- 1Key Laboratory of Carcinogenesis and Translational Research (Ministry of Education/Beijing), Department of Radiation Oncology, Peking University Cancer Hospital & Institute, Beijing, China
- 2Department of Otolaryngology, Head and Neck Surgery, Peking University People’s Hospital, Beijing, China
- 3Institute of Medical Technology, Peking University Health Science Center, Beijing, China
Purpose: Repeated cone-beam CT (CBCT) scans for image-guided radiotherapy (IGRT) increase the health risk of radiation-induced malignancies. Patient-enrolled studies to optimize scan protocols are inadequate. We proposed a virtual clinical trial-based approach to evaluate projection-reduced low-dose CBCT for IGRT.
Materials and methods: A total of 71 patients were virtually enrolled with 26 head, 23 thorax and 22 pelvis scans. Projection numbers of full-dose CBCT scans were reduced to 1/2, 1/4, and 1/8 of the original to simulate low-dose scans. Contrast-to-noise ratio (CNR) values in fat and muscle were measured in the full-dose and low-dose images. CBCT images were registered to planning CT to derive 6-degree-of-freedom couch shifts. Registration errors were statistically analyzed with the Wilcoxon paired signed-rank test.
Results: As projection numbers were reduced, CNR values descended and the magnitude of registration errors increased. The mean CNR values of full-dose and half-dose CBCT were >3.0. For full-dose and low-dose CBCT (i.e. 1/2, 1/4 and 1/8 full-dose), the mean registration errors were< ± 0.4 mm in translational directions (LAT, LNG, VRT) and ±0.2 degree in rotational directions (Pitch, Roll, Yaw); the mean magnitude of registration errors were< 1 mm in translation and< 0.5 degree in rotation. The couch shift differences between full-dose and low-dose CBCT were not statistically significant (p>0.05) in all the directions.
Conclusion: The results indicate that while the impact of dose-reduction on CBCT couch shifts is not significant, the impact on CNR values is significant. Further validation on optimizing CBCT imaging dose is required.
1 Introduction
On-board cone-beam computed tomography (CBCT) plays a considerable role in modern radiotherapy, as it is able to provide three-dimensional anatomy images with high-resolution. The use of CBCT facilitates patient positioning verification and target localization by anatomy alignment with reference to planning CT images, therefore substantially improving the accuracy, safety and effectiveness of image-guided radiotherapy (IGRT) (1–3). Despite the numerous advantages, CBCT comes at increased x-ray exposure compared with 2D radiographic imaging (4, 5). Since patients mostly receive recurrent CBCT scans over the entire treatment course, the health risk of imaging radiation induced by CBCT has raised serious concerns (6–11). For instance, it was estimated by Zhou et al. (8) that the average lifetime risk of cancer attributed to cumulative imaging doses per 100,000 individuals was 78 for brain cancer, 271 for lung cancer, and 510 for leukemia. A recent international survey on imaging practices in radiotherapy (12) shows that most centers used vendor-provided CBCT protocols in routine practice and less than 50% optimize the protocols to some extent. Growing efforts have been made to reduce imaging dose, and several strategies are recommended (4, 13, 14). A common and clinically available strategy of dose reduction is to reduce x-ray exposure via three techniques. The first technique is to use a short-arc scan over about 200 degrees instead of a 360-degree full-arc scan. The data acquired over short arcs are sufficient to reconstruct images of small objects, and therefore short-arc configuration has become the default for head-and-neck and pediatric protocols. The second is to decrease tube output (mAs) so as to reduce incident x-ray photons per projection, and the third is to increase angular separation to reduce exposure frequency. Several studies demonstrate that reduced exposure leads to CBCT image quality degradation. Wood et al. (15) evaluated mAs reduction on a pelvis phantom and made size-based recommendation of mAs settings. Yan et al. (16) investigated the relationship between the image quality and imaging dose and established a mAs-dependent empirical function. Lu et al. (17) evaluated the effect of reducing projection number on image quality and registration accuracy, concluding the projection-number reduction technique could achieve uncompromised registration accuracy under conscientious care. Similarly, Men et al. (18) evaluated the image quality, dose and registration errors acquired with different CBCT angular separations, indicating that CBCT images with fewer projections impair image quality and registration accuracy. Olch et al. (19) compared the image quality and registration accuracy between kV-pair imaging and low-dose CBCT acquired with reduced mAs and projections. The study demonstrates that CBCT dose can be reduced to a level comparable to kV-pair imaging with similar or enhanced patient positioning accuracy.
