- 1Department of Urology, Emory University, Atlanta, GA, United States
- 2Department of Urology, Northwestern University, Chicago, IL, United States
- 3Ferring Pharmaceuticals, International PharmaScience Center, Copenhagen, Denmark
- 4Carolina Urologic Research Center, Myrtle Beach, SC, United States
- 5Department of Urology, Tufts University School of Medicine, Boston, MA, United States
- 6Allina Health Cancer Institute, Minneapolis, MN, United States
Effective bladder-preserving therapeutic options are needed for patients with bacillus Calmette-Guérin unresponsive non–muscle-invasive bladder cancer. Nadofaragene firadenovec-vncg (Adstiladrin®) was approved by the US Food and Drug Administration as the first gene therapy in urology and the first intravesical gene therapy indicated for the treatment of adult patients with high-risk bacillus Calmette-Guérin–unresponsive non–muscle-invasive bladder cancer with carcinoma in situ with or without papillary tumors. The proposed mechanism of action underlying nadofaragene firadenovec efficacy is likely due to the pleiotropic nature of interferon-α and its direct and indirect antitumor activities. Direct activities include cell death and the mediation of an antiangiogenic effect, and indirect activities are those initiated through immunomodulation of the innate and adaptive immune responses. The sustained expression of interferon-α that results from this treatment modality contributes to a durable response. This review provides insight into potential mechanisms of action underlying nadofaragene firadenovec efficacy.
1 Introduction
Bladder cancer (BCa) is the tenth most commonly diagnosed cancer globally, with an estimated 573,278 new cases in 2020 (1). Of all BCa cases, approximately 75% are non–muscle-invasive bladder cancer (NMIBC) confined to the mucosa (stage Ta, carcinoma in situ [CIS]) or submucosa (stage T1) (2). High-grade (HG) tumors, accounting for approximately 31% of NMIBC cases (3), exhibit poorly differentiated cells and are more likely to progress to muscle-invasive disease (4). Guidelines by the American Urological Association, Society of Urologic Oncology, European Association of Urology, and National Comprehensive Cancer Network generally define high-risk NMIBC as CIS or HG Ta or T1 pathology (Ta ≤3 cm is considered intermediate risk) (5, 6). Individuals recently diagnosed with high-risk NMIBC have a 60–70% likelihood of recurrence and a 10–45% risk of disease progression to muscle-invasive or metastatic BCa within 5 years (6).
Although the growth of BCa is largely determined by the inherent proliferative capacity of urothelial carcinoma cells, recent advances in tumor immunology have revealed the importance of tumor-mediated immune surveillance subversion in promoting BCa growth (7, 8). Specifically, BCa has been shown to upregulate and secrete anti-apoptotic factors and immunosuppressive cytokines, such as transforming growth factor-ß, interleukin-6 (IL-6) and IL-10, to lose expression of immunogenic tumor antigens and to downregulate cell-surface major histocompatibility complex class I (MHC-I) and co-stimulatory molecules required to initiate T cell−mediated antitumor immune responses (7, 9). The cumulative effects of these immunosuppressive phenomena, coupled with the fact that the BCa microenvironment is tolerogenic and immune privileged due to the accumulation of several types of immune cells with immunosuppressive phenotypes (e.g., myeloid-derived suppressor cells, programmed death-ligand 1 (PD-L1)−expressing tolerogenic dendritic cells (DCs), and tumor-associated macrophages), result in BCa tissue being poorly immunogenic (7). By bolstering the innate and adaptive immune responses against BCa cells through vaccination with BCa-associated antigen or by administering checkpoint inhibitors of PD-L1/programmed cell death protein 1, immunotherapy attempts to induce a potent immune response to promote BCa regression. Although this goal appears straightforward, effective immunotherapy remains elusive due to problems such as T cell tolerance to BCa (self) antigen, induction of a Th2−polarized immune response, BCa heterogeneity, and limited clinical responses to immune checkpoint inhibitors (7).
Bacillus Calmette-Guérin (BCG) is a nonspecific immunotherapy that received approval by the US Food and Drug Administration (FDA) in 1990 for the treatment of patients with NMIBC (5). In clinical trials, intravesical BCG demonstrated initial complete response (CR) rates between 55% and 65% for papillary tumors and 70% and 75% for CIS (10). However, approximately one third of patients with NMIBC will not respond to BCG, and 50% will experience recurrence or progression following an initial response to BCG (11). The FDA defines BCG-unresponsive NMIBC as (1) persistent or recurrent CIS alone or with recurrent Ta/T1 (noninvasive papillary disease/tumor invades the subepithelial connective tissue) disease within 12 months of completion of adequate BCG therapy (defined as ≥5 of 6 doses of initial induction course plus either 2 of 3 doses of maintenance or ≥2 of 6 doses of a second induction course); (2) recurrent HG Ta/T1 disease within 6 months of completion of adequate BCG therapy; or (3) T1 HG disease at the first evaluation following an induction course of BCG (12). Regardless, a persistent global BCG shortage restricts access to BCG to such an extent that American Urological Association, Society of Urologic Oncology, European Association of Urology, and National Comprehensive Cancer Network guidelines have advised that BCG should be reserved for high-risk patients only (13).
