- 1Cancer Biology Laboratory, Department of Biosciences and Bioengineering, Indian Institute of Technology Guwahati (IITG), Guwahati, Assam, India
- 2Radiological Sciences Department, College of Applied Medical Sciences, King Khalid University, Abha, Saudi Arabia
- 3BioImaging Unit, Space Research Centre, Michael Atiyah Building, University of Leicester, Leicester, United Kingdom
- 4Electrical Engineering Department, College of Engineering, King Khalid University, Abha, Saudi Arabia
- 5Chair of Vegetative Anatomy, Ludwig-Maximilians-University Munich, Munich, Germany
- 6Department of Pharmacology, Yong Loo Lin School of Medicine, National University of Singapore, Singapore, Singapore
- 7National University of Singapore (NUS) Center for Cancer Research, Yong Loo Lin School of Medicine, National University of Singapore, Singapore, Singapore
- 8School of Basic Medicine, Health Science Center, Yangtze University, Jingzhou, Hubei, China
Ovarian cancer (OVC) is one of the most common causes of cancer-related deaths in women worldwide. Despite advancements in detection and therapy, the prognosis of OVC remains poor due to late diagnosis and the lack of effective therapeutic options at advanced stages. Therefore, a better understanding of the biology underlying OVC is essential for the development of effective strategies for early detection and targeted therapies. Nuclear receptors (NRs) are a superfamily of 48 transcription factors that, upon binding to their specific ligand, play a vital role in regulating various cellular processes such as growth, development, metabolism, and homeostasis. Accumulating evidence from several studies has shown that their aberrant expression is associated with multiple human diseases. Numerous NRs have shown significant effects in the development of various cancers, including OVC. This review summarizes the recent findings on the role of NRs in OVC, as well as their potential as prognostic and therapeutic markers. Further, the basic structure and signaling mechanism of NRs have also been discussed briefly. Moreover, this review highlights their cellular and molecular mechanisms in chemoresistance and chemosensitization. Further, the clinical trials targeting NRs for the treatment of OVC have also been discussed.
1 Introduction
Ovarian cancer (OVC) is one of the most intractable diseases, with an increasing number of mortalities in women worldwide (1, 2). As per the report of GLOBOCAN 2020, OVC ranks eighth in terms of both the number of new cases (313,959) and deaths (207,252) across the world (3). Even though chemotherapy and surgery have proven effective against OVC over the past few years, their undesirable side effects that affect the quality of life of patients including fatigue, neurotoxicity, and tumor recurrence, pose a major concern (4–6). Despite the emergence of various multimodal treatment strategies such as immunotherapy and targeted therapies, OVC remains life-threatening due to its high molecular heterogeneity, peritoneal dissemination, and late-stage diagnosis (2, 7, 8). Moreover, the chemoresistance of tumor cells also stands as the Achilles’ heel in overcoming the consequences of this disease (9). Hence, there is an imperative need for the identification of potential targets and novel drugs that could lead to the development of safe, efficacious, and innovative therapeutic strategies circumventing OVC. As nuclear receptors (NRs) play a pivotal role in the development of different malignancies, in the present review, we have highlighted the role of NRs in the development and progression of OVC and the potential of agonists and antagonists of NRs for the treatment of this cancer. In addition, the compounds derived from natural sources also showed potential effects in the prevention and treatment of different cancers, including OVC (10–14). Hence, we have also included the role of natural products in modulating NRs in OVC cells.
NRs are members of a large superfamily of transcription factors (TFs) that are present in all metazoans, except plants and yeast (15, 16). These proteins are thought to have a significant role in maintaining homeostasis, immunoregulation, and regular physiological processes like cell growth and differentiation (15, 17–21). Most of these receptors have well-defined ligands, apart from a few, named ‘orphan receptors’ which lack specific ligands. Unlike other TFs, NR’s activity can be regulated according to the ligand that binds to it. Their ligands are small lipophilic molecules that include thyroid hormone, some oxysterols, retinoic acid (RA), or steroid hormones like estrogen and progesterone that could permeate through the cell membrane and interact with NRs in the cytoplasm (22, 23). NRs, upon activation, modulate the transcription of numerous genes that are involved in essential biological activities such as cell differentiation, circadian functions, metabolism, reproduction, etc (24). NRs perform integrative roles in regulating the transcription of different genes in various cell types and tissues that control development and homeostasis (15, 23). The basic structure of NRs includes a variable A/B domain at the amino-terminal, which contains an activation function 1 (AF1) that interacts with various co-regulator proteins, a C domain which is a centrally conserved DNA-binding domain (DBD) with two zinc finger motifs, a D domain that serves as a short hinge region between DBD and ligand binding domain (LBD) which also facilitates nuclear localization and a fairly well-conserved E domain, found at the carboxy-terminal (summarized in Figure 1) (30). Ligands, along with their coactivators or corepressors control the action of NRs by regulating the activation function 2 (AF2) present at the LBD (31). Selective modulation of AF2 helix by specific synthetic agonists/antagonists can alter the activities of NRs in a favorable manner. For example, it was observed that the binding of estrogen antagonist, dihydroxytamoxifen results in a distorted configuration of AF2 helix (Figure 2A) (31).
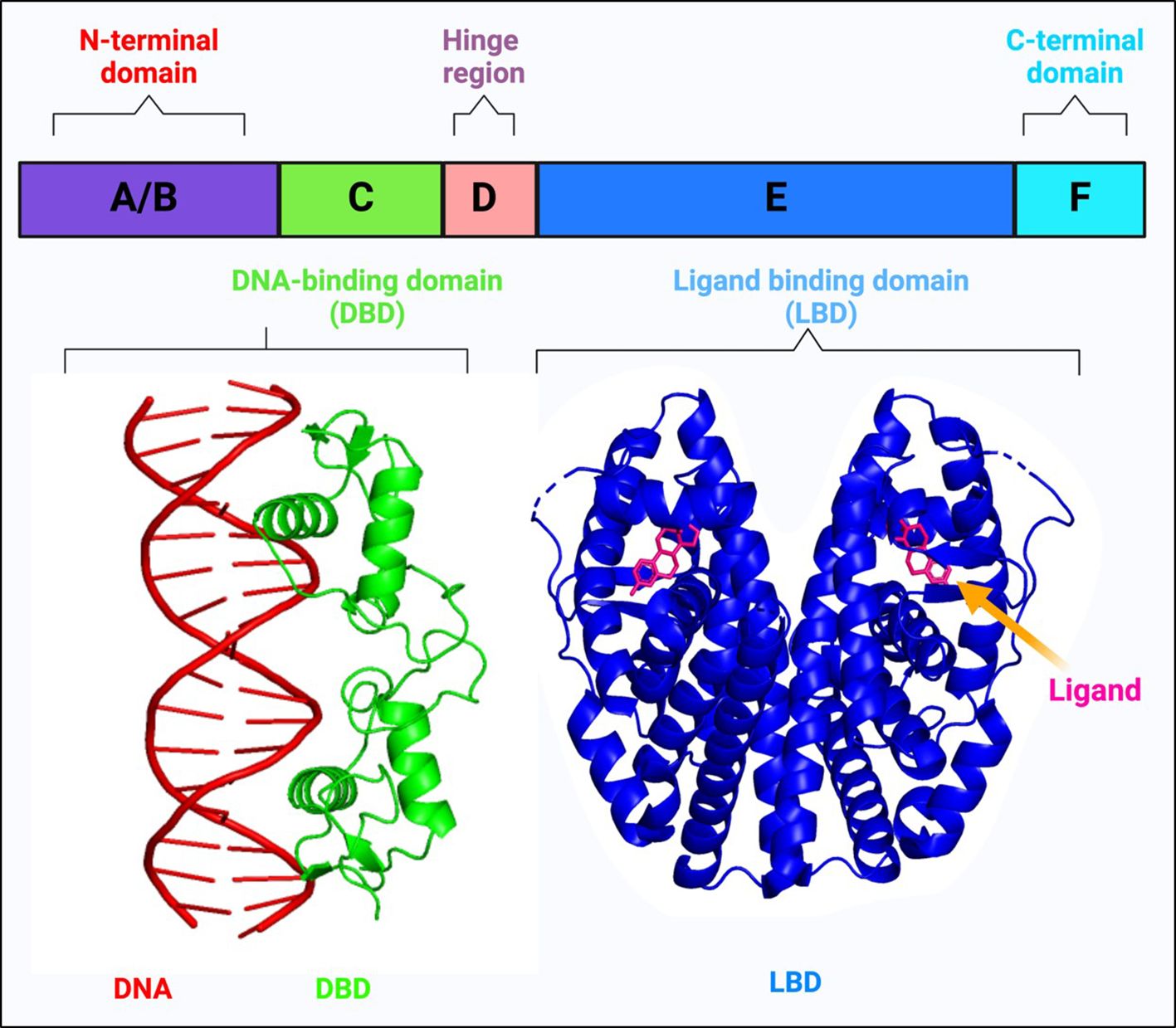
Figure 1 Schematic representation of the basic structure of a nuclear receptor, highlighting its different domains: The N-terminal domain (A, B), the DNA-binding domain (C), the hinge region (D), the ligand-binding domain (E), and the C-terminal domain (F). The DNA-binding domain (DBD) interacts with a double-stranded DNA helix shown in red (The crystal structure of the estrogen receptor DNA-binding domain bound to DNA, PDB ID: 1HCQ). This interaction is critical for the nuclear receptor’s ability to regulate gene expression. The structure of the ligand-binding domain (LBD) demonstrates how ligands can bind to nuclear receptors, influencing their conformation and function (Structure of complex between human estrogen receptor alpha-LBD in complex with 17-β-estradiol, PDB ID: 1GWR is depicted). The ligands typically modulate the receptor’s activity, affecting its ability to interact with co-regulators and its gene-regulating capability (25–29).
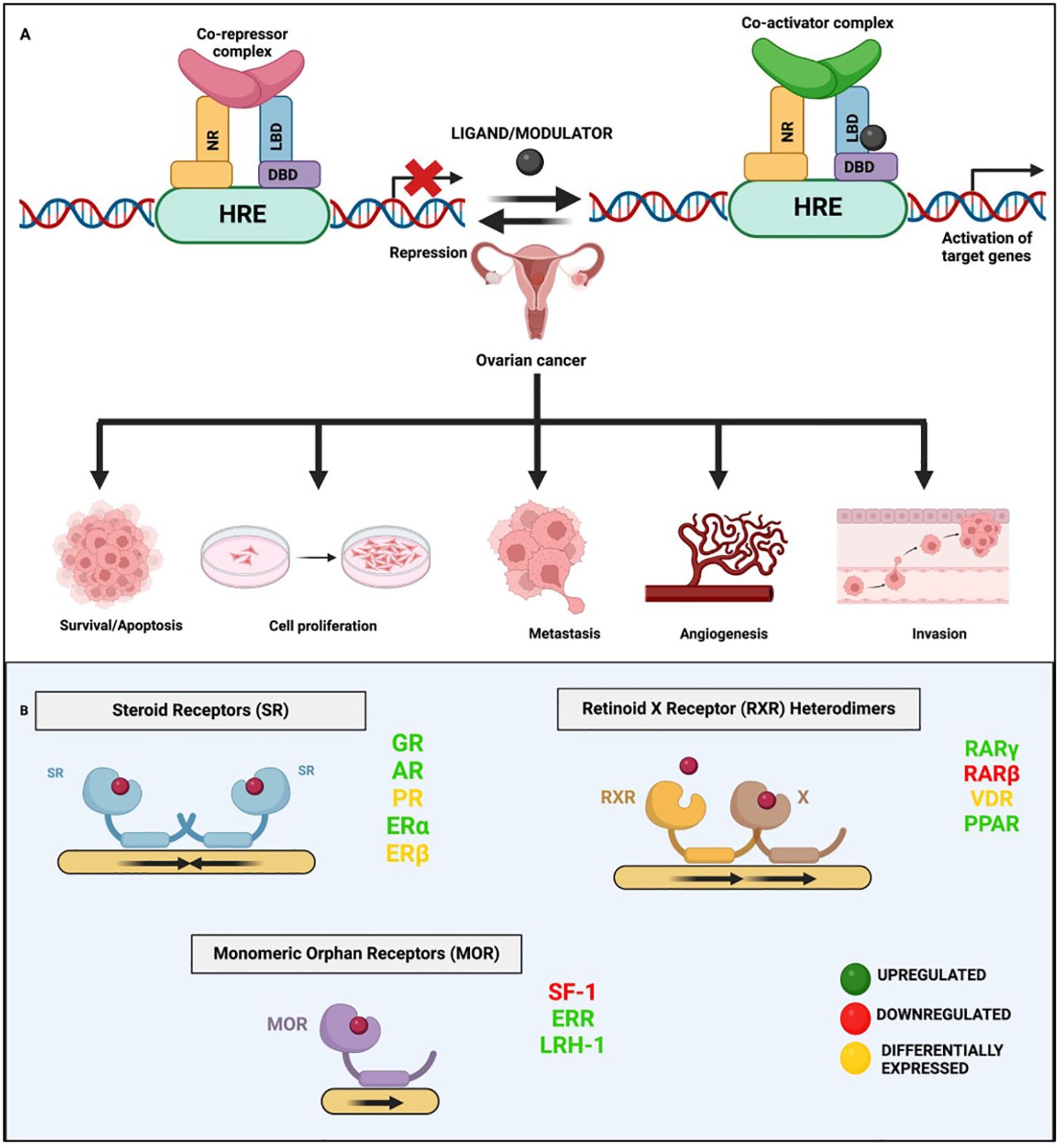
Figure 2 General mechanism of nuclear receptor signaling. (A) This panel illustrates the dual roles of NRs in gene regulation via binding to hormone response elements (HREs). In the case of Type II NR signaling, in the absence of a ligand/modulator, the NR forms a complex with co-repressors that bind to HREs, repressing gene expression. Upon ligand binding, the receptor undergoes a conformational change, displacing co-repressors and recruiting co-activators, leading to the activation/suppression of target genes including the ones involved in various hallmarks of OVC. (B) This panel depicts the different types of NRs implicated in OVC and the general mechanism of their binding as monomers, homo- or hetero- dimers.
Clear lines of evidence have emerged elucidating the role of NRs in various pathologies, including asthma, diabetes, rheumatoid arthritis, and different cancers, including OVC (32–36). Multiple studies have proved the role of NRs in regulating significant hallmarks of cancer, such as proliferation, survival, epithelial-mesenchymal transition (EMT), invasion, migration, apoptosis, etc (37–44). NRs exhibit differential expression in cancers, where some act as oncogenes while others act as tumor suppressors (45–47). Numerous NRs like estrogen receptor (ER), peroxisome-proliferator-activator receptor (PPAR), progesterone receptors (PR), retinoic acid receptors (RAR), and vitamin D receptors (VDR) have been linked to several malignancies (48–51). Many preclinical and clinical studies have revealed the role of dysregulated NRs as therapeutic targets for OVC. Consequently, there is an opportunity to develop selective agonists and antagonists for these receptors (22, 23, 31). Over recent years, there has been a quantum leap in the development of several drugs for the treatment of OVC that specifically modulate NR signaling in tumor cells. For instance, letrozole, an aromatase (an enzyme that converts androgens to estrogens) inhibitor, is undergoing Phase III clinical trial for the treatment of OVC due to the high level of estrogen (52). Although several studies have uncovered the role of NRs as important molecular markers and targets in diagnosis and therapy, there is currently no comprehensive compilation of the various preclinical and clinical studies on NRs in OVC. Therefore, in this review, we focus on the role of NRs in the development of OVC, the modulation of NRs by multiple agonists or antagonists, their mechanistic underpinnings, and the ongoing clinical trials delineating their potential as a therapeutic option for clinical management of this deadly cancer.
2 NRs in OVC
NRs have long been at the cutting edge of cancer research, where they are known to play crucial roles in regulating these malignancies, including OVC. The fact that many of the identified NRs are responsive to hormones and can bind with drugs or small molecules makes them potential targets for OVC therapeutics. NRs regulate differentiation and development and maintain homeostasis in normal cells, but they control cellular growth, apoptosis, invasion, and migration, by dysregulating major signaling processes in a variety of OVC cell types. For instance, few of the NRs, primarily hormone/steroid receptors including ER and androgen receptor (AR), show tremendous upregulation, but others, such as VDR and RAR, are downregulated or differentially expressed in OVC (Figure 2B) (53–56). The expression of various NRs in OVC is depicted in Table 1 (49, 53–55, 57–74). Hence, we attempt to provide a deeper comprehension of the multifaceted aspects of variable expression of NRs in OVC tumor cells and their underlying molecular mechanism upon agonist/antagonist binding, (Table 2) which envisages their significant role in the treatment of OVC. The mechanism of action of NRs upon agonist/antagonist binding is briefly depicted in Figure 3.

Table 2 Mechanistic role of various nuclear receptors in ovarian cancer in the presence of their agonists/antagonists.
