- 1Department of General Surgery, General Hospital of Northern Theater Command, Shenyang, Liaoning, China
- 2Department of Breast Surgery, The First Hospital of China Medical University, Shenyang, China
- 3Department of General Surgery, Xinqiao Hospital, Army Medical University, Third Military Medical University, Chongqing, China
Early-onset colorectal cancer (EOCRC) is defined as diagnosed at younger than 50 years of age and indicates a health burden globally. Patients with EOCRC have distinct risk factors, clinical characteristics, and molecular pathogenesis compared with older patients with CRC. Further investigations have identified different roles of obesity between EOCRC and late-onset colorectal cancer (LOCRC). Most studies have focused on the clinical characteristics of obesity in EOCRC, therefore, the mechanism involved in the association between obesity and EOCRC remains inconclusive. This review further states that obesity affects the carcinogenesis of EOCRC as well as its development and progression, which may lead to obesity-related metabolic syndrome, intestinal dysbacteriosis, and intestinal inflammation.
1 Introduction
Obesity is characterized by an excessive accumulation of adipose tissue and defined in terms of body mass index (BMI) (1). An imbalance between energy intake and energy consumption was correlated with the gain in weight. Obesity is defined as a BMI value > 30 kg/m2 through the formula weight (kg)/height2 (m2) (30.0-34.9, grade I; 35.0-39.9, grade II; and ≥40, grade III) (2). In the last decades, the prevalence of obesity has been increasing and obesity has become an epidemic problem (3). Obesity is also strongly correlated with environment and socio-economic status, which may accelerate the development of cancer through gene-environment interaction (3). In China, obesity rates tripled between 1991 and 2006 (4), with 10.5% prevalence of obesity in men in China (5).Obesity is a well-known risk factor for colorectal cancer (CRC). The risk of CRC increased by 1.2-1.5 folds in patients who were overweight and by 1.5-1.8 folds in patients with obesity (6). Previous investigations have implicated that obesity acted differently between early-onset colorectal cancer (EOCRC) and late-onset colorectal cancer (LOCRC) (7, 8). EOCRC is defined as CRC diagnosed at younger than 50 years of age (9). Moreover, CRC occurring in patients older than 50 years is defined as LOCRC (10). EOCRC accounts for nearly 10% of newly diagnosed cases of CRC; an increase in global incidence of EOCRC has been noted globally, especially in high-income regions (11). Adhari Al Zaabi et al. explained obesity as a modifiable risk factor for EOCRC (12). Obesity resulted in an increasing incident rate of EOCRC (13, 14). However, the clinical and biological plausible explanation of the association of obesity with EOCRC needs further research (15).
In this review, we first provide an overview of differences between EOCRC and LOCRC, association between obesity and EOCRC, and summarizing the main clinical differences and biological mechanisms, with an emphasis on: 1) clinical evidence between obesity and EOCRC 2) obesity-associated metabolic disorder in EOCRC, 3) obesity-induced dysfunction of intestinal microbiota, and 4) obesity-induced inflammation. It is crucial to elucidate the association between obesity and EOCRC for the development of preventive, diagnostic, and therapeutic strategies against cancer.
2 Difference between early-onset colorectal cancer and late-onset colorectal cancer
2.1 Different onset processes of early-onset colorectal cancer
CRC results from the accumulation of multiple genetic changes, however, most CRCs share only a few mutations (16). The main differential features between CRC of both age groups have been summarized (Figure 1). Genetic features in EOCRC are distinct from LOCRC. The incidence of EOCRC may be attributed to the accumulation of multiple rare genetic variants. Chromosome instability (CIN), microsatellite instability (MSI), and CpG island methylation (CIMP) are the three main processes involved in the onset and development of CRC (17). CIN is one of the most common forms of genomic instability, and is associated with poor survival, metastases, and resistance to cancer therapy (18). Along with higher rates of advanced histologic features and later stages, CIN is observed in most patients with EOCRC (19). CIN is also associated with the progression of adenoma to carcinoma, while MSI and CIMP are associated with the progression of sessile serrated polyps to invasive carcinomas (16). MSI results from mismatch repair deficiency and is associated with the primary loss of function of mismatch repair proteins (MLH1, MSH2, MSH6, and PMS6) (20). Research has showed that EOCRC is enriched in MSI, which is a distinct genetic pathway for CRC carcinogenesis (21, 22). One-third of EOCRC patients with MSI have been diagnosed with a defined inherited syndrome (23). EOCRC patients with MSI are also less responsive to 5fluorouracil-based chemotherapy (24, 25). Along with hereditary characteristics of EOCRC, Lynch syndrome (LS) is regarded as the most common hereditary cancer syndrome related to MSI and occurs at a higher frequency in EOCRC (17, 21). In LS, the most frequently mutated genes were MLH1 and MLH2, while mutations in MSH6 and PMS2 were rather lower (26). Correlations between germline deletions of epithelial cell adhesion molecules and silencing of MSH2 have also been observed in CRC patients with LS (20). c-MYC proto-oncogene (MYC) and Adenomatous polyposis coli (APC) have been indicated as initial factors of CRC. MYC, a basic-helix-loop-helix transcription factor binding to MAX, is involved in the regulation of the cell cycle, cell survival, metabolism, and ribosome biogenesis (27). The mutation in APC is attributed to the activation of the Wnt/β-catenin cascade and accelerated CRC processes (28). Compared with LOCRC, EOCRC is classified as the low-MYC expression group and dysregulated MYC contributes to CRC carcinogenesis in patients with EOCRC (29). APC, a tumor-suppressor gene, was also found in patients with EOCRC (30). These pieces of evidence prove that there are indeed differences in onset processes between EOCRC and LOCRC.
2.2 Different clinical symptoms of early-onset colorectal cancer
EOCRC exhibits differences in symptoms, pathogenesis sites, and clinical pathological features, with symptoms such as rectal bleeding, abdominal pain, and anemia being more common (21). Abdominal pain and rectal bleeding, the two most common symptoms, were observed in 47% and 46% of EOCRC, according to the study aggregated from 2014-2018 (31). The overall incidence of CRC, especially distal colon, and rectum cancer, has decreased in individuals older than 50 years (10). Studies have reported the occurrence of EOCRC mostly in the left colon and rectum, however, LOCRC tended to occur mostly in the right colon (32, 33). This difference may be explained by different embryologic structures of the left- and right-side colon. The right side of the colon develops from the midgut, while the left develops from the hindgut. In addition, the left- and right sides of the colon are supplied with two different blood vessels (34). EOCRC has been observed to be a poorly differentiated and advanced stage compared with LOCRC (32). Most CRCs are adenocarcinomas and comprise three key subtypes, including classical adenocarcinoma (CA), mucinous adenocarcinoma (MA), and signet-ring cell carcinoma (SRCC) (35). EOCRC is likely an SRCC and MA subtype (36, 37). Compared with classical adenocarcinoma, SRCC, and MA are positively associated with a higher possibility of lymphatic invasion, perineural infiltration, and a second primary malignancy (SPM) (36, 37). Patients with SRCC were also younger and the tumor was likely to be poorly differentiated (37). In a few studies, young patients were likely to have SPM (38). Similarly, the rate of SPM was also higher in patients with EOCRC in the first 6-11 months compared to patients with LOCRC (39). Distant metastasis is more frequent in EOCRC rather than in LOCRC (40). Moreover, with the liver being the primary organ of metastasis, nearly 14% of diagnosed EOCRC exhibited liver metastasis (LM) (40). Most patients with EOCRC were found to have distant metastasis or regionally advanced cancer at the time of diagnosis (41). However, studies showed that specific metastasis features were not independent of stage. The frequency of lymphovascular invasion was found to be higher only in stage 2, while peri-neural invasion was higher in stage 3 in EOCRC (42). Compared to LOCRC, patients with EOCRC also had a higher relapse rate and a worse survival rate (43, 44).
3 Distinct role of obesity in early-onset colorectal cancer
Even though an elevated incidence and a worse prognosis of EOCRC has been predicted in younger age, the prevention and early diagnosis of EOCRC still remain difficult as the causes of the disease are unknown (45). Multivariable analysis demonstrated that after controlling for staging and demographics, younger ages itself is no longer an indicator of worse outcomes (45). Therefore, the effect of age in EOCRC may be correlated with other risk factors. Interestingly, obesity, as a well-known CRC risk factor, correlated positively in younger patients with CRC (17). Nevertheless, the role of obesity in EOCRC and LOCRC still remains unclear. Obesity is highly correlated with metabolic syndrome, intestinal microbes, and inflammation (46, 47). In fact, low-grade inflammation also correlates with metabolic disorders and alterations of intestinal microbiota (48, 49). Therefore, we now summarize the clinical evidence and potential biological mechanism of obesity-induced EOCRC (Figure 2).
