- 1Medical Technology College of Qiqihar Medical College, Qiqihar, Heilongjiang, China
- 2School of Stomatology, Qiqihar Medical College, Qiqihar, Heilongjiang, China
- 3School of Basic Medical Sciences, Qiqihar Medical College, Qiqihar, Heilongjiang, China
The tumor microenvironment is a complex ecosystem where various cellular and molecular interactions shape the course of cancer progression. Macrophage colony-stimulating factor (M-CSF) plays a pivotal role in this context. This study delves into the biological properties and functions of M-CSF in regulating tumor-associated macrophages (TAMs) and its role in modulating host immune responses. Through the specific binding to its receptor colony-stimulating factor 1 receptor (CSF-1R), M-CSF orchestrates a cascade of downstream signaling pathways to modulate macrophage activation, polarization, and proliferation. Furthermore, M-CSF extends its influence to other immune cell populations, including dendritic cells. Notably, the heightened expression of M-CSF within the tumor microenvironment is often associated with dismal patient prognoses. Therefore, a comprehensive investigation into the roles of M-CSF in tumor growth advances our comprehension of tumor development mechanisms and unveils promising novel strategies and approaches for cancer treatment.
1 Introduction
Cancer has emerged as a highly fatal disease on a global scale, posing a significant threat to human health and life (1–3). As medical research advances, it has become increasingly evident that the immune system plays a pivotal regulatory role in the initiation and progression of cancer. Among the various immune cells in tumor tissues, macrophages constitute the most abundant population and exert multifaceted functions in tumor onset, development, metastasis, and therapeutic responses.
Macrophages primarily serve as integral components of the human immune system, with distinct subtypes performing diverse immunological functions. Notably, M1 macrophages are responsible for identifying and eradicating cancerous cells. Their direct anti-tumor actions involve phagocytosis and destruction of malignant cells. Additionally, M1 macrophages modulate immune responses by secreting pro-inflammatory cytokines and chemical factors and influencing the activities of other immune cells, thereby stimulating the host’s immune reactions against tumors. Macrophage colony-stimulating factors (M-CSFs) are crucial in this regulatory process. As a pivotal cytokine, M-CSF exerts a multifaceted influence on macrophage behavior, enhancing phagocytic and chemotactic activities, and augmenting cytotoxicity against tumor cells.
The tumor microenvironment represents a complex ecological system, comprising tumor cells, immune cells, extracellular matrix components, and a plethora of cytokines (4). Beyond their regulatory interactions with tumor-associated macrophages (TAMs), M-CSF engages in intricate cross-talk with various other cell types within the tumor microenvironment, impacting diverse physiological processes such as immunoregulation and metabolism, thereby forming a complex regulatory network (5, 6). This intricate regulation can profoundly influence tumor growth, invasion, metastasis, and immune evasion, underscoring the far-reaching implications of M-CSF in the context of cancer.
In clinical practice, an increasing body of research has demonstrated a strong correlation between the elevated expression of M-CSF and poor patient prognosis across various types of tumors (7, 8). This association underscores the potential of M-CSF as a promising target for novel therapeutic strategies in cancer management. For instance, interventions aimed at inhibiting M-CSF or their receptor, colony-stimulating factor 1 receptor (CSF-1R), hold the potential to modulate the tumor microenvironment, thereby impeding tumor progression and offering improved survival prospects for patients. In recent years, extensive research has underscored the pivotal role of M-CSF in tumor development. However, the precise regulation mechanisms and their potential as therapeutic and predictive indicators remain focal points of current research.
This study explored the role of M-CSF in tumor development. Initially, an analysis was conducted of the structural attributes, expression patterns, and regulatory mechanisms of M-CSF and their receptor CSF-1R, specifically emphasizing their interactions with host immune responses. Subsequently, the impact of M-CSF on existing clinical therapeutic approaches was investigated, while considering the modulation strategies involving other cytokines. Lastly, a perspective was provided on the future of M-CSF in cancer research, focusing on elucidating their interactions with TAMs and tumor cells, the development of novel diagnostic and therapeutic strategies, and the potential for personalized treatments.
2 Gene structure and biochemical characteristics of M-CSF
M-CSF, also known as CSF-1, is a cytokine that selectively stimulates the proliferation of hematopoietic progenitor cells, guiding their specific differentiation into the mononuclear phagocyte lineage (9). As a chemotactic factor, M-CSF plays a vital role in the survival, proliferation, and differentiation of mononuclear phagocytes within hematopoietic stem cells.
The gene encoding M-CSF is localized on the human chromosome 5. This gene undergoes transcription, resulting in multiple mRNA isoforms that can translate into various protein isoforms. The M-CSF protein is typically a glycoprotein modified with numerous N-glycan and glycosyl residues. These modifications are critically important for its stability and activity. Specifically, M-CSF exhibits three biologically active isoforms: secreted glycoprotein (80-100kDa), secreted protein-polysaccharide (130-160kDa), and cell surface glycoprotein spanning the cell membrane (68-86kDa). Structurally, the M-CSF protein comprises multiple functional domains, with the most crucial being the domain responsible for binding to its receptor CSF-1R (Figure 1).
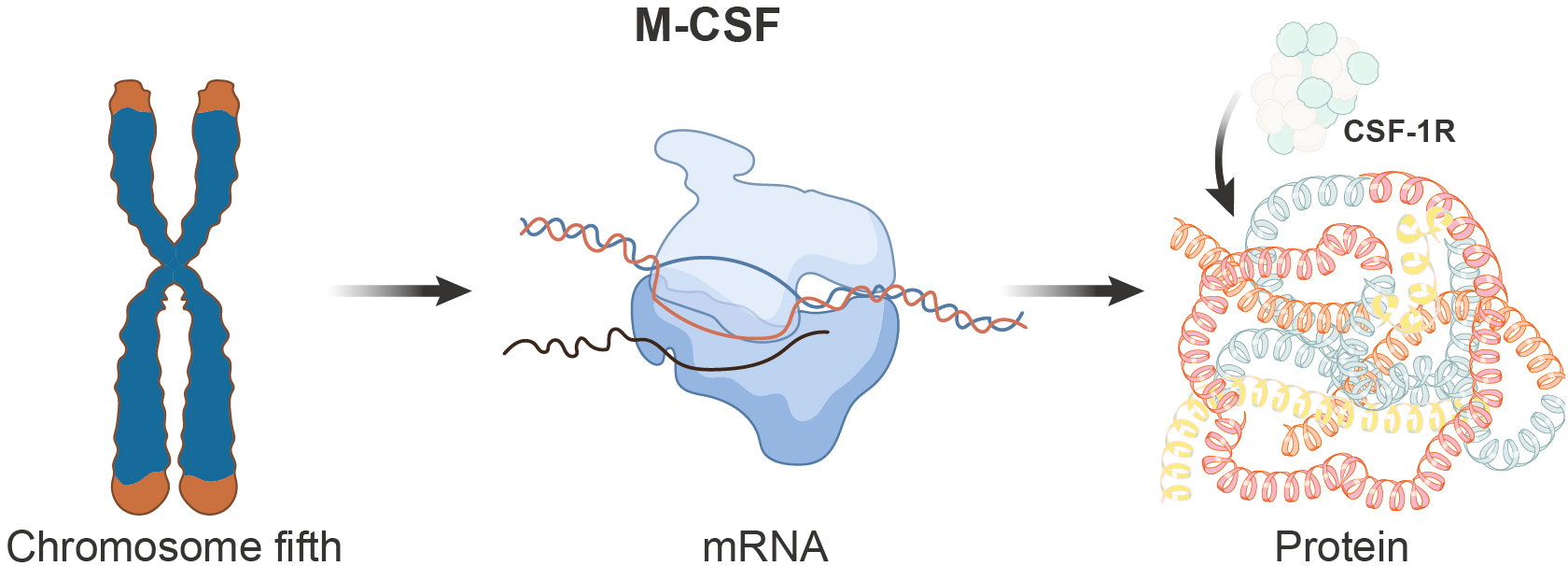
Figure 1 Schematic depicting the genomic, transcriptional, and translational aspects of the M-CSF gene. The M-CSF gene is located on chromosome 5 in humans and undergoes transcription to produce multiple mRNA isoforms, which subsequently translate into distinct protein isoforms. The M-CSF protein encompasses various functional domains, with the domain responsible for binding to its receptor, CSF-1R, being of paramount importance.
2.1 Biosynthesis and cellular origins of M-CSF
The biosynthesis of M-CSF commences with the transcription of its gene. Following translation, the initial M-CSF precursor protein enters the rough endoplasmic reticulum, where it undergoes initial folding and glycosylation modifications. Subsequently, M-CSF is transported to the Golgi apparatus, where final modifications and packaging occur. In the Golgi apparatus, M-CSF acquires its ultimate glycosylation form and is packaged into vesicles, which subsequently fuse with the cell membrane, leading to the secretion of M-CSF into the extracellular space. It is noteworthy that M-CSF can be produced by various cells, including monocytes, fibroblasts, osteoblasts, activated macrophages, neurons, epithelial cells, bone marrow stromal cells, activated endothelial cells, and tumor cells. This wide range of cellular origins enables M-CSF to exert its effects under different physiological and pathological conditions.
2.2 Mechanisms of M-CSF in regulating host immune responses
M-CSF, a crucial cytokine in the immune system, assumes a significant role in the regulation of host immune responses. It serves as the principal regulatory factor for the survival, proliferation, and differentiation of macrophages. M-CSF promotes the survival of monocytes, their differentiation into macrophages, and subsequent macrophage proliferation. Furthermore, it initiates and enhances macrophage-mediated cytotoxicity against tumor cells and microbes, regulates the release of cytokines and other inflammatory modulators by macrophages, and stimulates phagocytosis.
Macrophages, positioned at the forefront of the immune system, assume a central role in clearing both exogenous and endogenous pathogens. M-CSF modulates the survival, proliferation, differentiation, and functions of macrophages (10–12). Particularly during the early stages of the host’s immune response, the expression and secretion of M-CSF increase, facilitating the differentiation of hematopoietic progenitor cells in the bone marrow into macrophage lineages (13, 14). Additionally, M-CSF activates a series of signaling pathways by binding to its receptor, CSF-1R, to regulate various functions of macrophages (13, 15, 16). Notably, the influence of M-CSF on macrophage polarization is of particular significance, as it drives macrophages toward an M2 polarization state, characterized by anti-inflammatory and tissue repair functions (11, 17–19).
