and RAD51 (108). The potential for mechanistic synergism between ATR inhibitors (ATRi) and PARPis has been demonstrated in HRD and HRP ovarian cancer cells in preclinical models (108, 185, 186). Acquisition of PARPi resistance was shown to be associated with increased ATR-CHK1 activity, further supporting the potential benefit of combining of PARPis with ATR inhibitors (185). Patient-derived xenograft models of BRCA-wildtype and CCNE1-amplified platinum-resistant ovarian cancer, which are associated with increased baseline activation of ATR/CHK1, demonstrated tumor reduction and a significant increase in OS when treated with the combination of PARPi and ATRi (185). Based on these preclinical data, an ongoing Phase II clinical trial of ceralasertib (AZD6738) in combination with olaparib was developed and initial results demonstrated the potential of ATRi to overcome PARPi resistance in an HRD HGSC patient population (187).
ATRis are also being investigated as potential monotherapy, and preliminary anti-tumor activity has been demonstrated in heavily pretreated tumors across a range of histologic types and gene alterations (188). Initial results from TRESR, a phase I trial of ATRi monotherapy with camonsertib, support preclinical findings that ATRi may be clinically active in other patient populations beyond those with loss of function of ataxia telangiectasia mutated (ATM) kinase, including those with other gene alterations (e.g., ARID1A, CCNE1, and MYC) or phenotypic (replication) markers (188, 189). The functional assessment of replication stress biomarkers is thought to be a better predictive biomarker for ATRi response than single aberrant genes in ovarian cancer (190). This statement can also be applied to the selective CHK1/2 inhibitor prexasertib, which showed an increased sensitivity to platinum and olaparib in mouse tumor transplantation models and monotherapy efficacy in BRCA-wildtype platinum-resistant ovarian cancer (191, 192). To date, however, there is limited data on the safety and anti-tumor activity of CHK inhibitors, and a phase II trial of prexasertib was recently terminated prematurely due to COVID-19 and a shortage of investigational drug supplies (193).
The altered expression of HDACs (histone deacetylases) has been associated with resistance to platinum-based chemotherapy and poor prognosis (194) and HDAC inhibition leads to impaired HRR in cancer cells through reduced expression of critical genes such as BRCA1 and RAD51 (195, 196). Konstantinopoulos et al. provided a preclinical rationale for the use of HDAC inhibitors (HDACi) to reduce HRR in HRP ovarian cancer, including CCNE1-amplified tumors, as a means to enhance PARPi activity (197). This approach has been confirmed by further preclinical studies showing that HDACi such as suberoylanilide hydroamic acid (SAHA), romidepsin, panobinostat and entinostat are synergistic with PARPi in HRP ovarian cancer cells (197, 198). HDACi downregulate genes in the cyclin E/CDK and HR signaling pathways and thus show a synergistic cytotoxic effect in combination with a PARPi (198–200). Based on these preclinical results, there is an ongoing Phase I dose-escalation trial (NCT04703920) of the combination of the PARPi talazoparib and the HDACi belinostat in metastatic ovarian, breast and prostate cancer.
Another attempt to extend the benefit of PARPis to HRP patients is their combination with the heat shock protein 90 (HSP90) inhibitors. HSP90 mediates the maturation, stability and activation of several key proteins involved in DNA repair and HRR, such as CDK1, BRCA1 and BRCA2 (201). Due to its abundant expression, its dependence on adenosine ATP (adenosine triphosphate), and its massive protein interactome, it is an ideal target for pharmacological inhibition (201). Inhibition of HSP90 by ganetespib (STA-9090), a second-generation HSP90 inhibitor, sensitized HRP HGSC cells to talazoparib (201). HSP90 inhibition resulted in downregulation of BRCA1 and RAD51, HRR impairment and increased DNA damage (202). A recent Phase I dose-escalation study showed that the combination of the HSP90i onalespib and olaparib resulted in prolonged disease stabilization, without dose limiting toxicities, in a heavily pretreated patient population with advanced solid tumors (162). Due to limited efficacy as a monotherapy and in other combination studies, further development of onalespib was discontinued (162). However, preclinical and clinical data may support future evaluation of novel combinations of PARPis with other HSP90 inhibitors, such as pimitespib (203). While HSP90 inhibition has the potential to sensitize HRP HGSC to PARPi and other DNA-damaging agents, further clinical research is needed.
The BET (bromodomain and extraterminal) protein family includes BRD4, an epigenetic transcription modulator involved in the expression of proteins that regulate the cell cycle and DNA repair (204). BRD4 has been shown to be a necessary factor for the proliferation and survival of HGSC cells (205). In addition, BRD4 amplification is mutually exclusive with BRCA1 and BRCA2 mutations and tends to co-occur with CCNE1 amplification in HGSC, so BET inhibition may be particularly promising in the HRP group (38, 206–208). Preclinical studies have shown that BET inhibitors (BETis) suppress the expression of WEE1 and TOPBP1 (DNA Topoisomerase II Binding Protein 1) (209, 210). WEE1 and TOPBP1 play critical roles in cellular processes related to DNA damage response and cell cycle regulation. WEE1 is a protein kinase that regulates the G2/M checkpoint in the cell cycle, controlling entry into mitosis and allowing time for DNA repair (173, 176, 211, 212). TOPBP1 acts as a scaffold protein that coordinates the activation of ATR kinase in response to DNA damage, thereby initiating signaling cascades essential for DNA repair and cell cycle arrest (213). Dysfunction or dysregulation of these proteins can lead to genomic instability and contribute to the development of diseases such as cancer. Additionally, increased BRD4 expression has been identified as a factor contributing to PARPi resistance in HGSC (210). The specific BRD4 inhibitor INCB054329 was able to directly decrease the activity of both BRCA1 and RAD51 and induce an HRD phenotype (108, 209). Consequently, in combination with PARPis, a synergistic effect is observed with decreased HR activity, increased DNA damage, and consequently increased tumor cytotoxicity (108, 214). Unfortunately, initial clinical studies involving single agent use of BET inhibitors in various tumor types were disappointing, as preclinical results could not be replicated and resistance to therapy occurred rapidly in some cases (215). Specific evidence in ovarian cancer will be provided by an ongoing Phase II clinical trial (NCT05071937) of the BETi ZEN003694 in combination with the PARPi talazoparib in patients with recurrent ovarian cancer who have progressed on prior PARPi therapy.
The HRP HGSC subgroup exhibits complex molecular heterogeneity combined with an immune depleted microenvironment, and these are associated with therapy resistance and a poor prognosis. A subset of these cancers are driven by CCNE1 amplification and PI3K/AKT alterations that contribute to cell cycle dysregulation and thus these pathways represent promising targets for novel therapeutic approaches. However, a significant subset of HRP HGSC lack CCNE1 amplification, and the molecular drivers of these cancers are still being defined. Additional studies, including the use of cell lines and potentially the use of existing data from systematic knockdown and knockout genetic screens (216, 217) in the HRP non-CCNE1 amplified subgroup may define critical dependencies.
A large proportion of HRP HGSC are relatively immune depleted, likely in part due to a reduced mutational burden associated with intact DNA repair. The development of novel immunotherapies to boost the anti-tumor immune response remains a key area of focus for HRP tumors, including personalized approaches to enhance T-cell infiltration with therapeutic vaccines or adoptive cell therapy. Several new combination treatments are under investigation, which aim to sensitize HRP cancers to existing therapies, such as platinum and PARPis, by targeting the HRR pathway and impairing the ability of cells to functionally repair DNA. Antibody drug conjugates also represent a promising class of therapies to increase the potency and specificity of highly potent cytotoxic agents, while reducing toxicity.
These new approaches offer the opportunity to expand the otherwise very limited treatment options for patients with HRP HGSC. Importantly, explicit identification and enrollment of patients with HGSC tumors known to have intact HRR in clinical trials is crucial for the development of effective therapies for this medically underserved group.
NS: Writing – review & editing, Writing – original draft, Visualization, Methodology, Investigation, Formal analysis, Data curation. DG: Writing – review & editing, Validation, Supervision, Resources, Methodology, Investigation, Funding acquisition. GA-Y: Writing – review & editing, Validation, Supervision, Resources, Project administration, Methodology. DB: Writing – review & editing, Supervision, Resources, Project administration, Methodology. VH-S: Writing – review & editing, Writing – original draft, Supervision, Resources, Project administration, Methodology, Conceptualization. TZ: Writing – review & editing, Writing – original draft, Visualization, Supervision, Resources, Project administration, Methodology, Investigation, Funding acquisition, Formal analysis, Data curation, Conceptualization.
The author(s) declare financial support was received for the research, authorship, and/or publication of this article. This work was supported by the Swiss National Foundation (P500PM_20726/1, 2021), Bangerter-Rhyner Stiftung (0297, 2021), and Freie Gesellschaft Basel (2022) to TZ. DG is supported by a Victorian Cancer Agency/Ovarian Cancer Australia Low-Survival Cancer Philanthropic Mid-Career Research Fellowship (MCRF22018), and the National Health and Medical Research Council (NHMRC) of Australia (1186505 and 2029088).
DB reports research support grants from AstraZeneca, Roche-Genentech and BeiGene paid to institution outside the submitted work; also personal consulting fees from Exo Therapeutics, that are outside the submitted work. GA-Y reports research support grants from AstraZeneca and Roche-Genentech paid to institution outside the submitted work.
The remaining authors declare that the research was conducted in the absence of any commercial or financial relationships that could be construed as a potential conflict of interest.
All claims expressed in this article are solely those of the authors and do not necessarily represent those of their affiliated organizations, or those of the publisher, the editors and the reviewers. Any product that may be evaluated in this article, or claim that may be made by its manufacturer, is not guaranteed or endorsed by the publisher.