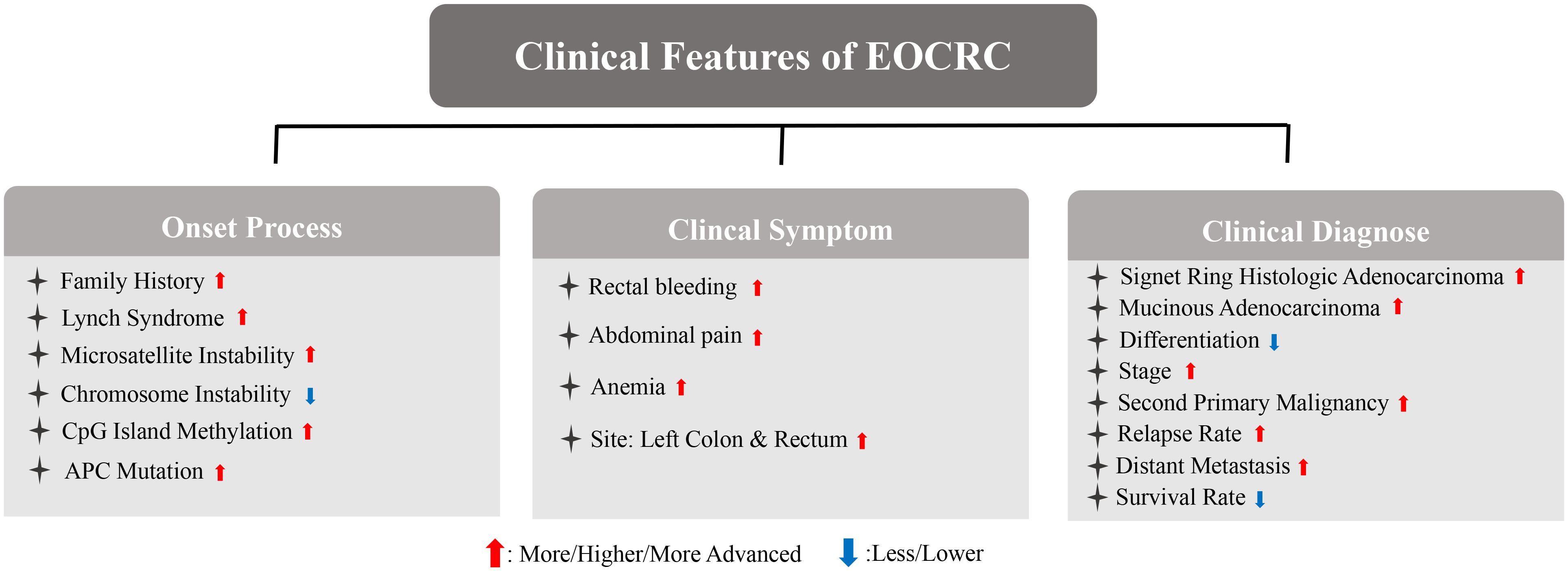
Figure 2 Association between obesity and EOCRC synopsizing the main clinical differences and biological mechanisms.
3.1 Clinical evidence between obesity and early-onset colorectal cancer
Patients with EOCRC are more likely to be obese. The degree of obesity is positively correlated with the occurrence and development of CRC, according to epidemiological evidence. Based on the Jass classification of CRC (MSI-H, CIMP-low/negative, BRAF-wildtype, and KRAS-wildtype), the large team of collaborating investigators found that only Jass type p5 or Lynch syndrome was not related to BMI (50). Therefore, obesity may play a potentially significant role in CRC. Regular physical activity and weight loss by bariatric surgery, respectively resulted in nearly 31% and 27% reduction in CRC risk, and obesity was identified as the risk factor for CRC (51, 52). Interestingly, reducing body weight at a young age decreased the risk of CRC in later ages (53). The average value of BMI in EOCRC patients, which is greater than 24, is significantly higher than that of patients with LOCRC (44). A retrospective study on 651 patients with EOCRC showed that 64.6% were overweight or obese (54). Weight gain in early- and mid-adulthood is associated with a significantly higher risk for CRC (55). Based on a propensity-weight analysis of 133008 patients with EOCRC, the association between EOCRC and a higher BMI (≥30kg/m2) was proved to be strong, with increased risks of advanced adenoma amongst obese EOCRC patients (36). Compared with sex, age, smoking, alcohol consumption, regular exercise, low-income status, and other risk factors of CRC, obesity is significantly associated with an increased risk of EOCRC. When compared with individuals with normal weight, the overweight group had an aHR of 1.10 (95% CI, 1.04–1.17), the obese group had an aHR of 1.19 (95% CI, 1.12–1.25), and the severely obese group had an aHR of 1.45 (95% CI, 1.31–1.61) for EOCRC (56). This evidence proved that patients with EOCRC are likely to be clinically obese and obesity may be a more dangerous factor in younger patients with CRCs compared with other risk factors of CRC. However, the potential mechanisms remain unclear.
Epidemiological evidence demonstrated that the incidence of EOCRC is triggered by environmental factors but not genetic evolutionary factors (17, 39, 57). Serrated polyps (SPs) and conventional adenomas (CAs) are important precursors of CRC. Interestingly, risks of SPs and CAs were significantly associated with obesity in young patients with CRC, while this association was weaker in aging CRC patients (58). Another study also showed that a higher BMI in early adulthood increased the incidence of serrated polyps and conventional adenomas (53). The high-fat diet, which is linked to obesity, is strongly associated with the specific molecular subtypes of EOCRC (59). Western diets and lack of activity, proven to accelerate the progression of obesity, also correlate with the rising incidence of EOCRC (60). High-sugar foods may accelerate de novo lipogenesis, obesity, and metabolic syndromes; accordingly, a prediction model showed the correlation of sweet and fried food with an increased risk of EOCRC (61). Moreover, in those EOCRCs patients having HNF1 mutation, high-fat diet (HFD) accelerates the formation of colonic polyps through the β-catenin/Cdx2 pathway (62). This specific subtype was also associated with conventional adenoma-carcinoma sequences, a well-known precursor of EOCRC (63). These studies emphasized the significant role of obesity in the initial process of EOCRC.
Obesity is also associated with postoperative complications. It distorts anatomical planes and reduces the operative space, resulting in technical difficulty and raising the anastomotic leak rate (64). Anastomotic leak was proven to increase local recurrence rates and decrease 5-year survival rates (64). Higher rates of shock, bleeding, wound disruption, postoperative infection, and digestive system complications are likely to appear in patients with obesity, which accelerates CRC progression by increasing the time of hospital stay and associated mortality (65).. Obesity is also positively associated with CRC recurrence and associated mortality rates (66, 67). Patients prediagnosed with an obesity in terms of BMI had worst outcomes than those with an overweight or normal BMI (68). Accumulation of body weight resulted in a higher proportion of visceral adipose tissue and subcutaneous adipose tissue, which are the principal deposition of fat (69). It was confirmed that visceral adipose tissue and subcutaneous adipose tissue increased the risk of CRC mortality (70). Interestingly, obesity also results in a rather worse outcome of EOCRC than of LOCRC. A systematic review and a multi-variable logistic regression model predicted BMI as an independent risk factor of EOCRC (71–73). A linear relationship of BMI with EOCRC was noted in a dose-response manner (71). One study of EOCRC in the UK showed that a higher BMI is correlated with a higher occurrence of tumor budding (TB) (74). TB is significantly associated with CRC metastasis, locoregional recurrences, and worse disease-free survival (75, 76). TB was also frequently found in SRCC, and this evidence corresponded with the specific histology of EOCRC (77). Patients with EOCRC have a higher perirectal fat area, which is strongly correlated with cancer metastasis and a worse survival (78). However, obesity results in a rather good outcome for LOCRC compared with EOCRC. In a recent study, a higher BMI was regarded as a protective factor of CRC prognosis, in which the participants were mainly patients with LOCRC (79). This inverse association proved that the risk of obesity in EOCRC was significantly increased compared with LOCRC. The association of obesity with EOCRC may be attributed to its involvement in the occurrence and development of CRC by affecting the metabolism and inflammatory factors, including insulin and insulin-like growth factors, sex hormones, and adipokines.
3.2 Obesity-associated metabolic disorder in early-onset colorectal cancer
A higher BMI is associated with an increase in metabolic disorders parallelly (80). Metabolic syndrome is a cluster of metabolic disorders, including obesity, diabetes mellitus type 2, hyperlipidemia, and hypertension, which showed a concurrent increase in incidence with the increase in obesity (80). Abnormal fat accumulation could also be seen in most individuals with metabolic syndrome, and excess fat plays a central role in such individuals (81). The pooled result of two research demonstrated that the cancer-specific mortality in CRC patients with metabolic syndrome was nearly two times higher than that of patients without metabolic syndrome (82). Metabolic syndrome may participate in the carcinogenesis of CRC through several mechanisms: i) insulin resistance, which leads to the accumulation of reactive oxygen species (ROS) and results in DNA damage and mutation; ii) increasing levels of leptin and adipokines promote the occurrence of CRC (83). Moreover, the CRC risk of metabolic syndrome in males was found to be higher than in females and this may result in estrogen and progesterone (83, 84). Studies have shown a greater sensitivity of EOCRC to obesity-induced metabolic syndrome compared to LOCRC. In one report, a history of metabolic disorders (including type 2 diabetes, mellitus, primary hypertension, disorders of lipoprotein metabolism and other dyslipidemias, overweight and obesity, or fatty change of liver) doubled the incidence of EOCRC (85). The number of metabolic disorders in patients was directly associated with the risk of EOCRC, increased risks of CRC are observed only among LOCRC patients who had more than three metabolic syndromes (86). Metformin, aspirin, statins, and other medications that treat metabolic syndrome can reduce the risk of CRC (87). The widespread use of these drugs for maintenance medication in obese patients with LOCRC might reduce the incidence of CRC compared with obese patients with EOCRC (87).
