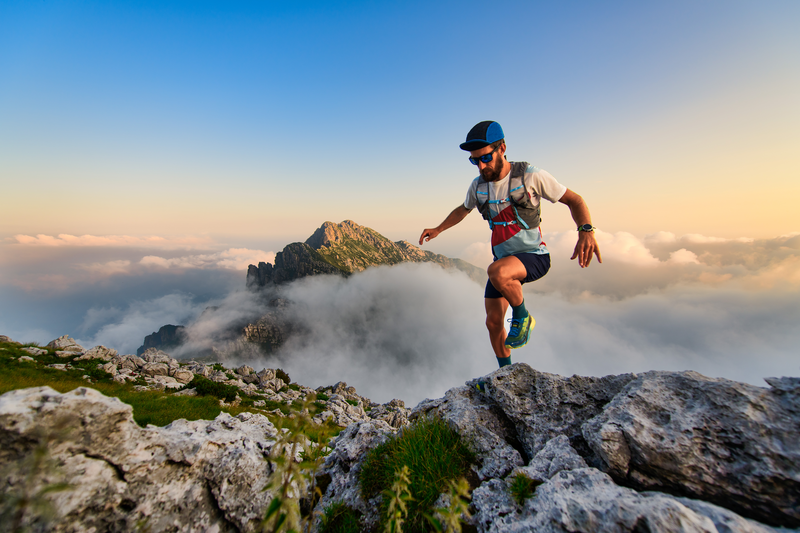
94% of researchers rate our articles as excellent or good
Learn more about the work of our research integrity team to safeguard the quality of each article we publish.
Find out more
REVIEW article
Front. Oncol. , 22 May 2024
Sec. Thoracic Oncology
Volume 14 - 2024 | https://doi.org/10.3389/fonc.2024.1380527
The detection rate of ground glass nodules (GGNs) has increased in recent years because of their malignant potential but relatively indolent biological behavior; thus, correct GGN recognition and management has become a research focus. Many scholars have explored the underlying mechanism of the indolent progression of GGNs from several perspectives, such as pathological type, genomic mutational characteristics, and immune microenvironment. GGNs have different major mutated genes at different stages of development; EGFR mutation is the most common mutation in GGNs, and p53 mutation is the most abundant mutation in the invasive stage of GGNs. Pure GGNs have fewer genomic alterations and a simpler genomic profile and exhibit a gradually evolving genomic mutation profile as the pathology progresses. Compared to advanced lung adenocarcinoma, GGN lung adenocarcinoma has a higher immune cell percentage, is under immune surveillance, and has less immune escape. However, as the pathological progression and solid component increase, negative immune regulation and immune escape increase gradually, and a suppressive immune environment is established gradually. Currently, regular computer tomography monitoring and surgery are the main treatment strategies for persistent GGNs. Stereotactic body radiotherapy and radiofrequency ablation are two local therapeutic alternatives, and systemic therapy has been progressively studied for lung cancer with GGNs. In the present review, we discuss the characterization of the multidimensional molecular evolution of GGNs that could facilitate more precise differentiation of such highly heterogeneous lesions, laying a foundation for the development of more effective individualized treatment plans.
Due to the greater use of high-resolution cross sectional imaging, an increasing number of cases with pulmonary nodules have been detected each year (1, 2). Pulmonary nodules can be classified into solid nodules (SNs), part-solid nodules (PSNs), which consist of a portion of SN and ground-glass opacity (GGO) components, or pure ground glass nodules (GGNs), according to radiographic textures. GGNs are defined as pulmonary parenchymal blurred opacity observed on CT scans that do not obscure the underlying bronchi and pulmonary vascular structures (3). They have malignant potential but relatively indolent biological behavior; therefore, their proper recognition and management have become a research focus. Administrative strategies for GGNs are mainly generated based on their radiological characteristics, such as the maximum diameter of the nodule, proportion of solid components, and growth rate (4). However, no consensus has been reached for surgical intervention timing and computed tomography (CT) monitoring frequency during conservative treatment (5–10). The clinical practice also frequently encounters cases that contradict existing guidelines (11). Different pathologic types can manifest as GGNs; in particular, minimally invasive adenocarcinoma (MIA) or invasive adenocarcinoma (IAC)-presenting GGNs are more likely to proliferate aggressively and have been the subject of numerous clinical investigations (12–17). Currently, there are not many clinical studies on GGNs alone. We reviewed the prior clinical studies on GGNs alone or including GGNs and outlined the underlying molecular characteristics and immunological features of the indolent biological behavior of GGNs to provide a theoretical foundation for more individualized clinical decision-making and management.
Pathologically, GGNs may be benign lesions (including focal interstitial fibrosis, inflammation, and hemorrhage) or malignant tumors (precancerous lesions and invasive lung adenocarcinoma [ADC]) (18).
GGNs can also be caused by inflammation, and infectious diseases are the main factors, such as mycoplasma, cytomegalovirus, and pneumocystis jirovecii pneumonia (19–21). GGNs caused by mycoplasma infection are characterized by lobular distribution, which may appear as solid micronodules or consolidations as the disease progresses (19). While GGO with cytomegalovirus could manifest as dense consolidation, bronchial wall thickening, or bronchiectasis (20), CT findings of P. jirovecii infection may present as an isolated ground glass infiltrate without other radiographic changes (21). One less common cause of GGO could be pulmonary hemorrhage, with varying etiology. In the condition, lesions can always be observed on CT scans, such as GGO and interlobular septal thickening, in addition to a ground-glass halo surrounding the pulmonary hemorrhagic foci and a patchy shadow moving down the hilum toward the periphery of pulmonary hemorrhage.
Atypical adenomatous hyperplasia (AAH) and adenocarcinoma in situ (AIS) are precancerous lesions that manifest as GGNs. AAH is defined as the focal hyperplasia of atypical cells located within the alveolar wall and respiratory bronchioles with irregular borders, consisting of alveolar type II cells and/or Clara cells (22). AAH appears as low-density pure GGO on CT images, usually less than 5 mm in size; however, some can reach 12 mm (18). AIS refers to the complete growth of ADC cells along the alveolar wall (adherent-like) without stromal, vascular, or pleural invasion, with no tumor cells in the alveolar space. On CT images, non-mucinous AIS usually presents as pure GGO, whereas mucinous AIS presents as SN or PSN (23).
MIA and IAC can also present as GGNs. MIA is observed as small solitary ADCs with tumor penetration of no more than 5 mm into the stroma and no pleural, lymphatic, or blood vessel invasions. MIA is usually non-mucinous but may be mucinous in rare cases. Non-mucinous MIA appears as a PSN in the CT scan, with the solid component being less than 5 mm (24). Mucinous MIA can be classified as SN or PSN (25). The histological subtypes of IAC are defined as a tumor invasion greater than 5 mm or invasion into the lymphatic vessels, vessels, and pleura. They can be divided into squamous, acinar, micropapillary, papillary, and solid invasive ADCs according to their histological subtypes. On CT scans, they may present as PSNs containing varying amounts of solid and ground glass. In the future, with the continuous development of artificial intelligence, imaging histology can improve the recognition of benign and malignant GGNs, thus providing more appropriate diagnosis and treatment options for patients.
