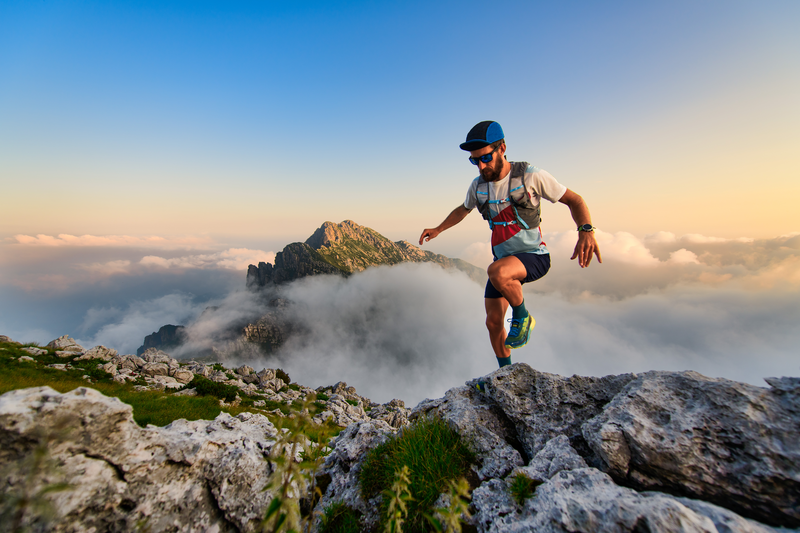
94% of researchers rate our articles as excellent or good
Learn more about the work of our research integrity team to safeguard the quality of each article we publish.
Find out more
REVIEW article
Front. Oncol. , 23 April 2024
Sec. Pharmacology of Anti-Cancer Drugs
Volume 14 - 2024 | https://doi.org/10.3389/fonc.2024.1387251
Cancer treatment is associated with long lasting cognitive impairment in cancer survivors. This cognitive impairment is often termed cancer related cognitive impairment (CRCI). Cancer survivors treated for tumors outside the central nervous system are increasingly diagnosed with CRCI. The development of strategies to mitigate the negative effects of cancer treatment on the brain are crucial. Although neuroimaging research has proposed several candidate mechanisms, the pathogenic underpinnings of CRCI remain uncertain. As such, preventative and treatment strategies have not been identified. To fill these gaps, animal models play a vital role in isolating underlying contributing mechanisms that promote CRCI and in testing new therapeutic approaches.
There are an estimated 18.1 million cancer survivors in the United States as of 2022 (1). Advances in early screening techniques and cancer treatment have extended cancer patients survival (2–5). With this increased longevity of cancer patients, the importance of quality of life is a vital survivorship issue (2, 4). Among the adverse effects of cancer treatment is a syndrome of cognitive impairment known as cancer related cognitive impairment (CRCI) (5). Cancer survivors affected by CRCI report visual and verbal memory impairment, learning and attention impairment, and difficulty processing new information and multitasking (6–8). CRCI decreases cancer survivors’ quality of life by negatively impacting their daily tasks and personal relationships, negatively impacting work performance and, decreasing their ability to return to work (9–12). This review will cover the proposed mechanisms for CRCI in cancer patients and the animal models being used to determine potential therapeutic targets.
Cancer related cognitive impairment affects cancer patients with non-central nervous system cancers before, during and after treatment (2, 13). The combined adverse effects of both cancer and cancer treatment cause CRCI (11, 13). The cancer treatments implicated in cognitive impairment include chemotherapy, radiation therapy, surgery, endocrine therapy, and immunotherapy (8, 11, 13). CRCI is estimated to effect 75% of cancer patients for durations up to 10 years following treatment for solid tumors including breast, lung, intestinal, ovarian, prostatic and testicular tumors (5, 7). CRCI is documented most thoroughly to date in women with breast cancer (BC), which represents 22% of all cancer survivors in the United States (2). BC survivors have reported cognitive dysfunction for 5-10 years following treatment with chemotherapy and/or radiation therapy (7, 14).
The diagnosis of CRCI is challenging as there is no clear consensus on a diagnostic algorithm best applying the numerous measures that assess cognitive function (13). The International Cancer and Cognitive Task Force (ICCTF) established criteria for determining cognitive impairment in cancer patients and recommends the use of neuropsychological tests that are sensitive for the cognitive domains most impaired by cancer treatment (15). Such neuropsychological objective tests remain the gold standard to objectively assess cognitive impairment (15, 16). Specifically, the ICCTF recommends the Hopkins Verbal Learning Test-Revised (HVLT-R), the Trail Making Test (TMT) and the Controlled Oral Word Association (COWA) or the Multilingual Aphasia Examination (16). These tests measure learning, memory, attention, processing speed and executive function, which are the principal cognitive domains affected in CRCI (16). These neuropsychological tests in cancer patients fail to reliably correlate with patients’ clinical cognitive impairment (15). This gap between neuropsychological test performance and self-reported clinical signs may result from compensatory activation of unaffected brain regions in cancer survivors to preserve performance on neuropsychological testing (15). Alternatively, patient self-reported measures, including the Functional Assessment of Cancer Therapy-Cognitive (FACT-Cog) have been shown to more strongly associate with the symptoms reported by cancer patients compared to their performance on objective neuropsychological testing (2, 15).
Standard neuroimaging in patients suffering from CRCI is frequently unremarkable (2, 17). Advanced imaging studies using volumetric magnetic resonance imaging (MRI), diffusion tensor imaging (DTI) and positron emission tomography have shown widespread reductions in gray matter volume, changes in white matter connectivity, alterations in brain activation and neuroinflammation (activation of microglia and astrocytes) in cancer patients with CRCI (2, 4, 5, 18, 19).
Chemotherapy has been implicated in causing CRCI, with terms such as “chemobrain” or “chemofog” often used to refer to this syndrome (7, 20–23). The cognitive domains affected in chemotherapy-associated CRCI include learning, memory, attention, executive function, language and processing speed (2, 8). In longitudinal studies, the incidence of CRCI after chemotherapy is reported from 17% to over 70% among cancer patients (2). Known risk factors that increase incidence of CRCI after chemotherapy include non-cancer related comorbidities, anemia, and genetic predisposition (24, 25). The proposed mechanisms for chemotherapy-associated CRCI include decreased neurogenesis, axonal degeneration and demyelination, reduction in dendrite volume and length, increased oxidative stress and free radical formation, neuroinflammation, toxicity to CNS progenitor cells, microglia apoptosis, blood brain barrier disruption, brain blood flow alterations, myelin degradation, production of damage associated molecular patterns, cytokine-induced neurotoxicity, reduced glucose metabolism, alteration in brain water content, and fluctuations in neurotransmitter levels (5, 14, 22, 23, 25, 26). Select chemotherapy drugs, including 5-fluorouracil (5-FU), carmustine (BCNU), lomustine (CCNU), temozolomide (TMZ) cross the intact blood brain barrier to directly injure the brain. Additional drugs such as methotrexate (MTX), paclitaxel, docetaxel (DTX), cisplatin may cross in small concentrations to negatively affect brain tissue (11, 22, 25–27). However, for drugs like doxorubicin (DOX), known to be associated with CRCI, it is unclear how it alters neural cells. Chemotherapy-associated CRCI is often mild to moderate in severity and can be dependent on the chemotherapy agent received and the dose (2, 8, 28). Chemotherapy agents such as MTX, BCNU, fludarabine, cytarabine and 5-FU can cause severe neuropathological diseases including encephalopathy and dementia (2).
The bulk of our understanding of chemotherapy-associated CRCI stems from studies of cognitive impairment in BC survivors during and after chemotherapy treatment (summarized in Table 1) (19, 29, 64, 80–82). BC patients after chemotherapy treatment report deficits in cognitive domains consisting of executive function, working memory, attention, concentration, processing speed, cognitive flexibility, visual and verbal memory (83). Various structural brain changes have been described in these BC patients, including reduction in gray matter, decreases in white matter integrity, altered brain activation, neuroinflammation and changes in brain functional connectivity (4, 8, 15, 18, 28, 84–86). PET imaging acquired 5-10 years after treatment in BC patients demonstrated altered frontal cortex activation during a short term verbal memory task in survivors treated with chemotherapy compared to survivors not treated with chemotherapy (84). One study used PET-MR imaging and neuropsychological testing to demonstrate that compared to patients treated with surgery alone, BC patients treated with surgery and adjuvant chemotherapy had higher brain local translocator protein expression, a measure of neuroinflammation that was associated with worse cognitive performance (4).
Table 1 Summary of findings from clinical studies evaluating cancer treatments effect on cognitive function.
Mechanistically, numerous studies have implicated systemic inflammation as a major contributor to chemotherapy-associated CRCI in BC patients. Chemotherapy associated inflammation is a potential significant contributor to CRCI; a thorough review of this subject is beyond the scope of the paper but has been well described in recent years (15, 80, 81). Chemotherapy agents have been reported to elevate multiple pro-inflammatory markers including interlukin-6 (IL-6), IL-1 beta (IL-1β), IL-5, IL-10, reactive oxygen species (ROS), IL-8, interferon gamma (IFN-α), IL-12, IL-17, and tumor necrosis factor alpha (TNF-α) (15, 82). Cognitive performance in chemotherapy-treated BC patients have specifically been linked to serum inflammatory markers (11, 29, 30, 64). One study found an association between increased concentrations of soluble tumor necrosis factor receptor I and II and decline in visual memory (29). Another study demonstrated a significant interaction between serum IL-6, TNF-α levels and verbal memory difficulties in BC patients treated with chemotherapy (64). In BC patients, one study showed that higher elevations in IL-6 and IL-1β were associated with more severe cognitive impairment (31) These studies support that select chemotherapy agents increase circulating inflammatory markers which subsequently cross the blood brain barrier to activate microglia and induce neuronal cell death (23, 87).
Several CRCI studies have evaluated the long-term impact of the adjuvant chemotherapy protocol CMF that includes cyclophosphamide (CYP), MTX and 5-FU for early-stage breast carcinoma (32). One study evaluated cognitive impairment via neuropsychological testing and self-reporting in BC patients 21 years following adjuvant CMF chemotherapy (65). These survivors performed worse on cognitive tests of learning, verbal memory, information processing speed, inhibition and psychomotor speed compared to non-cancer-bearing women (65). BC survivors also reported memory complaints that were not associated with neuropsychological testing, a commonly reported phenomena in other studies (20, 32, 33, 65). One study compared cognitive impairment at 2 years in BC survivors treated with surgery and adjuvant CMF and age-matched BC survivors treated with surgery only (32). In this study, CMF-treated survivors reported significantly more difficulties with memory and concentration and increased incidence of cognitive impairment than BC patients treated with surgery alone (32). An additional study evaluated stage I and II breast carcinoma patients 3-18 months after CMF treatment and found that 75% of patients scored 2 standard deviations below test norms (corrected for age, education and gender) on one or more neuropsychological tests (34). Another study showed that BC survivors treated with CMF at least 10 years prior had significant impairment in cognitive domains of executive function, working memory and divided attention compared to age, education status matched non-cancer controls (66). The underlying mechanisms leading to altered cognition are not clear. However, one study showed that 20 years after adjuvant CMF treatment, BC survivors had increased levels of systemic inflammation (increased granulocyte count to lymphocyte count ratio (GLR), increased platelet count to lymphocyte count ratio and increased platelet count times GLR) and lower cognitive performance compared to healthy controls (67).