The therapeutic effect of BCG is associated with the induction of Th1 immune responses. Attachment of BCG to urothelial cells triggers the cellular release of cytokines and chemokines, including IL-1, IL-6, IL-8, and tumor necrosis factor-α, which leads to the recruitment of immune cells into the urothelium. The activation of professional antigen-presenting cells, including macrophages, neutrophils, and DCs, and changes in the cytokine environment stimulate the differentiation of naïve cluster of differentiation 4+ (CD4+) T cells into Th1 and/or Th2 cells, which play respective roles in cellular and humoral immunity. Th1 cytokines, including interferon-γ (IFNγ), are associated with BCG response, and Th2 cytokines, including IL-10, are associated with BCG failure. Blocking IL-10 or inducing IFNγ can lead to a Th1-dominated immunity that is essential for BCG-mediated BCa regression (14).
While BCG-stimulated activation of the Th1 immune response demonstrates efficacy in overcoming BCa-induced immunosuppression, the development of new treatment modalities with greater immunomodulatory activity are required to improve patient outcomes (15). BCG-unresponsive NMIBC is associated with poor prognosis and historically few treatment options (6, 16). Radical cystectomy has remained the standard of care for patients with BCG-unresponsive disease and is a preferred option for high-risk disease in multiple clinical practice guidelines but is not appropriate for all patients (6, 17). For patients with high-risk, BCG-unresponsive NMIBC who are ineligible for or who choose not to undergo radical cystectomy, the therapeutic landscape has recently expanded to include intravesical chemotherapy, pembrolizumab, and the novel intravesical gene therapy, nadofaragene firadenovec-vncg (Adstiladrin®) (17). These treatments have all shown benefit in patients with BCG-unresponsive disease (18–20) but have notable differences in administration schedules and adverse event profiles owing to different mechanisms of action that elicit varied physiological responses. Intravesical chemotherapy encompasses multiple treatment regimens and dosing schedules and is generally well tolerated; however, it may need to be administered as often as weekly during induction therapy (17, 21). A widely used intravesical chemotherapy currently is sequential gemcitabine and docetaxel. The use of this regimen is only supported by retrospective data at present, with prospective validation still pending (18). Pembrolizumab is given via intravenous infusion every 3 to 6 weeks, and the adverse event profile includes mechanism-related immune-mediated adverse events (19, 22). Nadofaragene firadenovec is instilled intravesically on an every-3-month treatment schedule and has a well-tolerated safety profile, with micturition urgency being the most common grade 3/4 study-drug–related adverse event (20, 23). Other emerging intravesical therapies include immune adjuvants and alternative gene therapies for which the data are either emerging or pending larger phase 3 studies (16).
2 Gene therapy for bladder cancer
Cancer gene therapy is defined as the introduction of a therapeutic gene into a tumor cell utilizing a viral or nonviral vector. Viral vectors are widely used gene delivery vehicles in cancer therapies; adenoviruses are the preferred choice because they can express therapeutic genes episomally and have no risk of integrating into the genome (24). The deletion of E1 and E3 genes from the human serotype 5 adenovirus, a nonenveloped, icosahedral capsid, double-stranded DNA virus, prevents viral replication and creates space for transgenes, respectively (25). Human epithelial cells, including urothelial carcinoma cells, are particularly receptive to adenoviral infection due to the ubiquitous expression of the coxsackie/adenovirus receptor (26). Adenoviruses interact with the coxsackie/adenovirus receptor, leading to intracellular incorporation of the virus and subsequent expression of the transgene; once translated, the resultant protein remains detectable for up to 10 days after adenoviral infection (27, 28).
The urothelium of the bladder is a complex, multilayer surface that acts as a barrier to pathogens and urinary waste products. Efficient viral transduction of the urothelium requires a robust means to permeate the protective glycosoaminoglycan layer of the bladder mucosa. Investigation into the structure of Big CHAP (N,N’-Bis(3-D-gluconamidoproply)cholamide), a nonionic detergent used as a transduction-promoting agent in early intravesical adenoviral vector studies, led to the discovery of Syn3, a polyamide surfactant and synthetic excipient that promotes adenoviral transduction across the glycosoaminoglycan layer of the inner wall of the bladder (10, 29, 30). The simultaneous administration of adenovirus in a formulation containing Syn3 markedly increased adenoviral-mediated gene transfer and expression, not only in the normal urothelial cells but also in human superficial transitional cell carcinoma growing within the bladder of athymic nude mice (30). Syn3 is an important additive for adenoviral-mediated gene transfer for the treatment of NMIBC by intravesical administration (31).
Nadofaragene firadenovec-vncg received FDA approval as the first gene therapy in urology and the first intravesical gene therapy indicated for the treatment of adult patients with high-risk BCG-unresponsive NMIBC with CIS with or without papillary tumors (23). Nadofaragene firadenovec is a nonreplicating adenoviral vector−based gene therapy that delivers human IFNα2b complementary DNA to urothelial cells and Syn3 to enhance viral transduction of the urothelium. IFNα2b complementary DNA is transcribed into IFNα2b protein in bladder epithelial cells, where it exhibits direct and indirect immunomodulatory effects inhibiting tumor growth (28) (Figure 1).
