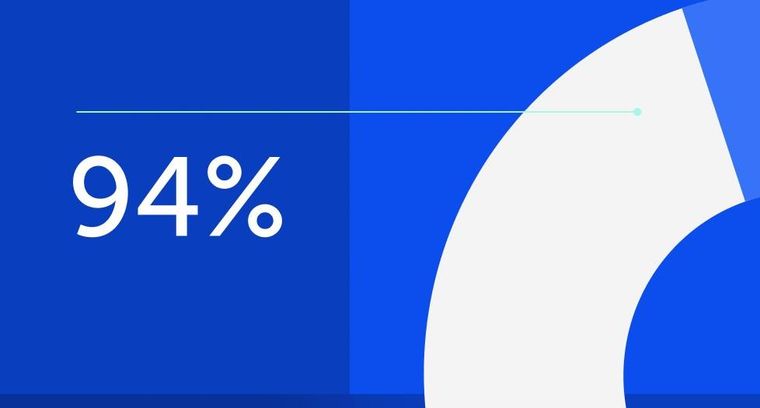
94% of researchers rate our articles as excellent or good
Learn more about the work of our research integrity team to safeguard the quality of each article we publish.
Find out more
ORIGINAL RESEARCH article
Front. Oncol., 02 August 2024
Sec. Neuro-Oncology and Neurosurgical Oncology
Volume 14 - 2024 | https://doi.org/10.3389/fonc.2024.1355202
This article is part of the Research TopicWomen in Neuro-Oncology and Neurosurgical Oncology Vol II: 2023View all 7 articles
Introduction: Glioblastoma IDH-wildtype (GBM) is the most malignant brain tumor in adults, with a poor prognosis of approximately 15 months after diagnosis. Most patients suffer from a recurrence in <1 year, and this renders GBM a life-threatening challenge. Among molecular mechanisms driving GBM aggressiveness, angiogenesis mediated by GBM endothelial cells (GECs) deserves consideration as a therapeutic turning point. In this scenario, calpains, a family of ubiquitously expressed calcium-dependent cysteine proteases, emerged as promising targets to be investigated as a novel therapeutic strategy and prognostic tissue biomarkers.
Methods: To explore this hypothesis, GECs were isolated from n=10 GBM biopsies and characterized phenotypically by immunofluorescence. The expression levels of calpains were evaluated by qRT-PCR and Western blot, and their association with patients’ prognosis was estimated by Pearson correlation and Kaplan–Meier survival analysis. Calpain targeting efficacy was assessed by a time- and dose-dependent proliferation curve, MTT assay for viability, caspase-3/7 activity, migration and angiogenesis in vitro, and gene and protein expression level modification.
Results: Immunofluorescence confirmed the endothelial phenotype of our primary GECs. A significant overexpression was observed for calpain-1/2/3 (CAPN) and calpain-small-subunits-1/2 (CAPNS1), whereas calpastatin gene, the calpain natural inhibitor, was reported to be downregulated. A significant negative correlation was observed between CAPN1/CAPNS1 and patient overall survival. GEC challenging revealed that the inhibition of calpain-1 exerts the strongest proapoptotic efficacy, so GEC mortality reached the 80%, confirmed by the increased activity of caspase-3/7. Functional assays revealed a strong affection of in vitro migration and angiogenesis. Gene and protein expression proved a downregulation of MAPK, VEGF/VEGFRs, and Bcl-2, and an upregulation of caspases and Bax-family mediators.
Conclusion: Overall, the differential expression of calpains and their correlation with patient survival suggest a novel promising target pathway, whose blockade showed encouraging results toward precision medicine strategies.
Tumors affecting the central nervous system (CNS) are a cluster of heterogeneous neoplasia that, despite arising in a common anatomical region, differ from each other in morphology, etiology, site of onset, molecular signature, and clinical course (1). With an incidence of approximately 200,000 people worldwide every year, CNS tumors represent approximately 2% of cancer deaths (2, 3), remaining among the most difficult cancers to treat, with a 5-year overall survival (OS) <35% (4).
Among these, glioblastoma IDH-wildtype (herein called GBM, World Health Organization grade 4) is the most frequent and malignant glioma in adults, and the median survival rate of patients diagnosed is 15 months, with a 5-year survival rate <5%, the bottommost long-term survival rate of malignant brain tumors (5).
The difficulty encountered when fighting GBM concerns the rapid progression, immune system escape, survival, angiogenesis, invasiveness, genetic instability, high frequency of relapse, and resistance to radio and chemotherapies. Currently, the standard therapeutic strategy consists of maximal resection (when possible), followed by radiotherapy in combination with temozolomide (TMZ) (6). Despite these aggressive regimens, most patients suffer from a recurrence in <1 year and the majority succumb to the disease within 2 years of diagnosis (7). The typical invasiveness of GBM into the surrounding tissues determines a progressive deterioration of patients’ cognitive skills, significantly increasing the morbidity associated with the disease (8).
During cancer progression, especially in aggressive tumors such as GBM, neoangiogenesis closely contributes to mass expansion, through the delicate synergy between cancer stem cells, neoplastic cells, and endothelial cells (ECs). Notably, the necrotic core of GBM is characterized by an hypoxic environment, able to increase the demand for oxygen and nutrient supply to support tumor survival (9), by the activation of pro-angiogenic mechanisms.
However, the pathological unbalance between pro- and antiangiogenic mediators often generates ultra-structurally abnormal vessels, dilated, irregularly branched, convoluted, and larger than the physiological ones, with increased permeability due to the lack of a complete basal membrane and the presence of a widely distributed fenestration (10). The hyperpermeability of tumor vasculature leads to local edema and extravasation of plasma, thus increasing the interstitial pressure and altering blood flow and leukocyte flux (11, 12). The leakiness of newly formed blood vessels means that large tumor areas are not supplied with blood flow and are therefore not reachable by circulating pharmacological molecules, nutrients, and oxygen, establishing large ischemic and necrotic regions, in a self-sustaining cycle (13).
Numerous observations reported that calpains, a well-conserved family of intracellular cysteine proteinases, are strongly activated by growth factors, primarily vascular endothelial growth factor (VEGF), so that calpains residing in the ECs have a considerable impact on tumor angiogenesis (14).
Belonging to a family of calcium-dependent cysteine proteases, calpains (encoded by CAPN genes) proteolytically process substrates to transform their structures and modulate activities. For their pleiotropic activities, it appears reasonable that calpain family is differentially expressed in human cancers, highlighting the key role of calpains in tumor onset and advancement (15).
Some reports demonstrated that calpain expression levels in many cancer subtypes, detected by immunohistochemistry or mRNA, are directly correlated to histopathological markers of malignancy and worst clinical course, fueling the consideration of calpains as negative prognostic markers. However, it should be considered that high expression levels are not automatically associated with high proteolytic activity, as high level of calpastatin, an inhibitor efficient in binding and blocking up to four calpain heterodimers simultaneously, may counteract calpain activity (16, 17).
The scientific literature reports an altered pattern of calpain family expression, with an overexpression of CAPN1, CAPN2, and the calpain subunit 1 (CAPNS1) in several cancers, as breast cancer (18–20), colorectal cancer (21), renal cell carcinoma (22), hepatocarcinoma (23), nasopharyngeal carcinoma (24), acute myeloid leukemia (25), schwannoma and meningioma (26), and glioma (27). Conversely, high levels of calpastatin have been found in endometrial carcinomas (28). Several studies have also highlighted a direct correlation between calpain expression and clinical outcome, including response to therapy, metastatic potential, and patients’ survival (29, 30).
In the present study, we looked at assessing the involvement of calpains in GBM angiogenesis and aggressiveness, by examining GECs, the key actors of tumor vascularization and infiltration. To this aim, we evaluated i) the gene and protein expression level of calpains in GECs, ii) their association with patients’ clinical outcomes to evaluate their potential as prognostic biomarkers, and iii) the anti-tumoral and anti-angiogenic effectiveness of calpain inhibitors, with the purpose to propose a novel promising therapeutic target, as a novel approach of personalized and precision medicine.