M-CSF exerts regulatory effects not only on innate immunity but also on adaptive immunity. In certain diseases such as cancer and autoimmune disorders, M-CSF modulates T cell activation and function through its regulation of macrophage functionality (14, 18, 20, 21). This regulation is partially mediated by M2-type macrophages induced by M-CSF, which can secrete anti-inflammatory factors like interleukin (IL)-10, thereby suppressing effector T cell activation and modulating immune responses (13, 22, 23). Research indicates that M-CSF also impacts the functionality of other immune cells, such as dendritic cells (18, 24). It has been implicated in dendritic cell differentiation and development, and it can also affect T-cell activation (18). Furthermore, M-CSF regulates the survival and proliferation of NK, T, and B cells and neutrophils, further expanding its role in immune responses (25, 26).
M-CSF not only assumes a crucial role in cancer but also exerts significant regulatory effects in non-neoplastic diseases. In autoimmune conditions like rheumatoid arthritis, M-CSF may be overexpressed, correlating with disease progression and exacerbation of pathology (27–29). Furthermore, due to its influence on adaptive immunity, M-CSF also plays a regulatory role in some immune-related disorders.
3 Regulatory role of M-CSF on TAMs
M-CSF plays a pivotal role in regulating macrophages within the immune system, exerting a critical influence on the roles of macrophages in diseases such as cancer. Macrophages can differentiate into two primary phenotypes: M1 and M2. M1 macrophages are associated with pro-inflammatory and anti-tumor functions, while M2 macrophages are typically linked to anti-inflammatory responses, tissue repair, and tumor promotion. Within the tumor microenvironment, macrophages can differentiate into TAMs, assuming multifaceted roles in tumor development, metastasis, and immune evasion. The influence of M-CSF on macrophage differentiation is particularly noteworthy. It tends to promote the differentiation of macrophages towards the M2 phenotype, which is associated with tumor-promoting properties (11). This M2 polarization, driven by M-CSF, supports tumor growth by suppressing anti-tumor immune responses and promoting tissue remodeling and angiogenesis, which are beneficial for tumor progression (30). The regulation of M-CSF and its impact on macrophage differentiation highlight the complex interplay between the immune system and cancer, underscoring the potential of M-CSF as a therapeutic target in cancer treatment (31).
3.1 Dual Role of TAMs in tumor microenvironment and their immune regulatory mechanisms
TAMs represent a prominent immune cell population within the tumor microenvironment, exerting pivotal roles in tumor development and metastasis (Figure 2) (17, 18, 32–36).
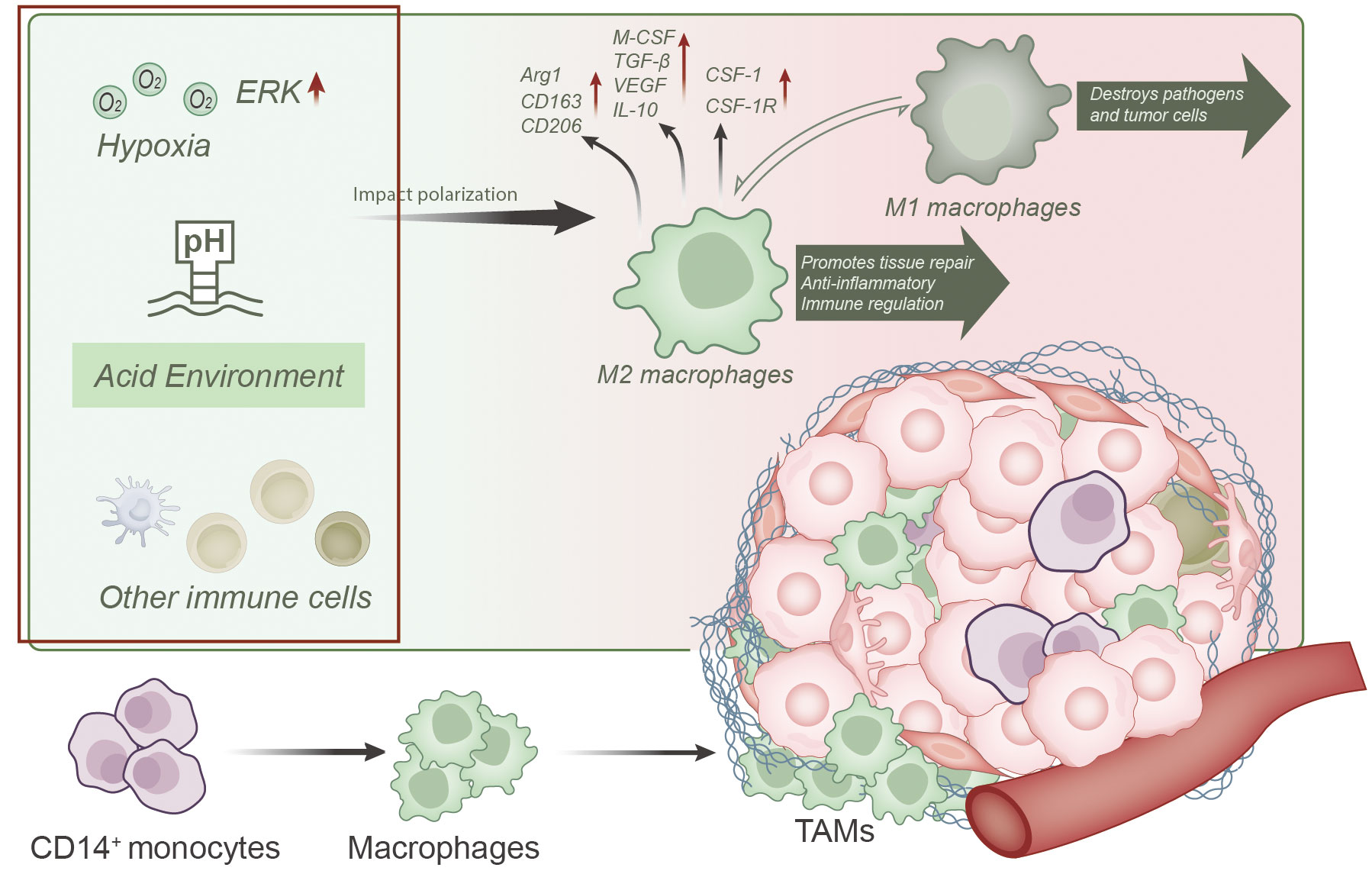
Figure 2 Overview of TAMs differentiation, function, and factors influencing their polarization within the tumor microenvironment. TAMs predominantly originate from CD14+ monocytes in the bone marrow and differentiate into macrophages within the tumor microenvironment. Various influencing factors, such as hypoxia, acidic conditions, and interactions with other immune cells, play a role in polarizing TAMs toward an M2-like phenotype. M2-like TAMs are characterized by elevated expression of markers like Arg1, CD163, and CD206, along with the production of immunosuppressive cytokines like M-CSF, TGF-β, VEGF, and IL-10. Overexpression of CSF-1 and CSF-1R on M2 macrophages is a key contributor to unfavorable tumor prognosis.
TAMs primarily originate from CD14+ monocytes from the bone marrow and differentiate into macrophages upon entering the tumor microenvironment (11, 13, 14, 16, 23), where they subsequently undergo local proliferation (13). TAMs dominate the tumor microenvironment (17, 18, 21, 34), particularly in the peritumoral regions, outnumbering intratumoral macrophages (23) and accounting for approximately 40% of total tumor cell population (35).
TAMs are typically categorized into two subtypes, known as the classically activated phenotype (M1 or M1-like phenotype) and alternatively activated phenotype (M2 or M2-like phenotype) (12, 14, 22, 23, 35, 37–39). Importantly, these M1 and M2 macrophages represent dynamically interconvertible subpopulations, and their phenotypes can reversibly switch (11, 12, 40). Consequently, TAMs exhibit a dualistic nature, capable of both inhibiting and promoting tumor development (11). In their tumor-suppressive role, TAMs engulf tumor cells and apoptotic cells while producing cytotoxic oxygen and nitrogen species, cytokines, and enzymes to restrain tumor growth (40, 41). Conversely, more recent research findings have highlighted TAMs’ pro-tumorigenic effects (12, 34), as they support tumor growth and metastasis through various mechanisms (36, 42), including promoting angiogenesis, suppressing anti-tumor immune responses (13, 22, 23), facilitating tumor cell invasion and metastasis (11, 21), and sustaining the survival of tumor stem cells (21).
In the tumor microenvironment, TAMs typically exhibit characteristics of M2 macrophages, which are associated with functions such as tissue repair promotion, anti-inflammatory responses, and immune regulation (12, 13, 17, 37, 42). These cells are characterized by the high expression of arginase-1 (Arg1), scavenger receptor CD163, and mannose receptor CD206 (42, 43). Importantly, these M2-like TAMs rely on cellular bioenergetic pathways, including the tricarboxylic acid (TCA) cycle, fatty acid oxidation (FAO), glycolysis, and mitochondrial oxidative phosphorylation (OxPhos) as their primary sources of cellular energy (22, 44). Furthermore, these M2-like TAMs produce various immunosuppressive cytokines such as M-CSF, transforming growth factor-β (TGF-β), vascular endothelial growth factor (VEGF), and IL-10, among others, which are categorized as Th2 cell-associated cytokines (38). These cytokines can promote tumor growth, immune evasion, and angiogenesis (13, 20, 22, 37). TAMs are frequently found in cancerous tumors, and their abundance correlates with poor prognosis (42). Overexpression of CSF-1 and CSF-1R on M2 macrophages represents a significant factor contributing to adverse tumor prognosis (19).
However, it is worth noting that the polarization state of TAMs is highly adaptable and influenced by various factors within the tumor microenvironment. For instance, hypoxic conditions can promote M2-like phenotype polarization by activating the ERK signaling pathway, thereby facilitating tumorigenesis (45). Additionally, factors such as the acidic conditions in the tumor microenvironment, factors secreted by tumor cells, and interactions with other immune cells are crucial determinants influencing TAM polarization (11, 13, 14, 33, 38, 46–49). These factors collectively form a complex regulatory network that dictates the multifaceted roles of TAMs in tumor development and immune evasion.