While CMF-treated BC patients have provided abundant information regarding this syndrome, CRCI has also been documented and evaluated following administration of many adjuvant chemotherapy protocols. Chemotherapy-treated BC patients (most of whom received 5-FU, DOX and CYP) in one study were more likely to show cognitive impairment on neurophysiological tests than healthy women controls (21). In another study, BC survivors treated with a wide variety of multi-agent treatment protocols incorporating DOX, CYP, DTX, paclitaxel, MTX, 5-FU or epirubicin, demonstrated deficits in episodic memory as compared to age- and sex-matched non-cancer controls 6 months following treatment (20). One-to-two years following treatment with either DOX and CYP or CYP, epirubicin and 5-FU, significantly more BC survivors demonstrated moderate to severe cognitive impairment compared to age, sex, and educational status matched non-cancer controls (35). A recent prospective study showed that BC patients treated with a diverse repertoire of multi-agent chemotherapy protocols were at a threefold greater risk of cognitive decline compared to BC patients treated with hormone therapy (36). Notably, a prospective study showed that cognitive impairment in chemotherapy-treated BC patients was worse in the presence of anxious or depressive symptoms (30).
Chemotherapy-induced CRCI has been demonstrated in other malignancies (Table 1), including hematopoietic malignancies and testicular cancer (TC) (37, 38, 40, 41, 43, 68). Cancer survivors, including patients treated for non-Hodgkin’s and Hodgkin’s lymphoma, have cognitive impairment in domains including memory, learning and executive function for at least 6 months following chemotherapy compared to healthy controls (41, 42, 44). Twenty percent of TC patients report worsening cognitive impairment 2 years following multiagent bleomycin, etoposide and cisplatin (BEP) therapy using quality-of-life questionnaires (37, 69). Interestingly, TC patients treated with BEP did not have significant cognitive differences on neuropsychological testing from TC patients treated with radiation therapy and surgery (69, 70, 74, 75). BEP therapy has also been shown to cause significant brain networking changes and altered white matter microstructure in TC patients for durations up to 14 years after treatment compared to TC patients who did not receive chemotherapy (39, 73). Additional studies of TC survivors with long-term follow up for at least 2 years post treatment have found cognitive impairment following multiagent chemotherapy with or without ECRT compared to survivors treated with surgery alone (71, 72).
Extracranial radiation therapy (ECRT) is associated with cognitive impairment in cancer patients. There are substantial health implications of this phenomenon because over 50% of all newly diagnosed cancer patients will receive ECRT as part of their treatment protocol (7). Importantly, radiotherapy is prescribed in an estimated 56% of breast and prostate cancer patients (88, 89). Unsurprisingly, brain irradiation for primary or metastatic tumors causes brain damage leading to neurocognitive decline (5, 6). Somewhat less intuitively, cognitive impairment has been associated with ECRT directed to tumor-bearing anatomic sites outside the brain (5, 7, 45, 46). Little is known regarding the potential mechanism(s) linking ECRT and CRCI.
Similar to chemotherapy-associated CRCI, clinical evidence for ECRT-associated CRCI has arisen from BC survivors (Table 1). Studies have shown impairment in multiple cognitive domains in BC survivors after ECRT including complex cognition, attention, memory, and executive function (7, 20, 23). One study showed that 6 months following surgery and ECRT (no chemotherapy), BC survivors demonstrated higher rates of impairment in attention compared to age matched non-cancer control women (20). A separate study that assessed cognitive function via neuropsychological tests and self-reporting showed that 6 months after treatment, BC patients treated with ECRT only or chemotherapy and ECRT (chemoRT) had similar prevalence of cognitive impairment (47). This cognitive dysfunction is robust and lasts years following ECRT. BC patients treated with ECRT only or chemoRT sustained cognitive impairment at 1 year post ECRT (45). An additional longitudinal study found that BC patients treated with ECRT or chemoRT demonstrated worse cognitive performance compared to age matched non-cancer-bearing women in areas of executive function and processing speed up to 3 years post treatment (76). Similar to chemotherapy-induced CRCI, cognitive dysfunction has been documented in patients treated with ECRT for a variety of solid and hematologic tumor types (48). Moreover, this broad study supported that the impact of treatment extends well beyond resolution of acute radiation toxicities, as cancer patients treated with chemotherapy, ECRT or chemoRT reported problems with memory and concentration up to 6 months post treatment (48). ECRT-induced CRCI has also been documented in TC survivors (69, 71). Indeed, in one study with follow-up of TC survivors for over 20 years post treatment documented cognitive impairment with ECRT compared to age matched healthy controls (75).Similar to the proposed mechanisms underlying the contribution of chemotherapy to CRCI, it has been hypothesized that ECRT exerts its bystander cognitive impact through the circulatory trafficking of ECRT-induced pro-inflammatory cytokines to the brain that subsequently induce cognitive impairment (23, 46, 90, 91). Pro-inflammatory cytokines that are produced after ECRT include IL-1β, IL-1α, TNF-α, IL-6, IL-4, INF-ϒ, IL-8 (91, 92). IL-6 has been implicated as a potential cytokine target, as one study demonstrated memory deficits identified in BC patients up to 7 months post-ECRT were partially mediated by elevated plasma IL-6 levels (46).
Chemotherapy and ECRT have garnered abundant attention as critical treatment modalities linked to CRCI. However, deleterious cognitive effects are reported with a diagnosis of cancer as well as with almost all cancer treatments, including surgery, endocrine therapy and immunotherapy (Table 1) (2, 93). Alarmingly, studies estimate that 40% of BC patients exhibit CRCI before the start of therapy; this may therefore be exacerbated with treatment (2). Early identification of patients at risk for CRCI, as well as knowledge of signals that contribute to CRCI, may aid in development of both preventive and mitigative strategies.
Endocrine therapy can cause cognitive impairment in cancer patients and appears to predominately affect the domains of verbal memory and processing speeds (2, 8, 94). Specific drugs associated with CRCI include aromatase inhibitors, selective estrogen receptor modulators/agonists and androgen deprivation therapy (8, 49, 77, 94, 95). The proposed mechanisms by which endocrine therapies cause CRCI are reduction in brain N-methy-D-aspartate receptor concentration, down regulation of brain derived neurotrophic factor expression, decreased antioxidant capacity of cells in the brain, and decreased amyloid β clearance (94). Use of tamoxifen, a commonly prescribed and important adjuvant therapy for women with hormone receptor positive BC, has been correlated with a reduction on measures of memory, verbal fluency, visuospatial functioning and processing speed (2, 77). In one study, women taking tamoxifen were more than twice as likely to report seeing a physician for memory issues as compared to BC patients not taking tamoxifen (96). Another study found that adjuvant hormonal therapy in BC patients has been associated with decreased verbal memory and processing speed (95). Tamoxifen may be given following chemotherapy or concurrent with chemotherapy, which may confound the effects of each individual drug. However, tamoxifen appears to play an important role in mediating CRCI as BC patients treated with tamoxifen and chemotherapy in one study had increased declines in visual memory and verbal working memory versus patients treated with chemotherapy alone (50). Prostate cancer patients treated with androgen deprivation therapies, including abiraterone acetate and enzalutamide, also report cognitive impairment (49, 94). One study found that patients with metastatic prostate cancer treated with enzalutamide had a significantly higher risk of clinically meaningful and worsening cognitive impairment than patients treated with abiraterone acetate plus prednisone up to 3 months after therapy (49).
Immunotherapies including cytokines, checkpoint inhibitors, chimeric antigen receptor (CAR) T cell therapies and hematopoietic stem cell transplantation have also been associated with CRCI (43, 51, 58, 59, 62, 94, 97). One study in patients with chronic myelogenous leukemia treated with interferon-alpha (INF-α) (with or without chemotherapy) showed declines in information processing and executive function when assessed by neuropsychological testing (51). This study also showed that 50% of the patients had significant declines in performance on one or more neuropsychological tests (51). Patients with melanoma, small cell lung cancer, non-small cell lung cancer and Merkel-cell carcinoma have exhibited neurotoxicity following immune checkpoint inhibitors five weeks after treatment (98). Approximately one-third of cancer patients had neurocognitive impairment after treatment with the popular immune checkpoint inhibitor pembrolizumab (52). Similar findings were found in a cross-sectional study, in which 41% of cancer survivors treated with the checkpoint inhibitor ipilimumab, had deficits in working memory and verbal memory (53). Cognitive impairment extends to CAR T-cell therapy as well, with 36% of 84 lymphoma patients treated with a CD19-targeted CAR T-cell developed severe cognitive impairment after treatment (54, 55). Up to 37% of patients with hematological neoplasms treated with CD-19 targeted CAR T-cell therapy reported cognitive impairment in one or more cognitive domains including memory, language, attention, and executive functioning, even up to 90 days after treatment (55, 56). Following allogeneic hematopoietic stem cell transplant for treatment of hematopoietic neoplasia, patients may demonstrate decline in verbal learning, reaction time, working memory, learning, executive function, attention, and psychomotor speed assessed by neuropsychological testing (58, 60, 61, 63, 78). In one study, up to 38% of patients with hematopoietic neoplasia had at least mild cognitive impairment 5 years following allogeneic bone marrow transplant (79). A separate study suggested that 41% of patients with hematopoietic neoplasia showed impairment in at least 1 cognitive domain one year post allogeneic bone marrow transplant (62).
Immunotherapies may contribute to CRCI via microglia-mediated neuronal death secondary to the production, circulation, and blood brain barrier penetration of proinflammatory cytokines (94). This supposition is supported by one study of non-CNS cancer patients demonstrating cognitive impairment that was correlated with pro-inflammatory plasma biomarkers including interferon-gamma (INF-ϒ), interleukin one beta (IL-1β), interleukin two (IL-2) and fibroblast growth factor two (FGF2) (57).
Clinical studies demonstrate serious cognitive impairment in cancer survivors that impacts quality-of-life measures for years following local and systemic treatments. Several treatments have been linked to changes in the brains of cancer patients, and to pro-inflammatory biomarkers in circulation. Future studies to identify reliable biomarkers may prove beneficial for detection, monitoring and response to treatment strategies aimed at preventing or mitigating CRCI.
Animal models of CRCI are critical to elucidating the mechanism(s) by which cancer treatments contribute to CRCI, and to the discovery of neuro-protective strategies that aim to improve quality of life (26, 99). The majority of preclinical studies use rodents including mice and rats to model CRCI in cancer patients (23, 99). In rodent studies of CRCI, experiments use a systemic approach to control for confounding variables in human cancer patients, including comorbidities, age, cancer type, disease progression, differences in baseline cognitive testing, and treatment regimens (26, 100). Through this approach, animal studies can account for age, sex, health status, and environment while also allowing for proper negative controls. Such rodent studies allowed for the assessment of the neurotoxic effects of a single cancer treatment agent, the characterization of potential mechanisms underlying cognitive deficits seen in cancer patients, and identified the cognitive domains affected by cancer treatments (23, 26). Preclinical studies have explored the cognitive and neurobiological effects of tumors with or without cancer treatments and investigated the effects of cancer treatment alone in healthy, tumor free rodents (23, 87, 101). Without question, selection of appropriate rodent models allow the acquisition of information that cannot be generated in human patients, thus addressing critical gaps in our knowledge of CRCI development.
