- 1Department of Medicine, Section of Internal Medicine, University of Verona, Verona, Italy
- 2EuroBloodNet Referral Center, Azienda Ospedaliera Universitaria Integrata Verona, Verona, Italy
- 3Istituto di Ricovero e Cura a Carattere Scientifico (IRCCS) Istituto Romagnolo per lo Studio dei Tumori (IRST) “Dino Amadori”, Meldola, Italy
Anemia is common in cancer patients and impacts on quality of life and prognosis. It is typically multifactorial, often involving different pathophysiological mechanisms, making treatment a difficult task. In patients undergoing active anticancer treatments like chemotherapy, decreased red blood cell (RBC) production due to myelosuppression generally predominates, but absolute or functional iron deficiency frequently coexists. Current treatments for chemotherapy-related anemia include blood transfusions, erythropoiesis-stimulating agents, and iron supplementation. Each option has limitations, and there is an urgent need for novel approaches. After decades of relative immobilism, several promising anti-anemic drugs are now entering the clinical scenario. Emerging novel classes of anti-anemic drugs recently introduced or in development for other types of anemia include activin receptor ligand traps, hypoxia-inducible factor-prolyl hydroxylase inhibitors, and hepcidin antagonists. Here, we discuss their possible role in the treatment of anemia observed in patients receiving anticancer therapies.
Introduction
Anemia represents a common problem in cancer patients, affecting nearly 30-49% of those with solid tumors at diagnosis, further increasing to nearly 70% or more among those undergoing anticancer treatments or those with advanced disease (1–4). It is typically multifactorial, often involving different pathophysiological mechanisms in the same individual (5, 6). This makes treatment a difficult task (4), leaving a substantial fraction of patients sub-optimally or even untreated (1, 6). Although blood loss (e.g., in gastrointestinal tumors, or iatrogenic due to frequent blood sampling) and reduced red blood cell (RBC) survival can occur, decreased RBC production predominates in most patients. In turn, reduced erythropoiesis can be due to different mechanisms, which include: 1) direct bone marrow toxicity by anticancer drugs; 2) absolute deficiency of essential micronutrients (mainly, but not only, iron); 3) functional iron deficiency due to hepcidin-induced iron sequestration into macrophages; 4) impaired bone marrow response to erythropoietin (EPO) due to tumor-associated systemic inflammation; and bone marrow substitution by metastatic cancer cells (7, 8). This review focuses on anemia mainly related to anticancer treatments. For a comprehensive review of the pathophysiology of anemia in cancer patients, the readers are referred elsewhere (4).
The term Cancer-Chemotherapy Related Anemia (CCRA) underlines what it is often the major driver of anemia in cancer patients but cannot be viewed as an absolute entity. Rather, the coexistence of other mechanism(s) is often the rule, with important implications for the treatment of this condition (see below). Moreover, it has to be taken into account that CCRA is not a peculiarity of traditional chemotherapy agents, but can also occur as off-target effect with a number of newer antineoplastic agents (4, 9), including tyrosine kinase inhibitors (10), monoclonal antibodies (11), and immunomodulatory agents (12). Drug-induced kidney dysfunction can also contribute to hypoproliferative anemia through inadequate EPO production. Rarely immunotherapeutic agents known as checkpoint inhibitors (e.g., nivolumab, ipilimumab, and pembrolizumab) can induce antibody-mediated hemolytic anemia due to immune dysregulation (13). Overall, anemia remains one of the most frequent adverse effects of anticancer therapies, with a prevalence exceeding 90 percent for patients receiving certain treatments (3). The ECAS (European Cancer Anemia Survey) study, involving more than 15,000 patients, found an increasing prevalence of anemia from 32 percent in those newly diagnosed before receiving any treatment, to 44-51 percent in those receiving concomitant chemotherapy/radiotherapy or chemotherapy alone, respectively (1). Another survey in the U.S. estimated a prevalence of anemia of 61 percent in patients receiving chemotherapy, but only 25 percent of them received any anemia treatment (14). Very recently, the CARENFER study enrolling 1,221 patients with different types of solid malignancies, most of them (75.4 percent) treated with chemotherapy, found a high prevalence of iron deficiency (57.9 percent) with or without anemia (15), pointing out the need of better detection of this micronutrient deficiency in cancer patients. Prevalence and severity of anemia vary depending on the extent of the disease, the type, schedule, and intensity of treatment, and whether the patient has received prior radiotherapy and/or chemotherapy (16).
CCRA is associated with decreased functional capacity and a diminished quality of life (QOL) (16, 17), particularly because of its contribution to fatigue, which is commonly recognized as the most debilitating symptom (18). The degree of anemia in patients receiving active anticancer treatment is classified according to the National Cancer Institute (NCI) Common Terminology Criteria for Adverse Effects (CTCAE) (available at https://ctep.cancer.gov/protocoldevelopment/electronic_applications/docs/ctcae_v5_quick_reference_5x7.pdf), ranging from grade 1 or mild (Hb levels < 120/130 g/L in females/males but ≥100 g/L) to grade 4 (Hb levels < 80 g/L and symptoms suggesting a life-threatening condition) (for the detailed classification, see (4). Anemia can represent a dose-limiting toxicity that prevents patients from being treated with the full dose of chemotherapy. In patients with both solid and liquid malignancies, the development of severe anemia during the first cycle of chemotherapy is associated with an increased risk of dose delay and/or dose reduction in the subsequent chemotherapy cycle. Finally, evidence suggests that anemia may be an independent prognostic factor associated with reduced survival (19). For example, Waters and colleagues reported that 86 percent of 906 patients treated with chemotherapy for lung cancer at a single institution in the United States between 1999 and 2001 developed anemia during treatment (or within 1 month after completing) (20). The median survival rates of patients whose Hb levels were maintained above 12.0 g/dl were significantly (p < 0.001) higher than those of patients with lower Hb levels (20). However, because of the concurrence of multiple confounders, including transfusions, a direct causal relationship between anemia and survival in cancer patients has not yet been established (21).
Therapeutic options for cancer-chemotherapy-related anemia
As for every type of anemia, elucidating the cause(s) is the cornerstone of an effective therapeutic approach. However, this is especially challenging in cancer patients. In its simplest form, CCRA can be the result of bone marrow toxicity in patients with early-stage cancer who were not anemic before starting chemotherapy. On the other hand, in patients with advanced disease, systemic inflammation, and micronutrient deficiencies (generally of iron, rarely of folate or B12 with the possible exception of low-middle income countries (22)) are often in the background, and chemotherapy merely unravels or exacerbates a pre-existing condition. In such cases the management should involve a multi-modal approach accounting for the severity of anemia, the associated inflammatory state, the impairment in iron metabolism, and the overall nutritional status.
Currently available treatments for anemia in patients receiving anticancer treatments include blood transfusions, iron supplementation, and erythropoiesis-stimulating agents (ESAs), like recombinant EPO (rhEPO) and darbepoetin-α. Each option has limitations, and guidelines restrict their use to specific settings (21, 23). Thereby, anemia in cancer patients is often poorly treated.
At present, we are witnessing an unprecedented development of novel anti-anemic drugs (24–26), many of them acting by ameliorating erythropoiesis. For this reason, since anemia in patients receiving anticancer treatments is essentially hypoproliferative due to bone marrow suppression, they could be theoretically of benefit also in this setting.
Below we review the current and future options for anemia in patients receiving active anticancer treatments (Figure 1).