3.2 Signaling pathways mediated by M-CSF and their key role in TAMs
M-CSF regulates macrophage functions primarily by binding to its receptor CSF-1R and initiating downstream signaling pathways (Figure 3) (33).
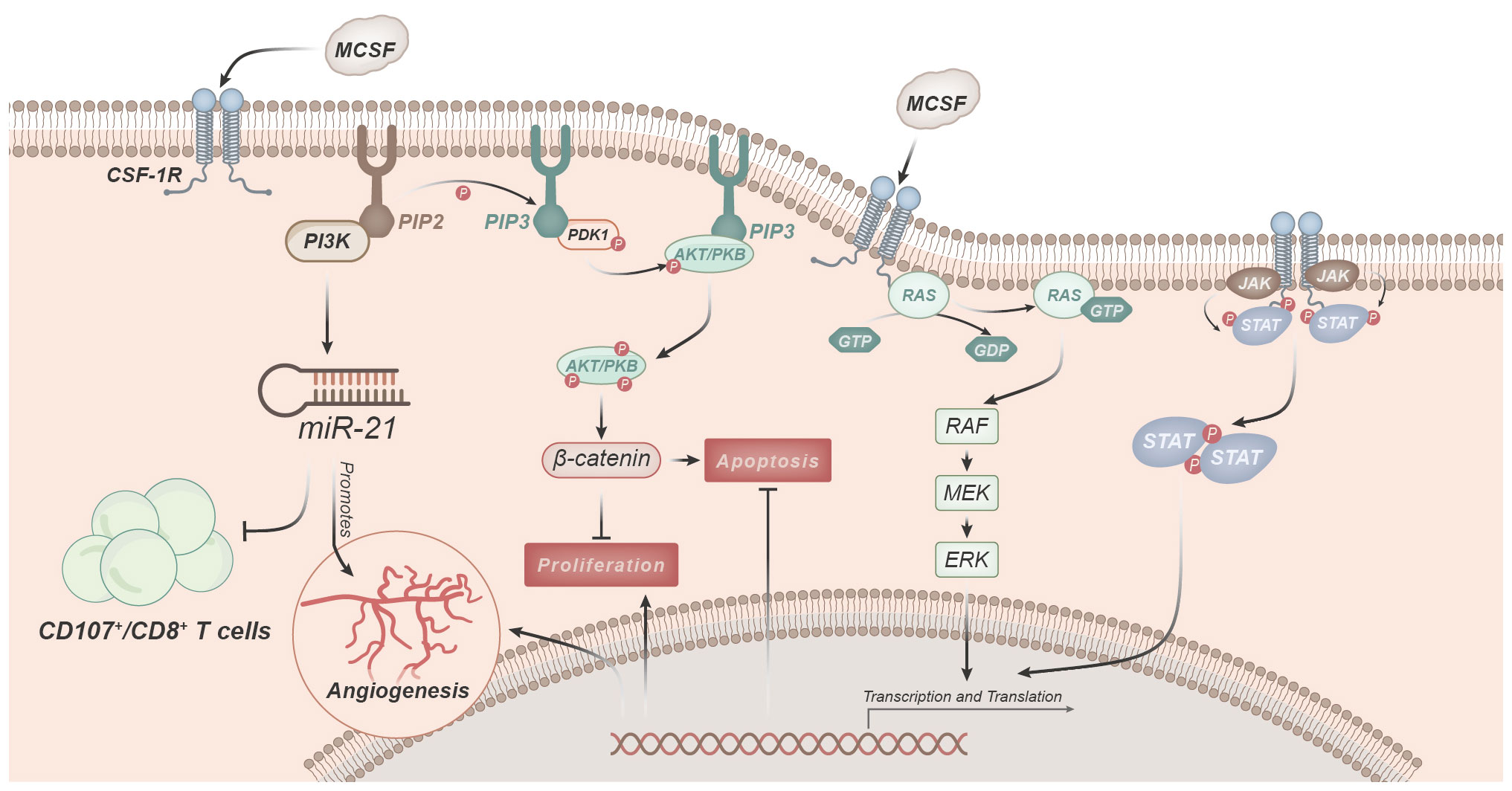
Figure 3 Signaling pathways involved in macrophage regulation and function mediated by M-CSF. M-CSF predominantly modulates macrophage functions by binding to its receptor, CSF-1R. Activation of CSF-1R triggers the PI3K pathway, leading to the conversion of PIP2 to PIP3. Subsequently, PIP3 facilitates the phosphorylation and activation of Akt kinase. Activated Akt, in turn, regulates various cellular functions, including macrophage survival, proliferation, and anti-apoptosis. CSF-1R signaling, through PI3K activation, induces miR-21 expression, promoting angiogenesis. Downstream signaling cascades initiated by CSF-1R, such as the Ras-Raf-MEK-ERK pathway, activate nuclear transcription factors, thereby initiating gene expression.
One of the key pathways involved is the PI3K-Akt pathway (11, 40, 47). Upon stimulation by M-CSF, activation of CSF-1R leads to the activation of PI3K, which catalyzes the conversion of phosphatidylinositol 4,5-bisphosphate (PIP2) to phosphatidylinositol 3,4,5-trisphosphate (PIP3). PIP3, in turn, promotes the phosphorylation and activation of Akt kinase (33). Activated Akt leads to the phosphorylation of GSK 3β, resulting in the release of β-catenin, which can mediate the regulation of various cellular functions (10, 24, 32, 33). CSF-1R signaling induces the expression of miR-21 through the activation of PI3K, thereby promoting angiogenesis. Simultaneously, this signal inhibits immune responses, such as reducing the number of CD107/CD8+ T cells in TAMs (11, 13, 14, 18, 36). Furthermore, transcriptional analysis also indicates the critical role of the PI3K-Akt signaling pathway in M-CSF-mediated regulation of macrophage functions (11).
Subsequently, the MAPK pathway comes into play. Downstream signaling pathways activated by CSF-1R, such as the Ras/Raf/MEK/ERK pathway, can activate nuclear transcription factors and initiate gene expression (11, 40). Macrophages activated by ERK are involved in cell growth, proliferation, differentiation, and inflammation regulation (14, 24, 32, 33, 50, 51). The MAPK/ERK pathway ultimately leads to an increase in the expression of biologically active VEGF, a key factor in the process of neovascularization. This regulation of VEGF primarily occurs at the gene transcription level, promoting the production of VEGF by monocytes and macrophages (52).
Moreover, the JAK-STAT pathway is also a crucial signaling pathway in M-CSF-mediated regulation of macrophage functions (32). Upon binding of M-CSF to CSF-1R, it activates JAK protein kinases, leading to their phosphorylation and activation. Subsequently, activated JAK further phosphorylates and activates STAT proteins. Phosphorylated STATs form homodimers or heterodimers that translocate to the cell nucleus, thereby controlling gene transcription and expression. This axis is a core intracellular signaling pathway that regulates various cytokine and growth factor signaling cascades, mediating downstream events such as inflammation infiltration, immune responses, repair, proliferation, differentiation, movement, and cell apoptosis (53).
In addition to the aforementioned signaling pathways, M-CSF can also regulate macrophage functions through pathways like NF-κB (54). The activation and interaction of these signaling pathways collectively regulate macrophage proliferation, survival, and polarization, enabling them to play a role in inflammation and immune responses.
In summary, the activation and interaction of these signaling pathways reflect the crucial role of macrophages in the immune system, not only in inflammatory responses but also in understanding their role in immune responses and diseases.
3.3 Regulatory role of M-CSF on TAM functions
M-CSF, as a pivotal regulatory molecule, confers a paramount role in the tumor microenvironment’s macrophages. Its influence extends beyond mere cellular survival and death, encompassing how these cells respond to stimuli from tumor cells. M-CSF exerts critical control over TAMs. This regulation is orchestrated through various molecular mechanisms, ensuring that macrophages exhibit functionality and adaptability within the tumor microenvironment. Furthermore, M-CSF provides the essential survival signals required by TAMs. This signaling cascade guarantees the proper protection of macrophages within the tumor microenvironment, averting premature cell death. In summary, M-CSF imparts crucial effects in regulating the fundamental functions of TAMs.
M-CSF exerts its effects not only through its intrinsic activity but also by activating various intracellular signaling pathways. Among these pathways, the PI3K-Akt signaling pathway serves as the principal avenue, responsible for promoting the survival, proliferation, anti-apoptosis, and migration of TAMs (55, 56). Additionally, M-CSF further refines its control over cells by activating the MAPK and JAK-STAT pathways (57, 58). These signaling pathways interact intricately within cells, forming a complex network that ensures cellular stability within the tumor environment. Particularly in the early stages of tumor progression, M-CSF, through these pathways, assures the survival and functionality of macrophages. In conclusion, M-CSF regulates the functionality of TAMs through multiple signaling pathways.
The tumor microenvironment represents a complex cellular network in which M-CSF serves as a bridging element. This bridge connects macrophages with tumor cells, leading macrophages to preferentially differentiate into M2-type macrophages within the tumor environment. These macrophages exhibit characteristics of immunosuppression and anti-inflammatory responses, further promoting tumor growth and development. Moreover, the overexpression of M-CSF reinforces this tendency, resulting in TAMs displaying more pronounced immunosuppressive traits. This feature is considered a crucial mechanism for tumor immune escape surveillance. In summary, the influence of M-CSF in the tumor microenvironment is both intricate and far-reaching.
Immunomodulation is another crucial role of M-CSF in the tumor microenvironment. M-CSF balances the degree of inflammatory reactions by regulating the production and release of inflammatory factors within macrophages (59, 60). For instance, it can inhibit the production of TNF-α and IL-6, thereby alleviating inflammatory responses. Simultaneously, M-CSF enhances macrophages’ capacity for inflammatory responses, enabling them to produce more lysozymes and oxygen radicals. This bidirectional action underscores the complexity of the role of M-CSF in immunomodulation. Therefore, M-CSF exerts a bidirectional and intricate role in regulating TAMs’ inflammatory responses.
Apart from the aforementioned primary functions, M-CSF has additional roles that are equally significant within the tumor microenvironment. For instance, M-CSF can induce macrophages to express certain immunosuppressive molecules, such as PD-L1 and PD-L2, contributing to the attenuation of macrophages’ anti-tumor activity (61). Moreover, M-CSF can regulate the formation of immune synapses in TAMs, which constitutes a critical interaction between immune cells and tumor cells. This interaction influences intercellular signal transduction and effector responses, further impacting tumor development. In summary, M-CSF assumes an indispensable role in regulating various functions of TAMs.