Patients of both sexes (n=10) with newly diagnosed GBM who underwent surgery for tumor excision at the Neurosurgery Unit of Fondazione IRCCS Ca’ Granda Ospedale Maggiore Policlinico from 2019 to 2021 were eligible for this study. The Institutional Review Board approved the protocol, and all patients provided informed consent. Inclusion criteria were as follows: 1) age between 18 and 80 years, 2) Karnofsky Performance Status (KPS) >60, 3) signed consent for the study, and 4) histologically proven diagnosis of GBM according to the WHO classification 2016 on review by two independent pathologists. Exclusion criteria were as follows: 1) previous brain surgery for other intracranial malignancies, 2) concomitant life-threatening disease, 3) history or presence of other malignancies, and 4) refusal or inability to consent to the study protocol. Demographic and clinical data covering the interval from the date of diagnosis to death or the last follow-up visit were collected from the patient’s records. Specifically, KPS, Ki-67 positivity, and MGMT promoter methylation, and IDH1/2 status were recorded.
Tissue samples deriving from tumor biopsies were transported from the operating room to the Laboratory of Experimental Neurosurgery and Cell Therapy under sterile conditions to be processed. From each sample, an aliquot was frozen dry at −80°C for successive molecular analysis, whereas another aliquot was manually and enzymatically digested with Trypsin (Gibco) for 1 h at 37°C. The resulting single cell suspension was plated into a 25-cm2 flask coated with bovine type I collagen (BD Biosciences, Milan, Italy) and cultured in endothelial proliferation medium (EndoPM) at 37°C, 5% CO2, 5% O2, according to our previous published protocols to isolate GECs (31–33). The media were changed one to two times a week and passaged at a split ratio of 1:4 every 14 days. Notably, this published and validated protocol for isolation, culture, and characterization allows us to maintain and manipulate primary GBM-derived endothelial cells (31–33).
GECs (1×104/well) were seeded into μ-Slide 8 Well, ibiTreat (Ibidi, Martinsried, Germany) collagen coated. When cells reached the desired confluence, they were fixed in paraformaldehyde 4% for 20 min at RT, washed twice with D-PBS (Euroclone) and incubated with 0.1 M glycine to quench auto-fluorescence. Then, the coverslips were incubated with PBS + 0.25%Triton ×100 to permeabilize cell membranes and then blocked in D-PBS + 5%BSA for 30 min at RT. Incubation with primary antibodies (Abs) diluted in blocking buffer was performed overnight at 4°C. The following primary Abs were used: anti-vascular endothelial growth factor (VEGF-A, Abcam), anti-Von Willebrand Factor (VWF, Sigma Aldrich), anti-VEGF receptor-1 (VEGFR1, Thermo Fisher Scientific), anti-VEGF receptor-2 (VEGFR2, Thermo Fisher Scientific), and anti-VE-Cadherin (Abcam), as specific markers for GECs. The day after, primary Abs were removed, and specific fluorescent secondary antibodies (goat anti-mouse and goat anti-rabbit, Life Technologies) were added for 45 min at RT, protected from light. After incubation and two washes with D-PBS, DAPI (1 μg/ml, Thermo Fisher) staining was performed for 3 min at RT, and coverlips were mounted with ProLong Gold Antifade Mountant (Thermo Fisher). Immunolabeling was acquired using an inverted DMI4 microscope equipped with DFC350xCCDcamera and LAS-X software (all from Leica Microsystems, Wetzlar, Germany).
DNA from the tumor samples was obtained using DNeasy Blood & Tissue Kits Isolation kit (Qiagen, USA), following manufacturer’s instructions. DNA quantification and integrity assessment were performed with NanoDrop 3000 (Thermo Fisher).
The assessment of hotspot mutations in IDH1, IDH2, and TERT promoter was performed as previously described (34). In brief, the molecular test investigates the following mutations in IDH1 and IDH2 genes, using MassARRAY Analyzer 4 system (Agena Bioscience, CA, USA): −IDH1, c.394C> T p.R132C, c.394C> G p.R132G, c. 394C> A p.R132S, c.395G> A p.R132H, c.395G> T p.R132L, c.395G> C p.R132P; and −IDH2, c.514A> C p.R172R, c.514A> G p.R172G, c.514A> T p.R172W, c.515G> A p.R172K, c.515G> C p.R172T, c. 515G> T p.R172M, c.516G> A p.R172R, c.516G> C p.R172S, c.516G> T p.R172S38. PCR, SAP, and IPLEX reactions were conducted as described in the manufacturer’s protocol (Agena Bioscience, San Diego, CA, USA). Samples were transferred to a SpectroCHIP (Agena Bioscience, San Diego, CA, USA) and analyzed by mass spectrometry. The spectral profiles generated by MALDI-TOF mass spectrometry were evaluated using Typer v.4.0 software (Agena Bioscience, San Diego, CA, USA).
The molecular test investigates the methylation of the MGMT gene promoter (GRCh37/hg19 chr10: 131.265.471–131.265.581), by pyrosequencing, with the “Pyromark Q96 ID System”. The protocol used is reported in Fontana et al. (35). DNA was extracted from FFPE tumor sample using QIAamp DNA FFPE kit (Qiagen, USA) and treated with sodium bisulfite using EZ DNA Methylation-GOLD kit (Euroclone, Italy). The test detects the methylation levels of 10 CpG sites located in the promoter region of the MGMT gene with a sensitivity of 95%. The methylation analysis was implemented as previously described (35). PCR was performed on at least 20 ng of bisulfite-treated DNA and approximately 10 pmol primers. Quantitative DNA methylation analysis was carried out on the Pyro Mark ID instrument using Pyro Gold Reagents (Qiagen) and 1 pmol of sequencing primer. Methylation data were analyzed by the Q-CpG software v1.9 (Qiagen), and the levels of methylation of each sample are represented by the mean of the methylation percentages at each CpG site of the investigated region. The analysis of the methylation levels of the MGMT gene promoter represents a prognostic and predictive marker of response to standard treatment with alkylating agents (e.g., TMZ).
In order to assess the effectiveness of calpain-targeting in GECs, calpain inhibitor-1 (against CAPN1) and calpain inhibitor-2 (against CAPN2) were administered at 25 μM, 50 μM, and 100 μM, whereas AC-calpastatin (inhibiting both CAPN1 and CAPN2) was administered at 10 μM and 20 μM, to evaluate dose-dependent efficacy and toxicity. All compounds were purchased by Calbiochem. TMZ (Schering-Plough, Milano, Italy) 200 μM was administered to evaluate the synergistic effect of the current alkylating agent currently used in GBM therapy, with tested compounds.
3-(4,5-Dimethylthiazol-2-yl)−2,5-diphenyltetrazolium bromide (MTT) assay was used to assess cell viability as a function of redox potential. GECs (5×103/well) were seeded and cultured in 96-well plate for 24 h. Then, culture media were replaced with fresh media containing the specific treatments. Tests were performed in triplicate following 48 h of treatment. At the end, culture media were replaced with 100 μl of fresh media + 10 μl of MTT 5 mg/ml in D-PBS. After 4 h of incubation, media were removed, and cells were lysed with 100 μl of 2-propanol/formic acid (95:5, by vol) for 10 min. Then, absorbance was read at 570 nm in a microplate reader. Notably, the same treatments were administered to healthy brain-derived cells as astrocytes (ABM, Cat. No. T0280) and neural progenitor stem cells (NPSC, CelProgen, Cat. No. 36057-02) to evaluate if the concentrations of calpain inhibitors tested would affect normal brain cells.
The LIVE/DEAD® Viability/Cytotoxicity Assay Kit provides a two-color fluorescence (Calcein AM and EthD-1) cell viability assay, which measures intracellular esterase activity and plasma membrane integrity, thus determining live and dead cells. GECs were seeded into 24-well plates (2×104/well) until confluence and then treated with the specific treatments for 72 h. Then, the mixture of Calcein AM and EthD-1 was prepared following the manufacturer’s instruction and administered to cell cultures. Fluorescence images were acquired with an Eclipse Ti-E microscope (Nikon Instruments, Italy).
The Caspase-Glo® 3/7 Assay is a luminescent assay added as an “add-mix-measure” format resulting in cell lysis, followed by caspase cleavage of the substrate and generation of a “glow-type” luminescent signal, produced by luciferase. GECs (5×103/well) were seeded and cultured in a 96-well plate for 24 h and then treated with the specific treatments. After 72 h, Caspase-Glo® 3/7 reagent was added in equal volume, and luminescence, proportional to the amount of caspase activity, was read with a microplate reader.