AAH, AIS, MIA, and IAC are currently diagnosed based on morphological analysis. However, this method does not accurately capture the biological characteristics of GGNs. In addition, although multiple studies have investigated mutational alterations in lung cancer (26), the gene mutational characteristics for the initiation and early progression of precancerous lesions remain poorly understood. Recent findings on gene mutation signatures in ground glass nodules have been summarized in Table 1.
In the preinvasive phase, EGFR is the most common gene mutation in GGNs, followed by ERBB2, RBM10, and KRAS mutations (12–14, 27, 28, 30, 31, 33–35). The exon 21 L858R mutation is reportedly the most common EGFR mutation among GGNs, followed by exon 19 deletion mutations (30, 33). GGNs with mutations in RBM10 tend to present with a pathologically lepidic pattern. Lepidic pattern growth is characterized by tumor cell proliferation along intact alveolar walls. In addition, patients with lepidic pattern have a better prognosis than those with non-lepidic pattern tumors, suggesting that RBM10 mutations are associated with a better prognosis (13, 31). However, in some studies on lung adenocarcinoma, RBM10 acts as a tumor suppressor. When cancer is about to develop, RBM10 is mutated to reduce its inhibitory effect on c-Myc, which ultimately promotes tumor growth (36, 37). More studies may be needed to determine the role of RBM10 in the development of lung cancer. Affyl CoA synthase long chain (ACSL) plays a crucial role in the uncontrolled proliferation of cancer cells through abnormal lipid synthesis and extracellular lipid uptake (38). As the tissues change from normal to AAH and ADC, ACSL5 expression increases gradually, supporting the notion that ACSL5 contributes to tumor progression (32). In addition, FUT2 and SERINC2, which are involved in metabolism, are aberrantly expressed in precancerous lesions, aiding the identification of early-stage lung cancer (32).
EGFR, ERBB2, NRAS, and BRAF mutations contribute to the early clonal genomic events in lung ADC. In contrast, mutations in TP53 and genes associated with cell migration, gap junctions, and metastasis (such as FRG1, DOCK7, SH3BP1, and GJA1) may be late events that occur during subclonal diversification and tumor progression (12, 14). Similar proportions of EGFR mutations were observed in IAC and MIA/AIS, whereas TP53 was mutated in IAC at a much higher proportion than in MIA/AIS (12). TP53 mutation was the most abundant in the invasive phase, followed by EGFR and RB1 mutations when compared with the preinvasive and microinvasive phases. The frequency of loss of heterozygosity of human leukocyte antigen (HLA) also increased in the invasive phase (13). Conversely, the mutation frequencies of EGFR, RBM10, and TP53 in IAC cases presenting with GGNs were all significantly higher than those of AAH, AIS, and MIA (30).
The tumor mutational burden (TMB) increases gradually from AAH, AIS, and MIA to IAC (13, 30). TMB in lung adenocarcinoma (LUAD) presented as SN was significantly higher than that in LUAD with GGO components, and the proportion of GGO components was negatively correlated with TMB (35). Copy number variations (CNVs) reflect chromosomal instability, and gene gains and losses caused by CNVs can result in tumorigenesis. In a previous study, GGO displayed significantly lower leverage of arm-level CNVs and lower focal CNVs than LUAD, further indicating that GGO had fewer genomic alterations and simpler genomic profiles than LUAD (14). Li et al. discovered that malignant cells in GGNs have CNV patterns similar to those in SN, indicating that different radiological appearances are not correlated with genomic features (39). The CNV patterns of malignant cells within GGNs and solid component cells are similar. This indicates that genomic characteristics do not correspond to radiological characteristics (38). Hu et al. proposed that progression before tumor formation mainly follows the clonal clearance model, whereby partial clonal mutations discovered in early indeterminate lung nodules (IPNs) become clones in later IPNs, whereas inappropriate subclones are eliminated (29). Furthermore, somatic copy number alterations and allelic imbalance may occur during the transition from AAH to AIS and MIA to AIS, respectively (32) (Figure 1).
Figure 1 EGFR was the most frequently mutated in GGNs, followed by mutations in RBM10, TP53, STK11, and KRAS. EGFR, TP53, RBM10, and ARID1B mutation frequencies increased with SSNs with a solid component (30). TMB in lung adenocarcinoma (LUAD) presented as SN was significantly higher than that in LUAD with GGO components (35). Li et al. discovered that malignant cells in GGN have CNV patterns similar to those in SN (39). EGFR, epidermal growth factor receptor; RBM10, RNA-binding protein 10; TP53, tumor Protein 53; STK11, serine/threonine kinase 11; KRAS, Kirsten ratsarcoma viral oncogene homolog; CNV, Copy number variation; ARID1B, AT-Rich Interaction Domain 1B.
Approximately 20% of patients with GGNs (3% of the screened population) have synchronous multiple ground-glass nodules (SM-GGNs) (11). Clinical decisions regarding the treatment of SM-GGNs are complicated by the difficulty in distinguishing synchronous multiple primary lung cancers from intrapulmonary metastases. According to some studies, SM-GGNs may have a common origin, and intrapulmonary metastasis may occur even in the early stages of lung cancer (11, 31, 40). However, some studies have found that SM-GGNs mostly originate independently (28, 33). There is no genetic linkage between independently cloned SM-GGNs, and they do not have the same driver mutations, nor typical susceptibility mutations. Therefore, they occur randomly (28). SM-GGNs that were not surgically resected did not exhibit changes in size or characteristics during long-term follow-up (41). It is recommended to interpret patients with partially solid GGN lesions as surgical candidates even if they have multiple lesions (42). A good prognosis after surgical resection of SM-GGNs has been reported, supporting the strategy of considering multiple GGNs in patients as primary tumors, and resection may be an appropriate option for the subgroup (33). Most previous studies have shortcomings, such as small sample sizes, relatively low purity of tumors in GGN samples, and geographic limitations of the study population. Thus, in the future, more prospective clinical trials are needed to better understand GGNs in greater depth.
Targeting tumor cells or the tumor microenvironment (TME) are the two major fundamental principles of antitumor therapies. The TME comprises all the non-cancerous host cells in the tumor and its non-cellular components. One of the most important components of TME is the immune component. During tumor development, a variety of immune cells, including but not limited to macrophages, dendritic cells (DCs), neutrophils, B cells, and T cells, are recruited into the microenvironment surrounding tumor cells, which together with elements such as cancer associated fibroblasts (CAF), and the extracellular matrix constitute the tumor immune microenvironment (43). Accumulating evidence suggests that the tumor immune microenvironment plays an essential role in the regulation of tumor growth and metastasis. Recent findings of studies characterizing the immune microenvironment in ground glass nodules have been summarized in Table 2.
Table 2 Results of studies reporting characteristics of the immune microenvironment in ground glass nodules.
T cells are key mediators of tumor destruction, and their specificity for tumor-expressed antigens is of paramount importance. CD3+ in T lymphocyte subsets is responsible for antigen presentation on the surface of mature T cells and assists in the recognition of T cell antigen receptors. CD4+ T cells are known as helper T cells (Th cells) and act to modulate immune responses by producing cytokines to enhance or suppress inflammation (49). CD4+ T cells can be differentiated into Th1 (antiviral/antitumor), Th2 (allergic), Th17 (autoimmune and pathogen responses), and Tregs (immunosuppressive) (50–53). CD8+ T cells are known as cytotoxic T cells (TC cells) and act to kill pathogen infected or malignant cells by secreting inflammatory cytokines along with cell lytic molecules such as perforin and granzyme (54). Studies have shown that most T cells, macrophages, mast cells, and type I alveolar epithelial cells are derived from normal tissues adjacent to tumors. Most plasma, dendritic, endothelial, and B cells are usually derived from tumor tissues, suggesting that tumors can stimulate their infiltration (15).
