- 1Department of Medical Immunology, School of Medicine, Tehran University of Medical Sciences, Tehran, Iran
- 2Department of Medical Biotechnology, School of Allied Medical Sciences, Iran University of Medical Sciences, Tehran, Iran
- 3Virology Department, Pasteur Institute of Iran, Tehran, Iran
- 4Department of Pharmaceutical Biotechnology, School of Pharmacy, Tehran University of Medical Sciences, Tehran, Iran
Introduction: Chimeric Antigen Receptor (CAR) T cell therapy has demonstrated remarkable success in treating hematological malignancies. However, its efficacy against solid tumors, including cervical cancer, remains a challenge. Hypoxia, a common feature of the tumor microenvironment, profoundly impacts CAR T cell function, emphasizing the need to explore strategies targeting hypoxia-inducible factor-1α (HIF-1α).
Methods: In this study, we evaluated the effects of the HIF-1α inhibitor PX-478 on mesoCAR T cell function through in-silico and in vitro experiments. We conducted comprehensive analyses of HIF-1α expression in cervical cancer patients and examined the impact of PX-478 on T cell proliferation, cytokine production, cytotoxicity, and exhaustion markers.
Results: Our in-silico analyses revealed high expression of HIF-1α in cervical cancer patients, correlating with poor prognosis. PX-478 effectively reduced HIF-1α levels in T and HeLa cells. While PX-478 exhibited dose-dependent inhibition of antigen-nonspecific T and mesoCAR T cell proliferation, it had minimal impact on antigen-specific mesoCAR T cell proliferation. Notably, PX-478 significantly impaired the cytotoxic function of mesoCAR T cells and induced terminally exhausted T cells.
Discussion: Our results underscore the significant potential and physiological relevance of the HIF-1α pathway in determining the fate and function of both T and CAR T cells. However, we recognize the imperative for further molecular investigations aimed at unraveling the intricate downstream targets associated with HIF-1α and its influence on antitumor immunity, particularly within the context of hypoxic tumors. These insights serve as a foundation for the careful development of combination therapies tailored to counter immunosuppressive pathways within hypoxic environments and fine-tune CAR T cell performance in the intricate tumor microenvironment.
1 Introduction
Cervical cancer (CC) ranks as the fourth most prevalent cancer among women (1). Despite the existence of various preventive and treatment modalities for CC, such as HPV screening, prophylactic vaccines, surgical interventions, radiotherapy, and chemotherapy, the global burden of the disease remains substantial (2). Therefore, there is an urgent need for new treatment strategies to improve the prognosis of patients with CC.
In recent years, the development of chimeric antigen receptor (CAR) T cell therapies for treating solid tumors has garnered significant interest (3). Currently, numerous clinical trials are underway to assess the effectiveness of CAR-T cell therapy in cervical cancer patients (NCT01583686) (NCT04556669) (NCT03356795). Mesothelin (MSLN) stands out as a crucial target antigen in the pursuit of novel immunotherapies for solid tumors (4). Clinical trials involving anti-MSLN CAR T cells have demonstrated commendable safety profiles but limited efficacy (5). The tumor microenvironment (TME) is widely recognized as a major obstacle, as it impedes T cell survival, proliferation, and cytotoxicity, thereby limiting the application of CAR T cell therapies in the clinical management of solid tumors (6). A common feature of the TME is hypoxia, characterized by inadequate oxygen supply (less than 2% O2) due to heightened metabolic demands and inefficient vasculature, in stark contrast to healthy tissues with oxygen levels of 5%–10% (7). Hypoxia is clinically associated with a poor prognosis and resistance to chemotherapy and radiotherapy (8–10) and it significantly compromises the fitness and efficacy of CAR T cells (11).
At the heart of the cellular response to hypoxia is hypoxia-inducible factor-1 (HIF-1), a master transcription factor that orchestrates the regulation of numerous downstream targets (12). HIF-1 exists as a heterodimeric transcription factor composed of the oxygen-sensitive HIF-1α subunit and the constitutively expressed HIF-1β (ARNT) subunit (13). Under normal oxygen conditions (normoxia), HIF-1α is rapidly degraded through ubiquitin-mediated pathways, primarily governed by proline hydroxylation (14). However, during hypoxia, the inhibition of HIF-1α hydroxylases interferes with VHL-HIF binding, leading to the stabilization of HIF-1α protein and enabling HIF-1 dimerization, which, in turn, activates its transcriptional function (14).
Numerous studies have unveiled the connection between HIF-1α overexpression and poorer prognosis in cervical cancer patients (15–18). Currently, several HIF-1α inhibitors are in development for various cancer types, exhibiting promising antitumor efficacy and manageable toxicity profiles (19–21). Nonetheless, it remains uncertain whether agents that inhibit HIF-1α can enhance the response to CAR-T cell therapy. To elucidate these questions, we explore the impact of the selective HIF-1α inhibitor PX-478 on the antitumoral function of second-generation mesoCAR T cells.
2 Material and methods
2.1 Bioinformatics analysis
Gene expression analysis was conducted using the GEPIA2 database (http://gepia2.cancer-pku.cn), which utilizes data from the TCGA and GTEx databases (22). To compare HIF-1α gene expression levels between squamous cell carcinoma of the cervix (SESC) and corresponding normal tissues, the “box plot” function for expression analysis was employed. The following statistical parameters were utilized: a Log2FC (Logarithm to the base 2-fold change) cutoff value of 1, and a p-value cutoff value of 0.01. Additionally, GEPIA2 was employed to assess the overall survival of SESC patients using the “Survival Analysis” module, with the Group Cutoff set to the Quartile. Hazard ratios (HRs) with 95% confidence intervals (CIs) and log-rank P-values were computed to ascertain survival outcomes. The representative immunohistochemistry image of HIF-1α expression was obtained from the Human Protein Atlas (HPA) database (23).
2.2 Cell lines
HEK293T, Jurkat, Hela, and PANC-1 cell lines were acquired from the Iranian Biological Resource Center (IBRC). HEK293T, Hela, and PANC-1 cells were maintained in D10 media, comprising DMEM (Gibco, Life Technologies), 10% fetal bovine serum (FBS), and 1% penicillin/streptomycin (Gibco, Life Technologies). Jurkat cells were cultured in R10 media, containing RPMI-1640 (Gibco, Life Technologies) supplemented with 10% FBS, 25 mM HEPES (Sigma Aldrich), 2 mM glutamine (Gibco), and 1% penicillin/streptomycin. Flow cytometry was used to validate mesothelin expression in the relevant cell lines prior to experiments. Regular mycoplasma contamination checks were conducted on all cell lines.
2.3 Primary human cells
Peripheral blood mononuclear cells (PBMCs) were isolated from fresh blood using standard methods with Histopaque®-1077 (Sigma Aldrich). Primary human T cells were negatively selected with immunomagnetic beads (Pan T Cell Isolation Kit, Miltenyi Biotec) and stored at -80°C. T cells were cultured in TM10 media, composed of TexMACS™ Medium (Miltenyi Biotec), supplemented with 10% human serum and 100 IU/mL premium-grade rhIL-2 (Miltenyi Biotec). Blood samples were obtained from healthy volunteers under approval from the Research Ethics Committees of the School of Medicine, Tehran University of Medical Sciences [IR.TUMS.BLC.1402.015].
2.4 Lentiviral vector production
Lentiviral vectors were produced following previously established protocols (24). HEK293T cells were transfected with lentiviral CAR and packaging plasmids using the calcium phosphate method. Lentiviral supernatants were collected at 48- and 72-hour time points post-transfection and then concentrated through high-speed centrifugation. The concentrated lentivirus batches were resuspended in cold RPMI-1640 media and stored at -80°C. Titration of lentiviral vectors was performed using Jurkat cells.