μ-Plate angiogenesis 96 wells (Ibidi) were coated with 12.5 mg/mL Matrigel (BD Bioscience), 10 μL/well on ice. After gentle agitation to ensure complete coating, plates were incubated for 30 min at 37°C to allow solidification of Matrigel. GECs (1×104/well) were seeded in triplicate in EndoPM, in the presence or absence of the described treatments, and incubated at 37°C, 5% CO2, and 5% O2. Cord formation was monitored for 24 h with an inverted Eclipse Ti-E microscope (Nikon Instruments, Florence, Italy), equipped with a high-resolution cSMOS camera (Andor Zyla, Andor Technology, Belfast, UK) and NIS_Elements 4.51 software, using differential interference contrast (DIC). After 24 h of incubation, five random images were acquired and analyzed with “Angiogenesis Analyzer” plugin in ImageJ.
GECs were seeded (1×104 cells, each side) into Ibidi Culture Inserts (Ibidi) and cultured until 95% confluence was reached. After that, the inserts were removed, and cells were challenged with the aforementioned treatments. After 24 h, GECs were stained with 1 mg/mL Calcein AM (Thermo Fisher Scientific) for 30 min at 37°C, and images of GECs migrated into cells-free gap were acquired with an inverted Leica DMI6000B widefield microscope at ×20 magnifications in five random fields. Cells that migrated into the gap were then counted using “Analyze Particles” in ImageJ.
GECs (1×105), were seeded into 25 cm2 collagen-coated culture flasks. When 90% confluence was reached or at the end of the above listed treatment (72h), total RNA was extracted following Invitrogen™ TRI Reagent™ (Thermo Fisher Scientific) manufacturer’s protocol and quantified with a NanoDrop 1000 spectrophotometer (Thermo Fisher Scientific). Reverse transcriptase reaction was executed using TranScriba Kit (A&A Biotechnology), loading 1 μg of RNA (A260/A280 > 1.8), according to the manufacturer’s instructions. Quantitative real-time PCR analysis (qRT-PCR) was performed using StepOnePlus™ (Thermo Fisher Scientific) using 1 μg of cDNA, forward and reverse primers (250 nM each one) and Titan HotTaq EvaGreen® qPCR Universal Mix (BioAtlas). Data were normalized to 18S expression, used as endogenous control. Relative gene expression was determined using the 2−ΔΔCt method. The primer sequences are provided in Table 1. Notably, the gene expression level of GECs was calculated as a fold-change versus human brain microvascular endothelial cells (HBMECs, Creative Biolabs), in comparison also to endothelial cells isolated from low-grade gliomas (LGG-ECs), using the same protocol described for GECs (32).
GECs were seeded into 25-cm2 collagen-coated culture flasks precoated with Collagen Bovine Type I and cultured in control condition to assess basal calpain expression levels (2×105), or in the presence of the desired anti-calpains treatment for 72 h (5×105, each condition). In both cases, cells were lysed with M-PER Protein Extraction Reagent (Thermo Fisher Scientific) in the presence of Halt Protease Inhibitor Cocktail (Thermo Fisher Scientific) and quantified by the Pierce Detergent Compatible BCA Assay Kit (Thermo Fisher Scientific). Protein lysates (30 μg, for basal GEC expression level compared to HBMECs and 20 μg for GECs treated with calpain inhibitors) were separated in Bolt 10% Bis–Tris Plus Gels (Thermo Fisher Scientific) in Mini Gel Tank (Thermo Fisher Scientific) and transferred onto nitrocellulose iBlot 2 Transfer Stacks using iBlot 2 Dry Blotting System (Thermo Fisher Scientific). After transfer, the membrane was blocked in Tris-buffered saline/Tween20, 5% milk solution and incubated separately with anti-GAPDH, anti-calpain-1, anti-calpain-2, anti-calpain-3, anti-calpain small subunit 1, anti-calpain small subunit 2 and anti-calpastatin (all purchased from Abcam), anti-ERK1, anti-pERK1/2 (ThermoFisher Scientific), anti-Akt (Abcam), anti-MEK1 (SantaCruz Biotechnology), and anti-VEGFA (Abcam), overnight at 4°C. After incubation with HRP-labeled secondary antibody (goat-anti-rabbit IgG and goat anti-mouse IgG, Invitrogen, Carlsbad, CA, USA), protein bands were scanned with SuperSignal West Pico PLUS Chemiluminescent Substrate (Thermo Fisher Scientific) and detected by ChemiDoc XRSþ (Bio-Rad, Hercules, CA, USA). Densitometric analyses were performed using ImageJ. Notably, as for qRT-PCR, the protein expression of calpains in basal GECs was visualized and calculated as a ratio to HBMECs, in comparison also to LGG-ECs, as a lower grade brain tumor subtype.
All analyses were done with GraphPad Prism (GraphPad Software, Inc., La Jolla, CA, USA) and IBM SPSS (Version 29.0). Continuous variables are presented as median and interquartile range (IQR). Categorical variables are presented as counts and percentages. Parameters were compared and analyzed by a one-way analysis of variance. When significant differences were detected, Dunnet post-hoc comparisons versus control group were made. The Pearson correlation test and Kaplan–Meier survival analysis were performed to assess the association between calpain gene expression and patient overall survival. Data reported represent the mean±standard deviation of at least three independent experiments run in triplicate. Differences were considered statistically significant for p<0.05.
The demographic, clinical, and molecular characteristics of patients, whose GBM biopsies were used to isolate GECs, are listed in Table 2. The median age of patients at diagnosis was 60 years (IQR, 47–76) and 67% were men. The median value of MGMT promoter methylation was 14 (IQR, 6.5–31.5), and all GBMs were wild type for IDH.
The successful isolation of GECs from tissue biopsies was proved by immunophenotypic characterization performed by immunofluorescence analysis. GECs displayed positivity to the principal endothelial and angiogenesis-related mediators as VEGF-A and its receptors (VEGFR1-2), von Willebrand factor (VWF) and Ve-cadherin (Figure 1), accordingly to our previous reports in the field (31, 32).
Figure 1 Immunophenotypic characterization of GECs by immunofluorescence for VEGF (red fluorescent labeling), VEGFR-1 (red fluorescent labeling), VEGFR-2 (red fluorescent labeling), VWF (green fluorescent labeling), and VE-Cadherin (red fluorescent labeling). Nuclei were counterstained with DAPI (blue fluorescent labeling). The white dotted boxes contain the respective isotype control for each antibody. Scale bar, 50 μm.
Once the GEC endothelial phenotype was confirmed, we proceeded with the gene expression screening of calpains, revealing a significant overexpression of CAPN1-2–3, and their small regulator subunits CAPNS1-2, in GECs compared to LGG-ECs and HBMECs, used as controls. An opposite trend was observed for CAST gene, encoding for the endogenous inhibitor of calpains (Figure 2A). These molecular alterations were confirmed also at the protein level, as Western blot analysis revealed a significant upregulation of calpains in GECs to the detriment of CAST (Figures 2B, C).
Figure 2 Gene and protein expression analysis of calpain 1, 2, and a3 (CAPN1, CAPN2, and CAPN3), calpastatin (CAST) and calpain small subunits 1 and 2 (CAPNS1 and CAPNS2) on human brain microvascular endothelial cells (HBMECs), low-grade glioma-derived endothelial cells (LGG-ECs), and glioblastoma endothelial cells (GECs). (A) Gene expression obtained by quantitative real-time PCR and reported as fold change to HBMECs, used as control. (B) Representative images of Western blot for calpain signaling and relative densitometric analysis (C) obtained by Fiji ImageJ, to quantify protein expression level, normalized to GAPDH expression level, used as endogenous control, and to untreated CTRL. Data are the mean±SD of at least three independent experiments run in triplicate. *p<0.05, **p<0.01, ***p<0.001 vs. HBMECs.
In order to evaluate the potential prognostic value of CAPN expression, we performed a Pearson bivariate correlation, surprisingly revealing that, despite the small sample size, a statistically significative negative association between the gene expression level of CAPN1 and CAPNS1 and patients’ OS exists (Figures 3A, B). Interestingly enough, we performed a Kaplan–Meier survival analysis to assess the impact of calpain expression on OS, identifying a valid statistically significant cutoff for CAPN1 (fold change >2.1 compared to HBMECs, p=0.041) and CAPNS1 (fold change >2.0, p=0.022) as negative prognostic factors for a OS < 12 months (Figures 3C, D). Notably, to perform this analysis, we selected the median gene expression value of our patient cohort as potential cutoff.
Figure 3 Pearson correlation between patients’ overall survival (OS) and gene expression levels of CAPN1 (A) and CAPNS1 (B). A negative association is indicated by a negative correlation coefficient (r). (C, D) Kaplan–Meier survival analysis was performed to assess the association between CAPN1/CAPNS1 gene expression levels and OS. To stratify our patient cohort, the values of 2.1 for CAPN1and 2.0 for CAPNS1 (the median expression fold-change to healthy cells) were used as cutoff to evaluate the cumulative 1-year survival. Data were obtained by IBM SPSS (Version 29.0). Exact p-values are specified in the graphs.