Rodent studies have characterized the scope and mechanistic underpinnings of the cognitive impact of cancer treatments in both tumor-bearing and non-tumor bearing rodents. This has included studies of chemotherapy (single or multi-agent), ECRT, surgery, hormonal therapy and immunotherapy (5, 23, 26, 87, 93, 102, 103). Within the chemotherapy space, rodent studies have evaluated the effects of intravenous or intraperitoneal administration of alkylating agents (CYP, temozolomide, thiotepa), platinums (cisplatin, oxaliplatin, carboplatin), antimetabolites (MTX, 5-FU, cytosine arabinoside, cytarabine), anthracycline (DOX, epirubicin), antimicrotubule agents (vincristine, paclitaxel, DTX), cytosine arabinoside (cytarabine) and topoisomerase I inhibitor (topotecan) (5, 23, 26, 87, 99). Rodent studies investigating the mechanisms of ECRT induced CRCI, include ECRT models of non-CNS cancer treatment (5, 7, 12, 102). In this model, ECRT is represented by a single dose of ECRT to an extracranial region, such as the skin of the hindlimb, in tumor-free or tumor-bearing animals (5, 7, 12, 102).
Behavioral testing in rodents (Table 2) has bolstered the findings of long-lasting, potentially severe cognitive deficits following chemotherapy from observational studies of human cancer patients (15). Importantly, behavioral tests used in rodent CRCI studies evaluate similar cognitive domains as those reported to be affected in human CRCI studies, maximizing translational strength of these models. Testing in rodents evaluates memory, learning, executive function, attention and cognitive flexibility (5, 26, 99). In exploring the impact of chemotherapy on cognition in rodents, researchers have evaluated a wide variety of chemotherapy regimens in both tumor-free and tumor-bearing animals (23, 26). This work has revealed deficits in spatial working memory in rodents treated with cisplatin, vincristine, CYP, DOX, BCNU, topotecan MTX + 5-FU, CYP + DOX or CYP + DOX + 5-FU (99, 104–111, 152). Additionally, recognition/visual memory was reduced in rodents following single agent administration of cisplatin, DOX, MTX, 5-FU, CYP, DTX, thiotepa and oxaliplatin (OXP) (5, 100, 112–122). Multi-agent chemotherapy including CYP + DOX and OXP + 5-FU also resulted in impaired recognition/visual memory (123–127). Rodent learning was diminished after administration of several single and multiagent protocols, including cisplatin, BCNU, MTX, CYP, DOX, OXP, TMZ, MTX + 5-FU, CYP + DOX (128–138). Rodents have decreased executive function after treatment with the following chemotherapy agents MTX, 5-FU, cytarabine, and MTX + 5-FU (139–143). Some chemotherapy agents including carboplatin, DTX, CYP and topotecan also increase attention deficits (99, 144). Finally, cognitive flexibility appears to be impaired in rodents treated with 5-FU, MTX + cytarabine, paclitaxel and cisplatin (139, 145–148). Collectively, the broad and significant negative impact that systemic chemotherapy has on cognitive function in rodent models mirrors cognitive impairment described in pediatric and adult cancer patients.
Table 2 Summary of findings from pre-clinical studies evaluating cancer treatments effect on cognitive function.
The impact of ECRT on cognition has been less thoroughly explored in rodent models but is of recent interest. Most notably, one study demonstrated that hippocampal dependent memory was impaired in mice treated with ECRT as compared to untreated control mice (5). Importantly, the memory deficits in these mice were comparable to mice treated with DOX and DOX + ECRT (5). The results of this study complement those reported in a carcinoma-bearing mouse model in which combination immunotherapy and ECRT lead to impairment in visual/recognition memory as compared to non-treated, tumor free control mice (102).
Hormonal therapy in intact rodents has also been shown to negatively impact memory, learning and executive functioning (103, 141). One study found that tumor-free mice treated with tamoxifen, MTX and/or 5-FU had compromised learning and executive function as compared to untreated control mice (141). This work parallels other studies that have shown that tamoxifen alone can cause impairment in memory, learning and executive function (149–151). Finally, surgical anesthesia has been shown to induce deficits in working memory and recognition/visual memory (93). A study reported that rats anesthetized with sevoflurane inhalant demonstrate diminished working and recognition memory as compared to untreated control rats (93).
While the descriptive confirmation of the impact of cancer treatment on the development of CRCI has been well documented in rodent models, studies have also provided invaluable insights into the mechanistic links between treatment and CRCI. Such work has shown that cancer treatments result in reduced neurogenesis, neuroinflammation, reduced brain glucose metabolism, alterations in brain neurotransmitter levels, decreased brain myelination, detrimental changes in neuron axons and dendrites, increased cellular damage in the hippocampus, reduction of white matter integrity, DNA damage, oxidative stress and mitochondrial dysfunction in the hippocampus (26, 121, 134, 148).
Multiple studies have positively correlated the behavioral deficits observed in treated mice to pathological changes within the brain (106, 142). One study demonstrated a positive correlation between DNA damage in the brain cells of mice treated with 5-FU and the learning deficit seen in these mice (142). Another study found in rats treated with 5-FU and methotrexate a significant correlation between decreased neurogenesis in the hippocampus of these rats and impairment of hippocampal memory (106). Similar associations have been found in BC patients treated with chemotherapy (4).
The impact of single-agent and multi-agent chemotherapy on neurogenesis, particularly within the hippocampus, is well-documented (26, 87, 153). Specifically, 14 independent rodent studies have shown that treatment with BCNU, MTX, 5-FU, CYP, DOX, TMZ, cisplatin, thiotepa, DOX + CYP, MTX+5-FU and CYP + DOX + 5-FU results in decreased neurogenesis in the hippocampal dentate gyrus as compared to non-chemotherapy treated rodents (100, 106, 111, 112, 122, 124, 129, 132, 135, 145, 148, 154–156). One study in rats expanded upon these findings and demonstrated that treatment with MTX+5-FU resulted in detectable cognitive dysfunction that correlated with reduced hippocampal neurogenesis (106). It is not yet clear how systemic chemotherapy impacts neurogenesis. Decreased levels of hippocampal brain-derived neurotropic factor and doublecortin may disrupt neurogenesis, based on one study in rats following 5-FU administration (116). Reduced neurogenesis following chemotherapy is one of several potential mechanisms by which chemotherapy alters cognition. This occurrence has profound implications in human patients because reduced neurogenesis may also contribute to depression and accelerate cognitive diseases like Alzheimer’s (157).
There is also robust evidence that cancer treatments induce neuroinflammation, which can lead to cognitive dysfunction itself while also contributing to reduced neurogenesis. Numerous studies have documented neuroinflammation or upregulation of brain proinflammatory cytokines, or both in rodents treated with chemotherapy, ECRT, immunotherapy or combination therapy (5, 100, 158). Specific chemotherapy agents that cause neuroinflammation in rodents include CYP, DOX and MTX (100, 133, 159). Two recent murine studies have also shown that hindlimb irradiation with appropriate blocking of CNS tissue, results in widespread brain astrocytosis and microgliosis (components of neuroinflammation) up to 30 days after ECRT treatment compared to control mice (5, 7). A similar finding was described in both tumor-free and tumor-bearing mice treated with immunotherapy or hindlimb irradiation or both had increased activated microglia in the hippocampus and cortex as compared to untreated control mice (102). These studies are complemented by work documenting increased levels of pro-inflammatory reactive oxygen species (ROS) in rodent models of CRCI. Cancer treatments in rodents increase ROS characterized by increased oxidative stress and DNA damage (87). The chemotherapy agents cisplatin, carboplatin, CYP, BCNU, cytarabine, DOX, and MTX have specifically been shown to increase brain oxidative stress (138, 160–168). Of import to cancer patients undergoing surgical procedures, the anesthetic agent sevoflurane has also been shown to cause increased hippocampal oxidative stress in rats (93).
Metabolic derangement has been highlighted in other studies as an important potential contributing factor to CRCI. Decreased brain glucose metabolism has been observed during FDG (fluorodeoxyglucose)-PET scan in rodents treated with either chemotherapy or ECRT (7, 136, 159, 169). Rats treated with CYP or DOX in one study showed decreased glucose metabolism in the medial cortex with both treatments and in the hippocampus in the DOX-treated rats (136). Similar findings were seen in a study in which DOX-treated rats had decreased prefrontal cortical glucose metabolism 30 days post treatment compared to untreated controls (169). MTX treatment to rats also caused reduced hippocampal glucose metabolism with decreased blood vessel density compared to untreated rats (159). Mice treated with hindlimb irradiation alone also developed reduced brain glucose metabolism after treatment compared to their pre-ECRT brain imaging (7).
Alterations in neurotransmitter levels and neurotransmission has been shown in the brains of rodents treated with MTX, 5-FU, carboplatin and DOX (6, 130, 144, 170, 171). Decreased levels of hippocampal norepinephrine, dopamine and serotonin were measured following MTX administration (130). Additionally, impaired dopamine release and update occurred following carboplatin and 5-FU administration in rats (144, 170). In mice, treatment with DOX results in impaired glutamatergic signaling (171). Finally, changes in the structures of rodent neuronal dendrites and axons have been described after chemotherapy (26, 121). Specifically, CYP, cisplatin and cytarabine have shown to reduce dendritic length, volume, spine density and number of branch points (26, 100, 117, 172). Cisplatin given to rats has also resulted in reduced hippocampal pyramidal neuron dendritic branching and spine density up to 28 days after administration (121).
Collectively, animal studies have demonstrated impairment in cognitive domains similar to cancer survivors after systemic and local antineoplastic treatment. Cognitive impairment has been correlated to neuropathological findings in the brain of rodents, which has led to the identification of potential mechanisms of cancer therapy-induced CRCI. Mechanisms implicated in the cause of CRCI include decreased neurotransmission, disruption of the blood brain barrier, impaired neurogenesis, increased neuronal oxidative stress, neuroinflammation, and decreased cerebral blood flow (87). Decreased neurotransmission is thought to be due to pro-inflammatory cytokines such as IL-1β that can lead to the catabolism of neurotransmitters and dysregulation of neurotransmission that can cause cognitive impairment (13, 87). Disruption of the blood brain barrier via peripheral cytokine dysregulation can lead to neuroinflammation causing cognitive impairment (13). Rodent animal models have highlighted that cancer treatments decreases neurogenesis through the production of pro-inflammatory cytokines such as TNF-α and subsequent activation of glial cells (22, 87, 94). Most cancer treatments induce the production of reactive oxygen species and oxidative stress, which in turn leads to neuronal DNA damage (22, 87, 91, 94). Pro-inflammatory cytokines produced in the tumor microenvironment can enter circulation and cross the blood brain barrier, leading to the activation of glial cells (13). Activated glial cells contribute to neuron damage and cognitive impairment (6, 173). Finally, several preclinical models have shown that cancer treatment can reduce cerebral blood flow and glucose metabolism, further contributing to cognitive impairment (7, 87, 94, 174). Importantly, most of the proposed mechanisms include a significant initial contribution from pro-inflammatory mediators. Further work in animal models is needed to target these pro-inflammatory mediators in attempts to elucidate new approaches that may mitigate CRCI.