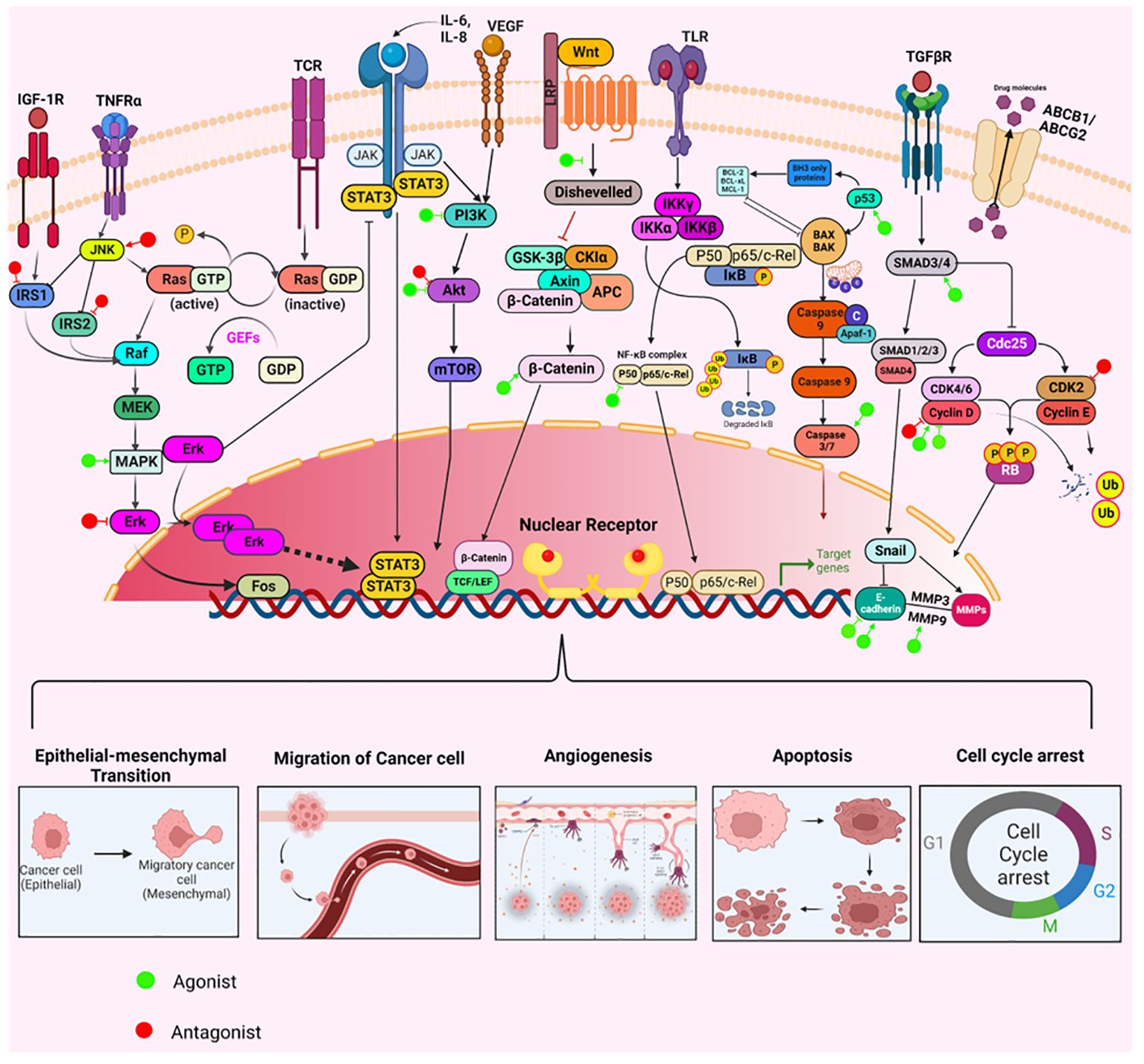
Figure 3 The mechanisms through which agonists or antagonists of various nuclear receptors influence different cellular processes regulating ovarian cancer pathogenesis. These compounds modulate key cellular behaviors like survival, proliferation, epithelial-mesenchymal transition (EMT), invasion, angiogenesis, migration, apoptosis, and cell cycle arrest. This modulation occurs through the upregulation or downregulation of specific pathways integral to the development and progression of ovarian cancer.
2.1 Androgen receptor
AR, also known as nuclear receptor subfamily 3, group C, gene 4 (NR3C4), located at Xq12, has been extensively studied as a TF that is dependent on ligand binding and is classified as a member of the NR superfamily (187–189). AR expression can be found in the regions of the body like the breast, cervix, endometrium, epididymis, fallopian tube, kidney, seminal vesicle, and testis (190).
Accumulating studies have revealed that AR expression has been upregulated, and androgen/AR signaling stimulated tumor growth and metastasis in OVC (86, 88, 191, 192). For instance, several studies in the past few decades have shown the effects of dihydrotestosterone (DHT), a synthetic androgen, in promoting AR translocation into the nucleus and increasing its expression in both OVC cells and in vivo models (76, 78–81). DHT acts as an agonist for AR, thereby inducing the proliferation and invasiveness of various OVC cells (78, 81). However, an intriguing study by Gogoi and colleagues showed that DHT and medroxyprogesterone acetate, an androgenic ligand, act by upregulating the inflammatory cytokines, IL-6 and IL-8 and downregulating the matrix metalloproteinases, MMP-2 and MMP-9 in OVC cells (79). Similarly, in another study, DHT increased cell proliferation in SKOV3 cells by elevating the IL-6 and IL-8 levels, which was reversed by treatment with an AR antagonist, flutamide (78). Further, the addition of R1881 (a synthetic anabolic androgenic steroid) caused cell growth and invasiveness by increasing the AR expression (82). It was observed that treatment with strong antagonists of AR, such as flutamide, OH-flutamide, anandron, and 3-β-hydroxysteroid dehydrogenase inhibitor, epostane reduced the survival and proliferation of OV166, OVC NOVA, and OV1225 cells adapted to DHT (75). Besides, OVCAR-3 and OSE2 cells, when treated with Casodex (bicalutamide), a potent anti-androgenic drug, inhibited cell proliferation and related genes by reducing AR level in the nucleus and resulted in cleavage of poly (ADP)-ribose polymerase (PARP) (80).
Taxol/paclitaxel, a mitotoxin, is well known for its growth-inhibitory properties of many cancers, including OVC. Yet, in patients, the effectiveness of this treatment is constrained by the acquisition of taxol resistance (txr). Kohli et al. reported that txr in OVC results from the upregulation of the linker histone H1.0 and the ABCB1 and ABCG2 drug transporters, followed by the recruitment of GCN5 and AR (88). Besides, Sun et al. demonstrated that FK506-binding protein 5 (FKBP5) was significantly upregulated in txr SKOV3 cells and knockdown of this protein resulted in increased sensitivity to taxol by downregulating several txr-related genes, including ABCB1, BMP5, FAT3, FGFR2, H1F0, SRCRB4D, STAG3, and TMPRSS15. Moreover, this study showed that FKBP5 forms a protein complex with AR which modulated the sensitivity of OVC cells towards taxol (82). Furthermore, the same group demonstrated that silencing of AR in txr OVC cells, resulted in increased apoptotic rate and decreased expression of AR mRNA and several multidrug resistance genes (83). In addition, they also found that suppression of ABCB1 gene helps in sensitizing txr OVC cells to paclitaxel or bicalutamide treatment (84). Another study demonstrated that AR expression and its nuclear translocation are influenced by the suppression of an AR molecular chaperone called small glutamine-rich tetratricopeptide repeat-containing protein alpha (SGTA) (81). Chung and colleagues demonstrated that overexpression of AR enhanced the cell growth, survival, and expression of the ABCG2 gene. This study also confirmed that the combinatorial treatment of paclitaxel with ASC-J9, an AR-degradation enhancer markedly reduced colony formation and survival of OVC cells (85).
Another study revealed the role of AR in promoting the growth of ovarian teratocarcinoma cells. AR overexpression improved the self-renewal capacity of cancer stem/progenitor cells (CSPCs). It was worth noting that the addition of DHT did not significantly affect cell growth or morphology, suggesting that these activities were carried out in a ligand-independent manner (57). Another study revealed the crosstalk between two NR signaling pathways where OVCAR-3 cells were treated with DHT and 1, 25-dihydroxy vitamin D3 [1,25(OH)2D3], which is an agonist of VDR. Notably, AR expression was enhanced by 1,25(OH)2D3 treatment but led to a reduction of growth in these cells (77). Another study demonstrated the treatment of txr OVC cells with androgen activated toll-like receptor 4 (TLR4) thereby and AR, thereby leading to AR-based txr. Hence, targeting TLR4 pathway can be used as an efficient strategy in overcoming txr in these cells (87). Collectively, the above studies illustrated that AR is upregulated in OVC, and therapeutic approaches targeting AR could be utilized for the prevention and management of this disease.
2.2 Estrogen receptor
ER, otherwise known as nuclear receptor subfamily 3 group A genes 1 (ERα) and 2 (ERβ) are positioned at 6q25.1 and 14q23.2, respectively (189, 193). ERα is predominantly present in reproductive tissues like the uterus and ovary and in the breast, kidney, bone, white adipose tissue, and liver. On the other hand, ERβ is expressed in various tissues such as the ovary, central nervous system, cardiovascular system, immune system, lung, male reproductive organs, prostate, colon, and kidney (193). The specific targeting of ERα and ERβ isoforms could be a valuable strategy for treating diseases, considering their overlapping and distinct nonoverlapping functions, as well as the tissue-specific differences in their relative abundance (187).
A plethora of research has illustrated the vital role of ER in cancer therapeutics. For instance, a number of studies have depicted that 17-β-estradiol or E2, a natural female steroid hormone, resulted in increased cell proliferation, growth, viability, and migration of various OVC cells and caused an increase in tumor growth and size in vivo through ER signaling pathway (7, 76, 89–93, 95, 97, 98, 102, 104, 108, 111). Moreover, many studies have clearly illustrated that ERα is often highly expressed in OVC and its natural ligand E2 has tumorigenic effects (55, 97, 104). Hung et al. reported that non-genomic ERα signaling increased the proliferation, invasion, and migration of immature ovarian teratoma cells by elevating the expression of microRNA-21 (125). Further, Parker et al. demonstrated that 24 genes specifically involved with ERs showed differential expression in various OVC cell lines, when treated with either E2 or genistein, a phytochemical with estrogenic potentials. This includes genes that regulate survival, invasion, migration, and other aspects critical to the development and progression of OVC (100). In addition, bisphenol A (BPA), a potent endocrine disrupting chemical (EDC) and an environmental estrogen mimic, was also shown to contribute significantly towards OVC progression via ER pathway by enhancing cell proliferation, adhesion, invasion, angiogenesis, and migration, as well as elevating cell growth-related genes (99, 110, 111, 117, 127). Further, di-n-butyl phthalate and hexabromocyclododecane, two EDCs were shown to induce estrogenic effects in OVC cells by inducing their proliferation (103). Therefore, a large number of synthetic ligands collectively known as selective estrogen receptor modulators (SERMs) are being employed for the therapeutic targeting of ER (187). For example, several studies suggested that treatment with tamoxifen and its derivatives profoundly reduced cell proliferation, and tumor growth, and induced apoptosis in preclinical OVC models (89, 90, 94, 98, 101, 122, 129, 194). Interestingly, a few studies explicitly conducted on ERβ indicated that E2 treatment alone or combined with ERβ overexpression resulted in reduced cell proliferation and motility by inducing the expression of p21 and fibulin-1c and downregulating the expression of MMP-2 and cyclin A2 (131, 132). Verardi et al. reported that an indole derivative interacted with ERβ and resulted in the suppression of growth of OVC cells (136). Further, another study showed that overexpression of ERβ5 promotes migration, invasion, and proliferation of OVC cells through FAK/c-Src activation establishing it as potential target for OVC treatment (134).
In addition, another study demonstrated that genistein and daidzein, two isoflavones from soy foods also exhibited anti-estrogenic effects in numerous OVC cells by inducing apoptosis, cell cycle arrest, and reducing cancer cell viability, sphere formation, invasion, and migration (96, 113). Besides, another study utilized two ERβ agonists, liquiritigenin, and S-equol, which is isolated from soy isoflavone daidzein, where both the agonists suppressed invasion and migration and induced apoptosis in preclinical models of OVC (133). Further, these compounds were reported to downregulate PI3K/Akt/Wnt signaling pathways in OVC cell lines such as SKOV3, NIH: OVCAR-3, CAOV3, and A2780 (96). Another study demonstrated that aconitine, a toxin from Aconitum plant inhibited the proliferation, motility, and colony formation along with inducing apoptosis and DNA damage of OVC cells (135). A few studies demonstrated that leptin promoted cell growth and invasion of SKOV3 cells by inducing estrogen and ERα expression. Nevertheless, treatment with selective ERα antagonist methyl-piperidine-pyrazole (MPP) substantially suppressed the growth of these cells (121, 128). Likewise, a couple of studies have reported the anti-carcinogenic effects of MPP in ERα-expressing OVC cells (106, 117). Moreover, another study showed that 3-butoxy-1,8,9-trihydroxy-6H-benzofuro [3,2-c]benzopyran-6-one (BTB), derived from wedelolactone, selectively blocked the E2-induced transactivation of ERs and hindered the growth of ER-positive OVC cells (107).
A plethora of preclinical studies provided evidence that anti-estrogen ICI 182, 780 or fulvestrant caused a significant decrease in OVC growth and metastasis by degrading ER and inducing apoptosis and cell cycle arrest (7, 94, 97, 99, 105, 108, 110, 114, 121, 122, 128). Many studies revealed that benzophenone-1 or 4-tert-octylphenol mimicked estrogenic effects by enhancing cell migration and the expression of proteins like N-cadherin, Snail, and Slug along with downregulating E-cadherin via ER-dependent manner (112, 124, 130). An interesting study reported that cadmium has strong estrogenic effects and it activated ER, which was reversed by the addition of ICI 182, 780 wherein it inhibited cell growth and both ERα and ERβ expression (115). Another study showed that suramin, an anticancer agent resulted in an unexpected increase in the growth of ER-positive OVC cells, thereby providing insights into the complex pathways through which ER modulates OVC progression (120). A number of studies have exploited the ERα specific agonistic action of 1, 3, 5-Tris (4-hydroxyphenyl)-4-propyl-1H-pyrazole (PPT) in upregulating the ERα expression, cell growth and migration in many OVC cell lines. Nonetheless, these effects could be potentially reversed by a strong antagonist of this receptor (99, 106, 123).
Another study portrayed the effects of pesticides, which are found in trace amounts in harvested fruits and vegetables on the development of OVC. This study showed that fenhexamid and cyprodinil, two potential fungicides, apparently increased cell proliferation and metastasis in BG-1 OVC cell line and increased tumor volume in mice models. However, treatment with the anti-estrogen, ICI 182, 780 suppressed cell proliferation by downregulating cathepsin D, PCNA, cyclin D, and E (109). In another study, the synergistic effect of trametinib and tamoxifen was explored in OVCAR-3 and SKOV3 cell lines previously treated with estrogen. It was found that treatment led to no significant impact on the growth of these cells. However, the treatment with trametinib or erlotinib alone showed a substantial reduction in cell growth (116). In addition, another study reported that micro RNA-26b suppressed the proliferation, invasion and migration of OVC cells by inhibiting ERα (118). Moreover, few studies have demonstrated that siRNA-mediated repression of ERα caused reduction in cell growth of various OVC cell lines and subsequent treatments with a potent ERα antagonist had enhanced anticancer effects (99, 127, 128). Interestingly, overexpression of ERβ in numerous lines of OVC cells, potentially reduced cell growth and migration and increased apoptosis (99, 131). Another study showed that the ERβ agonist, LY500307, inhibited growth, sphere formation, and self-renewal of ovarian cancer stem cells, while also inducing apoptosis and cell cycle arrest in these cells (137). Besides, another study revealed that OSU-ERb-12, an ERβ agonist suppressed OVC progression and inhibited cancer stem cell (CSC) subpopulation by preventing non-CSC to CSC conversion (119). Similarly, the treatment of OVC cell lines with the ERβ-specific antagonist 2,3-bis (4-hydroxy-phenyl)-propionitrile or DPN, also resulted in decreased growth and enhanced apoptosis (99, 106). Another study demonstrated semaphorin 4D expression, positively correlated with OVC progression, where it was upregulated by ERα which accelerated the proliferation of OVC cells, and was downregulated by ERβ which inhibited cell multiplication (126). In essence, these studies have uncovered the distinct roles of both the isoforms of ER and their agonists and antagonists that might pave the way for the therapeutic interventions of OVC.