It is important to note that all the aforementioned studies are all phantom-based without any patient-involved validation. To our knowledge, there were only two relevant clinical study on low-dose CBCT for IGRT. The first study was by Alcorn et al. (20), which was an international study to evaluate the feasibility of a pre-defined low-dose CBCT protocol in pediatrics that received central neural system radiotherapy. The other study was by Bryce-Atkinson et al. (21) using CBCT scans from 7 pediatric patients to simulate low-dose scans by either adding noise or halving the number of projections. Due to the limited patient category, imaging site and cohort size, further validation is required. To this end, this study aims to comprehensively evaluate the image quality, image dose, and registration accuracy of CBCT images reconstructed using projections acquired with different combinations of angular range. The key innovation and highlight are: 1) we used a virtual clinical trial-based approach retrospectively enrolling a total of 71 real patients, and 2) we evaluated the clinical viability of low-dose CBCT for head, thorax and pelvis IGRT.
2 Materials and methods
2.1 Patient enrollment and data acquisition
A total of 71 patients treated at our institution (Peking University Cancer Hospital, China) were retrospectively enrolled under the approval of the institutional review board. The patients were all adults (age ranging from 27 to 68) without any metal implant and were treated on either an Edge linac or VitalBeam linac (Varian Medical Systems, Palo Alto, USA) from March 2022 to July 2022. The patients were categorized into three groups according to imaging sites, i.e., 26 head scan patients (10 with brain metastasis, 9 with nasopharyngeal carcinoma and 7 with glioma), 23 thorax scan patients (all with lung cancer) and 22 pelvis scan patients (9 with cervical cancer, 9 with rectal cancer and 4 with liver cancer). For the head scan group, patients were all immobilized with the open-face Double-Shell Positioning System (MacroMedics, Belgium) (22). For the thorax scan group, 15 patients were immobilized with thermoplastic and the other 8 with vacuum bag cushions. For the pelvis scan group, 4 liver patients were immobilized with ZiFix™ abdominal compression belt (Qfix, USA).
The pertinent imaging protocols were listed in Table 1 (23). For each patient, the raw scan data of the first CBCT scan was off-line retrieved from the linac imaging node. The CBCT imaging dose was defined in weighted CT dose index (CTDIw), and was recalibrated previous to this study with the aid of on-site Varian service team.
2.2 Generation of projection-reduced low-dose CBCT
For each clinical CBCT scan, three low-dose scans were derived by picking the first projection of every two, four and eight projections to simulate 1/2, 1/4 and 1/8 the clinical sampling rate. This data resampling approach was to simulate a virtual clinical trial, in which a patient was simultaneously scanned at four dose levels, i.e., default (full-dose), 1/2, 1/4, and 1/8 the full dose levels. Therefore, the scan series were referred to as S1, S1/2, S1/4, and S1/8, respectively.
All the scan data were preprocessed and reconstructed in MATLAB R2022b (Mathworks, USA) using TIGRE-VarianCBCT, a validated open-source toolkit for on-board CBCT imaging (24). The reconstruction algorithm was FDK (Feldkamp, Davis and Kress), which is identical to the algorithm used in the current state of clinical practice.
2.3 Impact evaluation
2.3.1 Soft tissue contrast
The soft tissue contrast was a key aspect to reflect image quality. Herein, we adopted the muscle and fat contrast-to-noise (CNR) proposed by Bryce-Atkinson et al. (21) to define the soft-tissue contrast in Equation 1 as
where M and σ were the mean HU value and standard deviation over the selected region-of-interest (ROI). In this study, the soft tissue contrast performance was defined as acceptable if the CNR exceeded 3 as per the Rose criterion (25), in line with the study by Bryce-Atkinson et al. (21).
For the head scan patients, the ROI was defined at the nasopharynx level: lateral pterygoid was selected as the muscle region, and the subcutaneous fat was fat region, as shown in Figure 2A. For the thorax and pelvis scan patients, subcutaneous fat and muscle regions were selected as shown in Figures 2B, C.
2.3.2 Relative registration errors
The CBCT images of different dose levels were rigidly registered to planning CT to acquire couch shifts. The registration was performed in 3D Slicer (version 5.2.1) (26), open source software application for medical image analysis. While 3D Slicer was not intended for clinical use, the key reason we used it was that the registration algorithm (mutual information based rigid registration) in 3D Slicer was identical with the clinical system. The CBCT-to-CT registration was rigid and in 6-degree-of-freedom (6DoF), i.e., three translational directions (VRT, LAT and LNG) and three rotational directions (Yaw, Pitch and Roll). The registration result of the full-dose level (S1) was used as gold standard benchmark, and registration accuracy between the low-dose CBCT scans and the respective benchmark were compared to derive relative registration errors in each direction. The magnitude of the relative registration errors were calculated in Equations 2, 3 as
where ε was the relative registration error, and Tx and Rx were the magnitudes of translational and rotational relative errors respectively.