Quality of life for cancer survivors deserves the spotlight of attention because of its widespread impact on psychosocial and economic well-being. Published literature clearly and repeatedly documents that cancer therapy results in cognitive impairment that persists for years after treatment. However, there are limitations in interpreting published studies in cancer patients due to heterogeneity in patient populations, differences in treatment regimens, a lack of standardized neuropsychologic testing approach, wide variability in the control patient population and lack of assessing the cognitive impact of a cancer diagnosis over time (20, 25, 33, 35, 45–48, 76). These limitations with interpretation of existing data serve as a platform upon which to build prospective preclinical and clinical studies. Future clinical studies should include standardized assessment of cognitive function prior to treatment, during therapy, and with serial monitoring protocols, ideally for the life of the patient. The addition of biomarkers, such as known pro-inflammatory serum cytokines, and functional imaging studies may be a helpful addition to established cognitive function protocols. These studies would provide critical data to aid in the design of optimal cognitive monitoring. The Common Terminology Criteria for Adverse Events (CTCAE) v5.0 guidelines currently includes monitoring for cognitive dysfunction. However, as studies isolate specific proinflammatory mediators or distinguish types of dysfunctions, future versions of CTCAE may opt to include measures for improved monitoring.
Because studies in cancer survivors are often limited by multiple confounding variables, animal models are essential to investigate the neurobiological mechanism by which cancer treatment disrupts the brain. Collectively, CRCI animal models support multiple factors including reduced neurogenesis, neuroinflammation, metabolic derangements, DNA damage, oxidative stress and altered neurotransmission create a cycle of signals to promote cognitive dysfunction (87, 93, 138, 160–168). These studies have provided insight into potential molecular processes by identifying protein and gene differentially expressed in the brain of rodents treated with cancer therapies used in human oncology. Future studies are needed to evaluate equivalent dosing strategies to human cancer therapy including using multi-agent treatment since most cancer patients are exposed to more than one type of anti-cancer therapy (5, 7). Identification of critical signaling disruptions that occur with therapy is vital to the development of effective preventive and mitigative strategies that allow cancer survivors to maintain high cognitive function (5).
KD-D: Writing – original draft, Writing – review & editing. JL: Writing – original draft, Writing – review & editing. DS: Writing – original draft, Writing – review & editing.
The author(s) declare financial support was received for the research, authorship, and/or publication of this article. This study was supported by the University of Minnesota.
The authors declare that the research was conducted in the absence of any commercial or financial relationships that could be construed as a potential conflict of interest.
All claims expressed in this article are solely those of the authors and do not necessarily represent those of their affiliated organizations, or those of the publisher, the editors and the reviewers. Any product that may be evaluated in this article, or claim that may be made by its manufacturer, is not guaranteed or endorsed by the publisher.
CRCI, cancer related cognitive impairment; breast cancer, BC; International Cancer and Cognitive Task Force, (ICCTF); MRI, magnetic resonance imaging; PET, positron emission tomography; 5-FU, 5-fluorouracil; BCNU, carmustine; CCNU, lomustine; TMZ, temozolomide; MTX, methotrexate; DTX, docetaxel; DOX, doxorubicin; CMF, cyclophosphamide, methotrexate and 5-fluorouracil; CYP, cyclophosphamide; FAC, 5-fluorouracil, doxorubicin, cyclophosphamide; IL-6, interlukin-6; TNF-α, tumor necrosis factor alpha; testicular cancer, TC; BEP, bleomycin, etoposide and cisplatin; ECRT, extracranial radiation therapy; chemoRT, chemotherapy and extracranial radiation therapy; CAR, chimeric antigen receptor; OXP, oxaliplatin.
1. Society AC. Cancer Treatment & Survivorship Facts & Figures 2022-2024. Atlanta: American Cancer Society (2022).
2. Wefel JS, Kesler SR, Noll KR, Schagen SB. Clinical characteristics, pathophysiology, and management of noncentral nervous system cancer-related cognitive impairment in adults. CA Cancer J Clin. (2015) 65:123–38. doi: 10.3322/caac.21258
3. Kerstens C, Wildiers HPMW, Schroyen G, Almela M, Mark RE, Lambrecht M, et al. A systematic review on the potential acceleration of neurocognitive aging in older cancer survivors. Cancers (Basel). (2023) 15(4):1215. doi: 10.3390/cancers15041215
4. Schroyen G, Blommaert J, Van Weehaeghe D, Sleurs C, Vandenbulcke M, Dedoncker N, et al. Neuroinflammation and its association with cognition, neuronal markers and peripheral inflammation after chemotherapy for breast cancer. Cancers. (2021) 13:4198. doi: 10.3390/cancers13164198
5. Demos-Davies K, Lawrence J, Rogich A, Lind E, Seelig D. Cancer treatment induces neuroinflammation and behavioral deficits in mice. Front Behav Neurosci. (2022) 16:1067298. doi: 10.3389/fnbeh.2022.1067298
6. Gibson EM, Monje M. Microglia in cancer therapy-related cognitive impairment. Trends Neurosci. (2021) 44:441–51. doi: 10.1016/j.tins.2021.02.003
7. Feiock C, Yagi M, Maidman A, Rendahl A, Hui S, Seelig D. Central nervous system injury – A newly observed bystander effect of radiation. PloS One. (2016) 11:e0163233. doi: 10.1371/journal.pone.0163233
8. Pendergrass JC, Targum SD, Harrison JE. Cognitive impairment associated with cancer: A brief review. Innov Clin Neurosci. (2018) 15:36–44.
9. Janelsins MC, Kohli S, Mohile SG, Usuki K, Ahles TA, Morrow GR. An update on cancer- and chemotherapy-related cognitive dysfunction: current status. Semin Oncol. (2011) 38:431–8. doi: 10.1053/j.seminoncol.2011.03.014
10. Oppegaard KR, Armstrong TS, Anguera JA, Kober KM, Kelly DL, Laister RC, et al. Blood-based biomarkers of cancer-related cognitive impairment in non-central nervous system cancer: A scoping review. Crit Rev Oncol Hematol. (2022) 180:103822. doi: 10.1016/j.critrevonc.2022.103822
11. Hardy SJ, Krull KR, Wefel JS, Janelsins M. Cognitive changes in cancer survivors. Am Soc Clin Oncol Educ Book. (2018) 38:795–806. doi: 10.1200/EDBK_201179
12. Demos-Davies K, Lawrence J, Ferreira C, Seelig D. The distant molecular effects on the brain by cancer treatment. Brain Sci. (2023) 14(2):22. doi: 10.3390/brainsci14010022
13. Olson B, Marks DL. Pretreatment cancer-related cognitive impairment-mechanisms and outlook. Cancers (Basel). (2019) 11(5):687. doi: 10.3390/cancers11050687
14. Pang L, Li W, Yao S, Jing Y, Yin X, Cheng H. Psychological distress is involved in CRCI in breast cancer survivors via mediating cytokine levels. Cancer Med. (2023) 12:11806–15. doi: 10.1002/cam4.5847
15. Lange M, Joly F, Vardy J, Ahles T, Dubois M, Tron L, et al. Cancer-related cognitive impairment: an update on state of the art, detection, and management strategies in cancer survivors. Ann Oncol. (2019) 30:1925–40. doi: 10.1093/annonc/mdz410
16. Wefel JS, Vardy J, Ahles T, Schagen SB. International Cognition and Cancer Task Force recommendations to harmonise studies of cognitive function in patients with cancer. Lancet Oncol. (2011) 12:703–8. doi: 10.1016/S1470-2045(10)70294-1
17. Gibson EM, Monje M. Emerging mechanistic underpinnings and therapeutic targets for chemotherapy-related cognitive impairment. Curr Opin Oncol. (2019) 31:531–9. doi: 10.1097/CCO.0000000000000578
18. Zhuang Y, Guo L, Huang W, Bo G, Zhang J, Zhu Z, et al. Altered resting-state hippocampal functional connectivity in breast cancer survivors with chemotherapy-induced amenorrhea. Brain Behav. (2023) 13:e3039. doi: 10.1002/brb3.3039
19. Streit WJ, Mrak RE, Griffin WS. Microglia and neuroinflammation: a pathological perspective. J Neuroinflammation. (2004) 1:14. doi: 10.1186/1742-2094-1-14
20. Jim HS, Donovan KA, Small BJ, Andrykowski MA, Munster PN, Jacobsen PB. Cognitive functioning in breast cancer survivors: a controlled comparison. Cancer. (2009) 115:1776–83. doi: 10.1002/cncr.24192
21. Shilling V, Jenkins V, Morris R, Deutsch G, Bloomfield D. The effects of adjuvant chemotherapy on cognition in women with breast cancer—preliminary results of an observational longitudinal study. Breast (Edinburgh). (2005) 14:142–50. doi: 10.1016/j.breast.2004.10.004
22. Ren X, Boriero D, Chaiswing L, Bondada S, St Clair DK, Butterfield DA. Plausible biochemical mechanisms of chemotherapy-induced cognitive impairment ("chemobrain"), a condition that significantly impairs the quality of life of many cancer survivors. Biochim Biophys Acta Mol Basis Dis. (2019) 1865:1088–97. doi: 10.1016/j.bbadis.2019.02.007
23. Santos JC, Pyter LM. Neuroimmunology of behavioral comorbidities associated with cancer and cancer treatments. Front Immunol. (2018) 9:1195. doi: 10.3389/fimmu.2018.01195
24. Lustberg MB, Kuderer NM, Desai A, Bergerot C, Lyman GH. Mitigating long-term and delayed adverse events associated with cancer treatment: implications for survivorship. Nat Rev Clin Oncol. (2023) 20(8):527–42. doi: 10.1038/s41571-023-00776-9
25. Argyriou AA, Assimakopoulos K, Iconomou G, Giannakopoulou F, Kalofonos HP. Either called "chemobrain" or "chemofog," the long-term chemotherapy-induced cognitive decline in cancer survivors is real. J Pain Symptom Manage. (2011) 41:126–39. doi: 10.1016/j.jpainsymman.2010.04.021
