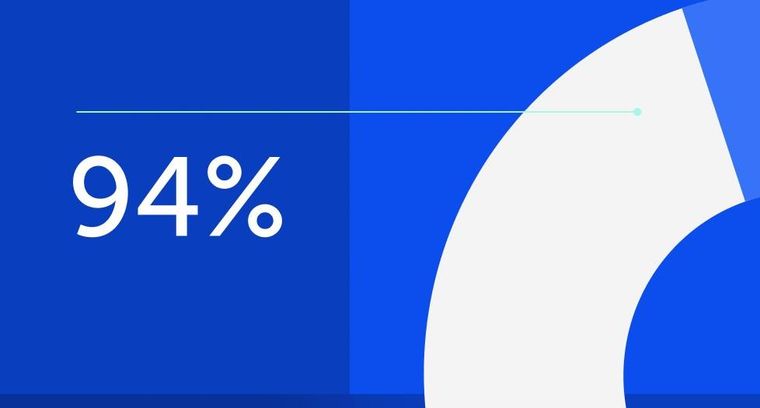
94% of researchers rate our articles as excellent or good
Learn more about the work of our research integrity team to safeguard the quality of each article we publish.
Find out more
REVIEW article
Front. Oncol., 15 February 2024
Sec. Head and Neck Cancer
Volume 14 - 2024 | https://doi.org/10.3389/fonc.2024.1353800
Head and neck cancer continues to be among the most prevalent types of cancer globally, yet it can be managed with appropriate treatment approaches. Presently, chemotherapy and radiotherapy stand as the primary treatment modalities for various groups and regions affected by head and neck cancer. Nonetheless, these treatments are linked to adverse side effects in patients. Moreover, due to tumor resistance to multiple drugs (both intrinsic and extrinsic) and radiotherapy, along with numerous other factors, recurrences or metastases often occur. Electrochemotherapy (ECT) emerges as a clinically proven alternative that offers high efficacy, localized effect, and diminished negative factors. Electrochemotherapy involves the treatment of solid tumors by combining a non-permeable cytotoxic drug, such as bleomycin, with a locally administered pulsed electric field (PEF). It is crucial to employ this method effectively by utilizing optimal PEF protocols and drugs at concentrations that do not possess inherent cytotoxic properties. This review emphasizes an examination of diverse clinical practices of ECT concerning head and neck cancer. It specifically delves into the treatment procedure, the choice of anti-cancer drugs, pre-treatment planning, PEF protocols, and electroporation electrodes as well as the efficacy of tumor response to the treatment and encountered obstacles. We have also highlighted the significance of assessing the spatial electric field distribution in both tumor and adjacent tissues prior to treatment as it plays a pivotal role in determining treatment success. Finally, we compare the ECT methodology to conventional treatments to highlight the potential for improvement and to facilitate popularization of the technique in the area of head and neck cancers where it is not widespread yet while it is not the case with other cancer types.
The incidence of head and neck cancer (HNC; including lip/oral cavity, larynx, oropharynx, hypopharynx, salivary glands, and nasopharynx) in Europe is approximately 21.8 per 100,000, with a mortality rate of approximately 15.6 per 100,000 (1). Squamous cell carcinoma is the most common type of cancer in the HNC region; it accounts for more than 90% of the cases (2). Smoking and heavy alcohol consumption are still the main risk factors, and their joint effect increases the risk for cancer even more (3). Furthermore, the underlying causes of some of the sites in the head and neck (H&N) region are changing. There has been a constant rise in the incidence of oropharyngeal cancers due to increasing rates of human papillomavirus infection (4). Over a hundred varieties of human papillomavirus (HPV) have been identified. The most oncogenic types are 16 and 18, which account for more than 90% of cases of oropharyngeal cancer related to HPV (5).
As can be seen in Figure 1, H&N cancer accounts for 5% of all cancer cases worldwide (6). Although H&N cancer accounts for a smaller percentage compared to other cancers, its incidence rate continues to rise. It is estimated that by 2030 the incidence rate will rise by 30% annually (7, 8).
Based on the precise anatomical sites where the cancer arises, head and neck cancer is often divided into several main subregions (Table 1). These subregions are important for diagnosing and treating the cancer effectively. Different regions usually have distinct types of squamous cell carcinoma (SCC), i.e., cancer differs in the larynx vs. that in the oral cavity, etc.