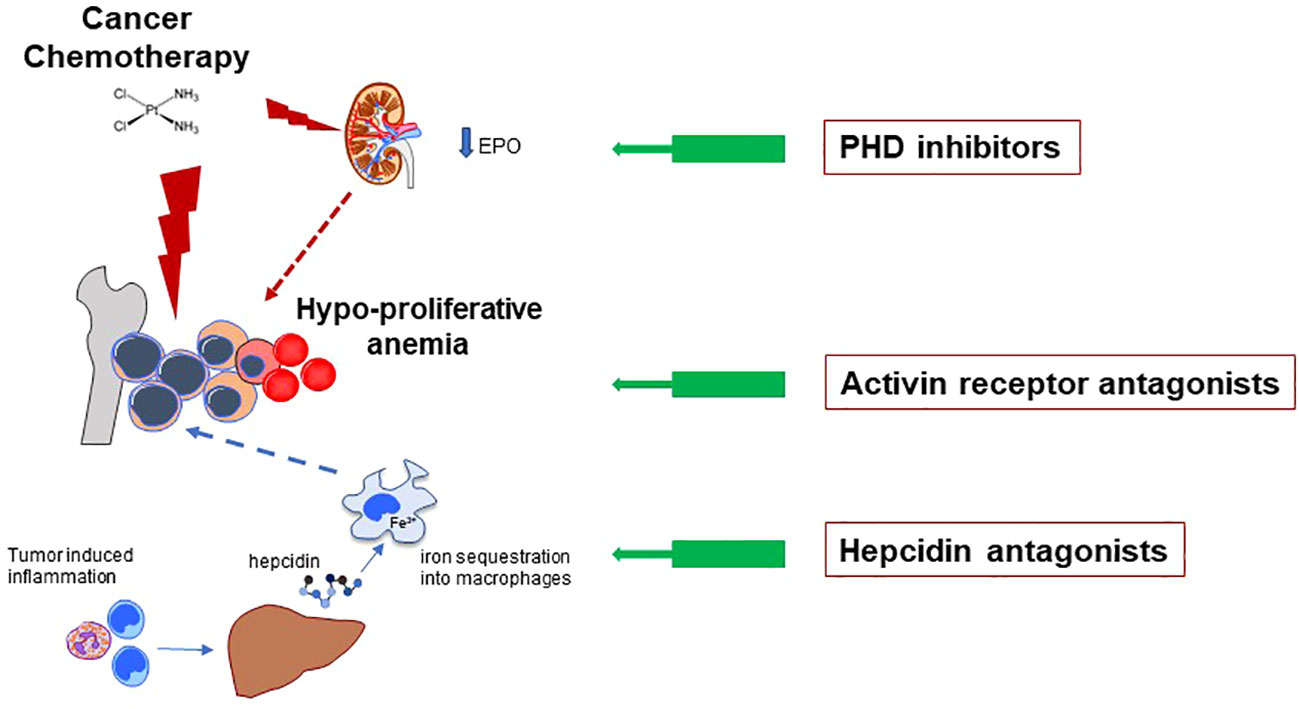
Figure 1 CCRA is essentially a hypoproliferative anemia mainly caused by toxic effects of anticancer drugs on rapidly proliferating RBC precursors in the bone marrow (major red lightning). However, the pathophysiology is often more complex, including drug-induced kidney damage with reduced production of endogenous EPO (minor red lightning), and low iron availability for erythropoiesis (dotted blue arrow). The latter can be due to either absolute or functional iron deficiency (FID, see main text). FID, in turn, is mainly determined by inflammatory-related increased hepcidin levels, leading to iron se-questration into macrophages. The general mechanisms of action of the innovative anti-anemic drug classes discussed in text are depicted. All the three classes inhibit path-way/molecule that modulate erythropoiesis, ultimately increasing RBCs production. Hypoxia Inducible Factor – Prolyl Hydroxylase inhibitors (HIF-PHIs) stabilize HIF leading to increased production of endogenous EPO. They represent an important alternative to the traditional administration of exogenous (recombinant) EPO. Moreover, they also have some favorable effects on iron metabolism (see main text). Activin Receptor (ACVR) ligand traps bind several members of the Transforming Growth Factor – beta (TGF-β) superfamily, thus reducing a key signaling pathway which negatively modulates late-stage erythropoiesis. Hepcidin antagonists increase iron availability for erythropoiesis.
RBC transfusions
Until the advent of ESAs in the 1990s, RBC transfusions—which were usually administered empirically when hemoglobin concentrations declined <100 g/L —were the only/primary treatment of cancer-related anemia. While transfusions are effective in providing an immediate increase in Hb, benefits are transient and risks are far from negligible, including anaphylactic reactions, transfusion-related acute lung injury (TRALI), circulatory overload, pathogens transmission, and increased susceptibility to infections possibly due to immunosuppressive modulation (27, 28). Of note, RBC transfusions have been independently associated with an increased risk for adverse outcomes in cancer patients undergoing surgery in terms of increased mortality, hospital length of stay, and tumor recurrence (29–31). Regarding CCRA, the Hb threshold for transfusion is not as clear as in other settings (32). The use of a liberal transfusion regimen (e.g., Hb threshold of 90 to 100 g/L) has not proven advantageous in terms of risk/benefit (33–35). A systematic review showed that restrictive transfusion regimens (e.g., Hb thresholds set at 70 to 80 g/L) in oncologic patients decrease blood utilization without increasing mortality and morbidity (36). The most recent guidelines issued by the Association for the Advancement of Blood and Biotherapies (AABB) in 2023 recommend a restrictive transfusion strategy in hemodynamically stable hospitalized adult patients with hematologic and oncologic disorders considering transfusion when the hemoglobin concentration is less than 70 g/L (37). However, the Authors recognize that the level of certainty evidence is low due to the few numbers of trials and patients enrolled. Thus, whether a rigorous restrictive regimen in cancer patients is as safe as a liberal regimen remains debated. Ad hoc studies investigating the optimal patient blood management strategy in CCRA are warranted. For the moment, especially in outpatients, an individualized approach putting first a comprehensive clinical judgment rather than mere laboratory abnormalities remains the best option (4).
Iron supplementation
As mentioned above, iron deficiency (ID) in cancer patients is common and multifactorial (15, 38). For a comprehensive review of the numerous mechanisms possibly leading to ID in cancer patients, readers are referred elsewhere (6). Briefly, absolute ID (AID) corresponds to depletion of iron stores, which can be due, for example to bleeding in gastrointestinal cancer, malnutrition in patients with advanced disease, and malabsorption in patients submitted to wide bowel resections. Even more common in cancer patients is iron-restricted erythropoiesis, also sometimes referred to as functional ID (FID), which corresponds to insufficient iron availability despite theoretically adequate iron stores. Iron sequestration typically takes place in macrophages due to the upregulation of hepcidin (see below) by proinflammatory cytokines (8). Generally speaking, anemia in cancer patients can be reminiscent of the anemia of inflammation (39) when all other causes of anemia have been excluded. Finally, a particular type of FID can occur in CCRA patients treated with ESAs, where expanded erythropoiesis not infrequently outpaces iron delivery from stores, ultimately leading to ESAs unresponsiveness unless supplemental iron is given (40).
Indeed, numerous studies have proven the efficacy of intravenous (IV) iron in combination with ESAs in CCRA (41–43). An earlier systematic review and meta-analysis (performed in 2013) of randomized controlled trials comparing IV iron with no iron or oral iron in CCRA confirmed the efficacy of IV iron in combination with ESAs (44). The same meta-analysis also reported the efficacy of IV iron alone, while only a few smaller trials were available at that time (44). Indeed, with the recent advent of modern third-generation IV iron preparations (45), evidence is accumulating on the efficacy of iron monotherapy in CCRA (46–49). CCRA patients with concomitant AID are the most suitable candidates for this approach, but diagnosing AID in cancer patients using traditional iron biomarkers is challenging (6), due, for example, to ferritin upregulation by tumor necrosis factor-alpha (TNF-a) and pro-inflammatory cytokines (50, 51). To identify patients that could benefit from iron supplementation, different ferritin cut-offs have been proposed, ranging from 100 (21) to up to 800 (52) μg/L, also depending on the presence or absence of concomitant low transferrin saturation (TSAT <20%). However, a consensus is still lacking, and there is an urgent need for better biomarkers (8). The most promising one in this sense is hepcidin, which has the potential to distinguish absolute iron deficiency even in inflammatory conditions (53). Indeed, at least three independent studies have shown its usefulness in predicting AID (and hence the response to IV iron) in CCRA (48, 54, 55). Nevertheless, due to the lack of harmonization between different valuable assays (56, 57), a universal cutoff is not yet available. On the other hand, there is substantial agreement on the little usefulness of oral iron in the majority of CCRA patients due to several caveats, including side effects (e.g., gastrointestinal discomfort), poor compliance, especially in patients on polypharmacy, and inflammation-induced malabsorption through increased hepcidin (4–6, 9). Iron administration in CCRA should be prescribed to patients within the framework of currently available guidelines (21, 52).