All metabolic syndrome components were shown to correlate independently with an increased risk of EOCRC (56). Obesity was present in almost 65%-80% of those diagnosed with type II diabetes (88). Increased body weight also impacts insulin clearance and results in insulin resistance, a kind of metabolic disorder (89). Dysglycemia resulted in a 20% to 38% increase in the risk of CRC (90). Weichuan Dong et al. suggested that diabetes has a stronger effect on EOCRC compared to LOCRC (91). The metabolic syndrome-related CRC might result in subsequent insulin resistance, which is linked to increased levels of insulin growth factor-1 (IGF-1) and IGFR (R) (92, 93). Elevated IGF-1 levels also induced CRC migration and proliferation via the phosphoinositide 3-kinase (PI3K)/Akt pathway (51, 94). Higher blood glucose increases the proliferation and metastases of CRC cells, and the insulin-growth factor receptor IGFR (R) pathway plays a pivotal role in the whole process (95). Dysglycemia is associated with the risk of EOCRC incidence and mortality. In one Swedish cohort study, patients diagnosed with diabetes had a 1.9-fold higher risk of developing EOCRC (39). Another research showed the correlation between sugar intake and a higher risk of EOCRC, while reduction of sugar intake was a protective strategy against EOCRC (96).
Hyperlipidemia, the subsyndrome of metabolic syndrome, showed no significant effects on CRC outcomes independently (82). However, one research showed that dyslipidemia was not associated with incident CRC (97). Another research showed the level of serum hyperlipidemia, was inversely correlated with the risk of colon cancer (98). However, one study including 1447 patients showed higher triglyceride levels in patients with EOCRC than those with LOCRC (99). Dyslipidemia was then found to have a strong association with EOCRC (OR=2.39, p<0.001) and was a risk factor (100). A meta-analysis of 20 studies demonstrated the correlation between hyperlipidemia (higher triglycerides and cholesterol levels) and obesity with EOCRC development (11). Likewise, in other studies, nearly 30% of patients with EOCRC were diagnosed with hyperlipidemia (80), and levels of HDL-c were also lower in EOCRC (99). These findings affirmed the important role of obesity-related hyperlipidemia in EOCRC.
Contrary to dysglycemia and hyperlipidemia, the role of hypertension in CRC is poorly understood (101). Nonetheless, one large prospective cohort study showed that hypertension is not associated with the risk and mortality of CRC (102). However, the Dietary Approaches to Stop Hypertension diet can reduce morbidity and prolong the survival of patients with CRC (103). Therefore, the exact role of hypertension in CRC still remains unclear. However, several cohort studies shown that hypertension was a risk factor in EOCRC compared with the normal cohort; surprisingly, hypertension also functioned as a protective role in EOCRC compared with LOCRC (73, 104). Moreover, EOCRC was likely to exhibit MSI, and hypertension was regarded as clinically independent of MSI status in CRC (105).
3.3 Obesity-induced dysfunction of intestinal microbiota in early-onset colorectal cancer
The intestinal microbes have crucial roles in intestinal homeostasis and are perceived as the second genome. The colonic microbial system comprises various microbes (bacteria, fungi, viruses, eukaryotes, and archaea) and play an important role in the development of CRC (106, 107). Intestinal microbes accelerate the occurrence of CRC via immune-regulation, gene interaction, inflammation, and other mechanisms (108). The abundance of intestinal microbes, including Fusobacterium nucleatum, enterotoxigenic Bacteroides fragilis, and Escherichia coli, increases significantly in the intestine (109). The interaction between microbiota and mitochondria also promotes the initial carcinogenesis of CRC. In one study, Peptostreptococcus anaerobiusactivate was found to activate toll-like receptor 2 (TLR2) and TLR4 to increase intracellular levels of ROS, contributing to cholesterol biosynthesis and cell proliferation in the development of CRC (110). Furthermore, two species of Propionibacterium were correlated with loss of mitochondrail transmembrane proteins and generation of ROS in CRC cells (111, 112). Tumor development and CRC metastasis are complex processes involving the tumor microenvironment (TME), of which, the microbes are the main component (113). Intestinal microbes invade CRC tissues and change the TME (114).
The intestinal microbiome regulates aging-related changes in cognitive function, innate immunity, and inflammation (115). The increasing incidence of EOCRC is multifactorial, while the microbiome is the key factor (116). In fact, the microbial diversity of patients with EOCRC was lower than those of patients with LOCRC (109, 117). Through metagenomic sequencing analysis, another study also showed significantly lower fecal alpha diversity in EOCRC compared with LOCRC and healthy individuals, indicating that intestinal microbes may be of great importance in EOCRC (117). The Flavonifractor plautii was enriched in EOCRC and exhibited a positive association with cancer metabolites (115). Therefore, the interaction between obesity and EOCRC may be based on intestinal microbes. Obesity can alter the intestinal microbiome, consequently leading to dysbiosis and metabolic dysfunction (118). Obesity-induced by HFDs leads to intestinal dysbiosis and the accumulation of toxic substances, such as lipopolysaccharides (LPS), secreted by intestinal microbes (119). In vivo, HFD resulted in the accumulation of LPS in EOCRC, which promoted CRC (60, 120). Cross-sectional research showed higher blood levels of LPS in patients with adenomas than in normal individuals. Moreover, levels of LPS in individuals with villous adenomas were higher than those with tubular adenomas (107). However, the link between dysbiosis in obese patients and its associations with EOCRC remains difficult to state, and require further investigations.
A sequencing of 16s ribosomal RNA gene revealed the differential components of intestinal microbes between patients with EOCRC and LOCRC (121). Firmicutes, Blautia, and Clostridia are three species abundant in EOCRC, and are positively correlated with HFD-induced obese individuals (81). The abundance of Firmicutes was higher in ob/ob mice (81). Clostridia plays a key role in fat accumulation and metabolic syndrome. Likewise, Blautia is positively correlated with blood levels of triglycerides, diglycerides, and Cardiolipin, which are common lipids in obese individuals (122). Clostridia also regulates the function of CD36, which is associated with lipid absorption (123). Actinomyces, identified as the key microbiota in the EOCRC group, was also abundant in obese individuals (109, 124). Bifidobacteriaceae was higher in older patients with CRC (121), and negatively associated with levels of total cholesterol, low-density lipoprotein-1 (LDL-1), and LDL-2. This evidence demonstrated the lesser significant role of obesity in LOCRC (125). Xiong. et al. indicated Fusobacteria and Bacteroidales as independent markers of EOCRC and LOCRC (117). Fusobacterium and Bacteroidales are both the pathobiont in individuals with CRC, but they perform differently in response to obesity (126, 127). The abundance of Fusobacterium increases significantly in obese people, and that of Bacteroidales decreased in glucocorticoid-induced obesity (128, 129). Fusobacterium also induce inflammation and suppresses host immunity in CRC, the abundance of Fusobacterium also correlates with shorter survival of patients with CRC (126). The levels of short-chain fatty acids (SCFAs) decrease in obese patients with CRC and have anti-cancer roles (130, 131). In fact, Mitsuokella. multacida, a bacterium that produced SCFA in the intestine, was absent in patients with EOCRC (60). This finding, based on intestinal SCFA levels, indicated that the risk of obesity may be higher in EOCRC. Thus, patients with EOCRC tend to exhibit obesity-related changes in gut microbes, suggesting a potential role of obesity in EOCRC.
3.4 Obesity-induced inflammation in early-onset colorectal cancer
Rather than attributing microbiome dysbiosis and metabolic syndrome, obesity was regarded as a cause of inflammation (60, 132). Therefore, a proposed mechanism linking obesity and cancer involves obesity-induced low-grade inflammation (133). Later, the state of low-grade chronic inflammation was regarded as a risk factor for CRC and causes DNA and protein damage in the occurrence and development of CRC (132, 134). Markers of systemic inflammation are also elevated in obese patients with CRC metastasis and correlate with a worse prognosis (68). Similarly, inflammation is a higher risk factor for CRC in youth rather than in middle-aged and older patients (132). Therefore, obesity-induced inflammation may have a more important role in EOCRC carcinogenesis (135). In one study, obesity-induced low-grade systemic inflammation in the carcinogenic milieu of CRC, was associated with the generation of inflammatory cytokines through the interaction between infiltrating immune cells and adipocytes (136). Another study showed that systemic chronic inflammation accelerates oncogenic processes in the colon through the tumor-associated macrophages, which is associated with tumor growth and worse survival in patients with EOCRC (45). Leptin and adiponectin, two well-known hormones related to obesity, are associated with macrophage metabolism and polarization towards a proinflammatory state (137). Therefore, the distinct effect of obesity in EOCRC might account for inflammation.