2.5 Lentiviral transduction
mesoCAR T cells were generated as per previous descriptions (25). Briefly, 1 × 106 T cells were seeded in each well of 12-well tissue culture plates and activated using Dynabeads™ Human T-Expander CD3/CD28 (Gibco, Life Technologies, 11161D) at a 1:1 ratio in TM10 media. Activated T cells were infected with lentiviral vectors supplemented with 8 mg/mL Polybrene (Santacruz) 24 hours after activation. Centrifugation at 850g for 1 hour at 32°C was employed to enhance transduction efficiency. Two hours later, 2 mL/well of TM10 media was added to the transduced T cells. At day 4 post-transduction, Dynabeads™ were removed from transduced T cells using a DynaMag™ magnet, and GFP expression, indicative of mesoCAR expression, was assessed via flow cytometry.
2.6 PX-478 dose-response
PX-478 (MedChemExpress, USA) was dissolved in Dimethyl sulfoxide (DMSO). To assess the impact of PX-478 on T cell proliferation, 2 × 105 CFSE-labeled T cells were seeded in 96-well tissue culture plates and exposed to varying concentrations of PX-478. Human T Cell-Expander Dynabeads™ CD3/CD28 (Gibco, Life Technologies, 11161D) were used at a 1:1 ratio in TM10 medium to activate T cells. After three days, T cells were harvested, and their proliferation was evaluated via flow cytometry.
2.7 Protein extraction and western blotting
Adherent cells were washed twice with PBS, scraped, and transferred to 1.5 ml tubes. T cells were also harvested and washed twice with PBS. After centrifugation, cells were lysed using RIPA buffer containing 1mM PMSF at a ratio of 60 μl per 106 cells. Proteins were separated by 10% SDS-PAGE under reducing conditions and subsequently transferred to PVDF membrane. The membrane was then blocked for 1 hour using a 5% BSA blocking reagent in Tris-Buffered Saline (pH=7.5) containing 0.05% Tween-20 (v/v) (TBST) and incubated with Rabbit anti-HIF-1α antibody diluted at 1:2,000 (Novus Biologicals NB100-449, Centennial, Colorado, USA) or Rabbit anti-β-actin antibody (Sigma-Aldrich) diluted at 1:2,000, overnight at 4˚C. The blots were further incubated with anti-Rabbit horseradish peroxidase-conjugated antibodies for 1 hour. Protein bands were detected using ECL method and X-ray film was used for visualization. Quantification was conducted using image J (imagej.org).
2.8 Hypoxia assay
Culture plates were incubated either under normoxic conditions (37°C in humidified air, 5% CO2) or under hypoxic conditions (1% O2, 5% CO2, 94% N2). Hypoxia was induced using a hypoxia incubator chamber (StemCell Technologies, Inc.) purged at 25L/min for 4 minutes with a gas mixture containing 1% O2, 5% CO2, and 94% nitrogen as a balance before sealing the chamber.
2.9 In vitro cytotoxicity assay
For in vitro cytotoxicity assays, 1x104 target cells were seeded in 96-well U‐bottomed tissue culture plates and pretreated with 25µM PX-478 for 24 hours under both hypoxic and normoxic conditions. Transduced or non-transduced T cells were then added to the wells at effector-to-target ratios of 1:1, 10:1, and 20:1 for 4 hours in TM10 media, with a final volume of 200 ml/well. To distinguish between effector and target cells, effector cells were stained with CFSE. Prior to flow cytometry analysis, 7-AAD (Miltenyi Biotec) was added to stain dead cells. Flow cytometry analysis utilized CFSE and 7-AAD staining to differentiate T cells from dead tumor cells. The frequency of lysed target cells (CFSE-/7-AAD+ cells) was calculated by subtracting the percentage of spontaneous lysis of target cells from the percentage of lysis of target cells in coculture with mesoCAR T cells. Normalized lysis of target cells (Specific lysis) was reported based on mesothelin expression on target cells.
2.10 In vitro proliferation and cytokine production assays
Target cells were treated with 50 mg/ml of mitomycin C (Sigma Aldrich) for 30 minutes at 37°C and subsequently washed. 2x105 target cells were seeded in 48-well tissue culture plates and pretreated with 25µM PX-478 for 24 hours under both hypoxic and normoxic conditions before removal of the media. For cell proliferation analysis, mesoCAR T cells and untransduced T cells were stained with 5 mM CFSE at room temperature for 8 minutes. An equal amount of FBS was added to halt the reaction. After washing three times with complete RPMI 1640 medium, CFSE-labeled cells (0.2 × 106/well) were cocultured with either target cells or media, in the absence of exogenous IL‐2, in 48‐well plates, with a final volume of 800 µl/well. After 24 hours, 200 µl of the supernatants were harvested and stored at −80°C. The subsequent cytokine analysis was carried out by enzyme-linked immunosorbent assay (ELISA) to quantify IFN-γ and IL-2. After 72 hours, cells were stained with PerCP-conjugated anti-human CD3 antibody (Clone: HIT3a, BioLegend), and CFSE dilution of CD3+ cells was determined by flow cytometry, as an indicator of proliferation.
2.11 Flow cytometric analysis
The purity of isolated T cells was confirmed using APC-conjugated anti-human CD3 (Clone: UCHT1, BioLegend). PE-conjugated anti-human mesothelin (Clone: #420411, R&D Systems) was used to detect mesothelin expression. FITC-conjugated anti-human CD3 (Clone: HIT3a, BioLegend), PE-conjugated anti-human CD279 (PD-1) (Clone: EH12.2H7, BioLegend), and APC-conjugated anti-human CD366 (Tim-3) (Clone: F38-2E2, BioLegend) antibodies were used to measure the expression of exhaustion markers. For proliferation assays, cells were loaded with CellTrace™ CFSE (Life Technologies, #C34554) according to manufacturer’s instructions, and T cells were detected using PerCP-conjugated anti-human CD3 antibody (Clone: HIT3a, BioLegend). Data were collected using a BD FACSCalibur (BD Biosciences) and analyzed with FlowJo software (v10.6). All assays were performed in duplicate and repeated two to three times.
2.12 Statistical analysis
Normality tests and one-way/two-way analysis of variance (ANOVA) were performed using GraphPad Prism software (v9) to identify differences among various treatment groups. p-values below 0.05 were considered statistically significant.
3 Results
3.1 Association of HIF-1α overexpression with adverse prognosis in cervical cancer patients
To assess the significance of HIF-1α expression in cervical cancer, we utilized the GEPIA2 database to visualize the mRNA expression levels of HIF-1α in cervical cancer. The analysis involved 13 normal tissue samples and 306 samples from cervical cancer patients. Our data unequivocally demonstrate an upregulation of HIF-1α in cervical cancer patients (Figure 1A). Complementing this, the immunohistochemistry image of HIF-1α protein levels in tissue samples from the Human Protein Atlas (HPA) dataset confirmed similar findings (Figure 1B).