The Supplementary Material for this article can be found online at: https://www.frontiersin.org/articles/10.3389/fonc.2024.1387281/full#supplementary-material
1. Ledermann JA, Matias-Guiu X, Amant F, Concin N, Davidson B, Fotopoulou C, et al. ESGO-ESMO-ESP consensus conference recommendations on ovarian cancer: pathology and molecular biology and early, advanced and recurrent disease. Ann Oncol. (2024) 35(3):248–66. doi: 10.1016/j.annonc.2023.11.015
2. Bowtell DD, Böhm S, Ahmed AA, Aspuria PJ, Bast RC Jr., Beral V, et al. Rethinking ovarian cancer II: reducing mortality from high-grade serous ovarian cancer. . Nat Rev Cancer. (2015) 15(11):668–79. doi: 10.1038/nrc4019
3. González-Martín A, Harter P, Leary A, Lorusso D, Miller RE, Pothuri B, et al. Newly diagnosed and relapsed epithelial ovarian cancer: ESMO Clinical Practice Guideline for diagnosis, treatment and follow-up. Ann Oncol. (2023) 34(10):833–48. doi: 10.1016/j.annonc.2023.07.011
4. Vergote I, Tropé CG, Amant F, Kristensen GB, Ehlen T, Johnson N, et al. Neoadjuvant chemotherapy primary Surg stage IIIC IV Ovarian cancer. N Engl J Med. (2010) 363(10):943–53. doi: 10.1056/NEJMoa0908806
5. Kehoe S, Hook J, Nankivell M, Jayson GC, Kitchener H, Lopes T, et al. Primary chemotherapy versus primary surgery for newly diagnosed advanced ovarian cancer (CHORUS): an open-label, randomised, controlled, non-inferiority trial. Lancet. (9990) 2015:386. doi: 10.1016/s0140-6736(14)62223-6
6. Onda T, Satoh T, Ogawa G, Saito T, Kasamatsu T, Nakanishi T, et al. Comparison of survival between primary debulking surgery and neoadjuvant chemotherapy for stage III/IV ovarian, tubal and peritoneal cancers in phase III randomised trial. Eur J Cancer. (2020) 130:114–25. doi: 10.1016/j.ejca.2020.02.020
7. Fagotti A, Ferrandina MG, Vizzielli G, Pasciuto T, Fanfani F, Gallotta V, et al. Randomized trial of primary debulking surgery versus neoadjuvant chemotherapy for advanced epithelial ovarian cancer (SCORPION-NCT01461850). . Int J Gynecol Cancer. (2020) 30(11):1657–64. doi: 10.1136/ijgc-2020-001640
8. Melamed A, Rauh-Hain JA, Gockley AA, Nitecki R, Ramirez PT, Hershman DL, et al. Association Between Overall Survival and the Tendency for Cancer Programs to Administer Neoadjuvant Chemotherapy for Patients With Advanced Ovarian Cancer. JAMA Oncol. (2021) 7(12):1782–90. doi: 10.1001/jamaoncol.2021.4252
9. Coleridge SL, Bryant A, Kehoe S, Morrison J. Neoadjuvant chemotherapy before surgery versus surgery followed by chemotherapy for initial treatment in advanced ovarian epithelial cancer. Cochrane Database Syst Rev. (2021) 7(7):Cd005343. doi: 10.1002/14651858.CD005343.pub6
10. Sims T, Floyd J, Sood A, Westin S, Fellman B, Unke J, et al. Correlation of BRCA and HRD status with clinical and survival outcomes in patients with advanced-stage ovarian cancer in the age of PARPi maintenance therapy (187). Gynecologic Oncol. (2022) 166:S107–S8. doi: 10.1016/S0090-8258(22)01414-7
11. Sims TT, Sood AK, Westin SN, Fellman BM, Unke J, Rangel KM, et al. Correlation of HRD status with clinical and survival outcomes in patients with advanced-stage ovarian cancer. J Clin Oncol. (2021) 39(15_suppl):5568. doi: 10.1200/JCO.2021.39.15_suppl.5568
12. González-Martín A, Pothuri B, Vergote I, Graybill W, Lorusso D, McCormick CC, et al. Progression-free survival and safety at 3.5years of follow-up: results from the randomised phase 3 PRIMA/ENGOT-OV26/GOG-3012 trial of niraparib maintenance treatment in patients with newly diagnosed ovarian cancer. Eur J Cancer. (2023) 189:112908. doi: 10.1016/j.ejca.2023.04.024
13. Monk BJ, Parkinson C, Lim MC, O'Malley DM, Oaknin A, Wilson MK, et al. A Randomized, Phase III Trial to Evaluate Rucaparib Monotherapy as Maintenance Treatment in Patients With Newly Diagnosed Ovarian Cancer (ATHENA-MONO/GOG-3020/ENGOT-ov45). J Clin Oncol. (2022) 40(34):3952–64. doi: 10.1200/jco.22.01003
14. Vaughan S, Coward JI, Bast RC Jr., Berchuck A, Berek JS, Brenton JD, et al. Rethinking ovarian cancer: recommendations for improving outcomes. Nat Rev Cancer. (2011) 11(10):719–25. doi: 10.1038/nrc3144
15. Cabasag CJ, Arnold M, Rutherford M, Ferlay J, Bardot A, Morgan E, et al. Shifting incidence and survival of epithelial ovarian cancer (1995-2014): A SurvMark-2 study. Int J Cancer. (2023) 152(9):1763–77. doi: 10.1002/ijc.34403
16. Network CSE. Ovarian Cancer Survival Rates 2004-2020. Available at: https://seer.cancer.gov/statistics-network/explorer/application.html?site=61&data_type=4&graph_type=2&compareBy=relative_survival_interval&chk_relative_survival_interval_1=1&chk_relative_survival_interval_3=3&chk_relative_survival_interval_5=5&hdn_sex=3&race=1&age_range=1&stage=101&advopt_precision=1&advopt_show_ci=on&hdn_view=0&advopt_show_apc=on&advopt_display=2#resultsRegion0.
17. Ray-Coquard I, Leary A, Pignata S, Cropet C, González-Martín A, Marth C, et al. Olaparib plus bevacizumab first-line maintenance in ovarian cancer: final overall survival results from the PAOLA-1/ENGOT-ov25 trial. Ann Oncol. (2023) 34(8):681–92. doi: 10.1016/j.annonc.2023.05.005
18. Ray-Coquard I, Pautier P, Pignata S, Pérol D, González-Martín A, Berger R, et al. Olaparib plus Bevacizumab as First-Line Maintenance in Ovarian Cancer. N Engl J Med. (2019) 381(25):2416–28. doi: 10.1056/NEJMoa1911361
19. Moore K, Colombo N, Scambia G, Kim BG, Oaknin A, Friedlander M, et al. Maintenance Olaparib in Patients with Newly Diagnosed Advanced Ovarian Cancer. N Engl J Med. (2018) 379(26):2495–505. doi: 10.1056/NEJMoa1810858
20. González-Martín A, Pothuri B, Vergote I, DePont Christensen R, Graybill W, Mirza MR, et al. Niraparib in Patients with Newly Diagnosed Advanced Ovarian Cancer. N Engl J Med. (2019) 381(25):2391–402. doi: 10.1056/NEJMoa1910962
21. González-Martín A, Desauw C, Heitz F, Cropet C, Gargiulo P, Berger R, et al. Maintenance olaparib plus bevacizumab in patients with newly diagnosed advanced high-grade ovarian cancer: Main analysis of second progression-free survival in the phase III PAOLA-1/ENGOT-ov25 trial. Eur J Cancer. (2022) 174:221–31. doi: 10.1016/j.ejca.2022.07.022
22. DiSilvestro P, Banerjee S, Colombo N, Scambia G, Kim BG, Oaknin A, et al. Overall Survival With Maintenance Olaparib at a 7-Year Follow-Up in Patients With Newly Diagnosed Advanced Ovarian Cancer and a BRCA Mutation: The SOLO1/GOG 3004 Trial. J Clin Oncol. (2023) 41(3):609–17. doi: 10.1200/jco.22.01549
23. Banerjee S, Moore KN, Colombo N, Scambia G, Kim BG, Oaknin A, et al. Maintenance olaparib for patients with newly diagnosed advanced ovarian cancer and a BRCA mutation (SOLO1/GOG 3004): 5-year follow-up of a randomised, double-blind, placebo-controlled, phase 3 trial. Lancet Oncol. (2021) 22(12):1721–31. doi: 10.1016/s1470-2045(21)00531-3
24. Swisher EM, Lin KK, Oza AM, Scott CL, Giordano H, Sun J, et al. Rucaparib in relapsed, platinum-sensitive high-grade ovarian carcinoma (ARIEL2 Part 1): an international, multicentre, open-label, phase 2 trial. Lancet Oncol. (2017) 18(1):75–87. doi: 10.1016/s1470-2045(16)30559-9
25. Monk BJ, Coleman RL, Fujiwara K, Wilson MK, Oza AM, Oaknin A, et al. ATHENA (GOG-3020/ENGOT-ov45): a randomized, phase III trial to evaluate rucaparib as monotherapy (ATHENA-MONO) and rucaparib in combination with nivolumab (ATHENA-COMBO) as maintenance treatment following frontline platinum-based chemotherapy in ovarian cancer. Int J Gynecol Cancer. (2021) 31(12):1589–94. doi: 10.1136/ijgc-2021-002933
26. Coleman RL, Oza AM, Lorusso D, Aghajanian C, Oaknin A, Dean A, et al. Rucaparib maintenance treatment for recurrent ovarian carcinoma after response to platinum therapy (ARIEL3): a randomised, double-blind, placebo-controlled, phase 3 trial. Lancet. (2017) 390(10106):1949–61. doi: 10.1016/s0140-6736(17)32440-6
27. Coleman RL, Fleming GF, Brady MF, Swisher EM, Steffensen KD, Friedlander M, et al. Veliparib with First-Line Chemotherapy and as Maintenance Therapy in Ovarian Cancer. N Engl J Med. (2019) 381(25):2403–15. doi: 10.1056/NEJMoa1909707
28. Farmer H, McCabe N, Lord CJ, Tutt AN, Johnson DA, Richardson TB, et al. Targeting the DNA repair defect in BRCA mutant cells as a therapeutic strategy. Nature. (2005) 434(7035):917–21. doi: 10.1038/nature03445
29. Tattersall A, Ryan N, Wiggans AJ, Rogozińska E, Morrison J. Poly(ADP-ribose) polymerase (PARP) inhibitors for the treatment of ovarian cancer. Cochrane Database Syst Rev. (2022) 2(2):Cd007929. doi: 10.1002/14651858.CD007929.pub4
30. Nguyen L, WMM J, Van Hoeck A, Cuppen E. Pan-cancer landscape of homologous recombination deficiency. Nat Commun. (2020) 11(1):5584. doi: 10.1038/s41467-020-19406-4