2.3 Estrogen-related receptor
ERR, also known as nuclear receptor 3 group B (NR3B), has three isoforms ERRα (NR3B1), ERRβ (NR3B2), and ERRγ (NR3B3), and are located at the 11q13.1, 14q24.3, and 1q41 position respectively (https://www.genenames.org/data/gene-symbol-report/#!/hgnc_id/3471, https://www.genenames.org/data/gene-symbol-report/#!/hgnc_id/3473, https://www.genenames.org/data/gene-symbol-report/#!/hgnc_id/3474) (187). ERRα is present throughout the body except in regions like the oral mucosa, ovary, smooth muscle, spleen, and vagina. ERRβ is expressed in regions like amygdala, basal ganglia, breast, cerebellum, cerebral cortex, choroid plexus, epididymis, fallopian tube, heart muscle, hippocampal formation, hypothalamus, kidney, medulla oblongata, midbrain, pons, prostate, retina, skeletal muscle, spinal cord, spleen, and stomach, testis, thalamus, thymus, tongue, and white matter. ERRγ is found in regions like the stomach, kidney, cerebral cortex, cerebellum, nasopharynx, bronchus, lung, esophagus, colon, rectum, testis, prostate, breast, heart muscle, smooth muscle, and skeletal muscle (190). ERRs exhibit a relatively similar sequence to that of ERs, particularly in the DBD regions, with a sequence identity of over 60% between ERRα and ERα (195). ERRs have been found to exert differential effects on estrogen signaling, and studies examining ERR expression in ovarian tumors have identified ERRα and ERRγ as potential prognostic markers for these cancers (196, 197). Moreover, studies have also reported that ERRα, ERRβ, and ERRγ are upregulated in OVC tissues compared to normal ovarian tissues (63, 64). An interesting study revealed that the overexpression of ERRα elevated the levels of Snail and promoted EMT whereas specific inhibition of ERRα led to the suppression of Snail, upregulation of E-cadherin expression, and reduction in stem cell properties. It was found that the miR-200 family has a role in the post-transcriptional control of Snail mediated by ERRα. Inhibition of miR-200a/b was found to counteract the downregulation of Snail caused by ERRα depletion in vitro. Further, the silencing of ERRα in vivo led to a substantial decrease in tumor burden, ascites formation, and metastatic peritoneal nodules (138). In addition, Wang et al. reported that cordycepin, an antitumor compound inhibited ERRα (a co-TF associated with mitochondrial fusion), EMT, metastasis and migration of OVC cells by repressing their mitochondrial activity (139). However, more studies in the future could lead to a better understanding of ERR action in OVC.
2.4 Glucocorticoid receptor
GR is a glucocorticoid-activated receptor protein that belongs to the superfamily 3 of the NRs. It is encoded by a single gene, NR3C1 and is positioned at 5q31–32, and contains a highly conserved sequence (187). GRs are expressed throughout the human body and can regulate the transcription of various genes involved in development, metabolism, and inflammation by binding to various glucocorticoid response elements (GRE). Their effects on cancer cells vary depending on the cell type, making their use in cancer therapy context-dependent (198). However, in OVC, this receptor shows upregulated expression and has tumorigenic potentials (58, 144).
Several lines of evidence implicated that a synthetic glucocorticoid, dexamethasone (DEX) plays a vital role in regulating cell proliferation and survival in different OVC cell lines. For instance, Yin and colleagues revealed that DEX upregulated fibronectin and MUC1 resulting in enhanced cell adhesion and survival by upregulating PI3K/Akt pathway and survival genes in SKOV3 and HO-8910 cell lines (144). Another study reported that the treatment of OVC cell lines with DEX resulted in suppression of paclitaxel-mediated apoptosis. DEX upregulated two important pro-survival genes, serum and glucocorticoid-regulated kinase 1 (SGK1), and map kinase phosphatase 1 (MKP1)/dual specificity phosphatase 1 (DUSP1) (142). Further, these effects were evident in human trials, where pharmacological doses of DEX were administered to patients undergoing chemotherapy (142). In essence, these results suggest that activation of the GR may lead to an increase in anti-apoptotic gene expression in cancer patients, thus hindering chemotherapy-induced apoptosis (142). Accordingly, similar results were observed when OVC cells were treated with DEX in combination with carboplatin and gemcitabine. Here, DEX upregulated SGK1 and MKP1/DUSP1 and significantly suppressed carboplatin or gemcitabine-induced cell death. However, the treatment with GR antagonists, mifepristone, or CORT125134 partially abrogated this effect. Further, a significant decrease in tumor volume was observed when OVC xenografts were treated with carboplatin or gemcitabine along with mifepristone (143). These results suggested that antagonists of GR enhanced the sensitivity of GR-positive OVC cells to chemotherapy-induced cell death by impeding the GR-mediated cellular survival pathways (143). Furthermore, preclinical and clinical studies revealed that the selective GR modulation via relacorilant (a selective glucocorticoid receptor modulator), when used in combination with nab-paclitaxel, may result in enhanced efficacy of chemotherapy (146). Another study demonstrated that DEX inhibited the proliferation of 3AO cells in both time and concentration-dependent manner. Additionally, treatment with DEX resulted in an increase in alkaline phosphatase (ALP) activity and a decrease in the expression of the CA125 tumor marker in 3AO cells. These findings suggested that glucocorticoids are critical regulators of 3AO cell proliferation and differentiation (140). Moreover, an in vitro study demonstrated that 3AO cells express functional GR that can be downregulated by DEX at both protein and mRNA levels (141). ORIC-101 (structure-based modified mifepristone), is a highly potent steroidal GR antagonist with reduced AR agonistic activity, making it suitable for use in AR-positive tumors. It has also shown an improved inhibition profile for CYP2C8 and CYP2C9, reducing the potential for drug-drug interactions. Unlike mifepristone, ORIC-101 can be co-administered with chemotherapeutic agents metabolized by CYP2C8, such as paclitaxel. Furthermore, ORIC-101 exhibited antitumor activity in vivo by enhancing the chemotherapy response in the GR-positive OVCAR-5 OVC xenograft model. Moreover, the safety and therapeutic potential of ORIC-101 is currently being evaluated in clinical studies (145). Taken together, these findings suggest a crucial role of GR in ovarian tumorigenesis, and effective interventions of GR at clinical levels could be a valuable therapeutic option targeting OVC.
2.5 NR1D1
Nuclear receptor subfamily 1 group D member 1 (NR1D1), also known as REV-ERBα, is a member of the nuclear hormone receptor family that plays a critical role in the regulation of various physiological processes, including metabolism, inflammation, and circadian rhythm (199, 200). Several studies over the years have uncovered the role of NR1D1 in the pathophysiology of cancer (201, 202). Wang et al. demonstrated that overexpression of NR1D1 suppressed proliferation and induced apoptosis of OVCAR-3 cells. Further, overexpression of NR1D1 remarkably downregulated JAK/STAT3 pathway by upregulating suppressor of cytokine signaling (SOCS) 3 in both OVC cell lines and xenografts (147). However, more studies are warranted to validate the tumor suppressor function of this receptor in OVC.
2.6 Nur77/TR3
Nur77, also known as NR4A1, NGFI-B, or TR3, is a member of the NR superfamily that has a role in the regulation of both the survival and death of cancerous cells (187, 203, 204). It is located at the 12q13.13 position of the chromosome (189). Nur77 is expressed throughout the body except in regions like the adipose tissue, bone marrow, liver, parathyroid gland, prostate, and spleen (190). It has been found that Nur77 signaling is dysregulated in numerous types of cancer and serves as a crucial target for cancer therapy (205). For instance, vitamin K2 treatment of PA-1 OVC cell line resulted in increased Nur77 expression in both the mitochondria and nuclei potentially implicating the induction of apoptosis mediated by vitamin K2, suggesting that this vitamin may possess therapeutic potential for the treatment of OVC (148). Another in vitro study showed that Nur77 is a crucial regulator of apoptosis and a key mediator of the response to cytotoxic chemotherapy, such as cisplatin, in OVC. Nevertheless, this study also suggested that upregulating Nur77 expression and promoting its nuclear export could represent a rational therapeutic strategy for counteracting cisplatin resistance in OVC (149). Another study reported that the expression of Nur77 is higher in ovarian tissue samples than in other tissues and the overexpression of this orphan NR is correlated with worse PFS. Besides, Nur77 expression showed heterogeneity among different high grade serous ovarian carcinoma (HGSOC) cell lines and samples and showed localization in cytoplasm and nucleus (206). However, further research is crucial to understand the functional role of Nur77 in modulating ovarian tumorigenesis.
2.7 Progesterone receptor
The PR or NR3C2 is a hormone-regulated TF located on chromosome 11 at q22 position and employs two different promoters to give rise to isoforms, PR-A and PR-B. The well-documented ligand of PR is progesterone (4-pregnene-3,20-dione) or P4 and both the ligand and its receptor play an inevitable role in the regulation of reproductive genes. Upon binding of ligand, PR dimerizes and enters the nucleus where it binds to progesterone receptor DNA-response element (PRE) and regulates the transcription of numerous genes (187, 198). Importantly, both the isoforms of PR are shown to be highly expressed in OVC issues than in normal ovaries and hence ligands/agents that target PR could act as potential therapeutic agents against this cancer (68).
Several lines of evidence have unequivocally proved that P4 binding to PR and the subsequent upregulation of this receptor have protective effects on cancer development (68, 91). Various studies have revealed that estrogen and its analogs greatly induce the expression of PR and increase the growth and proliferation of OVC cells (68, 91, 165). Besides, this hormone also induces tumor growth and volume in xenograft models. However, when OVC cells were treated with estrogen alone or combined with P4, cell proliferation, survival, and tumor growth were reduced significantly (68, 91). Another study demonstrated that tamoxifen and megestrol acetate (a progestin medication) substantially reduced tumor volume in PE04 cell xenografts (93). Another study reported that primary cell cultures derived from patients with EOC revealed that the isolated cells expressed ER and PR, with the ER positive/PR positive combination being the most prevalent. After a 72 hour culture period, both ER and PR expression levels declined. The survival rates of cells cultured in P4 appeared inversely correlated with PR expression, while reductions in 17-β-estradiol and testosterone levels in the cultures were associated with decreased cell survival. These findings suggest a significant impact of sex steroids on PR expression and the survival of ovarian epithelial tumor cells (162). Takahashi et al. demonstrated that treatment with cAMP activated the PR-B, leading to cellular senescence and suppression of tumorigenicity in SKOV (p16 and p53 null) OVC cells (163). Another study revealed that P4 safeguards OVC cells from cisplatin -induced apoptosis by activating the PI3K/Akt survival pathway and modulating the expression profiles of PGR and 1/2. Consequently, combining PI3K/Akt signaling inhibitors or a PGRMC antagonist with platinum-based chemotherapy could improve treatment outcomes and prognosis in OVC patients (164). Lima et al. showed that P4 enhanced the expression levels of a disintegrin and metalloproteinase with thrombospondin motifs (ADAMTS) in lysates from ES2 cells, and in lysates and conditioned media from NIH-OVCAR-3 cells, suggesting P4 acts via the PR to regulate ADAMTS levels (1 and 4) in OVC cells (166). Another study reported that progestins and vitamin D exhibit a synergistic effect in reducing cell viability and inducing apoptosis in OVC cells. Progestins also inhibit calcitriol (CAL)-induced CYP24A1 expression through PR-dependent mechanisms, thereby prolonging CAL activity. This combination therapy of progestins and vitamin D warrants further investigation as a potential strategy to inhibit ovarian carcinogenesis (167). Additionally, another study highlighted the critical role of P4 and the presence of PRs in reducing disease progression in endometrioid ovarian carcinoma (168). Further, HGSOC tumors exhibiting weak PR-B expression were linked to increased platinum resistance and poor survival outcomes. In preclinical settings, P4 and PR-B expression enhanced the sensitivity of HGSC cells to cisplatin by promoting cisplatin-induced apoptosis. Furthermore, preclinical evaluations demonstrated that P4 treatment could re-sensitize platinum-resistant HGSC cells to cisplatin, suggesting this approach could be clinically valuable in enhancing the effectiveness of cisplatin therapy in the treatment and management of OVC (50). Although the role of PR in OVC has been elucidated, further research is required to identify agents that could modulate the PR expression as a viable strategy in the treatment of OVC.
2.8 Peroxisome-proliferator-activator receptor
PPAR, also known as nuclear receptor 1, group 3 has three isoforms PPAR α (NR1C1), PPAR β (NR1C2), and PPARγ (NR1C3), and regulates energy metabolism (187, 207). These isoforms are located at 22q13.31, 6p21.31, and 3p25.2 positions (https://www.genenames.org/data/gene-symbol-report/#!/hgnc_id/9232; https://www.genenames.org/data/gene-symbol-report/#!/hgnc_id/9235; https://www.genenames.org/data/gene-symbol-report/#!/hgnc_id/9236). PPARα is expressed throughout the body. PPARβ expression is found in regions like the liver, prostate, heart, and skeletal muscles. PPARγ is present in the regions like appendix, cervix, colon, epididymis, esophagus, oral mucosa, placenta, rectum, stomach, tonsil, urinary bladder, adrenal gland, duodenum, gallbladder, hippocampus, lung, salivary gland, skin, small intestine, thyroid gland, vagina, adipose tissue, bone marrow, breast, bronchus, caudate, cerebellum cerebral cortex, kidney, skeletal muscle, spleen, and testis (190). In addition, PPARs are significantly expressed in the immune cells, which displayed their crucial involvement in immune cell differentiation and fate determination (207). PPARα and PPARδ play a pivotal role in regulating genes associated with substrate delivery, oxidative phosphorylation, and the maintenance of energy homeostasis (207). On the other hand, PPARγ primarily governs lipogenesis and lipid synthesis, with the highest expression levels observed in white adipose tissue (207). PPARs also differ in ligand specificity, and response to agonists and antagonists (208). PPARs have emerged as promising therapeutic targets for a wide range of diseases, such as metabolic, autoimmune, and cancers, including OVC (208–213).
Studies have reported the upregulation of PPAR in OVC compared to adjacent normal tissues (66, 67). An intriguing study reported that the treatment of oroxylin A (OA), a phytochemical, upregulated the expression of PPARγ and inhibited both the migratory capacity and viability of SKOV3 cells. Further, OA induced apoptotic cell death and restored the expression of the PGRMC1/2 family in OVC cells (159). Leng and colleagues demonstrated that low-level exposure to mono(2-ethylhexyl) phthalate (MEHP), a major toxicant from plastics activated the PI3K/Akt/NF-κB pathway in a PPARα-dependent manner, promoting OVC progression in both in vitro and in vivo (160). Several studies have reported that the treatment of PPAR modulators, such as ciglitazone, pioglitazone, troglitazone, clofibric acid, GW9662, GW6471 and rosiglitazone alone or in combination with other compounds like BPA, clioquinol, and DHA, resulted in multiple anticancer activities such as induction of apoptosis, necrosis and suppression of cell growth, viability, colony formation and glucose intake. These studies also showed that treatment of OVC cells with these compounds reduced tumor growth, inhibition of MMP-9, and COX-2 expression in pre-clinical settings in a PPAR-dependent manner (51, 67, 151–156). In addition, Hoffmann and team reported that BPA promoted the expression of apelin, which is an endogenous adipokine involved in the proliferation and migration of many cancers, in a PPARγ-dependent manner (158). However, a different study conducted by this team reported that BPA and its derivatives suppressed the expression of chemerin, another adipokine, thereby inducing the proliferation of OVC cells (51). Further, another study reported that the treatment of telmisartan, an angiotensin receptor blocker, resulted in the induction of apoptosis, activation of caspase-3, upregulation of PPARγ expression, and inhibition of cell growth and MMP-9 expression in HEY cells (157). Collectively, these studies suggest that targeting PPAR could result in several antitumorigenic effects leading to better management and treatment of OVC.