26. Matsos A, Johnston IN. Chemotherapy-induced cognitive impairments: A systematic review of the animal literature. Neurosci Biobehav Rev. (2019) 102:382–99. doi: 10.1016/j.neubiorev.2019.05.001
27. Deeken JF, Löscher W. The blood-brain barrier and cancer: transporters, treatment, and Trojan horses. Clin Cancer Res. (2007) 13:1663–74. doi: 10.1158/1078-0432.CCR-06-2854
28. Li X, Chen H, Lv Y, Chao HH, Gong L, Li CR, et al. Diminished gray matter density mediates chemotherapy dosage-related cognitive impairment in breast cancer patients. Sci Rep. (2018) 8:13801. doi: 10.1038/s41598-018-32257-w
29. Williams AM, Shah R, Shayne M, Huston AJ, Krebs M, Murray N, et al. Associations between inflammatory markers and cognitive function in breast cancer patients receiving chemotherapy. J Neuroimmunol. (2018) 314:17–23. doi: 10.1016/j.jneuroim.2017.10.005
30. Rodríguez Martín B, Fernández Rodríguez EJ, Rihuete Galve MI, Cruz Hernández JJ. Study of chemotherapy-induced cognitive impairment in women with breast cancer. Int J Environ Res Public Health. (2020) 17(23):8896. doi: 10.3390/ijerph17238896
31. Cheung YT, Ng T, Shwe M, Ho HK, Foo KM, Cham MT, et al. Association of proinflammatory cytokines and chemotherapy-associated cognitive impairment in breast cancer patients: a multi-centered, prospective, cohort study. Ann Oncol. (2015) 26:1446–51. doi: 10.1093/annonc/mdv206
32. Schagen SB, van Dam FS, Muller MJ, Boogerd W, Lindeboom J, Bruning PF. Cognitive deficits after postoperative adjuvant chemotherapy for breast carcinoma. Cancer. (1999) 85:640–50. doi: 10.1002/(ISSN)1097-0142
33. van Dam FS, Schagen SB, Muller MJ, Boogerd W, vd Wall E, Droogleever Fortuyn ME, et al. Impairment of cognitive function in women receiving adjuvant treatment for high-risk breast cancer: high-dose versus standard-dose chemotherapy. J Natl Cancer Inst. (1998) 90:210–8. doi: 10.1093/jnci/90.3.210
34. Wieneke MH, Dienst ER. Neuropsychological assessment of cognitive functioning following chemotherapy for breast cancer. Psycho-oncology. (1995) 4:61–6. doi: 10.1002/pon.2960040108
35. Fan HG, Houédé-Tchen N, Yi QL, Chemerynsky I, Downie FP, Sabate K, et al. Fatigue, menopausal symptoms, and cognitive function in women after adjuvant chemotherapy for breast cancer: 1- and 2-year follow-up of a prospective controlled study. J Clin Oncol. (2005) 23:8025–32. doi: 10.1200/JCO.2005.01.6550
36. Stewart A, Collins B, Mackenzie J, Tomiak E, Verma S, Bielajew C. The cognitive effects of adjuvant chemotherapy in early stage breast cancer: a prospective study. Psycho-oncology (Chichester England). (2008) 17:122–30. doi: 10.1002/pon.1210
37. Fosså SD, de Wit R, Roberts JT, Wilkinson PM, de Mulder PH, Mead GM, et al. Quality of life in good prognosis patients with metastatic germ cell cancer: a prospective study of the European Organization for Research and Treatment of Cancer Genitourinary Group/Medical Research Council Testicular Cancer Study Group (30941/TE20). J Clin Oncol. (2003) 21:1107–18. doi: 10.1200/JCO.2003.02.075
38. Wefel JS, Vidrine DJ, Marani SK, Swartz RJ, Veramonti TL, Meyers CA, et al. A prospective study of cognitive function in men with non-seminomatous germ cell tumors. Psychooncology. (2014) 23:626–33. doi: 10.1002/pon.3453
39. Amidi A, Hosseini SMH, Leemans A, Kesler SR, Agerbæk M, Wu LM, et al. Changes in brain structural networks and cognitive functions in testicular cancer patients receiving cisplatin-based chemotherapy. J Natl Cancer Inst. (2017) 109(12). doi: 10.1093/jnci/djx085
40. Skaali T, Fosså SD, Andersson S, Cvancarova M, Langberg CW, Lehne G, et al. Self-reported cognitive problems in testicular cancer patients: relation to neuropsychological performance, fatigue, and psychological distress. J Psychosom Res. (2011) 70:403–10. doi: 10.1016/j.jpsychores.2010.12.004
41. Trachtenberg E, Mashiach T, Ben Hayun R, Tadmor T, Fisher T, Aharon-Peretz J, et al. Cognitive impairment in hodgkin lymphoma survivors. Br J Haematol. (2018) 182:670–8. doi: 10.1111/bjh.15448
42. Fayette D, Juríčková V, Kozák T, Mociková H, Gaherová L, Fajnerová I, et al. Cognitive impairment associated with Hodgkin's lymphoma and chemotherapy. Neurosci Lett. (2023) 797:137082. doi: 10.1016/j.neulet.2023.137082
43. Zimmer P, Mierau A, Bloch W, Strüder HK, Hülsdünker T, Schenk A, et al. Post-chemotherapy cognitive impairment in patients with B-cell non-Hodgkin lymphoma: a first comprehensive approach to determine cognitive impairments after treatment with rituximab, cyclophosphamide, doxorubicin, vincristine and prednisone or rituximab and bendamustine. Leuk Lymphoma. (2015) 56:347–52. doi: 10.3109/10428194.2014.915546
44. Janelsins MC, Mohamed M, Peppone LJ, Magnuson A, Belcher EK, Melnik M, et al. Longitudinal changes in cognitive function in a nationwide cohort study of patients with lymphoma treated with chemotherapy. J Natl Cancer Inst. (2022) 114:47–59. doi: 10.1093/jnci/djab133
45. Noal S, Levy C, Hardouin A, Rieux C, Heutte N, Ségura C, et al. One-year longitudinal study of fatigue, cognitive functions, and quality of life after adjuvant radiotherapy for breast cancer. Int J Radiat Oncol Biol Phys. (2011) 81:795–803. doi: 10.1016/j.ijrobp.2010.06.037
46. Shibayama O, Yoshiuchi K, Inagaki M, Matsuoka Y, Yoshikawa E, Sugawara Y, et al. Association between adjuvant regional radiotherapy and cognitive function in breast cancer patients treated with conservation therapy. Cancer Med. (2014) 3:702–9. doi: 10.1002/cam4.174
47. Donovan KA, Small BJ, Andrykowski MA, Schmitt FA, Munster P, Jacobsen PB. Cognitive functioning after adjuvant chemotherapy and/or radiotherapy for early-stage breast carcinoma. Cancer. (2005) 104:2499–507. doi: 10.1002/cncr.21482
48. Kohli S, Griggs JJ, Roscoe JA, Jean-Pierre P, Bole C, Mustian KM, et al. Self-reported cognitive impairment in patients with cancer. J Oncol Pract. (2007) 3:54–9. doi: 10.1200/JOP.0722001
49. Thiery-Vuillemin A, Hvid Poulsen M, Lagneau E, Ploussard G, Birtle A, Dourthe LM, et al. Impact of abiraterone acetate plus prednisone or enzalutamide on fatigue and cognition in patients with metastatic castration-resistant prostate cancer: initial results from the observational AQUARiUS study. ESMO Open. (2018) 3:e000397. doi: 10.1136/esmoopen-2018-000397
50. Bender CM, Sereika SM, Berga SL, Vogel VG, Brufsky AM, Paraska KK, et al. Cognitive impairment associated with adjuvant therapy in breast cancer. Psycho-oncology (Chichester England). (2006) 15:422–30. doi: 10.1002/pon.964
51. Scheibel RS, Valentine AD, O'Brien S, Meyers CA. Cognitive dysfunction and depression during treatment with interferon-alpha and chemotherapy. J neuropsychiatry Clin neurosciences. (2004) 16:185–91. doi: 10.1176/jnp.16.2.185
52. Rogiers A, Leys C, De Cremer J, Awada G, Schembri A, Theuns P, et al. Health-related quality of life, emotional burden, and neurocognitive function in the first generation of metastatic melanoma survivors treated with pembrolizumab: a longitudinal pilot study. Supportive Care cancer. (2020) 28:3267–78. doi: 10.1007/s00520-019-05168-3
53. Rogiers A, Leys C, Lauwyck J, Schembri A, Awada G, Schwarze JK, et al. Neurocognitive function, psychosocial outcome, and health-related quality of life of the first-generation metastatic melanoma survivors treated with ipilimumab. J Immunol Res. (2020) 2020:2192480–11. doi: 10.1155/2020/2192480
54. Belin C, Devic P, Ayrignac X, Dos Santos A, Paix A, Sirven-Villaros L, et al. Description of neurotoxicity in a series of patients treated with CAR T-cell therapy. Sci Rep. (2020) 10:18997–93. doi: 10.1038/s41598-020-76055-9
55. Ruark J, Mullane E, Cleary N, Cordeiro A, Bezerra ED, Wu V, et al. Patient-reported neuropsychiatric outcomes of long-term survivors after chimeric antigen receptor T cell therapy. Biol Blood marrow transplantation. (2020) 26:34–43. doi: 10.1016/j.bbmt.2019.09.037
56. Hoogland AI, Barata A, Logue J, Kommalapati A, Hyland KA, Nelson AM, et al. Change in neurocognitive performance among patients with non-hodgkin lymphoma in the first year after chimeric antigen receptor T cell therapy. Transplant Cell Ther. (2022) 28:305.e1–.e9. doi: 10.1016/j.jtct.2022.03.023
57. Myers JS, Parks AC, Mahnken JD, Young KJ, Pathak HB, Puri RV, et al. First-line immunotherapy with check-point inhibitors: prospective assessment of cognitive function. Cancers. (2023) 15:1615. doi: 10.3390/cancers15051615
58. Friedman MA, Fernandez M, Wefel JS, Myszka KA, Champlin RE, Meyers CA. Course of cognitive decline in hematopoietic stem cell transplantation: a within-subjects design. Arch Clin Neuropsychol. (2009) 24:689–98. doi: 10.1093/arclin/acp060
59. Nakamura ZM, Heiling HM, Park EM, Deal AM, Quillen LJ, Chien SA, et al. Impact of patient and clinical characteristics on cognitive changes after allogeneic hematopoietic stem cell transplantation. Bone marrow Transplant (Basingstoke). (2021) 56:3100–3. doi: 10.1038/s41409-021-01481-w
60. Schulz-Kindermann F, Mehnert A, Scherwath A, Schirmer L, Schleimer B, Zander AR, et al. Cognitive function in the acute course of allogeneic hematopoietic stem cell transplantation for hematological Malignancies. Bone Marrow Transplant. (2007) 39:789–99. doi: 10.1038/sj.bmt.1705663
61. Jones D, Vichaya EG, Wang XS, Sailors MH, Cleeland CS, Wefel JS. Acute cognitive impairment in patients with multiple myeloma undergoing autologous hematopoietic stem cell transplant. Cancer. (2013) 119:4188–95. doi: 10.1002/cncr.28323
62. Scherwath A, Schirmer L, Kruse M, Ernst G, Eder M, Dinkel A, et al. Cognitive functioning in allogeneic hematopoietic stem cell transplantation recipients and its medical correlates: a prospective multicenter study. Psychooncology. (2013) 22:1509–16. doi: 10.1002/pon.3159