31. Marquard AM, Eklund AC, Joshi T, Krzystanek M, Favero F, Wang ZC, et al. Pan-cancer analysis of genomic scar signatures associated with homologous recombination deficiency suggests novel indications for existing cancer drugs. biomark Res. (2015) 3:9. doi: 10.1186/s40364-015-0033-4
32. Radhakrishnan SK, Jette N, Lees-Miller SP. Non-homologous end joining: Emerging themes and unanswered questions. DNA Repair. (2014) 17:2–8. doi: 10.1016/j.dnarep.2014.01.009
33. Colombo N, Sessa C, du Bois A, Ledermann J, McCluggage WG, McNeish I, et al. ESMO-ESGO consensus conference recommendations on ovarian cancer: pathology and molecular biology, early and advanced stages, borderline tumours and recurrent disease†. Ann Oncol. (2019) 30(5):672–705. doi: 10.1093/annonc/mdz062
34. Landen CN, Molinero L, Hamidi H, Sehouli J, Miller A, Moore KN, et al. Influence of Genomic Landscape on Cancer Immunotherapy for Newly Diagnosed Ovarian Cancer: Biomarker Analyses from the IMagyn050 Randomized Clinical Trial. Clin Cancer Res. (2023) 29(9):1698–707. doi: 10.1158/1078-0432.Ccr-22-2032
35. Fong PC, Yap TA, Boss DS, Carden CP, Mergui-Roelvink M, Gourley C, et al. Poly(ADP)-ribose polymerase inhibition: frequent durable responses in BRCA carrier ovarian cancer correlating with platinum-free interval. J Clin Oncol. (2010) 28(15):2512–9. doi: 10.1200/jco.2009.26.9589
36. Garsed DW, Pandey A, Fereday S, Kennedy CJ, Takahashi K, Alsop K, et al. The genomic and immune landscape of long-term survivors of high-grade serous ovarian cancer. Nat Genet. (2022) 54(12):1853–64. doi: 10.1038/s41588-022-01230-9
37. Pennington KP, Walsh T, Harrell MI, Lee MK, Pennil CC, Rendi MH, et al. Germline and somatic mutations in homologous recombination genes predict platinum response and survival in ovarian, fallopian tube, and peritoneal carcinomas. Clin Cancer Res. (2014) 20(3):764–75. doi: 10.1158/1078-0432.Ccr-13-2287
38. Bell D, Berchuck A, Birrer M, Chien J, Cramer DW, Dao F, et al. Integrated genomic analyses of ovarian carcinoma. Nature. (2011) 474(7353):609–15. doi: 10.1038/nature10166
39. Lord CJ, Ashworth A. The DNA damage response and cancer therapy. Nature. (2012) 481(7381):287–94. doi: 10.1038/nature10760
40. Mukhopadhyay A, Elattar A, Cerbinskaite A, Wilkinson SJ, Drew Y, Kyle S, et al. Development of a functional assay for homologous recombination status in primary cultures of epithelial ovarian tumor and correlation with sensitivity to poly(ADP-ribose) polymerase inhibitors. Clin Cancer Res. (2010) 16(8):2344–51. doi: 10.1158/1078-0432.Ccr-09-2758
41. Ledermann JA. First-line treatment of ovarian cancer: questions and controversies to address. Ther Adv Med Oncol. (2018) 10:1758835918768232. doi: 10.1177/1758835918768232
42. Ledermann JA, Raja FA, Fotopoulou C, Gonzalez-Martin A, Colombo N, Sessa C. Newly diagnosed and relapsed epithelial ovarian carcinoma: ESMO Clinical Practice Guidelines for diagnosis, treatment and follow-up. Ann Oncol. (2018) 29. doi: 10.1093/annonc/mdy157
43. Patch A-M, Christie EL, Etemadmoghadam D, Garsed DW, George J, Fereday S, et al. Whole–genome characterization of chemoresistant ovarian cancer. Nature. (2015) 521(7553):489–94. doi: 10.1038/nature14410
44. Konstantinopoulos PA, Ceccaldi R, Shapiro GI, D'Andrea AD. Homologous Recombination Deficiency: Exploiting the Fundamental Vulnerability of Ovarian Cancer. Cancer Discovery. (2015) 5(11):1137–54. doi: 10.1158/2159-8290.Cd-15-0714
45. Burdett NL, Willis MO, Pandey A, Fereday S, Bowtell D, Chenevix-Trench G, et al. Small-scale mutations are infrequent as mechanisms of resistance in post-PARP inhibitor tumour samples in high grade serous ovarian cancer. Sci Rep. (2023) 13(1):21884. doi: 10.1038/s41598-023-48153-x
46. Miller RE, Leary A, Scott CL, Serra V, Lord CJ, Bowtell D, et al. ESMO recommendations on predictive biomarker testing for homologous recombination deficiency and PARP inhibitor benefit in ovarian cancer. Ann Oncol. (2020) 31(12):1606–22. doi: 10.1016/j.annonc.2020.08.2102
47. Capoluongo ED, Pellegrino B, Arenare L, Califano D, Scambia G, Beltrame L, et al. Alternative academic approaches for testing homologous recombination deficiency in ovarian cancer in the MITO16A/MaNGO-OV2 trial. ESMO Open. (2022) 7(5):100585. doi: 10.1016/j.esmoop.2022.100585
48. Castroviejo-Bermejo M, Cruz C, Llop-Guevara A, Gutiérrez-Enríquez S, Ducy M, Ibrahim YH, et al. A RAD51 assay feasible in routine tumor samples calls PARP inhibitor response beyond BRCA mutation. EMBO Mol Med. (2018) 10(12):e9172. doi: 10.15252/emmm.201809172
49. Guffanti F, Alvisi MF, Anastasia A, Ricci F, Chiappa M, Llop-Guevara A, et al. Basal expression of RAD51 foci predicts olaparib response in patient-derived ovarian cancer xenografts. Br J Cancer. (2022) 126(1):120–8. doi: 10.1038/s41416-021-01609-1
50. Michelena J, Lezaja A, Teloni F, Schmid T, Imhof R, Altmeyer M. Analysis of PARP inhibitor toxicity by multidimensional fluorescence microscopy reveals mechanisms of sensitivity and resistance. Nat Commun. (2018) 9(1):2678. doi: 10.1038/s41467-018-05031-9
51. Fuh K, Mullen M, Blachut B, Stover E, Konstantinopoulos P, Liu J, et al. Homologous recombination deficiency real-time clinical assays, ready or not? Gynecol Oncol. (2020) 159(3):877–86. doi: 10.1016/j.ygyno.2020.08.035
52. Le Page C, Chung J, Rahimi K, Köbel M, Provencher D, Mes-Masson AM. Exploring the Clinical Impact of Predictive Biomarkers in Serous Ovarian Carcinomas. Curr Drug Targets. (2020) 21(10):974–95. doi: 10.2174/1389450120666191016143836
53. Davis A, Tinker AV, Friedlander M. "Platinum resistant" ovarian cancer: what is it, who to treat and how to measure benefit? Gynecol Oncol. (2014) 133(3):624–31. doi: 10.1016/j.ygyno.2014.02.038
54. Stockler MR, Hilpert F, Friedlander M, King MT, Wenzel L, Lee CK, et al. Patient-reported outcome results from the open-label phase III AURELIA trial evaluating bevacizumab-containing therapy for platinum-resistant ovarian cancer. J Clin Oncol. (2014) 32(13):1309–16. doi: 10.1200/jco.2013.51.4240
55. Poveda AM, Selle F, Hilpert F, Reuss A, Savarese A, Vergote I, et al. Bevacizumab Combined With Weekly Paclitaxel, Pegylated Liposomal Doxorubicin, or Topotecan in Platinum-Resistant Recurrent Ovarian Cancer: Analysis by Chemotherapy Cohort of the Randomized Phase III AURELIA Trial. J Clin Oncol. (2015) 33(32):3836–8. doi: 10.1200/jco.2015.63.1408
56. Haun MW, Estel S, Rücker G, Friederich HC, Villalobos M, Thomas M, et al. Early palliative care for adults with advanced cancer. Cochrane Database Syst Rev. doi: 10.1002/14651858.CD011129.pub2
57. Roncolato FT, Joly F, O'Connell R, Lanceley A, Hilpert F, Buizen L, et al. Reducing Uncertainty: Predictors of Stopping Chemotherapy Early and Shortened Survival Time in Platinum Resistant/Refractory Ovarian Cancer-The GCIG Symptom Benefit Study. Oncologist. (2017) 22(9):1117–24. doi: 10.1634/theoncologist.2017-0047
58. Koole SN, Schouten PC, Hauke J, Kluin RJC, Nederlof P, Richters LK, et al. Effect of HIPEC according to HRD/BRCAwt genomic profile in stage III ovarian cancer: Results from the phase III OVHIPEC trial. Int J Cancer. (2022) 151(8):1394–404. doi: 10.1002/ijc.34124
59. Lord CJ, Ashworth A. BRCAness revisited. Nat Rev Cancer. (2016) 16(2):110–20. doi: 10.1038/nrc.2015.21
60. Lord CJ, Ashworth A. PARP inhibitors: Synthetic lethality in the clinic. Science. (2017) 355(6330):1152–8. doi: 10.1126/science.aam7344
61. Heeke AL, Pishvaian MJ, Lynce F, Xiu J, Brody JR, Chen WJ, et al. Prevalence of Homologous Recombination-Related Gene Mutations Across Multiple Cancer Types. JCO Precis Oncol. (2018) 2018:PO.17.00286. doi: 10.1200/po.17.00286
62. du Bois A, Reuss A, Pujade-Lauraine E, Harter P, Ray-Coquard I, Pfisterer J. Role of surgical outcome as prognostic factor in advanced epithelial ovarian cancer: A combined exploratory analysis of 3 prospectively randomized phase 3 multicenter trials. Cancer. (2009) 115(6):1234–44. doi: 10.1002/cncr.24149
63. Kotsopoulos J, Zamani N, Rosen B, McLaughlin JR, Risch HA, Kim SJ, et al. Impact of germline mutations in cancer-predisposing genes on long-term survival in patients with epithelial ovarian cancer. Br J Cancer. (2022) 127(5):879–85. doi: 10.1038/s41416-022-01840-4
64. Karst AM, Jones PM, Vena N, Ligon AH, Liu JF, Hirsch MS, et al. Cyclin E1 deregulation occurs early in secretory cell transformation to promote formation of fallopian tube-derived high-grade serous ovarian cancers. Cancer Res. (2014) 74(4):1141–52. doi: 10.1158/0008-5472.Can-13-2247
65. Gorski JW, Ueland FR, Kolesar JM. CCNE1 Amplification as a Predictive Biomarker of Chemotherapy Resistance in Epithelial Ovarian Cancer. Diagnostics. (2020) 10(5):279.