Apart from systemic inflammation, intestinal inflammation is also widely known as a CRC-promoting factor, especially in inflammatory bowel disease (IBD)-related CRC (138). IBD causes chronic nonspecific inflammation in the intestinal tract (139). Studies have shown a poorer differentiation of IBD-related CRC compared with sporadic CRC, however, the tumor stage and survival rate did not differ significantly (140, 141). However, when patients were categorized by age, those younger than 50 had worse survival in the IBD-related CRC group (138, 142). Patients with EOCRC were more likely to have IBD than healthy individuals (143). Accordingly, a cohort study from 2011 to 2017 showed that patients with EOCRC have a higher rate of IBD than patients with LOCRC and the prevalence of IBD in EOCRC was nearly seven times as much in LOCRC (60, 144). Patients with IBD also had a threefold risk of developing EOCRC rather than those with LOCRC (143), and a sixfold higher incidence of EOCRC (85). Vajravelu et al. ranked inflammatory bowel disease, rather than a family history of CRC/polyps, as the important risk factor for EOCRC (31). Besides the clinical evidence, Arif et al. demonstrated that IBD-related EOCRC has a higher rate of perineural metastasis, poorer differentiation, and lymphovascular invasion (140). Therefore, IBD was confirmed as a risk factor for EOCRC, although the incidence of EOCRC remained lower in Asia than in the West (56). Interestingly, one retrospective study showed ulcerative colitis, as a stronger hazard factor of EOCRC compared with Crohn’s disease (145). Apart from a traditional normal-adenoma-adenocarcinoma sequence of CRC, colitis-associated CRC (CAC) follows the inflammation-dysplasia-carcinoma sequence and inflammation is regarded as the core process in CAC carcinogenesis (146). CAC correlates with intestinal inflammation, and has been proven to promote genetic alteration, oxidative stress-induced DNA damage, and immune dysregulation, indicating a worse prognosis than patients with no history of IBD (147, 148). Even though IBD and metabolic disorders were proven to be risk factors in obesity-related EOCRC, patients with IBD and having metabolic disease exhibited a lower incidence of EOCRC compared with those without metabolic disease (85).
4 Conclusions and future directions
However, it is crucial to recognize that not all individuals with EOCRC engage in unhealthy lifestyles or present with risk factors commonly linked to the condition. This observation suggests the existence of alternative etiological factors that may contribute to the pathogenesis of EOCRC in such individuals. A genetic predisposition, characterized by germline mutations in high-penetrance cancer susceptibility genes, is likely to be more prevalent among individuals with EOCRC, irrespective of the absence of overt lifestyle risk factors (149). For instance, germline mutations affecting DNA repair genes, exemplified by those observed in Lynch syndrome, can precipitate a heightened susceptibility to CRC at a younger age, independent of lifestyle elements (150). Furthermore, the gut microbiome and its interactions with host factors are gaining recognition as significant contributors to the etiology of CRC, encompassing early-onset presentations (151).
In recent decades, there has been a persistent increase in both the incidence and mortality of EOCRC, and the features of EOCRC are distinct from LOCRC. Compared to LOCRC, the risk of obesity appears to be higher in EOCRC. This review provides an insight into the possible mechanism of obesity-EOCRC interactions. The crosstalk between EOCRC and obesity is supported by clinical evidence, gut microbe, metabolic syndrome, and inflammation. Interestingly, glucagon-like peptide-1 (GLP-1) agonists play an effective role in treating type 2 diabetes and preventing obesity (152). The application of GLP-1 agonists might be a potential therapy for EOCRC. Therefore, future studies may focus on obesity in the prevention and treatment of EOCRC.
Author contributions
PX: Writing – original draft. ZT: Writing – original draft. HY: Writing – review & editing. CZ: Writing – review & editing.
Funding
The author(s) declare that no financial support was received for the research, authorship, and/or publication of this article.
Acknowledgments
A generative AI technology (Kimi, version 1.2.0, MOE model, https://kimi.moonshot.cn/) was used for writing statement “GLP-1 Agonists Might Improve the Outlook for Early Onset Colorectal Cancer”. We are responsible for checking the factual accuracy of any content created by the generative AI technology. We also clarified that the manuscript and figures were not written or produced by the generative AI technology.
Conflict of interest
The authors declare that the research was conducted in the absence of any commercial or financial relationships that could be construed as a potential conflict of interest.
Publisher’s note
All claims expressed in this article are solely those of the authors and do not necessarily represent those of their affiliated organizations, or those of the publisher, the editors and the reviewers. Any product that may be evaluated in this article, or claim that may be made by its manufacturer, is not guaranteed or endorsed by the publisher.
Glossary
References
1. Ng M, Fleming T, Robinson M, Thomson B, Graetz N, Margono C, et al. Global, regional, and national prevalence of overweight and obesity in children and adults during 1980-2013: a systematic analysis for the Global Burden of Disease Study 2013. Lancet. (2014) 384:766–81. doi: 10.1016/S0140-6736(14)60460-8
2. Caruso A, Gelsomino L, Panza S, Accattatis FM, Naimo GD, Barone I, et al. Leptin: A heavyweight player in obesity-related cancers. Biomolecules. (2023) 13:1084. doi: 10.3390/biom13071084
3. Bastien M, Poirier P, Lemieux I, Despres JP. Overview of epidemiology and contribution of obesity to cardiovascular disease. Prog Cardiovasc Dis. (2014) 56:369–81. doi: 10.1016/j.pcad.2013.10.016
4. von Deneen KM, Wei Q, Tian J, Liu Y. Obesity in China: what are the causes? Curr Pharm Des. (2011) 17:1132–9. doi: 10.2174/138161211795656765
5. Wang Y, Zhao L, Gao L, Pan A, Xue H. Health policy and public health implications of obesity in China. Lancet Diabetes Endocrinol. (2021) 9:446–61. doi: 10.1016/S2213-8587(21)00118-2
6. Bardou M, Rouland A, Martel M, Loffroy R, Barkun AN, Chapelle N. Review article: obesity and colorectal cancer. Aliment Pharmacol Ther. (2022) 56:407–18. doi: 10.1111/apt.17045
7. Nguyen LH, Cao Y, Batyrbekova N, Roelstraete B, Ma W, Khalili H, et al. Antibiotic therapy and risk of early-onset colorectal cancer: A national case-control study. Clin Transl Gastroenterol. (2022) 13:e437. doi: 10.14309/ctg.0000000000000437
8. Di Leo M, Zuppardo RA, Puzzono M, Ditonno I, Mannucci A, Antoci G, et al. Risk factors and clinical characteristics of early-onset colorectal cancer vs. late-onset colorectal cancer: a case-case study. Eur J Gastroenterol Hepatol. (2021) 33:1153–60. doi: 10.1097/MEG.0000000000002000
9. Foppa C, Maroli A, Lauricella S, Luberto A, La Raja C, Bunino F, et al. Different oncologic outcomes in early-onset and late-onset sporadic colorectal cancer: A regression analysis on 2073 patients. Cancers (Basel). (2022) 14:6239. doi: 10.3390/cancers14246239
10. Li J, Ma X, Chakravarti D, Shalapour S, DePinho RA. Genetic and biological hallmarks of colorectal cancer. Genes Dev. (2021) 35:787–820. doi: 10.1101/gad.348226.120
11. O’Sullivan DE, Sutherland RL, Town S, Chow K, Fan J, Forbes N, et al. Risk factors for early-onset colorectal cancer: A systematic review and meta-analysis. Clin Gastroenterol Hepatol. (2022) 20:1229–40. doi: 10.1016/j.cgh.2021.01.037
12. Al ZA, Al SA, Sayed S, Al AH, Al FF, Al AO, et al. Early onset colorectal cancer in Arabs, are we dealing with a distinct disease? Cancers (Basel). (2023) 15:889. doi: 10.3390/cancers15030889
13. Stoffel EM, Murphy CC. Epidemiology and mechanisms of the increasing incidence of colon and rectal cancers in young adults. Gastroenterology. (2020) 158:341–53. doi: 10.1053/j.gastro.2019.07.055