These results prompted the potential to test inhibitors of calpain signaling, to curb the angiogenic process. To this aim, the effect of calpain-1 and calpain-2 inhibitors and calpastatin, the natural inhibitor of both calpain-1 and calpain-2 was assessed on GEC viability, proliferation, apoptosis, and functionality. Toxicity test was performed by MTT and Live and Dead assays with a dose escalation. These tests revealed a dose–response trend, especially for calpain-1 inhibitor, whose maximum effect was observed at the concentration of 100 μM (Figure 4A). Contrary, Live, and Dead assay revealed a toxic effect at this concentration, with a high percentage of necrotic cells (Figure 4C), suggesting carrying out further experiments using our optimal concentration of 50 μM. The doses of the other compounds were chosen accordingly. Once the optimal dose was identified, we challenged healthy brain-derived cells as astrocytes and NPSC, to evaluate the possible, but not observed (Figure 4B), toxic effect of calpain inhibition.
Figure 4 (A) MTT assay performed after the administration of different doses of calpain inhibitor 1–2 and calpastatin. (B) MTT assay performed on healthy brain cells, astrocytes, and neural progenitor stem cells (NPSC) to assess the possible toxic effect of calpain inhibitors. (C) Representative images of Live and Dead assay conducted to assess the toxicity of tested compounds. Data are the mean±SD of at least three independent experiments run in triplicate. **p<0.01, ***p<0.001.
The viability test on GECs showed that calpain inhibitor 1 had the strongest effect in decreasing GEC viability and promoting cell death (Figure 5A), as the viability reached the 20% and the mortality reached the 400% compared to untreated control. These data were confirmed by the statistically significant increased activity of caspase-3 and caspase-7, considered as effector caspases of apoptotic events (Figure 5B). Interestingly, the analysis of viability also revealed a synergic activity of calpain inhibitors and TMZ, the standard treatment for GBM patients, suggesting the potential efficacy of a combined treatment (Figure 5C).
Figure 5 (A) Estimation of viable and dead GECs after treatment with calpain inhibitors 1–2 and calpastatin. (B) Caspase-3 and caspase-7 activity assay performed after GEC treatment. (C) Viability test conducted by MTT on GECs treated with calpain inhibitors 1–2 and calpastatin, alone or in combination with TMZ. Data are the mean±SD of at least three independent experiments run in triplicate. *p<0.05, **p<0.01, ***p<0.001.
Then, to test the effect of calpain inhibitors on angiogenic potential, in vitro tube-like structure assay on primary GECs confirmed the inhibition of vascular network formation (Figure 6A), with a statistically significant reduction in total tube length (Figure 6B). Moreover, the same effect was observed on GEC migration, as the administration of calpain inhibitors, especially calpain inhibitor-1, resulted in a significant reduction in cell migration across into the gap (Figures 6C, D).
Figure 6 (A) Tube-like structure assay and (C) migration assay performed on GEC after treatment with calpain inhibitors. The estimation of treatment efficacy was performed by calculating the total tube length (B) and the number of cells migrated into the gap (D). Data are the mean±SD of at least three independent experiments run in triplicate. p<0.05, **p<0.01, ***p<0.001.
Finally, following scientific literature and our previous studies, we explored the molecular pathways potentially impacted by calpain inhibition, through a gene (Figure 7) and protein (Figures 8A, B) expression screening. According to the previous results on GEC proliferation, survival, and functionality, calpain inhibitor 1 was found to be particularly effective in inhibiting proliferative signaling by the downregulation of proliferative signaling as MAPK, as RAF/RAS/MERK/ERK, proangiogenic pathways mediated by VEGF and its receptors and anti-apoptotic regulators as Bcl-2, and by upregulating proapoptotic mediators, as caspases and Bax-family mediators.
Figure 7 Gene expression analysis was conducted on GECs after treatment with calpain inhibitors by qRT-PCR. Data are the mean±SD of at least three independent experiments run in triplicate. *p<0.05, **p<0.01, ***<0.001.
Figure 8 (A) Representative images of Western blot analyses conducted on GECs treated with calpain inhibitors for 72 h. (B) Densitometric quantification to assess protein expression levels of tested markers, normalized to GAPDH expression level, used as endogenous control, and to untreated CTRL. Data were obtained by Fiji ImageJ. Data are the mean±SD of at least three independent experiments run in triplicate. *p<0.05, **p<0.01.
GBM is the most challenging primary brain tumor in adults, resulting in the assignment of the highest grade in the WHO classification. Accounting for 54% of all gliomas, it is almost always lethal (36). Despite the aggressive therapeutic regimens, consisting of gross total resection, followed by radiotherapy with concomitant and adjuvant therapies with TMZ, the majority of patients experience a relapse during the first year after diagnosis.
The urgency to identify a novel targeted treatment able to counteract the extremely malignant behavior of GBM drove the hypothesis that a targetable molecular signature may be involved in patient’s response to treatment and, then, in patient’s survival. To verify this hypothesis, a preliminary study, whose overall results are currently the subject of bioinformatic analysis that will be reported in a manuscript in preparation, was conducted using comparative genomic hybridization (array-CGH) to identify distinctive copy number variations in patients classified as long-term survivors (LTS, OS>24 months) and short-term survivors (STS, OS<12 months).
Notably, due to short life expectancy, GBM-LTS, defined as patients who survive longer than 2 years post-diagnosis, comprise <15% of all cases; thus, comparative studies on molecular differences between LTS and STS are challenging and promising, with a potential enormous impact on clinical practice.
The analysis of chromosomic unbalances exclusive of LTS group showed the upregulation of oncosuppressor pathways, regulating cell proliferation, survival, angiogenesis, and response to treatment. The CNV investigation together with the gene ontology analysis reported in LTS patients, an enrichment of pathways related to cell activation, cell cycle process, proteolysis, calcium-dependent cysteine-type endopeptidase activity, negative regulation of apoptotic process, and positive regulation of cell survival, revealing also an altered pattern of calpain family.
Calpains represent a conserved family of cysteine proteinases able to catalyze the controlled proteolysis of many specific substrates. The biological activity of calpains influences many central cellular processes, such as proliferation, apoptosis, survival, signaling, and cytoskeleton remodeling. From these premises, we decided to examine the active involvement of calpain in GBM aggressiveness, particularly focusing on angiogenic mechanisms promoted by ECs.
Using our already developed protocols, we started isolating GECs from GBM biopsies, confirming the endothelial phenotype by immunofluorescent analysis for endothelial and angiogenesis-related markers. GECs showed positivity to the most characterized pro-angiogenic mediators, VEGF-A and its receptors VEGFR1–VEGFR2, whose signaling has been widely recognized as crucial contributor of pathogenic angiogenesis and consequent formation of abnormal, fragile, and permeable microvessels (37, 38). The action of VEGF is primarily exerted on ECs, of which it promotes proliferation, migration, and survival, so that a positive correlation between VEGF, microvascular density, and clinical outcome has been frequently reported (39, 40). Another critical pro-angiogenic factor found was Von Willebrand Factor (VWF), a multimeric plasma glycoprotein that assist platelet adhesion to EC surface and subendothelial matrix, acting also as a circulating carrier for coagulation factor VIII (41). VWF is stored within the Weibel–Palade bodies in ECs, whose activation determines its release in tumor microenvironment and blood circulation (42). This, in turn, drives platelet recruitment, aggregation, and activation, with the consequent release of pro-angiogenic platelet content, in a self-sustaining autocrine and paracrine cycle (42). Previous studies by our research team demonstrated for the first time that GBM patients presenting a preoperative VWF: Ag higher than a specifically identified cutoff experience a poorer prognosis, with a threefold higher risk of death (37).
Finally, it has been widely reported that ECs express relatively high levels of VE-cadherin (43), which is actively involved in angiogenesis, inflammation, regeneration, vasculogenesis, and tumor progression (44).
Therefore, we used GECs as a reliable model of GBM angiogenesis, and we proceeded with the examination of calpain expression in GECs, revealing an upregulation of the most active calpains, CAPN1 and CAPN2, and their small regulator subunits (CAPNS1 and CAPNS2), together with a downregulation of CAST, their endogenous inhibitor. Notably, the overexpression of CAPN1 and CAPNS1 was found to significantly correlate with patient OS, suggesting their potential to serve as novel prognostic biomarkers. Our data are consistent with those published on the Human Protein Atlas, which reports a statistically significant increase of glioma patients’ survival associated with a lower gene expression in a cohort of n=153 patients (p=0.048).