66. Jones RM, Mortusewicz O, Afzal I, Lorvellec M, García P, Helleday T, et al. Increased replication initiation and conflicts with transcription underlie Cyclin E-induced replication stress. Oncogene. (2013) 32(32):3744–53. doi: 10.1038/onc.2012.387
67. Creeden JF, Nanavaty NS, Einloth KR, Gillman CE, Stanbery L, Hamouda DM, et al. Homologous recombination proficiency in ovarian and breast cancer patients. BMC Cancer. (2021) 21(1):1154. doi: 10.1186/s12885-021-08863-9
68. Gallo D, Young JTF, Fourtounis J, Martino G, Álvarez-Quilón A, Bernier C, et al. CCNE1 amplification is synthetic lethal with PKMYT1 kinase inhibition. Nature. (2022) 604(7907):749–56. doi: 10.1038/s41586-022-04638-9
69. Sapoznik S, Aviel-Ronen S, Bahar-Shany K, Zadok O, Levanon K. CCNE1 expression in high grade serous carcinoma does not correlate with chemoresistance. Oncotarget. (2017) 8(37):62240.
70. Au-Yeung G, Lang F, Azar WJ, Mitchell C, Jarman KE, Lackovic K, et al. Selective Targeting of Cyclin E1-Amplified High-Grade Serous Ovarian Cancer by Cyclin-Dependent Kinase 2 and AKT Inhibition. Clin Cancer Res. (2017) 23(7):1862–74. doi: 10.1158/1078-0432.Ccr-16-0620
71. Foster KI, Shaw KRM, Jin J, Westin SN, Yap TA, Glassman DM, et al. Clinical implications of tumor-based next-generation sequencing in high-grade epithelial ovarian cancer. Cancer. (2023) 129(11):1672–80. doi: 10.1002/cncr.34724
72. Dall'Acqua A, Bartoletti M, Masoudi-Khoram N, Sorio R, Puglisi F, Belletti B, et al. Inhibition of CDK4/6 as Therapeutic Approach for Ovarian Cancer Patients: Current Evidences and Future Perspectives. Cancers (Basel). (2021) 13(12):3035. doi: 10.3390/cancers13123035
73. Zhang Z, Golomb L, Meyerson M. Functional Genomic Analysis of CDK4 and CDK6 Gene Dependency across Human Cancer Cell Lines. Cancer Res. (2022) 82(11):2171–84. doi: 10.1158/0008-5472.Can-21-2428
74. Dietrich C, Trub A, Ahn A, Taylor M, Ambani K, Chan KT, et al. INX-315, a selective CDK2 inhibitor, induces cell cycle arrest and senescence in solid tumors. Cancer Discovery. (2024) 14(3):446–67. doi: 10.1158/2159-8290.Cd-23-0954
75. Yap TA, Elhaddad AM, Grisham RN, Hamm JT, Marks DK, Shapiro G, et al. First-in-human phase 1/2a study of a potent and novel CDK2-selective inhibitor PF-07104091 in patients (pts) with advanced solid tumors, enriched for CDK4/6 inhibitor resistant HR+/HER2- breast cancer. J Clin Oncol. 202341(16_suppl):3010. doi: 10.1200/JCO.2023.41.16_suppl.3010
76. Brown V, Ramsden P, House N, Vargas R, Guo J, Wang R, et al. Abstract 2306: BLU-222, an investigational, potent, and selective CDK2 inhibitor, demonstrated robust antitumor activity in CCNE1-amplified ovarian cancer models. Cancer Res. 82(12_Supplement):2306. doi: 10.1158/1538-7445.Am2022-2306
77. Dubbury SJ, Boutz PL, Sharp PA. CDK12 regulates DNA repair genes by suppressing intronic polyadenylation. Nature. (2018) 564(7734):141–5. doi: 10.1038/s41586-018-0758-y
78. Zhang H, Liu T, Zhang Z, Payne SH, Zhang B, McDermott JE, et al. Integrated Proteogenomic Characterization of Human High-Grade Serous Ovarian Cancer. Cell. (2016) 166(3):755–65. doi: 10.1016/j.cell.2016.05.069
79. Dou Y, Kawaler EA, Cui Zhou D, Gritsenko MA, Huang C, Blumenberg L, et al. Proteogenomic Characterization of Endometrial Carcinoma. Cell. (2020) 180(4):729–48.e26. doi: 10.1016/j.cell.2020.01.026
80. Krug K, Jaehnig EJ, Satpathy S, Blumenberg L, Karpova A, Anurag M, et al. Proteogenomic Landscape of Breast Cancer Tumorigenesis and Targeted Therapy. Cell. (2020) 183(5):1436–56.e31. doi: 10.1016/j.cell.2020.10.036
81. Vasaikar S, Huang C, Wang X, Petyuk VA, Savage SR, Wen B, et al. Proteogenomic Analysis of Human Colon Cancer Reveals New Therapeutic Opportunities. Cell. (2019) 177(4):1035–49.e19. doi: 10.1016/j.cell.2019.03.030
82. Sato E, Olson SH, Ahn J, Bundy B, Nishikawa H, Qian F, et al. Intraepithelial CD8+ tumor-infiltrating lymphocytes and a high CD8+/regulatory T cell ratio are associated with favorable prognosis in ovarian cancer. Proc Natl Acad Sci U.S.A. (2005) 102(51):18538–43. doi: 10.1073/pnas.0509182102
83. Zhang L, Conejo-Garcia JR, Katsaros D, Gimotty PA, Massobrio M, Regnani G, et al. Intratumoral T cells, recurrence, and survival in epithelial ovarian cancer. N Engl J Med. (2003) 348(3):203–13. doi: 10.1056/NEJMoa020177
84. Kandalaft LE, Dangaj Laniti D, Coukos G. Immunobiology of high-grade serous ovarian cancer: lessons for clinical translation. Nat Rev Cancer. (2022) 22(11):640–56. doi: 10.1038/s41568-022-00503-z
85. Consortium OTTA. Dose-Response Association of CD8+ Tumor-Infiltrating Lymphocytes and Survival Time in High-Grade Serous Ovarian Cancer. JAMA Oncol. (2017) 3(12):e173290–e. doi: 10.1001/jamaoncol.2017.3290
86. Garsed DW, Alsop K, Fereday S, Emmanuel C, Kennedy CJ, Etemadmoghadam D, et al. Homologous Recombination DNA Repair Pathway Disruption and Retinoblastoma Protein Loss Are Associated with Exceptional Survival in High-Grade Serous Ovarian Cancer. Clin Cancer Res. (2018) 24(3):569–80. doi: 10.1158/1078-0432.Ccr-17-1621
87. Le DT, Durham JN, Smith KN, Wang H, Bartlett BR, Aulakh LK, et al. Mismatch repair deficiency predicts response of solid tumors to PD-1 blockade. Science. (2017) 357(6349):409–13. doi: 10.1126/science.aan6733
88. Ciombor KK, Goldberg RM. Hypermutated Tumors and Immune Checkpoint Inhibition. Drugs. (2018) 78(2):155–62. doi: 10.1007/s40265-018-0863-0
89. Mouw KW, Goldberg MS, Konstantinopoulos PA, D'Andrea AD. DNA Damage and Repair Biomarkers of Immunotherapy Response. Cancer Discovery. (2017) 7(7):675–93. doi: 10.1158/2159-8290.Cd-17-0226
90. Disis ML, Patel MR, Pant S, Hamilton EP, Lockhart AC, Kelly K, et al. Avelumab (MSB0010718C; anti-PD-L1) in patients with recurrent/refractory ovarian cancer from the JAVELIN Solid Tumor phase Ib trial: Safety and clinical activity. J Clin Oncol. (2016) 34(15_suppl). doi: 10.1200/JCO.2016.34_suppl.5533
91. Hamanishi J, Mandai M, Ikeda T, Minami M, Kawaguchi A, Murayama T, et al. Safety and antitumor activity of Anti-PD-1 antibody, nivolumab, in patients with platinum-resistant ovarian cancer. J Clin Oncol. (2015) 33(34):4015–22. doi: 10.1200/JCO.2015.62.3397
92. Varga A, Piha-Paul SA, Ott PA, Mehnert JM, Berton-Rigaud D, Morosky A, et al. Pembrolizumab in patients (pts) with PD-L1–positive (PD-L1+) advanced ovarian cancer: updated analysis of KEYNOTE-028. J Clin Oncol. (2017) 35(15_suppl). doi: 10.1200/JCO.2017.35.15_suppl.5513
93. Ledermann JA, Colombo N, Oza AM, Fujiwara K, Birrer MJ, Randall LM, et al. Avelumab in combination with and/or following chemotherapy vs chemotherapy alone in patients with previously untreated epithelial ovarian cancer: Results from the phase 3 javelin ovarian 100 trial. . Gynecologic Oncol. (2020) 159:13–4. doi: 10.1016/j.ygyno.2020.06.025
94. Moore KN, Bookman M, Sehouli J, Miller A, Anderson C, Scambia G, et al. Atezolizumab, Bevacizumab, and Chemotherapy for Newly Diagnosed Stage III or IV Ovarian Cancer: Placebo-Controlled Randomized Phase III Trial (IMagyn050/GOG 3015/ENGOT-OV39). J Clin Oncol. (2021) 39(17):1842–55. doi: 10.1200/jco.21.00306
95. Matulonis UA, Shapira R, Santin A, Lisyanskaya AS, Pignata S, Vergote I, et al. Final results from the KEYNOTE-100 trial of pembrolizumab in patients with advanced recurrent ovarian cancer. J Clin Oncol. (2020) 38(15_suppl):6005. doi: 10.1200/JCO.2020.38.15_suppl.6005
96. Gaillard SL, Secord AA, Monk B. The role of immune checkpoint inhibition in the treatment of ovarian cancer. Gynecol Oncol Res Pract. (2016) 3:11. doi: 10.1186/s40661-016-0033-6
97. Bruand M, Barras D, Mina M, Ghisoni E, Morotti M, Lanitis E, et al. Cell-autonomous inflammation of BRCA1-deficient ovarian cancers drives both tumor-intrinsic immunoreactivity and immune resistance via STING. Cell Rep. (2021) 36(3):109412. doi: 10.1016/j.celrep.2021.109412
98. Gao Y, Wang Y, Luo F, Chu Y. Optimization of T Cell Redirecting Strategies: Obtaining Inspirations From Natural Process of T Cell Activation. Front Immunol. (2021) 12:664329. doi: 10.3389/fimmu.2021.664329
