- 1Department of Urology, First Hospital of Shanxi Medical University, Taiyuan, China
- 2The First Clinical Medical College of Shanxi Medical University, Taiyuan, China
- 3Public Experiment Center, University of Shanghai for Science and Technology, Shanghai, China
- 4The Second Clinical Medical College of Shanxi Medical University, Taiyuan, China
Objective: This study aimed to investigate the feasibility and effectiveness of using indocyanine green (ICG) injected intracutaneously through the lower limbs and perineum for visualized tracking, localization, and qualitative assessment of pelvic lymph nodes (LNs) in bladder cancer to achieve their accurate resection.
Methods: First, ICG was injected into the LN metastasis model mice lower limbs, and real-time and dynamic in vivo and ex vivo imaging was conducted by using a near-infrared fluorescence imaging system. Additionally, 26 patients with bladder cancer were enrolled and divided into intracutaneous group and transurethral group. A near-infrared fluorescence imaging device with internal and external imaging probes was used to perform real-time tracking, localization, and resection of the pelvic LNs.
Results: The mice normal LNs and the metastatic LNs exhibited fluorescence. The metastatic LNs showed a significantly higher signal-to-background ratio than the normal LNs (3.9 ± 0.2 vs. 2.0 ± 0.1, p < 0.05). In the intracutaneous group, the accuracy rate of fluorescent-labeled LNs was 97.6%, with an average of 11.3 ± 2.4 LNs resected per patient. Six positive LNs were detected in three patients (18.8%). In the transurethral group, the accuracy rate of fluorescent-labeled LNs was 84.4%, with an average of 8.6 ± 2.3 LNs resected per patient. Two positive LNs were detected in one patient (12.5%).
Conclusion: Following the intracutaneous injection of ICG into the lower limbs and perineum, the dye accumulates in pelvic LNs through lymphatic reflux. By using near-infrared fluorescence laparoscopic fusion imaging, physicians can perform real-time tracking, localization, and precise resection of pelvic LNs.
1 Introduction
Bladder cancer is one of the top 10 most commonly occurring cancers worldwide. In 2020, there were approximately 573,000 new cases globally, with over three-quarters of the cases occurring in males (1). The American Cancer Society estimates that in 2023, there will be over 82,000 new cases and over 16,000 deaths from bladder cancer in the United States; these numbers indicate a significant burden on human health (2). Radical cystectomy (RC) is an important surgical approach for treating muscle-invasive bladder cancer (MIBC). However, the prognosis of patients undergoing RC alone is poor, with a 5-year survival rate of only 42% (3). This is primarily due to the high propensity of MIBC to metastasize to the lymph node (LN). Approximately 25% of MIBC patients already have LN metastasis at the time of surgical treatment (4), and patients with metastasis have a relatively poor prognosis (5). Therefore, RC combined with pelvic LN dissection (PLND) is considered the gold standard for treating MIBC.
PLND is a surgical procedure that involves the removal of lymphatic tissue surrounding the blood vessels within a specific range in the pelvic region. It has diagnostic and therapeutic significance in surgical procedures for bladder cancer (6), prostate cancer (7), and colorectal cancer (8). RC combined with PLND can improve patient survival outcomes, even in patients with negative LNs. This is because PLND allows for more accurate LN staging and may remove micrometastases, thereby reducing tumor burden (9, 10).
In the past few decades, the concept and diagnostic approach of sentinel lymph node biopsy (SLNB) have gained significant attention in bladder cancer. Morton (11) first introduced the concept of sentinel lymph node (SLN) in 2004 to describe metastatic LN. The SLN is the first regional LN that directly receives lymphatic drainage from the primary tumor site. In theory, by detecting the SLN, it is possible to determine whether metastasis has occurred in all LNs draining from the cancer site, thus enabling to determine the extent of LN dissection.
In recent years, the use of near-infrared (NIR) fluorescence imaging-guided SLN detection has become a real-time and visually accessible technique. Indocyanine green (ICG) is an FDA-approved NIR fluorescent contrast agent that has been used in the medical field for over 60 years. Compared to the visible light range (400–700 nm), both the excitation light (780 nm) and emission light (820 nm) of ICG are within the NIR range (700–900nm), thereby reducing tissue scattering and autofluorescence interference and increasing imaging depth. Therefore, ICG has potential advantages for use in imaging (12). ICG-guided SLN detection has been successfully applied in clinical practice for the diagnosis of breast cancer (13), penile cancer (14), and melanoma (15). However, the complexity of bilateral lymphatic drainage in the bladder leads to a high false-negative rate and low detection rate, which limits the application of ICG in bladder cancer SLNB (16, 17).
In a preliminary study, Rietbergen et al. (18) demonstrated the feasibility of the hybrid tracer ICG-99mTc-nanocolloid for SLNB in clinical node-negative MIBC patients scheduled for RC with extended PLND (ePLND). However, its study was limited by sample size and lack of outcome data. In another study (19), ICG fluorescence-guided dissection of sentinel regions was able to visualize the lymphatic drainage pathways of the tumors in all RC patients.
The main routes of ICG injection include intravenous injection, intradermal injection, submucosal injection, and intracavitary injection (20). Currently, the reported injection sites for ICG tracing of pelvic LNs in bladder cancer include submucosal or detrusor muscle around the tumors. However, factors such as complex operation techniques and unstable imaging effects have led to a low success rate of visualization (21).
Thompson et al. (22) first proposed the concept of indirect lymphangiography in 1966, which is safer and simpler than direct lymphangiography. The principle of this method involves intracutaneous or subcutaneous injection of the contrast agent, which then reaches the lymphatic vessels through tissue spaces and is subsequently drained into the LNs, thereby allowing for local visualization of the LNs and lymphatic vessels by X-ray imaging. Shinaoka et al. (23) established a new lymphatic imaging protocol by performing detailed anatomical analysis of the LNs and lymphatic vessels through subcutaneous injection of ICG into the lower limbs. Therefore, based on the successful development of the Saians® 4K fluorescence imaging medical device, our research group combined indirect lymphangiography and NIR fluorescence imaging technology to design a novel approach by using intracutaneous injection of ICG through the lower limbs and perineum for labeling pelvic LNs in bladder cancer. This approach aims to provide real-time visualization guidance for accurate resection during surgery. We also performed intravesical injection of ICG around the bladder tumor through the urethra to differentiate the two injection methods on the basis of localization effects on pelvic LNs and positive LNs. This further validates the effectiveness and feasibility of the intracutaneous injection of ICG through the lower limbs and perineum.
2 Materials and methods
2.1 Animal model
2.1.1 Cell line
The present study used the green fluorescent protein (GFP)-labeled 5637 bladder cancer cell line (5637-GFP). The cell line was cultured in RPMI-1640 medium supplemented with 10% fetal bovine serum and 1% antibiotics. The cells were incubated at 37°C in a humidified atmosphere containing 5% CO2.