4 Impact of M-CSF on tumor development
Macrophage infiltration confers a direct or indirect regulatory role in the formation and progression of many tumors. In particular, the regulation of TAMs by M-CSF provides a favorable microenvironment for tumors, thus influencing tumor growth, metastasis, and immune evasion. Furthermore, the interactions between M-CSF and other cells and molecules in the tumor microenvironment also drive tumor progression. Understanding these effects of M-CSF provides us with a deeper insight and aids in the development of novel therapeutic strategies aimed at intervening in the tumor microenvironment, ultimately aiming to inhibit tumors or enhance the effectiveness of immunotherapy.
4.1 Catalytic role of M-CSF in tumor progression
With the advancement of scientific research, it has been firmly established that M-CSF plays a pivotal role in the field of tumor biology, particularly in the processes of tumor initiation, progression, and metastasis. The expression of M-CSF is intricately linked to tumor growth and development.
On the one hand, both tumor cells and other cells within their microenvironment, such as TAMs and tumor-associated dendritic cells, release various growth factors, including M-CSF, MCP-1, MCP-2, macrophage inflammatory protein-1α, and macrophage inflammatory protein-1β. Collectively, these cytokines drive tumor proliferation and growth by promoting the survival and proliferation of tumor cells, supplying them with essential nutrients, and further facilitating their migration and metastasis. In order to create a more favorable growth environment for tumor cells, it has been observed that tumor cells can produce M-CSF, consequently guiding the accumulation of surrounding tissue cells towards the tumor. This process may involve epithelial-to-mesenchymal transition (EMT), which enhances the migratory and invasive capabilities of tumor cells. Specifically, M-CSF can activate a series of transcription factors, such as Snail, Slug, and Twist, which inhibit the expression of the cell adhesion protein E-cadherin, thereby regulating the EMT process (62). Moreover, M-CSF can modulate the expression of cell cycle-related proteins within tumor cells, further driving their proliferation (63). On the other hand, M-CSF, through its binding with its specific receptor CSF-1R, activates multiple critical downstream signaling pathways such as PI3K/AKT and MAPK/ERK, thereby promoting tumor cell migration (64).
However, the impact of M-CSF extends beyond tumor cells alone. It plays a crucial role in the regulation of immune cells within the tumor microenvironment. For instance, M-CSF can enhance the proliferation of tumor-associated dendritic cells while reducing their activation and antigen-presenting capacity, leading to functional impairments and an inability to induce the proliferation of tumor-specific CD4+ and CD8+ T cells. Consequently, this affects the recognition and clearance of tumors by other immune cells. This also explains the close association between M-CSF expression levels and the degree of immune infiltration in tumors, patient prognosis, and treatment responsiveness.
In summary, M-CSF exerts multifaceted effects on the formation and progression of tumors. It not only directly influences tumor cells but also modulates immune cells within the tumor microenvironment and impacts the secretion of relevant molecules by immune cells, ultimately affecting tumor growth.
4.2 Regulatory mechanism of M-CSF on TAM-mediated tumor cell proliferation
Tumor development is a complex biological process involving interactions among various cell types. Particularly within the tumor microenvironment, M-CSF exerts a significant influence on tumor cell proliferation through the regulation of TAMs.
M-CSF not only directly stimulates the proliferation of liver cancer cells (65). More importantly, it plays a pivotal role in regulating TAMs. Multiple studies have confirmed that M-CSF can induce TAMs to differentiate into M2-type macrophages (66). In comparison to traditional M1-type macrophages, M2-type macrophages exhibit unique roles in immune modulation, primarily characterized by their anti-inflammatory and immunosuppressive properties. These cells release immunosuppressive factors such as TGF-β and IL-10, which can inhibit the activity of cytotoxic T cells, thus creating a more favorable growth environment for tumor cells.
Furthermore, M-CSF engages in close interactions with tumor cells and other cells within their microenvironment. For instance, tumor cells can produce M-CSF, attracting and guiding surrounding macrophages to accumulate at the tumor site, collectively creating a microenvironment conducive to tumor growth. These recruited TAMs, in turn, release more M-CSF, binding to CSF-1R on the surface of tumor cells, forming a positive feedback loop that facilitates continuous growth and survival of tumor cells.
4.3 Role of M-CSF in TAM-mediated tumor cell migration
The migration and infiltration of tumor cells are critical factors in tumor development and metastasis. Therefore, it is imperative to explore the role of M-CSF and its regulation of TAMs in this process.
In co-culture models of macrophages and tumor cells, macrophages predominantly exhibit M2-like characteristics. These macrophages not only promote the EMT process of colorectal cancer cells, enhancing their migratory and invasive capabilities (63), but also indirectly regulate the migration of tumor cells through various mechanisms. M-CSF can induce TAMs to differentiate towards the M2 phenotype (14, 17), thereby promoting the migration of tumor cells by secreting various cytokines such as epidermal growth factor (EGF), TNFs, and ILs (38). Tumor cells also self-secrete M-CSF, which not only attracts nearby macrophages to gather at the tumor site but also stimulates macrophages to release more M-CSF, binding to CSF-1R on the surface of tumor cells, forming a potent positive feedback mechanism (32, 67). Through the paracrine loop involving CSF-1R and epidermal growth factor, this mechanism enhances the interaction between tumor cells and macrophages, further promoting the migration and infiltration of tumor cells.
Hence, M-CSF holds a pivotal role in the regulation of tumor cell migration and infiltration. Its influence extends beyond direct effects on tumor cells, as it also modulates the behaviors of macrophages within the tumor microenvironment. This novel mechanistic understanding offers valuable insights and potential therapeutic targets for the treatment of cancer.
4.4 Interactions of M-CSF-mediated TAMs with tumor cell signaling pathways
The interaction between M-CSF and its receptor CSF-1R holds a central position in regulating the tumor microenvironment, particularly in the context of signaling crosstalk between TAMs and tumor cells.
Firstly, M-CSF influences the communication between TAMs and tumor cells by promoting TAM differentiation and proliferation (13, 14, 16). TAMs are a major immune cell type within the tumor microenvironment and play crucial roles in tumor growth, invasion, and metastasis (36). M-CSF, through binding to its receptor CSF-1R, not only activates signaling pathways such as PI3K/Akt and MAPK, driving TAM survival and proliferation, but also induces TAM polarization towards the immunosuppressive M2-like macrophages, reinforcing their supportive role in promoting tumor cell growth (18, 21, 67).
Secondly, M-CSF modulates TAM cytokine secretion, including IL-2, VEGF, EGF, TGF-β, and CCL22 (13, 21, 68), thereby impacting the behavior of tumor cells. These cytokines play diverse roles in tumor development, including stimulating angiogenesis, suppressing T cell-mediated immune responses, and promoting tumor cell survival and proliferation (13, 23, 36). Particularly under M-CSF regulation, TAMs promote angiogenesis and tumor cell proliferation by secreting VEGF and EGF, creating a favorable microenvironment for tumor progression (22).
Furthermore, M-CSF also regulates the expression of surface molecules on TAMs, such as PD-L1 and CD206, enhancing the signaling crosstalk between TAMs and tumor cells. The expression of PD-L1, an immune checkpoint molecule, on TAMs inhibits the activity of CD8+ T cells, creating favorable conditions for tumor growth and metastasis. Additionally, CD80 and CD86 as co-stimulatory molecules are expressed on TAMs, influencing immune responses in the tumor microenvironment by regulating T cell activation and responses (20, 21, 36). CD206, as a marker of M2-like macrophages, further supports the polarization of TAMs towards the M2-like phenotype following M-CSF action (36).
Therefore, M-CSF exerts its influence on the tumor microenvironment through multiple mechanisms to modulate TAM behavior, subsequently affecting tumor cell growth, migration, and infiltration. This finding provides a novel theoretical foundation and strategic direction for immunotherapy approaches against cancer.
5 Clinical applications of M-CSF: therapeutic strategies and outcomes
Given the pivotal role of M-CSF in tumor development, it has become a focal point of research in cancer treatment strategies. The following sections delve into the clinical applications of M-CSF and how its activity can be modulated by other cytokines to enhance treatment outcomes for patients.
5.1 Characteristics of M-CSF in tumor tissues
The role of M-CSF in tumor tissues is often significant, playing a critical role in tumor progression. High expression of M-CSF has been observed in various cancer types, including breast cancer, lung cancer, gastric cancer, and colorectal cancer (15, 32). Furthermore, studies have found that M-CSF expression correlates with disease severity and stages in ovarian cancer, glioblastoma, and triple-negative breast cancer (TNBC) (38, 46, 69). In recent years, a plethora of research has shown that M-CSF expression increases with the growth of tumor tissues. For instance, a previous study has noted that M-CSF expression is higher in lung cancer at advanced stages than at early stages (38, 70), and elevated M-CSF levels in the serum of patients with various types of cancer, including pancreatic and cervical cancers, were associated with advanced-stage tumors (71, 72). High levels of M-CSF expression were also significantly correlated with the distribution of CD163/CD68 TAMs in grade IV glioblastomas (38, 69). Furthermore, TNBC secretes more M-CSF compared to non-TNBC (46).
High M-CSF expression is not limited to tumor cells alone. In the tumor microenvironment, M-CSF is also produced by other cells, such as TAMs and stromal cells (38). These cytokines significantly impact the growth, differentiation, and functional expression of macrophages by binding to their specific receptor, c-fms (14). Additionally, M-CSF can regulate TAM polarization, promoting their transformation into an M2-like phenotype, further supporting tumor growth and metastasis (18).
It is worth noting that M-CSF is not only associated with tumor growth and metastasis but also with challenging aspects of cancer treatment, such as immune evasion, drug resistance, and radiotherapy resistance. Therefore, research on M-CSF not only aids in gaining a deeper understanding of the biological characteristics of tumors but may also provide new strategies and approaches for cancer treatment.
5.2 Correlation analysis of the role of M-CSF with clinical prognosis in cancer patients
In the context of cancer treatment and management, the identification and assessment of prognostic factors are of paramount importance. These factors not only offer predictions about potential clinical outcomes for patients but also provide essential information for tailoring individualized treatment plans. In this context, future research should pay attention to the functional relevance of M-CSF in the tumor microenvironment, particularly its regulation of TAMs.