99. Kim HS, Kim JY, Lee YJ, Kim SH, Lee JY, Nam EJ, et al. Expression of programmed cell death ligand 1 and immune checkpoint markers in residual tumors after neoadjuvant chemotherapy for advanced high-grade serous ovarian cancer. Gynecol Oncol. (2018) 151(3):414–21. doi: 10.1016/j.ygyno.2018.08.023
100. Pearce OMT, Delaine-Smith RM, Maniati E, Nichols S, Wang J, Böhm S, et al. Deconstruction of a Metastatic Tumor Microenvironment Reveals a Common Matrix Response in Human Cancers. Cancer Discovery. (2018) 8(3):304–19. doi: 10.1158/2159-8290.Cd-17-0284
101. Aghajanian C, Swisher EM, Okamoto A, Steffensen KD, Bookman MA, Fleming GF, et al. Impact of veliparib, paclitaxel dosing regimen, and germline BRCA status on the primary treatment of serous ovarian cancer – an ancillary data analysis of the VELIA trial. Gynecologic Oncol. (2022) 164(2):278–87. doi: 10.1016/j.ygyno.2021.12.012
102. Katsumata N, Yasuda M, Isonishi S, Takahashi F, Michimae H, Kimura E, et al. Long-term results of dose-dense paclitaxel and carboplatin versus conventional paclitaxel and carboplatin for treatment of advanced epithelial ovarian, fallopian tube, or primary peritoneal cancer (JGOG 3016): a randomised, controlled, open-label trial. Lancet Oncol. (2013) 14(10):1020–6. doi: 10.1016/S1470-2045(13)70363-2
103. Clamp AR, James EC, McNeish IA, Dean A, Kim J-W, O'Donnell DM, et al. Weekly dose-dense chemotherapy in first-line epithelial ovarian, fallopian tube, or primary peritoneal carcinoma treatment (ICON8): primary progression free survival analysis results from a GCIG phase 3 randomised controlled trial. Lancet. (2019) 394(10214):2084–95. doi: 10.1016/S0140-6736(19)32259-7
104. Yanaihara N, Yoshino Y, Noguchi D, Tabata J, Takenaka M, Iida Y, et al. Paclitaxel sensitizes homologous recombination-proficient ovarian cancer cells to PARP inhibitor via the CDK1/BRCA1 pathway. Gynecologic Oncol. (2023) 168:83–91. doi: 10.1016/j.ygyno.2022.11.006
105. Aronson SL, Lopez-Yurda M, Koole SN, Schagen van Leeuwen JH, Schreuder HWR, Hermans RHM, et al. Cytoreductive surgery with or without hyperthermic intraperitoneal chemotherapy in patients with advanced ovarian cancer (OVHIPEC-1): final survival analysis of a randomised, controlled, phase 3 trial. Lancet Oncol. (2023) 24(10):1109–18. doi: 10.1016/S1470-2045(23)00396-0
106. Schouten PC, Richters L, Vis DJ, Kommoss S, van Dijk E, Ernst C, et al. Ovarian Cancer-Specific BRCA-like Copy-Number Aberration Classifiers Detect Mutations Associated with Homologous Recombination Deficiency in the AGO-TR1 Trial. Clin Cancer Res. (2021) 27(23):6559–69. doi: 10.1158/1078-0432.Ccr-21-1673
107. Ghirardi V, Trozzi R, Scambia G, Fagotti A. Current and future trials about HIPEC in ovarian cancer. Bull du Cancer. (2024) 111(3):254–60. doi: 10.1016/j.bulcan.2023.01.016
108. Goel N, Foxall ME, Scalise CB, Wall JA, Arend RC. Strategies in Overcoming Homologous Recombination Proficiency and PARP Inhibitor Resistance. Mol Cancer Ther. (2021) 20(9):1542–9. doi: 10.1158/1535-7163.Mct-20-0992
109. Pascal JM. The comings and goings of PARP-1 in response to DNA damage. DNA Repair (Amst). (2018) 71:177–82. doi: 10.1016/j.dnarep.2018.08.022
110. Mirza MR, Monk BJ, Herrstedt J, Oza AM, Mahner S, Redondo A, et al. Niraparib Maintenance Therapy in Platinum-Sensitive, Recurrent Ovarian Cancer. N Engl J Med. (2016) 375(22):2154–64. doi: 10.1056/NEJMoa1611310
111. del Campo JM, Matulonis UA, Malander S, Provencher D, Mahner S, Follana P, et al. Niraparib Maintenance Therapy in Patients With Recurrent Ovarian Cancer After a Partial Response to the Last Platinum-Based Chemotherapy in the ENGOT-OV16/NOVA Trial. J Clin Oncol. (2019) 37(32):2968–73. doi: 10.1200/JCO.18.02238
112. You B, Sehgal V, Hosmane B, Huang X, Ansell PJ, Dinh MH, et al. CA-125 KELIM as a Potential Complementary Tool for Predicting Veliparib Benefit: An Exploratory Analysis From the VELIA/GOG-3005 Study. J Clin Oncol. (2023) 41(1):107–16. doi: 10.1200/jco.22.00430
113. Swisher EM, Aghajanian C, O'Malley DM, Fleming GF, Kaufmann SH, Levine DA, et al. Impact of homologous recombination status and responses with veliparib combined with first-line chemotherapy in ovarian cancer in the Phase 3 VELIA/GOG-3005 study. Gynecologic Oncol. (2022) 164(2):245–53.
114. Shibuya M. Vascular Endothelial Growth Factor (VEGF) and Its Receptor (VEGFR) Signaling in Angiogenesis: A Crucial Target for Anti- and Pro-Angiogenic Therapies. Genes Cancer. (2011) 2(12):1097–105. doi: 10.1177/1947601911423031
115. Tewari KS, Burger RA, Enserro D, Norquist BM, Swisher EM, Brady MF, et al. Final Overall Survival of a Randomized Trial of Bevacizumab for Primary Treatment of Ovarian Cancer. J Clin Oncol. (2019) 37(26):2317–28. doi: 10.1200/jco.19.01009
116. Nakai H, Matsumura N. The roles and limitations of bevacizumab in the treatment of ovarian cancer. Int J Clin Oncol. (2022) 27(7):1120–6. doi: 10.1007/s10147-022-02169-x
117. Chan N, Bristow RG. "Contextual" synthetic lethality and/or loss of heterozygosity: tumor hypoxia and modification of DNA repair. Clin Cancer Res. (2010) 16(18):4553–60. doi: 10.1158/1078-0432.Ccr-10-0527
118. Ribeiro ARG, Salvadori MM, de Brot L, Bovolin G, Mantoan H, Ilelis F, et al. Retrospective analysis of the role of cyclin E1 overexpression as a predictive marker for the efficacy of bevacizumab in platinum-sensitive recurrent ovarian cancer. Ecancermedicalscience. (2021) 15:1262. doi: 10.3332/ecancer.2021.1262
119. Mirza MR, Åvall Lundqvist E, Birrer MJ, dePont Christensen R, Nyvang GB, Malander S, et al. Niraparib plus bevacizumab versus niraparib alone for platinum-sensitive recurrent ovarian cancer (NSGO-AVANOVA2/ENGOT-ov24): a randomised, phase 2, superiority trial. Lancet Oncol. (2019) 20(10):1409–19. doi: 10.1016/s1470-2045(19)30515-7
120. Norquist BM, Brady MF, Harrell MI, Walsh T, Lee MK, Gulsuner S, et al. Mutations in Homologous Recombination Genes and Outcomes in Ovarian Carcinoma Patients in GOG 218: An NRG Oncology/Gynecologic Oncology Group Study. Clin Cancer Res. (2018) 24(4):777–83. doi: 10.1158/1078-0432.Ccr-17-1327
121. Teresa Z, Intidhar L-G, Maria Del G, Cristiana S, Ilaria C. The clinical challenges of homologous recombination proficiency in ovarian cancer: from intrinsic resistance to new treatment opportunities. Cancer Drug Resistance. (2023) 6(3):499–516. doi: 10.20517/cdr.2023.08
122. Lim JJ, Yang K, Taylor-Harding B, Wiedemeyer WR, Buckanovich RJ. VEGFR3 inhibition chemosensitizes ovarian cancer stemlike cells through down-regulation of BRCA1 and BRCA2. Neoplasia. (2014) 16(4):343–53. doi: 10.1016/j.neo.2014.04.003
123. Liu JF, Barry WT, Birrer M, Lee JM, Buckanovich RJ, Fleming GF, et al. Combination cediranib and olaparib versus olaparib alone for women with recurrent platinum-sensitive ovarian cancer: a randomised phase 2 study. Lancet Oncol. (2014) 15(11):1207–14. doi: 10.1016/s1470-2045(14)70391-2
124. Bizzaro F, Fuso Nerini I, Taylor MA, Anastasia A, Russo M, Damia G, et al. VEGF pathway inhibition potentiates PARP inhibitor efficacy in ovarian cancer independent of BRCA status. J Hematol Oncol. (2021) 14(1):186. doi: 10.1186/s13045-021-01196-x
125. Lee J-M, Moore RG, Ghamande S, Park MS, Diaz JP, Chapman J, et al. Cediranib in Combination with Olaparib in Patients without a Germline BRCA1/2 Mutation and with Recurrent Platinum-Resistant Ovarian Cancer: Phase IIb CONCERTO Trial. Clin Cancer Res. (2022) 28(19):4186–93. doi: 10.1158/1078-0432.Ccr-21-1733
126. Liu JF, Brady MF, Matulonis UA, Miller A, Kohn EC, Swisher EM, et al. Olaparib With or Without Cediranib Versus Platinum-Based Chemotherapy in Recurrent Platinum-Sensitive Ovarian Cancer (NRG-GY004): A Randomized, Open-Label, Phase III Trial. J Clin Oncol. (2022) 40(19):2138–47. doi: 10.1200/jco.21.02011
127. Cunnea P, Curry EW, Christie EL, Nixon K, Kwok CH, Pandey A, et al. Spatial and temporal intra-tumoral heterogeneity in advanced HGSOC: Implications for surgical and clinical outcomes. Cell Rep Med. (2023) 4(6):101055. doi: 10.1016/j.xcrm.2023.101055