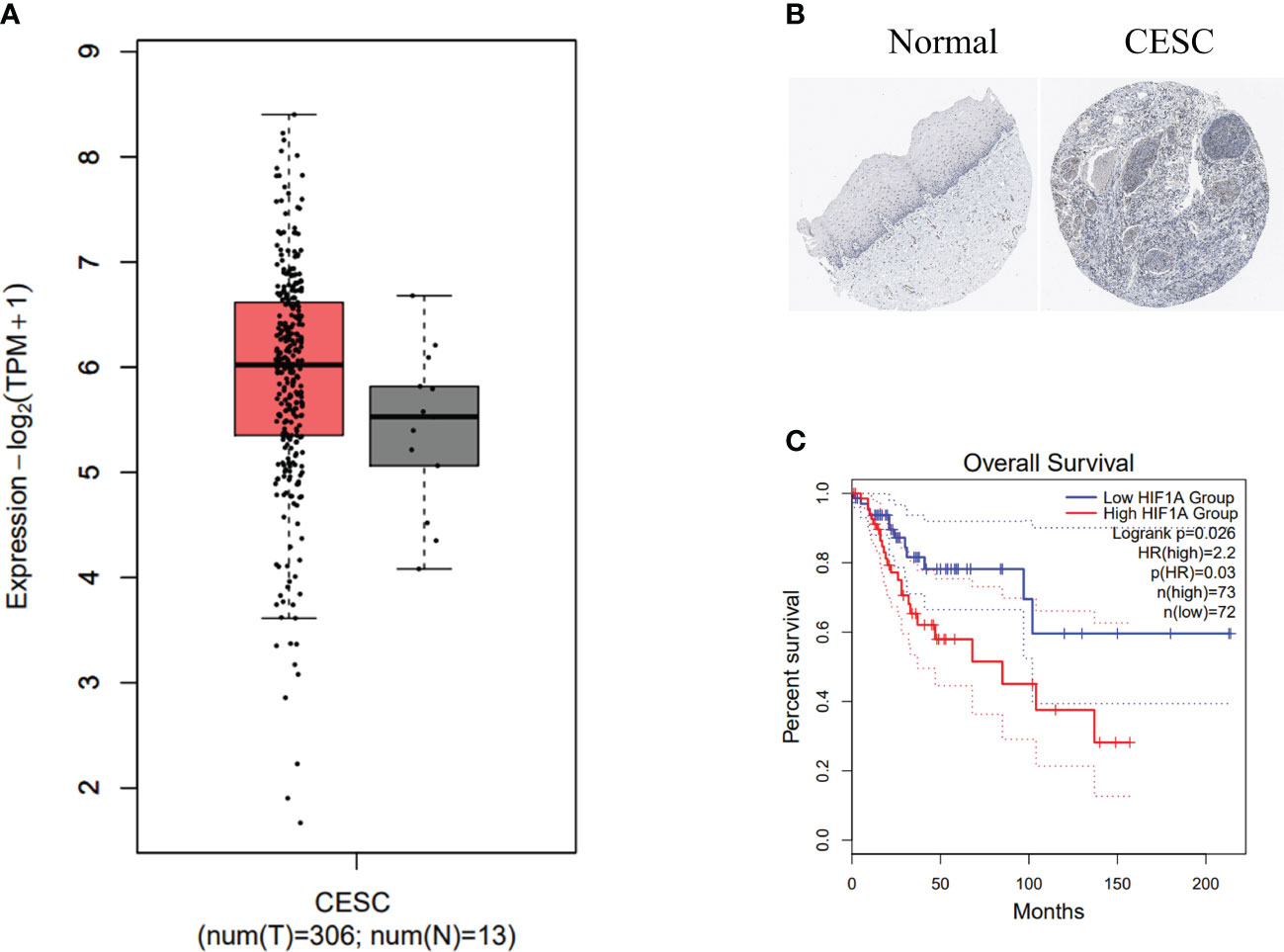
Figure 1 HIF-1α overexpression is associated with poor prognosis in cervical cancer patients. (A) HIF1A expression levels in biopsies from cervical cancer (CC) patients (highlighted in red) and corresponding normal tissue samples (depicted in grey) were analyzed using data from the TCGA dataset. The data was log2 transformed (TPM+1). (B) Representative images from the Human Protein Atlas database illustrate HIF-1α expression in normal (left) and CC (right) tissue samples. (C) Kaplan-Meier survival curves present the overall survival time of CC patients categorized into high and low HIF-1α expression groups. Dotted lines indicate a 95% confidence interval.
We further conducted a Kaplan-Meier survival analysis using GEPIA2 to investigate the prognostic value of HIF-1α. Our results reveal a statistically significant association between high expression of the HIF1A gene and shorter overall survival in cervical cancer patients (Figure 1C).
3.2 PX-478 reduces HIF-1α protein levels under hypoxic conditions
PX-478, previously identified as a compound that decreases cellular HIF-1α levels, was evaluated in our study. To validate the increase of HIF-1α under hypoxic conditions and assess the inhibitory effect of PX-478, HeLa and T cells were cultured under normoxic and hypoxic (1% O2) conditions while exposed to varying doses of PX-478 for 24 hours. Western blot analysis confirmed the efficient stabilization of HIF1α in hypoxic conditions and demonstrated that PX-478 inhibits the hypoxia-induced increase in HIF-1α protein levels in a dose-dependent manner (Figures 2A-D).
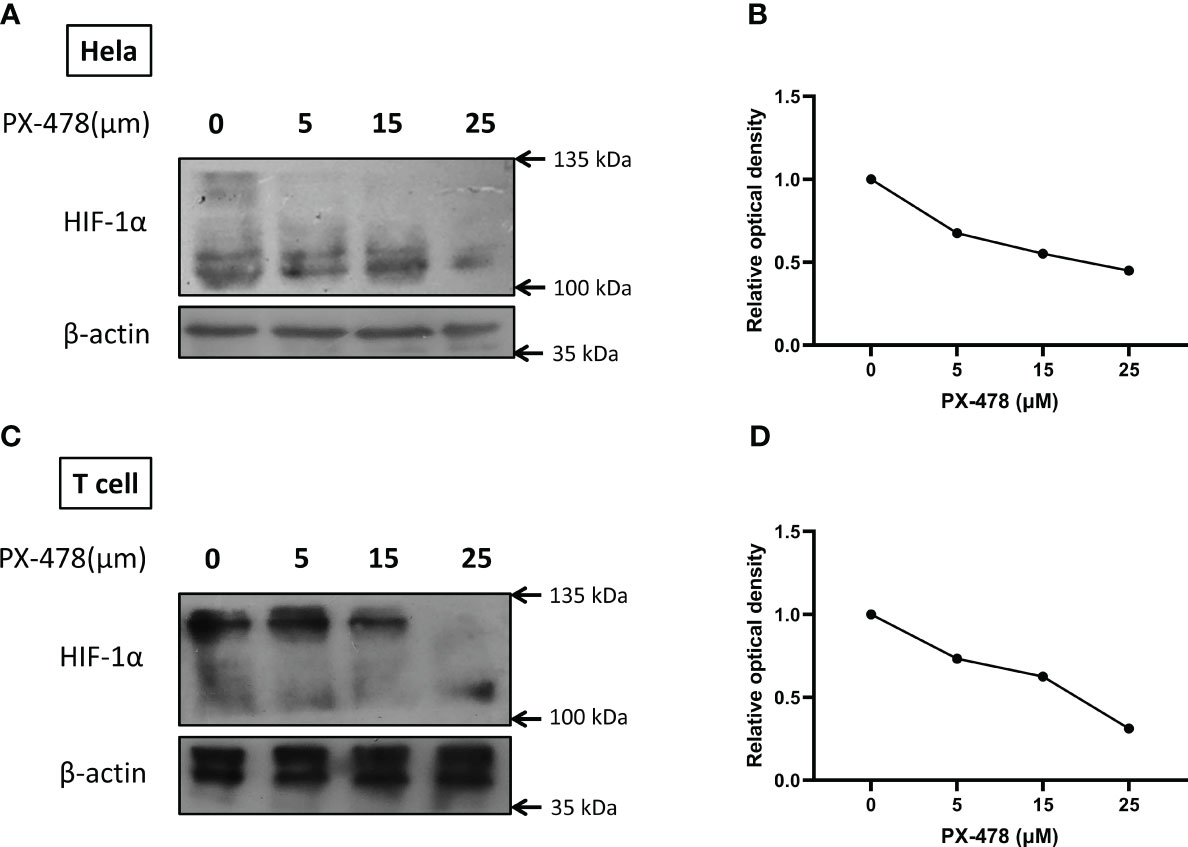
Figure 2 PX-478 decreases HIF-1α protein levels in a dose-dependent manner. (A, C) Hela and T cells were exposed to varying concentrations of PX-478 for 24 hours under hypoxic conditions, and the levels of HIF-1α protein were assessed through Western blot analysis. (B, D) PX-478 demonstrates a dose-dependent inhibition of HIF-1α protein expression. Densitometric quantification of the blots was performed relative to β-actin as a reference protein. Each experiment was repeated two to three times.