14. Ochs-Balcom HM, Kanth P, Farnham JM, Abdelrahman S, Cannon-Albright LA. Colorectal cancer risk based on extended family history and body mass index. Genet Epidemiol. (2020) 44:778–84. doi: 10.1002/gepi.22338
15. Chang SH, Patel N, Du M, Liang PS. Trends in early-onset vs late-onset colorectal cancer incidence by race/ethnicity in the United States cancer statistics database. Clin Gastroenterol Hepatol. (2022) 20:e1365–77. doi: 10.1016/j.cgh.2021.07.035
16. Anupriya S, Chakraborty A, Patnaik S. Clonal evolution and expansion associated with therapy resistance and relapse of colorectal cancer. Mutat Res Rev Mutat Res. (2022) 790:108445. doi: 10.1016/j.mrrev.2022.108445
17. Mauri G, Sartore-Bianchi A, Russo AG, Marsoni S, Bardelli A, Siena S. Early-onset colorectal cancer in young individuals. Mol Oncol. (2019) 13:109–31. doi: 10.1002/1878-0261.12417
18. Chen B, Dragomir MP, Fabris L, Bayraktar R, Knutsen E, Liu X, et al. The long noncoding RNA CCAT2 induces chromosomal instability through BOP1-AURKB signaling. Gastroenterology. (2020) 159:2146–62. doi: 10.1053/j.gastro.2020.08.018
19. Nfonsam V, Wusterbarth E, Gong A, Vij P. Early-onset colorectal cancer. Surg Oncol Clin N Am. (2022) 31:143–55. doi: 10.1016/j.soc.2021.11.001
20. Taieb J, Svrcek M, Cohen R, Basile D, Tougeron D, Phelip JM. Deficient mismatch repair/microsatellite unstable colorectal cancer: Diagnosis, prognosis and treatment. Eur J Cancer. (2022) 175:136–57. doi: 10.1016/j.ejca.2022.07.020
21. Cercek A, Chatila WK, Yaeger R, Walch H, Fernandes G, Krishnan A, et al. A comprehensive comparison of early-onset and average-onset colorectal cancers. J Natl Cancer Inst. (2021) 113:1683–92. doi: 10.1093/jnci/djab124
22. Xie Y, Jiao X, Zeng M, Fan Z, Li X, Yuan Y, et al. Clinical significance of Fusobacterium nucleatum and microsatellite instability in evaluating colorectal cancer prognosis. Cancer Manag Res. (2022) 14:3021–36. doi: 10.2147/CMAR.S382464
23. Zaborowski AM. Colorectal cancer in the young: research in early age colorectal cancer trends (REACCT) collaborative. Cancers. (2023) 15:2979. doi: 10.3390/cancers15112979
24. Willauer AN, Liu Y, Pereira A, Lam M, Morris JS, Raghav K, et al. Clinical and molecular characterization of early-onset colorectal cancer. Cancer Am Cancer Soc. (2019) 125:2002–10. doi: 10.1002/cncr.31994
25. Ribic CM, Sargent DJ, Moore MJ, Thibodeau SN, French AJ, Goldberg RM, et al. Tumor microsatellite-instability status as a predictor of benefit from fluorouracil-based adjuvant chemotherapy for colon cancer. N Engl J Med. (2003) 349:247–57. doi: 10.1056/NEJMoa022289
26. Dos SW, de Andrade ES, Garcia F, Campacci N, Sabato C, Melendez ME, et al. Whole-exome sequencing identifies pathogenic germline variants in patients with lynch-like syndrome. Cancers (Basel). (2022) 14:4233. doi: 10.3390/cancers14174233
27. Liu K, Lei S, Kuang Y, Jin Q, Long D, Liu C, et al. A novel mechanism of the c-Myc/NEAT1 axis mediating colorectal cancer cell response to photodynamic therapy treatment. Front Oncol. (2021) 11:652831. doi: 10.3389/fonc.2021.652831
28. Zhang L, Shay JW. Multiple roles of APC and its therapeutic implications in colorectal cancer. J Natl Cancer Inst. (2017) 109:djw332. doi: 10.1093/jnci/djw332
29. Marx OM, Mankarious MM, Eshelman MA, Ding W, Koltun WA, Yochum GS. Transcriptome analyses identify deregulated MYC in early onset colorectal cancer. Biomolecules. (2022) 12:1223. doi: 10.3390/biom12091223
30. Aitchison A, Hakkaart C, Day RC, Morrin HR, Frizelle FA, Keenan JI. APC mutations are not confined to hotspot regions in early-onset colorectal cancer. Cancers (Basel). (2020) 12:3829. doi: 10.3390/cancers12123829
31. Vajravelu RK, Mehta SJ, Lewis JD. Understanding characteristics of who undergoes testing is crucial for the development of diagnostic strategies to identify individuals at risk for early-age onset colorectal cancer. Gastroenterology. (2021) 160:993–8. doi: 10.1053/j.gastro.2020.11.058
32. Christodoulides N, Lami M, Malietzis G, Rasheed S, Tekkis P, Kontovounisios C. Sporadic colorectal cancer in adolescents and young adults: a scoping review of a growing healthcare concern. Int J Colorectal Dis. (2020) 35:1413–21. doi: 10.1007/s00384-020-03660-5
33. Xi Y, Yuefen P, Wei W, Quan Q, Jing Z, Jiamin X, et al. Analysis of prognosis, genome, microbiome, and microbial metabolome in different sites of colorectal cancer. J Transl Med. (2019) 17:353. doi: 10.1186/s12967-019-2102-1
34. Weinberg BA, Marshall JL. Colon cancer in young adults: trends and their implications. Curr Oncol Rep. (2019) 21:3. doi: 10.1007/s11912-019-0756-8
35. Nitsche U, Zimmermann A, Spath C, Muller T, Maak M, Schuster T, et al. Mucinous and signet-ring cell colorectal cancers differ from classical adenocarcinomas in tumor biology and prognosis. Ann Surg. (2013) 258:775–82, 782-3. doi: 10.1097/SLA.0b013e3182a69f7e
36. Wu CW, Lui RN. Early-onset colorectal cancer: Current insights and future directions. World J Gastrointest Oncol. (2022) 14:230–41. doi: 10.4251/wjgo.v14.i1.230
37. Wu M, Huang M, He C, Chen C, Li H, Wang J, et al. Risk of second primary Malignancies based on the histological subtypes of colorectal cancer. Front Oncol. (2021) 11:650937. doi: 10.3389/fonc.2021.650937
38. He X, Wu W, Ding Y, Li Y, Si J, Sun L. Excessive risk of second primary cancers in young-onset colorectal cancer survivors. Cancer Med. (2018) 7:1201–10. doi: 10.1002/cam4.1437
39. Venugopal A, Carethers JM. Epidemiology and biology of early onset colorectal cancer. Excli J. (2022) 21:162–82. doi: 10.17179/excli2021-4456
40. Ding X, Yang X, Wu D, Huang Y, Dai Y, Li J, et al. Nomogram predicting the cancer-specific survival of early-onset colorectal cancer patients with synchronous liver metastasis: a population-based study. Int J Colorectal Dis. (2022) 37:1309–19. doi: 10.1007/s00384-022-04175-x
41. Mikaeel RR, Young JP, Li Y, Smith E, Horsnell M, Uylaki W, et al. Survey of germline variants in cancer-associated genes in young adults with colorectal cancer. Genes Chromosomes Cancer. (2022) 61:105–13. doi: 10.1002/gcc.23011
42. Kibriya MG, Raza M, Quinn A, Kamal M, Ahsan H, Jasmine F. A transcriptome and methylome study comparing tissues of early and late onset colorectal carcinoma. Int J Mol Sci. (2022) 23:14261. doi: 10.3390/ijms232214261
43. Fontana E, Meyers J, Sobrero A, Iveson T, Shields AF, Taieb J, et al. Early-onset colorectal adenocarcinoma in the IDEA database: treatment adherence, toxicities, and outcomes with 3 and 6 months of adjuvant fluoropyrimidine and oxaliplatin. J Clin Oncol. (2021) 39:4009–19. doi: 10.1200/JCO.21.02008
44. Alvarez K, Cassana A, de la Fuente M, Canales T, Abedrapo M, Lopez-Kostner F. Clinical, pathological and molecular characteristics of Chilean patients with early-, intermediate- and late-onset colorectal cancer. Cells-Basel. (2021) 10:631. doi: 10.3390/cells10030631
45. McClelland PH, Liu T, Ozuner G. Early-onset colorectal cancer in patients under 50 years of age: demographics, disease characteristics, and survival. Clin Colorectal Cancer. (2022) 21:e135–44. doi: 10.1016/j.clcc.2021.11.003
46. Andersen CJ, Murphy KE, Fernandez ML. Impact of obesity and metabolic syndrome on immunity. Adv Nutr. (2016) 7:66–75. doi: 10.3945/an.115.010207
47. Cox AJ, West NP, Cripps AW. Obesity, inflammation, and the gut microbiota. Lancet Diabetes Endocrinol. (2015) 3:207–15. doi: 10.1016/S2213-8587(14)70134-2
48. Harlid S, Myte R, Van Guelpen B. The metabolic syndrome, inflammation, and colorectal cancer risk: an evaluation of large panels of plasma protein markers using repeated, prediagnostic samples. Mediators Inflammation. (2017) 2017:4803156. doi: 10.1155/2017/4803156
49. Esposito K, Chiodini P, Colao A, Lenzi A, Giugliano D. Metabolic syndrome and risk of cancer: a systematic review and meta-analysis. Diabetes Care. (2012) 35:2402–11. doi: 10.2337/dc12-0336
50. Colditz GA. Combined individual participant data: highest-level evidence on obesity and colorectal cancer molecular subtypes. J Natl Cancer Inst. (2023) 115:120–1. doi: 10.1093/jnci/djac216