128. Hall M, Savvatis K, Nixon K, Kyrgiou M, Hariharan K, Padwick M, et al. Maximal-Effort Cytoreductive Surgery for Ovarian Cancer Patients with a High Tumor Burden: Variations in Practice and Impact on Outcome. Annals of Surgical Oncology. (2019) 26(9):2943–51. doi: 10.1245/s10434-019-07516-3
129. Shi T, Zhu J, Feng Y, Tu D, Zhang Y, Zhang P, et al. Secondary cytoreduction followed by chemotherapy versus chemotherapy alone in platinum-sensitive relapsed ovarian cancer (SOC-1): a multicentre, open-label, randomised, phase 3 trial. Lancet Oncol. (2021) 22(4):439–49. doi: 10.1016/s1470-2045(21)00006-1
130. Harter P, Sehouli J, Vergote I, Ferron G, Reuss A, Meier W, et al. Randomized Trial of Cytoreductive Surgery for Relapsed Ovarian Cancer. N Engl J Med. (2021) 385(23):2123–31. doi: 10.1056/NEJMoa2103294
131. Coleman RL, Spirtos NM, Enserro D, Herzog TJ, Sabbatini P, Armstrong DK, et al. Secondary Surgical Cytoreduction for Recurrent Ovarian Cancer. New Engl J Med. (2019) 381(20):1929–39. doi: 10.1056/NEJMoa1902626
132. Petrillo M, Sozzi G, Dessole M, Capobianco G, Dessole S, Madonia M, et al. The role of surgery in platinum-resistant ovarian cancer: A call to the scientific community. Semin Cancer Biol. (2021) 77:194–202. doi: 10.1016/j.semcancer.2021.02.009
133. Zwimpfer TA, Tal O, Geissler F, Coelho R, Rimmer N, Jacob F, et al. Low grade serous ovarian cancer - A rare disease with increasing therapeutic options. Cancer Treat Rev. (2023) 112:102497. doi: 10.1016/j.ctrv.2022.102497
134. Petrillo M, Pedone Anchora L, Tortorella L, Fanfani F, Gallotta V, Pacciani M, et al. Secondary cytoreductive surgery in patients with isolated platinum-resistant recurrent ovarian cancer: A retrospective analysis. Gynecologic Oncol. (2014) 134(2):257–61. doi: 10.1016/j.ygyno.2014.05.029
135. Musella A, Marchetti C, Palaia I, Perniola G, Giorgini M, Lecce F, et al. Secondary Cytoreduction in Platinum-Resistant Recurrent Ovarian Cancer: A Single-Institution Experience. Ann Surg Oncol. (2015) 22(13):4211–6. doi: 10.1245/s10434-015-4523-2
136. Tuninetti V, Di Napoli M, Ghisoni E, Maggiorotto F, Robella M, Scotto G, et al. Cytoreductive Surgery for Heavily Pre-Treated, Platinum-Resistant Epithelial Ovarian Carcinoma: A Two-Center Retrospective Experience. Cancers. (2020) 12(8):2239.
137. Marchetti C, De Leo R, Musella A, D'Indinosante M, Capoluongo E, Minucci A, et al. BRCA Mutation Status to Personalize Management of Recurrent Ovarian Cancer: A Multicenter Study. Ann Surg Oncol. (2018) 25(12):3701–8. doi: 10.1245/s10434-018-6700-6
138. Fan CA, Reader J, Roque DM. Review of Immune Therapies Targeting Ovarian Cancer. Curr Treat Options Oncol. (2018) 19(12):74. doi: 10.1007/s11864-018-0584-3
139. Colombo I, Karakasis K, Suku S, Oza AM. Chasing Immune Checkpoint Inhibitors in Ovarian Cancer: Novel Combinations and Biomarker Discovery. Cancers (Basel). (2023) 15(12):3220. doi: 10.3390/cancers15123220
140. Lee EK, Xiong N, Cheng SC, Barry WT, Penson RT, Konstantinopoulos PA, et al. Combined pembrolizumab and pegylated liposomal doxorubicin in platinum resistant ovarian cancer: A phase 2 clinical trial. Gynecol Oncol. (2020) 159(1):72–8. doi: 10.1016/j.ygyno.2020.07.028
141. Pujade-Lauraine E, Fujiwara K, Ledermann JA, Oza AM, Kristeleit R, Ray-Coquard IL, et al. Avelumab alone or in combination with chemotherapy versus chemotherapy alone in platinum-resistant or platinum-refractory ovarian cancer (JAVELIN Ovarian 200): an open-label, three-arm, randomised, phase 3 study. Lancet Oncol. (2021) 22(7):1034–46. doi: 10.1016/s1470-2045(21)00216-3
142. Monk BJ, Colombo N, Oza AM, Fujiwara K, Birrer MJ, Randall L, et al. Chemotherapy with or without avelumab followed by avelumab maintenance versus chemotherapy alone in patients with previously untreated epithelial ovarian cancer (JAVELIN Ovarian 100): an open-label, randomised, phase 3 trial. Lancet Oncol. (2021) 22(9):1275–89. doi: 10.1016/s1470-2045(21)00342-9
143. Riccardi F, Dal Bo M, Macor P, Toffoli G. A comprehensive overview on antibody-drug conjugates: from the conceptualization to cancer therapy. Front Pharmacol. (2023) 14:1274088. doi: 10.3389/fphar.2023.1274088
144. Moore KN, Angelergues A, Konecny GE, García Y, Banerjee S, Lorusso D, et al. Mirvetuximab Soravtansine in FRα-Positive, Platinum-Resistant Ovarian Cancer. New Engl J Med. (2023) 389(23):2162–74. doi: 10.1056/NEJMoa2309169
145. Kalli KR, Oberg AL, Keeney GL, Christianson TJ, Low PS, Knutson KL, et al. Folate receptor alpha as a tumor target in epithelial ovarian cancer. Gynecol Oncol. (2008) 108(3):619–26. doi: 10.1016/j.ygyno.2007.11.020
146. Morand S, Devanaboyina M, Staats H, Stanbery L, Nemunaitis J. Ovarian Cancer Immunotherapy and Personalized Medicine. Int J Mol Sci. (2021) 22(12):6532. doi: 10.3390/ijms22126532
147. Oh J, Barve M, Matthews CM, Koon EC, Heffernan TP, Fine B, et al. Phase II study of Vigil® DNA engineered immunotherapy as maintenance in advanced stage ovarian cancer. Gynecologic Oncol. (2016) 143(3):504–10. doi: 10.1016/j.ygyno.2016.09.018
148. Bristow RE, Baldwin RL, Yamada SD, Korc M, Karlan BY. Altered expression of transforming growth factor-β ligands and receptors in primary and recurrent ovarian carcinoma. Cancer. (1999) 85(3):658–68. doi: 10.1002/(SICI)1097-0142(19990201)85:3<658::AID-CNCR16>3.0.CO;2-M
149. Zhang Y, Zhang L, Zhao Y, Wang S, Feng L. Efficacy and safety of Gemogenovatucel-T (Vigil) immunotherapy for advanced ovarian carcinoma: A systematic review and meta-analysis of randomized controlled trials. Front Oncol. (2022) 12:945867. doi: 10.3389/fonc.2022.945867
150. Senzer N, Barve M, Nemunaitis J, Kuhn J, Melnyk A, Beitsch P, et al. Long term follow up: phase I trial of “bi-shRNA furin/GMCSF DNA/autologous tumor cell” immunotherapy (FANG™) in advanced cancer. J Vaccines Vaccin. (2013) 4(8):209. doi: 10.4172/2157-7560.1000209
151. Rocconi RP, Grosen EA, Ghamande SA, Chan JK, Barve MA, Oh J, et al. Gemogenovatucel-T (Vigil) immunotherapy as maintenance in frontline stage III/IV ovarian cancer (VITAL): a randomised, double-blind, placebo-controlled, phase 2b trial. Lancet Oncol. (2020) 21(12):1661–72. doi: 10.1016/S1470-2045(20)30533-7
152. Rocconi RP, Monk BJ, Walter A, Herzog TJ, Galanis E, Manning L, et al. Gemogenovatucel-T (Vigil) immunotherapy demonstrates clinical benefit in homologous recombination proficient (HRP) ovarian cancer. Gynecol Oncol. (2021) 161(3):676–80. doi: 10.1016/j.ygyno.2021.03.009
153. McGranahan N, Furness AJS, Rosenthal R, Ramskov S, Lyngaa R, Saini SK, et al. Clonal neoantigens elicit T cell immunoreactivity and sensitivity to immune checkpoint blockade. Science. (2016) 351(6280):1463–9. doi: 10.1126/science.aaf1490
154. Preston CC, Goode EL, Hartmann LC, Kalli KR, Knutson KL. Immunity and immune suppression in human ovarian cancer. Immunotherapy. (2011) 3(4):539–56. doi: 10.2217/imt.11.20
155. Rocconi RP, Stevens EE, Bottsford-Miller JN, Ghamande SA, Elder J, DeMars LL, et al. Proof of principle study of sequential combination atezolizumab and Vigil in relapsed ovarian cancer. Cancer Gene Ther. (2022) 29(3-4):369–82. doi: 10.1038/s41417-021-00317-5
156. Sarivalasis A, Morotti M, Mulvey A, Imbimbo M, Coukos G. Cell therapies in ovarian cancer. Ther Adv Med Oncol. (2021) 13:17588359211008399. doi: 10.1177/17588359211008399
157. Drew Y, Kim JW, Penson RT, O'Malley DM, Parkinson C, Roxburgh P, et al. Olaparib plus Durvalumab, with or without Bevacizumab, as Treatment in PARP Inhibitor-Naïve Platinum-Sensitive Relapsed Ovarian Cancer: A Phase II Multi-Cohort Study. Clin Cancer Res. (2024) 30(1):50–62. doi: 10.1158/1078-0432.Ccr-23-2249
158. Harter P, Trillsch F, Okamoto A, Reuss A, Kim J-W, Rubio-Pérez MJ, et al. Durvalumab with paclitaxel/carboplatin (PC) and bevacizumab (bev), followed by maintenance durvalumab, bev, and olaparib in patients (pts) with newly diagnosed advanced ovarian cancer (AOC) without a tumor BRCA1/2 mutation (non-tBRCAm): Results from the randomized, placebo (pbo)-controlled phase III DUO-O trial. J Clin Oncol. (2023) 41(17_suppl):LBA5506–LBA. doi: 10.1200/JCO.2023.41.17_suppl.LBA5506