3.3 Effective antitumor activity of mesoCAR T cells against cervical cancer cells
We generated and characterized second-generation mesoCAR T cells, as described previously (25). Briefly, human CD3+ T cells were efficiently infected with lentiviral particles encoding the second-generation mesoCAR transgene (Figure 3A). We subsequently assessed the in vitro antitumor capacity of these cells. We used PANC-1 and HeLa cells, which represent mesothelin-negative and positive tumor cells respectively (Figures 3B, C). T cells expressing the mesoCAR transgene exhibited specific cytotoxicity against mesothelin-positive HeLa cells, while no cytotoxicity was observed against mesothelin-negative PANC-1 cells (Figure 3D). To test the effectiveness of mesoCAR T cells against HeLa cells, we investigated their proliferation and their capacity to produce IL-2 and IFN-γ cytokines in vitro. mesoCAR T cells demonstrated a high mesothelin-specific proliferation rate comparable to untransduced T cells after being stimulated with Hela and PANC-1 cells (Figure 3E). After CAR T cell stimulation with HeLa, mesoCAR T cells showed high mesothelin-specific proliferation rates and produced large amounts of IFN-γ and IL-2, comparable to untransduced T cells (Figures 3E-G). No IL-2 and IFN-γ secretion was detected in cultures of T cells alone, tumor cells alone, or when irrelevant target cells like PANC-1 were involved (Figures 3F, G).
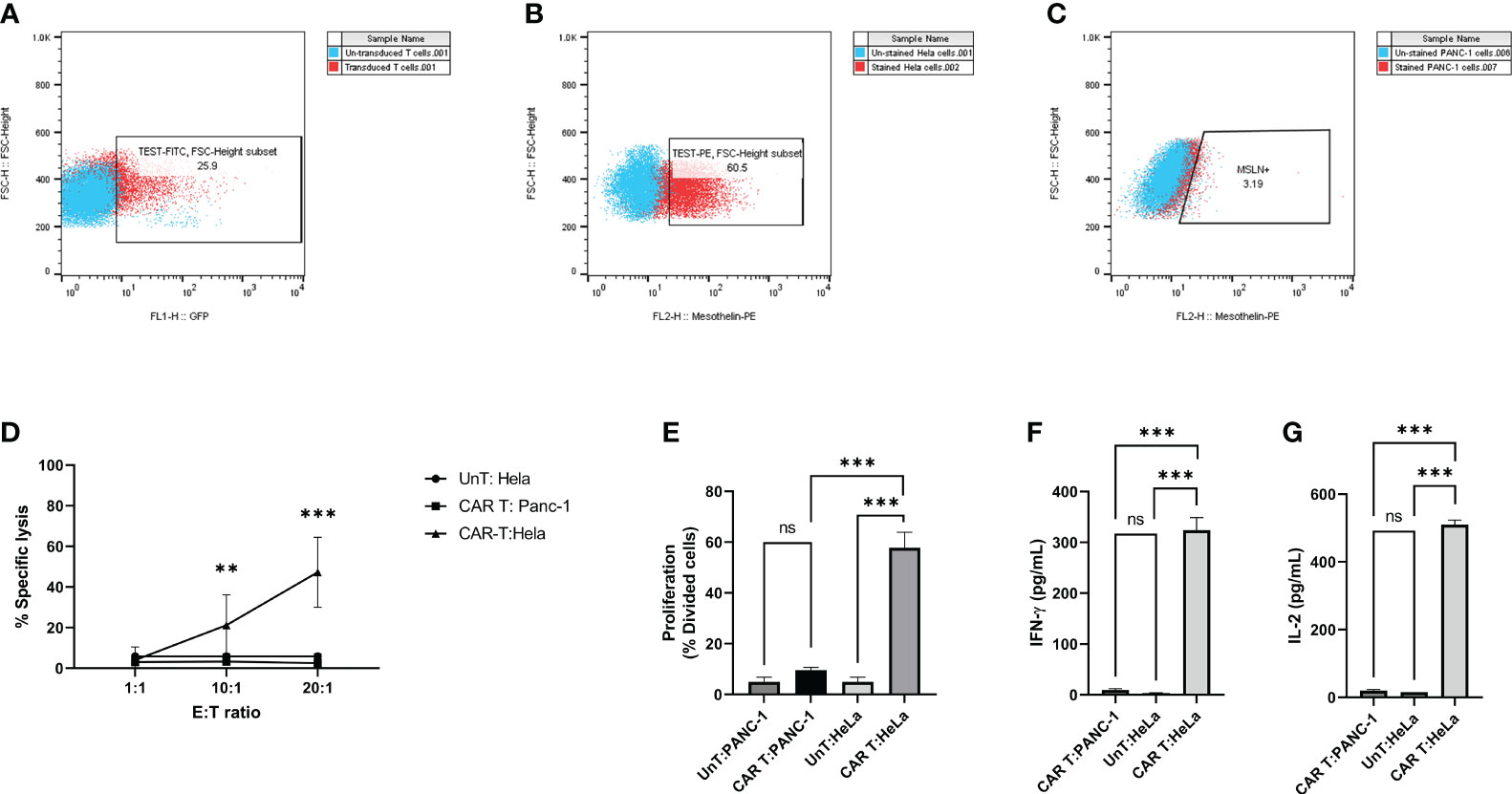
Figure 3 Antigen specificity of mesoCAR T cells against HeLa cells. (A) Assessment of chimeric antigen receptor (CAR) expression on mesoCAR T cells. (B, C) Representative dot plots illustrating mesothelin expression on HeLa and PANC-1 cells, respectively. (D) MesoCAR T cells demonstrate specific cytotoxicity against target cells at varying effector-to-target ratios. (E) Proliferation of mesoCAR T cells in response to target cells. (F, G) Production of IFN-γ and IL-2 by mesoCAR T cells in coculture with target cells. Statistical analysis was conducted using ordinary one-way ANOVA (E–G) and two-way ANOVA (D), followed by Tukey’s multiple comparison test. Significance denoted by ***(P < 0.001). Data are presented as mean ± SD. ns, non significant
3.4 Impact of PX-478 on mesoCAR T cell proliferation
The efficacy of CAR T cell immunotherapies against solid tumors hinges on T cell proliferation, persistence, and accumulation (26). To investigate the influence of PX-478 on mesoCAR T cell proliferation, we performed a series of experiments. Initially, T cells activated with anti-CD3/CD28-coated beads were exposed to varying concentrations of orally available PX-478, revealing that PX-478 can dose-dependently reduce antigen-nonspecific T cell proliferation (Figure 4A), while concurrently maintaining T cell viability unchanged (Figure 4B).