In the immune microenvironment, the infiltration of regulatory T (Treg) cells and CD8+ cytotoxic T cells are predominant, whereas the differential Treg genes are enriched in the G2M checkpoint pathways and E2F target pathways (15, 48). In addition, the proportions of effector CD4+ T cells, cytotoxic CD8+ T cells, DCs, and natural killer cells are higher in subsolid nodules (SSNs) than in advanced adenocarcinoma. In contrast, the proportions of regulatory and depleted CD4+ T and depleted CD8+ T are lower in advanced lung adenocarcinoma. These findings suggest that immune surveillance functions well in SSNs (46). CD4+ T cells, Tregs, and DC subtypes in GGN-ADC are comparable to those in solid nodular lung adenocarcinoma (SN-ADC) but significantly higher than those in normal lung tissues. However, the cytotoxicity scores of CD8+ effector memory T cells, gzmk+CD8+ effector T cells, and the natural killer (NK) cell subtypes are reportedly significantly lower in SN-ADC than in GGN-ADC, indicating similar immunity in both radiological components but attenuated cytotoxic function in the more aggressive components (39). This may be because CD8+ T cells derived from GGN-ADC enable cells to function by activating metabolic pathways, oxidative phosphorylation, and enhanced antigen presentation and processing pathways (44). The immunosuppressive microenvironment plays a major role in the occurrence and development of tumors, and T cell-mediated immunosuppression begins in preinvasive nodules and continues to increase in intensity during invasive tumor progression. Increased Treg density and T cell exclusion from cancer nests are the major immunosuppressive mechanisms of pure non-solid (PNS) nodules (17).
T cell types and numbers vary across pathological subtypes. A gradual decrease in CD8+ T lymphocytes, B lymphocytes, NK cells, and granulocytes occurs from normal lungs to AAH, AIS, MIA, and IAC, whereas the CD4+ T lymphocytes and CD4/CD8 ratio increase significantly under such conditions, suggesting that adaptive immune responses and immune escape begin in the preneoplastic stage (15, 32, 45, 47). In addition, exhausted CD8+ T cells and Tregs increase continuously as the pathology progresses, indicating further negative immune regulation as the tumor progresses (47). Meanwhile, as tumor heterogeneity increases with the transition from AIS to IAC, CD8+ effector T cells, NK cells, and myeloid cells act as promoters, and B cells act as suppressors of tumor heterogeneity (15).
T cells are at the core of antitumor immunity in various tumors, and the T cell receptor immune repertoire reflects the strength and diversity of T cell responses; however, chromosomal instability and HLA loss inhibit T cell responses (47, 55). Dejima et al. (47) observed that T-cell clonal strength and clonality were the highest in normal lung tissues and decreased gradually in AAH, AIS, MIA, and invasive ADC. Conversely, T-cell density and diversity increased gradually. They also reported that T-cell receptor oligoclonality and the proportion of the top 10 clonal expansions in PSN-containing GGO components were indistinguishable from those in benign nodules but lower than those in SN, suggesting that T-cell clonal expansion was lower in GGNs (35).In addition, it was found that the infiltration of activated CD4+ T cells, activated CD8+ T cells, and regulatory T cells was negatively correlated with the proportion of GGO components (35).
B cells play a key role in acquired immunity. They can perform various antibody-independent functions, including engulfing and processing antigens for T cell presentation, secreting soluble mediators (such as interleukin [IL]-10), providing costimulatory signals, and participating in lymphoid tissue development (56). B cells play diverse roles in the tumor immune microenvironment. They can aggregate with T cells and dendritic cells to form tertiary lymphoid structures as an adaptive immune response to persistent tumor antigens (57). Immune escape from tumors is facilitated by regulatory B cells, which have potent immunosuppressive properties (58). GGN-ADC has significantly stronger immune responses of B cells, and antigen processing and presentation are enhanced significantly; NK cell-mediated cytotoxicity and allogeneic rejection pathways are also enhanced significantly in GGN-ADC compared to SN-ADC (44). Studies have shown that B cells in SSN exhibit inflammatory gene expression patterns, whereas B cells in advanced lung ADC are actively transcribed and have a stronger secretory phenotype (46). B cells increase gradually from normal lung tissues to ADC (32). This indicates that as the disease progresses, the number and function of B cells change further.
As the primary effector cells of the natural immune system, NK cells play an important role in controlling tumor development (59). NK cells maintain homeostasis through the expression of regulatory receptors related to their killing function. NK cells have two cell subtypes, NK-C1 and NK-C2. Compared to NK-C2, NK-C1 represents the most cytotoxic cluster. Normal lung tissues and SSN have comparable percentages of NK cells; however, NK-C1 cells in SSN have a markedly higher cytotoxic proportion than in LUAD with lymph node metastasis (46). Multiple studies have revealed that NK cell activation induces a stronger immune response in GGN-ADC than in SN-ADC, and the number of NK cells decreases gradually with pathological progression, which may promote a more aggressive PNS nodule nature and immune escape (32, 39, 44).
Macrophages are critical components of the innate immune system. Their functions include phagocytosis, antigen presentation, defense, repair, and metabolism. In GGNs, macrophages tend to polarize toward M1, suggesting a stronger proinflammatory function than in SN (39). Macrophages derived from GGO-LUAD also secrete more proinflammatory cytokines (IL-1 and TNF-α) and chemokines (CCL4), whereas proinflammatory cytokines can recruit cytotoxic T cells to attack cancer cells (44). The macrophage subtype in precancerous lesions is dominated by alveolar macrophages, whereas that in invasive ADC is dominated by tumor-associated macrophages (TAMs), which promote tumor angiogenesis, migration, and invasion and form an immunosuppressive TME (45). In a previous study, TAM exhibited significantly higher infiltration in IAC than in AIS/MIA, whereas TAM infiltration was significantly lower in GGO-LUAD than in SN-LUAD (16). The myeloid subset of AIS is dominated by mast cells, whereas MIA and IAC are dominated by macrophages.
CAFs, a heterogeneous population, are the most prominent stromal components outside tumor cells; they can secrete various cytokines to regulate the occurrence and development of tumor cells (60). Fibroblast activation protein-α (FAP) is one of the specific markers of CAFs and is generally not expressed in normal tissue cells but is highly expressed in more than 90% of malignant CAFs derived from epithelial cells; it promotes tumor cell proliferation, metastasis, and immunosuppression (61). A previous study demonstrated that GGN-ADC fibroblasts expressed lower levels of collagen than cells derived from SN-ADC, whereas the cell metabolism of the derived fibroblasts was inhibited (44). However, GGN-ADC also had a similar distribution of fibroblast subtypes as SN-ADC (39). Reproducible detection of myofibroblasts (ACTA2 and RGS5) in AAH and AIS is achievable, indicating that they may represent the TME in early-stage lung ADC (45). Xing et al. discovered that fibroblasts from SSN and normal lung tissues were enriched in immunoregulatory features; however, fibroblasts from advanced lung ADC exhibited tumor-supportive features, such as oxidative phosphorylation, angiogenesis, epithelial–mesenchymal transition, and active transcriptional pathways (46). Furthermore, tumor-related fibroblasts negatively regulate protein kinase activity and proliferation of endothelial cells (48). The preinvasive state is characterized by genetic variations that affect the extracellular matrix and fibroblasts (17).