High expression of M-CSF has been linked to adverse clinical outcomes in various cancer types (32, 69). Notably, in non-small cell lung cancer, studies have revealed a positive correlation between M-CSF expression and the degree of TAM infiltration, which, in turn, significantly correlates with 5-year overall survival and disease-free survival rates. Specifically, patients with high M-CSF expression have a markedly lower 5-year overall survival rate of only 19.6%, compared to 51.3% in patients with lower expression levels (11, 38, 73).
The composition of immune cells within the tumor microenvironment, especially the presence and functional status of TAMs, provides crucial clues for assessing tumor progression. Immunological and stromal scores aid in evaluating the extent of immune cell infiltration and tumor purity in the tumor microenvironment (69). TAMs, particularly M2-like macrophages, are directly associated with the regulation of M-CSF and the release of other factors. M2-like macrophages are believed to exert inhibitory effects on the anti-tumor immune response. Prior research has indicated that TAMs are the predominant inflammatory cells in various cancers and are closely linked to disease progression and prognosis (73). Cytokines derived from M2 macrophages, such as IL-10 and TGF-β, are considered major mechanisms for suppressing anti-tumor immune activity (14).
In summary, the interplay between M-CSF and TAMs, especially M2-like macrophages, significantly impacts the immune microenvironment of tumors and patient prognosis. Clinical research has demonstrated the association of high M-CSF expression with adverse outcomes. Therefore, a thorough exploration of the role of M-CSF is crucial for improving treatment strategies and prognostic assessments.
5.3 Role of M-CSF in clinical therapies and strategies for modulation
M-CSF has been recognized as a crucial therapeutic target in cancer treatment (5, 38, 46). Multiple studies have demonstrated that inhibiting the interaction of CSF-1/CSF-1R, leading to the elimination of TAMs, is an effective strategy in cancer therapy (5, 11, 20, 21, 24, 32). Inhibitors targeting M-CSF or its receptor c-fms have shown significant effects in suppressing tumor growth and improving prognosis (20, 34). For instance, daily treatment with an anti-CSF-1R therapeutic antibody (50 mg/kg) has been proven to reduce the number of macrophages in a peritumoral osteosarcoma mouse model. Axatilimab, a novel humanized monoclonal antibody, can block the binding of M-CSF to its receptor, thereby inhibiting CSF-1R signaling and macrophage development (74). Moreover, active components of traditional Chinese medicine, such as cannabidiol, have been found to inhibit M-CSF secretion by melanoma cells, thereby affecting the immunosuppressive tumor microenvironment (49). Thus, the targeting of M-CSF in cancer therapy and the application of its inhibitors in combination therapy provide a promising and novel strategy for clinical cancer treatment.
M-CSF has been extensively reported to control immune responses, particularly processes related to tumor immune responses. It can stimulate the production and activation of M2 macrophages, resulting in a decrease in the number of antigen-presenting cells within the tumor microenvironment. Furthermore, M-CSF can inhibit macrophage phagocytosis and cytotoxicity, further reducing the body’s attack on tumor cells. Therefore, the regulatory role of M-CSF may impact the effectiveness of tumor treatment. When macrophage functions are suppressed, the body’s ability to attack tumor cells is diminished. Hence, understanding and manipulating the role of M-CSF in tumor immune responses are crucial for the development of more effective cancer treatment strategies.
M-CSF is closely associated with the process of angiogenesis in tumors. Research indicates that M-CSF can enhance the angiogenic capacity of tumor cells, thereby providing more blood supply and nutrients to the tumor (13, 23). M-CSF achieves this effect by inducing the survival of vascular endothelial cells and promoting the expression of factors related to angiogenesis, such as VEGF (75). In a mouse osteosarcoma model, inhibiting M-CSF activity selectively suppressed tumor angiogenesis and lymphangiogenesis, offering possibilities for exploring novel treatment strategies (76).
M-CSF is involved in the recruitment of macrophages in the tumor microenvironment (13, 20, 77). This process is crucial for sustaining tumor growth and metastasis. M-CSF antibodies have been demonstrated to significantly inhibit macrophage migration, thereby reducing the content of F-actin (46).
Although M-CSF and its receptor inhibitors have shown some effectiveness in cancer treatment, combining them with other treatment methods is more likely to yield significant therapeutic benefits. For example, PLX3397 is a known M-CSF receptor inhibitor that not only depletes TAMs but also reduces FOXP3+ regulatory T cells, promoting the migration and infiltration of CD8+ T cells into the tumor (78). Research has also found that the combination of CSF1R inhibitors with anti-PD-L1 therapy is more effective in preventing tumor growth than single treatments (18).
M-CSF is closely related to chemotherapy and radiotherapy. Chemotherapeutic drugs, such as cyclophosphamide (CTX), have been shown to reduce M-CSF levels in tissues, thereby inhibiting tumor cell survival (68). Furthermore, M-CSF is associated with the response to radiotherapy, as the expression of M-CSF in glioblastoma cells is related to resistance to the anti-tumor drug 5-FU (38).
M-CSF assumes a substantial role in alleviating the side effects of cancer treatment. Since cancer treatments may lead to bone marrow suppression and immune function decline, the use of M-CSF can promote the proliferation and differentiation of hematopoietic stem cells, thereby improving bone marrow function and immune function. For example, the use of hydrolyzed protein injection upregulated the expression of IL-2 and M-CSF, altering the composition of bone marrow hematopoietic cell populations, increasing the numbers of hematopoietic stem cells, B lymphocytes, macrophages, and granulocytes (68). Therefore, the use of M-CSF can alleviate the side effects during treatment and improve the quality of life for patients.
M-CSF affects the interactions between immune cells and tumor cells in the tumor microenvironment and the efficacy of chemotherapy and radiotherapy. It is worth noting that while the use of M-CSF or its receptor inhibitors alone has shown therapeutic potential in some cases, more research suggests that combining them with other treatment methods may enhance therapeutic efficacy. Additionally, the application of M-CSF contributes to alleviating side effects associated with cancer treatment and improving patients’ quality of life. Therefore, in-depth research into the role of M-CSF in tumor biology and its potential as part of comprehensive treatment strategies is of paramount importance.
5.4 Research on cytokine-mediated regulation of M-CSF
After extensive investigation into the role of M-CSF in cancer development, researchers have acknowledged the potential interactions between M-CSF and other cytokines, sparking exploration into the potential implications of these interactions for cancer treatment. The related research has yielded novel treatment strategies and expanded our perspective on cancer research.
The utilization of strategies involving other cytokines to modulate M-CSF presents innovative avenues for cancer treatment. For example, IFN-γ has the potential to not only disrupt the signaling of M-CSF, inhibiting tumor growth, but also enhance the efficacy of various anti-tumor therapies (79). Additionally, the anti-inflammatory properties of Interleukin-10 (IL-10) are also related to its ability to inhibit M-CSF, potentially opening up new avenues for its application in cancer treatment (36). TNF-α and IL-1β, as inflammatory cytokines, play a crucial role in regulating the expression of M-CSF. They may collaboratively participate in modulating the tumor microenvironment, thereby influencing the effectiveness of immunotherapy. However, these interactions are not always beneficial, especially in the presence of M-CSF, as TNF-α may promote the proliferation of macrophages and tumor cells (11). TGF-β and EGF play important roles in regulating M-CSF. They can alter the tumor microenvironment and potentially synergize with M-CSF, either enhancing or inhibiting the effectiveness of cancer treatment.
In summary, the interactions between M-CSF and other cytokines provide a new perspective for gaining a deeper understanding of the mechanisms of cancer development and developing novel treatment strategies.
6 Future perspectives in M-CSF research
As our understanding of the role of M-CSF in tumor development grows, we are recognizing its pivotal involvement in regulating interactions between immune cells and tumor cells. M-CSF not only directly affects tumor cell proliferation, migration, and invasion but also exerts influence on immune cells within the tumor microenvironment, such as by promoting the polarization of macrophages and inducing an inhibitory phenotype. Furthermore, the interactions of M-CSF with signaling molecules like the Wnt, Notch, and Hedgehog pathways may further modulate tumor progression. Consequently, a thorough examination of the intricate interplay between M-CSF and these pathways, along with a systematic investigation into their role in orchestrating tumor progression, presents substantial opportunities for the identification of novel cancer therapy strategies.
Emerging technologies, such as single-cell sequencing, artificial intelligence, microfluidic chips, tumor organoid cultures, and in vivo imaging techniques are revolutionizing M-CSF research. For instance, microfluidic chip technology can simulate cell-to-cell interactions in the tumor microenvironment, aiding our better understanding of how M-CSF affects cellular behavior. In vivo imaging techniques can monitor the expression and distribution of M-CSF in animal models, providing critical information for drug development and assessment.
The potential of whole-genome sequencing to unveil genetic and epigenetic characteristics of tumors, when combined with data on M-CSF expression patterns, paves the way for personalized treatment regimens. These tailored approaches aim to maximize therapeutic benefits for each patient, emphasizing the importance of individualized care in the fight against cancer.
Moreover, the integration of M-CSF inhibitors with other treatment modalities, such as radiation therapy, immunotherapy, and other targeted therapies, such as EGFR inhibitors, BRAF inhibitors, and mTOR inhibitors, holds promise. The concurrent inhibition of M-CSF and pathways like EGFR, for instance, might synergistically enhance treatment efficacy, offering new hope for more effective cancer treatments.
While early clinical trials have shown some therapeutic efficacy of M-CSF inhibitors, there is a pressing need for further research. It is crucial to determine the optimal dosage, treatment duration, and combination strategies with other therapies. Given the variations among patients, such as genetic polymorphisms, tumor heterogeneity, and immune status, personalized treatment plans need to be developed to ensure that each patient attains the best therapeutic outcomes. Meanwhile, challenges such as drug resistance, side effects, and the potential impact on normal physiological processes due to M-CSF inhibition must not be overlooked. Future research must strive to balance treatment efficacy with minimizing side effects, ensuring not only the effectiveness of therapy but also the quality of life for patients.
In conclusion, the advances in our understanding of the mechanism of M-CSF and the application of new technologies offer promising prospects for M-CSF-related strategies in oncology. The integration of M-CSF-related target therapies with other treatment modalities, the implementation of clinical applications and translational research, the formulation of personalized treatment strategies, and the resolution of potential issues and challenges emerge as crucial avenues for further investigation (Figure 4). Through continuous dedication and efforts, M-CSF-related target therapies holds the promise of ushering in a more optimistic future for cancer patients.