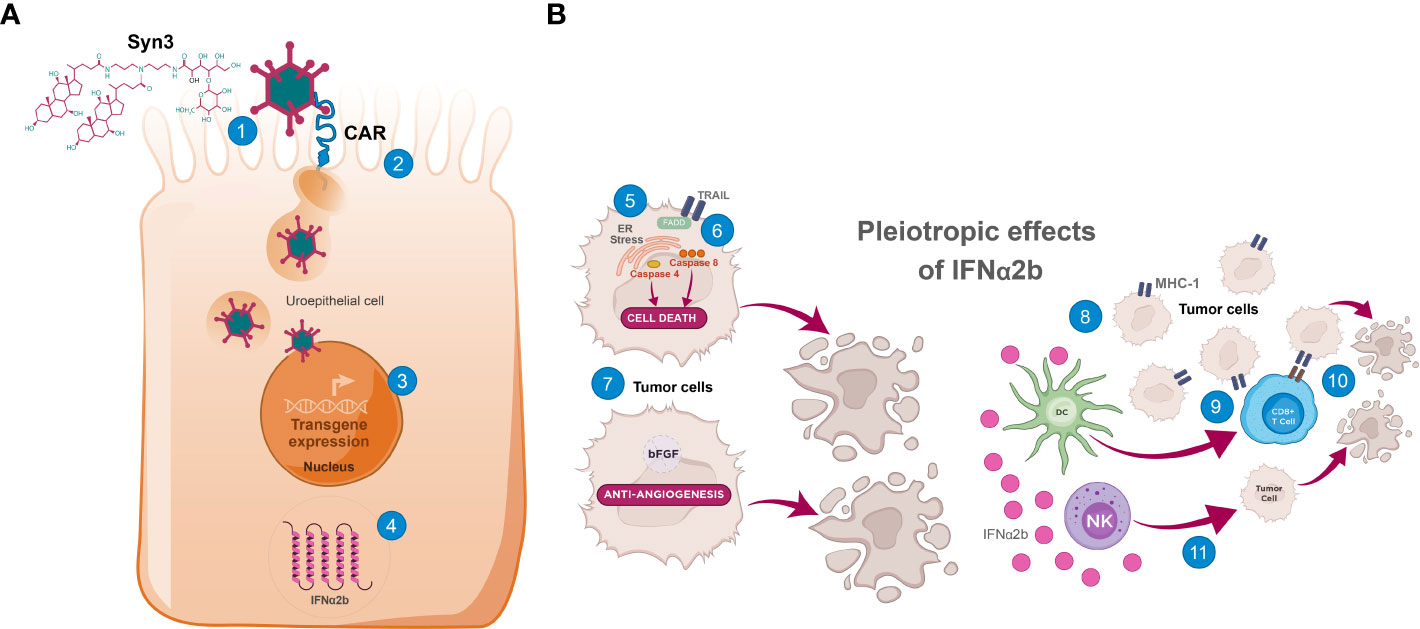
Figure 1 The mechanism of action of nadofaragene firadenovec. (A) Internalization of adenoviral vector into uroepithelial cells and transcription/translation of IFNα2b. (1) Syn3 promotes adenoviral vector access to uroepithelial cells (29); (2) After CAR-mediated endocytosis into the uroepithelial cell and escape from endosomes, the adenoviral capsid translocates to the nuclear envelope where it disassembles, and the human IFNα2b transgene is imported into the nucleus (26); (3) After import into the nucleus, IFNα2b cDNA is transcribed into mRNA; (4) IFNα2b mRNA is translated, leading to sustained production of IFNα2b (27, 28, 30). (B) Summary of putative direct and indirect effects of IFNα2b. IFNα2b exerts pleiotropic antitumor effects directly and indirectly in the tumor microenvironment (32). Direct cytotoxic effects include (5) induced ER stress in bladder cancer cells, leading to caspase 4 activation and cell death (33), (6) increased expression of TRAIL, leading to caspase 8 activation and cell death (34), and (7) antiangiogenic effects through downregulation of growth factors including bFGF (35, 36), leading to tumor hypoxia and central necrosis. Direct immunomodulatory effects are elicited through (8) upregulation of the presentation of surface tumor-associated antigens via augmentation of MHC-I class molecules, increasing the immunogenicity of tumor cells (37). Indirect immunomodulatory effects occur through (9) stimulation of DC-priming of cytotoxic CD8+ T cells, which (10) kill MHC-I+ tumors, and (11) increased antitumor activity of NK cells, which preferentially kill BCa cells lacking MHC-I (downregulation or loss of MHC-I is common in BCa cells) (32). BCa, bladder cancer; bFGF, basic fibroblast growth factor; CAR, coxsackievirus and adenovirus receptor; CD, cluster of differentiation; cDNA, complementary deoxyribonucleic acid; DC, dendritic cell; ER, endoplasmic reticulum; FADD, Fas-associated protein with death domain; IFNα2b, interferon α2b; MHC-I, major histocompatibility complex class I; mRNA, messenger ribonucleic acid; NK, natural killer; TRAIL, tumor necrosis factor−related apoptosis-inducing ligand.
IFNα has been used as a monotherapy and in combination with other agents, including BCG (38–40). Phase 2 studies of intravesical IFNα2b monotherapy at doses of 50–100 MIU have shown CRs, albeit of short duration, in up to 40% of patients with NMIBC, with most patients relapsing within 1 year (41). Limitations observed with intravesical IFNα2b therapy are likely due to a short drug exposure time rather than a lack of inherent antitumor activity (42). Local gene delivery with nadofaragene firadenovec can maximize transgene expression in the urothelium with prolonged local exposure of tissues to IFNα while minimizing systemic adenovirus distribution. In an orthotopic model of human BCa in nude mice to test the delivery and efficacy of nadofaragene firadenovec, high urinary IFN levels and marked tumor regression were observed following treatment (30). Adenoviral interferon-alpha 2b (AdIFNα) treatment also had cytotoxic effects on cells that were previously shown to be resistant to recombinant human IFNα (rhIFNα), which was attributed to a strong bystander effect in neighboring cells that potentially targeted tumor cells that were not effectively transduced during their initial exposure. Elevated bladder tissue IFNα levels were observed for ≥7 days following nadofaragene firadenovec therapy compared with rhIFNα levels and declined rapidly after treatment (30). This important discovery emphasized the benefit of gene therapy in overcoming the issue of durability with intravesically administered rhIFNα.