Calpains residing in the ECs are key participants of tumor angiogenesis. Their levels are induced by factors, primarily VEGF, whose axis VEGF/VEGFR2 has been found to stimulate calpain-2 dependent activation of PI3K/AMPK/Akt/eNOS pathway and consequent nitric oxide production and physiological angiogenesis (14). Hypoxic conditions characterizing aggressive tumors as GBM represent a further enhancer of calpain contribution in this pro-angiogenic self-vicious cycle.
The rapid progression of GBM necessitates a huge amount of oxygen and nutrients, supplied by angiogenic processes and pro-angiogenic factor secretion. The abundance and the key role of VEGF in this mechanism have been widely discussed, but it has been reported that VEGF in ECs is effective in activating calpain-2, so the administration of calpain inhibitors or siRNA may abolish VEGF-induced endothelial NO production and therefore angiogenesis (45–48). Furthermore, the faster growth of tumor cells due to hypoxic conditions leads to the upregulation of calpain expression and activity in ECs (49–51). Interestingly, calpain in tumor cells serves as a newly identified regulator of the hypoxia-inducible-factor (HIF-l α)/VEGF pathway (52). In particular, HIF-1 is known to induce transcription of more than 60 genes, including VEGF and erythropoietin, which assist in promoting and increasing oxygen delivery to hypoxic regions, thus promoting tumor progression.
For example, Zheng et al. have shown that hypoxia promotes calpain-induced filamin-A proteolysis in melanoma cells, which in turn facilitates HIF-1α nuclear translocation. In a tumor xenograft model, it has been observed that since VEGF is transcriptionally activated by HIF-1α, the overexpression of filamin-A is able to increase HIF-1α recruitment to VEGF promoter, thus promoting tumor angiogenesis. However, calpeptin inhibition of calpain attenuates HIF-1α nuclear accumulation and transactivation (52).
In order to investigate the involvement of calpains in tumor angiogenesis, Miyazaki et al. examined tumors and surrounding normal tissues from patients suffering from malignant astrocytoma, colon, and lung adenocarcinomas. The analysis of calpastatin immunostaining proved a significant loss of expression in tumor ECs compared to normal vessels. Furthermore, using mice harboring EC-specific transgene of calpastatin, the same authors observed a weakening of tumor angiogenesis in a Lewis lung carcinoma allograft transplantation model, potentially mediated by the inhibition of VEGF-C production through calpain/SOCS3/STAT3 (53).
Following this promising evidence about the potential pro-angiogenic role of calpains, we decided to test calpain inhibitors in order to restore the physiological condition and overcome GBM angiogenesis. To this aim, calpain inhibitors 1–2 and calpastatin were administered to GECs (Figure 9).
Figure 9 Schematic representation of study hypothesis: the hyperactivation of calpain signaling, mainly mediated by the binding of proliferative growth factors to their membrane receptors, contributes to aberrant GBM angiogenesis. Our data, consistent with scientific literature, suggest that the administration of calpain inhibitors, especially calpain inhibitor-1, may impair angiogenic mechanisms overcoming tumor invasiveness, progression, and infiltrative behavior.
Our data demonstrated for the first time on primary GBM-derived endothelial cells, a great ability of calpain inhibitors and calpastatin to slow down GEC proliferation and survival, by inducing also apoptotic mechanisms mediated by caspase-3 and caspase-7 activation. Notably, calpain inhibitors succeeded also in inhibiting GEC functionality, as migration and angiogenesis in vitro. The most robust effect was observed after the administration of calpain inhibitor 1, which was able to strongly arrest cell proliferation and viability and counteract tube-like structure formation and cell migration. Of relevance, the investigation of the potential molecular mechanisms underlying these effects revealed a downregulation of MAPK and an upregulation of pro-apoptotic mediators as BAX family. The Ras/RAF/MEK/ERK (MAPK) signaling represents one of the best-characterized pathways in cancer biology, and its hyperactivation is involved in over 40% of human cancer cases. The MAPK signaling acts by switching on proliferative genes controlling cellular overgrowth and simultaneously enables cells to overcome metabolic stress by inhibiting AMPK signaling. Mechanistically, upon binding of RTKs or other stimulations, Ras small GTPases are activated by GTP/GDP exchange factors (GEFs), which in turn recruit RAF/MEK complexes to the plasma membrane and trigger the RAF/MEK/ERK kinase cascade (54).
The translocation of active ERKs into the nuclei or in the cytoplasm induces the phosphorylation of substrates implicated in cell functions, such as proliferation and survival (55–57). Hence, the aberrant activation of MAPK signaling frequently induces proliferative disorders as human cancers, as what happens in GBM (58, 59). In our data, the downregulation of MAPK is complemented by the Bcl-2 reduced expression, which impacts cell death mechanisms, including apoptosis, autophagy, and necrosis, thus operating as nodal points at the junction of multiple crucial pathways in oncology. The overexpression of Bcl-2 family proteins causes the inhibition of cell death induced by hypoxia, growth factor deprivation, and oxidative stress, so the effect of calpain inhibitors in decreasing Bcl-2 may explain the induction of apoptosis (60). These results have been further confirmed by the overexpression of Bax and Bid, the pro-apoptotic members of Bcl-2 family, and those of caspase-3 and caspase-7, the major executioner caspases of apoptosis mechanisms. Irrespective of the specific death-initiating stimulus, caspase-3 and caspase-7 are both universally activated during apoptosis, coordinating the demolition phase of apoptosis by cleaving a diverse subset of protein substrates (61). Interesting data arise also from the upregulation of p53, p21, and p27 by calpain inhibitors. The TP53 gene encodes a protein acting as a transcription factor crucial for carcinogenesis. The inactivating mutation of TP53 is frequently detected in human cancers. The role of p53 consists in tumor suppression in response to cellular stress. The presence of the heritable TP53 mutant allele is responsible for the Li–Fraumeni disease, which predisposes patients to the development of different types of malignant tumors (62). With a similar mechanism, both p21and p27 inhibit cell cycle acting as an anti-proliferative mediators, so their deregulation, accelerated degradation, or mislocalization are often found in many cancers (63). Finally, calpain inhibitors were able to interfere with VEGF signaling, downregulating its expression and that of its two major receptors, VEGFR1 and VEGFR2, suggesting the disruption of the most active pro-angiogenic axis.
Most of the scientific literature on the relation between calpains and cancer are focused on the correlation between their expression and patient prognosis. The limited number of reports investigating their blockade are still controversial, as although there are numerous protumoral pathways induced by calpains, they are able to sensitize cancer cell to chemotherapy (18). According to our results, it was observed that calpain inhibition with calpeptin, and with a synthetic calpain inhibitor (ALLN), was able to suppress cell cycle progression and proliferation of cancer transformed cells and their anchorage-independent growth. Similarly, the inhibition of calpain activity by different inhibitors was effective in repressing the effects of the transformation induced by other oncoproteins such as v-Jun, v-Myc, v12k-Ras, and v-Fos (64). Furthermore, it has been reported that the simultaneous inhibition of calpains and ERK/MAPK pathway coupled with an activation of p38 MAPK was sufficient to restore the ability of v-Src-transformed myoblasts to differentiate (65). Interestingly, calpain inhibition was also shown to induce apoptosis of transformed cells, thanks to an accumulation of c-Myc, previously identified as a calpain substrate (66).
Furthermore, calpain inhibition, with calpeptin for example, proved to reduce lung cancer cell invasiveness by impeding cancer cell migration (67). Interestingly, the addition of C2-ceramide activating the phosphatase PP2A induces calpain dephosphorylation and inactivation, with a functional impairment of tumor invasion (68). In addition, the inhibition of m-calpain using calpain inhibitor I reduces the invasiveness of prostate carcinoma cells (69). Very similar results were obtained with rhabdomyosarcoma treated with calpeptin. Indeed, the invasiveness of these cells was dramatically reduced in the presence of calpeptin, restoring a condition close to normal myoblasts (70). Of relevance, the inhibition of calpains can also diminish the expression of metalloproteinases (MMPs). Indeed, the treatment of leukemic cells with the specific inhibitor CP1B, derived from calpastatin, affects the expression and secretion of MMP-2/MMP-9, reducing matrix degradation and thus tumor invasion (71).