51. Ye P, Xi Y, Huang Z, Xu P. Linking obesity with colorectal cancer: epidemiology and mechanistic insights. Cancers (Basel). (2020) 12:1408. doi: 10.3390/cancers12061408
52. Wolin KY, Yan Y, Colditz GA, Lee IM. Physical activity and colon cancer prevention: a meta-analysis. Br J Cancer. (2009) 100:611–6. doi: 10.1038/sj.bjc.6604917
53. Dairi O, Anderson JC, Butterly LF. Why is colorectal cancer increasing in younger age groups in the United States? Expert Rev Gastroenterol Hepatol. (2021) 15:623–32. doi: 10.1080/17474124.2021.1876561
54. Low EE, Demb J, Liu L, Earles A, Bustamante R, Williams CD, et al. Risk factors for early-onset colorectal cancer. Gastroenterology. (2020) 159:492–501. doi: 10.1053/j.gastro.2020.01.004
55. Karahalios A, English DR, Simpson JA. Weight change and risk of colorectal cancer: a systematic review and meta-analysis. Am J Epidemiol. (2015) 181:832–45. doi: 10.1093/aje/kwu357
56. Jin EH, Han K, Lee DH, Shin CM, Lim JH, Choi YJ, et al. Association between metabolic syndrome and the risk of colorectal cancer diagnosed before age 50 years according to tumor location. Gastroenterology. (2022) 163:637–48. doi: 10.1053/j.gastro.2022.05.032
57. Chen FW, Yang L, Cusumano VT, Chong MC, Lin JK, Partida D, et al. Early-onset colorectal cancer is associated with a lower risk of metachronous advanced neoplasia than traditional-onset colorectal cancer. Dig Dis Sci. (2022) 67:1045–53. doi: 10.1007/s10620-021-06902-w
58. Lo CH, He X, Hang D, Wu K, Ogino S, Chan AT, et al. Body fatness over the life course and risk of serrated polyps and conventional adenomas. Int J Cancer. (2020) 147:1831–44. doi: 10.1002/ijc.32958
59. Carroll KL, Fruge AD, Heslin MJ, Lipke EA, Greene MW. Diet as a risk factor for early-onset colorectal adenoma and carcinoma: A systematic review. Front Nutr. (2022) 9:896330. doi: 10.3389/fnut.2022.896330
60. Abdullah M, Sukartini N, Nursyirwan SA, Pribadi RR, Maulahela H, Utari AP, et al. Gut microbiota profiles in early- and late-onset colorectal cancer: A potential diagnostic biomarker in the future. Digestion. (2021) 102:823–32. doi: 10.1159/000516689
61. Deng JW, Zhou YL, Dai WX, Chen HM, Zhou CB, Zhu CQ, et al. Noninvasive predictive models based on lifestyle analysis and risk factors for early-onset colorectal cancer. J Gastroenterol Hepatol. (2023) 38:1768–77. doi: 10.1111/jgh.16243
62. Song H, Sontz RA, Vance MJ, Morris JM, Sheriff S, Zhu S, et al. High-fat diet plus HNF1A variant promotes polyps by activating beta-catenin in early-onset colorectal cancer. JCI Insight. (2023) 8:e167163. doi: 10.1172/jci.insight.167163
63. Nguyen LH, Cao Y, Hur J, Mehta RS, Sikavi DR, Wang Y, et al. The sulfur microbial diet is associated with increased risk of early-onset colorectal cancer precursors. Gastroenterology. (2021) 161:1423–32. doi: 10.1053/j.gastro.2021.07.008
64. Nugent TS, Kelly ME, Donlon NE, Fahy MR, Larkin JO, McCormick PH, et al. Obesity and anastomotic leak rates in colorectal cancer: a meta-analysis. Int J Colorectal Dis. (2021) 36:1819–29. doi: 10.1007/s00384-021-03909-7
65. Chung KC, Lee KC, Chen HH, Cheng KC, Wu KL, Song LC. Path analysis of the impact of obesity on postoperative outcomes in colorectal cancer patients: A population-based study. J Clin Med. (2021) 10:2904. doi: 10.3390/jcm10132904
66. O’Keefe SJ. Diet, microorganisms and their metabolites, and colon cancer. Nat Rev Gastroenterol Hepatol. (2016) 13:691–706. doi: 10.1038/nrgastro.2016.165
67. Ecker J, Benedetti E, Kindt A, Horing M, Perl M, Machmuller AC, et al. The colorectal cancer lipidome: identification of a robust tumor-specific lipid species signature. Gastroenterology. (2021) 161:910–23. doi: 10.1053/j.gastro.2021.05.009
68. Davis JS, Chavez JC, Kok M, San MY, Lee HY, Henderson H, et al. Association of prediagnosis obesity and postdiagnosis aspirin with survival from stage IV colorectal cancer. JAMA. (2022) 5:e2236357. doi: 10.1001/jamanetworkopen.2022.36357
69. Porro S, Genchi VA, Cignarelli A, Natalicchio A, Laviola L, Giorgino F, et al. Dysmetabolic adipose tissue in obesity: morphological and functional characteristics of adipose stem cells and mature adipocytes in healthy and unhealthy obese subjects. J Endocrinol Invest. (2021) 44:921–41. doi: 10.1007/s40618-020-01446-8
70. Feliciano E, Winkels RM, Meyerhardt JA, Prado CM, Afman LA, Caan BJ. Abdominal adipose tissue radiodensity is associated with survival after colorectal cancer. Am J Clin Nutr. (2021) 114:1917–24. doi: 10.1093/ajcn/nqab285
71. Shen J, Wu Y, Mo M, Feng X, Zhou C, Wang Z, et al. Risk factors associated with early-onset colorectal neoplasm in Chinese youth: A prospective population-based study. Front Oncol. (2021) 11:702322. doi: 10.3389/fonc.2021.702322
72. Li H, Boakye D, Chen X, Hoffmeister M, Brenner H. Association of body mass index with risk of early-onset colorectal cancer: systematic review and meta-analysis. Am J Gastroenterol. (2021) 116:2173–83. doi: 10.14309/ajg.0000000000001393
73. Elangovan A, Skeans J, Landsman M, Ali S, Elangovan AG, Kaelber DC, et al. Colorectal cancer, age, and obesity-related comorbidities: A large database study. Dig Dis Sci. (2021) 66:3156–63. doi: 10.1007/s10620-020-06602-x
74. Gan T, Schaberg KB, He D, Mansour A, Kapoor H, Wang C, et al. Association between obesity and histological tumor budding in patients with nonmetastatic colon cancer. JAMA Netw Open. (2021) 4:e213897. doi: 10.1001/jamanetworkopen.2021.3897
75. Nakamura T, Mitomi H, Kikuchi S, Ohtani Y, Sato K. Evaluation of the usefulness of tumor budding on the prediction of metastasis to the lung and liver after curative excision of colorectal cancer. Hepatogastroenterology. (2005) 52:1432–5. doi: 10.1136/gut.2005.070656
76. Okuyama T, Nakamura T, Yamaguchi M. Budding is useful to select high-risk patients in stage II well-differentiated or moderately differentiated colon adenocarcinoma. Dis Colon Rectum. (2003) 46:1400–6. doi: 10.1007/s10350-004-6757-0
77. Kang G, Pyo JS, Kim NY, Kang DW. Clinicopathological significances and prognostic role of intratumoral budding in colorectal cancers. J Clin Med. (2022) 11:5540. doi: 10.3390/jcm11195540
78. Pan AF, Zheng NX, Wang J, Kabemba J, Zheng K, Shen F, et al. Role of perirectal fat in the carcinogenesis and development of early-onset rectal cancer. J Oncol. (2022) 2022:4061142. doi: 10.1155/2022/4061142
79. Almasaudi AS, Dolan RD, Edwards CA, McMillan DC. Hypoalbuminemia reflects nutritional risk, body composition and systemic inflammation and is independently associated with survival in patients with colorectal cancer. Cancers (Basel). (2020) 12:1986. doi: 10.3390/cancers12071986
80. Reddy S, Mouchli A, Bierle L, Gerrard M, Walsh C, Mir A, et al. Assessing presenting symptoms, co-morbidities, and risk factors for mortality in underserved patients with non-hereditary early-onset colorectal cancer. Cureus. (2021) 13:e16117. doi: 10.7759/cureus.16117
81. Boulange CL, Neves AL, Chilloux J, Nicholson JK, Dumas ME. Impact of the gut microbiota on inflammation, obesity, and metabolic disease. Genome Med. (2016) 8:42. doi: 10.1186/s13073-016-0303-2
82. Lu B, Qian JM, Li JN. The metabolic syndrome and its components as prognostic factors in colorectal cancer: A meta-analysis and systematic review. J Gastroenterol Hepatol. (2023) 38:187–96. doi: 10.1111/jgh.16042
83. Tran TT, Gunathilake M, Lee J, Kim J. Association between metabolic syndrome and its components and incident colorectal cancer in a prospective cohort study. Cancer Am Cancer Soc. (2022) 128:1230–41. doi: 10.1002/cncr.34027
84. Han F, Wu G, Zhang S, Zhang J, Zhao Y, Xu J. The association of Metabolic Syndrome and its Components with the Incidence and Survival of Colorectal Cancer: A Systematic Review and Meta-analysis. Int J Biol Sci. (2021) 17:487–97. doi: 10.7150/ijbs.52452
85. Lundqvist E, Myrberg IH, Boman SE, Saraste D, Weibull CE, Landerholm K, et al. Autoimmune and metabolic diseases and the risk of early-onset colorectal cancer, a nationwide nested case-control study. Cancers (Basel). (2023) 15:688. doi: 10.3390/cancers15030688
86. Chen H, Zheng X, Zong X, Li Z, Li N, Hur J, et al. Metabolic syndrome, metabolic comorbid conditions and risk of early-onset colorectal cancer. Gut. (2021) 70:1147–54. doi: 10.1136/gutjnl-2020-321661