The most common site for H&N cancer is the oral cavity (including the lip), which accounts for more than 40% of the new cases. The next is laryngeal cancer with almost 20% of all H&N tumors. At third place is nasopharyngeal cancer with almost 15%, followed by hypopharyngeal and oropharyngeal cancer with more than 10% and 9%, respectively. The smallest group of new cancer cases includes salivary gland cancers; they account for more than 5% (6, 9). The most common histopathological (HP) type in this oral and lip region is SCC; it accounts for over 90% of the cases (10). It is followed by rarer HP types, such as basal cell carcinoma (BCC), verrucous carcinoma, adenocarcinoma, adenoid cyst carcinoma, mucoepidermoid carcinoma, lymphoma, sarcoma, and melanoma (11). The majority of larynx malignancies (over 98%) are well differentiated SCC (12). The other types that can be found in HP assessment could be chondrosarcomas, leiomyosarcomas, and melanomas (13). The vast majority of oropharyngeal cancer cases are SCC (over 95%) (14). The other HP types that can be found in the oropharynx are melanoma, primary lymphoid malignant tumors, minor salivary gland tumors, sarcomas, etc. (15). The dominant HP type (over 95%) in the hypopharynx is SCC (16). Other tumor types include lymphoma, sarcoma, and adenocarcinoma (17). In other subsites, SCC carcinoma is found as well in the vast majority of nasopharyngeal cases (>95%) (18). The less frequent types of HP are adenocarcinoma and lymphoma (19). While SCC stands as the most prevalent cancer type, its behavior differs based on the site as well as the influencing developmental factors.
Despite advances in detection and therapy, more than 65% of people with head and neck squamous cell carcinoma have recurrent or metastatic disease (or both) (20). Distant metastases to the head and neck are uncommon compared to other regions, but in 20%–35% of instances, they may be the first sign of an otherwise undetected malignancy (21). Additionally, distant metastases significantly impact the prognosis, often signaling a widespread or disseminated disease. The standard treatment for the head and neck region depends on the site, often encompassing a surgical intervention if viable, along with radiotherapy, chemotherapy, or a combination of these methods. Despite a complex, site-specific treatment, a large number of patients will experience disease recurrence, with up to 60% of patients experiencing local failure and 30% experiencing distant failure (22). There is a high risk to develop a second primary tumor after remission. The risk is up to 2.18% in the follow-up period of 31 months and drops to 1.59% for a period of 10 years but does not decrease further in a period of 30 years (23).
The treatment of recurrences is extremely challenging for physicians. In many instances, radiotherapy is no longer a viable choice due to prior irradiation, potential toxicity, and apprehensions about the impacts on function and quality of life (24). For the patients, when all treatment options are exhausted, they are left with palliative treatment. This option usually involves chemotherapy. It might involve single-agent chemotherapy, combinations of platinum-based doublets, or even triplet drug options (25). However, this therapy typically fails to improve the overall survival rate and can reduce the quality of life (26). Due to these reasons, novel treatment methods are on high demand. Among these approaches is electrochemotherapy (ECT), which displays encouraging outcomes by demonstrating high effectiveness and minimal side effects while preserving organ function. Nevertheless, the number of publications (Clarivate Analytics Web of Science, CA WoS) that specifically addresses ECT in head and neck cancer is still low (Figure 2), while the application of ECT in the context of solid organs is by several orders of magnitude higher (27). Based on CA WoS, there are currently only 98 papers featuring the keywords “electroporation” and “head and neck cancer” (access date: 2023-11-06).
Figure 2 Number of publications and citations in electroporation head and neck cancer research area. Different colors represent the publication types.
This paper has reviewed the available literature, characterized the potential applications of ECT in H&N cancer context, systemized available clinical cases, and identified the limitations or challenges, which might affect the success rate of electroporation-based treatment for H&N cancer treatment.