2.9 Retinoic acid receptor
RAR belongs to the nuclear receptor subfamily 1 group B of the NR superfamily, which binds to ligands such as RA and its isomers. RAR has three isoforms RARα, RARβ, and RARγ located on chromosomes, 17q21.2, 3p24.2, and 12q13.13, respectively (189, 214). RARs and RXRs are both regulated by their natural ligand retinoids, which upon binding regulate the transcription of several important genes. The dysregulation of the RA signaling pathway is suggested to be the underlying cause of a broad range of malignancies, including OVC, leukemia, skin cancer, head and neck cancer, lung cancer, breast cancer, prostate cancer, renal cell carcinoma, pancreatic cancer, liver cancer, glioblastoma, and neuroblastoma (214). RARα and RARγ are expressed throughout the body, but the expression of RARβ is constricted to regions except parathyroid gland, duodenum, liver, kidney, prostate, ovary, heart muscle, adipose tissue, lymph node, tonsil, and bone marrow (190). Treatment of OVC cell lines either alone or in combination with retinoids, and their derivatives, forskolin, 8-Cl-cAMP, 6-[-(1-Admantyl)-4-hydroxyphenyl]-2-naphthalene carboxylic acid (AHPN/CD437), N-(4-hydroxyphenyl) retinamide (4HPR or fenretinide), N-(4-methoxyphenyl) retinamide (4MPR), PD153035, and 2-(6-carboxy-2-naphthalenyl)-2-(5,6,7,8-tetrahydro-5,5,8,8-tetramethyl-2-naphthalenyl)-1,3-dithione (MM11253) inhibited different hallmarks of cancer such as growth, proliferation, colony formation, and apoptosis (56, 169–173). For instance, OVC cells when treated with the combination of RA and 8-Cl-cAMP resulted in increased RARβ expression followed by a reduction in colony formation ability by initiating fragmentation of nucleosomes resulting in apoptosis, as evidenced by an increase in the expression of cleaved caspase-3 and PARP (170). Another study demonstrated that overexpression of RARα and RXRα resulted in significant growth inhibition in both SKOV3 and CAOV3 cells when treated with RA. This study suggests that RAR/RXR profiles could be valuable in determining the potential therapeutic benefits of RA or receptor-specific retinoid derivatives in treating OVC (56). Another study showed that the treatment of OVCAR-3 cells with EGFR inhibitor PD153035 resulted in cellular inhibition and the induction of RARβ expression through the demethylation of its promoter sequences (174). In addition, siRNA-mediated knockdown of RARγ led to decreased cell proliferation and colony number in OVC cells. Further, the knockdown of RARγ in A2780 xenografts resulted in decreased tumor weight and volume along with reduced expression of RARγ, Ki-67, and PCNA (49). Another study showed that RARβ is involved in reducing the proliferation of OVC cells and increasing the sensitivity of these cells to a synthetic retinoid derivative, N-(4-hydroxyphenyl) retinamide (4HPR or fenretinide), suggesting RARs might have a tumor-suppressing effect in ovarian tumorigenesis (172). Taken together, these results suggest that further research is needed to better understand the role of RARs in OVC, which could provide a more holistic picture of the mechanistic action of this receptor in ovarian tumor biology.
2.10 Retinoid X receptor
In 1990, Mangelsdorf et al. discovered RXRs as orphan receptors, initially identified without any known ligands (215). However, the high sequence similarity between RXRs and RARs, along with their capacity to activate various genes in the presence of all-trans RA, highlighted their close association with RARs (216). Subsequently, 9-cis-RA was identified as their natural ligand (22, 215). RXRs exist in three distinct isoforms in mammals: RXRα, RXRβ, and RXRγ, also known as NR2B1, NR2B2, and NR2B3. These isoforms are encoded by separate genes located on human chromosomes 9q34.2, 6p21.32, and 1q23.3, respectively (215). Several studies have demonstrated the role of RXRs in OVC development. For instance, Holmes et al. reported that there is a higher apoptotic index in OVC cells overexpressing RXRα than in parental cells (171). In addition, another study showed that resveratrol induced apoptosis in carboplatin-resistant OVC cells by elevating the levels of RXRα and downregulating sirtunin 1 expression (175). Further, another study revealed the responsiveness of OVC cells to all-trans RA treatment depends significantly on the expression levels and activity of RARs and RXRs, particularly RARα and RXRα. This study found that modulating the expression of these receptors in resistant cell lines can restore sensitivity to RA, leading to effective therapeutic strategies for this cancer (56).
2.11 Vitamin D receptor
VDR belongs to the NR subfamily 1 group I of the nuclear receptor superfamily located on 12q13.11 (189). Upon activation with endogenous ligands, VDR modulates the expression of its target genes by heterodimerizing with RXR isoforms that translocate to the nucleus and bind to the vitamin D response elements (217).
VDR regulates different cellular processes such as cell cycle progression, proliferation, growth, survival, apoptosis, etc., upon activation with its ligands alone or in combination, especially with 1α,25-dihydroxy vitamin D3, MT19c, calcitriol, progesterone, cisplatin, paclitaxel, carboplatin, and synthetic vitamin D analogs (48, 183, 185). For instance, treatment with 1, 25VD suppressed the growth of OVCAR-3 cells and induced cell cycle arrest by inhibiting Cdc2 kinase and cyclin B1 levels. In addition, GADD45 was identified as an immediate early response gene that mediated the inhibitory effects of 1, 25VD on cell growth and cell cycle progression (176). In addition, it has been reported that vitamin D3 inhibits the growth and migration of the A2780 cells by phosphorylating MAPK and Akt proteins. Besides, it also inhibited metastatic potential and induced apoptosis in murine teratocarcinoma cells. However, this study also showed that vitamin D3 stimulated the growth of normal embryonic stem cells. Therefore, this study suggests that vitamin D3 may inhibit the proliferation of malignant cells while potentially protecting normal stem cells crucial for development and tissue regeneration (180). Another study reported that the treatment of ES2 OVC cells with a combination of calcitriol and progesterone, induced VDR expression and suppressed cell proliferation along with abating the expression of TGF-β, SMAD signaling proteins, and CYP24A1 (48). An intriguing study showed that treatment of OVC cells with a combination of vitamin D and TGF-β1 to OVC cells resulted in the reversal of TGF-β1 induced EMT by restoring the E-cadherin coupled with decreasing α-SMA, N-cadherin, slug, MMP-2, and MMP-9 expression (186). Another study showed that the treatment of SKOV3 cells with VDR antagonist, MT19c exhibited decreased cell proliferation, increased cell cycle arrest, and induced caspase-dependent apoptosis. Further, it reduced the IRS-1/2 pathway and its downstream target genes by MAPK/JNK activation (177). In addition, another study showed that MT19c specifically targets the metabolic pathways of cancer cells, particularly by inhibiting fatty acid synthase (FASN) functions and disrupting de novo lipogenesis, which is a characteristic feature of cancer cell metabolism in both in vitro and in vivo (179). Another study demonstrated that treatment with progesterone and calcitriol led to the inhibition of IκBα phosphorylation, suppression of NF-κB activation, and reduced expression of NF-κB-regulated genes that promote metastasis. These findings suggest that progesterone and calcitriol could be effective in managing ovarian tumors (178). Ji et al. demonstrated that 1α,25-dihydroxyvitamin D3 (1α,25(OH)2D3) restricts the stem cell-like properties of OVC cells by upregulating the expression of VDR, promoting the cytoplasmic β-catenin expression, and suppressing the CD44 expression (181). Another study demonstrated that 1α,25(OH)2D3 enhances radiosensitivity in a VDR-dependent manner and activates the NADPH oxidase- ROS-apoptosis pathway. This suggests that combining 1α,25(OH)2D3 with radiation therapy could enhance radiosensitivity in ovarian cells, potentially offering a novel therapeutic strategy for this disease (182). Further, it was reported that the combined treatment of calcitriol and cisplatin in SKOV3 OVC cells resulted in enhanced antiproliferative, apoptotic, and anti-angiogenic effects compared to cisplatin alone (184). Collectively, these studies indicate that targeting the VDR with its modulators induces various anticancer effects, including apoptosis, cell cycle arrest, inhibition of cell growth, and reduction of tumor growth, suggesting it might positively impact the treatment and management of OVC.
3 Clinical trials of NR-targeted drugs in OVC
Multiple clinical studies have been carried out in OVC patients for developing potential NR-based anticancer drugs. The clinical trials employing agonists/antagonists of NRs have been summarized in Table 3 (116, 142, 146, 218–232). For instance, the treatment of anti-estrogen fulvestrant to ER-positive recurrent ovarian cancer patients resulted in enhanced progression-free survival (PFS) of these patients (219). Another study revealed that the administration of everolimus and letrozole in combination resulted in 47% 12-week PFS in nine out of nineteen ER-positive HGSOC patients enrolled (220). Further, several studies engaged the treatment of recurrent/metastatic OVC patients with the aromatase inhibitor anastrozole, which resulted in increased PFS and tumoricidal activity and reduced inhibin levels, pain, and fatigue (222–225, 228). Furthermore, anastrozole and everolimus treatment resulted in complete or partial response in a significant percentage of patients with aberrations in multiple signaling pathways (232). Many clinical trials have been conducted where an aromatase inhibitor, letrozole alone or in combination with ribociclib was administered to OVC patients. As a result, the overall clinical benefit rate (CBR) and survival of patients were improved (226, 227, 229, 230). Another study reported that treating stage III or IV EOC patients with tamoxifen resulted in elevated ER levels and achieved 10% complete response and 8% partial response (221). In another study, a 28-year-old low grade OVC patient, when treated with trametinib and tamoxifen combination, increased tumor mass and cancer antigen 125 (CA125) levels. However, combinatorial treatment of trametinib and letrozole resulted in reduced tumor mass and CA125 (116). Nonetheless, another study revealed that ERβ expression led to poor survival in patients treated with carboplatin/docetaxel with or without celecoxib (231). Another study showed that treatment of OVC patients with DEX led to the increased expression of prosurvival genes such as SGK1 and MKP1/DUSP1, suggesting that high doses of glucocorticoids might reduce chemotherapy effectiveness by enhancing anti-apoptotic gene expression (142). Further, Munster et al. evaluated the combinatorial treatment of GR modulator relacorilant and paclitaxel in OVC patients which resulted in an increased response to paclitaxel with minimal toxicity (146). Cancer of the ovary abiraterone or CORAL represents the first ever AR-targeted phase II clinical trial initiated in AR-positive EOC patients. Even though the response to the abiraterone was minimal, a subset of the patients showed enhanced CBR (218). Therefore, an extensive evaluation of clinical studies and their outcomes is crucial and unavoidable for the development of effective NR-targeted drugs for the treatment of OVC. Besides, more studies are needed to understand the potential and efficacy of these drugs in clinical settings.
4 Conclusion
OVC, being one of the most dreadful diseases, kills more than two lakhs women around the globe annually. The current therapeutic regimens have led to increased drug resistance, debilitating side effects, and toxicity in patients. However, understanding the causative factors and disease etiology could help in identifying targets for the development of treatment regimens against OVC. NRs are a family of TFs that are activated by various small molecule ligands, such as hormones, and can modulate the expression of genes involved in development, metabolism, and inflammation. This comprehensive review has elaborated the role of NRs as promising therapeutic targets in OVC, where they regulate cell signaling pathways and transcription of genes associated with cancer cell survival, proliferation, EMT, invasion, angiogenesis, and migration. Further, studies involving agonists and antagonists of NRs investigated in preclinical and clinical settings, showing promising results in reducing tumor growth and promoting apoptosis have also been briefly described. For instance, anastrozole, letrozole, ribociclib, tamoxifen, bicalutamide, enzalutamide, fulvestrant, etc., have been successfully established as NR-targeted therapeutic interventions for OVC.
It is worth noting that NR-targeted therapeutic interventions for OVC present several strengths, limitations, and challenges. A notable strength of these therapies is their capacity to precisely target and modulate the activity of NRs, which are integral to the regulation of cell proliferation, apoptosis, and hormone signaling in OVC. For instance, several aforementioned studies have shown that targeting ERs can be particularly effective in ER-positive subtypes of OVC, potentially reducing tumor growth and enhancing patient outcomes. Additionally, the use of NR agonists and antagonists can increase the sensitivity of OVC cells to various chemotherapeutic agents, offering a strategic advantage in treatment protocols. It is worth noting that letrozole (Femara®), anastrozole (Arimidex®), and fulvestrant (Faslodex®) are FDA-approved drugs widely used for hormone receptor-positive metastatic breast cancers (233–235). However, they may be used off-label or in clinical trials for OVC treatment, especially when there is evidence of hormonal involvement in the tumor’s growth dynamics. Nevertheless, these therapeutic strategies also encounter significant limitations. The heterogeneity of OVC suggests that responses to these therapies can vary widely among patients, complicating the standardization of treatment approaches. Besides, the modulation of hormonal pathways can lead to potential side effects that affect a broad spectrum of bodily functions, potentially causing considerable discomfort or adverse health effects in patients. It is also noteworthy that most NR-targeted therapies demonstrate effectiveness primarily in hormone-positive types of OVC.
Therefore, the identification of NR ligands and the development of selective, safe, and efficacious modulators may provide new therapeutic options for OVC. Further research into the molecular and cellular mechanisms underlying the role of NRs in OVC is needed to develop effective strategies for early detection and targeted therapies. Conclusively, as NRs have already revolutionized cancer treatment strategies in the past, they will likely continue as a major source of novel approaches to OVC therapeutics in the future.
Author contributions
AS: Conceptualization, Data curation, Investigation, Methodology, Writing – original draft. BB: Conceptualization, Methodology, Writing – original draft. MM: Conceptualization, Investigation, Writing – review & editing. MSA: Conceptualization, Investigation, Writing – review & editing. MA: Conceptualization, Investigation, Writing – review & editing. MS: Conceptualization, Investigation, Writing – review & editing. GS: Conceptualization, Writing – review & editing. ZM: Conceptualization, Funding acquisition, Investigation, Resources, Writing – review & editing. AK: Conceptualization, Funding acquisition, Investigation, Resources, Writing – review & editing.
Funding
The author(s) declare financial support was received for the research, authorship, and/or publication of this article. This work was supported by the BT/556/NE/U-Excel/2016 grant awarded to AK by the Department of Biotechnology (DBT), Government of India. This work was supported by the following research grants from the National Foreign Experts Program of China (DL2023027001L).
Acknowledgments
AS acknowledges Council of Scientific and Industrial Research (CSIR), Ministry of Education (MoE), Govt. of India for providing her the fellowship. BB (PMRF ID: 1903287) acknowledges the Prime Minister’s Research Fellowship (PMRF) program, Ministry of Education (MoE), Government of India for providing him with the fellowship. The authors extend their appreciation to the Deanship of Research and Graduate Studies at King Khalid University for funding this work through Large Research Project under grant number RGP2/581/45.
Conflict of interest
The authors declare that the research was conducted in the absence of any commercial or financial relationships that could be construed as a potential conflict of interest.
Publisher’s note
All claims expressed in this article are solely those of the authors and do not necessarily represent those of their affiliated organizations, or those of the publisher, the editors and the reviewers. Any product that may be evaluated in this article, or claim that may be made by its manufacturer, is not guaranteed or endorsed by the publisher.
References
1. Jayson GC, Kohn EC, Kitchener HC, Ledermann JA. Ovarian cancer. Lancet. (2014) 384:1376–88. doi: 10.1016/S0140-6736(13)62146-7
2. Khatoon E, Parama D, Kumar A, Alqahtani MS, Abbas M, Girisa S, et al. Targeting pd-1/pd-L1 axis as new horizon for ovarian cancer therapy. Life Sci. (2022) 306:120827. doi: 10.1016/j.lfs.2022.120827
3. Sung H, Ferlay J, Siegel RL, Laversanne M, Soerjomataram I, Jemal A, et al. Global cancer statistics 2020: globocan estimates of incidence and mortality worldwide for 36 cancers in 185 countries. CA Cancer J Clin. (2021) 71:209–49. doi: 10.3322/caac.21660
4. Akter S, Rahman MA, Hasan MN, Akhter H, Noor P, Islam R, et al. Recent advances in ovarian cancer: therapeutic strategies, potential biomarkers, and technological improvements. Cells. (2022) 11:650. doi: 10.3390/cells11040650
5. Gernier F, Ahmed-Lecheheb D, Pautier P, Floquet A, Nadeau C, Frank S, et al. “Chronic fatigue, quality of life and long-term side-effects of chemotherapy in patients treated for non-epithelial ovarian cancer: national case-control protocol study of the gineco-vivrovaire rare tumors inca french network for rare Malignant ovarian tumors”. BMC Cancer. (2021) 21:1147. doi: 10.1186/s12885-021-08864-8
6. Paffenholz SV, Salvagno C, Ho YJ, Limjoco M, Baslan T, Tian S, et al. Senescence induction dictates response to chemo- and immunotherapy in preclinical models of ovarian cancer. Proc Natl Acad Sci USA. (2022) 119(5). doi: 10.1073/pnas.2117754119
7. Benhadjeba S, Edjekouane L, Sauve K, Carmona E, Tremblay A. Feedback control of the cxcr7/cxcl11 chemokine axis by estrogen receptor alpha in ovarian cancer. Mol Oncol. (2018) 12:1689–705. doi: 10.1002/1878-0261.12362
8. Huang X, Li XY, Shan WL, Chen Y, Zhu Q, Xia BR. Targeted therapy and immunotherapy: diamonds in the rough in the treatment of epithelial ovarian cancer. Front Pharmacol. (2023) 14:1131342. doi: 10.3389/fphar.2023.1131342
9. Wen Y, Hou Y, Yi X, Sun S, Guo J, He X, et al. Ezh2 activates chk1 signaling to promote ovarian cancer chemoresistance by maintaining the properties of cancer stem cells. Theranostics. (2021) 11:1795–813. doi: 10.7150/thno.48101
10. Ahmed SA, Parama D, Daimari E, Girisa S, Banik K, Harsha C, et al. Rationalizing the therapeutic potential of apigenin against cancer. Life Sci. (2021) 267:118814. doi: 10.1016/j.lfs.2020.118814
11. Babu BH, Jayram HN, Nair MG, Ajaikumar KB, Padikkala J. Free radical scavenging, antitumor and anticarcinogenic activity of gossypin. J Exp Clin Cancer Research: CR. (2003) 22:581–9.