159. Shapiro G, Do K, Tolaney S, Hilton J, Cleary J, Wolanski A, et al. Abstract CT047: Phase 1 dose-escalation study of the CDK inhibitor dinaciclib in combination with the PARP inhibitor veliparib in patients with advanced solid tumors. Cancer Res. (2017) 77:CT047–CT. doi: 10.1158/1538-7445.AM2017-CT047
160. Westin SN, Coleman RL, Fellman BM, Yuan Y, Sood AK, Soliman PT, et al. EFFORT: EFFicacy Of adavosertib in parp ResisTance: A randomized two-arm non-comparative phase II study of adavosertib with or without olaparib in women with PARP-resistant ovarian cancer. J Clin Oncol. (2021) 39(15_suppl):5505. doi: 10.1200/JCO.2021.39.15_suppl.5505
161. Konstantinopoulos PA, Gonzalez-Martin A, Cruz FM, Friedlander M, Glasspool R, Lorusso D, et al. EPIK-O/ENGOT-OV61: alpelisib plus olaparib vs cytotoxic chemotherapy in high-grade serous ovarian cancer (phase III study). Future Oncol. (2022) 18(31):3481–92. doi: 10.2217/fon-2022-0666
162. Konstantinopoulos PA, Cheng SC, Supko JG, Polak M, Wahner-Hendrickson AE, Ivy SP, et al. Combined PARP and HSP90 inhibition: preclinical and Phase 1 evaluation in patients with advanced solid tumours. Br J Cancer. (2022) 126(7):1027–36. doi: 10.1038/s41416-021-01664-8
163. Fang D, Huang S, Su SB. Cyclin E1-CDK 2, a potential anticancer target. Aging (Albany NY). (2016) 8(4):571–2. doi: 10.18632/aging.100946
164. Chen XX, Xie FF, Zhu XJ, Lin F, Pan SS, Gong LH, et al. Cyclin-dependent kinase inhibitor dinaciclib potently synergizes with cisplatin in preclinical models of ovarian cancer. Oncotarget. (2015) 6(17):14926–39. doi: 10.18632/oncotarget.3717
165. Johnson N, Li YC, Walton ZE, Cheng KA, Li D, Rodig SJ, et al. Compromised CDK1 activity sensitizes BRCA-proficient cancers to PARP inhibition. Nat Med. (2011) 17(7):875–82. doi: 10.1038/nm.2377
166. Johnson SF, Johnson N, Chi D, Primack B, D'Andrea AD, Lim E, et al. Abstract 1788: The CDK inhibitor dinaciclib sensitizes triple-negative breast cancer cells to PARP inhibition. Cancer Res. 2013 73(8_Supplement):1788–. doi: 10.1158/1538-7445.Am2013-1788
167. Chen P, Lee NV, Hu W, Xu M, Ferre RA, Lam H, et al. Spectrum and Degree of CDK Drug Interactions Predicts Clinical Performance. Mol Cancer Ther. (2016) 15(10):2273–81. doi: 10.1158/1535-7163.Mct-16-0300
168. Au-Yeung G, Bressel M, Prall O, Surace D, Andrews J, Mongta S, et al. IGNITE: A phase II signal-seeking trial of adavosertib targeting recurrent high-grade, serous ovarian cancer with cyclin E1 overexpression with and without gene amplification. J Clin Oncol. (2022) 40(16_suppl):5515–. doi: 10.1200/JCO.2022.40.16_suppl.5515
169. Johnson N, Cai D, Kennedy RD, Pathania S, Arora M, Li YC, et al. Cdk1 participates in BRCA1-dependent S phase checkpoint control in response to DNA damage. Mol Cell. (2009) 35(3):327–39. doi: 10.1016/j.molcel.2009.06.036
170. Turner N, Tutt A, Ashworth A. Hallmarks of 'BRCAness' in sporadic cancers. Nat Rev Cancer. (2004) 4(10):814–9. doi: 10.1038/nrc1457
171. Kausar T, Schreiber JS, Karnak D, Parsels LA, Parsels JD, Davis MA, et al. Sensitization of Pancreatic Cancers to Gemcitabine Chemoradiation by WEE1 Kinase Inhibition Depends on Homologous Recombination Repair. Neoplasia. (2015) 17(10):757–66. doi: 10.1016/j.neo.2015.09.006
172. Parsels LA, Karnak D, Parsels JD, Zhang Q, Vélez-Padilla J, Reichert ZR, et al. PARP1 Trapping and DNA Replication Stress Enhance Radiosensitization with Combined WEE1 and PARP Inhibitors. Mol Cancer Res. (2018) 16(2):222–32. doi: 10.1158/1541-7786.Mcr-17-0455
173. Schutte T, Embaby A, Steeghs N, van der Mierden S, van Driel W, Rijlaarsdam M, et al. Clinical development of WEE1 inhibitors in gynecological cancers: A systematic review. Cancer Treat Rev. (2023) 115:102531. doi: 10.1016/j.ctrv.2023.102531
174. Zhou S, Yung Chan S, Cher Goh B, Chan E, Duan W, Huang M, et al. Mechanism-based inhibition of cytochrome P450 3A4 by therapeutic drugs. Clin Pharmacokinet. (2005) 44(3):279–304. doi: 10.2165/00003088-200544030-00005
175. Heijink AM, Blomen VA, Bisteau X, Degener F, Matsushita FY, Kaldis P, et al. A haploid genetic screen identifies the G1/S regulatory machinery as a determinant of Wee1 inhibitor sensitivity. Proc Natl Acad Sci U.S.A. (2015) 112(49):15160–5. doi: 10.1073/pnas.1505283112
176. Domínguez-Kelly R, Martín Y, Koundrioukoff S, Tanenbaum ME, Smits VA, Medema RH, et al. Wee1 controls genomic stability during replication by regulating the Mus81-Eme1 endonuclease. J Cell Biol. (2011) 194(4):567–79. doi: 10.1083/jcb.201101047
177. Fruman DA, Chiu H, Hopkins BD, Bagrodia S, Cantley LC, Abraham RT. The PI3K Pathway in Human Disease. Cell. (2017) 170(4):605–35. doi: 10.1016/j.cell.2017.07.029
178. LoRusso PM. Inhibition of the PI3K/AKT/mTOR Pathway in Solid Tumors. J Clin Oncol. (2016) 34(31):3803–15. doi: 10.1200/jco.2014.59.0018
179. Ibrahim YH, García-García C, Serra V, He L, Torres-Lockhart K, Prat A, et al. PI3K inhibition impairs BRCA1/2 expression and sensitizes BRCA-proficient triple-negative breast cancer to PARP inhibition. Cancer Discovery. (2012) 2(11):1036–47. doi: 10.1158/2159-8290.Cd-11-0348
180. Liu JF, Palakurthi S, Zeng Q, Zhou S, Ivanova E, Huang W, et al. Establishment of Patient-Derived Tumor Xenograft Models of Epithelial Ovarian Cancer for Preclinical Evaluation of Novel Therapeutics. Clin Cancer Res. (2017) 23(5):1263–73. doi: 10.1158/1078-0432.Ccr-16-1237
181. Konstantinopoulos PA, Barry WT, Birrer M, Westin SN, Cadoo KA, Shapiro GI, et al. Olaparib and α-specific PI3K inhibitor alpelisib for patients with epithelial ovarian cancer: a dose-escalation and dose-expansion phase 1b trial. Lancet Oncol. (2019) 20(4):570–80. doi: 10.1016/s1470-2045(18)30905-7
182. Rascio F, Spadaccino F, Rocchetti MT, Castellano G, Stallone G, Netti GS, et al. The Pathogenic Role of PI3K/AKT Pathway in Cancer Onset and Drug Resistance: An Updated Review. Cancers (Basel). (2021) 13(16):3949. doi: 10.3390/cancers13163949
183. Westin SN, Labrie M, Litton JK, Blucher A, Fang Y, Vellano CP, et al. Phase Ib Dose Expansion and Translational Analyses of Olaparib in Combination with Capivasertib in Recurrent Endometrial, Triple-Negative Breast, and Ovarian Cancer. Clin Cancer Res. (2021) 27(23):6354–65. doi: 10.1158/1078-0432.Ccr-21-1656
184. Turner NC, Oliveira M, Howell SJ, Dalenc F, Cortes J, Gomez Moreno HL, et al. Capivasertib in Hormone Receptor–Positive Advanced Breast Cancer. New Engl J Med. (2023) 388(22):2058–70. doi: 10.1056/NEJMoa2214131
185. Kim H, Xu H, George E, Hallberg D, Kumar S, Jagannathan V, et al. Combining PARP with ATR inhibition overcomes PARP inhibitor and platinum resistance in ovarian cancer models. Nat Commun. (2020) 11(1):3726. doi: 10.1038/s41467-020-17127-2
186. Wethington SL, Shah PD, Martin LP, Tanyi JL, Latif NA, Morgan MA, et al. Combination of PARP and ATR inhibitors (olaparib and ceralasertib) shows clinical activity in acquired PARP inhibitor-resistant recurrent ovarian cancer. J Clin Oncol. (2021) 39(15_suppl):5516–. doi: 10.1200/JCO.2021.39.15_suppl.5516
187. Wethington SL, Shah PD, Martin L, Tanyi JL, Latif N, Morgan M, et al. Combination ATR (ceralasertib) and PARP (olaparib) Inhibitor (CAPRI) Trial in Acquired PARP Inhibitor-Resistant Homologous Recombination-Deficient Ovarian Cancer. Clin Cancer Res. (2023) 29(15):2800–7. doi: 10.1158/1078-0432.Ccr-22-2444
188. Yap TA, Fontana E, Lee EK, Spigel DR, Højgaard M, Lheureux S, et al. Camonsertib in DNA damage response-deficient advanced solid tumors: phase 1 trial results. Nat Med. (2023) 29(6):1400–11. doi: 10.1038/s41591-023-02399-0
189. Yap TA, Tan DSP, Terbuch A, Caldwell R, Guo C, Goh BC, et al. First-in-Human Trial of the Oral Ataxia Telangiectasia and RAD3-Related (ATR) Inhibitor BAY 1895344 in Patients with Advanced Solid Tumors. Cancer Discovery. (2021) 11(1):80–91. doi: 10.1158/2159-8290.Cd-20-0868
190. Bradbury A, Zenke FT, Curtin NJ, Drew Y. The Role of ATR Inhibitors in Ovarian Cancer: Investigating Predictive Biomarkers of Response. Cells. (2022) 11(15):2361. doi: 10.3390/cells11152361