2.1.2 Establishment of animal models and NIR fluorescence imaging
All experimental procedures, including animal handling and care, were conducted in accordance with the ethical guidelines of the Ethics Committee for Animal Welfare of Shanxi Medical University. Twelve female BALB/C-nu mice (age: 4–6 weeks, weight: 12–16 g, obtained from Beijing Huafukang Bio-technology Co.,China) were randomly assigned to a control group (n = 6) and a footpad tumor LN metastasis experimental group (n = 6). In the experimental group, 5637-GFP cells (4 × 106) were injected into the right footpad of mice. After 4 weeks, a footpad tumor metastasis model was established. The control group received no treatment or intervention.
ICG (25 mg, Dandong Medical Creation Pharmaceutical Co., China) was prepared as a 0.25 mg/mL solution. Forty microliters of the solution was intracutaneously injected into the right footpad of both experimental and control group mice. Real-time dynamic fluorescence imaging was performed to observe the distribution of ICG in mice. After 6 h, the fluorescent-labeled tissues were excised under fluorescence guidance. The fluorescence intensity of the labeled tissues and the surrounding tissues was analyzed using ImageJ software, and the signal-to-background ratio (SBR) was calculated. The tissues were then prepared as frozen sections. Under a fluorescence microscope, the GFP signal was detected at excitation and emission wavelengths of 480 and 520 nm, respectively, while the ICG signal was detected at excitation and emission wavelengths of 780 and 820 nm, respectively. Finally, tissue pathological analysis was performed by hematoxylin-eosin staining.
2.2 Patients and methods
2.2.1 Study population
This study was approved by the Ethics Committee of the First Hospital of Shanxi Medical University and registered with the Chinese Clinical Trial Registry. From June 2022 to December 2022, based on imaging examinations and cystoscopy, and after excluding contraindications, we included a total of 26 patients who were diagnosed with MIBC before surgery and planned to undergo RC+PLND. On another hand, the exclusion criteria are also considered that including the patients with a history of pelvic surgery or the tumors outside the bladder. The patients were divided into the lower limbs and perineum intracutaneous injection group (intracutaneous group) and transurethral intravesical injection group (transurethral group) according to the randomization principle. Finally, 16 patients were included in the intracutaneous group and 10 patients were included in the transurethral group.
2.2.2 Imaging system equipment
The Saians® 4K fluorescence imaging system (Saians Technology Development Co., Ltd., Taiyuan, Shanxi, China) is equipped with an ex vivo imaging lens and a laparoscopic lens for in vivo imaging. Both lenses offer visible light, fluorescence, and fusion pseudo-color modes. The visible light mode provides clear anatomical images, the fluorescence mode displays white fluorescence images, and the fusion mode simultaneously displays both anatomical and fluorescence images in a pixel-level fused green pseudo-color image within the same surgical field. These three modes do not require switching during the operation, which enables to achieve real-time intraoperative visualization. The imaging schematic is shown in Figure 1.
2.2.3 Methods of ICG injection
ICG was diluted to 2.5 mg/ml concentration by using sterile injectable water. For the intracutaneous group, at 1.5 h before the surgery, 0.5 ml ICG was injected intracutaneously at a point 2 cm above the medial malleolus on each side and on both sides of the midline of the perineum; the total volume was 2 ml. Immediately after the injection, each injection site was gently massaged. The ex vivo imaging lens of the imaging system was used to observe the fluorescence image of ICG traveling upward along the injection sites, and the time to reach the inguinal LNs was recorded. For the transurethral group, at 1.5 h before the surgery, 2 ml of ICG was injected into the submucosa around the bladder tumor by using a bladder injection needle, and the bladder was then filled with normal saline for 30 min to provide moderate bladder distension, thereby promoting lymphatic drainage.
2.2.4 Methods of fluorescence-guided PLND
The surgeries in both groups were performed by the same team. The patients were induced with general anesthesia and placed in the supine position with their head down and their feet elevated. A fluorescence laparoscope was inserted using the conventional laparoscopic approach. The procedure consisted of two steps: fluorescence-guided PLND (F-PLND) followed by RC. During F-PLND, a fluorescence-guided localization and excision technique was used. First, the position of the fluorescence image was observed before the peritoneum was incised. The peritoneum was then opened along the external iliac vessels, thereby exposing the external iliac arteries and veins, internal iliac arteries and veins, common iliac vessels, and obturator nerve. The tissue marked with fluorescence was then separated along the edge observed in the fluorescence image, excised, and subsequently numbered and sent for histopathological examination.
2.3 Statistical analysis
All graphs, calculations, and data analysis were performed using GraphPad Prism software. For quantitative data conforming to a normal distribution, we represented them using mean ± standard deviation and two independent-sample t-test was used to analyze the difference in SBR and the difference in the number of LN detected between the two groups. For qualitative data, we represented them using probabilities and percentages, and chi-square test (χ2-test) and Fisher probability method was used to analyze the difference in LN accuracy rate and the difference in LN positive detection rate between the two groups. P-values were considered statistically significant at P < 0.05.
3 Results
3.1 Fluorescence imaging characteristics of ICG injected in the lower limbs in normal and LN metastasis mice
The LN metastasis mouse model was successfully established by injecting tumor cells through the footpad, as confirmed by the GFP signal in the tissues and pathological examination (Figure 2). After 6h of ICG injection in the lower limbs, fluorescence imaging was observed in both the control group with normal LNs and the experimental group with metastatic LNs. However, the experimental group showed significantly higher SBR than the control group (3.9 ± 0.2 vs. 2.0 ± 0.1). The t-test was used to analyze the difference in SBR between the two groups of mice, and the results were statistically significant (p<0.05). Microscopic imaging revealed that the fluorescence imaging of ICG in the metastatic LNs overlapped partially with the GFP-labeled tumor cells, thus indicating a higher accumulation of ICG in the LNs with tumor cell metastasis.