12. Banik K, Khatoon E, Harsha C, Rana V, Parama D, Thakur KK, et al. Wogonin and its analogs for the prevention and treatment of cancer: A systematic review. Phytother Res. (2022) 36:1854–83. doi: 10.1002/ptr.7386
13. Buhrmann C, Yazdi M, Popper B, Kunnumakkara AB, Aggarwal BB, Shakibaei M. Induction of the epithelial-to-mesenchymal transition of human colorectal cancer by human tnf-β (Lymphotoxin) and its reversal by resveratrol. Nutrients. (2019) 11:704. doi: 10.3390/nu11030704
14. Kunnumakkara AB, Sung B, Ravindran J, Diagaradjane P, Deorukhkar A, Dey S, et al. Zyflamend suppresses growth and sensitizes human pancreatic tumors to gemcitabine in an orthotopic mouse model through modulation of multiple targets. Int J Cancer. (2012) 131:E292–303. doi: 10.1002/ijc.26442
15. McEwan IJ. Nuclear receptors: one big family. Nucl Receptor Superfamily: Methods Protoc. (2009) 505:3–18.
16. Manickasamy MK, Sajeev A, BharathwajChetty B, Alqahtani MS, Abbas M, Hegde M, et al. Exploring the nexus of nuclear receptors in hematological Malignancies. Cell Mol Life Sci. (2024) 81:1–36. doi: 10.1007/s00018-023-05085-z
17. Clark RB, Bishop-Bailey D, Estrada-Hernandez T, Hla T, Puddington L, Padula SJ. The nuclear receptor pparγ and immunoregulation: pparγ Mediates inhibition of helper T cell responses. J Immunol. (2000) 164:1364–71. doi: 10.4049/jimmunol.164.3.1364
18. Gu P, Goodwin B, Chung AC-K, Xu X, Wheeler DA, Price RR, et al. Orphan nuclear receptor lrh-1 is required to maintain oct4 expression at the epiblast stage of embryonic development. Mol Cell Biol. (2005) 25:3492–505. doi: 10.1128/MCB.25.9.3492-3505.2005
19. Stergiopoulos A, Politis PK. The role of nuclear receptors in controlling the fine balance between proliferation and differentiation of neural stem cells. Arch Biochem Biophysics. (2013) 534:27–37. doi: 10.1016/j.abb.2012.09.009
20. Gangwar SK, Kumar A, Jose S, Alqahtani MS, Abbas M, Sethi G, et al. Nuclear receptors in oral cancer-emerging players in tumorigenesis. Cancer Lett. (2022) 536:215666. doi: 10.1016/j.canlet.2022.215666
21. Manickasamy MK, Jayaprakash S, Girisa S, Kumar A, Lam HY, Okina E, et al. Delineating the role of nuclear receptors in colorectal cancer, a focused review. Discover Oncol. (2024) 15:41. doi: 10.1007/s12672-023-00808-x
22. Mangelsdorf DJ, Thummel C, Beato M, Herrlich P, Schutz G, Umesono K, et al. The nuclear receptor superfamily: the second decade. Cell. (1995) 83:835–9. doi: 10.1016/0092-8674(95)90199-X
23. Sever R, Glass CK. Signaling by nuclear receptors. Cold Spring Harbor Perspect Biol. (2013) 5:a016709. doi: 10.1101/cshperspect.a016709
24. Olivares AM, Moreno-Ramos OA, Haider NB. Role of nuclear receptors in central nervous system development and associated diseases. J Exp Neurosci. (2015) 9:93–121. doi: 10.4137/JEN.S25480
25. Burley SK, Bhikadiya C, Bi C, Bittrich S, Chen L, Crichlow GV, et al. Rcsb protein data bank: powerful new tools for exploring 3d structures of biological macromolecules for basic and applied research and education in fundamental biology, biomedicine, biotechnology, bioengineering and energy sciences. Nucleic Acids Res. (2021) 49:D437–D51. doi: 10.1093/nar/gkaa1038
27. Schwabe JW, Chapman L, Finch JT, Rhodes D. The crystal structure of the estrogen receptor DNA-binding domain bound to DNA: how receptors discriminate between their response elements. Cell. (1993) 75:567–78. doi: 10.1016/0092-8674(93)90390-C
28. Seeliger D, de Groot BL. Ligand docking and binding site analysis with pymol and autodock/vina. J computer-aided Mol design. (2010) 24:417–22. doi: 10.1007/s10822-010-9352-6
29. Warnmark A, Treuter E, Gustafsson JA, Hubbard RE, Brzozowski AM, Pike AC. Interaction of transcriptional intermediary factor 2 nuclear receptor box peptides with the coactivator binding site of estrogen receptor alpha. J Biol Chem. (2002) 277:21862–8. doi: 10.1074/jbc.M200764200
30. Weikum ER, Liu X, Ortlund EA. The nuclear receptor superfamily: A structural perspective. Protein Sci. (2018) 27:1876–92. doi: 10.1002/pro.3496
31. Glass CK, Rosenfeld MG. The coregulator exchange in transcriptional functions of nuclear receptors. Genes Dev. (2000) 14:121–41. doi: 10.1101/gad.14.2.121
32. Chang MR, Lyda B, Kamenecka TM, Griffin PR. Pharmacologic repression of retinoic acid receptor–related orphan nuclear receptor Γ Is therapeutic in the collagen-induced arthritis experimental model. Arthritis Rheumatol. (2014) 66:579–88. doi: 10.1002/art.38272
33. Chen Y-T, Liao J-W, Tsai Y-C, Tsai F-J. Inhibition of DNA methyltransferase 1 increases nuclear receptor subfamily 4 group a member 1 expression and decreases blood glucose in type 2 diabetes. Oncotarget. (2016) 7:39162. doi: 10.18632/oncotarget.v7i26
34. Jayaprakash S, Hegde M, Girisa S, Alqahtani MS, Abbas M, Lee EHC, et al. Demystifying the functional role of nuclear receptors in esophageal cancer. Int J Mol Sci. (2022) 23:10952. doi: 10.3390/ijms231810952
35. Tiwari D, Ahuja N, Kumar S, Kalra R, Nanduri R, Gupta S, et al. Nuclear receptor nr1d1 alleviates asthma by abating gata3 gene expression and th2 cell differentiation. Cell Mol Life Sci. (2022) 79:308. doi: 10.1007/s00018-022-04323-0
36. BharathwajChetty B, Sajeev A, Vishwa R, Aswani BS, Alqahtani MS, Abbas M, et al. Dynamic interplay of nuclear receptors in tumor cell plasticity and drug resistance: shifting gears in Malignant transformations and applications in cancer therapeutics. Cancer Metastasis Rev. (2024) 43:321–62. doi: 10.1007/s10555-024-10171-0
37. Kim S, Lee JJ, Heo DS. Ppargamma ligands induce growth inhibition and apoptosis through P63 and P73 in human ovarian cancer cells. Biochem Biophys Res Commun. (2011) 406:389–95. doi: 10.1016/j.bbrc.2011.02.052
38. Joshi S, Guleria R, Pan J, DiPette D, Singh U. Retinoic acid receptors and tissue-transglutaminase mediate short-term effect of retinoic acid on migration and invasion of neuroblastoma sh-sy5y cells. Oncogene. (2006) 25:240–7. doi: 10.1038/sj.onc.1209027
39. Chen J-Y, Wang J-J, Lee H-C, Chi C-W, Lee C-H, Hsu Y-C. Combination of peroxisome proliferator–activated receptor gamma and retinoid X receptor agonists induces sodium/iodide symporter expression and inhibits cell growth of human thyroid cancer cells. J Chin Med Assoc. (2020) 83:923. doi: 10.1097/JCMA.0000000000000389
40. Neubauer NL, Ward EC, Patel P, Lu Z, Lee I, Blok LJ, et al. Progesterone receptor-B induction of birc3 protects endometrial cancer cells from ap1-59-mediated apoptosis. Hormones Cancer. (2011) 2:170–81. doi: 10.1007/s12672-011-0065-7
41. Gangwar SK, Kumar A, Yap KC-H, Jose S, Parama D, Sethi G, et al. Targeting nuclear receptors in lung cancer—Novel therapeutic prospects. Pharmaceuticals. (2022) 15:624. doi: 10.3390/ph15050624
42. Hegde M, Girisa S, Naliyadhara N, Kumar A, Alqahtani MS, Abbas M, et al. Natural compounds targeting nuclear receptors for effective cancer therapy. Cancer Metastasis Rev. (2022) 42:765–822. doi: 10.1007/s10555-022-10068-w
43. Girisa S, Henamayee S, Parama D, Rana V, Dutta U, Kunnumakkara AB. Targeting farnesoid X receptor (Fxr) for developing novel therapeutics against cancer. Mol biomed. (2021) 2:1–23. doi: 10.1186/s43556-021-00035-2
44. Girisa S, Rana V, Parama D, Dutta U, Kunnumakkara AB. Differential roles of farnesoid X receptor (Fxr) in modulating apoptosis in cancer cells. Adv Protein Chem Struct Biol. (2021) 126:63–90. doi: 10.1016/bs.apcsb.2021.02.006
45. Kiang DT, Frenning DH, Goldman AI, Ascensao VF, Kennedy BJ. Estrogen receptors and responses to chemotherapy and hormonal therapy in advanced breast cancer. N Engl J Med. (1978) 299:1330–4. doi: 10.1056/NEJM197812142992403
46. Alsous L, Bardaweel S. Selective estrogen receptor modulators (Serms) synergize with cisplatin, induce apoptosis and suppress cellular migration and colony formation of lung cancer cells. Anticancer Agents Med Chem. (2022) 22:1826–36. doi: 10.2174/1871520621666210908110902
47. Li S, Wang H, Hong L, Liu W, Huang F, Wang J, et al. Cryptotanshinone inhibits breast cancer cell growth by suppressing estrogen receptor signaling. Cancer Biol Ther. (2015) 16:176–84. doi: 10.4161/15384047.2014.962960
48. Paucarmayta A, Taitz H, Casablanca Y, Rodriguez GC, Maxwell GL, Darcy KM, et al. Tgf-beta signaling proteins and cyp24a1 may serve as surrogate markers for progesterone calcitriol treatment in ovarian and endometrial cancers of different histological types. Transl Cancer Res. (2019) 8:1423–37. doi: 10.21037/tcr.2019.07.36
49. Xiu L, Zhao Y, Li N, Zeng J, Liu J, Fu Y, et al. High expression of rarg accelerates ovarian cancer progression by regulating cell proliferation. Front Oncol. (2022) 12:1063031. doi: 10.3389/fonc.2022.1063031
50. Lin H, Lan KC, Ou YC, Wu CH, Kang HY, Chuang IC, et al. Highly expressed progesterone receptor B isoform increases platinum sensitivity and survival of ovarian high-grade serous carcinoma. Cancers (Basel). (2021) 13:5578. doi: 10.3390/cancers13215578
51. Hoffmann M, Rak A, Ptak A. Bisphenol a and its derivatives decrease expression of chemerin, which reverses its stimulatory action in ovarian cancer cells. Toxicol Lett. (2018) 291:61–9. doi: 10.1016/j.toxlet.2018.04.004
52. McLaughlin PM, Klar M, Zwimpfer TA, Dutilh G, Vetter M, Marth C, et al. Maintenance therapy with aromatase inhibitor in epithelial ovarian cancer (Matao): study protocol of a randomized double-blinded placebo-controlled multi-center phase iii trial. BMC Cancer. (2022) 22:1–12. doi: 10.1186/s12885-022-09555-8
53. Aghmesheh M, Edwards L, Clarke CL, Byth K, Katzenellenbogen BS, Russell PJ, et al. Expression of steroid hormone receptors in brca1-associated ovarian carcinomas. Gynecol Oncol. (2005) 97:16–25. doi: 10.1016/j.ygyno.2004.12.030
54. Kuhnel R, de Graaff J, Rao BR, Stolk JG. Androgen receptor predominance in human ovarian carcinoma. J Steroid Biochem. (1987) 26:393–7. doi: 10.1016/0022-4731(87)90106-3
55. Rutherford T, Brown WD, Sapi E, Aschkenazi S, Munoz A, Mor G. Absence of estrogen receptor-beta expression in metastatic ovarian cancer. Obstet Gynecol. (2000) 96:417–21. doi: 10.1016/s0029-7844(00)00917-0
56. Wu S, Zhang D, Zhang ZP, Soprano DR, Soprano KJ. Critical role of both retinoid nuclear receptors and retinoid-X-receptors in mediating growth inhibition of ovarian cancer cells by all-trans retinoic acid. Oncogene. (1998) 17:2839–49. doi: 10.1038/sj.onc.1202208
57. Chung WM, Chang WC, Chen L, Lin TY, Chen LC, Hung YC, et al. Ligand-independent androgen receptors promote ovarian teratocarcinoma cell growth by stimulating self-renewal of cancer stem/progenitor cells. Stem Cell Res. (2014) 13:24–35. doi: 10.1016/j.scr.2014.04.003
58. Zachos G, Varras M, Koffa M, Ergazaki M, Spandidos DA. Glucocorticoid and estrogen receptors have elevated activity in human endometrial and ovarian tumors as compared to the adjacent normal tissues and recognize sequence elements of the H-ras proto-oncogene. Jpn J Cancer Res. (1996) 87:916–22. doi: 10.1111/j.1349-7006.1996.tb02120.x
59. De Sousa Damiao R, Fujiyama Oshima CT, Stavale JN, Goncalves WJ. Analysis of the expression of estrogen receptor, progesterone receptor and chicken ovalbumin upstream promoter-transcription factor I in ovarian epithelial cancers and normal ovaries. Oncol Rep. (2007) 18:25–32. doi: 10.3892/or
60. Brandenberger AW, Tee MK, Jaffe RB. Estrogen receptor alpha (Er-alpha) and beta (Er-beta) mrnas in normal ovary, ovarian serous cystadenocarcinoma and ovarian cancer cell lines: down-regulation of er-beta in neoplastic tissues. J Clin Endocrinol Metab. (1998) 83:1025–8. doi: 10.1210/jcem.83.3.4788
61. Pujol P, Rey JM, Nirde P, Roger P, Gastaldi M, Laffargue F, et al. Differential expression of estrogen receptor-alpha and -beta messenger rnas as a potential marker of ovarian carcinogenesis. Cancer Res. (1998) 58:5367–73.
62. Hu Z, Zhu L, Tan M, Cai M, Deng L, Yu G, et al. The expression and correlation between the transcription factor foxp1 and estrogen receptors in epithelial ovarian cancer. Biochimie. (2015) 109:42–8. doi: 10.1016/j.biochi.2014.12.001
63. Schuler-Toprak S, Weber F, Skrzypczak M, Ortmann O, Treeck O. Expression of estrogen-related receptors in ovarian cancer and impact on survival. J Cancer Res Clin Oncol. (2021) 147:2555–67. doi: 10.1007/s00432-021-03673-9
64. Fujimoto J, Alam SM, Jahan I, Sato E, Sakaguchi H, Tamaya T. Clinical implication of estrogen-related receptor (Err) expression in ovarian cancers. J Steroid Biochem Mol Biol. (2007) 104:301–4. doi: 10.1016/j.jsbmb.2007.03.016
65. Chand AL, Pathirage N, Lazarus K, Chu S, Drummond AE, Fuller PJ, et al. Liver receptor homologue-1 expression in ovarian epithelial and granulosa cell tumours. Steroids. (2013) 78:700–6. doi: 10.1016/j.steroids.2013.03.001
66. Zhang GY, Ahmed N, Riley C, Oliva K, Barker G, Quinn MA, et al. Enhanced expression of peroxisome proliferator-activated receptor gamma in epithelial ovarian carcinoma. Br J Cancer. (2005) 92:113–9. doi: 10.1038/sj.bjc.6602244
67. Vignati S, Albertini V, Rinaldi A, Kwee I, Riva C, Oldrini R, et al. Cellular and molecular consequences of peroxisome proliferator-activated receptor-gamma activation in ovarian cancer cells. Neoplasia. (2006) 8:851–61. doi: 10.1593/neo.06433
68. Mukherjee K, Syed V, Ho SM. Estrogen-induced loss of progesterone receptor expression in normal and Malignant ovarian surface epithelial cells. Oncogene. (2005) 24:4388–400. doi: 10.1038/sj.onc.1208623
69. Bhagat R, Kumar SS, Vaderhobli S, Premalata CS, Pallavi VR, Ramesh G, et al. Epigenetic alteration of P16 and retinoic acid receptor beta genes in the development of epithelial ovarian carcinoma. Tumour Biol. (2014) 35:9069–78. doi: 10.1007/s13277-014-2136-1
70. Ramayya MS, Sheng M, Moroz K, Hill SM, Rowan BG. Human steroidogenic factor-1 (Hsf-1) regulates progesterone biosynthesis and growth of ovarian surface epithelial cancer cells. J Steroid Biochem Mol Biol. (2010) 119:14–25. doi: 10.1016/j.jsbmb.2009.11.006
71. Villena-Heinsen C, Meyberg R, Axt-Fliedner R, Reitnauer K, Reichrath J, Friedrich M. Immunohistochemical analysis of 1,25-dihydroxyvitamin-D3-receptors, estrogen and progesterone receptors and ki-67 in ovarian carcinoma. Anticancer Res. (2002) 22:2261–7.