An effective and viable alternative to traditional anticancer procedures lies in the utilization of electroporation-based treatment known as electrochemotherapy. ECT can serve as a valuable complement to enhance treatment outcomes while simultaneously reducing the side effects associated with conventional therapies (28). This approach ensures a safe and highly efficient procedure (75% to 99% tumor response) when employed in the treatment of subcutaneous and cutaneous lesions as well as metastases originating from various tumor types (28). ECT is a localized therapeutic approach, which combines the administration of electric pulses capable of temporary permeabilizing cell membrane and thus enables the intracellular delivery of chemotherapeutic drugs like bleomycin (BLM) or cisplatin (CP) (29). Adequate treatment planning ensures no harm to healthy cells or critical blood vessels in close proximity with the tumors (28), while the doses of drugs that are utilized by ECT are below minimal inhibitory concentrations if used without PEF, which minimizes the side effects and improves the tolerance of the procedure by patients. As a result, ECT can be effectively utilized in cases when the tumors are unresectable (30).
Additionally, ECT induces the release of damage-associated pattern molecules after treatment, leading to the increase of tumor antigens, which improves the immune reaction against tumor and increases the drug’s efficacy (31, 32). Immune checkpoint inhibitory therapy’s synergistic effects can further stimulate the antitumor immune response. Another phenomenon observed during ECT is the “vascular lock,” which specifically involves vascular disruption and hypoperfusion. The application of ECT creates a decreased blood flow in treated areas after the procedure. This increases the time of drug presence in the targeted volume and could prevent bleeding from well-vascularized structures (31). The principles of electrochemotherapy in the context of oral cancer are summarized in Figure 3.
The illustration of primary cancer refers to cancer that is not treated with electrochemotherapy and so requires a larger concentration of anti-cancer drugs to penetrate the cell membrane. While a larger concentration can be used, it may have a detrimental effect on healthy cells. Using a lower dose, on the other hand, increases the risk of primary tumor metastasis. At the same time, ECT provides localized treatment for cancerous tissues by utilizing reduced concentrations of anti-cancer drugs, thus avoiding damage to healthy tissues in the vicinity and thereby reducing the side effects and improving the quality of life for patients. Additionally, as mentioned above, ECT elicits an immune response and, due to destroyed blood vessels, helps prevent bleeding of the lesion following the treatment.
Currently, BLM, and cisplatin (CDDP) are the two drugs routinely used to treat cancer in the context of ECT (33). Bleomycin is a water-soluble glycopeptide antibiotic that breaks DNA, creating DNA fragmentation, chromosomal gaps, and deletions. So far, it has been employed in electroporation-based treatments of different cancers, including H&N (34). Cisplatin is a chemotherapeutic agent, which contains platinum. When cisplatin binds to DNA, it creates intrastrand and interstrand cross-links that cause DNA to adduct and prevent DNA replication and transcription and eventually cause cell death (35). When cisplatin enters the cell, it goes through a series of biochemical processes that produce its active metabolites, which can bind to DNA and crosslink it. Electroporation enhances cisplatin absorption into the cancer cells by increasing the DNA adduct production and boosting the cytotoxicity (36).
Other ECT agents, such as doxorubicin, 5-fluorouracil, gemcitabine, mitomycin C, and calcium, are also being introduced to the field (37). As an alternative to conventional ECT, the most promising so far is calcium ECT (38). The approach entails the precise intracellular delivery of cytotoxic calcium concentrations, causing ATP depletion, which results in cancer cell death and tumor necrosis (39). Additionally, elevated intracellular calcium levels open up mitochondrial pores, resulting in the dissipation of electrochemical gradient and formation of new ATPases (40). The other effect related to calcium and cell death is that it activates proteases and lipases and generates reactive oxygen species, which can also contribute to apoptosis (40, 41). Since calcium electroporation does not fall under the category of antineoplastic drugs, it is exempt from the precautions associated with these drugs with regard to storage, handling, disposal, toxicity, and mutagenicity. Calcium electroporation effectively induces tumor necrosis while causing less damage to normal tissue, making it a very promising ion for further clinical evaluation (42). It was already tested in clinical studies (43), including H&N cancer (44). Calcium is typically administered intratumorally, while BLM and CDDP are delivered either intratumorally or intravenously (37, 44).