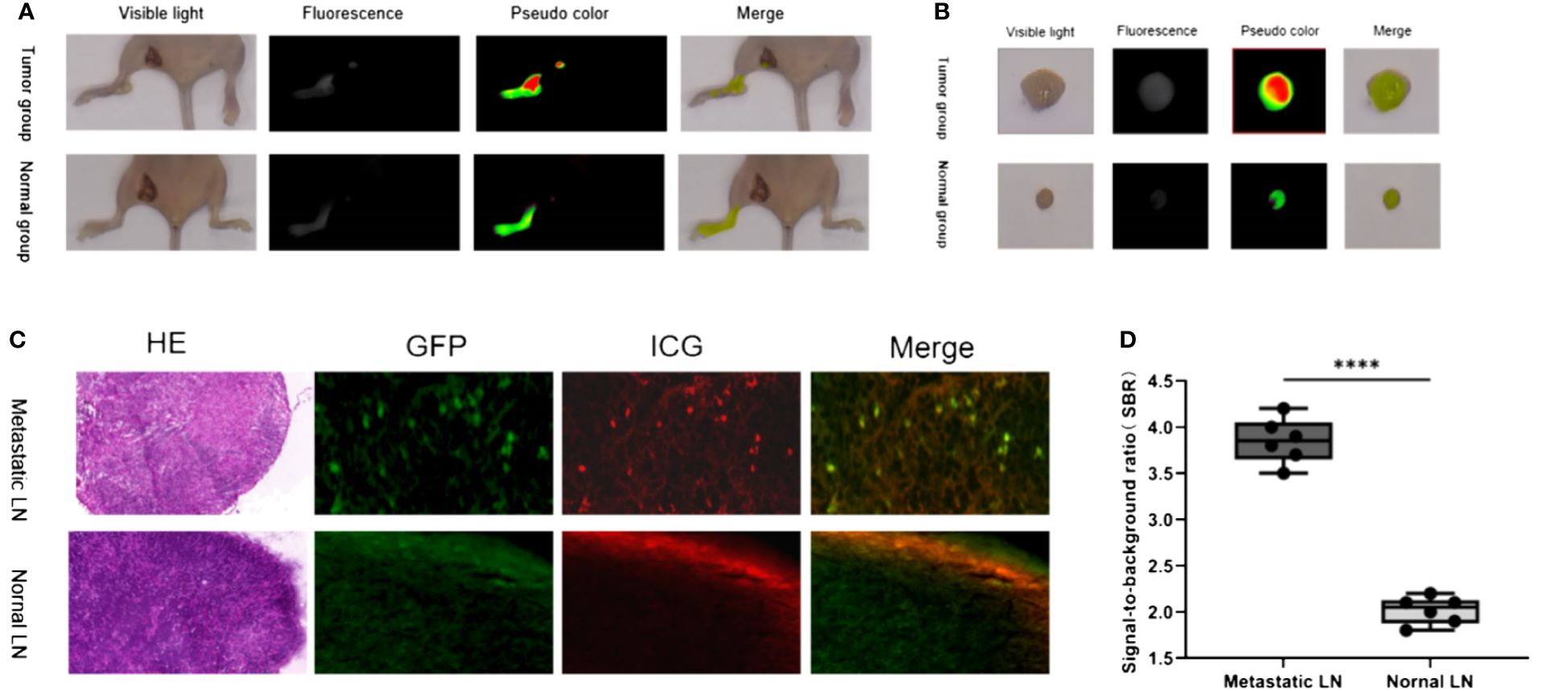
Figure 2 Fluorescence imaging characteristics of ICG injected in the lower limbs in normal and LN metastasis mice. (A) In vivo imaging of LN in tumor group and normal group; (B) In vitro imaging of LN in tumor group and normal group; (C) Representative H&E staining, GFP, ICG, and merged microscopic images of normal LNs and metastat LNs; (D) The SBR of metastatic and normal LNs. ****: P< 0.0001.
3.2 ICG-guided fluorescence imaging characteristics of pelvic LN resection in patients with bladder cancer
The surgeries in both groups of patients were successfully completed. No intraoperative or postoperative adverse reactions related to ICG injection were observed in any of the patients. In the intracutaneous group, successful visualization of pelvic LN was achieved in all 16 patients. In the transurethral group, successful visualization of pelvic LN was achieved in 8 of 10 patients, and the remaining 2 patients were not included in the statistical analysis. General patient information is provided in Table 1.
3.2.1 Imaging findings of the intracutaneous group
Following ICG injection, the external lens of the imaging system was initially focused on the injection site. A fluorescence image of the lower limbs was first acquired, and the system gradually moved toward the proximal end of the limbs. The system passed through the inner side of the calf, popliteal fossa, and inner side of the thigh, finally reaching the inguinal region. The time taken to reach the inguinal LN was 25–35 min (Figure 3). Before the peritoneum was opened intraoperatively, fluorescence imaging was performed at the distal and proximal ends of the iliac vessels and their surrounding areas. After opening the peritoneum along the external iliac vessels, circular, elliptical, and linear green fluorescence images were observed around the internal iliac vein, adjacent to the internal iliac artery, and near the iliolumbar vessel (Figure 4). We found that the fusion pseudo-color mode accurately displayed the LNs and lymphatic vessels, with a clear boundary from the surrounding nerves and blood vessels (Figure 5). Intracutaneous ICG injection into the lower limbs and perineum allowed visualization of the external iliac artery, internal iliac artery, common iliac vessels, and the obturator LN. The surgeons performed LN dissection using the fusion mode, and the LNs were numbered and sent for histopathological examination. 82 fluorescent-labeled tissues were removed from the 16 patients during the surgery. Postoperative pathological results showed 80 LN tissues and 2 fibroconnective tissues, with an accuracy rate of 97.6% for the fluorescent-labeled LN tissues. A total of 180 LNs were detected in the fluorescent-labeled tissues, with an average of 11.3 ± 2.4 LNs removed per patient. Three of these patients (18.8%) had a total of 6 positive LNs.
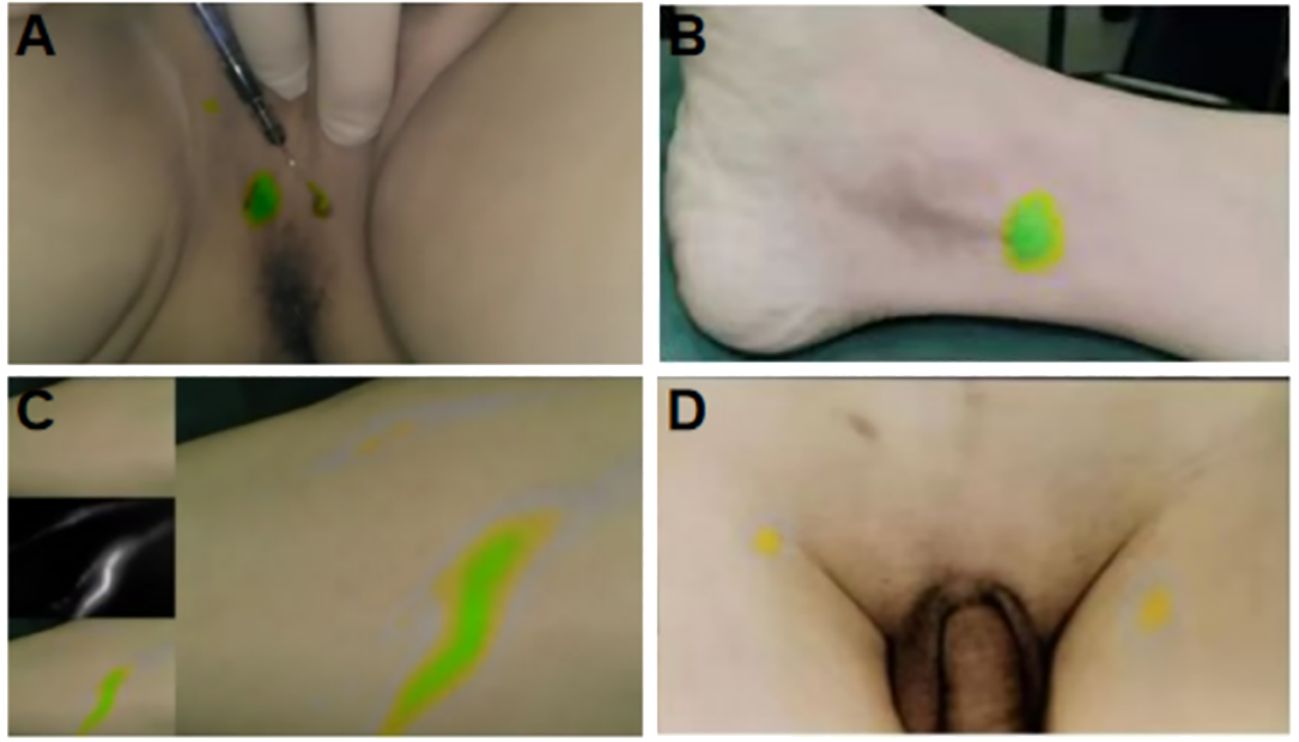
Figure 3 Fluorescent imaging of ICG injection site and its flow in lower limb LNs. (A) The injection site of lower limbs; (B) The injection site of perineum; (C) Fluorescent image of lymph flowing in lymphatic vessels after ICG lower limb injection; (D) Fluorescent imaging of inguinal LNs.