87. Lazarova D, Bordonaro M. Multifactorial causation of early onset colorectal cancer. J Cancer. (2021) 12:6825–34. doi: 10.7150/jca.63676
88. Lu L, Koo S, McPherson S, Hull MA, Rees CJ, Sharp L. Systematic review and meta-analysis: Associations between metabolic syndrome and colorectal neoplasia outcomes. Colorectal Dis. (2022) 24:681–94. doi: 10.1111/codi.16092
89. Hu C, Zhang Q, Jin X, Zhang L, Zhang Y, Zhu Q, et al. A paradox between preoperative overweight/obesity and change in weight during postoperative chemotherapy and its relationship to survival in stage II and III colorectal cancer patients. Clin Nutr. (2021) 40:2410–9. doi: 10.1016/j.clnu.2020.10.039
90. Yuhara H, Steinmaus C, Cohen SE, Corley DA, Tei Y, Buffler PA. Is diabetes mellitus an independent risk factor for colon cancer and rectal cancer? Am J Gastroenterol. (2011) 106:1911–21, 1922. doi: 10.1038/ajg.2011.301
91. Dong W, Kim U, Rose J, Hoehn RS, Kucmanic M, Eom K, et al. Geographic variation and risk factor association of early versus late onset colorectal cancer. Cancers (Basel). (2023) 15:1006. doi: 10.3390/cancers15041006
92. Yu GH, Li SF, Wei R, Jiang Z. Diabetes and colorectal cancer risk: clinical and therapeutic implications. J Diabetes Res. (2022) 2022:1747326. doi: 10.1155/2022/1747326
93. Feng Q, Tang LJ, Luo DH, Wang Y, Wu N, Chen H, et al. Metabolic syndrome-related hyperuricemia is associated with a poorer prognosis in patients with colorectal cancer: A multicenter retrospective study. Cancer Manag Res. (2021) 13:8809–19. doi: 10.2147/CMAR.S338783
94. Li Z, Pan W, Shen Y, Chen Z, Zhang L, Zhang Y, et al. IGF1/IGF1R and microRNA let-7e down-regulate each other and modulate proliferation and migration of colorectal cancer cells. Cell Cycle. (2018) 17:1212–9. doi: 10.1080/15384101.2018.1469873
95. Petrelli F, Ghidini M, Rausa E, Ghidini A, Cabiddu M, Borgonovo K, et al. Survival of colorectal cancer patients with diabetes mellitus: A meta-analysis. Can J Diabetes. (2021) 45:186–97. doi: 10.1016/j.jcjd.2020.06.009
96. Rosenberg K. Sugar-sweetened beverage intake linked to early-onset colorectal cancer risk. Am J Nurs. (2021) 121:52. doi: 10.1097/01.NAJ.0000794268.40128.c1
97. Jimba T, Kaneko H, Yano Y, Itoh H, Yotsumoto H, Seki H, et al. Relation of the metabolic syndrome to incident colorectal cancer in young adults aged 20 to 49 years. Am J Cardiol. (2021) 158:132–8. doi: 10.1016/j.amjcard.2021.07.049
98. van Duijnhoven FJ, Bueno-De-Mesquita HB, Calligaro M, Jenab M, Pischon T, Jansen EH, et al. Blood lipid and lipoprotein concentrations and colorectal cancer risk in the European Prospective Investigation into Cancer and Nutrition. Gut. (2011) 60:1094–102. doi: 10.1136/gut.2010.225011
99. Tang CT, Li J, Yang Z, Zeng C, Chen Y. Comparison of some biochemical markers between early-onset and late-onset colorectal precancerous lesions: A single-center retrospective study. J Clin Lab Anal. (2022) 36:e24637. doi: 10.1002/jcla.24637
100. Schumacher AJ, Chen Q, Attaluri V, McLemore EC, Chao CR. Metabolic risk factors associated with early-onset colorectal adenocarcinoma: A case-control study at Kaiser Permanente Southern California. Cancer Epidemiol Biomarkers Prev. (2021) 30:1792–8. doi: 10.1158/1055-9965.EPI-20-1127
101. Trabulo D, Ribeiro S, Martins C, Teixeira C, Cardoso C, Mangualde J, et al. Metabolic syndrome and colorectal neoplasms: An ominous association. World J Gastroenterol. (2015) 21:5320–7. doi: 10.3748/wjg.v21.i17.5320
102. Zhang Y, Song M, Chan AT, Meyerhardt JA, Willett WC, Giovannucci EL. Long-term use of antihypertensive medications, hypertension and colorectal cancer risk and mortality: a prospective cohort study. Br J Cancer. (2022) 127:1974–82. doi: 10.1038/s41416-022-01975-4
103. Zheng Y, Meng L, Liu H, Sun L, Nie Y, Wu Q, et al. Let food be thy medicine: the role of diet in colorectal cancer: a narrative review. J Gastrointest Oncol. (2022) 13:2020–32. doi: 10.21037/jgo
104. Syed AR, Thakkar P, Horne ZD, Abdul-Baki H, Kochhar G, Farah K, et al. Old vs new: Risk factors predicting early onset colorectal cancer. World J Gastrointest Oncol. (2019) 11:1011–20. doi: 10.4251/wjgo.v11.i11.1011
105. Ma Y, Lin C, Liu S, Wei Y, Ji C, Shi F, et al. Radiomics features based on internal and marginal areas of the tumor for the preoperative prediction of microsatellite instability status in colorectal cancer. Front Oncol. (2022) 12:1020349. doi: 10.3389/fonc.2022.1020349
106. Villeger R, Lopes A, Veziant J, Gagniere J, Barnich N, Billard E, et al. Microbial markers in colorectal cancer detection and/or prognosis. World J Gastroenterol. (2018) 24:2327–47. doi: 10.3748/wjg.v24.i22.2327
107. Song M, Chan AT, Sun J. Influence of the gut microbiome, diet, and environment on risk of colorectal cancer. Gastroenterology. (2020) 158:322–40. doi: 10.1053/j.gastro.2019.06.048
108. Han S, Zhuang J, Pan Y, Wu W, Ding K. Different characteristics in gut microbiome between advanced adenoma patients and colorectal cancer patients by metagenomic analysis. Microbiol Spectr. (2022) 10:e159322. doi: 10.1128/spectrum.01593-22
109. Xu Z, Lv Z, Chen F, Zhang Y, Xu Z, Huo J, et al. Dysbiosis of human tumor microbiome and aberrant residence of Actinomyces in tumor-associated fibroblasts in young-onset colorectal cancer. Front Immunol. (2022) 13:1008975. doi: 10.3389/fimmu.2022.1008975
110. Tsoi H, Chu E, Zhang X, Sheng J, Nakatsu G, Ng SC, et al. Peptostreptococcus anaerobius induces intracellular cholesterol biosynthesis in colon cells to induce proliferation and causes dysplasia in mice. Gastroenterology. (2017) 152:1419–33. doi: 10.1053/j.gastro.2017.01.009
111. Jan G, Belzacq AS, Haouzi D, Rouault A, Metivier D, Kroemer G, et al. Propionibacteria induce apoptosis of colorectal carcinoma cells via short-chain fatty acids acting on mitochondria. Cell Death Differ. (2002) 9:179–88. doi: 10.1038/sj/cdd/4400935
112. Zhang Y, Zhang J, Duan L. The role of microbiota-mitochondria crosstalk in pathogenesis and therapy of intestinal diseases. Pharmacol Res. (2022) 186:106530. doi: 10.1016/j.phrs.2022.106530
113. Park EM, Chelvanambi M, Bhutiani N, Kroemer G, Zitvogel L, Wargo JA. Targeting the gut and tumor microbiota in cancer. Nat Med. (2022) 28:690–703. doi: 10.1038/s41591-022-01779-2
114. Wong SH, Yu J. Gut microbiota in colorectal cancer: mechanisms of action and clinical applications. Nat Rev Gastroenterol Hepatol. (2019) 16:690–704. doi: 10.1038/s41575-019-0209-8
115. Kong C, Liang L, Liu G, Du L, Yang Y, Liu J, et al. Integrated metagenomic and metabolomic analysis reveals distinct gut-microbiome-derived phenotypes in early-onset colorectal cancer. Gut. (2023) 72:1129–42. doi: 10.1136/gutjnl-2022-327156
116. Yu I, Wu R, Tokumaru Y, Terracina KP, Takabe K. The role of the microbiome on the pathogenesis and treatment of colorectal cancer. Cancers (Basel). (2022) 14:5685. doi: 10.3390/cancers14225685
117. Xiong H, Wang J, Chang Z, Hu H, Yuan Z, Zhu Y, et al. Gut microbiota display alternative profiles in patients with early-onset colorectal cancer. Front Cell Infect Microbiol. (2022) 12:1036946. doi: 10.3389/fcimb.2022.1036946
118. Genua F, Raghunathan V, Jenab M, Gallagher WM, Hughes DJ. The role of gut barrier dysfunction and microbiome dysbiosis in colorectal cancer development. Front Oncol. (2021) 11:626349. doi: 10.3389/fonc.2021.626349
119. Huang P, Liu Y. A reasonable diet promotes balance of intestinal microbiota: prevention of precolorectal cancer. BioMed Res Int. (2019) 2019:3405278. doi: 10.1155/2019/3405278
120. Liu C, Yao Z, Wang J, Zhang W, Yang Y, Zhang Y, et al. Macrophage-derived CCL5 facilitates immune escape of colorectal cancer cells via the p65/STAT3-CSN5-PD-L1 pathway. Cell Death Differ. (2020) 27:1765–81. doi: 10.1038/s41418-019-0460-0
121. Zhang YK, Zhang Q, Wang YL, Zhang WY, Hu HQ, Wu HY, et al. A comparison study of age and colorectal cancer-related gut bacteria. Front Cell Infect Microbiol. (2021) 11:606490. doi: 10.3389/fcimb.2021.606490