Erythropoiesis stimulating agents
Since their appearance in the 1990s, erythropoiesis-stimulating agents (ESAs) substantially changed the scenario of CCRA treatment. The efficacy of either rhEPO (epoetin) or the analog darbepoetin in reducing transfusions and improving QoL has been documented by several studies and meta-analyses (58–60). Biosimilars are available, showing acceptable interchangeability (61). However, ESAs in cancer patients have raised major concerns, including increased risk of thrombosis (62) and decreased survival possibly due to tumor progression (63). The latter could be due to off-target ESAs binding to ephrin-B4 receptors, which in turn increase angiogenesis and tumor neovascularization (64). This has led to consistent restriction of ESAs use for treating anemia in cancer patients. According to recent guidelines jointly issued by the American Society of Hematology and the American Society of Clinical Oncology (for a comprehensive review, see (23), these drugs should be reserved for CCRA patients receiving chemotherapy with palliative intent and who are expected to have short survival. Outside the scenario of CCRA, ESAs are currently used in patients with low-risk myelodysplastic (MDS) syndromes.
Novel anti-anemic drugs with a potential use for CCRA
Activin type II receptor (ACVR) ligand traps
This novel class includes two drugs, luspatercept and sotatercept. They are recombinant fusion proteins that act by inhibiting negative regulators of late-stage erythropoiesis, like activin B and other transforming growth factor beta (TGF β) superfamily ligands (65–70). Thus, they can be viewed as molecular traps that facilitate erythropoiesis by removing biological brakes to the process (24, 26, 71). At variance with EPO, which stimulates the proliferation of RBC progenitors during the early stages of erythropoiesis, ACVR ligand traps distinctly favor late-stage erythropoiesis. Their effect, also through modulation of marrow stromal cells (66), is prevalent in differentiation rather than proliferation of RBC progenitors. This explains why these compounds work particularly well in conditions of pathologically expanded but ineffective erythropoiesis, like thalassemic syndromes and low-risk MDS (see below).
Of note, sotatercept was initially developed to increase bone mineral density in malignant bone disease and in osteoporosis (72, 73), with the unexpected observation of an erythroid response (73, 74). Later, the same effect was observed with the analog luspatercept (75). Improvement of ineffective erythropoiesis was subsequently confirmed in murine models of b-thalassemia (69). This recently translated into one of the major achievements in the treatment of patients with beta-thalassemic syndromes, where luspatercept has been proven to increase Hb and substantially decrease transfusion needs (76). Indeed, luspatercept has been preferentially developed in clinical studies because of a higher specificity activin B (rather than for activin A) as compared to sotatercept, with a lower probability of determining off-target effects (77). The sustained effect on erythropoiesis has also prompted the use of luspatercept for anemia associated with hematologic malignancies (77, 78). Low-risk MDS have been selected because of the need for alternative strategies to frequent RBC transfusions in anemic patients with prolonged anticipated survival. The MEDALIST trial enrolled 229 transfusion-dependent patients affected by MDS with ringed sideroblasts, who were either refractory or not candidates for ESAs because of high baseline EPO level (79). Transfusion independence for ≥8 weeks was observed in 38 percent of the patients in the luspatercept group, as compared with 13 percent in the placebo group (P<0.001). Such positive results have been recently confirmed by the COMMANDS trial, which enrolled 356 patients randomized to receive either luspatercept or epoetin alfa and showed a higher rate of transfusion independence in the luspatercept group (80). Luspatercept shows a favorable safety profile (79), and is now approved for low-risk MDS by both FDA and EMA. A post-hoc analysis of the MEDALIST study provided support for the efficacy of Luspatercept also in a subgroup of patients affected by myelodysplastic syndromes/myeloproliferative neoplasm with ring sideroblasts and thrombocytosis (MDS/MPN-RT-T) (81); Luspatercept is currently under active investigation for other types of anemia in hematological malignancies, like myeloproliferative neoplasm-associated myelofibrosis (NCT03194542). Preliminary results of the latter study have been recently presented showing that transfusion-independency was reached in nearly one-third of patients on ruxolitinib that were initially transfusion dependent (82). Such promises and successes in anemia associated with hematological malignancies make ACVR ligand traps an attractive option also for anemia in other malignancies. To date, only limited/incomplete data are available for sotatercept in CCRA. Two Phase II placebo-controlled studies evaluated this anti-anemic drug in patients with metastatic breast cancer receiving myelosuppressive chemotherapy or with advanced/metastatic solid tumors receiving platinum-based chemotherapy (83). Unfortunately, both studies were terminated early due to slow patient accrual. Nevertheless, preliminary results indicated that mean hemoglobin levels increased ≥10 g/L in up to 66.7 percent of patients receiving sotatercept, as compared to 0 percent in placebo groups (83). In both studies, the safety profile was comparable to that of placebo groups. While caution is needed in interpretation due to the small populations studied, both studies point out the potential benefit of ACVR ligand traps for CCRA.
Hepcidin antagonists
As mentioned above, hepcidin overexpression plays a major role in the anemia of inflammation (39, 84) and often contributes to anemia in cancer patients by reducing iron availability for erythropoiesis because of iron sequestration in cells, mainly in macrophages (39). This condition of FID can be, in principle, reversed by counteracting hepcidin activity (85). However, inhibiting hepcidin is not as simple as it could be theoretically anticipated. This hormone, which represents the master regulator of iron homeostasis (53), is continuously produced by the liver in discrete amounts (nM concentration). Direct hepcidin binders have been developed (85, 86), including a fully humanized monoclonal antibody (LY2787106), and a structured mirror-image RNA oligonucleotide (NOX-H94) (87), both with high affinity for human hepcidin. A phase 1 multicenter trial evaluated LY2787106 in 33 patients with different malignancies and anemia, 19 of whom received chemotherapy (88). LY2787106 was well-tolerated and induced initially dose-dependent increases in serum iron and transferrin saturation in patients with high hepcidin concentration. However, iron parameters quickly returned to baseline (within 1 week), and the primary efficacy endpoint (increased Hb level) was not reached (88). No further development of the drug has appeared in the literature.
NOX-H94 was initially evaluated in human volunteers subjected to experimental endotoxemia by lipopolysaccharide administration, with an observed increase of serum iron consistent with hepcidin inhibition (89). The drug was further shown to effectively inhibit hepcidin in a dose-dependent manner and was apparently well-tolerated (90). In 2014, a phase-II pilot study on 12 patients with anemia and hematological malignancies reported increased hemoglobin levels in 5 (91). However, again, no further developments have been reported. Another crucial point that makes hepcidin antagonization far from simple is represented by the substantial complexity of molecular regulation of hepcidin production (92, 93). Many strategies alternative to direct hepcidin neutralization have been reported, including antagonists of the bone morphogenetic protein 6 (BMP6) pathway with heparin derivatives (94–97), anti-BMP6 antibodies (98), dorsomorphin (99), and ferroportin stabilizers (100, 101). Further drugs that modulate the hepcidin-ferroportin axis include anti-hemojuvelin (HJV) antibodies (currently under evaluation in two clinical trials NCT05745883 and NCT05320198), and momelotinib. This latter drug is a promising and potent inhibitor of the ACVR1/ALK2 receptor, which has been developed for the treatment of anemia in patients with myelofibrosis (102–104). In a broad sense, numerous other drug classes indirectly regulate hepcidin (for a recent review, see (105)). Among them are interleukin 6 (IL-6) and interleukin-1 beta (IL-1b) inhibitors, signal transducer and activator of transcription 3 (STAT3) inhibitors, and Hypoxia-inducible factor–prolyl hydroxylase inhibitors (HIF-PHIs) (see below). While several clinical trials are underway (90), no data are available for patients with CCRA.