There are also limitations to the previous research on this topic. First, most of the samples studied were not large enough, and most studies were conducted in Asian non-smoking patients, requiring international collaborative studies in other ethnicities and smokers. Second, the biological complexity of GGNs may vary owing to differences in pathology and single-cell sequencing sampling locations, as well as intra-tumor heterogeneity. In the future, studies of larger cohorts of GGNs are needed to dissect the evolutionary trajectories of GGNs and their underlying molecular mechanisms using tools designed to deal with increasingly complex biological features.
PD-L1 positivity was observed in AIS, MIA, and IAC, indicating that immunoediting was initiated before invasion (12). Qu et al. observed that PD-L1 expression levels were similar in GGO-LUAD, SN-LUAD, different pathological subtypes, and multiple primary lung ADC (16). However, a meta-analysis revealed that PD-L1 expression in GGO was significantly lower than in SN (34). Furthermore, PD-L1 expression is higher in SN nodules than in PNS nodules, suggesting that immune checkpoint adjustment occurs in aggressive nodules (17). The different findings may be due to significant heterogeneity in the methods and cutoff values used to measure PD-L1 expression. In addition, the expression level of PD-1 differs during the evolution of lung nodules. During the AAH/AIS phase, PD-1 is highly expressed in Pan CK (-) tumor cells; however, in the MIA and IAS phases, PD-1 is expressed highly in Pan CK (+) tumor cells (45). In recent years, the development of immune checkpoint blockade targeting T cells via anti-PD-1 and anti-PD-L1 has revolutionized cancer treatment and has been used to treat early-stage lung cancer and precancerous lesions (62, 63). However, further studies are needed to determine whether treatment in lung nodules with immune checkpoint inhibitors should be based on the level of PD-1 or PD-L1.
Several metabolic pathways were upregulated in SN-LUAD than in GGO-LUAD, indicating that metabolic activity increases with cancer progression (35). The loss of HLA heterozygosity in lung ADC containing GGO components is significantly lower than in those without components containing GGO (35). Hu et al. observed that the proportion of aberrant methylation and intratumoral heterogeneity increased gradually as the pathology progressed; however, increased global hypomethylation was associated with higher mutational burden, CNV, AI, and Treg/CD8 ratio, demonstrating that methylation plays an important role in chromosomal instability and the tumor immune microenvironment during the early development of lung ADC (64). Another study showed that genes in advanced lesions exhibit promoter hypermethylation, which may suppress the expression of neoantigens, leading to antigen depletion and immune escape (47). However, the overall methylation levels were negatively correlated with CD4+ T cell infiltration, CD4/CD8 ratio, Treg infiltration, and Treg/CD8, which further suggests the potential impact of global genomic and methylation statuses on the immune microenvironment (47).
As GGNs tend to develop inertly, regular CT monitoring is one of the main international treatment strategies for persistent GGNs (4). GGNs have been studied more and treated more aggressively in Southeast Asian countries (10, 65, 66). And the timing of surgical resection intervention depends mainly on the size of the GGN and the dynamic changes in imaging during follow-up (65). Stereotactic body radiotherapy (SBRT) and radiofrequency ablation are two local therapeutic alternatives, and systemic therapy has been progressively studied in lung cancer with GGNs.
Video-assisted surgery is increasingly applied to the treatment of GGNs instead of conventional thoracotomy with fewer ports and smaller incisions. Different surgical approaches need to be developed depending on the size and location of the GGNs. Although lobectomy is the standard treatment for early-stage lung cancer because the tumor biology of GGNs may differ from a previous diagnosis of lung cancer, lung segmental resection and lung wedge resection are surgical options. In patients with mixed GGNs, wedge resection should be considered with caution because of the high recurrence rate (67). If the lesion is deeper but still within one of the lung segments, then segmental resection may be considered. If the lesion is located between two or more segments or in a bronchial root segment, then lobectomy is indicated (68). In addition, intraoperative frozen pathology is suggestive of the choice of surgical approach for early-stage lung cancer with GGNs. Multiple studies have drawn similar conclusions and reported no cases of hilar and mediastinal lymph node metastases in GGO-predominant lung tumors, and mediastinal lymph node sampling/selective lymph node dissection is acceptable in patients with c-stage I non-small-cell lung cancer (NSCLC) with GGO-predominant tumors (69, 70).
When multiple GGNs are present in different locations, an appropriate surgical approach based on the principles of surgical oncology that preserves lung function as much as possible is needed (71, 72). Several studies have demonstrated a satisfactory prognosis for patients undergoing resection of multiple GGNs (71–74). Previous studies on the surgical treatment of GGNs have been mostly retrospective, and further large-sample, prospective trials are needed to determine the effect of surgical modality on the prognosis of GGNs as well as interactions between multiple GGNs to determine the optimal surgical treatment strategy.
SBRT is an alternative therapy for patients with inoperable early-stage NSCLC who are unable or unwilling to undergo surgical resection. Multiple studies have reported no significant difference in survival between patients with stage I lung cancer with GGNs who underwent surgery or SBRT (75, 76). As a precise minimally invasive technique, image-guided thermal ablation has been increasingly used to treat early−stage lung cancer (77–79). Studies have found that either radiofrequency ablation, cryoablation, or microwave ablation (MWA) are safe, minimally invasive, and have good survival rates for the treatment of lung cancer with GGNs (80–82). Huang et al. (83) retrospectively evaluated the MWA efficacy in the treatment of pulmonary multiple concurrent GGNs. The results showed that CT-guided percutaneous MWA for the treatment of multiple simultaneous pulmonary GGNs was feasible, safe, and effective. Radiofrequency ablation may be a suitable option for patients with GGNs who refuse surgery.
Systemic therapy for lung cancer with GGNs has also been reported in some retrospective studies. It was found that chemotherapy was not efficacious against lung adenocarcinoma with GGNs; therefore, chemotherapy should not be a treatment option (84, 85). In multiple primary lung adenocarcinomas, most lesions show multiple GGNs in imaging, many of which have EGFR mutations (86). Cheng et al. (87) conducted a study to determine whether postoperative oral EGFR-TKIs were effective in patients with unresected GGNs with synchronous multiple primary lung cancer (sMPLC) and EGFR mutations. The results showed that EGFR-TKIs showed limited efficacy against these unresected GGO lesions. This may be related to the inability to access the genetic status of each GGN and the heterogeneity among GGNs. Xu et al. (88) showed that the use of immune checkpoint inhibitors was efficacious and safe for patients with sMPLC, which exhibited promise as a neoadjuvant or adjuvant immunotherapy for such patients. Additional multicenter prospective randomized controlled trials are needed to determine whether immunotherapy is effective for treating GGNs.
Early lung cancer development may be associated with GGO components, and GGO-related molecular features may reflect early lung cancer progression. Clinically, GGNs exhibit indolent development and a favorable prognosis, and multiple studies have reported that GGN-ADC exhibits different molecular characteristics in genomic mutations and immune microenvironments than SN-ADC. We reviewed the prior clinical studies on GGNs alone or including GGNs and outlined the underlying molecular characteristics and immunological features of the indolent biological behavior of GGNs.