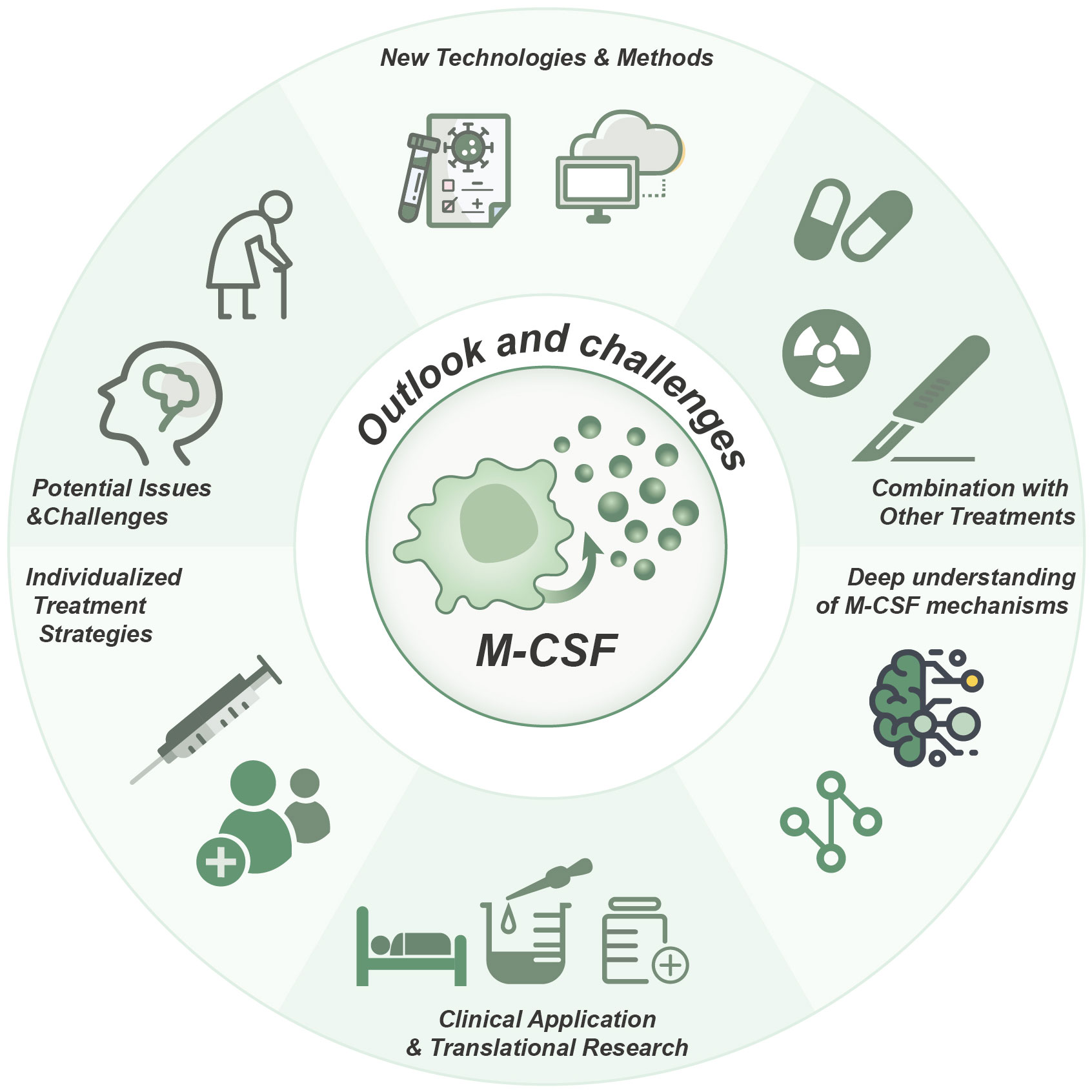
Figure 4 Role, strategies, and future prospects of M-CSF in cancer therapy. Targeting M-CSF as a therapeutic approach in cancer, particularly in combination therapies, holds significant promise for clinical applications. The development of individualized treatment strategies, a more profound comprehension of M-CSF mechanisms, and the utilization of innovative technologies are essential for achieving optimal therapeutic outcomes.
Author contributions
LY: Conceptualization, Methodology, Writing – original draft, Writing – review & editing. YG: Conceptualization, Formal analysis, Methodology, Writing – original draft, Writing – review & editing. ZC: Data curation, Supervision, Writing – review & editing. KT: Data curation, Formal analysis, Methodology, Writing – review & editing. PL: Data curation, Supervision, Writing – review & editing. HL: Data curation, Investigation, Software, Writing – review & editing. XX: Data curation, Formal analysis, Supervision, Writing – review & editing. QP: Data curation, Investigation, Writing – original draft, Writing – review & editing. XL: Conceptualization, Formal analysis, Funding acquisition, Methodology, Project administration, Writing – original draft, Writing – review & editing.
Funding
The author(s) declare financial support was received for the research, authorship, and/or publication of this article. This study was supported by Qiqihar Medical College 2023 Innovation and Entrepreneurship Training Program for College Students (Project No. X202311230028).
Conflict of interest
The authors declare that the research was conducted in the absence of any commercial or financial relationships that could be construed as a potential conflict of interest.
Publisher’s note
All claims expressed in this article are solely those of the authors and do not necessarily represent those of their affiliated organizations, or those of the publisher, the editors and the reviewers. Any product that may be evaluated in this article, or claim that may be made by its manufacturer, is not guaranteed or endorsed by the publisher.
References
1. Siegel RL, Miller KD, Fuchs HE, Jemal A. Cancer statistics, 2022. CA Cancer J Clin. (2022) 72(1):7–33. doi: 10.3322/caac.21708
2. Wang Y, Yan Q, Fan C, Mo Y, Wang Y, Li X, et al. Overview and countermeasures of cancer burden in china. Sci China Life Sci. (2023) 66(11):2515–26. doi: 10.1007/s11427-022-2240-6
3. Wadhwa V, Patel N, Grover D, Ali FS, Thosani N. Interventional gastroenterology in oncology. CA Cancer J Clin. (2023) 73(3):286–319. doi: 10.3322/caac.21766
4. Arneth B. Tumor microenvironment. Medicina (Kaunas). (2019) 56(1):15. doi: 10.3390/medicina56010015
5. Van Overmeire E, Stijlemans B, Heymann F, Keirsse J, Morias Y, Elkrim Y, et al. And GM-CSF receptor signaling differentially regulate monocyte maturation and macrophage polarization in the tumor microenvironment. Cancer Res. (2016) 76(1):35–42. doi: 10.1158/0008-5472.CAN-15-0869
6. Bonelli S, Geeraerts X, Bolli E, Keirsse J, Kiss M, Pombo Antunes AR, et al. Beyond the m-CSF receptor - novel therapeutic targets in tumor-associated macrophages. FEBS J. (2018) 285(4):777–87. doi: 10.1111/febs.14202
7. Wang Y, Lyu Z, Qin Y, Wang X, Sun L, Zhang Y, et al. FOXO1 promotes tumor progression by increased M2 macrophage infiltration in esophageal squamous cell carcinoma. Theranostics. (2020) 10(25):11535–48. doi: 10.7150/thno.45261
8. Akazawa Y, Kono H, Hara M, Furuya S, Nakata Y, Wakana H, et al. M-CSF receptor antagonists inhibit the initiation and progression of hepatocellular carcinoma in mice. Anticancer Res. (2019) 39(9):4787–94. doi: 10.21873/anticanres.13663
9. Mossadegh-Keller N, Sarrazin S, Kandalla PK, Espinosa L, Stanley ER, Nutt SL, et al. M-CSF instructs myeloid lineage fate in single haematopoietic stem cells. Nature. (2013) 497(7448):239–43. doi: 10.1038/nature12026
10. Rusiniak ME, Punch PR, Hait NC, Maiti A, Burns RT, Chapla D, et al. Extracellular ST6GAL1 regulates monocyte-macrophage development and survival. Glycobiology. (2022) 32(8):701–11. doi: 10.1093/glycob/cwac032
11. Chen YC, Lai YS, Hsuuw YD, Chang KT. Withholding of m-CSF supplement reprograms macrophages to M2-like via endogenous CSF-1 activation. Int J Mol Sci. (2021) 22(7):3532. doi: 10.3390/ijms22073532
12. Yadav S, Priya A, Borade DR, Agrawal-Rajput R. Macrophage subsets and their role: co-relation with colony-stimulating factor-1 receptor and clinical relevance. Immunol Res. (2023) 71(2):130–52. doi: 10.1007/s12026-022-09330-8
13. Schiopu A, Bengtsson E, Gonçalves I, Nilsson J, Fredrikson GN, Björkbacka H. Associations between macrophage colony-stimulating factor and monocyte chemotactic protein 1 in plasma and first-time coronary events: A nested case-control study. J Am Heart Assoc. (2016) 5(9):e002851. doi: 10.1161/JAHA.115.002851
14. Zuo B, Li T, Liu X, Wang S, Cheng J, Liu X, et al. Dipeptidyl peptidase 4 inhibitor reduces tumor-associated macrophages and enhances anti-PD-L1-mediated tumor suppression in non-small cell lung cancer. Clin Transl Oncol. (2023) 25(11):3188–202. doi: 10.1007/s12094-023-03187-5
15. Ushach I, Zlotnik A. Biological role of granulocyte macrophage colony-stimulating factor (GM-CSF) and macrophage colony-stimulating factor (M-CSF) on cells of the myeloid lineage. J Leukoc Biol. (2016) 100(3):481–9. doi: 10.1189/jlb.3RU0316-144R
16. Lee JM, Shin KS, Koh CH, Song B, Jeon I, Park MH, et al. Inhibition of topoisomerase i shapes antitumor immunity through the induction of monocyte-derived dendritic cells. Cancer Lett. (2021) 520:38–47. doi: 10.1016/j.canlet.2021.06.031
17. Jia D, Lu Y, Lv M, Wang F, Lu X, Zhu W, et al. Targeted co-delivery of resiquimod and a SIRPα variant by liposomes to activate macrophage immune responses for tumor immunotherapy. J Control Release. (2023) 360:858–71. doi: 10.1016/j.jconrel.2023.07.030
18. Magkouta SF, Vaitsi PC, Pappas AG, Iliopoulou M, Kosti CN, Psarra K, et al. CSF1/CSF1R axis blockade limits mesothelioma and enhances efficiency of anti-PDL1 immunotherapy. Cancers (Basel). (2021) 13(11):2546. doi: 10.3390/cancers13112546