Hypoxia-inducible factor-prolyl hydroxylase inhibitors
Hypoxia-inducible factors (HIFs) are transcription factors that mediate the cellular response to hypoxic conditions via the upregulation of several genes that are key to erythropoiesis, including those for EPO, the EPO receptor, and even for some critical proteins involved in iron metabolism (25, 106, 107). HIFs are heterodimers consisting of a constitutively expressed HIF-1β subunit and an O2-regulated HIF-α subunit. Under normoxic conditions, HIF is degraded by the hydroxylation of prolyl residues of the HIF-α subunit by HIF prolyl hydroxylases (PH). By preventing HIF degradation, HIF-PHIs stimulate endogenous EPO and promote erythropoiesis (25). Several HIF prolyl hydroxylase inhibitors have been developed and successfully used to treat anemia in chronic kidney disease (CKD) patients (108).
HIF-PHIs induce endogenous EPO at a concentration near the physiological range, representing a substantial difference with peaks determined by rhEPO administration and may explain the lower rate of cardiovascular adverse events. Other advantages over traditional ESAs include oral administration, lower costs, and lower immunogenicity. As mentioned above, it is worth noting that HIF-PHIs have pleiotropic favorable action on erythropoiesis beyond EPO stimulation (25). Indeed, critical players in iron metabolism like the Transferrin Receptor, Divalent Metal Transporter 1, and Ferroportin (the cell membrane receptor of hepcidin) are controlled by HIF through binding to their hypoxia-responsive elements, resulting in potent transcriptional activation (109–111). In this way, HIF-PHIs improve iron absorption from the gut and iron uptake and utilization by erythroid precursors through upregulation of transferrin receptor, ultimately leading to decreased levels of hepcidin, ferritin, and transferrin saturation (112). Roxadustat, one of the main HIF-PHIs already approved for anemia in CKD (113, 114), is currently being investigated in oncologic patients with anemia. MATTERHORN (NCT03263091) is a phase 3, randomized, double-blind, placebo-controlled study to assess the efficacy and safety of roxadustat in anemia of lower risk-MDS. In the dose-selection stage, Roxadustat was well-tolerated, and 37.5% of pts (9/24) with LR-MDS and low RBC transfusion burden achieved transfusion independence (TI) (115). In the MATTERHORN double-blind stage, a more significant percentage of pts in the Roxadustat arm compared with the placebo arm were TI responders (47.5% vs. 33.3%). However, this difference did not reach statistical significance (p=0.22) (116). A recent phase 2 open-label trial has investigated the efficacy and safety of Roxadustat for CCRA in various non-myeloid malignancies, including pancreas, breast, lung, and ovarian cancer, who have planned concurrent myelosuppressive therapies (117). Subgroup analyses demonstrated the efficacy of Roxadustat in correcting Hb regardless of tumor type and chemotherapy received. Adverse events were consistent with observations in patients with advanced-stage malignancies.
Despite their undoubtful benefit in the short-term correction of anemia, concerns have been raised about the possible off-target effects of HIF-PHIs during long-term use. Indeed, HIFs are ubiquitously expressed and regulate a broad spectrum of cell functions beyond erythropoiesis. Theoretically, HIF-PHIs may foster latent cancers since HIF activation in a hypoxic environment could promote cell proliferation and angiogenesis. This calls for caution, especially in older people. Nevertheless, no data thus far have revealed any effect of HIF-PHIs on tumor progression. On the other hand, chronic hypoxia caused by prolyl hydroxylase inhibition may inactivate cancer-associated fibroblasts, leading to decreased tumor metastatic spread. Ideally, future compounds able to selectively stimulate HIF2 could reduce undesirable off-target effects.
Conclusions and perspectives
Anemia in cancer represents a significant unmet clinical need because of its high prevalence, the impact on QoL, and the likely implications on survival. Currently available treatments, particularly RBC transfusions and ESAs, have significant limitations. This results in a substantial proportion of patients left untreated or treated inadequately (4, 21).
The first step of the treatment of anemia in these patients is disentangling the causes, particularly those that can be readily treated, like iron deficiency, which is highly prevalent in cancer patients (3, 14) and relatively simple to correct with the newer intravenous iron preparations.
Nowadays, the therapeutic armamentarium of anti-anemic drugs is rapidly expanding, with remarkable successes in conditions like CKD, thalassemic syndromes, and certain MDS. For the moment, only a minority of the agents belonging to the most promising classes shown in Table 1 are being specifically investigated in CCRA. Each of them shows promise and caveats.
Nevertheless, the multifactorial pathogenesis of anemia in cancer, which can also change during the evolution of the disease, makes it unlikely that a single drug will be successful in the heterogeneous population of anemic cancer patients. Rather, an individualized holistic approach, including a thorough evaluation of nutritional status and, possibly, the combined use of more than one drug/supplement targeting different steps of erythropoiesis, should be implemented to provide an effective treatment that improves survival and quality of life of anemic cancer patients.
Author contributions
CB: Writing – original draft, Writing – review & editing. FB: Writing – review & editing. GiaM: Writing – review & editing. AV: Writing – review & editing. CC: Writing – review & editing. GioM: Writing – review & editing. DG: Writing – original draft, Writing – review & editing.
Funding
The author(s) declare financial support was received for the research, authorship, and/or publication of this article. Partially supported by the Italian Ministry of Health grant no. CO-2026-02361206 to DG.
Conflict of interest
DG has received fees as a consultant (Advisory Board) from Vifor Pharma, and Kedrion.
The remaining authors declare that the research was conducted in the absence of any commercial or financial relationships that could be construed as a potential conflict of interest.
The author(s) declared that they were an editorial board member of Frontiers, at the time of submission. This had no impact on the peer review process and the final decision.
Publisher’s note
All claims expressed in this article are solely those of the authors and do not necessarily represent those of their affiliated organizations, or those of the publisher, the editors and the reviewers. Any product that may be evaluated in this article, or claim that may be made by its manufacturer, is not guaranteed or endorsed by the publisher.
References
1. Ludwig H, Van Belle S, Barrett-Lee P, Birgegard G, Bokemeyer C, Gascon P, et al. The European Cancer Anaemia Survey (ECAS): a large, multinational, prospective survey defining the prevalence, incidence, and treatment of anaemia in cancer patients. Eur J Cancer. (2004) 40:2293–306. doi: 10.1016/j.ejca.2004.06.019
2. Saint A, Viotti J, Borchiellini D, Hoch B, Raimondi V, Hebert C, et al. Iron deficiency during first-line chemotherapy in metastatic cancers: a prospective epidemiological study. Support Care Cancer. (2020) 28:1639–47. doi: 10.1007/s00520-019-04938-3
3. Maccio A, Madeddu C, Gramignano G, Mulas C, Tanca L, Cherchi MC, et al. The role of inflammation, iron, and nutritional status in cancer-related anemia: results of a large, prospective, observational study. Haematologica. (2015) 100:124–32. doi: 10.3324/haematol.2014.112813
4. Gilreath JA, Rodgers GM. How I treat cancer-associated anemia. Blood. (2020) 136:801–13. doi: 10.1182/blood.2019004017
5. Abdel-Razeq H, Hashem H. Recent update in the pathogenesis and treatment of chemotherapy and cancer induced anemia. Crit Rev oncology/hematol. (2020) 145:102837. doi: 10.1016/j.critrevonc.2019.102837
6. Busti F, Marchi G, Ugolini S, Castagna A, Girelli D. Anemia and iron deficiency in cancer patients: role of iron replacement therapy. Pharm (Basel). (2018) 11(4):94. doi: 10.3390/ph11040094
7. Weiss G, Ganz T, Goodnough LT. Anemia of inflammation. Blood. (2019) 133:40–50. doi: 10.1182/blood-2018-06-856500
8. Camaschella C, Girelli D. The changing landscape of iron deficiency. Mol Aspects Med. (2020) 75:100861. doi: 10.1016/j.mam.2020.100861
9. Madeddu C, Neri M, Sanna E, Oppi S, Maccio A. Experimental drugs for chemotherapy- and cancer-related anemia. J Exp Pharmacol. (2021) 13:593–611. doi: 10.2147/JEP.S262349
10. Zhou Y, Zhang X, Wu X, Zhou Y, Zhang B, Liu X, et al. A prospective multicenter phase II study on the efficacy and safety of dasatinib in the treatment of metastatic gastrointestinal stromal tumors failed by imatinib and sunitinib and analysis of NGS in peripheral blood. Cancer Med. (2020) 9:6225–33. doi: 10.1002/cam4.3319
11. Siena S, Di Bartolomeo M, Raghav K, Masuishi T, Loupakis F, Kawakami H, et al. Trastuzumab deruxtecan (DS-8201) in patients with HER2-expressing metastatic colorectal cancer (DESTINY-CRC01): a multicentre, open-label, phase 2 trial. Lancet Oncol. (2021) 22:779–89. doi: 10.1016/S1470-2045(21)00086-3
12. Zhang T, Harrison MR, O’Donnell PH, Alva AS, Hahn NM, Appleman LJ, et al. A randomized phase 2 trial of pembrolizumab versus pembrolizumab and acalabrutinib in patients with platinum-resistant metastatic urothelial cancer. Cancer. (2020) 126:4485–97. doi: 10.1002/cncr.33067
13. Friedman CF, Proverbs-Singh TA, Postow MA. Treatment of the immune-related adverse effects of immune checkpoint inhibitors: A review. JAMA Oncol. (2016) 2:1346–53. doi: 10.1001/jamaoncol.2016.1051
14. Tchekmedyian NS. Anemia in cancer patients: significance, epidemiology, and current therapy. Oncol (Williston Park). (2002) 16:17–24.