Regardless of whether the pathology is AAH, AIS, MIA, or IAC, it can manifest as GGNs on CT. GGNs have different major mutated genes at different developmental stages. In addition, pure GGNs have fewer genomic alterations and a simpler genomic profile and exhibit a progressively evolving genomic mutational profile as pathology progresses. Compared with advanced lung adenocarcinoma, GGN-ADC has a higher immune cell percentage, which is under immune surveillance and has less immune escape. However, as pathology progresses and the solid component increases, negative immune regulation, and immune escape increase gradually, and a suppressive immune environment is established gradually (16, 35, 39, 44, 47, 64).
Some studies have found malignant potential for rapid progression and even metastasis in generally inert GGNs, suggesting heterogeneity in GGNs. Currently, follow-up observation and surgery are the mainstay treatment of GGNs. SBRT and radiofrequency ablation are local therapeutic alternatives, and systemic therapy has been progressively studied in lung cancer with GGNs. Exploration of the characteristics of the molecular evolution of GGNs could provide avenues for distinguishing GGNs with different growth potentials to facilitate the selection of more effective and individualized treatment modalities. In the clinical setting, primary tumor tissue from the same patient is collected only once along the tumor progression trajectory, and serial samples are not available for longitudinal studies. However, with advancements in multiple detection methods, such as imaging omics, circulating tumor DNA, and body fluid metabolomics, it will be possible in the future to reflect the dynamic changes in the GGN genome and immune microenvironment in real-time using multiple detection methods to develop more rational treatment and follow-up protocols.
FC: Writing – original draft, Writing – review & editing. JL: Writing – original draft. LL: Writing – original draft. LT: Writing – review & editing. GW: Writing – review & editing. XZ: Writing – review & editing.
The author(s) declare financial support was received for the research, authorship, and/or publication of this article. This study was supported by the Beijing Medical Award Foundation [Grant number: YXJL-2022-0105-0039; Grant number: YXJL-2022-0665-0272; Grant number: YXJL-2023-0227-0132].
The authors declare that the research was conducted in the absence of any commercial or financial relationships that could be construed as a potential conflict of interest.
All claims expressed in this article are solely those of the authors and do not necessarily represent those of their affiliated organizations, or those of the publisher, the editors and the reviewers. Any product that may be evaluated in this article, or claim that may be made by its manufacturer, is not guaranteed or endorsed by the publisher.
1. Gould MK, Tang T, Liu IL, Lee J, Zheng C, Danforth KN, et al. Recent trends in the identification of incidental pulmonary nodules. Am J Respir Crit Care Med. (2015) 192:1208–14. doi: 10.1164/rccm.201505-0990OC
2. Hendrix W, Rutten M, Hendrix N, van Ginneken B, Schaefer-Prokop C, Scholten ET, et al. Trends in the incidence of pulmonary nodules in chest computed tomography: 10-year results from two Dutch hospitals. Eur Radiol. (2023) 33:8279–88. doi: 10.1007/s00330-023-09826-3
3. Bankier AA, MacMahon H, Colby T, Gevenois PA, Goo JM, Leung ANC, et al. Fleischner society: glossary of terms for thoracic imaging. Radiology. (2024) 310:e232558. doi: 10.1148/radiol.232558
4. Farjah F, Monsell SE, Smith-Bindman R, Gould MK, Banegas MP, Ramaprasan A, et al. Fleischner society guideline recommendations for incidentally detected pulmonary nodules and the probability of lung cancer. J Am Coll Radiol. (2022) 19:1226–35. doi: 10.1016/j.jacr.2022.06.018
5. MacMahon H, Naidich DP, Goo JM, Lee KS, Leung ANC, Mayo JR, et al. Guidelines for management of incidental pulmonary nodules detected on CT images: from the fleischner society 2017. Radiology. (2017) 284:228–43. doi: 10.1148/radiol.2017161659
6. Wood DE, Kazerooni EA, Baum SL, Eapen GA, Ettinger DS, Hou L, et al. Lung cancer screening, version 3.2018, NCCN clinical practice guidelines in oncology. J Natl Compr Canc Netw. (2018) 16:412–41. doi: 10.6004/jnccn.2018.0020
7. Pedersen JH, Rzyman W, Veronesi G, D’Amico TA, Van Schil P, Molins L, et al. Recommendations from the European Society of Thoracic Surgeons (ESTS) regarding computed tomography screening for lung cancer in Europe. Eur J Cardiothorac Surg. (2017) 51:411–20. doi: 10.1093/ejcts/ezw418
8. Bai C, Choi CM, Chu CM, Anantham D, Chung-Man Ho J, Khan AZ, et al. Evaluation of pulmonary nodules: clinical practice consensus guidelines for Asia. Chest. (2016) 150:877–93. doi: 10.1016/j.chest.2016.02.650
9. Van Gerpen R. Creating an incidental pulmonary nodule safety-net program. Chest. (2021) 159:2477–82. doi: 10.1016/j.chest.2020.12.053
10. Chen B, Li Q, Hao Q, Tan J, Yan L, Zhu Y, et al. Malignancy risk stratification for solitary pulmonary nodule: A clinical practice guideline. J Evid Based Med. (2022) 15:142–51. doi: 10.1111/jebm.12476
11. Li R, Li X, Xue R, Yang F, Wang S, Li Y, et al. Early metastasis detected in patients with multifocal pulmonary ground-glass opacities (GGOs). Thorax. (2018) 73:290–2. doi: 10.1136/thoraxjnl-2017-210169
12. Zhang C, Zhang J, Xu FP, Wang YG, Xie Z, Su J, et al. Genomic landscape and immune microenvironment features of preinvasive and early invasive lung adenocarcinoma. J Thorac Oncol. (2019) 14:1912–23. doi: 10.1016/j.jtho.2019.07.031
13. Chen H, Carrot-Zhang J, Zhao Y, Hu H, Freeman SS, Yu S, et al. Genomic and immune profiling of pre-invasive lung adenocarcinoma. Nat Commun. (2019) 10:5472. doi: 10.1038/s41467-019-13460-3
14. Cao P, Hu S, Kong K, Han P, Yue J, Deng Y, et al. Genomic landscape of ground glass opacities (GGOs) in East Asians. J Thorac Dis. (2021) 13:2393–403. doi: 10.21037/jtd-21-82
15. He Y, Liu X, Wang H, Wu L, Jiang M, Guo H, et al. Mechanisms of progression and heterogeneity in multiple nodules of lung adenocarcinoma. Small Methods. (2021) 5:e2100082. doi: 10.1002/smtd.202100082