122. Ai ZL, Zhang X, Ge W, Zhong YB, Wang HY, Zuo ZY, et al. Salvia miltiorrhiza extract may exert an anti-obesity effect in rats with high-fat diet-induced obesity by modulating gut microbiome and lipid metabolism. World J Gastroenterol. (2022) 28:6131–56. doi: 10.3748/wjg.v28.i43.6131
123. Petersen C, Bell R, Klag KA, Lee SH, Soto R, Ghazaryan A, et al. T cell-mediated regulation of the microbiota protects against obesity. Science. (2019) 365:eaat9351. doi: 10.1126/science.aat9351
124. Dong TS, Luu K, Lagishetty V, Sedighian F, Woo SL, Dreskin BW, et al. The intestinal microbiome predicts weight loss on a calorie-restricted diet and is associated with improved hepatic steatosis. Front Nutr. (2021) 8:718661. doi: 10.3389/fnut.2021.718661
125. Vitali F, Tortora K, Di Paola M, Bartolucci G, Menicatti M, De Filippo C, et al. Intestinal microbiota profiles in a genetic model of colon tumorigenesis correlates with colon cancer biomarkers. Sci Rep. (2022) 12:1432. doi: 10.1038/s41598-022-05249-0
126. Wang Y, Wen Y, Wang J, Lai X, Xu Y, Zhang X, et al. Clinicopathological differences of high Fusobacterium nucleatum levels in colorectal cancer: A review and meta-analysis. Front Microbiol. (2022) 13:945463. doi: 10.3389/fmicb.2022.945463
127. Perichon B, Lichtl-Hafele J, Bergsten E, Delage V, Trieu-Cuot P, Sansonetti P, et al. Detection of Streptococcus gallolyticus and Four Other CRC-Associated Bacteria in Patient Stools Reveals a Potential “Driver” Role for Enterotoxigenic Bacteroides fragilis. Front Cell Infect Microbiol. (2022) 12:794391. doi: 10.3389/fcimb.2022.794391
128. Qiu D, Xia Z, Deng J, Jiao X, Liu L, Li J. Glucorticoid-induced obesity individuals have distinct signatures of the gut microbiome. Biofactors. (2019) 45:892–901. doi: 10.1002/biof.1565
129. Duan M, Wang Y, Zhang Q, Zou R, Guo M, Zheng H. Characteristics of gut microbiota in people with obesity. PloS One. (2021) 16:e255446. doi: 10.1371/journal.pone.0255446
130. Gomes S, Baltazar F, Silva E, Preto A. Microbiota-derived short-chain fatty acids: new road in colorectal cancer therapy. Pharmaceutics. (2022) 14. doi: 10.3390/pharmaceutics14112359
131. Xiong S, Liu K, Yang F, Dong Y, Zhang H, Wu P, et al. Global research trends on inflammatory bowel diseases and colorectal cancer: A bibliometric and visualized study from 2012 to 2021. Front Oncol. (2022) 12:943294. doi: 10.3389/fonc.2022.943294
132. Liu T, Fan Y, Zhang Q, Wang Y, Yao N, Song M, et al. The combination of metabolic syndrome and inflammation increased the risk of colorectal cancer. Inflammation Res. (2022) 71:899–909. doi: 10.1007/s00011-022-01597-9
133. Shimi G, Pourvali K, Ghorbani A, Nooshin S, Zare KS, Iranirad R, et al. Alterations of DNA methylation and expression of genes related to thyroid hormone metabolism in colon epithelium of obese patients. BMC Med Genomics. (2022) 15:229. doi: 10.1186/s12920-022-01387-6
134. Cirillo F, Catellani C, Sartori C, Lazzeroni P, Amarri S, Street ME. Obesity, insulin resistance, and colorectal cancer: could miRNA dysregulation play A role? Int J Mol Sci. (2019) 20:2922. doi: 10.3390/ijms20122922
135. Scheurlen KM, Billeter AT, O’Brien SJ, Galandiuk S. Metabolic dysfunction and early-onset colorectal cancer - how macrophages build the bridge. Cancer Med. (2020) 9:6679–93. doi: 10.1002/cam4.3315
136. Harvey AE, Lashinger LM, Hursting SD. The growing challenge of obesity and cancer: an inflammatory issue. Ann N Y Acad Sci. (2011) 1229:45–52. doi: 10.1111/j.1749-6632.2011.06096.x
137. Scheurlen KM, Chariker JH, Kanaan Z, Littlefield AB, George JB, Seraphine C, et al. The NOTCH4-GATA4-IRG1 axis as a novel target in early-onset colorectal cancer. Cytokine Growth Factor Rev. (2022) 67:25–34. doi: 10.1016/j.cytogfr.2022.06.002
138. Andersen NN, Jess T. Has the risk of colorectal cancer in inflammatory bowel disease decreased? World J Gastroenterol. (2013) 19:7561–8. doi: 10.3748/wjg.v19.i43.7561
139. Zhao H, Zhang W, Cheng D, You L, Huang Y, Lu Y. Investigating dysbiosis and microbial treatment strategies in inflammatory bowel disease based on two modified Koch’s postulates. Front Med (Lausanne). (2022) 9:1023896. doi: 10.3389/fmed.2022.1023896
140. Arif AA, Chahal D, Ladua GK, Bhang E, Salh B, Rosenfeld G, et al. Hereditary and inflammatory bowel disease-related early onset colorectal cancer have unique characteristics and clinical course compared with sporadic disease. Cancer Epidemiol Biomarkers Prev. (2021) 30:1785–91. doi: 10.1158/1055-9965.EPI-21-0507
141. Reynolds IS, O’Toole A, Deasy J, McNamara DA, Burke JP. A meta-analysis of the clinicopathological characteristics and survival outcomes of inflammatory bowel disease associated colorectal cancer. Int J Colorectal Dis. (2017) 32:443–51. doi: 10.1007/s00384-017-2754-3
142. Bogach J, Pond G, Eskicioglu C, Seow H. Age-related survival differences in patients with inflammatory bowel disease-associated colorectal cancer: A population-based cohort study. Inflammation Bowel Dis. (2019) 25:1957–65. doi: 10.1093/ibd/izz088
143. Ahmad KS, Raja AR, Nawawi K, Ahmad HF, Mokhtar NM. Gut dysbiosis and intestinal barrier dysfunction: potential explanation for early-onset colorectal cancer. Front Cell Infect Microbiol. (2021) 11:744606. doi: 10.3389/fcimb.2021.744606
144. Gausman V, Dornblaser D, Anand S, Hayes RB, O’Connell K, Du M, et al. Risk factors associated with early-onset colorectal cancer. Clin Gastroenterol Hepatol. (2020) 18:2752–9. doi: 10.1016/j.cgh.2019.10.009
145. Danial D, Youssef ED, Maryam BM, Mohammad A, Moein BM, Liliane D. Risk factors of young-onset colorectal cancer: analysis of a large population-based registry. Can J Gastroenterol Hepatol. (2022) 2022:3582443. doi: 10.1155/2022/3582443
146. Nagao-Kitamoto H, Kitamoto S, Kamada N. Inflammatory bowel disease and carcinogenesis. Cancer Metastasis Rev. (2022) 41:301–16. doi: 10.1007/s10555-022-10028-4
147. Fantini MC, Guadagni I. From inflammation to colitis-associated colorectal cancer in inflammatory bowel disease: Pathogenesis and impact of current therapies. Dig Liver Dis. (2021) 53:558–65. doi: 10.1016/j.dld.2021.01.012
148. Jess T, Loftus EJ, Velayos FS, Harmsen WS, Zinsmeister AR, Smyrk TC, et al. Incidence and prognosis of colorectal dysplasia in inflammatory bowel disease: a population-based study from Olmsted County, Minnesota. Inflammation Bowel Dis. (2006) 12:669–76. doi: 10.1097/00054725-200608000-00001
149. Laskar RS, Qu C, Huyghe JR, Harrison T, Hayes RB, Cao Y, et al. Genome-wide association study and Mendelian randomization analyses provide insights into the causes of early-onset colorectal cancer. Ann Oncol. (2024) S0923-7534(24)00058-9. doi: 10.1016/j.annonc.2024.02.008
150. Al MH, Monahan KJ. The diagnostic yield of colonoscopic surveillance following resection of early age onset colorectal cancer. United Eur Gastroenterol J. (2024). doi: 10.1002/ueg2.12516
151. Dai R, Kelly BN, Ike A, Berger D, Chan A, Drew DA, et al. The impact of the gut microbiome, environment, and diet in early-onset colorectal cancer development. Cancers (Basel). (2024) 16:676. doi: 10.3390/cancers16030676
Keywords: obesity, EOCRC, metabolic syndrome, intestinal microbe, inflammation
Citation: Xu P, Tao Z, Yang H and Zhang C (2024) Obesity and early-onset colorectal cancer risk: emerging clinical evidence and biological mechanisms. Front. Oncol. 14:1366544. doi: 10.3389/fonc.2024.1366544
Received: 06 January 2024; Accepted: 22 April 2024;
Published: 02 May 2024.
Edited by:
Philip Rosenberg, National Cancer Institute (NIH), United StatesReviewed by:
Neha Nanda, Harvard Medical School, United StatesGuilherme Zweig Rocha, State University of Campinas, Brazil
Copyright © 2024 Xu, Tao, Yang and Zhang. This is an open-access article distributed under the terms of the Creative Commons Attribution License (CC BY). The use, distribution or reproduction in other forums is permitted, provided the original author(s) and the copyright owner(s) are credited and that the original publication in this journal is cited, in accordance with accepted academic practice. No use, distribution or reproduction is permitted which does not comply with these terms.
*Correspondence: Cheng Zhang, emhhbmdjMTEwOUAxNjMuY29t