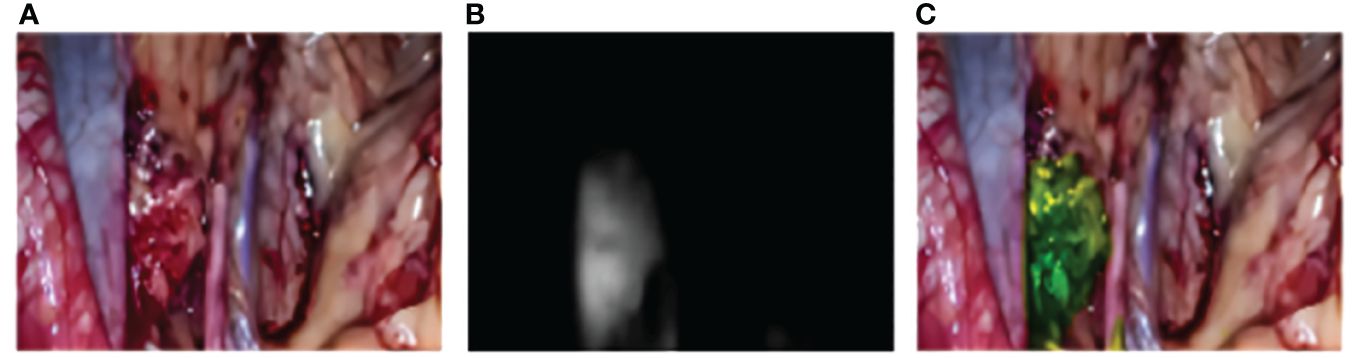
Figure 4 Intraoperative multimodal fluorescence imaging of obturator LN. (A) The visible light model; (B) The fluorescence model; (C) The merge model.
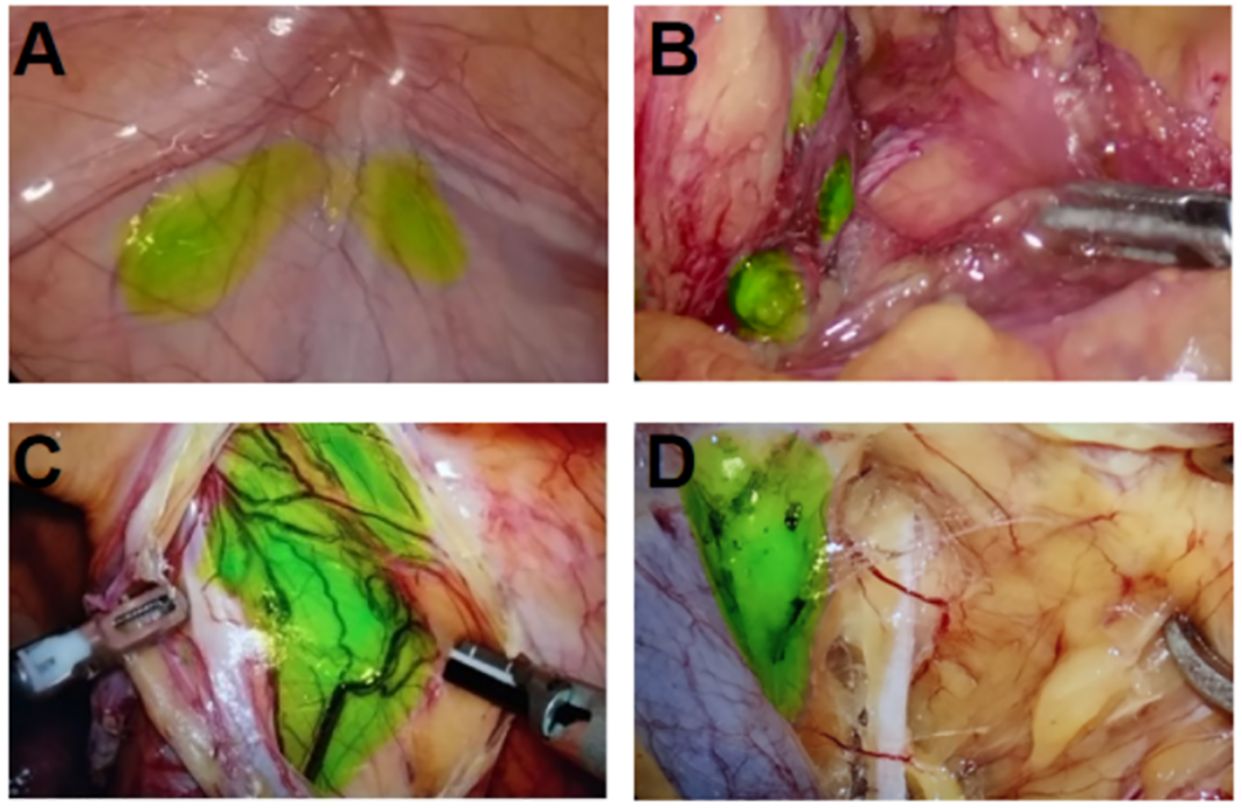
Figure 5 Resection of pelvic LNs in merge mode after ICG injection in the lower limbs combined with perineum. (A) Intra-abdominal iliac artery LN; (B) interiliac vessel LN; (C) Cloquet LN next to the circumflex iliac vein; (D) The obturator LN between the external iliac vein and the obturator nerve.
3.2.2 Imaging findings of the transurethral group
Among the 10 patients in the transurethral group, one patient experienced extensive leakage of ICG outside the bladder wall and peritoneum, resulting in blurred fluorescence imaging of the LNs and inability to identify them. One patient did not undergo bladder perfusion, resulting in nonvisualization of the LNs. In the remaining 8 patients, 32 fluorescent-labeled tissues were removed. Postoperative pathological results showed 27 LN tissues and 5 fibroconnective tissues, with an accuracy rate of 84.4% for the fluorescent-labeled LN tissues. A total of 69 LNs were detected in the fluorescent-labeled tissues, with an average of 8.6 ± 2.3 LNs removed per patient. Among these, 2 positive LNs were detected in one patient (12.5%).