19. Sun Y, Cronin MF, Mendonça MCP, Guo J, O'Driscoll CM. Sialic acid-targeted cyclodextrin-based nanoparticles deliver CSF-1R siRNA and reprogram tumour-associated macrophages for immunotherapy of prostate cancer. Eur J Pharm Sci. (2023) 185:106427. doi: 10.1016/j.ejps.2023.106427
20. Zhang W, Jiang X, Zou Y, Yuan L, Wang X. Pexidartinib synergize PD-1 antibody through inhibiting treg infiltration by reducing TAM-derived CCL22 in lung adenocarcinoma. Front Pharmacol. (2023) 14:1092767. doi: 10.3389/fphar.2023.1092767
21. Jeon Y, Kang H, Yang Y, Park D, Choi B, Kim J, et al. A novel selective Axl/Mer/CSF1R kinase inhibitor as a cancer immunotherapeutic agent targeting both immune and tumor cells in the tumor microenvironment. Cancers (Basel). (2022) 14(19):4821. doi: 10.3390/cancers14194821
22. Pizzurro GA, Bridges K, Jiang X, Vidyarthi A, Miller-Jensen K, Colegio OR. Functionally and metabolically divergent melanoma-associated macrophages originate from common bone-marrow precursors. Cancers (Basel). (2023) 15(13):3330. doi: 10.3390/cancers15133330
23. Lv YF, Liao ZQ, Bi QC, Xie CS, Wei XY, Yun Y, et al. Irreversible repolarization of tumour-associated macrophages by low-pi stress inhibits the progression of hepatocellular carcinoma. J Cell Mol Med. (2023) 27(19):2906–21. doi: 10.1111/jcmm.17861
24. Xiang C, Li H, Tang W. Targeting CSF-1R represents an effective strategy in modulating inflammatory diseases. Pharmacol Res. (2023) 187:106566. doi: 10.1016/j.phrs.2022.106566
25. Kandalla PK, Subburayalu J, Cocita C, de Laval B, Tomasello E, Iacono J, et al. M-CSF directs myeloid and NK cell differentiation to protect from CMV after hematopoietic cell transplantation. EMBO Mol Med. (2023) 15(11):e17694. doi: 10.15252/emmm.202317694
26. Ding J, Guo C, Hu P, Chen J, Liu Q, Wu X, et al. CSF1 is involved in breast cancer progression through inducing monocyte differentiation and homing. Int J Oncol. (2016) 49(5):2064–74. doi: 10.3892/ijo.2016.3680
27. Fuentelsaz-Romero S, Cuervo A, Estrada-Capetillo L, Celis R, García-Campos R, Ramírez J, et al. And macrophage polarization in joints of undifferentiated arthritis patients evolving to rheumatoid arthritis or psoriatic arthritis. Front Immunol. (2021) 11:613975. doi: 10.3389/fimmu.2020.613975
28. Lindau A, Härdtner C, Hergeth SP, Blanz KD, Dufner B, Hoppe N, et al. Atheroprotection through SYK inhibition fails in established disease when local macrophage proliferation dominates lesion progression. Basic Res Cardiol. (2016) 111(2):20. doi: 10.1007/s00395-016-0535-8
29. Schaller-Paule MA, Yalachkov Y, Steinmetz H, Friedauer L, Hattingen E, Miesbach W, et al. Analysis of CSF d-dimer to identify intrathecal fibrin-driven autoimmunity in patients with multiple sclerosis. Neurol Neuroimmunol Neuroinflamm. (2022) 9:):e1150. doi: 10.1212/NXI.0000000000001150
30. Yang Y, Qin J, Lan L, Li N, Wang C, He P, et al. M-CSF cooperating with NFκB induces macrophage transformation from M1 to M2 by upregulating c-jun. Cancer Biol Ther. (2014) 15(1):99–107. doi: 10.4161/cbt.26718
31. Liu H, Zhao D, Li H, Zhang W, Lin Q, Wang X, et al. Blocking iASPP/Nrf2/M-CSF axis improves anti-cancer effect of chemotherapy-induced senescence by attenuating M2 polarization. Cell Death Dis. (2022) 13(2):166. doi: 10.1038/s41419-022-04611-4
32. Mougel A, Adriaenssens E, Guyot B, Tian L, Gobert S, Chassat T, et al. Macrophage-Colony-Stimulating factor receptor enhances prostate cancer cell growth and aggressiveness In vitro and In vivo and increases osteopontin expression. Int J Mol Sci. (2022) 23(24):16028. doi: 10.3390/ijms232416028
33. Zhang L, Wang Y, Xiao F, Wang S, Xing G, Li Y, et al. CKIP-1 regulates macrophage proliferation by inhibiting TRAF6-mediated akt activation. Cell Res. (2014) 24(6):742–61. doi: 10.1038/cr.2014.53
34. Waaijer SJH, Suurs FV, Hau CS, Vrijland K, de Visser KE, de Groot DJA, et al. Radiolabeled monoclonal antibody against colony-stimulating factor 1 receptor specifically distributes to the spleen and liver in immunocompetent mice. Front Oncol. (2021) 11:786191. doi: 10.3389/fonc.2021.786191
35. Montemurro N, Pahwa B, Tayal A, Shukla A, De Jesus Encarnacion M, Ramirez I, et al. Macrophages in recurrent glioblastoma as a prognostic factor in the synergistic system of the tumor microenvironment. Neurol Int. (2023) 15(2):595–608. doi: 10.3390/neurolint15020037
36. Wu K, Lin K, Li X, Yuan X, Xu P, Ni P, et al. Redefining tumor-associated macrophage subpopulations and functions in the tumor microenvironment. Front Immunol. (2020) 11:1731. doi: 10.3389/fimmu.2020.01731
37. Han S, Bao X, Zou Y, Wang L, Li Y, Yang L, et al. -lactate modulates M2 tumor-associated macrophages and remodels immunosuppressive tumor microenvironment for hepatocellular carcinoma. Sci Adv. (2023) 9(29):eadg2697. doi: 10.1126/sciadv.adg2697
38. Lu CS, Shiau AL, Su BH, Hsu TS, Wang CT, Su YC, et al. Oct4 promotes M2 macrophage polarization through upregulation of macrophage colony-stimulating factor in lung cancer. J Hematol Oncol. (2020) 13(1):62. doi: 10.1186/s13045-020-00887-1
39. Manji GA, Van Tine BA, Lee SM, Raufi AG, Pellicciotta I, Hirbe AC, et al. A phase i study of the combination of pexidartinib and sirolimus to target tumor-associated macrophages in unresectable sarcoma and malignant peripheral nerve sheath tumors. Clin Cancer Res. (2021) 27(20):5519–27. doi: 10.1158/1078-0432.CCR-21-1779
40. Cai H, Zhang Y, Wang J, Gu J. Defects in macrophage reprogramming in cancer therapy: The negative impact of PD-L1/PD-1. Front Immunol. (2021) 12:690869. doi: 10.3389/fimmu.2021.690869
41. Sica A, Mantovani A. Macrophage plasticity and polarization: in vivo veritas. J Clin Invest. (2012) 122(3):787–95. doi: 10.1172/JCI59643
42. Tran NL, Lee IK, Kim H, Lee K, Kim SH, Oh SJ. Facile construction of tumour spheroids with induced M2 macrophage polarization for anticancer drug screening. BioMed Mater. (2022) 17(6).