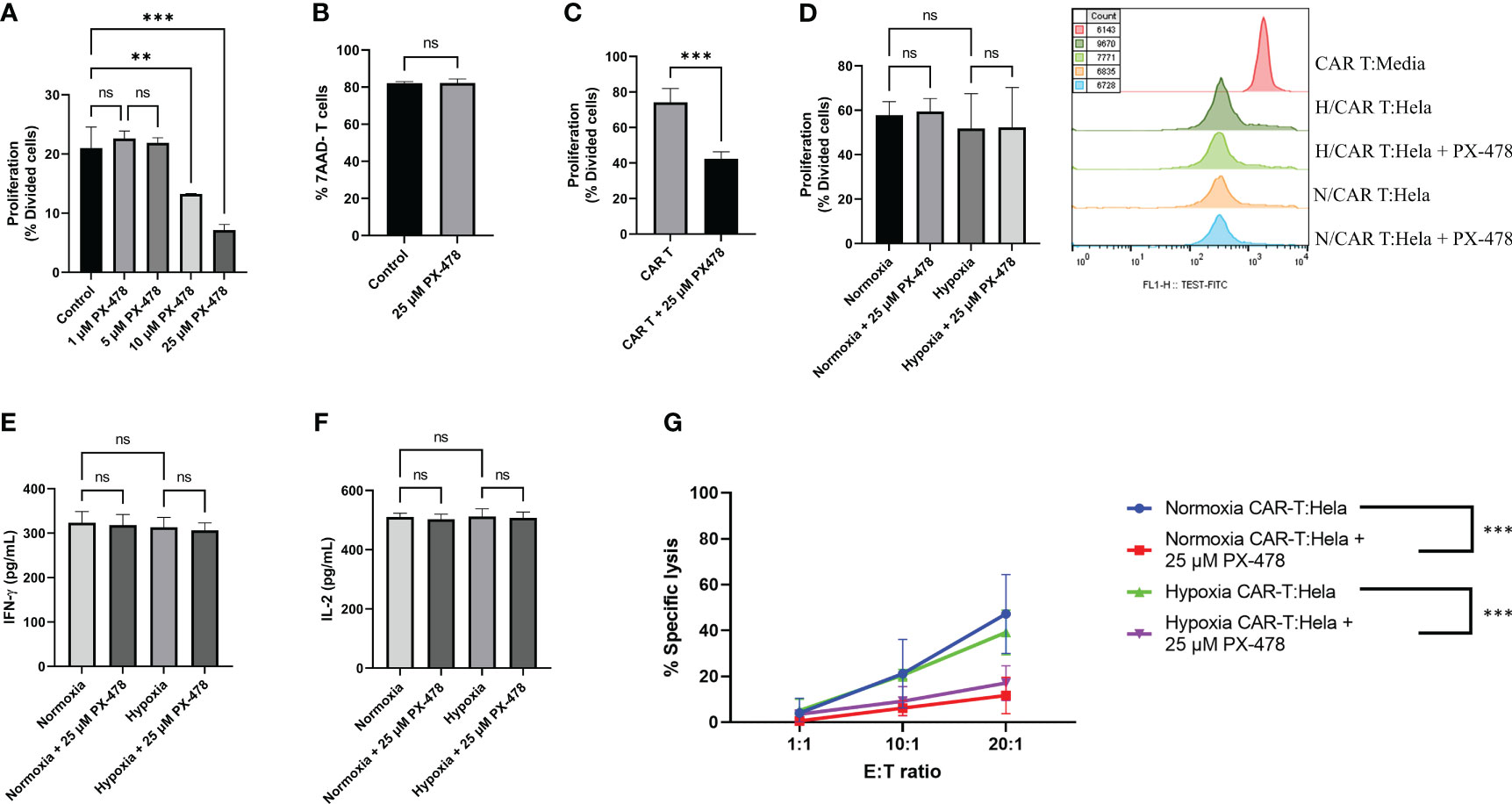
Figure 4 The effects of HIF-1α inhibitor PX-478 on the antitumor function of mesoCAR T cells. (A) PX-478 significantly inhibits antigen-nonspecific proliferation of T cells. (B) Viability of T cells remained unchanged in response to PX-478. (C) PX-478 significantly inhibits the IL-2-induced antigen-nonspecific proliferation of mesoCAR T cells. (D) Antigen-specific proliferative capacity and representative cell count of mesoCAR T cells over a three-day coculture with pre-treated Hela cells. (E, F) Production of IFN-γ and IL-2 by mesoCAR T cells in coculture with pre-treated Hela cells. (G) Overlaid plot demonstrating mesoCAR T cell cytotoxicity against Hela cells in the presence of PX-478. Statistical analysis was performed using ordinary one-way ANOVA (A, C-F), Student’s t-test (B), two-way ANOVA (G), and Tukey multiple comparison test. **P < 0.01; ***P < 0.001. Data are presented as mean ± SD. ns, non significant
Consistent with the data obtained from T cells, we observed that the antigen-nonspecific proliferation of mesoCAR T cells in an IL-2-containing medium was also impeded by PX-478 (Figure 4C). Given the observed direct inhibitory effect of PX-478 on T and mesoCAR T cell proliferation, we conducted experiments in which tumor target cells were pretreated with PX-478 for 24 hours under both hypoxia and normoxia conditions. After supernatant removal, mesoCAR T cells were introduced. Interestingly, pretreatment of tumor cells with PX-478 did not significantly impact mesoCAR cell proliferation (Figure 4D). This result was supported by the analysis of cytokine production, where no significant changes in the levels of IL-2 and IFN-γ were observed (Figures 4E, F). Collectively, our findings indicate that PX-478 directly inhibits antigen-nonspecific T and mesoCAR T cell proliferation, while pretreatment of tumor cells with PX-478 has no influence on mesoCAR T cell proliferation and cytokine production.
3.5 PX-478 impairs mesoCAR T cell cytotoxic function
Having established the effect of PX-478 on mesoCAR T cell proliferation, we sought to examine its impact on the cytotoxicity of these cells against tumor target cells. HeLa cervical cancer cells were cultured, pre-exposed to 25µM PX-478 for 24 hours, and then co-incubated with mesoCAR T cells under normoxic and hypoxic (1% O2) conditions. mesoCAR T cells demonstrated effective killing of HeLa cells under both hypoxic and normoxic conditions (Figure 4G). However, PX-478 significantly reduced the cytotoxicity of mesoCAR T cells under both hypoxic and normoxic conditions (Figure 4G).
In an effort to understand the underlying reason for the impairment of mesoCAR T cell cytotoxic function, we considered the possibility that these cells become exhausted in the presence of PX-478. Exhausted T cells can be categorized into progenitor-exhausted T cells (Tpex) and terminally exhausted T cells (Ttex) based on their function and phenotype (24, 27, 28). Tpex cells express PD-1 but not TIM3, retain stem-like characteristics, and remain polyfunctional. In contrast, Ttex cells express both PD-1 and TIM3 at high levels, have a limited lifespan, and cannot effectively suppress tumor growth (29). Immune checkpoint blockade can rejuvenate Tpex but not Ttex cells (30). To explore this, cells from our cocultures were analyzed via flow cytometry for the expression of PD-1 and TIM3. Our results revealed that pretreatment of tumor cells with PX-478 did not significantly alter the expression of PD-1 but led to an increased expression of TIM3 (Supplementary Figure S1A, B). Furthermore, the abundance of Ttex cells (PD-1+TIM3+) increased, while the abundance of Tpex cells (PD-1+TIM3-) decreased under both normoxic and hypoxic conditions (Supplementary Figure S1C).
4 Discussion
In this study, we delved into the impact of the HIF-1α inhibitor PX-478 on the antitumoral function of mesoCAR T cells. Our in-silico analysis compellingly indicated that the overexpression of HIF-1α in CC patients is strongly associated with an unfavorable prognosis. It is well-established that HIF-1α becomes stabilized within the hypoxic core of rapidly growing, poorly vascularized solid tumors (31). This stabilization of HIFs plays a pivotal role in promoting tumor survival and metastasis by orchestrating changes in glycolysis, nutrient uptake, waste disposal, angiogenesis, apoptosis, and cell migration (32–35).
To target HIF-1α, we employed PX-478, an orally available small molecule known to interfere with HIF-1α transcription and translation, thus leading to reduced deubiquitination of HIF-1α (36). Our Western blot analyses provided clear evidence that PX-478 effectively inhibited HIF-1α in a dose-dependent manner in both T and HeLa cells. Our investigation into the effects of PX-478 on T cell proliferation revealed a dose-dependent suppression. Notably, previous studies have shown that T cell receptor activation stabilizes HIF-1α in T lymphocytes (37), thereby facilitating a metabolic shift towards glycolysis to support T cell proliferation and effector functions (38, 39). Furthermore, PI3K/mTOR activity downstream of TCR and CD28 signaling induces HIF-1α expression by promoting transcription of two HIF-1α mRNA splice isoforms and driving increased protein translation in human and mouse T cells (37, 40). Additionally, PX-478 can prevent the G2/M transition by affecting proteins related to the G2 phase of the cell cycle, such as cyclin B1, thereby inhibiting cell proliferation (41).