The data results show that: the accuracy rate and detection number of fluorescent-labeled LN tissues were significantly higher in the intracutaneous group than in the transurethral group, and the results were statistically significant (p < 0.05). However, no significant difference was observed in the positive detection rate of LN between the two groups (p > 0.05). The results are shown in Figure 6.
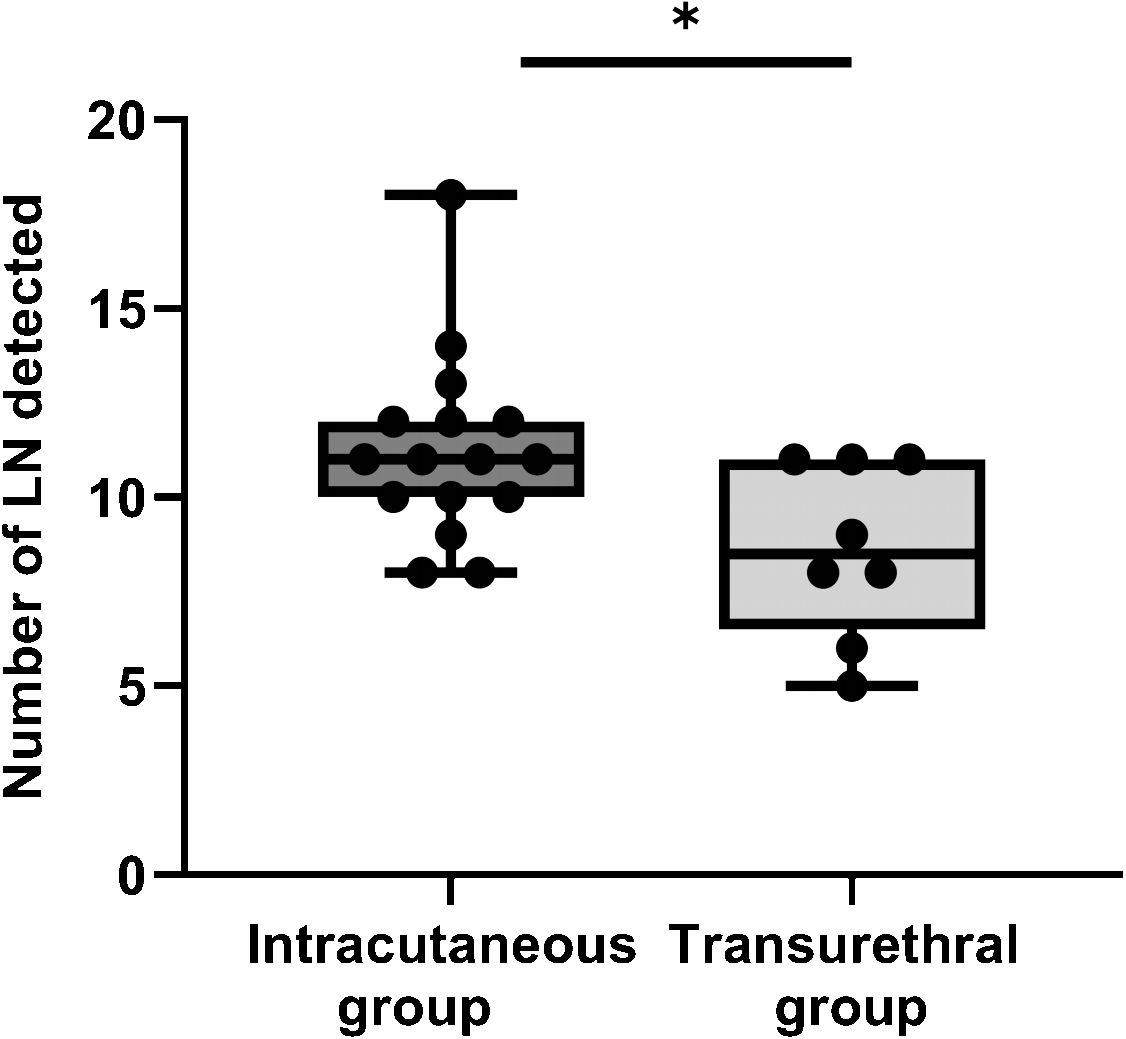
Figure 6 The number of LN detected between intracutaneous group and transurethral group. *: P< 0.05.
4 Discussion
Pelvic LNs are the primary route of metastasis in bladder cancer and an important indicator of patient prognosis (24). RC combined with PLND is the standard surgical approach for treating MIBC. However, the precise localization of pelvic LNs during the surgery remains a substantial challenge. F-PLND utilizes NIR fluorescence imaging to provide surgeons with high SBR for differentiating LN from the surrounding tissues, thereby effectively avoiding damage to the surrounding tissues and improving the efficiency of PLND.
The distribution of pelvic LN metastasis has always been a hot topic in urological tumors such as bladder cancer and prostate cancer (25). Gandaglia and Gondoputro evaluated the safety and effectiveness of 99mTc-based prostate-specific membrane antigen (PSMA) in robot-assisted radioguided surgery, and considered that this technology is valuable in detecting LN metastasis. However, its sensitivity is not high and therefore cannot replace conventional ePLND (26, 27). Francesco et al. correctly staged 98% of prostate cancer patients using ICG-guided lymphography, and the negative predictive value as high as 97% indicates that avoiding ePLND is safe for most patients (28). Similarly, another reported study evaluated conventional ePLND and PLND guided by ICG, it was found that adding fluorescence can enhance the identification rate of lymphatic drainage, and obtain more LNs and metastatic LNs, providing a more accurate local staging and prolonging recurrence-free survival (29).
In 2007, Knapp et al. (30) conducted the first study on intravesical injection of ICG for SLN mapping in an animal model. The authors emphasized that the control of intravesical pressure is a crucial factor to achieve the optimal imaging of SLNs and that the tracking of SLNs must be individualized because of anatomical variations in lymphatic drainage. In 2014, Schaafsma et al. (31) proposed that the intravesical injection of ICG with bladder distention is the optimal method to visualize SLNs. Subsequently, Manny et al. (32) identified SLNs in bladder cancer patients by using robot-assisted ICG fluorescence laparoscopy and reported 75% sensitivity and 52% specificity for positive LNs. However, Liedberg et al. (33) indicated that SLN detection in bladder cancer had a false negative rate of up to 19%.
The above-mentioned findings indicate that SLN detection in tumors of the urinary system, such as bladder cancer and prostate cancer, still lacks reliable theoretical basis (34). Transurethral intravesical injection of ICG not only carries the risk of urethral injury and infection but also poses challenges in controlling the injection site, dosage, and depth, leading to potential issues such as ICG leakage and intraoperative fluorescence contamination. The thickness of the bladder wall, intravesical pressure, and degree of bladder distention can also affect the stability of intraoperative ICG imaging. In the transurethral group, two patients did not show LN visualization intraoperatively because of inadequate control of parameters such as injection depth and intravesical pressure. Other studies have also confirmed that SLN mapping should be considered a supplementary approach to PLND during RC, rather than as a replacement of routine bilateral PLND (17).