In a multicenter phase 2 trial, 40 patients with HG BCG-refractory or relapsed NMIBC were randomly assigned to receive either low- or high-dose intravesical nadofaragene firadenovec; patients who responded at 3, 6, and 9 months were re-treated at 4, 7, and 10 months (43). The primary endpoint was freedom from HG disease recurrence at 12 months (defined by a negative result on for-cause or end-of-study biopsy), which was found to be similar between the two dose groups, with 7 patients (33%) and 7 patients (37%) achieving 12-month recurrence-free survival of HG disease in the low- and high-dose groups, respectively. In subgroup analyses, 50% of patients with papillary disease and 30% of patients with CIS had 12-month recurrence-free survival. The most common adverse (AEs) events were lower urinary tract symptoms; no grade 4 or 5 AEs occurred and no patient discontinued therapy due to an AE. The higher dose was selected for use in the subsequent multicenter phase 3 trial (20). A total of 53.4% of patients with CIS with or without HG Ta/T1 had a CR at the 3-month assessment visit; this response was maintained through 12 months in 45.5% of these patients. In the HG Ta/T1 cohort, 72.9% of patients had a CR at 3 months, with 43.8% remaining recurrence-free through 12 months. The most frequently reported drug-related AEs were discharge around the catheter during instillation, fatigue, bladder spasms, and micturition urgency. Most AEs were transient and classified as either grade 1 or 2. There was a low discontinuation rate (1.9%), and no patient died from treatment-related AEs during the 12-month follow-up period (20). A major strength of the study was its mandatory end-of-study biopsy at 12 months, which provided objective pathologic disease assessment as opposed to visual assessments alone. Additionally, in the CIS cohort, patients who had a CR had a significantly longer median time to cystectomy of 11.4 months, compared with only 6.4 months among those who did not.
Because the human population is largely seropositive for anti-adenovirus antibodies, a planned secondary analysis of the phase 3 data investigated whether anti-adenovirus antibody levels predicted the durability of response to nadofaragene firadenovec (44). Baseline titer levels did not predict treatment response, suggesting that pre-exposure to circulating adenovirus did not adversely affect the efficacy of nadofaragene firadenovec. A 3-month adenovirus titer level of >800 was associated with a higher likelihood of durable response, and peak posttreatment titer levels of >800 were noted in 89% of responders versus 59% of non-responders. Although reasons for an increase in anti-adenovirus antibody titer levels observed in durable responders remain to be fully elucidated (44, 45), serum anti-adenovirus antibodies may serve as a predictive marker for nadofaragene firadenovec response durability.
For patients with BCG-unresponsive NMIBC who prefer a bladder-preserving treatment approach, nadofaragene firadenovec offers a compelling novel treatment option. After its FDA approval in December 2022, nadofaragene firadeneovec was initially available to a limited number of clinical study sites and community clinics due to supply constraints. The manufacturing processes were subsequently optimized and as of January 2024, the product has become fully available across the United States for healthcare providers to prescribe for appropriate patients (46). As of February 2024, completion of construction is near final for two new, state-of-the-art facilities that will be dedicated to manufacturing of nadofaragene firadenovec for future long-term supply.
3 Mechanism of action of nadofaragene firadenovec
The antitumor efficacy of AdIFNα results from the direct and indirect pleiotropic antitumor effects of IFNα. All cell types, including DCs, produce IFNα in response to the presence of cancer cells or other immune stimuli. Interferons modulate direct and indirect antitumor activities, typically through their canonical signaling via the Janus kinase (JAK)/signal transducer and activator of transcription (STAT) pathway. IFNα, part of the IFN-I family, signals through the IFNα receptor (IFNAR). IFN-I binds a heterodimeric receptor formed by IFNAR1 and IFNAR2 chains, causing their respective, constitutively associated JAKs, TYK2 and JAK1, to activate and phosphorylate STAT1 and STAT2. Phosphorylated (p)-STAT1 and p-STAT2 bind to IFN regulatory factor 9 and form a transcriptional complex named IFN‐stimulated gene factor 3, which is recruited to the IFN-stimulated response elements and regulates the transcription of downstream IFN‐stimulated genes. Key IFN‐stimulated genes include IFNα-inducible protein 27, PD-L1, and tumor necrosis factor–related apoptosis-inducing ligand (TRAIL) (32, 47, 48).
IFNα exhibits direct cytotoxic effects through endoplasmic reticulum stress, caspase 4 activation (33), and induction of TRAIL expression (34, 49). In BCa cells, rhIFNα-induced TRAIL expression resulted in cell death via an IFN regulatory factor-1−dependent mechanism (34), which may be a key cell death pathway underlying direct IFNα activity, because elevated TRAIL levels have been found in patients with detectable urinary IFNα following transduction (50). IFNα also mediates an antiangiogenic effect (35, 36, 51); preclinical studies have shown that systemic administration of rhIFNα to bladder tumor−bearing mice was associated with decreased angiogenic factors, including basic fibroblast growth factor (35). In addition, IFNα can directly upregulate the presentation of surface tumor−associated antigens via augmentation of MHC-I molecules, increasing the immunogenicity of tumor cells and making them more vulnerable to identification and subsequent destruction by cytotoxic CD8+ T cells (37). Increased tumorigenicity is especially important in BCa cells, which are renowned for immune evasion (7).