Although many cancers respond to chemotherapy, a major obstacle to the treatment is multiple drug resistance (MDR). Based on the factors involved, drug resistance can be split into two categories: intrinsic resistance and extrinsic resistance (45). Intrinsic resistance implies the elements that are present in cancer cells or tissues themselves and responsible for lowering the efficiency of cancer chemotherapeutics prior to receiving chemotherapy. Extrinsic or acquired drug resistance, on the other hand, can arise during the treatment of tumors that were initially responsive to cytotoxic drugs and counteract their therapeutic effects as a result of a variety of adaptive responses, such as an increase in the expression of the therapeutic target and activation of alternative signaling pathways (45, 46). There are a lot of factors involved in MDR, and the main mechanisms of drug resistance are shown in Figure 4.
As can be seen in Figure 4, the main causes for MDR are as follows:
• Increased drug efflux: overexpression of ATP-binding cassette transporters (ABC), such as BCRL, MRP1, or P-glycoprotein (P-gp), which is one of the main causes of drug resistance. The intracellular concentration and effectiveness of chemotherapeutic medications are decreased by ABC transporting chemotherapy agents out of cancer cells (47, 48).
• Altered drug targets: Cancer cells may acquire genetic alterations that modify the targets of chemotherapy treatments, rendering them ineffective—for instance, mutations in drug target genes like the epidermal growth factor receptor (EGFR) in lung cancer, leading to resistance to EGFR inhibitors (49, 50).
• Inactivation of chemotherapy drugs: Cancer cells might produce enzymes capable of metabolizing chemotherapy agents and turning them into inactive variants. Resistance to platinum-based medicines, such as cisplatin, is characterized by an increased production of enzymes such as glutathione S-transferases (GSTs), which neutralizes the drug (48, 51).
• DNA repair mechanisms: In cancer cells, chemotherapy medicines cause DNA damage. However, enhanced DNA repair capacity can provide resistance by efficiently repairing drug-induced DNA damages via the activation of repair pathways such as nucleotide excision repair and homologous recombination (52, 53).
• Cell death inhibition: Cancer cells can develop a variety of ways to avoid chemotherapy-induced cell death, allowing them to survive and proliferate. Changes in apoptotic pathways (e.g., Bcl-2 protein family mutations), such as the overexpression of anti-apoptotic proteins, can prevent apoptosis and provide resistance to chemotherapy (54, 55). Furthermore, abnormalities in cell death signaling networks, such as the activation of survival pathways, might enhance cell survival and mitigate the cytotoxic effects of chemotherapy medications (56).
• Epigenetic modification: Epigenetic changes, such as DNA methylation and histone modifications, can affect gene expression patterns, contributing to drug resistance. These changes can quiet tumor suppressor genes or activate drug resistance genes, boosting cell survival and decreasing treatment sensitivity (57, 58).
The same issue arises not only with chemotherapy but also with radiotherapy. Radiotherapy resistance (RR) can occur due to multiple factors, including genetic mutations, altered DNA repair mechanisms, and changes in the tumor microenvironment (59). Among the various mechanisms proposed as potential reasons of RR, hypoxia has been emphasized. The presence of oxygen in the tissues enhances the effects of ionizing radiation; tumors with lower oxygen levels tend to exhibit greater resistance to ionizing radiation damage (60). Hypoxic regions are common in H&N SCC and may be related with resistance to ionizing radiation (61). Changes in various intracellular pathways, especially those involved in DNA repair, cell replication, cell cycle, and apoptosis, have been found to counteract ionizing radiation-induced cell death and so result in RR (62). There are numerous routes involved in H&N cancer RR, and it is one of the reasons why it is so difficult to overcome this obstacle. There is a need for new radio-sensitizing agents, but unfortunately most of the clinical trials fail during evaluation (63). There is a great need for alternative therapeutic modalities in the context of H&N cancer that will be able to overcome chemo/radio-resistance.