The real benefits of ICG are represented by the radiation free procedure, the easy access and visualization of the targeted LNs as lymphatic route. However, although SLN tracing based on transurethral intravesical injection of ICG has certain clinical application value, it also has limitations such as high false negative rate, non-standardized procedures and a range of imaging influencing factors, which require further improvement. Additionally, due to the limitation of penetration depth, ICG may affect target observation in obese patients (35). Therefore, we believe that the current role of ICG in RC+PLND is to provide targeted and accurate anatomical guidance rather than being used for SLN applications.
Although the three-tier template for PLND was proposed as early as 2004 by Leissner et al. (36), there is still no consensus on the optimal extent of PLND. Bruins et al. (37)found that any type of PLND template was superior to no PLND. A larger dissection range may remove more LNs and potentially provide better survival benefits. However, it can also lead to various complications such as lymphatic cysts, obturator nerve injury, and lymphedema (38). Therefore, the determination of the ideal dissection range for implementing more precise PLND is highly clinically relevant.
In the present study, we analyzed the lymphatic drainage system of the normal lower limbs, perineum, and bladder (39, 40). We found that the LNs draining the normal pelvic organs and those associated with tumor metastasis are the intrinsic LNs of the pelvic anatomy, including the internal and external iliac, obturator, and presacral LNs. A previous study (41) reported that the use of ICG and iodine injections into the lower limbs to determine the lymphatic reflux pathway provides a theoretical basis for our experiment. Therefore, we combined indirect lymphangiography techniques with NIR fluorescence imaging to investigate the feasibility of locating and identifying pelvic LNs through ICG injection into the lower limbs and perineum.
First, we established a model of LN metastasis in mice and analyzed LN imaging after ICG injection in the footpad. We found that this injection method was feasible, and the LNs in both control and experimental groups showed successful imaging, with higher SBR in the experimental group than in the control group. Based on these results, we suggest that both normal and metastatic LNs can be identified in mice.
A comparison with the classical approach of transurethral intravesical injection of ICG showed that ICG injection in the lower limbs and perineum can detect more LNs, with an accuracy rate of 97.3% for LN identification. This may be related to the fact that lymphatic vessels in fibroconnective tissues are fluorescently labeled and removed together. Additionally, we found that intraoperative damage to small lymphatic vessels or LNs may cause leakage of ICG, leading to fluorescence contamination in the local surgical field, thus affecting the localization and removal of LNs. Therefore, surgeons should try to be gentle during the operation and avoid excessive separation of large tissue. Both groups showed positive fluorescence imaging of LNs during the surgery, although the difference was not statistically significant. Inoue et al. (42) first reported that the intravesical injection of ICG could visualize metastatic LNs in patients with bladder cancer. The results showed a false-negative rate of 100%, and it was suggested that when the lymphatic pathway is completely blocked by tumor cells, an alternative pathway may be induced. ICG, as a nontargeted contrast agent, may be more suitable for detecting pelvic negative LNs rather than metastatic LNs. However, in another study (43), the intravenous injection of ICG successfully visualized metastatic LNs in mice and tumor patients with an accuracy rate of 83.3%. This finding holds promise as a new method for identifying LN metastasis.
The present study confirmed that ICG injection in the lower limbs and perineum can assist surgeons in the precise localization and removal of LNs during F-PLND, thereby minimizing unnecessary extensive LN dissection and reducing intraoperative damage. Considering the importance of LN dissection in the prognosis of bladder cancer, the localization and tracking of F-PLND should be a focus of future research studies (44).
Although we successfully visualized pelvic LNs by injecting ICG in the lower limbs and perineum, thereby effectively improving the accuracy of LN identification and the efficiency of LN dissection during surgery, the present study has some limitations. Firstly, we did not remove fluorescence-negative tissues to analyze the specificity of this injection method for visualizing pelvic LNs. This will be a direction of our further research. Secondly, this technique cannot evaluate the status of LNs intraoperatively. In the future, interdisciplinary research across multiple fields will be needed to exploit targeted probes to address this issue. Lastly, this is a single-center small sample study, and the results need further validation in multicenter studies with larger samples.
5 Conclusions
This study confirms that after intradermal injection of ICG in the lower limbs and perineum, ICG can accumulate in the pelvic LNs through lymphatic reflux. Compared to intravesical injection, the operation method is simpler, safer and the intraoperative imaging effect is more stable. Under the guidance of F-PLND, surgeons can real-time track, accurately locate and precisely remove pelvic LNs, greatly improving the efficiency of PLND. This technique will provide a new theory and method for further research on the accurate localization and qualitative analysis of pelvic LNs during surgery.
Data availability statement
The original contributions presented in the study are included in the article/supplementary material. Further inquiries can be directed to the corresponding author.
Ethics statement
The studies involving humans were approved by the ethics committee of the First Hospital of Shanxi Medical University. The studies were conducted in accordance with the local legislation and institutional requirements. The participants provided their written informed consent to participate in this study. The animal study was approved by the Ethics Committee for Animal Welfare of Shanxi Medical University. The study was conducted in accordance with the local legislation and institutional requirements. Written informed consent was obtained from the individual(s) for the publication of any potentially identifiable images or data included in this article.
Author contributions
YnZ: Writing – original draft, Writing – review & editing. XG: Writing – original draft, Writing – review & editing. YeZ: Writing – original draft, Writing – review & editing. JW: Writing – review & editing. PY: Writing – review & editing. HK: Writing – review & editing. YS: Writing – review & editing. CL: Writing – original draft, Writing – review & editing. XY: Writing – original draft, Writing – review & editing.
Funding
The author(s) declare financial support was received for the research, authorship, and/or publication of this article. This work was supported by The Central Guidance on Local Science and Technology Development Fund of Shanxi Province (No. YDZJSX2021C010), The Patent Conversion Project Fund of Shanxi Province (No. 202304008).
Acknowledgments
The authors thank all the patients and physicians who participated in this study. We also thank the pathology department of First Hospital of Shanxi Medical University for assistance with pathological evaluation.
Conflict of interest
The authors declare that the research was conducted in the absence of any commercial or financial relationships that could be construed as a potential conflict of interest.
Publisher’s note
All claims expressed in this article are solely those of the authors and do not necessarily represent those of their affiliated organizations, or those of the publisher, the editors and the reviewers. Any product that may be evaluated in this article, or claim that may be made by its manufacturer, is not guaranteed or endorsed by the publisher.