The indirect effects of IFNα, which include tumor microenvironment immunomodulation through the enhanced proliferation, maturation, and antigen presentation of immune cells such as DCs, macrophages, and natural killer (NK) cells, strengthen innate and adaptive antitumor immunity. IFNα is involved in antigen recognition and processing, leading to CD8+ T cell, NK cell, and DC activation. IFNα further promotes the adaptive antitumor response by stimulating the DC-priming of CD8+ T cells. In addition, stimulation of the adaptive immune response by type I IFNs is expected to complement immune checkpoint blockade. Together, the direct and indirect IFN-α2b effects can lead to BCa cell lysis and the release of BCa-associated antigen, further enhancing BCa immunogenicity and subsequent tumor regression (32, 48).
IFNα also augments the antitumor activity of NK cells, which preferentially kill MHC-I−deficient cells. Activated NK cells recognize and directly attack cancer cells by releasing cytotoxic granules containing perforin and granzymes. These substances create pores in the cancer cell membrane and induce apoptosis. Because downregulation or total loss of MHC-I expression is common in BCa cells (52), NK cells likely play a significant role in the antitumor immune activity of IFNα in BCa (32).
Taken together, these studies postulate that MHC-I−deficient BCa cells transduced with nadofaragene firadenovec produce IFNα2b, which may modulate NK cell responses by upregulating stress-induced ligands for activating NK cell receptors and promoting NK cell priming by DCs. Concurrently, MHC-I−expressing BCa cells transduced by nadofaragene firadenovec produce IFNα2b which may augment anti-BCa CD8+ T cell responses by upregulating MHC-I, promoting antigen presentation, and activating signaling pathways that augment T cell proliferation and cytotoxicity (32).
4 Conclusions
Nadofaragene firadenovec is the first FDA-approved gene therapy for high-risk BCG-unresponsive NMIBC with CIS with or without papillary tumors. The mechanism of action of nadofaragene firadenovec is hypothesized to result via direct and indirect IFNα2b activity. IFNα induces cell type-specific direct biological responses, including apoptosis and angiogenesis inhibition, affecting tumor cell initiation and progression. Indirect activity occurs through immunoregulation, by stimulating immune cells, including NK and T cells, and increasing antigen presentation by macrophages and DCs, which augments a more robust immune response.
Author contributions
VN: Conceptualization, Funding acquisition, Project administration, Supervision, Writing – original draft, Writing – review & editing. JM: Conceptualization, Writing – original draft, Writing – review & editing. JJ: Conceptualization, Writing – original draft, Writing – review & editing. NS: Conceptualization, Writing – original draft, Writing – review & editing. GS: Conceptualization, Writing – original draft, Writing – review & editing. BK: Conceptualization, Funding acquisition, Project administration, Supervision, Writing – original draft, Writing – review & editing.
Funding
The author(s) declare that financial support was received for the research, authorship, and/or publication of this article. This work was supported by Ferring Pharmaceuticals Inc.
Acknowledgments
Medical writing support, under the guidance of the authors, was provided by Paul Sobol, PhD, and Emma Bone, PhD (ApotheCom, Yardley, PA, USA), and was funded by Ferring Pharmaceuticals, Parsippany, NJ, USA.
Conflict of interest
Author JJ was employed by the company Ferring Pharmaceuticals Inc. VN: Served as a consultant and received funding from Ferring Pharmaceuticals Inc. and Ciox Health. JM: Served as a consultant for Merck, AstraZeneca, Incyte, Janssen, BMS, UroGen, Prokarium, Imvax, Pfizer, and Seagen/Astellas, Ferring; research funding from VHA, NIH, and DoD; compensation for talks/educational courses from AUA, OncLive, Olympus, and UroToday; clinical trials at SWOG, Genentech, Merck, AstraZeneca, and Incyte; and two patents: T1 and TCGA classifier. JJ: Employee of Ferring Pharmaceuticals Inc. NS: Served as a consultant received funding from Astellas, Dendreon, Janssen, Bayer, Myriad, MDxHealth, Tolmar, Myovant, Pfizer, EMD Serono Inc, AstraZeneca, Merck, Urogen, Guardant, Abbvie, Amgen, Bristol Myers Squibb, Boston Scientific, Exact Imaging, Foundation Medicine, CG Oncology, Invitae, Propella, Sanofi, Pacific Edge, Alessa, Amgen, Arquer, Asieris, Clarity, Ferring Pharmaceuticals Inc., Lantheus, Lilly, Minomic, Nanogen, Novartis, Photocure, PlatformQ, Profound, Promaxo, Protara, Speciality Networks, Telix, FIZE Medical, Accord, Antev, BioProtect, Aura Biosciences, Palette Life, and Preview. NS received funding from a leadership role in GenesisCare, Alessa, and Photocure. GS: Consultant to Ferring Pharmaceuticals Inc. BK: Served as a consultant/advisor and received funding from Photocure, Asieris Pharmaceuticals, Ferring Pharmaceuticals Inc., Illumina, Inc., and Abbott; and has ownership/investment interest in Astrin Biosciences and Styx Biotechnologies.
The authors declare that this study received funding from Ferring Pharmaceuticals Inc. The funder had involvement in the development, authorship, and publication of this manuscript.
The author(s) declared that they were an editorial board member of Frontiers, at the time of submission. This had no impact on the peer review process and the final decision.
Publisher’s note
All claims expressed in this article are solely those of the authors and do not necessarily represent those of their affiliated organizations, or those of the publisher, the editors and the reviewers. Any product that may be evaluated in this article, or claim that may be made by its manufacturer, is not guaranteed or endorsed by the publisher.