2.3.3 Statistical analysis
The mean, standard deviation, 25–75% percentile (Q1-Q3), minimum and maximum values over the CNR results and relative registration errors were calculated. In addition, the 6DoF couch shifts acquired by registration to planning-CT via different CBCT image series were compared. The couch shifts of S1/2, S1/4 and S1/8 were paired with the full-dose method in each direction and then tested with the Wilcoxon paired signed-rank test. In this study, data analysis was performed in OriginPro (version 2021a, OriginLab, USA), and p-value< 0.05 was considered statistically significant.
3 Results
Figure 1 shows representative reconstructed images of real patients that received head (a-row), thorax (b-row) and pelvis (c-row) scans respectively with the default (S1) and projection-reduced protocols (S1/2, S1/4, S1/8). As we reduced the projection number, image quality deteriorated as the dose was lowered. Compared with the full-dose reference images (left-column in Figure 1), image noise and strike artifacts in dose-reduced protocols became obvious, which were induced by increased angular separations. While image details were blurred, the loss of soft-tissue contrast was relatively greater than that of bony structures.
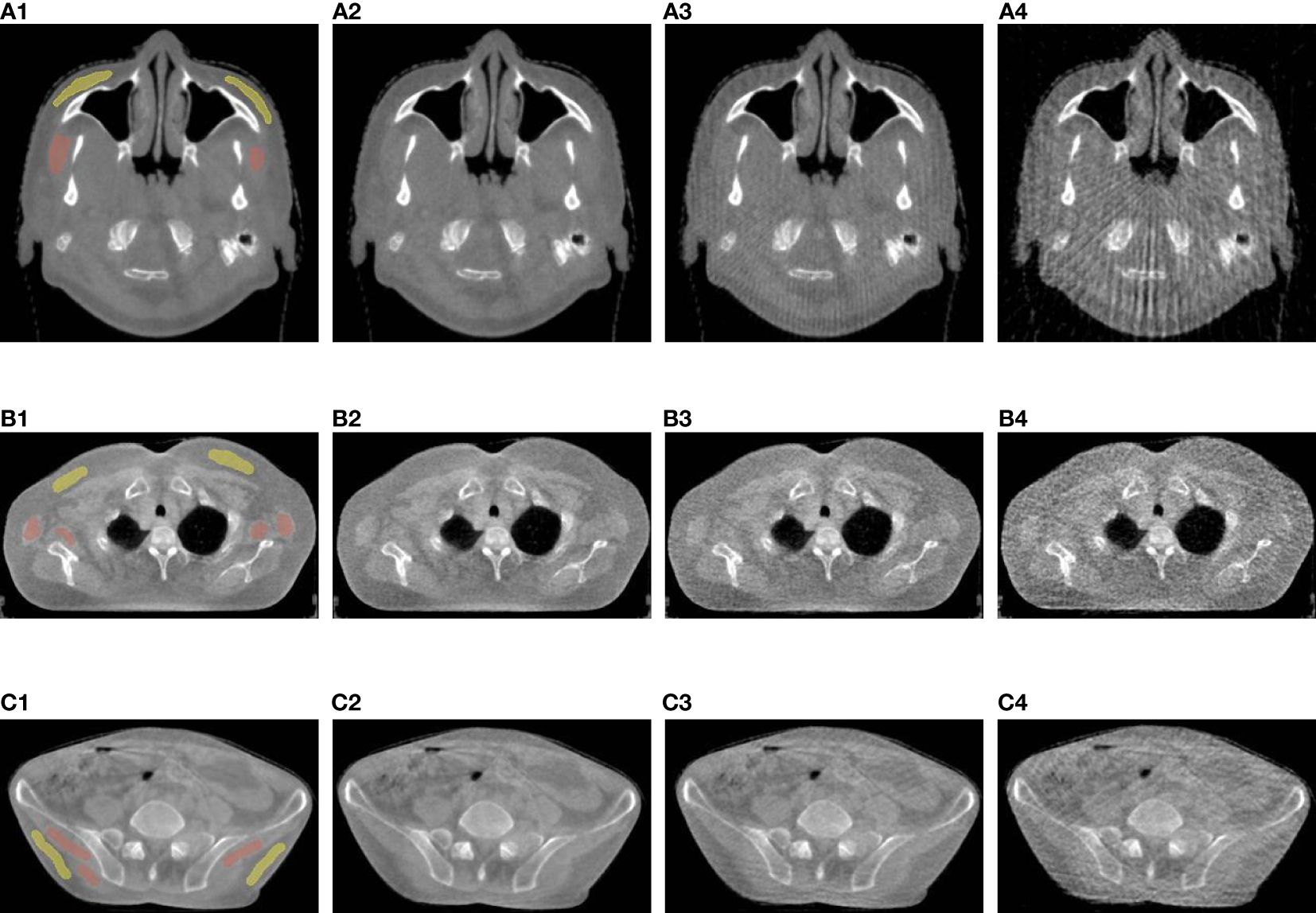
Figure 1 Visual image quality comparison of head (A-row), thorax (B-row), and pelvis (C-row) CBCT. The 1st column shows full-dose images, and 2nd, 3rd and 4th columns show S1/2, S1/4, and S1/8 images. The red and yellow annotations represent subcutaneous fat and muscle regions for CNR calculation.