Electrochemotherapy is indicated as one of the viable options to control cancer MDR. Since ECT triggers the increased cell chemotherapeutic agent’s uptake, cancerous cells become less capable of maintaining their intracellular parts and suppress DNA repair capabilities (27). M. Condello et al. demonstrated that in vitro EP with mitomycin C increased the drug cytotoxicity in oral and laryngeal cancer cells with intrinsic drug resistance compared to a single treatment by inhibiting the protective mechanism of autophagy in favor of apoptosis (64). Cemazar et al. concluded that membrane restriction is the major obstacle to cisplatin cytotoxicity in cisplatin-resistant murine sarcoma cells. Their study showed that cells were equally sensitive to cisplatin ECT in vitro. Similarly, the in vivo results indicated an increased effectiveness of cisplatin in both parental and cisplatin-resistant tumors, though a significantly higher cure rate was assessed in cisplatin-sensitive compared to cisplatin-resistant tumors: 85% and 6%, respectively, at the same 8-mg/kg dose (65). Thus, MDR remains a challenge even in electroporation-based treatments. Recently, novel methods and hypothesis over MDR-related cell response in the context of ECT were proposed. The utilization of multi-drug cocktails, namely, the combinations of different anti-cancer agents, is also possible (66). Such approach decreases the probability of drug resistance and minimizes concurrent toxicity while effectively eliminating tumor cells. MDR may also be managed through modulation of PEF parameters. Shorter-duration electric pulses (nanosecond range) were shown to be more effective in countering drug resistance mechanisms compared to conventional European Standard Operating Procedures of Electrochemotherapy (ESOPE) protocols employing 100-µs pulse trains (66).
For locally or locoregionally limited head and neck squamous cell carcinoma (HNSCC), surgery, radiotherapy (RT), and chemotherapy (CT) are the main treatment techniques. Planning a course of treatment should maximize function preservation while aiming for the best curative strategy. A single-modality intervention can yield cure rates of more than 80% for individuals with a small primary malignancy that involves just one node or with no clinical nodal involvement (resection or radiation) (67). Surgical resection followed by adjuvant RT or concurrent chemoradiation (CRT) with salvage surgery acting as salvage therapy—also known as the organ preservation approach—remains the cornerstone of treatment for locoregionally progressed HNSCC (68). However, every case is unique, and treatment is tailored to each patient, e.g., in the oral cavity, stage I and II cancers are usually treated with primary surgery or RT, while stage III and IV cases are treated with surgery followed by RT with or without CRT (69). The treatment method is dependent on the anatomical site, cancer type, stage of the disease, and general health of the patient.
Besides the benefits, most treatments have their own limitations and disadvantages. One major concern with CT is the significant side effects including nausea, fatigue, hair loss, neutropenia, and general toxicity (70). When comparing the side effects, cisplatin-based chemotherapy yields more adverse effects (toxicity, mucositis, nausea, vomiting), when compared to RT alone (71). Because ECT requires a smaller drug dose compared to CT, it leads to less toxicity and a reduced rate of side effects (72). Moreover, chemotherapy is a non-specific treatment, affecting both cancerous and healthy dividing cells. This lack of specificity leads to collateral damage to healthy tissues. This indiscriminate action can result in persistent issues such as compromised immune function, cardiovascular problems, and impaired cognitive abilities, affecting the overall health and wellbeing of individuals who have undergone chemotherapy (73). When treating HNSCC, it is important to consider both aesthetics and function. The impact of surgical therapy is primarily determined by site. The function is mostly compromised while surgically treating sites like tongue, lip, or larynx. When treating cancer on the face or nose, aesthetics is also important. ECT is effective in both of these circumstances since the function is nearly not impacted and the visual element is far less impaired than with surgery; it requires as well only one or two treatments to achieve high OR (74). Both CT and RT can lead to esthetic changes, mostly on pigmentation of the skin (75, 76). Side effects, like impaired function, depend on the treated site, and it can vary from difficulty in swallowing, xerostomia, and others (77). CT can lead to long-term sequelae, presenting challenges that extend beyond the active treatment period. One notable consequence is the potential for lasting damage to healthy cells and tissues. Less toxic treatment approaches are needed since chemotherapy-induced toxicity seems to be a major problem in the treatment of HNSCC (78). Furthermore, CT has been associated with an increased risk of secondary cancers. The exposure to these agents during the initial treatment may contribute to the development of new malignancies later in life, underscoring the complex and multifaceted nature of chemotherapy-related sequelae (79). The same goes for radiotherapy; in some cases, it increases secondary cancer risk in different locations (80, 81). This is because both CT and radiotherapy not only impact cancerous cells and cancer itself but also affect all the body cells, as in the case of CT, or specific areas and cells in the case of RT. There is also data that some chemotherapeutic drugs and radiotherapy are linked to a higher incidence of cardiovascular diseases (82). The various aspects of cancer treatments are summarized in Table 2.