63. Harder H, Van Gool AR, Duivenvoorden HJ, Cornelissen JJ, Eijkenboom WMH, Barge RMY, et al. Case-referent comparison of cognitive functions in patients receiving haematopoietic stem-cell transplantation for haematological Malignancies: Two-year follow-up results. Eur J Cancer (1990). (2007) 43:2052–9. doi: 10.1016/j.ejca.2007.06.005
64. Kesler S, Janelsins M, Koovakkattu D, Palesh O, Mustian K, Morrow G, et al. Reduced hippocampal volume and verbal memory performance associated with interleukin-6 and tumor necrosis factor-alpha levels in chemotherapy-treated breast cancer survivors. Brain Behav Immun. (2013) 30 Suppl:S109–16. doi: 10.1016/j.bbi.2012.05.017
65. Koppelmans V, Breteler MM, Boogerd W, Seynaeve C, Gundy C, Schagen SB. Neuropsychological performance in survivors of breast cancer more than 20 years after adjuvant chemotherapy. J Clin Oncol. (2012) 30:1080–6. doi: 10.1200/JCO.2011.37.0189
66. Yamada TH, Denburg NL, Beglinger LJ, Schultz SK. Neuropsychological outcomes of older breast cancer survivors: cognitive features ten or more years after chemotherapy. J Neuropsychiatry Clin Neurosci. (2010) 22:48–54. doi: 10.1176/appi.neuropsych.22.1.48
67. van der Willik KD, Koppelmans V, Hauptmann M, Compter A, Ikram MA, Schagen SB. Inflammation markers and cognitive performance in breast cancer survivors 20 years after completion of chemotherapy: a cohort study. Breast Cancer Res. (2018) 20:135. doi: 10.1186/s13058-018-1062-3
68. Ahles TA, Saykin AJ, Furstenberg CT, Cole B, Mott LA, Skalla K, et al. Neuropsychologic impact of standard-dose systemic chemotherapy in long-term survivors of breast cancer and lymphoma. J Clin Oncol. (2002) 20:485–93. doi: 10.1200/JCO.2002.20.2.485
69. Schagen SB, Boogerd W, Muller MJ, Huinink WT, Moonen L, Meinhardt W, et al. Cognitive complaints and cognitive impairment following BEP chemotherapy in patients with testicular cancer. Acta Oncol. (2008) 47:63–70. doi: 10.1080/02841860701518058
70. Pedersen AD, Rossen P, Mehlsen MY, Pedersen CG, Zachariae R, Von Der Maase H. Long-term cognitive function following chemotherapy in patients with testicular cancer. J Int Neuropsychol Society. (2009) 15:296–301. doi: 10.1017/S1355617709090316
71. Chovanec M, Vasilkova L, Setteyova L, Obertova J, Palacka P, Rejlekova K, et al. Long-term cognitive functioning in testicular germ-cell tumor survivors. Oncologist. (2018) 23:617–23. doi: 10.1634/theoncologist.2017-0457
72. Skoogh J, Steineck G, Stierner U, Cavallin-Ståhl E, Wilderäng U, Wallin A, et al. Testicular-cancer survivors experience compromised language following chemotherapy: findings in a Swedish population-based study 3-26 years after treatment. Acta Oncol. (2012) 51:185–97. doi: 10.3109/0284186X.2011.602113
73. Stouten-Kemperman MM, de Ruiter MB, Caan MW, Boogerd W, Kerst MJ, Reneman L, et al. Lower cognitive performance and white matter changes in testicular cancer survivors 10 years after chemotherapy. Hum Brain Mapp. (2015) 36:4638–47. doi: 10.1002/hbm.22942
74. Amidi A, Wu LM, Pedersen AD, Mehlsen M, Pedersen CG, Rossen P, et al. Cognitive impairment in testicular cancer survivors 2 to 7 years after treatment. Support Care Cancer. (2015) 23:2973–9. doi: 10.1007/s00520-015-2663-3
75. Stelwagen J, Meuleman AT, Lubberts S, Steursma G, Kruyt LM, Donkerbroek JW, et al. Cognitive impairment in long-term survivors of testicular cancer more than 20 years after treatment. Cancers (Basel). (2021) 13(22):5675. doi: 10.3390/cancers13225675
76. Phillips KM, Jim HS, Small BJ, Laronga C, Andrykowski MA, Jacobsen PB. Cognitive functioning after cancer treatment: a 3-year longitudinal comparison of breast cancer survivors treated with chemotherapy or radiation and noncancer controls. Cancer. (2012) 118:1925–32. doi: 10.1002/cncr.26432
77. Castellon SA, Ganz PA, Bower JE, Petersen L, Abraham L, Greendale GA. Neurocognitive performance in breast cancer survivors exposed to adjuvant chemotherapy and tamoxifen. J Clin Exp Neuropsychol. (2004) 26:955–69. doi: 10.1080/13803390490510905
78. Sharafeldin N, Bosworth A, Patel SK, Chen Y, Morse E, Mather M, et al. Cognitive functioning after hematopoietic cell transplantation for hematologic Malignancy: results from a prospective longitudinal study. J Clin Oncol. (2018) 36:463–75. doi: 10.1200/JCO.2017.74.2270
79. Syrjala KL, Artherholt SB, Kurland BF, Langer SL, Roth-Roemer S, Elrod JB, et al. Prospective neurocognitive function over 5 years after allogeneic hematopoietic cell transplantation for cancer survivors compared with matched controls at 5 years. J Clin Oncol. (2011) 29:2397–404. doi: 10.1200/JCO.2010.33.9119
80. Yao S, Zhang Q, Yao X, Zhang X, Pang L, Yu S, et al. Advances of neuroimaging in chemotherapy related cognitive impairment (CRCI) of patients with breast cancer. Breast Cancer Res Treat. (2023) 201:15–26. doi: 10.1007/s10549-023-07005-y
81. Maurer GS, Clayton ZS. Anthracycline chemotherapy, vascular dysfunction and cognitive impairment: burgeoning topics and future directions. Future Cardiol. (2023) 19:547–66. doi: 10.2217/fca-2022-0086
82. Behranvand N, Nasri F, Zolfaghari Emameh R, Khani P, Hosseini A, Garssen J, et al. Chemotherapy: a double-edged sword in cancer treatment. Cancer immunology Immunother. (2022) 71:507. doi: 10.1007/s00262-021-03013-3
83. Hodgson KD, Hutchinson AD, Wilson CJ, Nettelbeck T. A meta-analysis of the effects of chemotherapy on cognition in patients with cancer. Cancer Treat Rev. (2013) 39:297–304. doi: 10.1016/j.ctrv.2012.11.001
84. Silverman DH, Dy CJ, Castellon SA, Lai J, Pio BS, Abraham L, et al. Altered frontocortical, cerebellar, and basal ganglia activity in adjuvant-treated breast cancer survivors 5-10 years after chemotherapy. Breast Cancer Res Treat. (2007) 103:303–11. doi: 10.1007/s10549-006-9380-z
85. de Ruiter MB, Reneman L, Boogerd W, Veltman DJ, Caan M, Douaud G, et al. Late effects of high-dose adjuvant chemotherapy on white and gray matter in breast cancer survivors: converging results from multimodal magnetic resonance imaging. Hum Brain Mapp. (2012) 33:2971–83. doi: 10.1002/hbm.21422
86. McDonald BC, Conroy SK, Ahles TA, West JD, Saykin AJ. Gray matter reduction associated with systemic chemotherapy for breast cancer: a prospective MRI study. Breast Cancer Res Treat. (2010) 123:819–28. doi: 10.1007/s10549-010-1088-4
87. Seigers R, Fardell JE. Neurobiological basis of chemotherapy-induced cognitive impairment: A review of rodent research. Neurosci Biobehav Rev. (2011) 35:729–41. doi: 10.1016/j.neubiorev.2010.09.006
88. McGale P, Taylor C, Correa C, Cutter D, Duane F, Ewertz M, et al. Effect of radiotherapy after mastectomy and axillary surgery on 10-year recurrence and 20-year breast cancer mortality: meta-analysis of individual patient data for 8135 women in 22 randomised trials. Lancet. (2014) 383:2127–35. doi: 10.1016/S0140-6736(14)60488-8
89. Perez BA, Koontz BF. Radiotherapy before and after radical prostatectomy for high-risk and locally advanced prostate cancer. Urol Oncol. (2015) 33:226–34. doi: 10.1016/j.urolonc.2014.09.018
90. Kiprian D, Czarkowska-Paczek B, Wyczalkowska-Tomasik A, Fuksiewicz M, Kotowicz B, Paczek L. Radiotherapy and radiochemotherapy increase serum levels of pro-inflammatory interleukin-6 and C-reactive protein in patients with head and neck cancers. Trans Cancer Res. (2018) 7:41–7. doi: 10.21037/tcr
91. Schaue D, Kachikwu EL, McBride WH. Cytokines in radiobiological responses: A review. Radiat Res. (2012) 178:505–23. doi: 10.1667/RR3031.1
92. Stanojković TP, Matić IZ, Petrović N, Stanković V, Kopčalić K, Besu I, et al. Evaluation of cytokine expression and circulating immune cell subsets as potential parameters of acute radiation toxicity in prostate cancer patients. Sci Rep. (2020) 10:19002. doi: 10.1038/s41598-020-75812-0
93. Chen C, Zeng J, Luo B, Li S. Chrysin attenuates oxidative stress to alleviate sevoflurane-induced cognitive impairments in aged rats. Psychiatry Invest. (2023) 20:430–8. doi: 10.30773/pi.2022.0287
94. Fleming B, Edison P, Kenny L. Cognitive impairment after cancer treatment: mechanisms, clinical characterization, and management. BMJ. (2023) 380:e071726. doi: 10.1136/bmj-2022-071726
95. Collins B, Mackenzie J, Stewart A, Bielajew C, Verma S. Cognitive effects of chemotherapy in post-menopausal breast cancer patients 1 year after treatment. Psychooncology. (2009) 18:134–43. doi: 10.1002/pon.1379
96. Paganini-Hill A, Clark LJ. Preliminary assessment of cognitive function in breast cancer patients treated with tamoxifen. Breast Cancer Res Treat. (2000) 64:165–76. doi: 10.1023/A:1006426132338
97. Harrison RA, Sharafeldin N, Rexer JL, Streck B, Petersen M, Henneghan AM, et al. Neurocognitive impairment after hematopoietic stem cell transplant for hematologic Malignancies: phenotype and mechanisms. oncologist (Dayton Ohio). (2021) 26:e2021–e33. doi: 10.1002/onco.13867
98. Duong SL, Barbiero FJ, Nowak RJ, Baehring JM. Neurotoxicities associated with immune checkpoint inhibitor therapy. J neuro-oncology. (2021) 152:265–77. doi: 10.1007/s11060-021-03695-w
99. Seigers R, Loos M, Van Tellingen O, Boogerd W, Smit AB, Schagen SB. Cognitive impact of cytotoxic agents in mice. Psychopharmacol (Berl). (2015) 232:17–37. doi: 10.1007/s00213-014-3636-9
100. Christie L-A, Acharya MM, Parihar VK, Nguyen A, Martirosian V, Limoli CL. Impaired cognitive function and hippocampal neurogenesis following cancer chemotherapy. Clin Cancer Res. (2012) 18:1954–65. doi: 10.1158/1078-0432.CCR-11-2000
101. Schrepf A, Lutgendorf SK, Pyter LM. Pre-treatment effects of peripheral tumors on brain and behavior: neuroinflammatory mechanisms in humans and rodents. Brain Behav Immun. (2015) 49:1–17. doi: 10.1016/j.bbi.2015.04.010