The 6DoF registration errors of projection-reduced head, thorax, and pelvis scans relative to the full-dose scans are summarized in Figure 2, showing the median, mean ± standard deviation (SD) and 25–75% interpercentile range (Q1-Q3). We can clearly see that, regardless of imaging site or scan protocol, the mean and median values of relative errors across six directions were quite close to zero, ranging within ±0.4 mm in translation and within ±0.2 degrees in rotation. We use Q1-Q3 and mean ± SD to indicate uncertainties in relative errors. For translational directions, the Q1-Q3 values across all protocols ranged within ±0.5 mm in translation except that in LNG for pelvis scan protocols (± 0.7 mm), and a similar trend was identified for mean ± SD. For rotational directions, the uncertainties in head protocols were relatively larger than in those in thorax and pelvis protocols. This may be attributed to the uncertainties induced by cervical/neck flexion. In addition, the couch shifts of S1/2, S1/4 and S1/8 were paired with those of S1 in each direction to perform Wilcoxon paired signed-rank test. The p-values across all the tests were > 0.05, among which the smallest value was about 0.17, indicating the couch shift differences between projection-reduced protocols and the full-dose protocol were not statistically significant.
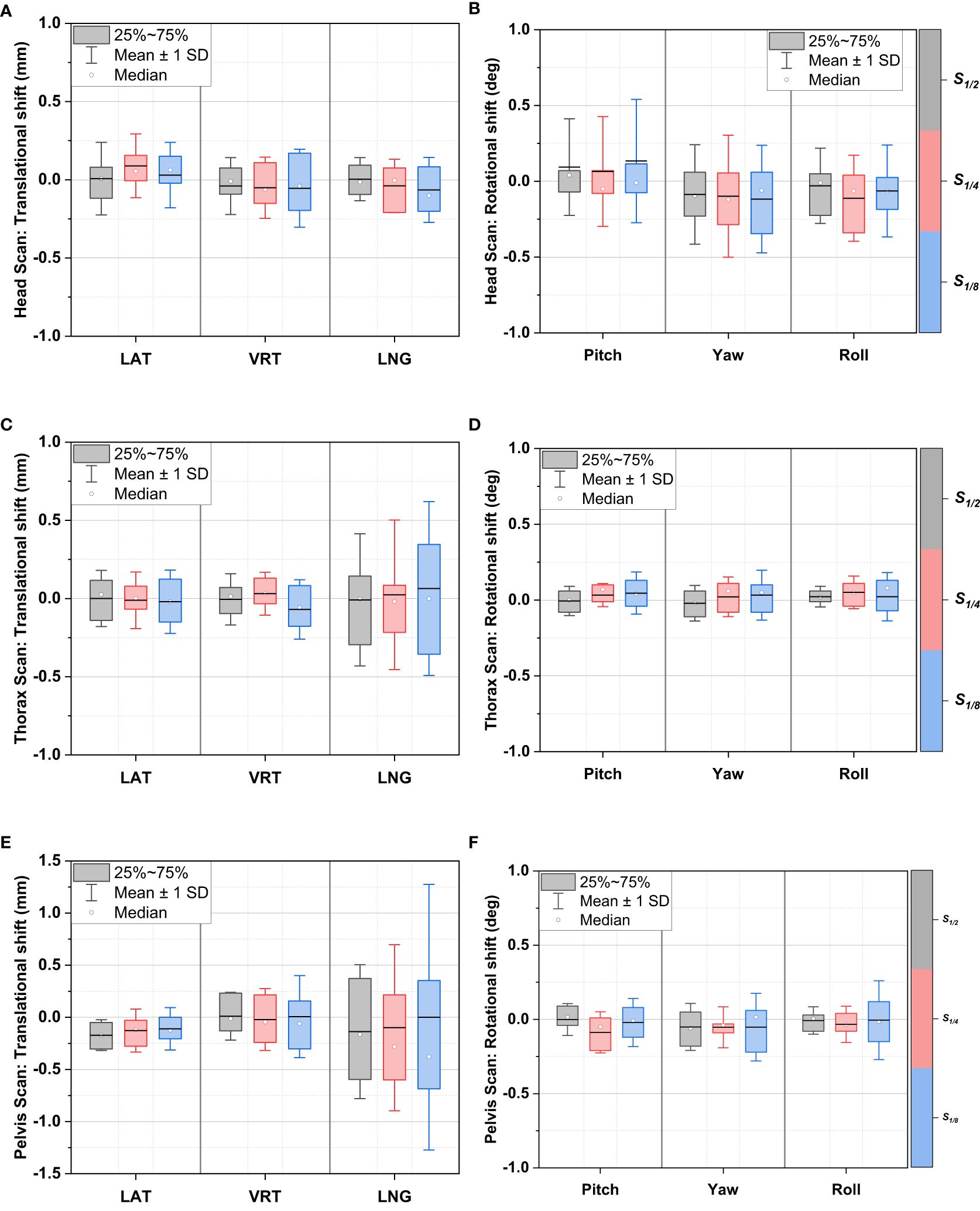
Figure 2 Relative registration error distributions between projection-reduced CBCT and full-dose CBCT in 6DoF: head scan translational shifts (A) and rotational shifts (B); thorax scan translational shifts (C) and rotational shifts (D); pelvis scan translational shifts (E) and rotational shifts (F).