Overall, the activity of calpain-1 and calpain-2, the two ubiquitous calpains, could be impaired by targeted treatment to impede cell transformation, suppress the enhanced motility, adhesion disassembly and the cell cycle progression, and by inducing tumor cell death. Interestingly, it has been reported that the inhibition of calpain activity could also be useful to improve the sensitivity of lung cancer cells to the proteasome inhibitor bortezomib (72). It is possible that while calpain inhibition may decrease apoptosis, the cells may be redirected to other modes of death. Still, the sum of the published data suggests that an interesting approach would be to target calpains to improve chemotherapy efficiency.
Furthermore, targeting calpain activity with specific inhibitors could be a novel approach to limiting the development of primary tumors and the formation of metastases, by inhibiting tumor cell migration and invasion, which allows dissemination and tumor neovascularization, which in turn allows tumor progression.
Taken together, our results led to the awareness that molecular mechanisms underlying GBM malignancy and aggressiveness need to be investigated deeper. Targeting calpains may be considered as a novel frontier of molecular target therapies, which may benefit from the molecular screening and consequent patient stratification. The development of target therapies for patients with brain cancer, through the modulation of angiogenesis, invasiveness, and pharmacological sensitivity/resistance, is urgently needed in the era of precision medicine. Furthermore, the discovery of novel molecular mediators, from genetics to epigenetics and proteomics, as potential prognostic and predictive biomarkers may be handy and recognizable by an “omic” approach and may impact clinical practice in terms of patient management.
The raw data supporting the conclusions of this article will be made available by the authors, without undue reservation.
The Institutional Review Board of Fondazione IRCCS Ca’ Granda Ospedale Maggiore Policlinico approved the protocol (IRB#1670/2015) and all patients provided a signed informed consent. The studies were conducted in accordance with the local legislation and institutional requirements. The participants provided their written informed consent to participate in this study.
LG: Conceptualization, Data curation, Formal analysis, Funding acquisition, Investigation, Methodology, Project administration, Validation, Visualization, Writing – original draft. SN: Conceptualization, Data curation, Formal analysis, Funding acquisition, Investigation, Methodology, Project administration, Validation, Visualization, Writing – original draft. LB: Data curation, Investigation, Methodology, Validation, Writing – review & editing. EB: Formal analysis, Supervision, Validation, Writing – review & editing. EG: Supervision, Validation, Writing – review & editing. RC: Data curation, Formal analysis, Investigation, Supervision, Validation, Writing – review & editing. MM: Investigation, Supervision, Writing – review & editing. LF: Supervision, Validation, Writing – review & editing. GA: Formal analysis, Supervision, Validation, Writing – review & editing. CC: Data curation, Methodology, Supervision, Formal analysis, Validation, Visualization, Software, Writing – review & editing. CG: Data curation, Supervision, Formal analysis, Validation, Writing – review & editing. LS: Resources, Validation, Writing – review & editing. AA: Resources, Validation, Writing – review & editing. LR: Formal analysis, Investigation, Supervision, Validation, Writing – review & editing. ML: Funding acquisition, Project administration, Resources, Supervision, Writing – review & editing. GM: Conceptualization, Data curation, Funding acquisition, Investigation, Project administration, Resources, Supervision, Validation, Writing – review & editing.
The author(s) declare financial support was received for the research, authorship, and/or publication of this article. This research was funded by Italian Ministry of Health, RC2022, RC2023, RC2024 and GR-2019-12370940, and by Fondazione Cariplo, 2019-1737.
We are immensely grateful to A-Tono: The World in Your Hand for the precious help in conducting and disseminating our research activity and to Associazione Amici della Neurochirurgia del Policlinico di Milano for the support of our scientific activity.
Authors EB, GA, LR have been involved, as researchers, thanks to the participation of Andremacon Srl in the study.
The remaining authors declare that the research was conducted in the absence of any commercial or financial relationships that could be construed as a potential conflict of interest.
The author(s) declared that they were an editorial board member of Frontiers, at the time of submission. This had no impact on the peer review process and the final decision.
All claims expressed in this article are solely those of the authors and do not necessarily represent those of their affiliated organizations, or those of the publisher, the editors and the reviewers. Any product that may be evaluated in this article, or claim that may be made by its manufacturer, is not guaranteed or endorsed by the publisher.
1. Crocetti E, Trama A, Stiller C. Epidemiology of glial and non-glial brain tumours in Europe. Eur J Cancer. (2012) 48:1532–42. doi: 10.1016/j.ejca.2011.12.013
2. Kheirollahi M, Dashti S, Khalaj Z, Nazemroaia F, Mahzouni P. Brain tumors: Special characters for research and banking. Adv Biomed Res. (2015) 4:4. doi: 10.4103/2277-9175.148261
3. Siegel RL, Miller KD, Jemal A. Cancer statistics. Cancer J Clin. (2020) 70:7–30. doi: 10.3322/caac.21590
4. Lapointe S, Perry A, Butowski NA. Primary brain tumours in adults. Lancet. (2018) 392:432–46. doi: 10.1016/S0140-6736(18)30990-5
5. Nakamura M, Masutomi K, Kyo S, Hashimoto M, Maida Y, Kanaya T, et al. Efficient inhibition of human telomerase reverse transcriptase expression by RNA interference sensitizes cancer cells to ionizing radiation and chemotherapy. Hum Gene Ther. (2005) 16:859–68. doi: 10.1089/hum.2005.16.859
6. Stupp R, Mason WP, van den Bent MJ, Weller M, Fisher B, Taphoorn MJ, et al. Radiotherapy plus concomitant and adjuvant temozolomide for glioblastoma. N Engl J Med. (2005) 352:987–96. doi: 10.1056/NEJMoa043330
7. Grossman SA, Ye X, Piantadosi S, Desideri S, Nabors LB, Rosenfeld M, et al. Survival of patients with newly diagnosed glioblastoma treated with radiation and temozolomide in research studies in the United States. Clin Cancer Res. (2010) 16:2443–9. doi: 10.1158/1078-0432.CCR-09-3106
8. Taphoorn MJ, Sizoo EM. Bottomley, A, Review on quality of life issues in patients with primary brain tumors. Oncologist. (2010) 15:618–26. doi: 10.1634/theoncologist.2009-0291
9. Nishida N, Yano H, Nishida T, Kamura T, Kojiro M. Angiogenesis in cancer. Vasc Heal Risk Manag. (2006) 2:213–9. doi: 10.2147/vhrm.2006.2.3.213