15. Luporsi E, Turpin A, Massard V, Morin S, Chauffert B, Carnot A, et al. Iron deficiency in patients with cancer: a prospective cross-sectional study. BMJ Support Palliat Care. (2021). doi: 10.1136/bmjspcare-2021-002913
16. Groopman JE, Itri LM. Chemotherapy-induced anemia in adults: incidence and treatment. J Natl Cancer Inst. (1999) 91:1616–34. doi: 10.1093/jnci/91.19.1616
17. Lind M, Vernon C, Cruickshank D, Wilkinson P, Littlewood T, Stuart N, et al. The level of haemoglobin in anaemic cancer patients correlates positively with quality of life. Br J Cancer. (2002) 86:1243–9. doi: 10.1038/sj.bjc.6600247
18. Holzner B, Kemmler G, Greil R, Kopp M, Zeimet A, Raderer M, et al. The impact of hemoglobin levels on fatigue and quality of life in cancer patients. Ann Oncol. (2002) 13:965–73. doi: 10.1093/annonc/mdf122
19. Caro JJ, Salas M, Ward A, Goss G. Anemia as an independent prognostic factor for survival in patients with cancer: a systemic, quantitative review. Cancer. (2001) 91:2214–21. doi: 10.1002/(ISSN)1097-0142
20. Waters JS, O’Brien ME, Ashley S. Management of anemia in patients receiving chemotherapy. J Clin Oncol. (2002) 20:601–3. doi: 10.1200/JCO.2002.20.2.601
21. Aapro M, Beguin Y, Bokemeyer C, Dicato M, Gascon P, Glaspy J, et al. Management of anaemia and iron deficiency in patients with cancer: ESMO Clinical Practice Guidelines. Ann Oncol. (2018) 29:iv271. doi: 10.1093/annonc/mdy323
22. Parikh PM, Aggarwal S, Biswas G, Gulia S, Agarwala V, Basade M, et al. Practical clinical consensus guidelines for the management of cancer associated anemia in low- and middle-income countries. South Asian J Cancer. (2023) 12:93–9. doi: 10.1055/s-0043-1771445
23. Bohlius J, Bohlke K, Castelli R, Djulbegovic B, Lustberg MB, Martino M, et al. Management of cancer-associated anemia with erythropoiesis-stimulating agents: ASCO/ASH clinical practice guideline update. Blood Adv. (2019) 3:1197–210. doi: 10.1182/bloodadvances.2018030387
24. Busti F, Marchi G, Lira Zidanes A, Castagna A, Girelli D. Treatment options for anemia in the elderly. Transfus Apher Sci. (2019) 58:416–21. doi: 10.1016/j.transci.2019.06.018
25. Busti F, Marchi G, Vianello A, Girelli D. Practical implications of the 2019 Nobel Prize in Physiology or Medicine: from molecular adaptation to hypoxia to novel anti-anemic drugs in the clinic. Intern Emerg Med. (2020) 15:911–5. doi: 10.1007/s11739-020-02417-w
26. Cappellini MD, Marcon A, Fattizzo B, Motta I. Innovative treatments for rare anemias. Hemasphere. (2021) 5:e576. doi: 10.1097/HS9.0000000000000576
27. Cata JP, Wang H, Gottumukkala V, Reuben J, Sessler DI. Inflammatory response, immunosuppression, and cancer recurrence after perioperative blood transfusions. Br J Anaesth. (2013) 110:690–701. doi: 10.1093/bja/aet068
28. Goodnough LT. Risks of blood transfusion. Anesthesiol Clin North Am. (2005) 23:241–52, v. doi: 10.1016/j.atc.2004.07.004
29. Acheson AG, Brookes MJ, Spahn DR. Effects of allogeneic red blood cell transfusions on clinical outcomes in patients undergoing colorectal cancer surgery: a systematic review and meta-analysis. Ann Surg. (2012) 256:235–44. doi: 10.1097/SLA.0b013e31825b35d5
30. Luan H, Ye F, Wu L, Zhou Y, Jiang J. Perioperative blood transfusion adversely affects prognosis after resection of lung cancer: a systematic review and a meta-analysis. BMC Surg. (2014) 14:34. doi: 10.1186/1471-2482-14-34
31. Prescott LS, Aloia TA, Brown AJ, Taylor JS, Munsell MF, Sun CC, et al. Perioperative blood transfusion in gynecologic oncology surgery: analysis of the National Surgical Quality Improvement Program Database. Gynecol Oncol. (2015) 136:65–70. doi: 10.1016/j.ygyno.2014.11.009
32. Carson JL, Guyatt G, Heddle NM, Grossman BJ, Cohn CS, Fung MK, et al. Clinical practice guidelines from the AABB: red blood cell transfusion thresholds and storage. JAMA: J Am Med Assoc. (2016) 316:2025–35. doi: 10.1001/jama.2016.9185
33. Park SH, Nam E, Bang SM, Cho EK, Shin DB, Lee JH. A randomized trial of anemia correction with two different hemoglobin targets in the first-line chemotherapy of advanced gastric cancer. Cancer Chemother Pharmacol. (2008) 62:1–9. doi: 10.1007/s00280-007-0561-1
34. Webert KE, Cook RJ, Couban S, Carruthers J, Lee KA, Blajchman MA, et al. A multicenter pilot-randomized controlled trial of the feasibility of an augmented red blood cell transfusion strategy for patients treated with induction chemotherapy for acute leukemia or stem cell transplantation. Transfusion. (2008) 48:81–91. doi: 10.1111/j.1537-2995.2007.01485.x
35. Jansen AJ, Caljouw MA, Hop WC, van Rhenen DJ, Schipperus MR. Feasibility of a restrictive red-cell transfusion policy for patients treated with intensive chemotherapy for acute myeloid leukaemia. Transfus Med. (2004) 14:33–8. doi: 10.1111/j.0958-7578.2004.00477.x
36. Prescott LS, Taylor JS, Lopez-Olivo MA, Munsell MF, VonVille HM, Lairson DR, et al. How low should we go: A systematic review and meta-analysis of the impact of restrictive red blood cell transfusion strategies in oncology. Cancer Treat Rev. (2016) 46:1–8. doi: 10.1016/j.ctrv.2016.03.010
37. Carson JL, Stanworth SJ, Guyatt G, Valentine S, Dennis J, Bakhtary S, et al. Red blood cell transfusion: 2023 AABB international guidelines. JAMA: J Am Med Assoc. (2023) 330:1892–902. doi: 10.1001/jama.2023.12914
38. Ludwig H, Muldur E, Endler G, Hubl W. Prevalence of iron deficiency across different tumors and its association with poor performance status, disease status and anemia. Ann Oncol. (2013) 24:1886–92. doi: 10.1093/annonc/mdt118
40. Goodnough LT, Nemeth E, Ganz T. Detection, evaluation, and management of iron-restricted erythropoiesis. Blood. (2010) 116:4754–61. doi: 10.1182/blood-2010-05-286260
41. Auerbach M, Ballard H, Trout JR, McIlwain M, Ackerman A, Bahrain H, et al. Intravenous iron optimizes the response to recombinant human erythropoietin in cancer patients with chemotherapy-related anemia: a multicenter, open-label, randomized trial. J Clin Oncol. (2004) 22:1301–7. doi: 10.1200/JCO.2004.08.119
42. Pedrazzoli P, Farris A, Del Prete S, Del Gaizo F, Ferrari D, Bianchessi C, et al. Randomized trial of intravenous iron supplementation in patients with chemotherapy-related anemia without iron deficiency treated with darbepoetin alpha. J Clin Oncol. (2008) 26:1619–25. doi: 10.1200/JCO.2007.12.2051
43. Mhaskar R, Wao H, Miladinovic B, Kumar A, Djulbegovic B. The role of iron in the management of chemotherapy-induced anemia in cancer patients receiving erythropoiesis-stimulating agents. Cochrane Database Syst Rev. (2016) 2:Cd009624. doi: 10.1002/14651858.CD009624.pub2
44. Gafter-Gvili A, Rozen-Zvi B, Vidal L, Leibovici L, Vansteenkiste J, Gafter U, et al. Intravenous iron supplementation for the treatment of chemotherapy-induced anaemia - systematic review and meta-analysis of randomised controlled trials. Acta Oncol. (2013) 52:18–29. doi: 10.3109/0284186X.2012.702921
45. Girelli D, Ugolini S, Busti F, Marchi G, Castagna A. Modern iron replacement therapy: clinical and pathophysiological insights. Int J Hematol. (2018) 107:16–30. doi: 10.1007/s12185-017-2373-3