The CNR, Tx and Rx values over the testing patients were summarized in Table 2 and Figure 3. As the projection numbers were reduced, the imaging dose decreased, leading to significant descending in CNR performance and ascending in Tx and Rx values. For CNR, while the S1/2 protocol exhibited inferior performance to the full-dose protocol, the mean values across the three sites were >3.0, indicating the soft-tissue contrast performance was generally acceptable even when the projection number was halved from the default setting. For Tx, the mean values were mostly less than 1 mm except for S1/8 protocol in pelvis scan. This is similar to the trend in Figure 2E, where S1/8 exhibited the largest uncertainties. For Rx, while the mean values for head scans were between 0.4 and 0.5 degree, those for thorax and pelvis scans were all less than 0.4 degree.
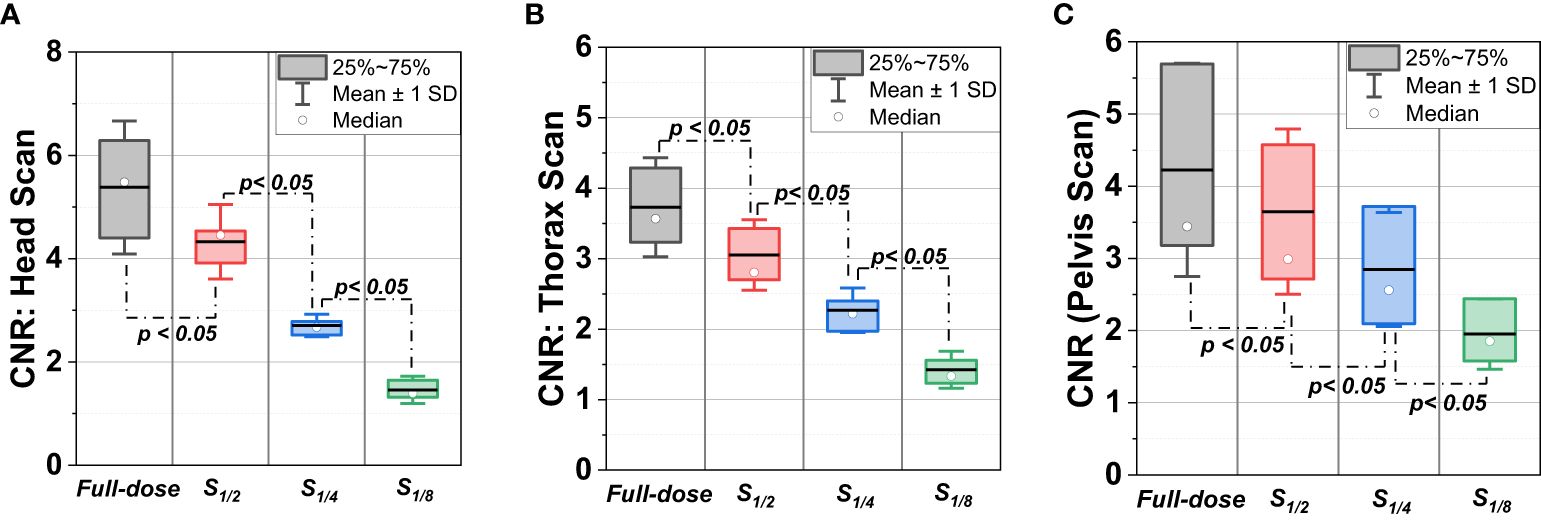
Figure 3 CNR distributions of full-dose CBCT scans and low-dose CBCT scans (S1/2, S1/4, and S1/8) in sites of (A) head, (B) thorax and (C) pelvis.