Considering the inhibitory effects of PX-478 on T cell proliferation, we explored the potential of pre-treatment with PX-478 prior to CAR-T cell therapy. We pre-treated tumor cells with PX-478 and meticulously evaluated its influence on the proliferation and cytokine production of mesoCAR T cells. Proliferation analyses provided no significant differences in mesoCAR T cell proliferation between hypoxic and normoxic conditions, as well as PX-478-treated and untreated groups. Likewise, our analysis of IFN-γ and IL-2 cytokine production showed no significant differences, thereby confirming the results on proliferation. We further assessed how PX-478 affected the cytotoxicity of mesoCAR T cells. Consistent with previous research, no significant difference was observed in the cytotoxicity of mesoCAR T cells under hypoxic and normoxic conditions (11, 42). However, PX-478 significantly impeded the cytotoxic function of mesoCAR T cells. Earlier studies have indicated that HIF-1α is vital for the cytotoxic function of CAR T cells. For instance, Palazon et al. demonstrated that the deletion of HIF-1α resulted in reduced expression of several proteins critical for tumor rejection by cytotoxic T lymphocytes (43). The genetic ablation of HIF-1α led to decreased production of effector cytokines such as IFN-γ and TNF-α, along with cytolytic molecules like granzyme B (43). HIF-1α hydroxylation at proline residues in normoxia leads to VHL-mediated proteasomal degradation (44). It has been demonstrated that VHL-deficient TILs accumulate and survive in tumors in an HIF-dependent manner, retaining polyfunctionality and cytolytic capacity (45), highlighting the essential role of HIF-1α in T cells’ antitumor function.
HIFs have also been found to play a pivotal role in regulating T cell exhaustion in the context of infections and malignancies (43, 45–47). Consequently, we investigated the impact of PX-478 pre-treatment of tumor cells on the expression pattern of exhaustion markers on mesoCAR T cells. Our findings indicated an increase in the percentage of TIM3+ T cells and Ttex cells under hypoxia conditions and in PX-478 treated groups. This aligns with previous studies that have shown that the expression of TIM-3, a marker of terminally exhausted T cells, is substantially up-regulated under hypoxic conditions (43, 48, 49). The decrease in cytotoxicity of mesoCAR T cells in the presence of PX-478 may be explained by the increase in Ttex cells, which have limited antitumor activity.
Nevertheless, it is essential to acknowledge the limitations of our study. PX-478 may not be entirely specific for reducing HIF-1α levels; prior research suggests that it may affect other intracellular factors as well (50). In order to exclude off-target effects of PX-478, more specific approaches targeting HIF-1α, such as genetic knockdown or using alternative pharmacological inhibitors, would provide clearer evidence for the role of HIF-1α signaling in regulating antitumor function of mesoCAR T cells. Additionally, selectively rescuing HIF-1α protein levels in the presence of PX-478 using stabilizing agents that do not broadly impact other cell mediators would further elucidate the specific contribution of HIF-1α to the observed phenotypes. Additionally, HIF-1α is physiologically activated by hypoxia and plays a critical role in regulating the expression of several genes, including GLUT1, LDHA, and VEGF (12). Consequently, some downstream genes may exert either a positive or negative influence on the antitumoral function of CAR T cells. Further investigations may uncover specific downstream targets of HIF-1α that modulate the antitumor function of CAR T cells in the tumor microenvironment. Considering previous studies demonstrating HIF-1α can directly regulate expression of T cell activation-related genes such as CD69 in tumor-infiltrating lymphocytes (51), it would be informative to examine how PX-478 impacts levels of canonical activation markers on mesoCAR T cells.
PX-478 has previously demonstrated antitumor efficacy across several human tumor models (41, 52). However, our present investigation exclusively focuses on the in vitro evaluation of the potential combination therapy involving PX-478 and mesoCAR T cells. Our data demonstrate PX-478 partially impairs mesoCAR T cell function, but do not reflect the compound’s direct effects on cervical tumor cells or overall therapeutic potential in vivo. As we only assessed a subset of responses using an isolated cell system, our results should not be interpreted as evidence for negative impacts of HIF-1α inhibitors in cervical cancer more broadly. It is worth noting that our study was limited to in vitro assessments using cell lines and CAR T cell cocultures, while prior research has suggested that PX-478 may inhibit tumor angiogenesis, resulting in antitumor effects in vivo. Therefore, future in vivo studies are essential to provide a more comprehensive understanding of the function of PX-478 in a natural tumor microenvironment.
5 Conclusions
In summary, our study demonstrates the significant impact of HIF-1α inhibition using the PX-478 inhibitor on mesoCAR T cell function within the cervical cancer microenvironment. The inhibition of HIF-1α markedly impairs the cytotoxicity of mesoCAR T cells while minimally affecting their proliferation and cytokine production. Our findings underscore the clinical relevance of HIF-1α overexpression in cervical cancer patients and highlight the potential challenges in targeting HIF-1α for enhancing CAR T cell therapy efficacy. Despite limitations in specificity and the need for further in vivo validation, our study provides crucial insights into the interplay between HIF-1α signaling and CAR T cell function, serving as a foundational framework for the development of combination therapies aimed at optimizing CAR T cell performance in solid tumors like cervical cancer.
Data availability statement
The original contributions presented in the study are included in the article/Supplementary Material. Further inquiries can be directed to the corresponding authors.
Ethics statement
This study involves human cell lines and was approved by Research Ethics Committees of Biosafety & Laboratory, Tehran University of Medical Sciences [IR.TUMS.BLC.1402.015]. Informed consent was obtained from all individual participants included in the study.
Author contributions
AP: Conceptualization, Data curation, Formal Analysis, Investigation, Visualization, Writing – original draft. BA: Conceptualization, Methodology, Project administration, Writing – original draft, Writing – review & editing. TS: Methodology, Writing – original draft. ZS: Investigation, Writing – original draft. MH: Investigation, Writing – original draft. JH: Supervision, Writing – review & editing. HM: Conceptualization, Funding acquisition, Supervision, Writing – review & editing.
Funding
The author(s) declare financial support was received for the research, authorship, and/or publication of this article. Research reported in this publication was supported by grants from Tehran University of Medical Sciences (grants nos. 50756 and 50760, awarded to HM), and the National Institute for Medical Research Development (NIMAD) of Iran (grant no. 942554, awarded to JH).
Conflict of interest
The authors declare that the research was conducted in the absence of any commercial or financial relationships that could be construed as a potential conflict of interest.
The author(s) declared that they were an editorial board member of Frontiers, at the time of submission. This had no impact on the peer review process and the final decision.
Publisher’s note
All claims expressed in this article are solely those of the authors and do not necessarily represent those of their affiliated organizations, or those of the publisher, the editors and the reviewers. Any product that may be evaluated in this article, or claim that may be made by its manufacturer, is not guaranteed or endorsed by the publisher.
Supplementary material
The Supplementary Material for this article can be found online at: https://www.frontiersin.org/articles/10.3389/fonc.2024.1357801/full#supplementary-material
Supplementary Figure 1 | The effects of HIF-1α inhibitor PX-478 on the expression pattern of exhaustion markers. (A) The percentage of PD1+ T cells. (B) The frequency of Tpex and Ttex cells. (C) The percentage of TIM3+ T cells. Statistical analysis was performed using ordinary one-way ANOVA (A-C), and Tukey multiple comparison test. *P < 0.05; **P < 0.01; ***P < 0.001. Data are presented as mean ± SD.
Abbreviations
CAR T cells, Chimeric antigen receptors; HIF-1α, Hypoxia-inducible factor-1α; IFN-γ, Interferon-gamma; IL-2, Interleukin 2; mesoCAR T, Fully human anti-mesothelin CAR T cells; TME, Tumor microenvironment; CC, Cervical cancer.