16. Qu R, Ye F, Hu S, Wang B, Qin S, Xiong J, et al. Distinct cellular immune profiles in lung adenocarcinoma manifesting as pure ground glass opacity versus solid nodules. J Cancer Res Clin Oncol. (2022) 146:3775–88. doi: 10.1007/s00432-022-04289-3
17. Altorki NK, Borczuk AC, Harrison S, Groner LK, Bhinder B, Mittal V, et al. Global evolution of the tumor microenvironment associated with progression from preinvasive invasive to invasive human lung adenocarcinoma. Cell Rep. (2022) 39:110639. doi: 10.1016/j.celrep.2022.110639
18. Gao JW, Rizzo S, Ma LH, Qiu XY, Warth A, Seki N, et al. Pulmonary ground-glass opacity: computed tomography features, histopathology and molecular pathology. Transl Lung Cancer Res. (2017) 6:68–75. doi: 10.21037/tlcr.2017.01.02
19. Reittner P, Müller NL, Heyneman L, Johkoh T, Park JS, Lee KS, et al. Mycoplasma pneumoniae pneumonia: radiographic and high-resolution CT features in 28 patients. AJR Am J Roentgenol. (2000) 174:37–41. doi: 10.2214/ajr.174.1.1740037
20. McGuinness G, Scholes JV, Garay SM, Leitman BS, McCauley DI, Naidich DP. Cytomegalovirus pneumonitis: spectrum of parenchymal CT findings with pathologic correlation in 21 AIDS patients. Radiology. (1994) 192:451–9. doi: 10.1148/radiology.192.2.8029414
21. Sider L, Gabriel H, Curry DR, Pham MS. Pattern recognition of the pulmonary manifestations of AIDS on CT scans. Radiographics. (1993) 13:771–84;discussion 85-6. doi: 10.1148/radiographics.13.4.8356267
22. Mori M, Rao SK, Popper HH, Cagle PT, Fraire AE. Atypical adenomatous hyperplasia of the lung: a probable forerunner in the development of adenocarcinoma of the lung. Mod Pathol. (2001) 14:72–84. doi: 10.1038/modpathol.3880259
23. Lee HY, Lee KS, Han J, Kim BT, Cho YS, Shim YM, et al. Mucinous versus nonmucinous solitary pulmonary nodular bronchioloalveolar carcinoma: CT and FDG PET findings and pathologic comparisons. Lung Cancer. (2009) 65:170–5. doi: 10.1016/j.lungcan.2008.11.009
24. Travis WD, Garg K, Franklin WA, Wistuba II, Sabloff B, Noguchi M, et al. Evolving concepts in the pathology and computed tomography imaging of lung adenocarcinoma and bronchioloalveolar carcinoma. J Clin Oncol. (2005) 23:3279–87. doi: 10.1200/JCO.2005.15.776
25. Sawada E, Nambu A, Motosugi U, Araki T, Kato S, Sato Y, et al. Localized mucinous bronchioloalveolar carcinoma of the lung: thin-section computed tomography and fluorodeoxyglucose positron emission tomography findings. Jpn J Radiol. (2010) 28:251–8. doi: 10.1007/s11604-009-0414-4
26. Jamal-Hanjani M, Wilson GA, McGranahan N, Birkbak NJ, Watkins TBK, Veeriah S, et al. Tracking the evolution of non-small-cell lung cancer. N Engl J Med. (2017) 376:2109–21. doi: 10.1056/NEJMoa1616288
27. Park E, Ahn S, Kim H, Park SY, Lim J, Kwon HJ, et al. Targeted sequencing analysis of pulmonary adenocarcinoma with multiple synchronous ground-glass/lepidic nodules. J Thorac O. (2018) 13:1776–83. doi: 10.1016/j.jtho.2018.07.097
28. Ren Y, Huang S, Dai C, Xie D, Zheng L, Xie H, et al. Germline predisposition and copy number alteration in pre-stage lung adenocarcinomas presenting as ground-glass nodules. Front Oncol. (2019) 9:288. doi: 10.3389/fonc.2019.00288
29. Hu X, Fujimoto J, Ying L, Fukuoka J, Ashizawa K, Sun W, et al. Multi-region exome sequencing reveals genomic evolution from preneoplasia to lung adenocarcinoma. Nat Commun. (2019) 10:2978. doi: 10.1038/s41467-019-10877-8
30. Li Y, Li X, Li H, Zhao Y, Liu Z, Sun K, et al. Genomic characterisation of pulmonary subsolid nodules: mutational landscape and radiological features. Eur Respir J. (2020) 55. doi: 10.1183/13993003.01409-2019
31. Wu N, Liu S, Li J, Hu Z, Yan S, Duan H, et al. Deep sequencing reveals the genomic characteristics of lung adenocarcinoma presenting as ground-glass nodules (GGNs). Transl Lung Cancer Res. (2021) 10:1239–55. doi: 10.21037/tlcr-20-1086
32. Lim J, Han YB, Park SY, Ahn S, Kim H, Kwon HJ, et al. Gene expression profiles of multiple synchronous lesions in lung adenocarcinoma. Cells. (2021) 10. doi: 10.3390/cells10123484
33. Wu C, Zhao C, Yang Y, He Y, Hou L, Li X, et al. High discrepancy of driver mutations in patients with NSCLC and synchronous multiple lung ground-glass nodules. J Thorac Oncol. (2015) 10:778–83. doi: 10.1097/JTO.0000000000000487
34. Wei Z, Wang Z, Nie Y, Zhang K, Shen H, Wang X, et al. Molecular alterations in lung adenocarcinoma with ground-glass nodules: A systematic review and meta-analysis. Front Oncol. (2021) 11:724692. doi: 10.3389/fonc.2021.724692
35. Chen K, Bai J, Reuben A, Zhao H, Kang G, Zhang C, et al. Multiomics analysis reveals distinct immunogenomic features of lung cancer with ground-glass opacity. Am J Respir Crit Care Med. (2021) 204:1180–92. doi: 10.1164/rccm.202101-0119OC
36. Bao Y, Zhang S, Zhang X, Pan Y, Yan Y, Wang N, et al. RBM10 loss promotes EGFR-driven lung cancer and confers sensitivity to spliceosome inhibition. Cancer Res. (2023) 83:1490–502. doi: 10.1158/0008-5472.CAN-22-1549
37. Lee H, Jung JH, Ko HM, Park H, Segall AM, Sheffmaker RL, et al. RNA-binding motif protein 10 inactivates c-Myc by partnering with ribosomal proteins uL18 and uL5. Proc Natl Acad Sci U S A. (2023) 120:e2308292120. doi: 10.1073/pnas.2308292120
38. Tang Y, Zhou J, Hooi SC, Jiang YM, Lu GD. Fatty acid activation in carcinogenesis and cancer development: Essential roles of long-chain acyl-CoA synthetases. Oncol Lett. (2018) 16:1390–6. doi: 10.3892/ol.2018.8843
39. Li Y, Li X, Chen H, Sun K, Li H, Zhou Y, et al. Single-cell RNA sequencing reveals the multi-cellular ecosystem in different radiological components of pulmonary part-solid nodules. Clin Transl Med. (2022) 12:e723. doi: 10.1002/ctm2.723
40. Zhou D, Liu QX, Li MY, Hou B, Yang GX, Lu X, et al. Utility of whole exome sequencing analysis in differentiating intrapulmonary metastatic multiple ground-glass nodules (GGNs) from multiple primary GGNs. Int J Clin Oncol. (2022) 27:871–81. doi: 10.1007/s10147-022-02134-8