191. Lee JM, Nair J, Zimmer A, Lipkowitz S, Annunziata CM, Merino MJ, et al. Prexasertib, a cell cycle checkpoint kinase 1 and 2 inhibitor, in BRCA wild-type recurrent high-grade serous ovarian cancer: a first-in-class proof-of-concept phase 2 study. Lancet Oncol. (2018) 19(2):207–15. doi: 10.1016/s1470-2045(18)30009-3
192. Gupta N, Huang TT, Nair JR, An D, Zurcher G, Lampert EJ, et al. BLM overexpression as a predictive biomarker for CHK1 inhibitor response in PARP inhibitor-resistant BRCA-mutant ovarian cancer. Sci Transl Med. (2023) 15(701):eadd7872. doi: 10.1126/scitranslmed.add7872
193. Giudice E, Huang TT, Nair JR, Zurcher G, McCoy A, Nousome D, et al. The CHK1 inhibitor prexasertib in BRCA wild-type platinum-resistant recurrent high-grade serous ovarian carcinoma: a phase 2 trial. Nat Commun. (2024) 15(1):2805. doi: 10.1038/s41467-024-47215-6
194. Kim MG, Pak JH, Choi WH, Park JY, Nam JH, Kim JH. The relationship between cisplatin resistance and histone deacetylase isoform overexpression in epithelial ovarian cancer cell lines. J Gynecol Oncol. (2012) 23(3):182–9. doi: 10.3802/jgo.2012.23.3.182
195. Koprinarova M, Botev P, Russev G. Histone deacetylase inhibitor sodium butyrate enhances cellular radiosensitivity by inhibiting both DNA nonhomologous end joining and homologous recombination. DNA Repair (Amst). (2011) 10(9):970–7. doi: 10.1016/j.dnarep.2011.07.003
196. Adimoolam S, Sirisawad M, Chen J, Thiemann P, Ford JM, Buggy JJ. HDAC inhibitor PCI-24781 decreases RAD51 expression and inhibits homologous recombination. Proc Natl Acad Sci U S A. (2007) 104(49):19482–7. doi: 10.1073/pnas.0707828104
197. Konstantinopoulos PA, Wilson AJ, Saskowski J, Wass E, Khabele D. Suberoylanilide hydroxamic acid (SAHA) enhances olaparib activity by targeting homologous recombination DNA repair in ovarian cancer. Gynecol Oncol. (2014) 133(3):599–606. doi: 10.1016/j.ygyno.2014.03.007
198. Wilson AJ, Sarfo-Kantanka K, Barrack T, Steck A, Saskowski J, Crispens MA, et al. Panobinostat sensitizes cyclin E high, homologous recombination-proficient ovarian cancer to olaparib. Gynecol Oncol. (2016) 143(1):143–51. doi: 10.1016/j.ygyno.2016.07.088
199. Wilson AJ, Lalani AS, Wass E, Saskowski J, Khabele D. Romidepsin (FK228) combined with cisplatin stimulates DNA damage-induced cell death in ovarian cancer. Gynecol Oncol. (2012) 127(3):579–86. doi: 10.1016/j.ygyno.2012.09.016
200. Gupta VG, Hirst J, Petersen S, Roby KF, Kusch M, Zhou H, et al. Entinostat, a selective HDAC1/2 inhibitor, potentiates the effects of olaparib in homologous recombination proficient ovarian cancer. Gynecol Oncol. (2021) 162(1):163–72. doi: 10.1016/j.ygyno.2021.04.015
201. Gabbasov R, Benrubi ID, O'Brien SW, Krais JJ, Johnson N, Litwin S, et al. Targeted blockade of HSP90 impairs DNA-damage response proteins and increases the sensitivity of ovarian carcinoma cells to PARP inhibition. Cancer Biol Ther. (2019) 20(7):1035–45. doi: 10.1080/15384047.2019.1595279
202. Choi YE, Battelli C, Watson J, Liu J, Curtis J, Morse AN, et al. Sublethal concentrations of 17-AAG suppress homologous recombination DNA repair and enhance sensitivity to carboplatin and olaparib in HR proficient ovarian cancer cells. Oncotarget. (2014) 5(9):2678–87. doi: 10.18632/oncotarget.1929
203. Hoy SM. Pimitespib: First Approval. Drugs. (2022) 82(13):1413–8. doi: 10.1007/s40265-022-01764-6
204. Donati B, Lorenzini E, Ciarrocchi A. BRD4 and Cancer: going beyond transcriptional regulation. Mol Cancer. (2018) 17(1):164. doi: 10.1186/s12943-018-0915-9
205. Baratta MG, Schinzel AC, Zwang Y, Bandopadhayay P, Bowman-Colin C, Kutt J, et al. An in-tumor genetic screen reveals that the BET bromodomain protein, BRD4, is a potential therapeutic target in ovarian carcinoma. Proc Natl Acad Sci U.S.A. (2015) 112(1):232–7. doi: 10.1073/pnas.1422165112
206. Rhyasen GW, Yao Y, Zhang J, Dulak A, Castriotta L, Jacques K, et al. BRD4 amplification facilitates an oncogenic gene expression program in high-grade serous ovarian cancer and confers sensitivity to BET inhibitors. PloS One. (2018) 13(7):e0200826. doi: 10.1371/journal.pone.0200826
207. Drumond-Bock AL, Bieniasz M. The role of distinct BRD4 isoforms and their contribution to high-grade serous ovarian carcinoma pathogenesis. Mol Cancer. (2021) 20(1):145. doi: 10.1186/s12943-021-01424-5
208. Pan-cancer analysis of whole genomes. Nature. (2020) 578(7793):82–93. doi: 10.1038/s41586-020-1969-6
209. Andrikopoulou A, Liontos M, Koutsoukos K, Dimopoulos MA, Zagouri F. Clinical perspectives of BET inhibition in ovarian cancer. Cell Oncol (Dordr). (2021) 44(2):237–49. doi: 10.1007/s13402-020-00578-6
210. Karakashev S, Zhu H, Yokoyama Y, Zhao B, Fatkhutdinov N, Kossenkov AV, et al. BET Bromodomain Inhibition Synergizes with PARP Inhibitor in Epithelial Ovarian Cancer. Cell Rep. (2017) 21(12):3398–405. doi: 10.1016/j.celrep.2017.11.095
211. Watanabe N, Broome M, Hunter T. Regulation of the human WEE1Hu CDK tyrosine 15-kinase during the cell cycle. EMBO J. (1995) 14(9):1878–91. doi: 10.1002/j.1460-2075.1995.tb07180.x
212. Mir SE, De Witt Hamer PC, Krawczyk PM, Balaj L, Claes A, Niers JM, et al. In silico analysis of kinase expression identifies WEE1 as a gatekeeper against mitotic catastrophe in glioblastoma. Cancer Cell. (2010) 18(3):244–57. doi: 10.1016/j.ccr.2010.08.011
213. Yan S, Michael WM. TopBP1 and DNA polymerase alpha-mediated recruitment of the 9-1-1 complex to stalled replication forks: implications for a replication restart-based mechanism for ATR checkpoint activation. Cell Cycle. (2009) 8(18):2877–84. doi: 10.4161/cc.8.18.9485
214. Wilson AJ, Stubbs M, Liu P, Ruggeri B, Khabele D. The BET inhibitor INCB054329 reduces homologous recombination efficiency and augments PARP inhibitor activity in ovarian cancer. Gynecol Oncol. (2018) 149(3):575–84. doi: 10.1016/j.ygyno.2018.03.049
215. Bechter O, Schöffski P. Make your best BET: The emerging role of BET inhibitor treatment in malignant tumors. Pharmacol Ther. (2020) 208:107479. doi: 10.1016/j.pharmthera.2020.107479
216. Bock C, Datlinger P, Chardon F, Coelho MA, Dong MB, Lawson KA, et al. High-content CRISPR screening. Nat Rev Methods Primers. (2022) 2(1):9. doi: 10.1038/s43586-022-00098-7
Keywords: ovarian cancer, homologous recombination proficiency, treatment resistance, PARP inhibitor, CDK inhibitor, PI3K inhibitor, antibody drug conjugate (ADC), vaccine
Citation: Stiegeler N, Garsed DW, Au-Yeung G, Bowtell DDL, Heinzelmann-Schwarz V and Zwimpfer TA (2024) Homologous recombination proficient subtypes of high-grade serous ovarian cancer: treatment options for a poor prognosis group. Front. Oncol. 14:1387281. doi: 10.3389/fonc.2024.1387281
Received: 17 February 2024; Accepted: 15 May 2024;
Published: 04 June 2024.
Edited by:
Robb Hollis, University of Edinburgh, United KingdomReviewed by:
Tricia Roxburgh, University of Glasgow, United KingdomCopyright © 2024 Stiegeler, Garsed, Au-Yeung, Bowtell, Heinzelmann-Schwarz and Zwimpfer. This is an open-access article distributed under the terms of the Creative Commons Attribution License (CC BY). The use, distribution or reproduction in other forums is permitted, provided the original author(s) and the copyright owner(s) are credited and that the original publication in this journal is cited, in accordance with accepted academic practice. No use, distribution or reproduction is permitted which does not comply with these terms.
*Correspondence: Tibor A. Zwimpfer, dGlib3IuendpbXBmZXJAdW5pYmFzLmNo
Disclaimer: All claims expressed in this article are solely those of the authors and do not necessarily represent those of their affiliated organizations, or those of the publisher, the editors and the reviewers. Any product that may be evaluated in this article or claim that may be made by its manufacturer is not guaranteed or endorsed by the publisher.
Research integrity at Frontiers
Learn more about the work of our research integrity team to safeguard the quality of each article we publish.