4 Discussion
Reducing imaging dose of CBCT has been a prominent concern due to the potential health risks associated with recurrent CBCT scans over the entire treatment course. A direct strategy to reducing dose is to obtain projections as few as feasible through expanding the angular gap in sampling, but with the challenge of ensuring that image quality and registration accuracy are not compromised. In the meantime, how to effectively evaluate the impact of projection reduction on image quality and registration accuracy in the clinical context without impairing treatment accuracy is a challenging issue as well.
Our study proposed a virtual clinical trial-based strategy to perform comprehensive clinical evaluation of projection-reduced low-dose CBCT for IGRT application. In this work, we enrolled a total of 71 real-world patients to quantitatively evaluate image quality and registration accuracy for head, thorax and pelvis CBCT scans. Hence, this virtual clinical trial-based study is more accurate in reflecting the clinical context than previous studies that are either highly controlled (20, 21) or phantom-based (15–19). In the meantime, we used the validated open-source research toolkit for image reconstruction (TIGRE-VarianCBCT) and registration (3D Slicer), justifying the reproducibility of all the results. In this context, our analysis yielded important insights.
Firstly, while the reduction of projection numbers led to increased image noise and strike artifacts, acceptable clinical image quality was maintained up to a decrease by half from the default projection number. This was justified by the CNR results in Table 2, demonstrating the potential to reduce the imaging dose without significant loss in image quality. However, as the projection number further decreased to 1/4 and 1/8 of the full dose, the CNR values fell below the acceptable threshold, implying substantial deterioration in soft-tissue contrast. This also indicates a limitation in the extent of projection-reduced low-dose CBCT while preserving adequate imaging quality.
Secondly, our study showed that the relative registration errors of projection-reduced CBCT scans compared to full-dose scans were negligible across six directions with mean error< 0.5 mm in translation and<0.4 degree in rotation. Moreover, the error magnitude was< 1.0 mm in translation (Tx) and< 0.4 degree in rotation (Rx). This further demonstrates that a reduction in projection numbers does not necessarily impair the registration accuracy and suggest the maintenance of registration accuracy even at reduced doses, the key concern in IGRT. This finding is in line with those in phantom-based studies by Men et al. (18) and Olch et al. (19). Also, as the projection number was further reduced, slight increase in uncertainties were observed, especially in the LNG direction in both thorax pelvis scans. This phenomenon we assume can be attributed to subtle pelvic anatomy changes along the LNG direction.
Moreover, it is worth noting that no statistically significant shift differences were identified between the dose-reduced protocols and the full-dose reference. The consistency of our findings with the literature (19, 21) reinforces the reliability and potential clinical viability of the projection-reduction strategy.
Our study has several limitations. First, we used auto-matching in CBCT-to-CT registration to minimize any personal bias. However, in clinical practice, therapists or physicists are probably to perform couch shifts with manual corrections. There may be some discrepancy here between the research world and how the shifts would look in a true clinical setting. Second, while the strategy of virtual clinical trial facilitated patient enrollment [for instance, only 6 patients were enrolled in the study by Bryce-Atkinson et al (21)], due to the limitation of the IRB approval, we only enrolled adult patients. It is well known that age is a key factor attributable to estimating patients’ risks (13), as it is pediatric patients that will benefit the most from imaging dose reduction. While we believe some findings in this study are also applicable to pediatric patients, further efforts including more extensive clinical trials are required for solid validation. Thirdly, while we used a virtual clinical trial design to ensure clinical applicability, further prospective clinical trials are needed for a more robust and comprehensive validation, especially in combination with other low-dose techniques. Besides, the retrospective study design, scan sites and the dose-reduction method are also limitations.
5 Conclusion
Our study indicates that while the impact of dose-reduction on CBCT couch shifts is not significant, the impact on CNR values is significant. Further validation on optimizing CBCT imaging dose is required.
Data availability statement
The original contributions presented in the study are included in the article, further inquiries can be directed to the corresponding authors.
Ethics statement
The studies involving humans were approved by Peking University Cancer Hospital. The studies were conducted in accordance with the local legislation and institutional requirements. Written informed consent for participation was not required from the participants or the participants’ legal guardians/next of kin in accordance with the national legislation and institutional requirements.
Author contributions
MW: Writing – original draft. KY: Writing – original draft. YZ: Writing – review & editing. JG: Writing – review & editing. XZ: Writing – review & editing. ZL: Writing – review & editing. YL: Writing – review & editing. HW: Writing – review & editing. YD: Writing – review & editing.