41. Chung JH, Choe G, Jheon S, Sung SW, Kim TJ, Lee KW, et al. Epidermal growth factor receptor mutation and pathologic-radiologic correlation between multiple lung nodules with ground-glass opacity differentiates multicentric origin from intrapulmonary spread. J Thorac Oncol. (2009) 4:1490–5. doi: 10.1097/JTO.0b013e3181bc9731
42. Asamura H, Suzuki K, Watanabe S, Matsuno Y, Maeshima A, Tsuchiya R. A clinicopathological study of resected subcentimeter lung cancers: a favorable prognosis for ground glass opacity lesions. Ann Thorac Surg. (2003) 76:1016–22. doi: 10.1016/S0003-4975(03)00835-X
43. Rajbhandary S, Dhakal H, Shrestha S. Tumor immune microenvironment (TIME) to enhance antitumor immunity. Eur J Med Res. (2023) 28:169. doi: 10.1186/s40001-023-01125-3
44. Lu T, Yang X, Shi Y, Zhao M, Bi G, Liang J, et al. Single-cell transcriptome atlas of lung adenocarcinoma featured with ground glass nodules. Cell Discovery. (2020) 6:69. doi: 10.1038/s41421-020-00200-x
45. Wang Z, Li Z, Zhou K, Wang C, Jiang L, Zhang L, et al. Deciphering cell lineage specification of human lung adenocarcinoma with single-cell RNA sequencing. Nat Commun. (2021) 12:6500. doi: 10.1038/s41467-021-26770-2
46. Xing X, Yang F, Huang Q, Guo H, Li J, Qiu M, et al. Decoding the multicellular ecosystem of lung adenocarcinoma manifested as pulmonary subsolid nodules by single-cell RNA sequencing. Sci Adv. (2021) 7. doi: 10.1126/sciadv.abd9738
47. Dejima H, Hu X, Chen R, Zhang J, Fujimoto J, Parra ER, et al. Immune evolution from preneoplasia to invasive lung adenocarcinomas and underlying molecular features. Nat Commun. (2021) 12:2722. doi: 10.1038/s41467-021-22890-x
48. Kim EY, Cha YJ, Lee SH, Jeong S, Choi YJ, Moon DH, et al. Early lung carcinogenesis and tumor microenvironment observed by single-cell transcriptome analysis. Transl Oncol. (2022) 15:101277. doi: 10.1016/j.tranon.2021.101277
49. Ziegler SF. Division of labour by CD4(+) T helper cells. Nat Rev Immunol. (2016) 16:403. doi: 10.1038/nri.2016.53
50. Sakaguchi S, Yamaguchi T, Nomura T, Ono M. Regulatory T cells and immune tolerance. Cell. (2008) 133:775–87. doi: 10.1016/j.cell.2008.05.009
51. Gonzalez H, Hagerling C, Werb Z. Roles of the immune system in cancer: from tumor initiation to metastatic progression. Genes Dev. (2018) 32:1267–84. doi: 10.1101/gad.314617.118
52. Schmidt-Weber CB, Akdis M, Akdis CA. TH17 cells in the big picture of immunology. J Allergy Clin Immunol. (2007) 120:247–54. doi: 10.1016/j.jaci.2007.06.039
53. Jankovic D, Liu Z, Gause WC. Th1- and Th2-cell commitment during infectious disease: asymmetry in divergent pathways. Trends Immunol. (2001) 22:450–7. doi: 10.1016/s1471-4906(01)01975-5
54. Zhang N, Bevan MJ. CD8(+) T cells: foot soldiers of the immune system. Immunity. (2011) 35:161–8. doi: 10.1016/j.immuni.2011.07.010
55. Reuben A, Zhang J, Chiou SH, Gittelman RM, Li J, Lee WC, et al. Comprehensive T cell repertoire characterization of non-small cell lung cancer. Nat Commun. (2020) 11:603. doi: 10.1038/s41467-019-14273-0
56. Wang Y, Liu J, Burrows PD, Wang JY. B cell development and maturation. Adv Exp Med Biol. (2020) 1254:1–22. doi: 10.1007/978-981-15-3532-1_1
57. Germain C, Gnjatic S, Tamzalit F, Knockaert S, Remark R, Goc J, et al. Presence of B cells in tertiary lymphoid structures is associated with a protective immunity in patients with lung cancer. Am J Respir Crit Care Med. (2014) 189:832–44. doi: 10.1164/rccm.201309-1611OC
58. Moore DK, Loxton AG. Regulatory B lymphocytes: development and modulation of the host immune response during disease. Immunotherapy. (2019) 11:691–704. doi: 10.2217/imt-2018-0185
59. Valipour B, Velaei K, Abedelahi A, Karimipour M, Darabi M, Charoudeh HN. NK cells: An attractive candidate for cancer therapy. J Cell Physiol. (2019) 234:19352–65. doi: 10.1002/jcp.28657
60. Wang B, Xi C, Liu M, Sun H, Liu S, Song L, et al. Breast fibroblasts in both cancer and normal tissues induce phenotypic transformation of breast cancer stem cells: a preliminary study. PeerJ. (2018) 6:e4805. doi: 10.7717/peerj.4805
61. Zi F, He J, He D, Li Y, Yang L, Cai Z. Fibroblast activation protein alpha in tumor microenvironment: recent progression and implications (review). Mol Med Rep. (2015) 11:3203–11. doi: 10.3892/mmr.2015.3197
62. Allinson JP, Brown J, Gibb K, Navani N. Immunotherapy in non-small cell lung cancer. Which patients and at which stage? Am J Respir Crit Care Med. (2019) 199:1277–9. doi: 10.1164/rccm.201810-1930RR
63. Chang JY, Lin SH, Dong W, Liao Z, Gandhi SJ, Gay CM, et al. Stereotactic ablative radiotherapy with or without immunotherapy for early-stage or isolated lung parenchymal recurrent node-negative non-small-cell lung cancer: an open-label, randomised, phase 2 trial. Lancet. (2023) 402:871–81. doi: 10.1016/s0140-6736(23)01384-3
64. Hu X, Estecio MR, Chen R, Reuben A, Wang L, Fujimoto J, et al. Evolution of DNA methylome from precancerous lesions to invasive lung adenocarcinomas. Nat Commun. (2021) 12:687. doi: 10.1038/s41467-021-20907-z
65. Zheng H, Zhang H, Wang S, Xiao F, Liao M. Invasive prediction of ground glass nodule based on clinical characteristics and radiomics feature. Front Genet. (2021) 12:783391. doi: 10.3389/fgene.2021.783391
66. Yankelevitz DF, Yip R, Smith JP, Liang M, Liu Y, Xu DM, et al. CT screening for lung cancer: nonsolid nodules in baseline and annual repeat rounds. Radiology. (2015) 277:555–64. doi: 10.1148/radiol.2015142554
67. Tsutani Y, Miyata Y, Nakayama H, Okumura S, Adachi S, Yoshimura M, et al. Oncologic outcomes of segmentectomy compared with lobectomy for clinical stage IA lung adenocarcinoma: propensity score-matched analysis in a multicenter study. J Thorac Cardiovasc Surg. (2013) 146:358–64. doi: 10.1016/j.jtcvs.2013.02.008
68. Gould MK, Donington J, Lynch WR, Mazzone PJ, Midthun DE, Naidich DP, et al. Evaluation of individuals with pulmonary nodules: when is it lung cancer? Diagnosis and management of lung cancer, 3rd ed: American College of Chest Physicians evidence-based clinical practice guidelines. Chest. (2013) 143:e93S–e120S. doi: 10.1378/chest.12-2351
69. Haruki T, Aokage K, Miyoshi T, Hishida T, Ishii G, Yoshida J, et al. Mediastinal nodal involvement in patients with clinical stage I non-small-cell lung cancer: possibility of rational lymph node dissection. J Thorac Oncol. (2015) 10:930–6. doi: 10.1097/JTO.0000000000000546