References
1. Sung H, Ferlay J, Siegel RL, Laversanne M, Soerjomataram I, Jemal A, et al. Global cancer statistics 2020: GLOBOCAN estimates of incidence and mortality worldwide for 36 cancers in 185 countries. CA Cancer J Clin. (2021) 71:209–49. doi: 10.3322/caac.21660
2. Babjuk M, Burger M, Capoun O, Cohen D, Compérat EM, Dominguez Escrig JL, et al. European association of urology guidelines on non-muscle-invasive bladder cancer (Ta, T1, and carcinoma in situ). Eur Urol. (2022) 81:75–94. doi: 10.1016/j.eururo.2021.08.010
3. Sylvester RJ, Rodríguez O, Hernández V, Turturica D, Bauerová L, Bruins HM, et al. European association of urology (EAU) prognostic factor risk groups for non-muscle-invasive bladder cancer (NMIBC) incorporating the WHO 2004/2016 and WHO 1973 classification systems for grade: an update from the EAU NMIBC guidelines panel. Eur Urol. (2021) 79:480–8. doi: 10.1016/j.eururo.2020.12.033
4. Mayo Clinic. Bladder cancer. Rochester, NY: Mayo Clinic (2023). Available at: https://www.mayoclinic.org/diseases-conditions/bladder-cancer/diagnosis-treatment/drc-20356109.
5. Packiam VT, Johnson SC, Steinberg GD. Non-muscle-invasive bladder cancer: Intravesical treatments beyond Bacille Calmette-Guérin. Cancer. (2017) 123:390–400. doi: 10.1002/cncr.30392
6. Chang SS, Boorjian SA, Chou R, Clark PE, Daneshmand S, Konety BR, et al. Diagnosis and treatment of non-muscle invasive bladder cancer: AUA/SUO guideline. J Urol. (2016) 196:1021–9. doi: 10.1016/j.juro.2016.06.049
7. Crispen PL, Kusmartsev S. Mechanisms of immune evasion in bladder cancer. Cancer Immunol Immunother. (2020) 69:3–14. doi: 10.1007/s00262-019-02443-4
8. Joseph M, Enting D. Immune responses in bladder cancer-role of immune cell populations, prognostic factors and therapeutic implications. Front Oncol. (2019) 9:1270. doi: 10.3389/fonc.2019.01270
9. Gil-Julio H, Perea F, Rodriguez-Nicolas A, Cozar JM, González-Ramirez AR, Concha A, et al. Tumor escape phenotype in bladder cancer is associated with loss of HLA Class I expression, T-cell exclusion and stromal changes. Int J Mol Sci. (2021) 22:7248. doi: 10.3390/ijms22147248
10. Narayan VM, Dinney CPN. Intravesical gene therapy. Urol Clin North Am. (2020) 47:93–101. doi: 10.1016/j.ucl.2019.09.011
11. Lebacle C, Loriot Y, Irani J. BCG-unresponsive high-grade non-muscle invasive bladder cancer: what does the practicing urologist need to know? World J Urol. (2021) 39:4037–46. doi: 10.1007/s00345-021-03666-w
12. US Department of Health and Human Services. BCG-Unresponsive nonmuscle invasive bladder cancer: developing drugs and biologics for treatment: guidance for industry. Rockville, MD: US Department of Health and Human Services (2018). Available at: https://www.fda.gov/media/101468/download.
13. Shore ND, Palou Redorta J, Robert G, Hutson TE, Cesari R, Hariharan S, et al. Non-muscle-invasive bladder cancer: An overview of potential new treatment options. Urol Oncol. (2021) 39:642–63. doi: 10.1016/j.urolonc.2021.05.015
14. Askeland EJ, Newton MR, O'Donnell MA, Luo Y. Bladder cancer immunotherapy: BCG and beyond. Adv Urol. (2012) 2012:181987. doi: 10.1155/2012/181987
15. Maruf M, Brancato SJ, Agarwal PK. Nonmuscle invasive bladder cancer: a primer on immunotherapy. Cancer Biol Med. (2016) 13:194–205. doi: 10.20892/j.issn.2095-3941.2016.0020
16. Al Hussein Al Awamlh B, Chang SS. Novel therapies for high-risk non-muscle invasive bladder cancer. Curr Oncol Rep. (2023) 25:83–91. doi: 10.1007/s11912-022-01350-9
17. National Comprehensive Cancer Network. NCCN Clinical Practice Guidelines in Oncology. Bladder Cancer. Version 3.2023. Plymouth Meeting, PA: National Comprehensive Cancer Network (2023). Available at: https://www.nccn.org/professionals/physician_gls/pdf/bladder.pdf.
18. Yim K, Melnick K, Mott SL, Carvalho FLF, Zafar A, Clinton TN, et al. Sequential intravesical gemcitabine/docetaxel provides a durable remission in recurrent high-risk NMIBC following BCG therapy. Urol Oncol. (2023) 41:458.e1–7. doi: 10.1016/j.urolonc.2023.06.018
19. Balar AV, Kamat AM, Kulkarni GS, Uchio EM, Boormans JL, Roumiguié M, et al. Pembrolizumab monotherapy for the treatment of high-risk non-muscle-invasive bladder cancer unresponsive to BCG (KEYNOTE-057): an open-label, single-arm, multicentre, phase 2 study. Lancet Oncol. (2021) 22:919–30. doi: 10.1016/s1470-2045(21)00147-9
20. Boorjian SA, Alemozaffar M, Konety BR, Shore ND, Gomella LG, Kamat AM, et al. Intravesical nadofaragene firadenovec gene therapy for BCG-unresponsive non-muscle-invasive bladder cancer: a single-arm, open-label, repeat-dose clinical trial. Lancet Oncol. (2021) 22:107–17. doi: 10.1016/s1470-2045(20)30540-4
21. Prasanna T, Craft P, Balasingam G, Haxhimolla H, Pranavan G. Intravesical gemcitabine versus intravesical bacillus calmette-guerin for the treatment of non-muscle invasive bladder cancer: an evaluation of efficacy and toxicity. Front Oncol. (2017) 7:260. doi: 10.3389/fonc.2017.00260
22. KEYTRUDA 50 mg powder for concentrate for solution for infusion. Harlem, Netherlands: Merck Sharp and Dohme BV (2020).