72. Silvagno F, Poma CB, Realmuto C, Ravarino N, Ramella A, Santoro N, et al. Analysis of vitamin D receptor expression and clinical correlations in patients with ovarian cancer. Gynecol Oncol. (2010) 119:121–4. doi: 10.1016/j.ygyno.2010.06.008
73. Thill M, Fischer D, Kelling K, Hoellen F, Dittmer C, Hornemann A, et al. Expression of vitamin D receptor (Vdr), cyclooxygenase-2 (Cox-2) and 15-hydroxyprostaglandin dehydrogenase (15-pgdh) in benign and Malignant ovarian tissue and 25-hydroxycholecalciferol (25(Oh2)D3) and prostaglandin E2 (Pge2) serum level in ovarian cancer patients. J Steroid Biochem Mol Biol. (2010) 121:387–90. doi: 10.1016/j.jsbmb.2010.03.049
74. Cordes T, Hoellen F, Dittmer C, Salehin D, Kummel S, Friedrich M, et al. Correlation of prostaglandin metabolizing enzymes and serum pge2 levels with vitamin D receptor and serum 25(Oh)2d3 levels in breast and ovarian cancer. Anticancer Res. (2012) 32:351–7.
75. Slotman BJ, Rao BR. Response to inhibition of androgen action of human ovarian cancer cells in vitro. Cancer Lett. (1989) 45:213–20. doi: 10.1016/0304-3835(89)90080-3
76. Sawada M, Terada N, Wada A, Mori Y, Yamasaki M, Saga T, et al. Estrogen- and androgen-responsive growth of human ovarian adenocarcinoma heterotransplanted into nude mice. Int J Cancer. (1990) 45:359–63. doi: 10.1002/ijc.2910450225
77. Ahonen MH, Zhuang YH, Aine R, Ylikomi T, Tuohimaa P. Androgen receptor and vitamin D receptor in human ovarian cancer: growth stimulation and inhibition by ligands. Int J Cancer. (2000) 86:40–6. doi: 10.1002/(sici)1097-0215(20000401)86:1<40::aid-ijc6>3.0.co;2-e
78. Wang Y, Yang J, Gao Y, Dong LJ, Liu S, Yao Z. Reciprocal regulation of 5alpha-dihydrotestosterone, interleukin-6 and interleukin-8 during proliferation of epithelial ovarian carcinoma. Cancer Biol Ther. (2007) 6:864–71. doi: 10.4161/cbt.6.6.4093
79. Gogoi R, Kudla M, Gil O, Fishman D. The activity of medroxyprogesterone acetate, an androgenic ligand, in ovarian cancer cell invasion. Reprod Sci. (2008) 15:846–52. doi: 10.1177/1933719108323446
80. Sheach LA, Adeney EM, Kucukmetin A, Wilkinson SJ, Fisher AD, Elattar A, et al. Androgen-related expression of G-proteins in ovarian cancer. Br J Cancer. (2009) 101:498–503. doi: 10.1038/sj.bjc.6605153
81. Butler MS, Ricciardelli C, Tilley WD, Hickey TE. Androgen receptor protein levels are significantly reduced in serous ovarian carcinomas compared with benign or borderline disease but are not altered by cancer stage or metastatic progression. Horm Cancer. (2013) 4:154–64. doi: 10.1007/s12672-013-0135-0
82. Sun NK, Huang SL, Chang PY, Lu HP, Chao CC. Transcriptomic profiling of taxol-resistant ovarian cancer cells identifies fkbp5 and the androgen receptor as critical markers of chemotherapeutic response. Oncotarget. (2014) 5:11939–56. doi: 10.18632/oncotarget.2654
83. Sun NK, Huang SL, Lu HP, Chang TC, Chao CC. Integrative transcriptomics-based identification of cryptic drivers of taxol-resistance genes in ovarian carcinoma cells: analysis of the androgen receptor. Oncotarget. (2015) 6:27065–82. doi: 10.18632/oncotarget.4824
84. Sun NK, Kohli A, Huang SL, Chang TC, Chao CC. Androgen receptor transcriptional activity and chromatin modifications on the abcb1/mdr gene are critical for taxol resistance in ovarian cancer cells. J Cell Physiol. (2019) 234:8760–75. doi: 10.1002/jcp.27535
85. Chung WM, Ho YP, Chang WC, Dai YC, Chen L, Hung YC, et al. Increase paclitaxel sensitivity to better suppress serous epithelial ovarian cancer via ablating androgen receptor/aryl hydrocarbon receptor-abcg2 axis. Cancers (Basel). (2019) 11:463. doi: 10.3390/cancers11040463
86. Hill A, Cristea M, He M, Frankel P, Neuhausen S, Pal SK, et al. Androgen receptor and pi3k pathway activity in ovarian cancer. J Cancer Res Ther Oncol. (2019) 7:103. doi: 10.17303/jcrto.2019.7.103
87. Huang SL, Chang TC, Chao CCK, Sun NK. Tlr4/il-6/irf1 signaling regulates androgen receptor expression: A potential therapeutic target to overcome taxol resistance in ovarian cancer. Biochem Pharmacol. (2021) 186:114456. doi: 10.1016/j.bcp.2021.114456
88. Kohli A, Huang SL, Chang TC, Chao CC, Sun NK. H1.0 induces paclitaxel-resistance genes expression in ovarian cancer cells by recruiting gcn5 and androgen receptor. Cancer Sci. (2022) 113:2616–26. doi: 10.1111/cas.15448
89. Nash JD, Ozols RF, Smyth JF, Hamilton TC. Estrogen and anti-estrogen effects on the growth of human epithelial ovarian cancer in vitro. Obstet Gynecol. (1989) 73:1009–16. doi: 10.1097/00006250-198906000-00021
90. Langdon SP, Hawkes MM, Lawrie SS, Hawkins RA, Tesdale AL, Crew AJ, et al. Oestrogen receptor expression and the effects of oestrogen and tamoxifen on the growth of human ovarian carcinoma cell lines. Br J Cancer. (1990) 62:213–6. doi: 10.1038/bjc.1990.263
91. Ochiai K, Isonishi S, Hirama Y, Yasuda M, Terashima Y. Effect of estrogen and progesterone on the growth of human ovarian dysgerminoma heterotransplanted to athymic nude mice. Asia Oceania J Obstet Gynaecol. (1991) 17:147–58. doi: 10.1111/j.1447-0756.1991.tb00039.x
92. Galtier-Dereure F, Capony F, Maudelonde T, Rochefort H. Estradiol stimulates cell growth and secretion of procathepsin D and a 120-kilodalton protein in the human ovarian cancer cell line bg-1. J Clin Endocrinol Metab. (1992) 75:1497–502. doi: 10.1210/jcem.75.6.1464654
93. Langdon SP, Ritchie A, Young K, Crew AJ, Sweeting V, Bramley T, et al. Contrasting effects of 17 beta-estradiol on the growth of human ovarian carcinoma cells in vitro and in vivo. Int J Cancer. (1993) 55:459–64. doi: 10.1002/ijc.2910550323
94. Ercoli A, Scambia G, Fattorossi A, Raspaglio G, Battaglia A, Cicchillitti L, et al. Comparative study on the induction of cytostasis and apoptosis by ici 182,780 and tamoxifen in an estrogen receptor-negative ovarian cancer cell line. Int J Cancer. (1998) 76:47–54. doi: 10.1002/(sici)1097-0215(19980330)76:1<47::aid-ijc9>3.0.co;2-y
95. Hayashido Y, Lucas A, Rougeot C, Godyna S, Argraves WS, Rochefort H. Estradiol and fibulin-1 inhibit motility of human ovarian- and breast-cancer cells induced by fibronectin. Int J Cancer. (1998) 75:654–8. doi: 10.1002/(sici)1097-0215(19980209)75:4<654::aid-ijc26>3.0.co;2-7
96. Chen X, Anderson JJ. Isoflavones inhibit proliferation of ovarian cancer cells in vitro via an estrogen receptor-dependent pathway. Nutr Cancer. (2001) 41:165–71. doi: 10.1080/01635581.2001.9680628
97. Hall JM, Korach KS. Stromal cell-derived factor 1, a novel target of estrogen receptor action, mediates the mitogenic effects of estradiol in ovarian and breast cancer cells. Mol Endocrinol. (2003) 17:792–803. doi: 10.1210/me.2002-0438
98. Stopper H, Schmitt E, Gregor C, Mueller SO, Fischer WH. Increased cell proliferation is associated with genomic instability: elevated micronuclei frequencies in estradiol-treated human ovarian cancer cells. Mutagenesis. (2003) 18:243–7. doi: 10.1093/mutage/18.3.243
99. Park SH, Cheung LW, Wong AS, Leung PC. Estrogen regulates snail and slug in the down-regulation of E-cadherin and induces metastatic potential of ovarian cancer cells through estrogen receptor alpha. Mol Endocrinol. (2008) 22:2085–98. doi: 10.1210/me.2007-0512
100. Parker LP, Taylor DD, Kesterson S, Gercel-Taylor C. Gene expression profiling in response to estradiol and genistein in ovarian cancer cells. Cancer Genomics Proteomics. (2009) 6:189–94.
101. Shukla J, Sharma U, Kar R, Varma IK, Juyal S, Jagannathan NR, et al. Tamoxifen-2-hydroxylpropyl-beta-cyclodextrin-aggregated nanoassembly for nonbreast estrogen-receptor-positive cancer therapy. Nanomed (Lond). (2009) 4:895–902. doi: 10.2217/nnm.09.69
102. Spillman MA, Manning NG, Dye WW, Sartorius CA, Post MD, Harrell JC, et al. Tissue-specific pathways for estrogen regulation of ovarian cancer growth and metastasis. Cancer Res. (2010) 70:8927–36. doi: 10.1158/0008-5472.CAN-10-1238
103. Park MA, Hwang KA, Lee HR, Yi BR, Jeung EB, Choi KC. Cell growth of bg-1 ovarian cancer cells is promoted by di-N-butyl phthalate and hexabromocyclododecane via upregulation of the cyclin D and cyclin-dependent kinase-4 genes. Mol Med Rep. (2012) 5:761–6. doi: 10.3892/mmr.2011.712
104. Li HH, Zhao YJ, Li Y, Dai CF, Jobe SO, Yang XS, et al. Estradiol 17beta and its metabolites stimulate cell proliferation and antagonize ascorbic acid-suppressed cell proliferation in human ovarian cancer cells. Reprod Sci. (2014) 21:102–11. doi: 10.1177/1933719113492211
105. Kim JY, Yi BR, Go RE, Hwang KA, Nam KH, Choi KC. Methoxychlor and triclosan stimulates ovarian cancer growth by regulating cell cycle- and apoptosis-related genes via an estrogen receptor-dependent pathway. Environ Toxicol Pharmacol. (2014) 37:1264–74. doi: 10.1016/j.etap.2014.04.013
106. Chan KK, Leung TH, Chan DW, Wei N, Lau GT, Liu SS, et al. Targeting estrogen receptor subtypes (Eralpha and erbeta) with selective er modulators in ovarian cancer. J Endocrinol. (2014) 221:325–36. doi: 10.1530/JOE-13-0500
107. Xu D, Lin TH, Yeh CR, Cheng MA, Chen LM, Chang C, et al. The wedelolactone derivative inhibits estrogen receptor-mediated breast, endometrial, and ovarian cancer cells growth. BioMed Res Int. (2014) 2014:713263. doi: 10.1155/2014/713263
108. Maleki J, Nourbakhsh M, Shabani M, Korani M, Nourazarian SM, Ostadali Dahaghi MR, et al. 17beta-estradiol stimulates generation of reactive species oxygen and nitric oxide in ovarian adenocarcinoma cells (Ovcar 3). Iran J Cancer Prev. (2015) 8:e2332. doi: 10.17795/ijcp2332
109. Go RE, Kim CW, Choi KC. Effect of fenhexamid and cyprodinil on the expression of cell cycle- and metastasis-related genes via an estrogen receptor-dependent pathway in cellular and xenografted ovarian cancer models. Toxicol Appl Pharmacol. (2015) 289:48–57. doi: 10.1016/j.taap.2015.09.001
110. Kim YS, Hwang KA, Hyun SH, Nam KH, Lee CK, Choi KC. Bisphenol a and nonylphenol have the potential to stimulate the migration of ovarian cancer cells by inducing epithelial-mesenchymal transition via an estrogen receptor dependent pathway. Chem Res Toxicol. (2015) 28:662–71. doi: 10.1021/tx500443p
111. Ptak A, Gregoraszczuk EL. Effects of bisphenol a and 17beta-estradiol on vascular endothelial growth factor a and its receptor expression in the non-cancer and cancer ovarian cell lines. Cell Biol Toxicol. (2015) 31:187–97. doi: 10.1007/s10565-015-9303-z
112. Shin S, Go RE, Kim CW, Hwang KA, Nam KH, Choi KC. Effect of benzophenone-1 and octylphenol on the regulation of epithelial-mesenchymal transition via an estrogen receptor-dependent pathway in estrogen receptor expressing ovarian cancer cells. Food Chem Toxicol. (2016) 93:58–65. doi: 10.1016/j.fct.2016.04.026
113. Chan KKL, Siu MKY, Jiang YX, Wang JJ, Leung THY, Ngan HYS. Estrogen receptor modulators genistein, daidzein and erb-041 inhibit cell migration, invasion, proliferation and sphere formation via modulation of fak and pi3k/akt signaling in ovarian cancer. Cancer Cell Int. (2018) 18:65. doi: 10.1186/s12935-018-0559-2
114. Wang K, Zhu G, Bao S, Chen S. Long non-coding rna linc00511 mediates the effects of esr1 on proliferation and invasion of ovarian cancer through mir-424-5p and mir-370-5p. Cancer Manag Res. (2019) 11:10807–19. doi: 10.2147/CMAR.S232140
115. Ataei N, Aghaei M, Panjehpour M. Evidences for involvement of estrogen receptor induced erk1/2 activation in ovarian cancer cell proliferation by cadmium chloride. Toxicol In Vitro. (2019) 56:184–93. doi: 10.1016/j.tiv.2019.01.015
116. Kato S, McFall T, Takahashi K, Bamel K, Ikeda S, Eskander RN, et al. Kras-mutated, estrogen receptor-positive low-grade serous ovarian cancer: unraveling an exceptional response mystery. Oncologist. (2021) 26:e530–e6. doi: 10.1002/onco.13702
117. Sang C, Song Y, Jin TW, Zhang S, Fu L, Zhao Y, et al. Bisphenol a induces ovarian cancer cell proliferation and metastasis through estrogen receptor-alpha pathways. Environ Sci Pollut Res Int. (2021) 28:36060–8. doi: 10.1007/s11356-021-13267-0
118. He G, Liu X, Liu L. Microrna-26b inhibits growth and cellular invasion of ovarian cancer cells by targeting estrogen receptor alpha. 3 Biotech. (2022) 12:168. doi: 10.1007/s13205-022-03222-2
119. Banerjee A, Cai S, Xie G, Li N, Bai X, Lavudi K, et al. A novel estrogen receptor beta agonist diminishes ovarian cancer stem cells via suppressing the epithelial-to-mesenchymal transition. Cancers (Basel). (2022) 14:2331. doi: 10.3390/cancers14092311
120. Kalli KR, Bradley SV, Fuchshuber S, Conover CA. Estrogen receptor-positive human epithelial ovarian carcinoma cells respond to the antitumor drug suramin with increased proliferation: possible insight into er and epidermal growth factor signaling interactions in ovarian cancer. Gynecol Oncol. (2004) 94:705–12. doi: 10.1016/j.ygyno.2004.05.059
121. Choi JH, Lee KT, Leung PC. Estrogen receptor alpha pathway is involved in leptin-induced ovarian cancer cell growth. Carcinogenesis. (2011) 32:589–96. doi: 10.1093/carcin/bgq276
122. Badia E, Docquier A, Busson M, Lapierre M, Pujol P, Balaguer P, et al. Long-term treatment with the pure anti-estrogen fulvestrant durably remodels estrogen signaling in bg-1 ovarian cancer cells. J Steroid Biochem Mol Biol. (2012) 132:176–85. doi: 10.1016/j.jsbmb.2012.05.006
123. Simpkins F, Hevia-Paez P, Sun J, Ullmer W, Gilbert CA, da Silva T, et al. Src inhibition with saracatinib reverses fulvestrant resistance in er-positive ovarian cancer models in vitro and in vivo. Clin Cancer Res. (2012) 18:5911–23. doi: 10.1158/1078-0432.CCR-12-1257
124. Park MA, Hwang KA, Lee HR, Yi BR, Jeung EB, Choi KC. Benzophenone-1 stimulated the growth of bg-1 ovarian cancer cells by cell cycle regulation via an estrogen receptor alpha-mediated signaling pathway in cellular and xenograft mouse models. Toxicology. (2013) 305:41–8. doi: 10.1016/j.tox.2012.12.021
125. Hung YC, Chang WC, Chen LM, Chang YY, Wu LY, Chung WM, et al. Non-genomic estrogen/estrogen receptor alpha promotes cellular Malignancy of immature ovarian teratoma in vitro. J Cell Physiol. (2014) 229:752–61. doi: 10.1002/jcp.24495
126. Liu Y, Hou Y, Ma L, Sun C, Pan J, Yang Y, et al. Regulation of semaphorin 4d expression and cell proliferation of ovarian cancer by eralpha and erbeta. Braz J Med Biol Res. (2017) 50:e6057. doi: 10.1590/1414-431X20166057
127. Shi XY, Wang Z, Liu L, Feng LM, Li N, Liu S, et al. Low concentrations of bisphenol a promote human ovarian cancer cell proliferation and glycolysis-based metabolism through the estrogen receptor-alpha pathway. Chemosphere. (2017) 185:361–7. doi: 10.1016/j.chemosphere.2017.07.027
128. Ghasemi A, Saeidi J, Mohtashami M, Hashemy SI. Estrogen-independent role of eralpha in ovarian cancer progression induced by leptin/ob-rb axis. Mol Cell Biochem. (2019) 458:207–17. doi: 10.1007/s11010-019-03544-5
129. Heublein S, Page S, Mayr D, Schmoeckel E, Trillsch F, Marme F, et al. Potential interplay of the gatipotuzumab epitope ta-muc1 and estrogen receptors in ovarian cancer. Int J Mol Sci. (2019) 20:295. doi: 10.3390/ijms20020295
130. Liu X, Zhan T, Gao Y, Cui S, Liu W, Zhang C, et al. Benzophenone-1 induced aberrant proliferation and metastasis of ovarian cancer cells via activated eralpha and wnt/beta-catenin signaling pathways. Environ pollut. (2022) 292:118370. doi: 10.1016/j.envpol.2021.118370
131. Treeck O, Pfeiler G, Mitter D, Lattrich C, Piendl G, Ortmann O. Estrogen receptor beta1 exerts antitumoral effects on sk-ov-3 ovarian cancer cells. J Endocrinol. (2007) 193:421–33. doi: 10.1677/JOE-07-0087
132. Zhu J, Hua K, Sun H, Yu Y, Jin H, Feng Y. Re-expression of estrogen receptor beta inhibits the proliferation and migration of ovarian clear cell adenocarcinoma cells. Oncol Rep. (2011) 26:1497–503. doi: 10.3892/or.2011.1430
133. Liu J, Viswanadhapalli S, Garcia L, Zhou M, Nair BC, Kost E, et al. Therapeutic utility of natural estrogen receptor beta agonists on ovarian cancer. Oncotarget. (2017) 8:50002–14. doi: 10.18632/oncotarget.18442
134. Chan KKL, Siu MKY, Jiang YX, Wang JJ, Wang Y, Leung THY, et al. Differential expression of estrogen receptor subtypes and variants in ovarian cancer: effects on cell invasion, proliferation and prognosis. BMC Cancer. (2017) 17:606. doi: 10.1186/s12885-017-3601-1
135. Wang X, Lin Y, Zheng Y. Antitumor effects of aconitine in A2780 cells via estrogen receptor beta−Mediated apoptosis, DNA damage and migration. Mol Med Rep. (2020) 22:2318–28. doi: 10.3892/mmr.2020.11322
136. Verardi L, Fiori J, Andrisano V, Locatelli A, Morigi R, Naldi M, et al. Indole derivative interacts with estrogen receptor beta and inhibits human ovarian cancer cell growth. Molecules. (2020) 25:4438. doi: 10.3390/molecules25194438
137. He Y, Alejo S, Venkata PP, Johnson JD, Loeffel I, Pratap UP, et al. Therapeutic targeting of ovarian cancer stem cells using estrogen receptor beta agonist. Int J Mol Sci. (2022) 23:7159. doi: 10.3390/ijms23137159
138. Lam SS, Mak AS, Yam JW, Cheung AN, Ngan HY, Wong AS. Targeting estrogen-related receptor alpha inhibits epithelial-to-mesenchymal transition and stem cell properties of ovarian cancer cells. Mol Ther. (2014) 22:743–51. doi: 10.1038/mt.2014.1
139. Wang CW, Hsu WH, Tai CJ. Antimetastatic effects of cordycepin mediated by the inhibition of mitochondrial activity and estrogen-related receptor alpha in human ovarian carcinoma cells. Oncotarget. (2017) 8:3049–58. doi: 10.18632/oncotarget.13829
140. Xu MJ, Fang GE, Liu YJ, Song LN. Effects of glucocorticoid on proliferation, differentiation, and glucocorticoid receptor expression in human ovarian carcinoma cell line 3ao. Acta Pharmacol Sin. (2002) 23:819–23.