46. Rodgers GM, Gilreath JA. The role of intravenous iron in the treatment of anemia associated with cancer and chemotherapy. Acta Haematol. (2019) 142:13–20. doi: 10.1159/000496967
47. Abdel-Razeq H, Saadeh SS, Malhis R, Yasser S, Abdulelah H, Eljaber R, et al. Treatment of anemia in cancer patients undergoing chemotherapy with intravenous ferric carboxymaltose without erythropoiesis-stimulating agents. Ther Adv Med Oncol. (2020) 12:1758835920953292. doi: 10.1177/1758835920953292
48. Makharadze T, Boccia R, Krupa A, Blackman N, Henry DH, Gilreath JA. Efficacy and safety of ferric carboxymaltose infusion in reducing anemia in patients receiving chemotherapy for nonmyeloid Malignancies: A randomized, placebo-controlled study (IRON-CLAD). Am J Hematol. (2021) 96:1639–46. doi: 10.1002/ajh.26376
49. Gluszak C, de Vries-Brilland M, Seegers V, Baroin C, Kieffer H, Delva R, et al. Impact of iron-deficiency management on quality of life in patients with cancer: A prospective cohort study (CAMARA study). Oncologist. (2022) 27:328–33. doi: 10.1093/oncolo/oyac005
50. Miller LL, Miller SC, Torti SV, Tsuji Y, Torti FM. Iron-independent induction of ferritin H chain by tumor necrosis factor. Proc Natl Acad Sci U S A. (1991) 88:4946–50. doi: 10.1073/pnas.88.11.4946
51. Torti FM, Torti SV. Regulation of ferritin genes and protein. Blood. (2002) 99:3505–16. doi: 10.1182/blood.V99.10.3505
52. Network NCC. NCCN Clinical Practice Guidelines in Oncology. Hematopoietic growth factors.2024 . Available online at: https://www.nccn.org/guidelines/guidelines-detail?category=3&id=1493.
53. Girelli D, Nemeth E, Swinkels DW. Hepcidin in the diagnosis of iron disorders. Blood. (2016) 127:2809–13. doi: 10.1182/blood-2015-12-639112
54. Steensma DP, Sasu BJ, Sloan JA, Tomita DK, Loprinzi CL. Serum hepcidin levels predict response to intravenous iron and darbepoetin in chemotherapy-associated anemia. Blood. (2015) 125:3669–71. doi: 10.1182/blood-2015-03-636407
55. Jang JH, Kim Y, Park S, Kim K, Kim SJ, Kim WS, et al. Efficacy of intravenous iron treatment for chemotherapy-induced anemia: A prospective Phase II pilot clinical trial in South Korea. PloS Med. (2020) 17:e1003091. doi: 10.1371/journal.pmed.1003091
56. Diepeveen LE, Laarakkers CMM, Martos G, Pawlak ME, Uğuz FF, Verberne K, et al. Provisional standardization of hepcidin assays: creating a traceability chain with a primary reference material, candidate reference method and a commutable secondary reference material. Clin Chem Lab medicine: CCLM/FESCC. (2019) 57:864–72. doi: 10.1515/cclm-2018-0783
57. van der Vorm LN, Hendriks JCM, Laarakkers CM, Klaver S, Armitage AE, Bamberg A, et al. Toward worldwide hepcidin assay harmonization: identification of a commutable secondary reference material. Clin Chem. (2016) 62:993–1001. doi: 10.1373/clinchem.2016.256768
58. Littlewood TJ, Bajetta E, Nortier JW, Vercammen E, Rapoport B. Effects of epoetin alfa on hematologic parameters and quality of life in cancer patients receiving nonplatinum chemotherapy: results of a randomized, double-blind, placebo-controlled trial. J Clin Oncol. (2001) 19:2865–74. doi: 10.1200/JCO.2001.19.11.2865
59. Vansteenkiste J, Pirker R, Massuti B, Barata F, Font A, Fiegl M, et al. Double-blind, placebo-controlled, randomized phase III trial of darbepoetin alfa in lung cancer patients receiving chemotherapy. J Natl Cancer Inst. (2002) 94:1211–20. doi: 10.1093/jnci/94.16.1211
60. Tonia T, Mettler A, Robert N, Schwarzer G, Seidenfeld J, Weingart O, et al. Erythropoietin or darbepoetin for patients with cancer. Cochrane Database Syst Rev. (2012) 12:CD003407. doi: 10.1002/14651858.CD003407.pub5
61. Trotta F, Belleudi V, Fusco D, Amato L, Mecozzi A, Mayer F, et al. Comparative effectiveness and safety of erythropoiesis-stimulating agents (biosimilars vs originators) in clinical practice: a population-based cohort study in Italy. BMJ Open. (2017) 7:e011637. doi: 10.1136/bmjopen-2016-011637
62. Bohlius J, Wilson J, Seidenfeld J, Piper M, Schwarzer G, Sandercock J, et al. Recombinant human erythropoietins and cancer patients: updated meta-analysis of 57 studies including 9353 patients. J Natl Cancer Inst. (2006) 98:708–14. doi: 10.1093/jnci/djj189
63. Bohlius J, Schmidlin K, Brillant C, Schwarzer G, Trelle S, Seidenfeld J, et al. Recombinant human erythropoiesis-stimulating agents and mortality in patients with cancer: a meta-analysis of randomised trials. Lancet. (2009) 373:1532–42. doi: 10.1016/S0140-6736(09)60502-X
64. Pradeep S, Huang J, Mora EM, Nick AM, Cho MS, Wu SY, et al. Erythropoietin stimulates tumor growth via ephB4. Cancer Cell. (2015) 28:610–22. doi: 10.1016/j.ccell.2015.09.008
65. Soderberg SS, Karlsson G, Karlsson S. Complex and context dependent regulation of hematopoiesis by TGF-beta superfamily signaling. Ann N Y Acad Sci. (2009) 1176:55–69. doi: 10.1111/j.1749-6632.2009.04569.x
66. Iancu-Rubin C, Mosoyan G, Wang J, Kraus T, Sung V, Hoffman R. Stromal cell-mediated inhibition of erythropoiesis can be attenuated by Sotatercept (ACE-011), an activin receptor type II ligand trap. Exp Hematol. (2013) 41:155–66 e17. doi: 10.1016/j.exphem.2012.12.002
67. Guerra A, Oikonomidou PR, Sinha S, Zhang J, Lo Presti V, Hamilton CR, et al. Lack of Gdf11 does not improve anemia or prevent the activity of RAP-536 in a mouse model of β-thalassemia. Blood. (2019) 134:568–72. doi: 10.1182/blood.2019001057
68. Camaschella C. GDF11 is not the target of luspatercept. Blood. (2019) 134:500–1. doi: 10.1182/blood.2019001983
69. Dussiot M, Maciel TT, Fricot A, Chartier C, Negre O, Veiga J, et al. An activin receptor IIA ligand trap corrects ineffective erythropoiesis in beta-thalassemia. Nat Med. (2014) 20:398–407. doi: 10.1038/nm.3468
70. Suragani RN, Cawley SM, Li R, Wallner S, Alexander MJ, Mulivor AW, et al. Modified activin receptor IIB ligand trap mitigates ineffective erythropoiesis and disease complications in murine beta-thalassemia. Blood. (2014) 123:3864–72. doi: 10.1182/blood-2013-06-511238
71. Carrancio S, Markovics J, Wong P, Leisten J, Castiglioni P, Groza MC, et al. An activin receptor IIA ligand trap promotes erythropoiesis resulting in a rapid induction of red blood cells and haemoglobin. Br J Haematol. (2014) 165:870–82. doi: 10.1111/bjh.12838
72. Pearsall RS, Canalis E, Cornwall-Brady M, Underwood KW, Haigis B, Ucran J, et al. A soluble activin type IIA receptor induces bone formation and improves skeletal integrity. Proc Natl Acad Sci U S A. (2008) 105:7082–7. doi: 10.1073/pnas.0711263105
73. Ruckle J, Jacobs M, Kramer W, Pearsall AE, Kumar R, Underwood KW, et al. Single-dose, randomized, double-blind, placebo-controlled study of ACE-011 (ActRIIA-IgG1) in postmenopausal women. J Bone Miner Res. (2009) 24:744–52. doi: 10.1359/jbmr.081208
74. Raje N, Vallet S. Sotatercept, a soluble activin receptor type 2A IgG-Fc fusion protein for the treatment of anemia and bone loss. Curr Opin Mol Ther. (2010) 12:586–97.