102. McGinnis GJ, Friedman D, Young KH, Torres ERS, Thomas JCR, Gough MJ, et al. Neuroinflammatory and cognitive consequences of combined radiation and immunotherapy in a novel preclinical model. Oncotarget. (2017) 8:9155–73. doi: 10.18632/oncotarget.v8i6
103. Buwalda B, Schagen SB. Is basic research providing answers if adjuvant anti-estrogen treatment of breast cancer can induce cognitive impairment? Life Sci (1973). (2013) 93:581–8. doi: 10.1016/j.lfs.2012.12.012
104. Shabani M, Larizadeh MH, Parsania S, Shekaari MA, Shahrokhi N. Profound destructive effects of adolescent exposure to vincristine accompanied with some sex differences in motor and memory performance. Can J Physiol Pharmacol. (2012) 90:379–86. doi: 10.1139/y11-132
105. Shabani M, Nazeri M, Parsania S, Razavinasab M, Zangiabadi N, Esmaeilpour K, et al. Walnut consumption protects rats against cisplatin-induced neurotoxicity. Neurotoxicology (Park For South). (2012) 33:1314–21. doi: 10.1016/j.neuro.2012.08.004
106. Winocur G, Wojtowicz JM, Tannock IF. Memory loss in chemotherapy-treated rats is exacerbated in high-interference conditions and related to suppression of hippocampal neurogenesis. Behav Brain Res. (2015) 281:239–44. doi: 10.1016/j.bbr.2014.12.028
107. Winocur G, Wojtowicz JM, Huang J, Tannock IF. Physical exercise prevents suppression of hippocampal neurogenesis and reduces cognitive impairment in chemotherapy-treated rats. Psychopharmacology. (2014) 231:2311–20. doi: 10.1007/s00213-013-3394-0
108. Winocur G, Vardy J, Binns MA, Kerr L, Tannock I. The effects of the anti-cancer drugs, methotrexate and 5-fluorouracil, on cognitive function in mice. Pharmacology Biochem behavior. (2006) 85:66–75. doi: 10.1016/j.pbb.2006.07.010
109. Inverardi F, Chikhladze M, Donzelli A, Moroni RF, Regondi MC, Pennacchio P, et al. Cytoarchitectural, behavioural and neurophysiological dysfunctions in the BCNU-treated rat model of cortical dysplasia. Eur J Neurosci. (2013) 37:150–62. doi: 10.1111/ejn.12032
110. Janelsins MC, Heckler CE, Thompson BD, Gross RA, Opanashuk LA, Cory-Slechta DA. A clinically relevant dose of cyclophosphamide chemotherapy impairs memory performance on the delayed spatial alternation task that is sustained over time as mice age. Neurotoxicology (Park For South). (2016) 56:287–93. doi: 10.1016/j.neuro.2016.06.013
111. Rendeiro C, Sheriff A, Bhattacharya TK, Gogola JV, Baxter JH, Chen H, et al. Long-lasting impairments in adult neurogenesis, spatial learning and memory from a standard chemotherapy regimen used to treat breast cancer. Behav Brain Res. (2016) 315:10–22. doi: 10.1016/j.bbr.2016.07.043
112. Lyons L, ElBeltagy M, Umka J, Markwick R, Startin C, Bennett G, et al. Fluoxetine reverses the memory impairment and reduction in proliferation and survival of hippocampal cells caused by methotrexate chemotherapy. Psychopharmacology. (2011) 215:105–15. doi: 10.1007/s00213-010-2122-2
113. Vijayanathan V, Gulinello M, Ali N, Cole PD. Persistent cognitive deficits, induced by intrathecal methotrexate, are associated with elevated CSF concentrations of excitotoxic glutamate analogs and can be reversed by an NMDA antagonist. Behav Brain Res. (2011) 225:491–7. doi: 10.1016/j.bbr.2011.08.006
114. Elbeltagy M, Mustafa S, Umka J, Lyons L, Salman A, Gloria Tu C-Y, et al. Fluoxetine improves the memory deficits caused by the chemotherapy agent 5-fluorouracil. Behav Brain Res. (2010) 208:112–7. doi: 10.1016/j.bbr.2009.11.017
115. Lyons L, Elbeltagy M, Bennett G, Wigmore P. Fluoxetine counteracts the cognitive and cellular effects of 5-Fluorouracil in the rat hippocampus by a mechanism of prevention rather than recovery. PloS One. (2012) 7:e30010. doi: 10.1371/journal.pone.0030010
116. Mustafa S, Walker A, Bennett G, Wigmore PM. 5-Fluorouracil chemotherapy affects spatial working memory and newborn neurons in the adult rat hippocampus. Eur J Neurosci. (2008) 28:323–30. doi: 10.1111/j.1460-9568.2008.06325.x
117. Acharya MM, Martirosian V, Chmielewski NN, Hanna N, Tran KK, Liao AC, et al. Stem cell transplantation reverses chemotherapy-induced cognitive dysfunction. Cancer Res (Chicago Ill). (2015) 75:676–86. doi: 10.1158/0008-5472.CAN-14-2237
118. Callaghan CK, O'Mara SM. Long-term cognitive dysfunction in the rat following docetaxel treatment is ameliorated by the phosphodiesterase-4 inhibitor, rolipram. Behav Brain Res. (2015) 290:84–9. doi: 10.1016/j.bbr.2015.04.044
119. Fardell JE, Vardy J, Monds LA, Johnston IN. The long-term impact of oxaliplatin chemotherapy on rodent cognition and peripheral neuropathy. Behav Brain Res. (2015) 291:80–8. doi: 10.1016/j.bbr.2015.04.038
120. Fardell JE, Vardy J, Johnston IN. The short and long term effects of docetaxel chemotherapy on rodent object recognition and spatial reference memory. Life Sci (1973). (2013) 93:596–604. doi: 10.1016/j.lfs.2013.05.006
121. Lomeli N, Di K, Czerniawski J, Guzowski JF, Bota DA. Cisplatin-induced mitochondrial dysfunction is associated with impaired cognitive function in rats. Free Radical Biol Med. (2017) 102:274–86. doi: 10.1016/j.freeradbiomed.2016.11.046
122. Mondie CM, Vandergrift KA, Wilson CL, Gulinello ME, Weber ET. The chemotherapy agent, thioTEPA, yields long-term impairment of hippocampal cell proliferation and memory deficits but not depression-related behaviors in mice. Behav Brain Res. (2010) 209:66–72. doi: 10.1016/j.bbr.2010.01.016
123. Kitamura Y, Hattori S, Yoneda S, Watanabe S, Kanemoto E, Sugimoto M, et al. Doxorubicin and cyclophosphamide treatment produces anxiety-like behavior and spatial cognition impairment in rats: Possible involvement of hippocampal neurogenesis via brain-derived neurotrophic factor and cyclin D1 regulation. Behav Brain Res. (2015) 292:184–93. doi: 10.1016/j.bbr.2015.06.007
124. Kitamura Y, Kanemoto E, Sugimoto M, Machida A, Nakamura Y, Naito N, et al. Influence of nicotine on doxorubicin and cyclophosphamide combination treatment-induced spatial cognitive impairment and anxiety-like behavior in rats. Naunyn-Schmiedeberg's Arch Pharmacol. (2017) 390:369–78. doi: 10.1007/s00210-016-1338-z
125. Salas-Ramirez KY, Bagnall C, Frias L, Abdali SA, Ahles TA, Hubbard K. Doxorubicin and cyclophosphamide induce cognitive dysfunction and activate the ERK and AKT signaling pathways. Behav Brain Res. (2015) 292:133–41. doi: 10.1016/j.bbr.2015.06.028
126. Iarkov A, Appunn D, Echeverria V. Post-treatment with cotinine improved memory and decreased depressive-like behavior after chemotherapy in rats. Cancer chemotherapy Pharmacol. (2016) 78:1033–9. doi: 10.1007/s00280-016-3161-0
127. Fardell JE, Vardy J, Shah JD, Johnston IN. Cognitive impairments caused by oxaliplatin and 5-fluorouracil chemotherapy are ameliorated by physical activity. Psychopharmacology. (2012) 220:183–93. doi: 10.1007/s00213-011-2466-2
128. Winocur G, Henkelman M, Wojtowicz JM, Zhang H, Binns MA, Tannock IF. The effects of chemotherapy on cognitive function in a mouse model: A prospective study. Clin Cancer Res. (2012) 18:3112–21. doi: 10.1158/1078-0432.CCR-12-0060
129. Nokia MS, Anderson ML, Shors TJ. Chemotherapy disrupts learning, neurogenesis and theta activity in the adult brain. Eur J Neurosci. (2012) 36:3521–30. doi: 10.1111/ejn.12007
130. Madhyastha S, Somayaji SN, Rao MS, Nalini K, Bairy KL. Hippocampal brain amines in methotrexate-induced learning and memory deficit. Can J Physiol Pharmacol. (2002) 80:1076–84. doi: 10.1139/y02-135
131. Song T-Y, Chen C-L, Liao J-W, Ou H-C, Tsai M-S. Ergothioneine protects against neuronal injury induced by cisplatin both in vitro and in vivo. Food Chem Toxicol. (2010) 48:3492–9. doi: 10.1016/j.fct.2010.09.030
132. Seigers R, Schagen SB, Coppens CM, van der Most PJ, van Dam FS, Koolhaas JM, et al. Methotrexate decreases hippocampal cell proliferation and induces memory deficits in rats. Behav Brain Res. (2009) 201:279–84. doi: 10.1016/j.bbr.2009.02.025
133. Yang M, Kim J-S, Kim J, Jang S, Kim S-H, Kim J-C, et al. Acute treatment with methotrexate induces hippocampal dysfunction in a mouse model of breast cancer. Brain Res bulletin. (2012) 89:50–6. doi: 10.1016/j.brainresbull.2012.07.003
134. Bianchi E, Di Cesare Mannelli L, Micheli L, Farzad M, Aglianò M, Ghelardini C. Apoptotic process induced by oxaliplatin in rat hippocampus causes memory impairment. Basic Clin Pharmacol toxicology. (2017) 120:14–21. doi: 10.1111/bcpt.12629
135. Hou J-g, Xue J-j, Lee M-r, Sun M-q, Zhao X-h, Zheng Y-n, et al. Compound K is able to ameliorate the impaired cognitive function and hippocampal neurogenesis following chemotherapy treatment. Biochem Biophys Res Commun. (2013) 436:104–9. doi: 10.1016/j.bbrc.2013.05.087
136. Lim I, Joung H-Y, Yu AR, Shim I, Kim JS. PET evidence of the effect of donepezil on cognitive performance in an animal model of chemobrain. BioMed Res Int. (2016) 2016:6945415–7. doi: 10.1155/2016/6945415
137. Konat GW, Kraszpulski M, James I, Zhang H-T, Abraham J. Cognitive dysfunction induced by chronic administration of common cancer chemotherapeutics in rats. Metab Brain disease. (2008) 23:325–33. doi: 10.1007/s11011-008-9100-y
138. Helal GK, Aleisa AM, Helal OK, Al-Rejaie SS, Al-Yahya AA, Al-Majed AA, et al. Metallothionein induction reduces caspase-3 activity and TNFalpha levels with preservation of cognitive function and intact hippocampal neurons in carmustine-treated rats. Oxid Med Cell Longev. (2009) 2:26–35. doi: 10.4161/oxim.2.1.7901
139. Bisen-Hersh EB, Hineline PN, Walker EA. Effects of early chemotherapeutic treatment on learning in adolescent mice: implications for cognitive impairment and remediation in childhood cancer survivors. Clin Cancer Res. (2013) 19:3008–18. doi: 10.1158/1078-0432.CCR-12-3764
140. Foley JJ, Raffa RB, Walker EA. Effects of chemotherapeutic agents 5-fluorouracil and methotrexate alone and combined in a mouse model of learning and memory. Psychopharmacologia. (2008) 199:527–38. doi: 10.1007/s00213-008-1175-y