43. Korotkaja K, Jansons J, Spunde K, Rudevica Z, Zajakina A. Establishment and characterization of free-floating 3D macrophage programming model in the presence of cancer cell spheroids. Int J Mol Sci. (2023) 24(13):10763. doi: 10.3390/ijms241310763
44. Jiang S, Fu W, Wang S, Zhu G, Wang J, Ma Y. Bacterial outer membrane vesicles loaded with perhexiline suppress tumor development by regulating tumor-associated macrophages repolarization in a synergistic way. Int J Mol Sci. (2023) 24(13):11222. doi: 10.3390/ijms241311222
45. Zhang J, Cao J, Ma S, Dong R, Meng W, Ying M, et al. Tumor hypoxia enhances non-small cell lung cancer metastasis by selectively promoting macrophage M2 polarization through the activation of ERK signaling. Oncotarget. (2014) 5(20):9664–77. doi: 10.18632/oncotarget.1856
46. Lu X, Yang R, Zhang L, Xi Y, Zhao J, Wang F, et al. Macrophage colony-stimulating factor mediates the recruitment of macrophages in triple negative breast cancer. Int J Biol Sci. (2019) 15(13):2859–71. doi: 10.7150/ijbs.39063
47. Zhang M, Zhang H, Tang F, Wang Y, Mo Z, Lei X, et al. Doxorubicin resistance mediated by cytoplasmic macrophage colony-stimulating factor is associated with switch from apoptosis to autophagic cell death in MCF-7 breast cancer cells. Exp Biol Med (Maywood). (2016) 241(18):2086–93. doi: 10.1177/1535370216660399
48. Chen YM, Hung WT, Chang WC, Hsieh CW, Chung WH, Lan JL, et al. Genetic association and expression correlation between colony-stimulating factor 1 gene encoding m-CSF and adult-onset still's disease. J Immunol Res. (2020) 2020:8640719. doi: 10.1155/2020/8640719
49. Wyrobnik I, Steinberg M, Gelfand A, Rosenblum R, Eid Mutlak Y, Sulimani L, et al. Decreased melanoma CSF-1 secretion by cannabigerol treatment reprograms regulatory myeloid cells and reduces tumor progression. Oncoimmunology. (2023) 12(1):2219164. doi: 10.1080/2162402X.2023.2219164
50. Chu CH, Wang S, Li CL, Chen SH, Hu CF, Chung YL, et al. Neurons and astroglia govern microglial endotoxin tolerance through macrophage colony-stimulating factor receptor-mediated ERK1/2 signals. Brain Behav Immun. (2016) 55:260–72. doi: 10.1016/j.bbi.2016.04.015
51. Digiacomo G, Ziche M, Dello Sbarba P, Donnini S, Rovida E. Prostaglandin E2 transactivates the colony-stimulating factor-1 receptor and synergizes with colony-stimulating factor-1 in the induction of macrophage migration via the mitogen-activated protein kinase ERK1/2. FASEB J. (2015) 29(6):2545–54. doi: 10.1096/fj.14-258939
52. Gallo CC, Honda TSB, Alves PT, Han SW. Macrophages mobilized by the overexpression of the macrophage-colony stimulating factor promote efficient recovery of the ischemic muscle functionality. Life Sci. (2023) 317:121478. doi: 10.1016/j.lfs.2023.121478
53. Liu Y, Wang W, Zhang J, Gao S, Xu T, Yin Y. JAK/STAT signaling in diabetic kidney disease. Front Cell Dev Biol. (2023) 11:1233259. doi: 10.3389/fcell.2023.1233259
54. Wang Y, Mo X, Piper MG, Wang H, Parinandi NL, Guttridge D, et al. M-CSF induces monocyte survival by activating NF-κB p65 phosphorylation at Ser276 via protein kinase C. PloS One. (2011) 6(12):e28081. doi: 10.1371/journal.pone.0028081
55. Wang L, Iorio C, Yan K, Yang H, Takeshita S, Kang S, et al. A ERK/RSK-mediated negative feedback loop regulates m-CSF-evoked PI3K/AKT activation in macrophages. FASEB J. (2018) 32(2):875–87. doi: 10.1096/fj.201700672RR
56. Rodriguez RM, Suarez-Alvarez B, Lavín JL, Ascensión AM, Gonzalez M, Lozano JJ, et al. Signal integration and transcriptional regulation of the inflammatory response mediated by the GM-/M-CSF signaling axis in human monocytes. Cell Rep. (2019) 29(4):860–872.e5. doi: 10.1016/j.celrep.2019.09.035
57. Matoba K, Kawanami D, Tsukamoto M, Kinoshita J, Ito T, Ishizawa S, et al. Rho-kinase regulation of TNF-α-induced nuclear translocation of NF-κB RelA/p65 and m-CSF expression via p38 MAPK in mesangial cells. Am J Physiol Renal Physiol. (2014) 307(5):F571–80. doi: 10.1152/ajprenal.00113.2014
58. Wang F, Zhang X, Liu W, Zhou Y, Wei W, Liu D, et al. Activated natural killer cell promotes nonalcoholic steatohepatitis through mediating JAK/STAT pathway. Cell Mol Gastroenterol Hepatol. (2022) 13(1):257–74. doi: 10.1016/j.jcmgh.2021.08.019
59. Simón-Fuentes M, Herrero C, Acero-Riaguas L, Nieto C, Lasala F, Labiod N, et al. TLR7 activation in m-CSF-Dependent monocyte-derived human macrophages potentiates inflammatory responses and prompts neutrophil recruitment. J Innate Immun. (2023) 15(1):517–30. doi: 10.1159/000530249
60. Popova A, Kzhyshkowska J, Nurgazieva D, Goerdt S, Gratchev A. Pro- and anti-inflammatory control of m-CSF-mediated macrophage differentiation. Immunobiology. (2011) 216(1-2):164–72. doi: 10.1016/j.imbio.2010.06.003
61. Peranzoni E, Lemoine J, Vimeux L, Feuillet V, Barrin S, Kantari-Mimoun C, et al. Macrophages impede CD8 t cells from reaching tumor cells and limit the efficacy of anti-PD-1 treatment. Proc Natl Acad Sci USA. (2018) 115(17):E4041–50. doi: 10.1073/pnas.1720948115
62. Chen Y, Zhao Z, Chen Y, Lv Z, Ding X, Wang R, et al. An epithelial-to-mesenchymal transition-inducing potential of granulocyte macrophage colony-stimulating factor in colon cancer. Sci Rep. (2017) 7(1):8265. doi: 10.1038/s41598-017-08047-1
63. Shi X, Kaller M, Rokavec M, Kirchner T, Horst D, Hermeking H. Characterization of a p53/miR-34a/CSF1R/STAT3 feedback loop in colorectal cancer. Cell Mol Gastroenterol Hepatol. (2020) 10(2):391–418. doi: 10.1016/j.jcmgh.2020.04.002
64. Stanley ER, Chitu V. CSF-1 receptor signaling in myeloid cells. Cold Spring Harb Perspect Biol. (2014) 6(6):a021857. doi: 10.1101/cshperspect.a021857
65. Wang J, Wang Y, Chu Y, Li Z, Yu X, Huang Z, et al. Tumor-derived adenosine promotes macrophage proliferation in human hepatocellular carcinoma. J Hepatol. (2021) 74(3):627–37. doi: 10.1016/j.jhep.2020.10.021
66. Sierra-Filardi E, Vega MA, Sánchez-Mateos P, Corbí AL, Puig-Kröger A. Heme oxygenase-1 expression in m-CSF-polarized M2 macrophages contributes to LPS-induced IL-10 release. Immunobiology. (2010) 215(9-10):788–95. doi: 10.1016/j.imbio.2010.05.020
67. Murga-Zamalloa C, Rolland DCM, Polk A, Wolfe A, Dewar H, Chowdhury P, et al. Colony-stimulating factor 1 receptor (CSF1R) activates AKT/mTOR signaling and promotes t-cell lymphoma viability. Clin Cancer Res. (2020) 26(3):690–703. doi: 10.1158/1078-0432.CCR-19-1486
68. Wang S, Zhang Y, Meng W, Dong Y, Zhang S, Teng L, et al. The involvement of macrophage colony stimulating factor on protein hydrolysate injection mediated hematopoietic function improvement. Cells. (2021) 10(10):2776. doi: 10.3390/cells10102776
69. Xiang T, Cheng N, Huang B, Zhang X, Zeng P. Important oncogenic and immunogenic roles of SPP1 and CSF1 in hepatocellular carcinoma. Med Oncol. (2023) 40(6):158. doi: 10.1007/s12032-023-02024-7
70. Baghdadi M, Endo H, Takano A, Ishikawa K, Kameda Y, Wada H, et al. High co-expression of IL-34 and m-CSF correlates with tumor progression and poor survival in lung cancers. Sci Rep. (2018) 8(1):418. doi: 10.1038/s41598-017-18796-8
71. Groblewska M, Mroczko B, Wereszczyńska-Siemiatkowska U, Myśliwiec P, Kedra B, Szmitkowski M. Serum levels of granulocyte colony-stimulating factor (G-CSF) and macrophage colony-stimulating factor (M-CSF) in pancreatic cancer patients. Clin Chem Lab Med. (2007) 45(1):30–4. doi: 10.1515/CCLM.2007.025
72. Lubowicka E, Zbucka-Kretowska M, Sidorkiewicz I, Zajkowska M, Gacuta E, Puchnarewicz A, et al. Diagnostic power of cytokine m-CSF, metalloproteinase 2 (MMP-2) and tissue inhibitor-2 (TIMP-2) in cervical cancer patients based on ROC analysis. Pathol Oncol Res. (2020) 26(2):791–800. doi: 10.1007/s12253-019-00626-z
73. Richardsen E, Uglehus RD, Johnsen SH, Busund LT. Macrophage-colony stimulating factor (CSF1) predicts breast cancer progression and mortality. Anticancer Res. (2015) 35(2):865–74.
74. Kitko CL, Arora M, DeFilipp Z, Zaid MA, Di Stasi A, Radojcic V, et al. Axatilimab for chronic graft-Versus-Host disease after failure of at least two prior systemic therapies: Results of a phase I/II study. J Clin Oncol. (2023) 41(10):1864–75. doi: 10.1200/JCO.22.00958
75. Curry JM, Eubank TD, Roberts RD, Wang Y, Pore N, Maity A, et al. M-CSF signals through the MAPK/ERK pathway via Sp1 to induce VEGF production and induces angiogenesis in vivo. PloS One. (2008) 3(10):e3405. doi: 10.1371/journal.pone.0003405
76. Kubota Y, Takubo K, Shimizu T, Ohno H, Kishi K, Shibuya M, et al. M-CSF inhibition selectively targets pathological angiogenesis and lymphangiogenesis. J Exp Med. (2009) 206(5):1089–102. doi: 10.1084/jem.20081605
77. Ries CH, Cannarile MA, Hoves S, Benz J, Wartha K, Runza V, et al. Targeting tumor-associated macrophages with anti-CSF-1R antibody reveals a strategy for cancer therapy. Cancer Cell. (2014) 25(6):846–59. doi: 10.1016/j.ccr.2014.05.016
78. Fujiwara T, Yakoub MA, Chandler A, Christ AB, Yang G, Ouerfelli O, et al. CSF1/CSF1R signaling inhibitor pexidartinib (PLX3397) reprograms tumor-associated macrophages and stimulates t-cell infiltration in the sarcoma microenvironment. Mol Cancer Ther. (2021) 20(8):1388–99. doi: 10.1158/1535-7163.MCT-20-0591
Keywords: macrophage colony-stimulating factor, tumor progression, CSF-1R, immune modulation, dendritic cells, cancer therapy
Citation: Yi L, Gai Y, Chen Z, Tian K, Liu P, Liang H, Xu X, Peng Q and Luo X (2024) Macrophage colony-stimulating factor and its role in the tumor microenvironment: novel therapeutic avenues and mechanistic insights. Front. Oncol. 14:1358750. doi: 10.3389/fonc.2024.1358750
Received: 20 December 2023; Accepted: 12 March 2024;
Published: 04 April 2024.
Edited by:
Bostjan Markelc, Institute of Oncology Ljubljana, SloveniaReviewed by:
Persio Dello Sbarba, University of Florence, ItalyQiang Wang, Houston Methodist Research Institute, United States
Copyright © 2024 Yi, Gai, Chen, Tian, Liu, Liang, Xu, Peng and Luo. This is an open-access article distributed under the terms of the Creative Commons Attribution License (CC BY). The use, distribution or reproduction in other forums is permitted, provided the original author(s) and the copyright owner(s) are credited and that the original publication in this journal is cited, in accordance with accepted academic practice. No use, distribution or reproduction is permitted which does not comply with these terms.
*Correspondence: Xiaoqing Luo, NDEwMTU4NDNAcXEuY29t