75. Attie KM, Allison MJ, McClure T, Boyd IE, Wilson DM, Pearsall AE, et al. A phase 1 study of ACE-536, a regulator of erythroid differentiation, in healthy volunteers. Am J Hematol. (2014) 89:766–70. doi: 10.1002/ajh.23732
76. Cappellini MD, Viprakasit V, Taher AT, Georgiev P, Kuo KHM, Coates T, et al. A phase 3 trial of luspatercept in patients with transfusion-dependent β-thalassemia. N Engl J Med. (2020) 382:1219–31. doi: 10.1056/NEJMoa1910182
77. Fenaux P, Kiladjian JJ, Platzbecker U. Luspatercept for the treatment of anemia in myelodysplastic syndromes and primary myelofibrosis. Blood. (2019) 133:790–4. doi: 10.1182/blood-2018-11-876888
78. Kubasch AS, Fenaux P, Platzbecker U. Development of luspatercept to treat ineffective erythropoiesis. Blood Adv. (2021) 5:1565–75. doi: 10.1182/bloodadvances.2020002177
79. Fenaux P, Platzbecker U, Mufti GJ, Garcia-Manero G, Buckstein R, Santini V, et al. Luspatercept in patients with lower-risk myelodysplastic syndromes. New Engl J Med. (2020) 382:140–51. doi: 10.1056/NEJMoa1908892
80. Platzbecker U, Della Porta MG, Santini V, Zeidan AM, Komrokji RS, Shortt J, et al. Efficacy and safety of luspatercept versus epoetin alfa in erythropoiesis-stimulating agent-naive, transfusion-dependent, lower-risk myelodysplastic syndromes (COMMANDS): interim analysis of a phase 3, open-label, randomised controlled trial. Lancet. (2023) 402:373–85. doi: 10.1016/S0140-6736(23)00874-7
81. Komrokji RS, Platzbecker U, Fenaux P, Zeidan AM, Garcia-Manero G, Mufti GJ, et al. Luspatercept for myelodysplastic syndromes/myeloproliferative neoplasm with ring sideroblasts and thrombocytosis. Leukemia. (2022) 36:1432–5. doi: 10.1038/s41375-022-01521-4
82. Gerds AT, Harrison C, Kiladjian JJ, Mesa R, Vannucchi A, Komrokji RS, et al. S167: efficacy and safety of luspatercept for the treatment of anemia in patients with myelofibrosis: results from the ace-536-mf-001 study. Hemasphere. (2023) 7:e0882611. doi: 10.1097/01.HS9.0000967580.08826.11
83. Raftopoulos H, Laadem A, Hesketh PJ, Goldschmidt J, Gabrail N, Osborne C, et al. Sotatercept (ACE-011) for the treatment of chemotherapy-induced anemia in patients with metastatic breast cancer or advanced or metastatic solid tumors treated with platinum-based chemotherapeutic regimens: results from two phase 2 studies. Support Care Cancer. (2016) 24:1517–25. doi: 10.1007/s00520-015-2929-9
84. Nemeth E, Ganz T. Hepcidin and iron in health and disease. Annu Rev Med. (2023) 74:261–77. doi: 10.1146/annurev-med-043021-032816
85. Poli M, Asperti M, Ruzzenenti P, Regoni M, Arosio P. Hepcidin antagonists for potential treatments of disorders with hepcidin excess. Front Pharmacol. (2014) 5:86. doi: 10.3389/fphar.2014.00086
86. Sasu BJ, Cooke KS, Arvedson TL, Plewa C, Ellison AR, Sheng J, et al. Antihepcidin antibody treatment modulates iron metabolism and is effective in a mouse model of inflammation-induced anemia. Blood. (2010) 115:3616–24. doi: 10.1182/blood-2009-09-245977
87. Schwoebel F, van Eijk LT, Zboralski D, Sell S, Buchner K, Maasch C, et al. The effects of the anti-hepcidin Spiegelmer NOX-H94 on inflammation-induced anemia in cynomolgus monkeys. Blood. (2013) 121:2311–5. doi: 10.1182/blood-2012-09-456756
88. Vadhan-Raj S, Abonour R, Goldman JW, Smith DA, Slapak CA, Ilaria RL Jr., et al. A first-in-human phase 1 study of a hepcidin monoclonal antibody, LY2787106, in cancer-associated anemia. J Hematol Oncol. (2017) 10:73. doi: 10.1186/s13045-017-0427-x
89. van Eijk LT, John AS, Schwoebel F, Summo L, Vauleon S, Zollner S, et al. Effect of the antihepcidin Spiegelmer lexaptepid on inflammation-induced decrease in serum iron in humans. Blood. (2014) 124:2643–6. doi: 10.1182/blood-2014-03-559484
90. Boyce M, Warrington S, Cortezi B, Zollner S, Vauleon S, Swinkels DW, et al. Safety, pharmacokinetics and pharmacodynamics of the anti-hepcidin Spiegelmer lexaptepid pegol in healthy subjects. Br J Pharmacol. (2016) 173:1580–8. doi: 10.1111/bph.13433
91. Georgiev P, Lazaroiu M, Ocroteala L, Grudeva-Popova J, Gheorghita E, Vasilica M, et al. Abstract 3847: The anti-hepcidin Spiegelmer® Lexaptepid Pegol (NOX-H94) as treatment of anemia of chronic disease in patients with multiple myeloma, low grade lymphoma, and CLL: A phase II pilot study. Cancer Res. (2014) 74:3847. doi: 10.1158/1538-7445.AM2014-3847
92. Camaschella C, Nai A, Silvestri L. Iron metabolism and iron disorders revisited in the hepcidin era. Haematologica. (2020) 105:260–72. doi: 10.3324/haematol.2019.232124
93. Muckenthaler MU, Rivella S, Hentze MW, Galy B. A red carpet for iron metabolism. Cell. (2017) 168:344–61. doi: 10.1016/j.cell.2016.12.034
94. Poli M, Girelli D, Campostrini N, Maccarinelli F, Finazzi D, Luscieti S, et al. Heparin: a potent inhibitor of hepcidin expression in vitro and in vivo. Blood. (2011) 117:997–1004. doi: 10.1182/blood-2010-06-289082
95. Poli M, Asperti M, Naggi A, Campostrini N, Girelli D, Corbella M, et al. Glycol-split nonanticoagulant heparins are inhibitors of hepcidin expression in vitro and in vivo. Blood. (2014) 123:1564–73. doi: 10.1182/blood-2013-07-515221
96. Poli M, Asperti M, Ruzzenenti P, Mandelli L, Campostrini N, Martini G, et al. Oversulfated heparins with low anticoagulant activity are strong and fast inhibitors of hepcidin expression in vitro and in vivo. Biochem Pharmacol. (2014) 92:467–75. doi: 10.1016/j.bcp.2014.09.007
97. Li L, Wang X, Zhang H, Chen Q, Cui H. Low anticoagulant heparin-iron complex targeting inhibition of hepcidin ameliorates anemia of chronic disease in rodents. Eur J Pharmacol. (2021) 897:173958. doi: 10.1016/j.ejphar.2021.173958