References
1. Sung H, Ferlay J, Siegel RL, Laversanne M, Soerjomataram I, Jemal A, et al. Global cancer statistics 2020: GLOBOCAN estimates of incidence and mortality worldwide for 36 cancers in 185 countries. CA Cancer J Clin. (2021) 71:209–49. doi: 10.3322/caac.21660
2. Siegel RL, Miller KD, Wagle NS, Jemal A. Cancer statistics, 2023. CA Cancer J Clin. (2023) 73:17–48. doi: 10.3322/caac.21763
3. Breau R, Karnes R, Farmer S, Thapa P, Cagiannos I, Morash C, et al. Progression to detrusor muscle invasion during urothelial carcinoma surveillance is associated with poor prognosis. BJU Int. (2014) 113:900–6. doi: 10.1111/bju.12403
4. Meeks J, Bellmunt J, Bochner B, Clarke N, Daneshmand S, Galsky M, et al. A systematic review of neoadjuvant and adjuvant chemotherapy for muscle-invasive bladder cancer. Eur Urol. (2012) 62:523–33. doi: 10.1016/j.eururo.2012.05.048
5. Del Bene G, Calabrò F, Giannarelli D, Plimack ER, Harshman LC, Yu EY, et al. Neoadjuvant vs. Adjuvant chemotherapy in muscle invasive bladder cancer (MIBC): analysis from the RISC database. Front Oncol. (2018) 8:463. doi: 10.3389/fonc.2018.00463
6. Student V, Tudos Z, Studentova Z, Cesak O, Studentova H, Repa V, et al. Effect of peritoneal fixation (PerFix) on lymphocele formation in robot-assisted radical prostatectomy with pelvic lymphadenectomy: results of a randomized prospective trial. Eur Urol. (2023) 83:154–62. doi: 10.1016/j.eururo.2022.07.027
7. Haiquel L, Cathelineau X, Sanchez-Salas R, Macek P, Secin F. Pelvic lymph node dissection in high-risk prostate cancer. Int Braz J urol. (2022) 48:54–66. doi: 10.1590/s1677-5538.Ibju.2020.1063
8. Law B, Yusuf Z, Ng Y, Aly E. Does adding lateral pelvic lymph node dissection to neoadjuvant chemotherapy improve outcomes in low rectal cancer? Int J colorectal Dis. (2020) 35:1387–95. doi: 10.1007/s00384-020-03656-1
9. Crozier J, Papa N, Perera M, Stewart M, Goad J, Sengupta S, et al. Lymph node yield in node-negative patients predicts cancer specific survival following radical cystectomy for transitional cell carcinoma. Investig Clin Urol. (2017) 58:416–22. doi: 10.4111/icu.2017.58.6.416
10. Nakagawa T. Lymph node dissection for bladder cancer: Current standards and the latest evidence. Int J Urol. (2021) 28:7–15. doi: 10.1111/iju.14398
11. Morton D, Cochran A. The case for lymphatic mapping and sentinel lymphadenectomy in the management of primary melanoma. Br J Dermatol. (2004) 151:308–19. doi: 10.1111/j.1365-2133.2004.06133.x
12. Olson M, Ly Q, Mohs A. Fluorescence guidance in surgical oncology: challenges, opportunities, and translation. Mol Imaging Biol. (2019) 21:200–18. doi: 10.1007/s11307-018-1239-2
13. Bargon C, Huibers A, Young-Afat D, Jansen B, Borel-Rinkes I, Lavalaye J, et al. Sentinel lymph node mapping in breast cancer patients through fluorescent imaging using indocyanine green: the INFLUENCE trial. Ann Surg. (2022) 276:913–20. doi: 10.1097/sla.0000000000005633
14. Brouwer O, van den Berg N, Mathéron H, van der Poel H, van Rhijn B, Bex A, et al. A hybrid radioactive and fluorescent tracer for sentinel node biopsy in penile carcinoma as a potential replacement for blue dye. Eur Urol. (2014) 65:600–9. doi: 10.1016/j.eururo.2013.11.014
15. Gilmore D, Khullar O, Gioux S, Stockdale A, Frangioni J, Colson Y, et al. Effective low-dose escalation of indocyanine green for near-infrared fluorescent sentinel lymph node mapping in melanoma. Ann Surg Oncol. (2013) 20:2357–63. doi: 10.1245/s10434-013-2905-x
16. Sinha A, West A, Hayes J, Teoh J, Decaestecker K, Vasdev N. Methods of sentinel lymph node detection and management in urinary bladder cancer-A narrative review. Curr Oncol (Toronto Ont). (2022) 29:1335–48. doi: 10.3390/curroncol29030114
17. Małkiewicz B, Jędrzejuk D, Gurwin A, Wilk K, Knecht-Gurwin K, Kiełb P, et al. Diagnostic value of the sentinel lymph node technique in patients with muscle-invasive bladder cancer. J Clin Med. (2023) 12:3092. doi: 10.3390/jcm12093092
18. Rietbergen DDD, van Gennep EJ, KleinJan GH, Donswijk M, Valdés Olmos RA, van Rhijn BW, et al. 2. Clin Nucl Med. (2022) 47:774–80. doi: 10.1097/rlu.0000000000004301
19. Püllen L, Costa PF, Darr C, Hess J, Kesch C, Rehme C, et al. Near-infrared fluorescence lymph node template region dissection plus backup lymphadenectomy in open radical cystectomy for bladder cancer using an innovative handheld device: A single center experience. J Surg Oncol. (2024). doi: 10.1002/jso.27618
20. Cassinotti E, Boni L, Baldari L. Application of indocyanine green (ICG)-guided surgery in clinical practice: lesson to learn from other organs-an overview on clinical applications and future perspectives. Updates Surg. (2023) 75:357–65. doi: 10.1007/s13304-022-01361-y
21. Zarifmahmoudi L, Ghorbani H, Sadri K, Tavakkoli M, Keshvari M, Salehi M, et al. Sentinel node biopsy in Urothelial carcinoma of the bladder: systematic review and meta-analysis. Urol Int. (2019) 103:373–82. doi: 10.1159/000497310
22. Thompson L, Futrell J, Anlyan W. Lymphangiography: direct and indirect utilizing an iodinated emulsion. Plast reconstructive Surg. (1966) 37:125–30. doi: 10.1097/00006534-196602000-00007
23. Shinaoka A, Koshimune S, Yamada K, Kumagishi K, Suami H, Kimata Y, et al. Correlations between tracer injection sites and lymphatic pathways in the leg: A near-infrared fluorescence lymphography study. Plast Reconstr Surg. (2019) 144:634–42. doi: 10.1097/prs.0000000000005982
24. Sathianathen N, Risk M, Konety B. Lymphadenectomy for muscle-invasive bladder cancer and upper tract urothelial cell carcinoma. Urologic Clinics North America. (2018) 45:215–28. doi: 10.1016/j.ucl.2017.12.006
25. Grivas N, van den Bergh RCN, Brouwer OR, KleinJan GH, Ramirez-Backhaus M, Wilthagen EA, et al. Pelvic lymph node distribution and metastases of prostate and bladder cancer: a systematic literature review and template proposal. World J Urol. (2021) 39:751–9. doi: 10.1007/s00345-020-03281-1