10. De Bock K, Cauwenberghs S, Carmeliet P. Vessel abnormalization: another hallmark of cancer? Molecular mechanisms and therapeutic implications. Curr Opin Genet Dev. (2011) 21:73–9. doi: 10.1016/j.gde.2010.10.008
11. Carmeliet P, Ferreira V, Breier G, Pollefeyt S, Kieckens L, Gertsenstein M, et al. Abnormal blood vessel development and lethality in embryos lacking a single VEGF allele. Nature. (1996) 380:435–9. doi: 10.1038/380435a0
12. Folkins C, Shaked Y, Man S, Tang T, Lee CR, Zhu Z, et al. Glioma tumor stem-like cells promote tumor angiogenesis and vasculogenesis via vascular endothelial growth factor and stromal-derived factor 1. Cancer Res. (2009) 69:7243–51. doi: 10.1158/0008-5472.CAN-09-0167
13. Baish JW, Stylianopoulos T, Lanning RM, Kamoun WS, Fukumura D, Munn LL, et al. Scaling rules for diffusive drug delivery in tumor and normal tissues. Proc Natl Acad Sci U.S.A. (2011) 108:1799–803. doi: 10.1073/pnas.1018154108
14. Zhang Y, Liu NM, Wang Y, Youn JY, Cai H. Endothelial cell calpain as a critical modulator of angiogenesis. Biochim Biophys Acta Mol Basis Dis. (2017) 1863:1326–35. doi: 10.1016/j.bbadis.2017.03.021
15. Emori Y, Kawasaki H, Imajoh S, Imahori K, Suzuki K. Endogenous inhibitor for calcium-dependent cysteine protease contains four internal repeats that could be responsible for its multiple reactive sites. Proc Natl Acad Sci U.S.A. (1987) 84:3590–4. doi: 10.1073/pnas.84.11.3590
16. Moldoveanu T, Gehring K, Green DR. Concerted multi-pronged attack by calpastatin to occlude the catalytic cleft of heterodimeric calpains. Nature. (2008) 456:404–8. doi: 10.1038/nature07353
17. Kulkarni S, Saju L, Farver C, Tubbs R. Calpain4 is required for activation of HER2 in breast cancer cells exposed to trastuzumab and its suppression decreases survival and enhances response. Int J Cancer. (2012) 131:2420–32. doi: 10.1002/ijc.27510
18. Wang H, Guo Z, Wu F, Long F, Cao X, Liu B, et al. PKA-mediated protein phosphorylation protects ezrin from calpain I cleavage. Biochem Biophys Res Commun. (2005) 333:496–501. doi: 10.1016/j.bbrc.2005.05.143
19. Libertini SJ, Robinson BS, Dhillon NK, Glick D, George M, Dandekar S, et al. Cyclin E both regulates and is regulated by calpain 2, a protease associated with metastatic breast cancer phenotype. Cancer Res. (2005) 65:10700–8. doi: 10.1158/0008-5472.CAN-05-1666
20. Lakshmikuttyamma A, Selvakumar P, Kanthan R, Kanthan SC, Sharma RK. Overexpression of m-calpain in human colorectal adenocarcinomas. Cancer Epidemiol Biomarkers Prev. (2004) 12:1604–9. doi: 10.1158/1055-9965.1604.13.10
21. Zhuang Q, Qian X, Cao Y, Fan M, Xu X, He X. Capn4 mRNA level is correlated with tumour progression and clinical outcome in clear cell renal cell carcinoma. J Int Med Res. (2014) 42:282–91. doi: 10.1177/0300060513505524
22. Chen B, Tang J, Guo YS, Li Y, Chen ZN, Jiang J. Calpains are required for invasive and metastatic potentials of human HCC cells. Cell Biol Int. (2013) 37:643–52. doi: 10.1002/cbin.10062
23. Zheng PC, Chen X, Zhu HW, Zheng W, Mao LH, Lin C, et al. Capn4 is a marker of poor clinical outcomes and promotes nasopharyngeal carcinoma metastasis via nuclear factor-κB-induced matrix metalloproteinase 2 expression. Cancer Sci. (2014) 105:630–8. doi: 10.1111/cas.12416
24. Niapour M, Farr C, Minden M, Berger SA. Elevated calpain activity in acute myelogenous leukemia correlates with decreased calpastatin expression. Blood Cancer J. (2012) 2:e51. doi: 10.1038/bcj.2011.50
25. Kimura Y, Koga H, Araki N, Mugita N, Fujita N, Takeshima H, et al. The involvement of calpain-dependent proteolysis of the tumor suppressor NF2 (merlin) in schwannomas and meningiomas. Nat Med. (1998) 4:915–22. doi: 10.1038/nm0898-915
26. Cai JJ, Qi ZX, Hua W, Zhu JJ, Zhang X, Yao Y, et al. Increased expression of Capn4 is associated with the Malignancy of human glioma. CNS Neurosci Ther. (2014) 20:521–7. doi: 10.1111/cns.12248
27. Salehin D, Fromberg I, Haugk C, Dohmen B, Georg T, Bohle RM, et al. Immunhistochemical analysis for expression of calpain 1, calpain 2 and calpastatin in endometrial cancer. Anticancer Res. (2010) 30:2837–43.
28. Storr SJ, Mohammed RA, Woolston CM, Green AR, Parr T, Spiteri I, et al. Calpastatin is associated with lymphovascular invasion in breast cancer. Breast. (2011) 2011:413–8. doi: 10.1016/j.breast.2011.04.002
29. Storr SJ, Pu X, Davis J, Lobo D, Reece-Smith AM, Parsons SL, et al. Expression of the calpain system is associated with poor clinical outcome in gastro-oesophageal adenocarcinomas. J Gastroenterol. (2013) 48:1213–21. doi: 10.1007/s00535-012-0743-4
30. Storr SJ, Zaitoun AM, Arora A, Durrant LG, Lobo DN, MadhuSudan S. Calpain system protein expression in carcinomas of the pancreas, bile duct and ampulla. BMC Cancer. (2012) 12:511. doi: 10.1186/1471-2407-12-511
31. Guarnaccia L, Navone SE, Trombetta E, Cordiglieri C, Cherubini A, Crisà FM, et al. Angiogenesis in human brain tumors: Screening of drug response through a patient-specific cell platform for personalized therapy. Sci Rep. (2018) 8:1–13. doi: 10.1038/s41598-018-27116-7
32. Navone SE, Guarnaccia L, Cordiglieri C, Crisà FM, Caroli M, Locatelli M, et al. Aspirin affects tumor angiogenesis and sensitizes human glioblastoma endothelial cells to temozolomide, bevacizumab, and sunitinib, impairing vascular endothelial growth factor-related signaling. World Neurosurg. (2018) 120:e380–91. doi: 10.1016/j.wneu.2018.08.080
33. Navone SE, Marfia G, Invernici G, Cristini S, Nava S, Balbi S. Isolation and expansion of human and mouse brain microvascular endothelial cells. Nat Protoc. (2013) 8:1680–93. doi: 10.1155/2019/2617030
34. Pesenti C, Navone SE, Guarnaccia L, Terrasi A, Costanza J, Silipigni R, et al. The genetic landscape of human glioblastoma and matched primary cancer stem cells reveals intratumour similarity and intertumour heterogeneity. Stem Cells Int. (2019) 2019:2617030. doi: 10.1155/2019/2617030
35. Fontana L, Tabano S, Bonaparte E, Marfia G, Pesenti C, Falcone R. MGMT-Methylated alleles are distributed heterogeneously within glioma samples irrespective of IDH status and chromosome 10q deletion. J Neuropathol Exp Neurol. (2016) 75:791–800. doi: 10.1093/jnen/nlw052
36. Marfia G, Navone SE, Fanizzi C, Tabano S, Pesenti C, Abdel Hadi L, et al. Prognostic value of preoperative von Willebrand factor plasma levels in patients with Glioblastoma. Cancer Med. (2016) 5:1783–90. doi: 10.1002/cam4.747
37. Olsson AK, Dimberg A, Kreuger J. VEGF receptor signalling - in control of vascular function. Nat Rev Mol Cell Biol. (2006) 7:359–71. doi: 10.1038/nrm1911
38. Ferrara N. VEGF-A: a critical regulator of blood vessel growth. Eur Cytokine Netw. (2009) 20:158–63. doi: 10.1684/ecn.2009.0170
39. Takano S, Yoshii Y, Kondo S, Suzuki H, Maruno T, Shirai S. Concentration of vascular endothelial growth factor in the serum and tumor tissue of brain tumor patients. Cancer Res. (1996) 56:2185–90.