References
1. Sung H, Ferlay J, Siegel RL, Laversanne M, Soerjomataram I, Jemal A, et al. Global cancer statistics 2020: globocan estimates of incidence and mortality worldwide for 36 cancers in 185 countries. CA Cancer J Clin. (2021) 71:209–49. doi: 10.3322/caac.21660
2. Tang Y, Zhang AX, Chen G, Wu Y, Gu W. Prognostic and therapeutic tils of cervical cancer—Current advances and future perspectives. Mol Therapy-Oncolytics. (2021) 22:410–30. doi: 10.1016/j.omto.2021.07.006
3. Johnson LA, June CH. Driving gene-engineered T cell immunotherapy of cancer. Cell Res. (2017) 27:38–58. doi: 10.1038/cr.2016.154
4. Lamberts LE, de Groot DJ, Bense RD, de Vries EG, Fehrmann RS. Functional genomic mrna profiling of a large cancer data base demonstrates mesothelin overexpression in a broad range of tumor types. Oncotarget. (2015) 6:28164–72. doi: 10.18632/oncotarget.4461
5. Zhai X, Mao L, Wu M, Liu J, Yu S. Challenges of anti-mesothelin car-T-cell therapy. Cancers (Basel). (2023) 15. doi: 10.3390/cancers15051357
6. Martinez M, Moon EK. Car T cells for solid tumors: new strategies for finding, infiltrating, and surviving in the tumor microenvironment. Front Immunol. (2019) 10:128. doi: 10.3389/fimmu.2019.00128
7. Muz B, de la Puente P, Azab F, Azab AK. The role of hypoxia in cancer progression, angiogenesis, metastasis, and resistance to therapy. Hypoxia (Auckl). (2015) 3:83–92. doi: 10.2147/hp.S93413
8. Nordsmark M, Bentzen SM, Rudat V, Brizel D, Lartigau E, Stadler P, et al. Prognostic value of tumor oxygenation in 397 head and neck tumors after primary radiation therapy. An international multi-center study. Radiother Oncol. (2005) 77:18–24. doi: 10.1016/j.radonc.2005.06.038
9. Guo Q, Lu L, Liao Y, Wang X, Zhang Y, Liu Y, et al. Influence of C-src on hypoxic resistance to paclitaxel in human ovarian cancer cells and reversal of fv-429. Cell Death Dis. (2018) 8:e3178. doi: 10.1038/cddis.2017.367
10. Overgaard J. Hypoxic modification of radiotherapy in squamous cell carcinoma of the head and neck–a systematic review and meta-analysis. Radiother Oncol. (2011) 100:22–32. doi: 10.1016/j.radonc.2011.03.004
11. Berahovich R, Liu X, Zhou H, Tsadik E, Xu S, Golubovskaya V, et al. Hypoxia selectively impairs car-T cells in vitro. Cancers (Basel). (2019) 11(5). doi: 10.3390/cancers11050602
12. Semenza GL. Targeting hif-1 for cancer therapy. Nat Rev Cancer. (2003) 3:721–32. doi: 10.1038/nrc1187
13. Lee JW, Bae SH, Jeong JW, Kim SH, Kim KW. Hypoxia-inducible factor (Hif-1)Alpha: its protein stability and biological functions. Exp Mol Med. (2004) 36:1–12. doi: 10.1038/emm.2004.1
14. Masoud GN, Li W. Hif-1α Pathway: role, regulation and intervention for cancer therapy. Acta Pharm Sin B. (2015) 5:378–89. doi: 10.1016/j.apsb.2015.05.007
15. Lu ZH, Wright JD, Belt B, Cardiff RD, Arbeit JM. Hypoxia-inducible factor-1 facilitates cervical cancer progression in human papillomavirus type 16 transgenic mice. Am J Pathol. (2007) 171:667–81. doi: 10.2353/ajpath.2007.061138
16. Abudoukerimu A, Hasimu A, Abudoukerimu A, Tuerxuntuoheti G, Huang Y, Wei J, et al. Hif-1α Regulates the progression of cervical cancer by targeting Yap/Taz. J Oncol. (2022) 2022:3814809. doi: 10.1155/2022/3814809
17. Yan B, Ma QF, Tan WF, Cai HN, Li YL, Zhou ZG, et al. Expression of Hif-1α Is a predictive marker of the efficacy of neoadjuvant chemotherapy for locally advanced cervical cancer. Oncol Lett. (2020) 20:841–9. doi: 10.3892/ol.2020.11596
18. Bachtiary B, Schindl M, Pötter R, Dreier B, Knocke TH, Hainfellner JA, et al. Overexpression of hypoxia-inducible factor 1alpha indicates diminished response to radiotherapy and unfavorable prognosis in patients receiving radical radiotherapy for cervical cancer. Clin Cancer Res. (2003) 9:2234–40.
19. Koh MY, Spivak-Kroizman T, Venturini S, Welsh S, Williams RR, Kirkpatrick DL, et al. Molecular mechanisms for the activity of Px-478, an antitumor inhibitor of the hypoxia-inducible factor-1α. Mol Cancer Ther. (2008) 7:90–100. doi: 10.1158/1535-7163.MCT-07-0463
20. Fallah J, Rini BI. Hif inhibitors: status of current clinical development. Curr Oncol Rep. (2019) 21:1–10. doi: 10.1007/s11912-019-0752-z
21. Jordan BF, Runquist M, Raghunand N, Baker A, Williams R, Kirkpatrick L, et al. Dynamic contrast-enhanced and diffusion Mri show rapid and dramatic changes in tumor microenvironment in response to inhibition of Hif-1α Using Px-478. Neoplasia. (2005) 7:475–85. doi: 10.1593/neo.04628
22. Tang Z, Kang B, Li C, Chen T, Zhang Z. Gepia2: an enhanced web server for large-scale expression profiling and interactive analysis. Nucleic Acids Res. (2019) 47:W556–w60. doi: 10.1093/nar/gkz430
23. Thul PJ, Åkesson L, Wiking M, Mahdessian D, Geladaki A, Ait Blal H, et al. A subcellular map of the human proteome. Science. (2017) 356:eaal3321. doi: 10.1126/science.aal3321
24. Akbari B, Soltantoyeh T, Shahosseini Z, Yarandi F, Hadjati J, Mirzaei HR. The inhibitory receptors pd1, tim3, and A2ar are highly expressed during mesocar T cell manufacturing in advanced human epithelial ovarian cancer. Cancer Cell Int. (2023) 23:104. doi: 10.1186/s12935-023-02948-0
25. Akbari B, Soltantoyeh T, Shahosseini Z, Jadidi-Niaragh F, Hadjati J, Brown CE, et al. Pge2-ep2/ep4 signaling elicits mesocar T cell immunosuppression in pancreatic cancer. Front Immunol. (2023) 14:1209572. doi: 10.3389/fimmu.2023.1209572
26. Ueda T, Shiina S, Iriguchi S, Terakura S, Kawai Y, Kabai R, et al. Optimization of the proliferation and persistency of car T cells derived from human induced pluripotent stem cells. Nat Biomed Eng. (2023) 7:24–37. doi: 10.1038/s41551-022-00969-0
27. Miller BC, Sen DR, Al Abosy R, Bi K, Virkud YV, LaFleur MW, et al. Subsets of exhausted cd8(+) T cells differentially mediate tumor control and respond to checkpoint blockade. Nat Immunol. (2019) 20:326–36. doi: 10.1038/s41590-019-0312-6
28. Beltra JC, Manne S, Abdel-Hakeem MS, Kurachi M, Giles JR, Chen Z, et al. Developmental relationships of four exhausted cd8(+) T cell subsets reveals underlying transcriptional and epigenetic landscape control mechanisms. Immunity. (2020) 52:825–41.e8. doi: 10.1016/j.immuni.2020.04.014