23. ADSTILADRIN® (nadofaragene firadenovec-vncg) suspension, for intravesical use Vol. 15. Kastrup, Denmark: Ferring Pharmaceuticals (2022).
24. Akbulut H. Immune gene therapy of cancer. Turk J Med Sci. (2020) 50:1679–90. doi: 10.3906/sag-2005-327
25. Crystal RG. Adenovirus: the first effective in vivo gene delivery vector. Hum Gene Ther. (2014) 25:3–11. doi: 10.1089/hum.2013.2527
26. Martini A, Tholomier C, Mokkapati S, Dinney CPN. Interferon gene therapy with nadofaragene firadenovec for bladder cancer: from bench to approval. Front Immunol. (2023) 14:1260498. doi: 10.3389/fimmu.2023.1260498
27. Connor RJ, Anderson JM, Machemer T, Maneval DC, Engler H. Sustained intravesical interferon protein exposure is achieved using an adenoviral-mediated gene delivery system: a study in rats evaluating dosing regimens. Urology. (2005) 66:224–9. doi: 10.1016/j.urology.2005.02.015
28. Dinney CP, Fisher MB, Navai N, O'Donnell MA, Cutler D, Abraham A, et al. Phase I trial of intravesical recombinant adenovirus mediated interferon-α2b formulated in Syn3 for Bacillus Calmette-Guérin failures in nonmuscle invasive bladder cancer. J Urol. (2013) 190:850–6. doi: 10.1016/j.juro.2013.03.030
29. Yamashita M, Rosser CJ, Zhou JH, Zhang XQ, Connor RJ, Engler H, et al. Syn3 provides high levels of intravesical adenoviral-mediated gene transfer for gene therapy of genetically altered urothelium and superficial bladder cancer. Cancer Gene Ther. (2002) 9:687–91. doi: 10.1038/sj.cgt.7700488
30. Benedict WF, Tao Z, Kim CS, Zhang X, Zhou JH, Adam L, et al. Intravesical Ad-IFNalpha causes marked regression of human bladder cancer growing orthotopically in nude mice and overcomes resistance to IFN-alpha protein. Mol Ther. (2004) 10:525–32. doi: 10.1016/j.ymthe.2004.05.027
31. Tao Z, Connor RJ, Ashoori F, Dinney CP, Munsell M, Philopena JA, et al. Efficacy of a single intravesical treatment with Ad-IFN/Syn 3 is dependent on dose and urine IFN concentration obtained: implications for clinical investigation. Cancer Gene Ther. (2006) 13:125–30. doi: 10.1038/sj.cgt.7700865
32. Green JL, Osterhout RE, Klova AL, Merkwirth C, McDonnell SRP, Zavareh RB, et al. Molecular characterization of type I IFN-induced cytotoxicity in bladder cancer cells reveals biomarkers of resistance. Mol Ther Oncol. (2021) 23:547–59. doi: 10.1016/j.omto.2021.11.006
33. Yang Z, Zhang XQ, Dinney CN, Benedict WF. Direct cytotoxicity produced by adenoviral-mediated interferon α gene transfer in interferon-resistant cancer cells involves ER stress and caspase 4 activation. Cancer Gene Ther. (2011) 18:609–16. doi: 10.1038/cgt.2011.26
34. Papageorgiou A, Dinney CP, McConkey DJ. Interferon-alpha induces TRAIL expression and cell death via an IRF-1-dependent mechanism in human bladder cancer cells. Cancer Biol Ther. (2007) 6:872–9. doi: 10.4161/cbt.6.6.4088
35. Dinney CP, Bielenberg DR, Perrotte P, Reich R, Eve BY, Bucana CD, et al. Inhibition of basic fibroblast growth factor expression, angiogenesis, and growth of human bladder carcinoma in mice by systemic interferon-alpha administration. Cancer Res. (1998) 58:808–14.
36. Slaton JW, Perrotte P, Inoue K, Dinney CP, Fidler IJ. Interferon-alpha-mediated down-regulation of angiogenesis-related genes and therapy of bladder cancer are dependent on optimization of biological dose and schedule. Clin Cancer Res. (1999) 5:2726–34.
37. Medrano RFV, Hunger A, Mendonça SA, Barbuto JAM, Strauss BE. Immunomodulatory and antitumor effects of type I interferons and their application in cancer therapy. Oncotarget. (2017) 8:71249–84. doi: 10.18632/oncotarget.19531
38. Joudi FN, Smith BJ, O'Donnell MA. Final results from a national multicenter phase II trial of combination bacillus Calmette-Guérin plus interferon alpha-2B for reducing recurrence of superficial bladder cancer. Urol Oncol. (2006) 24:344–8. doi: 10.1016/j.urolonc.2005.11.026
39. Lamm D, Brausi M, O'Donnell MA, Witjes JA. Interferon alfa in the treatment paradigm for non-muscle-invasive bladder cancer. Urol Oncol. (2014) 32:35.e21–30. doi: 10.1016/j.urolonc.2013.02.010
40. O'Donnell MA, Krohn J, DeWolf WC. Salvage intravesical therapy with interferon-alpha 2b plus low dose bacillus Calmette-Guerin is effective in patients with superficial bladder cancer in whom bacillus Calmette-Guerin alone previously failed. J Urol. (2001) 166:1300–4, discussion 4-5.