98. Petzer V, Tymoszuk P, Asshoff M, Carvalho J, Papworth J, Deantonio C, et al. A fully human anti-BMP6 antibody reduces the need for erythropoietin in rodent models of the anemia of chronic disease. Blood. (2020) 136:1080–90. doi: 10.1182/blood.2019004653
99. Yu PB, Hong CC, Sachidanandan C, Babitt JL, Deng DY, Hoyng SA, et al. Dorsomorphin inhibits BMP signals required for embryogenesis and iron metabolism. Nat Chem Biol. (2008) 4:33–41. doi: 10.1038/nchembio.2007.54
100. Witcher DR, Leung D, Hill KA, De Rosa DC, Xu J, Manetta J, et al. LY2928057, an antibody targeting ferroportin, is a potent inhibitor of hepcidin activity and increases iron mobilization in normal cynomolgus monkeys. Blood. (2013) 122:3433–. doi: 10.1182/blood.V122.21.3433.3433
101. Fung E, Sugianto P, Hsu J, Damoiseaux R, Ganz T, Nemeth E. High-throughput screening of small molecules identifies hepcidin antagonists. Mol Pharmacol. (2013) 83:681–90. doi: 10.1124/mol.112.083428
102. Asshoff M, Petzer V, Warr MR, Haschka D, Tymoszuk P, Demetz E, et al. Momelotinib inhibits ACVR1/ALK2, decreases hepcidin production, and ameliorates anemia of chronic disease in rodents. Blood. (2017) 129:1823–30. doi: 10.1182/blood-2016-09-740092
103. Tefferi A, Pardanani A, Gangat N. Momelotinib (JAK1/JAK2/ACVR1 inhibitor): mechanism of action, clinical trial reports, and therapeutic prospects beyond myelofibrosis. Haematologica. (2023) 108:2919–32. doi: 10.3324/haematol.2022.282612
104. Duminuco A, Chifotides HT, Giallongo S, Giallongo C, Tibullo D, Palumbo GA. ACVR1: A novel therapeutic target to treat anemia in myelofibrosis. Cancers (Basel). (2023) 16(1):154. doi: 10.3390/cancers16010154
105. Sagar P, Angmo S, Sandhir R, Rishi V, Yadav H, Singhal NK. Effect of hepcidin antagonists on anemia during inflammatory disorders. Pharmacol Ther. (2021) 226:107877. doi: 10.1016/j.pharmthera.2021.107877
106. Semenza GL. Involvement of oxygen-sensing pathways in physiologic and pathologic erythropoiesis. Blood. (2009) 114:2015–9. doi: 10.1182/blood-2009-05-189985
107. Renassia C, Peyssonnaux C. New insights into the links between hypoxia and iron homeostasis. Curr Opin Hematol. (2019) 26:125–30. doi: 10.1097/MOH.0000000000000494
108. Li M, Lan J, Dong F, Duan P. Effectiveness of hypoxia-induced factor prolyl hydroxylase inhibitor for managing anemia in chronic kidney disease: a systematic review and meta-analysis. Eur J Clin Pharmacol. (2021) 77:491–507. doi: 10.1007/s00228-020-03037-1
109. Lok CN, Ponka P. Identification of a hypoxia response element in the transferrin receptor gene. J Biol Chem. (1999) 274:24147–52. doi: 10.1074/jbc.274.34.24147
110. Qian ZM, Wu XM, Fan M, Yang L, Du F, Yung WH, et al. Divalent metal transporter 1 is a hypoxia-inducible gene. J Cell Physiol. (2011) 226:1596–603. doi: 10.1002/jcp.22485
111. Taylor M, Qu A, Anderson ER, Matsubara T, Martin A, Gonzalez FJ, et al. Hypoxia-inducible factor-2α mediates the adaptive increase of intestinal ferroportin during iron deficiency in mice. Gastroenterology. (2011) 140:2044–55. doi: 10.1053/j.gastro.2011.03.007
112. Pergola PE, Spinowitz BS, Hartman CS, Maroni BJ, Haase VH. Vadadustat, a novel oral HIF stabilizer, provides effective anemia treatment in nondialysis-dependent chronic kidney disease. Kidney Int. (2016) 90:1115–22. doi: 10.1016/j.kint.2016.07.019
113. Chen N, Hao C, Liu BC, Lin H, Wang C, Xing C, et al. Roxadustat treatment for anemia in patients undergoing long-term dialysis. New Engl J Med. (2019) 381:1011–22. doi: 10.1056/NEJMoa1901713
114. Chen N, Hao C, Peng X, Lin H, Yin A, Hao L, et al. Roxadustat for anemia in patients with kidney disease not receiving dialysis. New Engl J Med. (2019) 381:1001–10. doi: 10.1056/NEJMoa1813599
115. Henry DH, Glaspy J, Harrup R, Mittelman M, Zhou A, Carraway HE, et al. Roxadustat for the treatment of anemia in patients with lower-risk myelodysplastic syndrome: Open-label, dose-selection, lead-in stage of a phase 3 study. Am J Hematol. (2022) 97:174–84. doi: 10.1002/ajh.26397
116. Mittelman M, Henry DH, Glaspy J, Tombak A, Harrup RA, Madry K, et al. Efficacy and safety of roxadustat for treatment of anemia in patients with lower-risk myelodysplastic syndrome (LR-MDS) with low red blood cell (RBC) transfusion burden: results of phase III matterhorn study. Blood. (2023) 142:195. doi: 10.1182/blood-2023-180749
Keywords: anemia, activin receptor ligand traps, cancer, chemotherapy, hepcidin, iron, therapy, prolyl hydroxylase inhibitors
Citation: Bozzini C, Busti F, Marchi G, Vianello A, Cerchione C, Martinelli G and Girelli D (2024) Anemia in patients receiving anticancer treatments: focus on novel therapeutic approaches. Front. Oncol. 14:1380358. doi: 10.3389/fonc.2024.1380358
Received: 01 February 2024; Accepted: 19 March 2024;
Published: 02 April 2024.
Edited by:
Mohamed A. Yassin, Qatar University, QatarReviewed by:
Yelena Ginzburg, Icahn School of Medicine at Mount Sinai, United StatesMoshe Mittelman, Tel Aviv Sourasky Medical Center, Israel
Laura Silvestri, San Raffaele Scientific Institute (IRCCS), Italy
Copyright © 2024 Bozzini, Busti, Marchi, Vianello, Cerchione, Martinelli and Girelli. This is an open-access article distributed under the terms of the Creative Commons Attribution License (CC BY). The use, distribution or reproduction in other forums is permitted, provided the original author(s) and the copyright owner(s) are credited and that the original publication in this journal is cited, in accordance with accepted academic practice. No use, distribution or reproduction is permitted which does not comply with these terms.
*Correspondence: Domenico Girelli, domenico.girelli@univr.it