29. Chakravarti M, Dhar S, Bera S, Sinha A, Roy K, Sarkar A, et al. Terminally exhausted Cd8+ T cells resistant to pd-1 blockade promote generation and maintenance of aggressive cancer stem cells. Cancer Res. (2023) 83:1815–33. doi: 10.1158/0008-5472.Can-22-3864
30. Kallies A, Zehn D, Utzschneider DT. Precursor exhausted T cells: key to successful immunotherapy? Nat Rev Immunol. (2020) 20:128–36. doi: 10.1038/s41577-019-0223-7
31. Jun JC, Rathore A, Younas H, Gilkes D, Polotsky VY. Hypoxia-inducible factors and cancer. Curr Sleep Med Rep. (2017) 3:1–10. doi: 10.1007/s40675-017-0062-7
32. Luo D, Wang Z, Wu J, Jiang C, Wu J. The role of hypoxia inducible factor-1 in hepatocellular carcinoma. BioMed Res Int. (2014) 2014:409272. doi: 10.1155/2014/409272
33. Xie J, Xiao Y, Zhu XY, Ning ZY, Xu HF, Wu HM. Hypoxia regulates stemness of breast cancer Mda-Mb-231 cells. Med Oncol. (2016) 33:42. doi: 10.1007/s12032-016-0755-7
34. Nagaraju GP, Bramhachari PV, Raghu G, El-Rayes BF. Hypoxia inducible factor-1α: its role in colorectal carcinogenesis and metastasis. Cancer Lett. (2015) 366:11–8. doi: 10.1016/j.canlet.2015.06.005
35. Parks SK, Cormerais Y, Marchiq I, Pouyssegur J. Hypoxia optimises tumour growth by controlling nutrient import and acidic metabolite export. Mol Aspects Med. (2016) 47-48:3–14. doi: 10.1016/j.mam.2015.12.001
36. Palayoor ST, Mitchell JB, Cerna D, Degraff W, John-Aryankalayil M, Coleman CN. Px-478, an inhibitor of hypoxia-inducible factor-1alpha, enhances radiosensitivity of prostate carcinoma cells. Int J Cancer. (2008) 123:2430–7. doi: 10.1002/ijc.23807
37. Nakamura H, Makino Y, Okamoto K, Poellinger L, Ohnuma K, Morimoto C, et al. Tcr engagement increases hypoxia-inducible factor-1 alpha protein synthesis via rapamycin-sensitive pathway under hypoxic conditions in human peripheral T cells. J Immunol. (2005) 174:7592–9. doi: 10.4049/jimmunol.174.12.7592
38. Buck MD, O’Sullivan D, Pearce EL. T cell metabolism drives immunity. J Exp Med. (2015) 212:1345–60. doi: 10.1084/jem.20151159
39. Pearce EL, Poffenberger MC, Chang CH, Jones RG. Fueling immunity: insights into metabolism and lymphocyte function. Science. (2013) 342:1242454. doi: 10.1126/science.1242454
40. Lukashev D, Caldwell C, Ohta A, Chen P, Sitkovsky M. Differential regulation of two alternatively spliced isoforms of hypoxia-inducible factor-1 alpha in activated T lymphocytes. J Biol Chem. (2001) 276:48754–63. doi: 10.1074/jbc.M104782200
41. Zhu Y, Zang Y, Zhao F, Li Z, Zhang J, Fang L, et al. Inhibition of hif-1α by px-478 suppresses tumor growth of esophageal squamous cell cancer in vitro and in vivo. Am J Cancer Res. (2017) 7:1198–212.
42. Kosti P, Opzoomer JW, Larios-Martinez KI, Henley-Smith R, Scudamore CL, Okesola M, et al. Hypoxia-sensing car T cells provide safety and efficacy in treating solid tumors. Cell Rep Med. (2021) 2:100227. doi: 10.1016/j.xcrm.2021.100227
43. Palazon A, Tyrakis PA, Macias D, Veliça P, Rundqvist H, Fitzpatrick S, et al. An hif-1α/vegf-a axis in cytotoxic T cells regulates tumor progression. Cancer Cell. (2017) 32:669–83.e5. doi: 10.1016/j.ccell.2017.10.003
44. Palazon A, Goldrath AW, Nizet V, Johnson RS. Hif transcription factors, inflammation, and immunity. Immunity. (2014) 41:518–28. doi: 10.1016/j.immuni.2014.09.008
45. Liikanen I, Lauhan C, Quon S, Omilusik K, Phan AT, Bartrolí LB, et al. Hypoxia-inducible factor activity promotes antitumor effector function and tissue residency by Cd8+ T cells. J Clin Invest. (2021) 131. doi: 10.1172/jci143729
46. Doedens AL, Phan AT, Stradner MH, Fujimoto JK, Nguyen JV, Yang E, et al. Hypoxia-inducible factors enhance the effector responses of Cd8(+) T cells to persistent antigen. Nat Immunol. (2013) 14:1173–82. doi: 10.1038/ni.2714
47. Phan AT, Doedens AL, Palazon A, Tyrakis PA, Cheung KP, Johnson RS, et al. Constitutive glycolytic metabolism supports cd8(+) T cell effector memory differentiation during viral infection. Immunity. (2016) 45:1024–37. doi: 10.1016/j.immuni.2016.10.017
48. Voron T, Colussi O, Marcheteau E, Pernot S, Nizard M, Pointet AL, et al. Vegf-a modulates expression of inhibitory checkpoints on cd8+ T cells in tumors. J Exp Med. (2015) 212:139–48. doi: 10.1084/jem.20140559
49. Bannoud N, Dalotto-Moreno T, Kindgard L, García PA, Blidner AG, Mariño KV, et al. Hypoxia supports differentiation of terminally exhausted cd8 T cells. Front Immunol. (2021) 12:660944. doi: 10.3389/fimmu.2021.660944
50. Koh MY, Spivak-Kroizman T, Venturini S, Welsh S, Williams RR, Kirkpatrick DL, et al. Molecular mechanisms for the activity of px-478, an antitumor inhibitor of the hypoxia-inducible factor-1alpha. Mol Cancer Ther. (2008) 7:90–100. doi: 10.1158/1535-7163.Mct-07-0463
51. Labiano S, Meléndez-Rodríguez F, Palazón A, Teijeira Á, Garasa S, Etxeberria I, et al. Cd69 is a direct hif-1α Target gene in hypoxia as a mechanism enhancing expression on tumor-infiltrating T lymphocytes. Oncoimmunology. (2017) 6:e1283468. doi: 10.1080/2162402x.2017.1283468
Keywords: CAR T cell therapy, pharmacological targeting, HIF-1α, PX-478, cervical cancer, T cell exhaustion
Citation: Panahi Meymandi AR, Akbari B, Soltantoyeh T, Shahosseini Z, Hosseini M, Hadjati J and Mirzaei HR (2024) PX-478, an HIF-1α inhibitor, impairs mesoCAR T cell antitumor function in cervical cancer. Front. Oncol. 14:1357801. doi: 10.3389/fonc.2024.1357801
Received: 18 December 2023; Accepted: 30 January 2024;
Published: 15 February 2024.
Edited by:
Mazdak Ganjalikhani Hakemi, Isfahan University of Medical Sciences, IranReviewed by:
Vitaly Pozdeev, University of Luxembourg, LuxembourgDmitry Aleksandrovich Zinovkin, Gomel State Medical University, Belarus
Copyright © 2024 Panahi Meymandi, Akbari, Soltantoyeh, Shahosseini, Hosseini, Hadjati and Mirzaei. This is an open-access article distributed under the terms of the Creative Commons Attribution License (CC BY). The use, distribution or reproduction in other forums is permitted, provided the original author(s) and the copyright owner(s) are credited and that the original publication in this journal is cited, in accordance with accepted academic practice. No use, distribution or reproduction is permitted which does not comply with these terms.
*Correspondence: Jamshid Hadjati, hajatij@tums.ac.ir; Hamid Reza Mirzaei, h-mirzaei@tums.ac.ir