141. Xu M, Song L, Wang Z. Effects of dexamethasone on glucocorticoid receptor expression in a human ovarian carcinoma cell line 3ao. Chin Med J (Engl). (2003) 116:392–5.
142. Melhem A, Yamada SD, Fleming GF, Delgado B, Brickley DR, Wu W, et al. Administration of glucocorticoids to ovarian cancer patients is associated with expression of the anti-apoptotic genes sgk1 and mkp1/dusp1 in ovarian tissues. Clin Cancer Res. (2009) 15:3196–204. doi: 10.1158/1078-0432.CCR-08-2131
143. Stringer-Reasor EM, Baker GM, Skor MN, Kocherginsky M, Lengyel E, Fleming GF, et al. Glucocorticoid receptor activation inhibits chemotherapy-induced cell death in high-grade serous ovarian carcinoma. Gynecol Oncol. (2015) 138:656–62. doi: 10.1016/j.ygyno.2015.06.033
144. Yin L, Fang F, Song X, Wang Y, Huang G, Su J, et al. The pro-adhesive and pro-survival effects of glucocorticoid in human ovarian cancer cells. J Mol Endocrinol. (2016) 57:61–72. doi: 10.1530/JME-15-0142
145. Rew Y, Du X, Eksterowicz J, Zhou H, Jahchan N, Zhu L, et al. Discovery of a potent and selective steroidal glucocorticoid receptor antagonist (Oric-101). J Med Chem. (2018) 61:7767–84. doi: 10.1021/acs.jmedchem.8b00743
146. Munster PN, Greenstein AE, Fleming GF, Borazanci E, Sharma MR, Custodio JM, et al. Overcoming taxane resistance: preclinical and phase 1 studies of relacorilant, a selective glucocorticoid receptor modulator, with nab-paclitaxel in solid tumors. Clin Cancer Res. (2022) 28:3214–24. doi: 10.1158/1078-0432.CCR-21-4363
147. Wang H, Fu Y. Nr1d1 suppressed the growth of ovarian cancer by abrogating the jak/stat3 signaling pathway. BMC Cancer. (2021) 21:871. doi: 10.1186/s12885-021-08597-8
148. Sibayama-Imazu T, Fujisawa Y, Masuda Y, Aiuchi T, Nakajo S, Itabe H, et al. Induction of apoptosis in pa-1 ovarian cancer cells by vitamin K2 is associated with an increase in the level of tr3/nur77 and its accumulation in mitochondria and nuclei. J Cancer Res Clin Oncol. (2008) 134:803–12. doi: 10.1007/s00432-007-0349-z
149. Wilson AJ, Liu AY, Roland J, Adebayo OB, Fletcher SA, Slaughter JC, et al. Tr3 modulates platinum resistance in ovarian cancer. Cancer Res. (2013) 73:4758–69. doi: 10.1158/0008-5472.CAN-12-4560
150. Lei P, Abdelrahim M, Safe S. 1,1-bis(3’-indolyl)-1-(P-substituted phenyl)Methanes inhibit ovarian cancer cell growth through peroxisome proliferator-activated receptor-dependent and independent pathways. Mol Cancer Ther. (2006) 5:2324–36. doi: 10.1158/1535-7163.MCT-06-0184
151. Yokoyama Y, Xin B, Shigeto T, Umemoto M, Kasai-Sakamoto A, Futagami M, et al. Clofibric acid, a peroxisome proliferator-activated receptor alpha ligand, inhibits growth of human ovarian cancer. Mol Cancer Ther. (2007) 6:1379–86. doi: 10.1158/1535-7163.MCT-06-0722
152. Xin B, Yokoyama Y, Shigeto T, Futagami M, Mizunuma H. Inhibitory effect of meloxicam, a selective cyclooxygenase-2 inhibitor, and ciglitazone, a peroxisome proliferator-activated receptor gamma ligand, on the growth of human ovarian cancers. Cancer. (2007) 110:791–800. doi: 10.1002/cncr.22854
153. Zhang Y, Ba Y, Liu C, Sun G, Ding L, Gao S, et al. Pgc-1alpha induces apoptosis in human epithelial ovarian cancer cells through a ppargamma-dependent pathway. Cell Res. (2007) 17:363–73. doi: 10.1038/cr.2007.11
154. Shigeto T, Yokoyama Y, Xin B, Mizunuma H. Peroxisome proliferator-activated receptor alpha and gamma ligands inhibit the growth of human ovarian cancer. Oncol Rep. (2007) 18:833–40. doi: 10.3892/or
155. Tuller ER, Brock AL, Yu H, Lou JR, Benbrook DM, Ding WQ. Pparalpha signaling mediates the synergistic cytotoxicity of clioquinol and docosahexaenoic acid in human cancer cells. Biochem Pharmacol. (2009) 77:1480–6. doi: 10.1016/j.bcp.2009.02.002
156. Shin SJ, Kim JY, Kwon SY, Mun KC, Cho CH, Ha E. Ciglitazone enhances ovarian cancer cell death via inhibition of glucose transporter-1. Eur J Pharmacol. (2014) 743:17–23. doi: 10.1016/j.ejphar.2014.09.013
157. Pu Z, Zhu M, Kong F. Telmisartan prevents proliferation and promotes apoptosis of human ovarian cancer cells through upregulating ppargamma and downregulating mmp−9 expression. Mol Med Rep. (2016) 13:555–9. doi: 10.3892/mmr.2015.4512
158. Hoffmann M, Fiedor E, Ptak A. Bisphenol a and its derivatives tetrabromobisphenol a and tetrachlorobisphenol a induce apelin expression and secretion in ovarian cancer cells through a peroxisome proliferator-activated receptor gamma-dependent mechanism. Toxicol Lett. (2017) 269:15–22. doi: 10.1016/j.toxlet.2017.01.006
159. Shen JJ, Zhu XF, Xu J, Wang ZF, Gu WJ, Chen Y. Oroxylin a exerts anticancer effects on human ovarian cancer cells via the ppargamma−Dependent reversal of the progesterone receptor membrane component 1/2 expression profile. Oncol Rep. (2020) 43:1309–18. doi: 10.3892/or.2020.7509
160. Leng J, Li H, Niu Y, Chen K, Yuan X, Chen H, et al. Low-dose mono(2-ethylhexyl) phthalate promotes ovarian cancer development through pparalpha-dependent pi3k/akt/nf-kappab pathway. Sci Total Environ. (2021) 790:147990. doi: 10.1016/j.scitotenv.2021.147990
161. Langdon SP, Gabra H, Bartlett JM, Rabiaz GJ, Hawkins RA, Tesdale AL, et al. Functionality of the progesterone receptor in ovarian cancer and its regulation by estrogen. Clin Cancer Res. (1998) 4:2245–51.
162. Taube M, Hockenstrom T, Isaksson M, Lindgren PR, Backstrom T. Effects of sex steroids on survival and receptor expression in ovarian epithelial tumour cells. Int J Oncol. (2003) 22:1257–62. doi: 10.3892/ijo.22.6.1257
163. Takahashi A, Kato K, Kuboyama A, Inoue T, Tanaka Y, Kuhara A, et al. Induction of senescence by progesterone receptor-B activation in response to camp in ovarian cancer cells. Gynecol Oncol. (2009) 113:270–6. doi: 10.1016/j.ygyno.2008.12.032
164. Zhu X, Han Y, Fang Z, Wu W, Ji M, Teng F, et al. Progesterone protects ovarian cancer cells from cisplatin-induced inhibitory effects through progesterone receptor membrane component 1/2 as well as akt signaling. Oncol Rep. (2013) 30:2488–94. doi: 10.3892/or.2013.2680
165. Diep CH, Charles NJ, Gilks CB, Kalloger SE, Argenta PA, Lange CA. Progesterone receptors induce foxo1-dependent senescence in ovarian cancer cells. Cell Cycle. (2013) 12:1433–49. doi: 10.4161/cc.24550
166. Lima MA, da Silva SV, Freitas VM. Progesterone acts via the progesterone receptor to induce adamts proteases in ovarian cancer cells. J Ovarian Res. (2016) 9:9. doi: 10.1186/s13048-016-0219-x
167. Rodriguez GC, Turbov J, Rosales R, Yoo J, Hunn J, Zappia KJ, et al. Progestins inhibit calcitriol-induced cyp24a1 and synergistically inhibit ovarian cancer cell viability: an opportunity for chemoprevention. Gynecol Oncol. (2016) 143:159–67. doi: 10.1016/j.ygyno.2016.04.022
168. Pedernera E, Gomora MJ, Morales-Vasquez F, Perez-Montiel D, Mendez C. Progesterone reduces cell survival in primary cultures of endometrioid ovarian cancer. J Ovarian Res. (2019) 12:15. doi: 10.1186/s13048-019-0486-4
169. Harant H, Korschineck I, Krupitza G, Fazeny B, Dittrich C, Grunt TW. Retinoic acid receptors in retinoid responsive ovarian cancer cell lines detected by polymerase chain reaction following reverse transcription. Br J Cancer. (1993) 68:530–6. doi: 10.1038/bjc.1993.381
170. Srivastava RK, Srivastave AR, Cho-Chung YS. Synergistic effects of 8-cl-camp and retinoic acids in the inhibition of growth and induction of apoptosis in ovarian cancer cells: induction of retinoic acid receptor beta. Mol Cell Biochem. (2000) 204:1–9. doi: 10.1023/a:1007074814676
171. Holmes WF, Dawson MI, Soprano RD, Soprano KJ. Induction of apoptosis in ovarian carcinoma cells by ahpn/cd437 is mediated by retinoic acid receptors. J Cell Physiol. (2000) 185:61–7. doi: 10.1002/1097-4652(200010)185:1<61::AID-JCP5>3.0.CO;2-0
172. Pergolizzi R, Appierto V, Crosti M, Cavadini E, Cleris L, Guffanti A, et al. Role of retinoic acid receptor overexpression in sensitivity to fenretinide and tumorigenicity of human ovarian carcinoma cells. Int J Cancer. (1999) 81:829–34. doi: 10.1002/(sici)1097-0215(19990531)81:5<829::aid-ijc26>3.0.co;2-3
173. Sabichi AL, Hendricks DT, Bober MA, Birrer MJ. Retinoic acid receptor beta expression and growth inhibition of gynecologic cancer cells by the synthetic retinoid N-(4-hydroxyphenyl) retinamide. J Natl Cancer Inst. (1998) 90:597–605. doi: 10.1093/jnci/90.8.597
174. Grunt TW, Puckmair K, Tomek K, Kainz B, Gaiger A. An egf receptor inhibitor induces rar-beta expression in breast and ovarian cancer cells. Biochem Biophys Res Commun. (2005) 329:1253–9. doi: 10.1016/j.bbrc.2005.02.104
175. Chen F, Kolben T, Meister S, Czogalla B, Kolben TM, Hester A, et al. The role of resveratrol, sirtuin1 and rxralpha as prognostic markers in ovarian cancer. Arch Gynecol Obstet. (2022) 305:1559–72. doi: 10.1007/s00404-021-06262-w
176. Jiang F, Li P, Fornace AJ Jr., Nicosia SV, Bai W. G2/M arrest by 1,25-dihydroxyvitamin D3 in ovarian cancer cells mediated through the induction of gadd45 via an exonic enhancer. J Biol Chem. (2003) 278:48030–40. doi: 10.1074/jbc.M308430200
177. Brard L, Lange TS, Robison K, Kim KK, Ara T, McCallum MM, et al. Evaluation of the first ergocalciferol-derived, non hypercalcemic anti-cancer agent mt19c in ovarian cancer skov-3 cell lines. Gynecol Oncol. (2011) 123:370–8. doi: 10.1016/j.ygyno.2011.07.002
178. Kavandi L, Collier MA, Nguyen H, Syed V. Progesterone and calcitriol attenuate inflammatory cytokines cxcl1 and cxcl2 in ovarian and endometrial cancer cells. J Cell Biochem. (2012) 113:3143–52. doi: 10.1002/jcb.24191
179. Moore RG, Lange TS, Robinson K, Kim KK, Uzun A, Horan TC, et al. Efficacy of a non-hypercalcemic vitamin-D2 derived anti-cancer agent (Mt19c) and inhibition of fatty acid synthesis in an ovarian cancer xenograft model. PloS One. (2012) 7:e34443. doi: 10.1371/journal.pone.0034443
180. Abdelbaset-Ismail A, Pedziwiatr D, Suszynska E, Sluczanowska-Glabowska S, Schneider G, Kakar SS, et al. Vitamin D3 stimulates embryonic stem cells but inhibits migration and growth of ovarian cancer and teratocarcinoma cell lines. J Ovarian Res. (2016) 9:26. doi: 10.1186/s13048-016-0235-x
181. Ji M, Liu L, Hou Y, Li B. 1alpha,25−Dihydroxyvitamin D3 restrains stem cell−Like properties of ovarian cancer cells by enhancing vitamin D receptor and suppressing cd44. Oncol Rep. (2019) 41:3393–403. doi: 10.3892/or.2019.7116
182. Ji MT, Nie J, Nie XF, Hu WT, Pei HL, Wan JM, et al. 1alpha,25(Oh)(2)D(3) radiosensitizes cancer cells by activating the nadph/ros pathway. Front Pharmacol. (2020) 11:945. doi: 10.3389/fphar.2020.00945
183. Kuittinen T, Rovio P, Luukkaala T, Laurila M, Grenman S, Kallioniemi A, et al. Paclitaxel, carboplatin and 1,25-D3 inhibit proliferation of ovarian cancer cells in vitro. Anticancer Res. (2020) 40:3129–38. doi: 10.21873/anticanres.14294
184. Kim JH, Park WH, Suh DH, Kim K, No JH, Kim YB. Calcitriol combined with platinum-based chemotherapy suppresses growth and expression of vascular endothelial growth factor of skov-3 ovarian cancer cells. Anticancer Res. (2021) 41:2945–52. doi: 10.21873/anticanres.15076
185. Piatek K, Kutner A, Cacsire Castillo-Tong D, Manhardt T, Kupper N, Nowak U, et al. Vitamin D analogs regulate the vitamin D system and cell viability in ovarian cancer cells. Int J Mol Sci. (2021) 23:172. doi: 10.3390/ijms23010172
186. Kitami K, Yoshihara M, Tamauchi S, Sugiyama M, Koya Y, Yamakita Y, et al. Peritoneal restoration by repurposing vitamin D inhibits ovarian cancer dissemination via blockade of the tgf-beta1/thrombospondin-1 axis. Matrix Biol. (2022) 109:70–90. doi: 10.1016/j.matbio.2022.03.003
187. Burris TP, Solt LA, Wang Y, Crumbley C, Banerjee S, Griffett K, et al. Nuclear receptors and their selective pharmacologic modulators. Pharmacol Rev. (2013) 65:710–78. doi: 10.1124/pr.112.006833
188. Trapman J, Klaassen P, Kuiper GG, van der Korput JA, Faber PW, van Rooij HC, et al. Cloning, structure and expression of a cdna encoding the human androgen receptor. Biochem Biophys Res Commun. (1988) 153:241–8. doi: 10.1016/s0006-291x(88)81214-2
189. Seal RL, Braschi B, Gray K, Jones TE, Tweedie S, Haim-Vilmovsky L, et al. Genenames.org: the HGNC resources in 2023. Nucleic Acids Res. (2023) 51:D1003–D9. doi: 10.1093/nar/gkac888
190. Uhlen M, Fagerberg L, Hallstrom BM, Lindskog C, Oksvold P, Mardinoglu A, et al. Proteomics. Tissue-based map of the human proteome. Science. (2015) 347:1260419. doi: 10.1126/science.1260419
191. Chang C, Lee S, Yeh S, Chang T. Androgen receptor (Ar) differential roles in hormone-related tumors including prostate, bladder, kidney, lung, breast and liver. Oncogene. (2014) 33:3225–34. doi: 10.1038/onc.2013.274
192. Modugno F, Laskey R, Smith AL, Andersen CL, Haluska P, Oesterreich S. Hormone response in ovarian cancer: time to reconsider as a clinical target? Endocrine-related Cancer. (2012) 19:R255–R79. doi: 10.1530/ERC-12-0175
193. Jia M, Dahlman-Wright K, Gustafsson JA. Estrogen receptor alpha and beta in health and disease. Best Pract Res Clin Endocrinol Metab. (2015) 29:557–68. doi: 10.1016/j.beem.2015.04.008
194. Gallo MA, Kaufman D. Antagonistic and agonistic effects of tamoxifen: significance in human cancer. Semin Oncol. (1997) 24:S1–71-S1-80.