41. Nagabhushan TL, Maneval DC, Benedict WF, Wen SF, Ihnat PM, Engler H, et al. Enhancement of intravesical delivery with Syn3 potentiates interferon-α2b gene therapy for superficial bladder cancer. Cytokine Growth Factor Rev. (2007) 18:389–94. doi: 10.1016/j.cytogfr.2007.06.007
42. Duplisea JJ, Mokkapati S, Plote D, Schluns KS, McConkey DJ, Yla-Herttuala S, et al. The development of interferon-based gene therapy for BCG unresponsive bladder cancer: from bench to bedside. World J Urol. (2019) 37:2041–9. doi: 10.1007/s00345-018-2553-7
43. Shore ND, Boorjian SA, Canter DJ, Ogan K, Karsh LI, Downs TM, et al. Intravesical rAd-IFNα/syn3 for patients with high-grade, Bacillus Calmette-Guerin-refractory or relapsed non-muscle-invasive bladder cancer: A phase II randomized study. J Clin Oncol. (2017) 35:3410–6. doi: 10.1200/jco.2017.72.3064
44. Mitra AP, Narayan VM, Mokkapati S, Miest T, Boorjian SA, Alemozaffar M, et al. Antiadenovirus antibodies predict response durability to nadofaragene firadenovec therapy in BCG-unresponsive non-muscle-invasive bladder cancer: Secondary analysis of a phase 3 clinical trial. Eur Urol. (2022) 81:223–8. doi: 10.1016/j.eururo.2021.12.009
45. Zaiss AK, Vilaysane A, Cotter MJ, Clark SA, Meijndert HC, Colarusso P, et al. Antiviral antibodies target adenovirus to phagolysosomes and amplify the innate immune response. J Immunol. (2009) 182:7058–68. doi: 10.4049/jimmunol.0804269
46. Ferring announces full availability of ADSTILADRIN® (nadofaragene firadenovec-vncg) in the U.S (2024). Available online at: https://www.ferring.com/ferring-announces-full-availability-of-adstiladrin-nadofaragene-firadenovec-vncg-in-the-u-s/ (Accessed January 30, 2024).
47. Martin-Hijano L, Sainz B Jr. The interactions between cancer stem cells and the innate interferon signaling pathway. Front Immunol. (2020) 11:526. doi: 10.3389/fimmu.2020.00526
48. Xiong F, Wang Q, Wu GH, Liu WZ, Wang B, Chen YJ. Direct and indirect effects of IFN-α2b in Malignancy treatment: not only an archer but also an arrow. biomark Res. (2022) 10:69. doi: 10.1186/s40364-022-00415-y
49. Papageorgiou A, Lashinger L, Millikan R, Grossman HB, Benedict W, Dinney CP, et al. Role of tumor necrosis factor-related apoptosis-inducing ligand in interferon-induced apoptosis in human bladder cancer cells. Cancer Res. (2004) 64:8973–9. doi: 10.1158/0008-5472.Can-04-1909
50. Benedict WF, Fisher M, Zhang XQ, Yang Z, Munsell MF, Dinney CN. Use of monitoring levels of soluble forms of cytokeratin 18 in the urine of patients with superficial bladder cancer following intravesical Ad-IFNα/Syn3 treatment in a phase l study. Cancer Gene Ther. (2014) 21:91–4. doi: 10.1038/cgt.2014.1
51. von Marschall Z, Scholz A, Cramer T, Schäfer G, Schirner M, Oberg K, et al. Effects of interferon alpha on vascular endothelial growth factor gene transcription and tumor angiogenesis. J Natl Cancer Inst. (2003) 95:437–48. doi: 10.1093/jnci/95.6.437
Keywords: adenoviral-mediated interferon delivery, Adstiladrin, BCG-unresponsive, nadofaragene firadenovec-vncg, non-muscle-invasive bladder cancer, Syn3
Citation: Narayan VM, Meeks JJ, Jakobsen JS, Shore ND, Sant GR and Konety BR (2024) Mechanism of action of nadofaragene firadenovec-vncg. Front. Oncol. 14:1359725. doi: 10.3389/fonc.2024.1359725
Received: 29 December 2023; Accepted: 14 February 2024;
Published: 15 March 2024.
Edited by:
Nancy B. Davis, Merck Sharp & Dohme Corp, United StatesReviewed by:
Vignesh Packiam, Rutgers, The State University of New Jersey, United StatesWilliam Tabayoyong, University of Rochester, United States
Copyright © 2024 Narayan, Meeks, Jakobsen, Shore, Sant and Konety. This is an open-access article distributed under the terms of the Creative Commons Attribution License (CC BY). The use, distribution or reproduction in other forums is permitted, provided the original author(s) and the copyright owner(s) are credited and that the original publication in this journal is cited, in accordance with accepted academic practice. No use, distribution or reproduction is permitted which does not comply with these terms.
*Correspondence: Badrinath R. Konety, QmFkcmluYXRoLktvbmV0eUBhbGxpbmEuY29t