Funding
The author(s) declare financial support was received for the research, authorship, and/or publication of this article. This work was supported in part by National Natural Science Foundation of China (No. 12375335, 12005007), Beijing Natural Science Foundation (No. 1212011), National Key Research and Development Project (No. 2019YFF01014405), Peking University Educational Reform Foundation (No. 2299000138), and Clinical Research Project of Wu Jieping Medical Foundation (No. 320.6750.2022-3-59), Peking University People's Hospital Scientific Research Development Funds (No. RDE2023-09).
Conflict of interest
The authors declare that the research was conducted in the absence of any commercial or financial relationships that could be construed as a potential conflict of interest.
Publisher’s note
All claims expressed in this article are solely those of the authors and do not necessarily represent those of their affiliated organizations, or those of the publisher, the editors and the reviewers. Any product that may be evaluated in this article, or claim that may be made by its manufacturer, is not guaranteed or endorsed by the publisher.
Abbreviations
6DoF, 6-degree-of-freedom; CT, computed tomography; CBCT, cone-beam computed tomography; CTDIw, weighted computed tomography dose index; CNR, contrast-to-noise ratio; HU, Hounsfield unit; IGRT, image-guided radiotherapy; IRB, Institutional Review Board; LAT, lateral; LNG, longitudinal; VRT, vertical; Q1, first quartile; Q3, third quartile; SD, standard deviation.
References
1. Zelefsky MJ, Kollmeier M, Cox B, Fidaleo A, Sperling D, Pei X, et al. Improved clinical outcomes with high-dose image guided radiotherapy compared with non-IGRT for the treatment of clinically localized prostate cancer. Int J Radiat Oncol Biol Phys. (2012) 84:125–9. doi: 10.1016/j.ijrobp.2011.11.047
2. Bujold A, Craig T, Jaffray D, Dawson LA. Image-guided radiotherapy: has it influenced patient outcomes? Semin Radiat Oncol. (2012) 22:50–61. doi: 10.1016/j.semradonc.2011.09.001
3. Nabavizadeh N, Elliot DA, Chen Y, Kusano AS, Mitin T, Thomas CR Jr, et al. Image guided radiation therapy (IGRT) practice patterns and IGRT’s impact on workflow and treatment planning: results from a national survey of American Society for Radiation Oncology members. Int J Radiat Oncol Biol Phys. (2016) 94:850–7. doi: 10.1016/j.ijrobp.2015.09.035
4. Ding GX, Munro P. Radiation exposure to patients from image guidance procedures and techniques to reduce the imaging dose. Radiother Oncol. (2013) 108:91–8. doi: 10.1016/j.radonc.2013.05.034
5. Son K, Kim JS, Lee H, Cho S. Imaging dose of human organs from kV-CBCT in image-guided radiation therapy. Radiat Prot Dosimetry. (2017) 175:194–200. doi: 10.1093/rpd/ncw285
6. Hess CB, Thompson HM, Benedict SH, Seibert JA, Wong K, Vaughan AT, et al. Exposure risks among children undergoing radiation therapy: considerations in the era of image guided radiation therapy. Int J Radiat Oncol Biol Phys. (2016) 94:978–92. doi: 10.1016/j.ijrobp.2015.12.372
7. Dzierma Y, Mikulla K, Richter P, Bell K, Melchior P, Nuesken F, et al. Imaging dose and secondary cancer risk in image-guided radiotherapy of pediatric patients. Radiat Oncol. (2018) 13:168. doi: 10.1186/s13014-018-1109-8
8. Zhou L, Bai S, Zhang Y, Ming X, Zhang Y, Deng J. Imaging dose, cancer risk and cost analysis in image-guided radiotherapy of cancers. Sci Rep. (2018) 8:10076. doi: 10.1038/s41598-018-28431-9
9. Hua CH, Vern-Gross TZ, Hess CB, Olch AJ, Alaei P, Sathiaseelan V, et al. Practice patterns and recommendations for pediatric image-guided radiotherapy: a Children’s Oncology Group report. Pediatr Blood Cancer. (2020) 67:e28629. doi: 10.1002/pbc.28629
10. Ibbott GS. Patient doses from image-guided radiation therapy. Physica Med. (2020) 72:30–1. doi: 10.1016/j.ejmp.2020.03.005
11. Steiner E, Healy B, Baldock C. Dose from imaging at the time of treatment should be reduced. Phys Eng Sci Med. (2023) 46(3):959–62. doi: 10.1007/s13246-023-01298-5
12. Martin CJ, Kron T, Vassileva J, Wood TJ, Joyce C, Ung NM, et al. An international survey of imaging practices in radiotherapy. Physica Med. (2021) 90:53–65. doi: 10.1016/j.ejmp.2021.09.004