70. Zha J, Xie D, Xie H, Zhang L, Zhou F, Ying P, et al. Recognition of “aggressive” behavior in “indolent” ground glass opacity and mixed density lesions. J Thorac Dis. (2016) 8:1460–8. doi: 10.21037/jtd.2016.05.33
71. Shimada Y, Saji H, Otani K, Maehara S, Maeda J, Yoshida K, et al. Survival of a surgical series of lung cancer patients with synchronous multiple ground-glass opacities, and the management of their residual lesions. Lung Cancer. (2015) 88:174–80. doi: 10.1016/j.lungcan.2015.02.016
72. Yao F, Yang H, Zhao H. Single-stage bilateral pulmonary resections by video-assisted thoracic surgery for multiple small nodules. J Thorac Dis. (2016) 8:469–75. doi: 10.21037/jtd.2016.02.66
73. Nakata M, Sawada S, Yamashita M, Saeki H, Kurita A, Takashima S, et al. Surgical treatments for multiple primary adenocarcinoma of the lung. Ann Thorac Surg. (2004) 78:1194–9. doi: 10.1016/j.athoracsur.2004.03.102
74. Mun M, Kohno T. Efficacy of thoracoscopic resection for multifocal bronchioloalveolar carcinoma showing pure ground-glass opacities of 20 mm or less in diameter. J Thorac cardiov sur. (2007) 134:877–82. doi: 10.1016/j.jtcvs.2007.06.010
75. Onishi H, Shioyama Y, Matsumoto Y, Shibamoto Y, Miyakawa A, Suzuki G, et al. Stereotactic body radiotherapy in patients with lung tumors composed of mainly ground-glass opacity. J Radiat Res. (2020) 61:426–30. doi: 10.1093/jrr/rraa015
76. Tomita N, Okuda K, Osaga S, Miyakawa A, Nakanishi R, Shibamoto Y. Surgery versus stereotactic body radiotherapy for clinical stage I non-small-cell lung cancer: propensity score-matching analysis including the ratio of ground glass nodules. Clin Transl Oncol. (2021) 23:638–47. doi: 10.1093/jrr/rraa015
77. Green DB, Groner LK, Lee JJ, Shin J, Broncano J, Vargas D, et al. Overview of interventional pulmonology for radiologists. Radiographics. (2021) 41:1916–35. doi: 10.1148/rg.2021210046
78. Uhlig J, Ludwig JM, Goldberg SB, Chiang A, Blasberg JD, Kim HS. Survival Rates after Thermal Ablation versus Stereotactic Radiation Therapy for Stage 1 Non-Small Cell Lung Cancer: A National Cancer Database Study. Radiology. (2018) 289:862–70. doi: 10.1148/radiol.2018180979
79. Postmus PE, Kerr KM, Oudkerk M, Senan S, Waller DA, Vansteenkiste J, et al. Early and locally advanced non-small-cell lung cancer (NSCLC): ESMO Clinical Practice Guidelines for diagnosis, treatment and follow-up. Ann Oncol. (2017) 28:iv1–iv21. doi: 10.1093/annonc/mdx222
80. Kodama H, Yamakado K, Hasegawa T, Takao M, Taguchi O, Fukai I, et al. Radiofrequency ablation for ground-glass opacity-dominant lung adenocarcinoma. J Vasc Interv Radiol. (2014) 25:333–9. doi: 10.1016/j.jvir.2013.11.035
81. Liu S, Zhu X, Qin Z, Xu J, Zeng J, Chen J, et al. Computed tomography-guided percutaneous cryoablation for lung ground-glass opacity: A pilot study. J Cancer Res Ther. (2019) 15:370–4. doi: 10.4103/jcrt.JCRT_299_18
82. Yang X, Ye X, Lin Z, Jin Y, Zhang K, Dong Y, et al. Computed tomography-guided percutaneous microwave ablation for treatment of peripheral ground-glass opacity-Lung adenocarcinoma: A pilot study. J Cancer Res Ther. (2018) 14:764–71. doi: 10.4103/jcrt.JCRT_269_18
83. Huang G, Yang X, Li W, Wang J, Han X, Wei Z, et al. A feasibility and safety study of computed tomography-guided percutaneous microwave ablation: a novel therapy for multiple synchronous ground-glass opacities of the lung. Int J Hyperthermia. (2020) 37:414–22. doi: 10.1080/02656736.2020.1756467
84. Lu W, Cham MD, Qi L, Wang J, Tang W, Li X, et al. The impact of chemotherapy on persistent ground-glass nodules in patients with lung adenocarcinoma. J Thorac Dis. (2017) 9:4743–9. doi: 10.21037/jtd.2017.10.50
85. Zhang Y, Deng C, Ma X, Gao Z, Wang S, Zheng Q, et al. Ground-glass opacity-featured lung adenocarcinoma has no response to chemotherapy. J Cancer Res Clin. (2020) 146:2411–7. doi: 10.1007/s00432-020-03234-6
86. Kim HK, Choi YS, Kim J, Shim YM, Lee KS, Kim K. Management of multiple pure ground-glass opacity lesions in patients with bronchioloalveolar carcinoma. J Thorac Oncol. (2010) 5:206–10. doi: 10.1097/JTO.0b013e3181c422be
87. Cheng B, Li C, Zhao Y, Li J, Xiong S, Liang H, et al. The impact of postoperative EGFR-TKIs treatment on residual GGO lesions after resection for lung cancer. Signal Transduct Target Ther. (2021) 6:73. doi: 10.1038/s41392-020-00452-9
Keywords: ground glass nodules, lung adenocarcinoma, pathology, genomic mutation, immune cell infiltration, immune microenvironment
Citation: Chen F, Li J, Li L, Tong L, Wang G and Zou X (2024) Multidimensional biological characteristics of ground glass nodules. Front. Oncol. 14:1380527. doi: 10.3389/fonc.2024.1380527
Received: 01 February 2024; Accepted: 07 May 2024;
Published: 22 May 2024.
Edited by:
Mirella Marino, Hospital Physiotherapy Institutes (IRCCS), ItalyReviewed by:
Song Xu, Tianjin Medical University General Hospital, ChinaCopyright © 2024 Chen, Li, Li, Tong, Wang and Zou. This is an open-access article distributed under the terms of the Creative Commons Attribution License (CC BY). The use, distribution or reproduction in other forums is permitted, provided the original author(s) and the copyright owner(s) are credited and that the original publication in this journal is cited, in accordance with accepted academic practice. No use, distribution or reproduction is permitted which does not comply with these terms.
*Correspondence: Xuelin Zou, Mjg3NjkzMzYwQHFxLmNvbQ==
†These authors have contributed equally to this work
Disclaimer: All claims expressed in this article are solely those of the authors and do not necessarily represent those of their affiliated organizations, or those of the publisher, the editors and the reviewers. Any product that may be evaluated in this article or claim that may be made by its manufacturer is not guaranteed or endorsed by the publisher.
Research integrity at Frontiers
Learn more about the work of our research integrity team to safeguard the quality of each article we publish.