141. Walker EA, Foley JJ, Clark-Vetri R, Raffa RB. Effects of repeated administration of chemotherapeutic agents tamoxifen, methotrexate, and 5-fluorouracil on the acquisition and retention of a learned response in mice. Psychopharmacologia. (2011) 217:539–48. doi: 10.1007/s00213-011-2310-8
142. Krynetskiy E, Krynetskaia N, Rihawi D, Wieczerzak K, Ciummo V, Walker E. Establishing a model for assessing DNA damage in murine brain cells as a molecular marker of chemotherapy-associated cognitive impairment. Life Sci (1973). (2013) 93:605–10. doi: 10.1016/j.lfs.2013.03.013
143. Fardell JE, Vardy J, Logge W, Johnston I. Single high dose treatment with methotrexate causes long-lasting cognitive dysfunction in laboratory rodents. Pharmacology Biochem behavior. (2010) 97:333–9. doi: 10.1016/j.pbb.2010.08.019
144. Kaplan SV, Limbocker RA, Gehringer RC, Divis JL, Osterhaus GL, Newby MD, et al. Impaired brain dopamine and serotonin release and uptake in wistar rats following treatment with carboplatin. ACS Chem Neurosci. (2016) 7:689–99. doi: 10.1021/acschemneuro.5b00029
145. Dubois M, Lapinte N, Villier V, Lecointre C, Roy V, Tonon MC, et al. Chemotherapy-induced long-term alteration of executive functions and hippocampal cell proliferation: Role of glucose as adjuvant. Neuropharmacology. (2014) 79:234–48. doi: 10.1016/j.neuropharm.2013.11.012
146. Smith AE, Slivicki RA, Hohmann AG, Crystal JD. The chemotherapeutic agent paclitaxel selectively impairs learning while sparing source memory and spatial memory. Behav Brain Res. (2017) 320:48–57. doi: 10.1016/j.bbr.2016.11.042
147. Mu L, Wang J, Cao B, Jelfs B, Chan RHM, Xu X, et al. Impairment of cognitive function by chemotherapy: association with the disruption of phase-locking and synchronization in anterior cingulate cortex. Mol brain. (2015) 8:32–. doi: 10.1186/s13041-015-0125-y
148. Chiu GS, Maj MA, Rizvi S, Dantzer R, Vichaya EG, Laumet G, et al. Pifithrin-μ Prevents cisplatin-induced chemobrain by preserving neuronal mitochondrial function. Cancer Res (Chicago Ill). (2017) 77:742–52. doi: 10.1158/0008-5472.CAN-16-1817
149. Chen D, Wu CF, Shi B, Xu YM. Tamoxifen and toremifene cause impairment of learning and memory function in mice. Pharmacology Biochem behavior. (2002) 71:269–76. doi: 10.1016/S0091-3057(01)00656-6
150. Chen D, Wu C-F, Shi B, Xu Y-M. Tamoxifen and toremifene impair retrieval, but not acquisition, of spatial information processing in mice. Pharmacology Biochem behavior. (2002) 72:417–21. doi: 10.1016/S0091-3057(01)00782-1
151. Esmaeili B, Basseda Z, Gholizadeh S, Javadi Paydar M, Dehpour AR. Tamoxifen disrupts consolidation and retrieval of morphine-associated contextual memory in male mice: interaction with estradiol. Psychopharmacologia. (2009) 204:191–201. doi: 10.1007/s00213-008-1448-5
152. Shabani M, Larizadeh MH, Parsania S, Hajali V, Shojaei A. Evaluation of destructive effects of exposure to cisplatin during developmental stage: no profound evidence for sex differences in impaired motor and memory performance. Int J Neurosci. (2012) 122:439–48. doi: 10.3109/00207454.2012.673515
153. Palmer TD, Monje ML, Mizumatsu S, Fike JR. Irradiation induces neural precursor-cell dysfunction. Nat Med. (2002) 8:955–62. doi: 10.1038/nm749
154. ELBeltagy M, Mustafa S, Umka J, Lyons L, Salman A, Dormon K, et al. The effect of 5-fluorouracil on the long term survival and proliferation of cells in the rat hippocampus. Brain Res Bull. (2012) 88:514–8. doi: 10.1016/j.brainresbull.2012.05.005
155. Dietrich J, Han R, Yang Y, Mayer-Pröschel M, Noble M. CNS progenitor cells and oligodendrocytes are targets of chemotherapeutic agents in vitro and in vivo. J Biol (London England). (2006) 5:22–. doi: 10.1186/jbiol50
156. Mignone RG, Weber ET. Potent inhibition of cell proliferation in the hippocampal dentate gyrus of mice by the chemotherapeutic drug thioTEPA. Brain Res. (2006) 1111:26–9. doi: 10.1016/j.brainres.2006.06.093
157. Pereira Dias G, Hollywood R, Bevilaqua MC, da Luz AC, Hindges R, Nardi AE, et al. Consequences of cancer treatments on adult hippocampal neurogenesis: implications for cognitive function and depressive symptoms. Neuro Oncol. (2014) 16:476–92. doi: 10.1093/neuonc/not321
158. Chu C, Davis CM, Lan X, Hienz RD, Jablonska A, Thomas AM, et al. Neuroinflammation after stereotactic radiosurgery-induced brain tumor disintegration is linked to persistent cognitive decline in a mouse model of metastatic disease. Int J Radiat oncology biology physics. (2020) 108:745–57. doi: 10.1016/j.ijrobp.2020.05.027
159. Seigers R, Timmermans J, van der Horn HJ, de Vries EF, Dierckx RA, Visser L, et al. Methotrexate reduces hippocampal blood vessel density and activates microglia in rats but does not elevate central cytokine release. Behav Brain Res. (2010) 207:265–72. doi: 10.1016/j.bbr.2009.10.009
160. Husain K, Whitworth C, Hazelrigg S, Rybak L. Carboplatin-induced oxidative injury in rat inferior colliculus. Int J toxicology. (2003) 22:335–42. doi: 10.1080/713936678
161. Oboh G, Ogunruku OO. Cyclophosphamide-induced oxidative stress in brain: protective effect of hot short pepper (Capsicum frutescens L. var. abbreviatum). Exp Toxicol Pathol. (2010) 62:227–33. doi: 10.1016/j.etp.2009.03.011
162. Geller HM, Cheng KY, Goldsmith NK, Romero AA, Zhang AL, Morris EJ, et al. Oxidative stress mediates neuronal DNA damage and apoptosis in response to cytosine arabinoside. J Neurochem. (2001) 78:265–75. doi: 10.1046/j.1471-4159.2001.00395.x
163. Joshi G, Sultana R, Tangpong J, Cole MP, St Clair DK, Vore M, et al. Free radical mediated oxidative stress and toxic side effects in brain induced by the anti cancer drug adriamycin: Insight into chemobrain. Free Radical Res. (2005) 39:1147–54. doi: 10.1080/10715760500143478
164. Montilla P, Túnez I, Muñoz MC, Soria JV, López A. Antioxidative effect of melatonin in rat brain oxidative stress induced by Adriamycin. Rev Esp Fisiol. (1997) 53:301–5.
165. Rajamani R, Muthuvel A, Senthilvelan M, Sheeladevi R. Oxidative stress induced by methotrexate alone and in the presence of methanol in discrete regions of the rodent brain, retina and optic nerve. Toxicol letters. (2006) 165:265–73. doi: 10.1016/j.toxlet.2006.05.005
166. Oz E, Ilhan MN. Effects of melatonin in reducing the toxic effects of doxorubicin. Mol Cell Biochem. (2006) 286:11–5. doi: 10.1007/s11010-005-9003-8
167. Uzar E, Koyuncuoglu HR, Uz E, Yilmaz HR, Kutluhan S, Kilbas S, et al. The activities of antioxidant enzymes and the level of malondialdehyde in cerebellum of rats subjected to methotrexate: protective effect of caffeic acid phenethyl ester. Mol Cell Biochem. (2006) 291:63–8. doi: 10.1007/s11010-006-9196-5
168. Oz M, Nurullahoglu Atalik KE, Yerlikaya FH, Demir EA. Curcumin alleviates cisplatin-induced learning and memory impairments. Neurobiol Learn memory. (2015) 123:43–9. doi: 10.1016/j.nlm.2015.05.001
169. Barry RL, Byun NE, Tantawy MN, Mackey CA, Wilson GH, Stark AJ, et al. In vivo neuroimaging and behavioral correlates in a rat model of chemotherapy-induced cognitive dysfunction. Brain Imaging behavior. (2018) 12:87–95. doi: 10.1007/s11682-017-9674-2
170. Jarmolowicz DP, Gehringer R, Lemley SM, Sofis MJ, Kaplan S, Johnson MA. 5-Fluorouracil impairs attention and dopamine release in rats. Behav Brain Res. (2019) 362:319–22. doi: 10.1016/j.bbr.2019.01.007
171. Thomas TC, Beitchman JA, Pomerleau F, Noel T, Jungsuwadee P, Butterfield DA, et al. Acute treatment with doxorubicin affects glutamate neurotransmission in the mouse frontal cortex and hippocampus. Brain Res. (2017) 1672:10–7. doi: 10.1016/j.brainres.2017.07.003
172. Zhou W, Kavelaars A, Heijnen CJ. Metformin prevents cisplatin-induced cognitive impairment and brain damage in mice. PloS One. (2016) 11:e0151890–e. doi: 10.1371/journal.pone.0151890
173. Liddelow SA, Guttenplan KA, Clarke LE, Bennett FC, Bohlen CJ, Schirmer L, et al. Neurotoxic reactive astrocytes are induced by activated microglia. Nature. (2017) 541:481–7. doi: 10.1038/nature21029
Keywords: cancer related cognitive impairment, CRCI, cancer treatment, chemobrain, neurobehavior
Citation: Demos-Davies K, Lawrence J and Seelig D (2024) Cancer related cognitive impairment: a downside of cancer treatment. Front. Oncol. 14:1387251. doi: 10.3389/fonc.2024.1387251
Received: 17 February 2024; Accepted: 09 April 2024;
Published: 23 April 2024.
Edited by:
David A. Gewirtz, Virginia Commonwealth University, United StatesReviewed by:
Albrecht Reichle, University Medical Center Regensburg, GermanyCopyright © 2024 Demos-Davies, Lawrence and Seelig. This is an open-access article distributed under the terms of the Creative Commons Attribution License (CC BY). The use, distribution or reproduction in other forums is permitted, provided the original author(s) and the copyright owner(s) are credited and that the original publication in this journal is cited, in accordance with accepted academic practice. No use, distribution or reproduction is permitted which does not comply with these terms.
*Correspondence: Kimberly Demos-Davies, ZGVtb3MwMjdAdW1uLmVkdQ==
†Present address: Jessica Lawrence, Department of Surgical & Radiological Sciences, University of California, Davis, Davis, CA, United States
Disclaimer: All claims expressed in this article are solely those of the authors and do not necessarily represent those of their affiliated organizations, or those of the publisher, the editors and the reviewers. Any product that may be evaluated in this article or claim that may be made by its manufacturer is not guaranteed or endorsed by the publisher.
Research integrity at Frontiers
Learn more about the work of our research integrity team to safeguard the quality of each article we publish.