13. Murphy MJ, Balter J, Balter S, BenComo JA Jr, Das IJ, Jiang SB, et al. The management of imaging dose during image-guided radiotherapy: report of the AAPM Task Group 75. Med Phys. (2007) 34:4041–63. doi: 10.1118/1.2775667
14. Ding GX, Alaei P, Curran B, Flynn R, Gossman M, Mackie TR, et al. Image guidance doses delivered during radiotherapy: Quantification, management, and reduction: Report of the AAPM Therapy Physics Committee Task Group 180. Med Phys. (2018) 45:e84–99. doi: 10.1002/mp.12824
15. Wood TJ, Moore CS, Horsfield CJ, Saunderson JR, Beavis AW. Accounting for patient size in the optimization of dose and image quality of pelvis cone beam CT protocols on the Varian OBI system. BJR Open. (2015) 88:20150364. doi: 10.1259/bjr.20150364
16. Yan H, Cervino L, Jia X, Jiang SB. A comprehensive study on the relationship between the image quality and imaging dose in low-dose cone beam CT. Phys Med Biol. (2012) 57:2063–80. doi: 10.1088/0031-9155/57/7/2063
17. Lu B, Lu H, Palta J. A comprehensive study on decreasing the kilovoltage cone-beam CT dose by reducing the projection number. J Appl Clin Med Phys. (2010) 11:231–49. doi: 10.1120/jacmp.v11i3.3274
18. Men K, Dai J. A projection quality-driven tube current modulation method in cone-beam CT for IGRT: proof of concept. Technol Cancer Res Treat. (2017) 16:1179–86. doi: 10.1177/1533034617740283
19. Olch AJ, Alaei P. How low can you go? A CBCT dose reduction study. J Appl Clin Med Phys. (2021) 22:85–9. doi: 10.1002/acm2.13164
20. Alcorn SR, Zhou XC, Bojechko C, Rubo RA, Chen MJ, Dieckmann K, et al. Low-dose image-guided pediatric CNS radiation therapy: final analysis from a prospective low-dose cone-beam CT protocol from a multinational pediatrics consortium. Technol Cancer Res Treat. (2020) 19:153303382092065. doi: 10.1177/1533033820920650
21. Bryce-Atkinson A, De Jong R, Marchant T, Whitfield G, Aznar MC, Bel A, et al. Low dose cone beam CT for paediatric image-guided radiotherapy: Image quality and practical recommendations. Radiother Oncol. (2021) 163:68–75. doi: 10.1016/j.radonc.2021.07.027
22. Zhou S, Li J, Zhu X, Du Y, Yu S, Wang M, et al. Initial clinical experience of surface guided stereotactic radiation therapy with open-face mask immobilization for improving setup accuracy: a retrospective study. Radiat Oncol. (2022) 17:104. doi: 10.1186/s13014-022-02077-4
23. Varian Medical Systems. TrueBeam technical reference guide volume 2: imaging P1033684-002-B. Palo Alto, CA: Varian Medical Systems, Inc. (2022).
24. Du Y, Wang R, Biguri A, Zhao X, Peng Y, Wu H. TIGRE-VarianCBCT for on-board cone-beam computed tomography, an open-source toolkit for imaging, dosimetry and clinical research. Phys Med. (2022) 102:33–45. doi: 10.1016/j.ejmp.2022.08.013
25. Burgess AE. The Rose model, revisited. J Opt Soc Am A Opt Image Sci Vis. (1999) 16:633–46. doi: 10.1364/JOSAA.16.000633
Keywords: CBCT, low dose, image guided radiotherapy, virtual clinical trial, imaging dose
Citation: Wang M, Yao K, Zhao Y, Geng J, Zhu X, Liu Z, Li Y, Wu H and Du Y (2024) Virtual clinical trial-based study for clinical evaluation of projection-reduced low-dose cone-beam CT for image guided radiotherapy. Front. Oncol. 14:1369603. doi: 10.3389/fonc.2024.1369603
Received: 12 January 2024; Accepted: 12 June 2024;
Published: 05 July 2024.
Edited by:
Martin Bues, Mayo Clinic Arizona, United StatesReviewed by:
Christian Bäumer, West German Proton Therapy Centre Essen (WPE), GermanyMattison Flakus, Mayo Clinic Arizona, United States
Sara Bashir, Mayo Clinic Arizona, United States, in collaboration with reviewer MF
Copyright © 2024 Wang, Yao, Zhao, Geng, Zhu, Liu, Li, Wu and Du. This is an open-access article distributed under the terms of the Creative Commons Attribution License (CC BY). The use, distribution or reproduction in other forums is permitted, provided the original author(s) and the copyright owner(s) are credited and that the original publication in this journal is cited, in accordance with accepted academic practice. No use, distribution or reproduction is permitted which does not comply with these terms.
*Correspondence: Yixin Zhao, zhaoyixin16@163.com; Yi Du, yi.du@hotmail.com
†These authors have contributed equally to this work