26. Gandaglia G, Mazzone E, Stabile A, Pellegrino A, Cucchiara V, Barletta F, et al. Prostate-specific membrane antigen Radioguided Surgery to Detect Nodal Metastases in Primary Prostate Cancer Patients Undergoing Robot-assisted Radical Prostatectomy and Extended Pelvic Lymph Node Dissection: Results of a Planned Interim Analysis of a Prospective Phase 2 Study. Eur Urol. (2022) 82:411–8. doi: 10.1016/j.eururo.2022.06.002
27. Gondoputro W, Scheltema MJ, Blazevski A, Doan P, Thompson JE, Amin A, et al. Robot-assisted prostate-specific membrane antigen-radioguided surgery in primary diagnosed prostate cancer. J Nucl Med. (2022) 63:1659–64. doi: 10.2967/jnumed.121.263743
28. Claps F, de Pablos-Rodríguez P, Gómez-Ferrer Á, Mascarós JM, Marenco J, Collado Serra A, et al. Free-indocyanine green-guided pelvic lymph node dissection during radical prostatectomy. Urol Oncol. (2022) 40:489.e419–489.e426. doi: 10.1016/j.urolonc.2022.08.005
29. Claps F, Ramírez-Backhaus M, Mir Maresma MC, Gómez-Ferrer Á, Mascarós JM, Marenco J, et al. Indocyanine green guidance improves the efficiency of extended pelvic lymph node dissection during laparoscopic radical prostatectomy. Int J Urol. (2021) 28:566–72. doi: 10.1111/iju.14513
30. Knapp D, Adams L, Degrand A, Niles J, Ramos-Vara J, Weil A, et al. Sentinel lymph node mapping of invasive urinary bladder cancer in animal models using invisible light. Eur Urol. (2007) 52:1700–8. doi: 10.1016/j.eururo.2007.07.007
31. Schaafsma B, Verbeek F, Elzevier H, Tummers Q, van der Vorst J, Frangioni J, et al. Optimization of sentinel lymph node mapping in bladder cancer using near-infrared fluorescence imaging. J Surg Oncol. (2014) 110:845–50. doi: 10.1002/jso.23740
32. Manny TB, Hemal AK. Fluorescence-enhanced robotic radical cystectomy using unconjugated indocyanine green for pelvic lymphangiography, tumor marking, and mesenteric angiography: the initial clinical experience. Urology. (2014) 83:824–9. doi: 10.1016/j.urology.2013.11.042
33. Liedberg F, Chebil G, Davidsson T, Gudjonsson S, Månsson W. Intraoperative sentinel node detection improves nodal staging in invasive bladder cancer. J Urol. (2006) 175:84–8. doi: 10.1016/s0022-5347(05)00066-2
34. Małkiewicz B, Kiełb P, Kobylański M, Karwacki J, Poterek A, Krajewski W, et al. Sentinel lymph node techniques in urologic oncology: current knowledge and application. Cancers. (2023) 15:2495. doi: 10.3390/cancers15092495
35. Jewell EL, Huang JJ, Abu-Rustum NR, Gardner GJ, Brown CL, Sonoda Y, et al. Detection of sentinel lymph nodes in minimally invasive surgery using indocyanine green and near-infrared fluorescence imaging for uterine and cervical Malignancies. Gynecol Oncol. (2014) 133:274–7. doi: 10.1016/j.ygyno.2014.02.028
36. Leissner J, Ghoneim M, Abol-Enein H, Thüroff J, Franzaring L, Fisch M, et al. Extended radical lymphadenectomy in patients with urothelial bladder cancer: results of a prospective multicenter study. J Urol. (2004) 171:139–44. doi: 10.1097/01.ju.0000102302.26806.fb
37. Bruins H, Veskimae E, Hernandez V, Imamura M, Neuberger M, Dahm P, et al. The impact of the extent of lymphadenectomy on oncologic outcomes in patients undergoing radical cystectomy for bladder cancer: a systematic review. Eur Urol. (2014) 66:1065–77. doi: 10.1016/j.eururo.2014.05.031
38. Cacciamani G, Maas M, Nassiri N, Ortega D, Gill K, Dell'Oglio P, et al. Impact of pelvic lymph node dissection and its extent on perioperative morbidity in patients undergoing radical prostatectomy for prostate cancer: A comprehensive systematic review and meta-analysis. Eur Urol Oncol. (2021) 4:134–49. doi: 10.1016/j.euo.2021.02.001
39. Tilki D, Brausi M, Colombo R, Evans C, Fradet Y, Fritsche H, et al. Lymphadenectomy for bladder cancer at the time of radical cystectomy. Eur Urol. (2013) 64:266–76. doi: 10.1016/j.eururo.2013.04.036
40. Hugen C, Daneshmand S. Lymph node dissection in bladder cancer: Where do we stand? World J Urol. (2017) 35:527–33. doi: 10.1007/s00345-015-1751-9
41. Shinaoka A, Koshimune S, Suami H, Yamada K, Kumagishi K, Boyages J, et al. Lower-limb lymphatic drainage pathways and lymph nodes: A CT lymphangiography cadaver study. Radiology. (2020) 294:223–9. doi: 10.1148/radiol.2019191169
42. Inoue S, Shiina H, Mitsui Y, Yasumoto H, Matsubara A, Igawa M. Identification of lymphatic pathway involved in the spread of bladder cancer: Evidence obtained from fluorescence navigation with intraoperatively injected indocyanine green. Can Urol Assoc J. (2013) 7:E322–328. doi: 10.5489/cuaj.12096
43. Kim K, Han KN, Choi BH, Rho J, Lee JH, Eo JS, et al. Identification of metastatic lymph nodes using indocyanine green fluorescence imaging. Cancers (Basel). (2023) 15:1964. doi: 10.3390/cancers15071964
Keywords: bladder cancer, pelvic lymph node dissection, near-infrared fluorescence imaging, indocyanine green, precise visualization
Citation: Zhang Y, Guo X, Zhang Y, Wei J, Yan P, Kang H, Shu Y, Liu C and Yang X (2024) A preliminary investigation of precise visualization, localization, and resection of pelvic lymph nodes in bladder cancer by using indocyanine green fluorescence-guided approach through intracutaneous dye injection into the lower limbs and perineum. Front. Oncol. 14:1384268. doi: 10.3389/fonc.2024.1384268
Received: 09 February 2024; Accepted: 06 May 2024;
Published: 22 May 2024.
Edited by:
Evgenii Belykh, Rutgers University, United StatesReviewed by:
Francesco Claps, The Netherlands Cancer Institute (NKI), NetherlandsAlvand Sehat, The State University of New Jersey, United States
Copyright © 2024 Zhang, Guo, Zhang, Wei, Yan, Kang, Shu, Liu and Yang. This is an open-access article distributed under the terms of the Creative Commons Attribution License (CC BY). The use, distribution or reproduction in other forums is permitted, provided the original author(s) and the copyright owner(s) are credited and that the original publication in this journal is cited, in accordance with accepted academic practice. No use, distribution or reproduction is permitted which does not comply with these terms.
*Correspondence: Chao Liu, TGl1Y2hhb18wODMxQDE2My5jb20=; Xiaofeng Yang, eXhmeWxxQDE2My5jb20=
†These authors have contributed equally to this work
‡ORCID: Yunmeng Zhang, orcid.org/0009-0004-7810-4720