40. Fidler IJ, Ellis LM. Neoplastic angiogenesis–not all blood vessels are created equal. N Engl J Med. (2004) 351:215–6. doi: 10.1056/NEJMp048080
41. Bauer AT, Suckau J, Frank K, Desch A, Goertz L, Wagner AH, et al. von Willebrand factor fibers promote cancer-associated platelet aggregation in Malignant melanoma of mice and humans. Blood. (2015) 125:3153–63. doi: 10.1182/blood-2014-08-595686
42. Campanella R, Guarnaccia L, Cordiglieri C, Trombetta E, Caroli M, Carrabba G, et al. Tumor-educated platelets and angiogenesis in glioblastoma: another brick in the wall for novel prognostic and targetable biomarkers, changing the vision from a localized tumor to a systemic pathology. Cells. (2020) 9:294. doi: 10.3390/cells9020294
43. Etienne-Manneville S. P120catenin tuning of VE-cadherin endocytosis controls collective cell behavior during angiogenesis. J Cell Biol. (2020) 219(5):e202003005. doi: 10.1083/jcb.202003005
44. Viallard C, Larrivée B. Tumor angiogenesis and vascular normalization: alternative therapeutic targets. Angiogenesis. (2017) 20:409–26. doi: 10.1007/s10456-017-9562-9
45. Youn JY, Wang T. An ezrin/calpain/PI3K/AMPK/eNOSs1179 signaling cascade mediating VEGF-dependent endothelial nitric oxide production. Circ Res. (2009) 104:50–9. doi: 10.1161/CIRCRESAHA.108.178467
46. Su Y, Cui Z, Li Z. Calpain-2 regulation of VEGF-mediated angiogenesis. FASEB J. (2006) 20:1443–51. doi: 10.1096/fj.05-5354com
47. Zhang Y, Li Q, Youn JY. Protein phosphotyrosine phosphatase 1B (PTP1B) in calpain-dependent feedback regulation of vascular endothelial growth factor receptor (VEGFR2) in endothelial cells: IMPLICATIONS IN VEGF-DEPENDENT ANGIOGENESIS AND DIABETIC WOUND HEALING. J Biol Chem. (2017) 292:407–16. doi: 10.1074/jbc.M116.766832
48. Hein TW, Rosa RH Jr, Ren Y, Xu W. VEGF receptor-2-linked PI3K/calpain/SIRT1 activation mediates retinal arteriolar dilations to VEGF and shear stress. Invest Ophthalmol Vis Sci. (2015) 56:5381–9. doi: 10.1167/iovs15-16950
49. Hoang MV, Smith LE. Calpain inhibitors reduce retinal hypoxia in ischemic retinopathy by improving neovascular architecture and functional perfusion. Biochim Biophys Acta. (2011) 1812:549–57. doi: 10.1016/j.bbadis.2010.08.008
50. Mo XG, Chen QW, Li XS, Zheng MM, Ke DZ, Deng W, et al. Suppression of NHE1 by small interfering RNA inhibits HIF-1α-induced angiogenesis in vitro via modulation of calpain activity. Microvasc Res. (2011) 81:160–8. doi: 10.1016/j.mvr.2010.12.004
51. Aono Y, Ariyoshi H, Tsuji Y, Ueda A, Tokunaga M, Sakon M. Localized activation of m-calpain in human umbilical vein endothelial cells upon hypoxia. Thromb Res. (2001) 102:353–61. doi: 10.1016/S0049-3848(01)00238-9
52. Zheng X, Zhou AX, Rouhi P, Uramoto H, Borén J, Cao Y, et al. Hypoxia-induced and calpain-dependent cleavage of filamin A regulates the hypoxic response. Proc Natl Acad Sci U.S.A. (2014) 111:2560–5. doi: 10.1073/pnas.1320815111
53. Miyazaki T, Taketomi Y, Saito Y, Hosono T, Lei XF, Kim-Kaneyama JR, et al. Calpastatin counteracts pathological angiogenesis by inhibiting suppressor of cytokine signaling 3 degradation in vascular endothelial cells. Circ Res. (2015) 116:1170–81. doi: 10.1161/CIRCRESAHA.116.305363
54. Wojnowski L, Stancato LF, Larner AC, Rapp UR. Overlapping and specific functions of Braf and Craf-1 proto-oncogenes during mouse embryogenesis. Mech Dev. (2000) 91:97–104. doi: 10.1016/S0925-4773(99)00276-2
55. Baumann B, Weber CK, Troppmair J, Whiteside S, Israel A, Rapp UR. Raf induces NF-kappaB by membrane shuttle kinase MEKK1, a signaling pathway critical for transformation. Proc Natl Acad Sci U.S.A. (2000) 97:4615–200. doi: 10.1073/pnas.080583397
56. Zhang Z, Kobayashi S, Borczuk AC, Leidner RS, Laframboise T, Levine AD. Dual specificity phosphatase 6 (DUSP6) is an ETS-regulated negative feedback mediator of oncogenic ERK signaling in lung cancer cells. Carcinogenesis. (2010) 31:577–86. doi: 10.1093/carcin/bgq020
57. Ekerot M, Stavridis MP, Delavaine L, Mitchell MP, Staples C, Owens DM. Negative-feedback regulation of FGF signalling by DUSP6/MKP-3 is driven by ERK1/2 and mediated by Ets factor binding to a conserved site within the DUSP6/MKP-3 gene promoter. Biochem J. (2008) 412:287–98. doi: 10.1042/BJ20071512
58. Valis K. Targeting ERK-Hippo interplay in cancer therapy. Int J Mol Sci. (2020) 21:3236. doi: 10.3390/ijms21093236
59. Unni AM, Harbourne B, Oh MH, Wild S, Ferrarone JR, Lockwood WW. Hyperactivation of ERK by multiple mechanisms is toxic to RTK-RAS mutation-driven lung adenocarcinoma cells. Elife. (2018) 7:e33718. doi: 10.7554/eLife.33718.022
60. Yip KW. Bcl-2 family proteins and cancer. Oncogene. (2008) 27:6398–406. doi: 10.1038/onc.2008.307
62. Boutelle AM, Attardi LD. p53 and tumor suppression: it takes a network. Trends Cell Biol. (2021) 31:298–310. doi: 10.1016/j.tcb.2020.12.011
63. Chu IM, Hengst L. The Cdk inhibitor p27 in human cancer: prognostic potential and relevance to anticancer therapy. Nat Rev Cancer. (2008) 8:253–67. doi: 10.1038/nrc2347
64. Carragher NO, Fonseca BD. Calpain activity is generally elevated during transformation but has oncogene-specific biological functions. Neoplasia. (2004) 6:53–73. doi: 10.1016/S1476-5586(04)80053-8
65. Ciuffini L, Castellani L, Salvati E, Galletti S, Falcone G. Delineating v-Src downstream effector pathways in transformed myoblasts. Oncogene. (2008) 27:528–39. doi: 10.1038/sj.onc.1210665
66. Small GW, Chou TY, Dang CV. Evidence for involvement of calpain in c-Myc proteolysis in vivo. Arch Biochem Biophys. (2002) 400:151–61. doi: 10.1016/S0003-9861(02)00005-X
67. Xu L. Tobacco-specific nitrosamine 4-(methylnitrosamino)-1-(3-pyridyl)-1-butanone induces phosphorylation of mu- and m-calpain in association with increased secretion, cell migration, and invasion. J Biol Chem. (2004) 279:53683–90. doi: 10.1074/jbc.M409889200
68. Bertoli C, Copetti T, Lam EW, Demarchi F. Calpain small-1 modulates Akt/FoxO3A signaling and apoptosis through PP2A. Oncogene. (2009) 28:721–3. doi: 10.1038/onc.2008.425
69. Mamoune A, Luo JH, Lauffenburger DA. Calpain-2 as a target for limiting prostate cancer invasion. Cancer Res. (2003) 63:4632–40.
70. Roumes H, Leloup L, Dargelos E, Brustis JJ, Daury L. Calpains: markers of tumor aggressiveness? Exp Cell Res. (2010) 316:1587–99. doi: 10.1016/j.yexcr.2010.02.017
71. Popp O, Heidinger M, Ruiz-Heinrich L, Ries C, Jochum M. The calpastatin-derived calpain inhibitor CP1B reduces mRNA expression of matrix metalloproteinase-2 and -9 and invasion by leukemic THP-1 cells. Biol Chem. (2003) 384:951–8. doi: 10.1515/BC.2003.107
Keywords: glioblastoma, angiogenesis, calpains, tumor endothelial cells, target therapy
Citation: Guarnaccia L, Navone SE, Begani L, Barilla E, Garzia E, Campanella R, Miozzo M, Fontana L, Alotta G, Cordiglieri C, Gaudino C, Schisano L, Ampollini A, Riboni L, Locatelli M and Marfia G (2024) Testing calpain inhibition in tumor endothelial cells: novel targetable biomarkers against glioblastoma malignancy. Front. Oncol. 14:1355202. doi: 10.3389/fonc.2024.1355202
Received: 13 December 2023; Accepted: 01 July 2024;
Published: 02 August 2024.
Edited by:
Leonora Balaj, Massachusetts General Hospital and Harvard Medical School, United StatesReviewed by:
Omer Faruk Hatipoglu, Kindai University Hospital, JapanCopyright © 2024 Guarnaccia, Navone, Begani, Barilla, Garzia, Campanella, Miozzo, Fontana, Alotta, Cordiglieri, Gaudino, Schisano, Ampollini, Riboni, Locatelli and Marfia. This is an open-access article distributed under the terms of the Creative Commons Attribution License (CC BY). The use, distribution or reproduction in other forums is permitted, provided the original author(s) and the copyright owner(s) are credited and that the original publication in this journal is cited, in accordance with accepted academic practice. No use, distribution or reproduction is permitted which does not comply with these terms.
*Correspondence: Stefania Elena Navone, c3RlZmFuaWEubmF2b25lQHBvbGljbGluaWNvLm1pLml0
Disclaimer: All claims expressed in this article are solely those of the authors and do not necessarily represent those of their affiliated organizations, or those of the publisher, the editors and the reviewers. Any product that may be evaluated in this article or claim that may be made by its manufacturer is not guaranteed or endorsed by the publisher.
Research integrity at Frontiers
Learn more about the work of our research integrity team to safeguard the quality of each article we publish.