195. Horard B, Vanacker J. Estrogen receptor-related receptors: orphan receptors desperately seeking a ligand. J Mol Endocrinol. (2003) 31:349–57. doi: 10.1677/jme.0.0310349
196. Sun P, Sehouli J, Denkert C, Mustea A, Konsgen D, Koch I, et al. Expression of estrogen receptor-related receptors, a subfamily of orphan nuclear receptors, as new tumor biomarkers in ovarian cancer cells. J Mol Med (Berl). (2005) 83:457–67. doi: 10.1007/s00109-005-0639-3
197. Wu F, Wang J, Wang Y, Kwok TT, Kong SK, Wong C. Estrogen-related receptor alpha (Erralpha) inverse agonist xct-790 induces cell death in chemotherapeutic resistant cancer cells. Chem Biol Interact. (2009) 181:236–42. doi: 10.1016/j.cbi.2009.05.008
198. Zhao L, Zhou S, Gustafsson JA. Nuclear receptors: recent drug discovery for cancer therapies. Endocr Rev. (2019) 40:1207–49. doi: 10.1210/er.2018-00222
199. Cho H, Zhao X, Hatori M, Yu RT, Barish GD, Lam MT, et al. Regulation of circadian behaviour and metabolism by rev-erb-α and rev-erb-β. Nature. (2012) 485:123–7. doi: 10.1038/nature11048
200. Gibbs JE, Blaikley J, Beesley S, Matthews L, Simpson KD, Boyce SH, et al. The nuclear receptor rev-erbα Mediates circadian regulation of innate immunity through selective regulation of inflammatory cytokines. Proc Natl Acad Sci. (2012) 109:582–7. doi: 10.1073/pnas.1106750109
201. Wang X, Wang N, Wei X, Yu H, Wang Z. Rev-erbalpha reduction is associated with clinicopathological features and prognosis in human gastric cancer. Oncol Lett. (2018) 16:1499–506. doi: 10.3892/ol.2018.8809
202. Zhang H, Shu R, Liu X, Zhang X, Sun D. Downregulation of rev-erbalpha is associated with the progression of lung adenocarcinoma. Ann Transl Med. (2022) 10:56. doi: 10.21037/atm-21-6405
203. Zhang X-k. Targeting nur77 translocation. Expert Opin Ther Targets. (2007) 11:69–79. doi: 10.1517/14728222.11.1.69
204. Lith SC, de Vries CJ. Nuclear receptor nur77: its role in chronic inflammatory diseases. Essays Biochem. (2021) 65:927–39. doi: 10.1042/EBC20210004
205. To SK, Zeng J-Z, Wong AS. Nur77: A potential therapeutic target in cancer. Expert Opin Ther Targets. (2012) 16:573–85. doi: 10.1517/14728222.2012.680958
206. Delgado E, Boisen MM, Laskey R, Chen R, Song C, Sallit J, et al. High expression of orphan nuclear receptor nr4a1 in a subset of ovarian tumors with worse outcome. Gynecol Oncol. (2016) 141:348–56. doi: 10.1016/j.ygyno.2016.02.030
207. Christofides A, Konstantinidou E, Jani C, Boussiotis VA. The role of peroxisome proliferator-activated receptors (Ppar) in immune responses. Metabolism. (2021) 114:154338. doi: 10.1016/j.metabol.2020.154338
208. Wagner N, Wagner K-D. Ppar beta/delta and the hallmarks of cancer. Cells. (2020) 9:1133. doi: 10.3390/cells9051133
209. Dupont J, Reverchon M, Cloix L, Froment P, Ramé C. Involvement of adipokines, ampk, pi3k and the ppar signaling pathways in ovarian follicle development and cancer. Int J Dev Biol. (2012) 56:959–67. doi: 10.1387/ijdb.120134jd
210. Botta M, Audano M, Sahebkar A, Sirtori CR, Mitro N, Ruscica M. Ppar agonists and metabolic syndrome: an established role? Int J Mol Sci. (2018) 19:1197. doi: 10.3390/ijms19041197
211. Choi J-M, Bothwell AL. The nuclear receptor ppars as important regulators of T-cell functions and autoimmune diseases. Molecules Cells. (2012) 33:217–22. doi: 10.1007/s10059-012-2297-y
212. Gou Q, Gong X, Jin J, Shi J, Hou Y. Peroxisome proliferator-activated receptors (Ppars) are potential drug targets for cancer therapy. Oncotarget. (2017) 8:60704. doi: 10.18632/oncotarget.v8i36
213. Tachibana K, Yamasaki D, Ishimoto K. The role of ppars in cancer. PPAR Res. (2008) 2008:102737. doi: 10.1155/2008/102737
214. di Masi A, Leboffe L, De Marinis E, Pagano F, Cicconi L, Rochette-Egly C, et al. Retinoic acid receptors: from molecular mechanisms to cancer therapy. Mol aspects Med. (2015) 41:1–115. doi: 10.1016/j.mam.2014.12.003
215. Mangelsdorf DJ, Ong ES, Dyck JA, Evans RM. Nuclear receptor that identifies a novel retinoic acid response pathway. Nature. (1990) 345:224–9. doi: 10.1038/345224a0
216. Rowe A. Retinoid X receptors. Int J Biochem Cell Biol. (1997) 29:275–8. doi: 10.1016/S1357-2725(96)00101-X
217. Rochel N. Vitamin D. and its receptor from a structural perspective. Nutrients. (2022) 14:2847. doi: 10.3390/nu14142847
218. Banerjee S, Tovey H, Bowen R, Folkerd E, Kilburn L, McLachlan J, et al. Abiraterone in patients with recurrent epithelial ovarian cancer: principal results of the phase ii cancer of the ovary abiraterone (Coral) trial (Cruk - A16037). Ther Adv Med Oncol. (2020) 12:1758835920975352. doi: 10.1177/1758835920975352
219. Argenta PA, Thomas SG, Judson PL, Downs LS Jr., Geller MA, Carson LF, et al. A phase ii study of fulvestrant in the treatment of multiply-recurrent epithelial ovarian cancer. Gynecol Oncol. (2009) 113:205–9. doi: 10.1016/j.ygyno.2009.01.012
220. Colon-Otero G, Weroha SJ, Foster NR, Haluska P, Hou X, Wahner-Hendrickson AE, et al. Phase 2 trial of everolimus and letrozole in relapsed estrogen receptor-positive high-grade ovarian cancers. Gynecol Oncol. (2017) 146:64–8. doi: 10.1016/j.ygyno.2017.04.020
221. Hatch KD, Beecham JB, Blessing JA, Creasman WT. Responsiveness of patients with advanced ovarian carcinoma to tamoxifen. A gynecologic oncology group study of second-line therapy in 105 patients. Cancer. (1991) 68:269–71. doi: 10.1002/1097-0142(19910715)68:2<269::aid-cncr2820680209>3.0.co;2-o
222. Tang M, O’Connell RL, Amant F, Beale P, McNally O, Sjoquist KM, et al. Paragon: A phase ii study of anastrozole in patients with estrogen receptor-positive recurrent/metastatic low-grade ovarian cancers and serous borderline ovarian tumors. Gynecol Oncol. (2019) 154:531–8. doi: 10.1016/j.ygyno.2019.06.011
223. Kok PS, Beale P, O’Connell RL, Grant P, Bonaventura T, Scurry J, et al. Paragon (Anzgog-0903): A phase 2 study of anastrozole in asymptomatic patients with estrogen and progesterone receptor-positive recurrent ovarian cancer and ca125 progression. J Gynecol Oncol. (2019) 30:e86. doi: 10.3802/jgo.2019.30.e86
224. Banerjee SN, Tang M, O’Connell RL, Sjoquist K, Clamp AR, Millan D, et al. A phase 2 study of anastrozole in patients with oestrogen receptor and/progesterone receptor positive recurrent/metastatic granulosa cell tumours/sex-cord stromal tumours of the ovary: the paragon/anzgog 0903 trial. Gynecol Oncol. (2021) 163:72–8. doi: 10.1016/j.ygyno.2021.07.024
225. Bonaventura A, O’Connell RL, Mapagu C, Beale PJ, McNally OM, Mileshkin LR, et al. Paragon (Anzgog-0903): phase 2 study of anastrozole in women with estrogen or progesterone receptor-positive platinum-resistant or -refractory recurrent ovarian cancer. Int J Gynecol Cancer. (2017) 27:900–6. doi: 10.1097/IGC.0000000000000978
226. Colon-Otero G, Zanfagnin V, Hou X, Foster NR, Asmus EJ, Wahner Hendrickson A, et al. Phase ii trial of ribociclib and letrozole in patients with relapsed oestrogen receptor-positive ovarian or endometrial cancers. ESMO Open. (2020) 5:e000926. doi: 10.1136/esmoopen-2020-000926
227. Ramirez PT, Schmeler KM, Milam MR, Slomovitz BM, Smith JA, Kavanagh JJ, et al. Efficacy of letrozole in the treatment of recurrent platinum- and taxane-resistant high-grade cancer of the ovary or peritoneum. Gynecol Oncol. (2008) 110:56–9. doi: 10.1016/j.ygyno.2008.03.014
228. del Carmen MG, Fuller AF, Matulonis U, Horick NK, Goodman A, Duska LR, et al. Phase ii trial of anastrozole in women with asymptomatic mullerian cancer. Gynecol Oncol. (2003) 91:596–602. doi: 10.1016/j.ygyno.2003.08.021
229. Smyth JF, Gourley C, Walker G, MacKean MJ, Stevenson A, Williams AR, et al. Antiestrogen therapy is active in selected ovarian cancer cases: the use of letrozole in estrogen receptor-positive patients. Clin Cancer Res. (2007) 13:3617–22. doi: 10.1158/1078-0432.CCR-06-2878
230. Bowman A, Gabra H, Langdon SP, Lessells A, Stewart M, Young A, et al. Ca125 response is associated with estrogen receptor expression in a phase ii trial of letrozole in ovarian cancer: identification of an endocrine-sensitive subgroup. Clin Cancer Res. (2002) 8:2233–9.
231. van Kruchten M, van der Marel P, de Munck L, Hollema H, Arts H, Timmer-Bosscha H, et al. Hormone receptors as a marker of poor survival in epithelial ovarian cancer. Gynecol Oncol. (2015) 138:634–9. doi: 10.1016/j.ygyno.2015.06.032
232. Wheler JJ, Moulder SL, Naing A, Janku F, Piha-Paul SA, Falchook GS, et al. Anastrozole and everolimus in advanced gynecologic and breast Malignancies: activity and molecular alterations in the pi3k/akt/mtor pathway. Oncotarget. (2014) 5:3029–38. doi: 10.18632/oncotarget.1799
233. Bross PF, Cohen MH, Williams GA, Pazdur R. Fda drug approval summaries: fulvestrant. Oncologist. (2002) 7:477–80. doi: 10.1634/theoncologist.7-6-477
Keywords: ovarian cancer, nuclear receptors, targeted therapies, clinical trials, chemoresistance
Citation: Sajeev A, BharathwajChetty B, Manickasamy MK, Alqahtani MS, Abbas M, Shakibaei M, Sethi G, Ma Z and Kunnumakkara AB (2024) Nuclear receptors in ovarian cancer: changing paradigms in cancer therapeutics. Front. Oncol. 14:1383939. doi: 10.3389/fonc.2024.1383939
Received: 08 February 2024; Accepted: 31 May 2024;
Published: 15 July 2024.
Edited by:
Anurag Kumar Singh, Martin Luther University of Halle-Wittenberg, GermanyReviewed by:
Kwok-Ming Yao, The University of Hong Kong, Hong Kong SAR, ChinaAlka Singh, The University of Chicago, United States
Copyright © 2024 Sajeev, BharathwajChetty, Manickasamy, Alqahtani, Abbas, Shakibaei, Sethi, Ma and Kunnumakkara. This is an open-access article distributed under the terms of the Creative Commons Attribution License (CC BY). The use, distribution or reproduction in other forums is permitted, provided the original author(s) and the copyright owner(s) are credited and that the original publication in this journal is cited, in accordance with accepted academic practice. No use, distribution or reproduction is permitted which does not comply with these terms.
*Correspondence: Ajaikumar B. Kunnumakkara, kunnumakkara@iitg.ac.in; Gautam Sethi, phcgs@nus.edu.sg; Zhaowu Ma, mazw@yangtzeu.edu.cn