The response of individual tumors to ECT in clinical setting was classified by Mali et al. as complete response (CR), partial response (PR), no change (NC), or progressive disease (PD) (32). The concepts of objective response (OR, including CR and PR) and no response were introduced (32). This evaluation method is still applied today as Response Evaluation Criteria in Solid Tumors (RECIST). It is widely used to evaluate tumor response to ECT. In the first ECT studies, different protocols for electrochemotherapy were performed with different doses of chemotherapy drugs, different pulse parameters, and different electric pulse generators in conjunction with different electrode types. The first standard operating procedure (SOP) was introduced in 2006 as ESOPE (83). It standardized inclusion criteria, drug doses, pulse parameters, patient follow-up, and more. The main disadvantage is that it focuses mainly on skin cancers and metastases. It was updated in 2018 with new recommendations for indications for electrochemotherapy, pretreatment information and evaluation, and treatment choices as well as follow-up (84).
Most of the studies concentrate on palliative treatment objectives, primarily relying on the current standard operating procedure (SOP) of ESOPE. The prevailing inclusion criteria are outlined as follows:
— Cutaneous metastases, of any histology, which are symptomatic due to bleeding, ulceration, oozing, Odorkor pain.
— Progression of cutaneous metastases, where the development of symptoms, as listed above, is expected.
— Primary skin cancers, including recurrent tumors, where other treatment modalities (surgery, radiotherapy, and systemic therapies) have failed or are not possible.
— Patients who are receiving systemic therapy, but where cutaneous metastases are progressing or not responding despite a satisfactory response to systemic therapy in internal organs.
— Patient preference for electrochemotherapy after other treatment possibilities have been thoroughly explained to the patient (84).
All the studies and protocols involved and mentioned going forward are summarized and presented in Supplementary Table S3.
The DAHANCA 32, a clinical phase 2 study published in 2018, demonstrated promising results of recurrent mucosal head and neck tumor treatment using ECT. All 26 patients included in the study were pretreated with radiotherapy and had no other curative options. The treatment was conducted according to ESOPE guidelines with intravenous bleomycin injection (15,000 IU/m2). The overall tumor response rate was 58% by CT RESIST criteria (five tumors demonstrated a complete response and 10 had partial). Moreover, 16 out of 17 performed post-treatment biopsies (4 weeks after ECT) showed no remaining cancer cells (85). An analogous previous multicentral study of 43 cases, the EURECA project (2017), showed an overall response of 56%. The only factor influencing outcomes was tumor size, and the response rates were higher if the tumor was smaller than 3 cm (86).
A 2018 prospective study by B. Pichi et al., including 36 cases of any type of recurrent head and neck tumors, demonstrated a 100% overall response rate, though it was mostly partial (72). A similar study of 93 cases conducted by F. Longo et al. in 2019 showed 45% overall response with 5% complete response as well as improved pain and bleeding control (87). In both studies, the median survival rate was 9 months (72, 87).
A study by G. Riva et al. demonstrated a significantly higher global health status and social functioning in patients treated with ECT for head and neck tumors as well as decreased use of pain medications and better appetite (88). The previously mentioned EURECA project demonstrated an increase in wellbeing at 2 and 4 months with the EQ-5D questionnaire, unchanged scores with the EORTC QLQ-C30 (except “diarrhea”), and the EORTC QLQ-H&N35 (except significantly poorer swallowing) questionnaires (86). The DAHANCA study showed similar results (85).
One of the largest recent studies was conducted by Longo et al. in 2019 (87). In this setting, all 93 patients had a recurrent and/or metastatic disease and were treated with at least two chemotherapy and/or radiotherapy lines and were not suitable for surgery or chemo/radiotherapy. The overall response (OR) rate was 45%, with a complete response rate (CR) of 5%. This research found that the OR rate was significantly influenced by the tumor size. If the tumor was smaller than 3 cm (30 patients), the OR rate was 54.5%; if it was larger than 3 cm (10 patients), the OR rate was 26.3%. Similar findings were found in other studies that measured the size of the tumor. Most of the studies showed that smaller tumors (≤3 cm) have better response to ECT compared to larger tumors (88–91).
The tumor size and treatment response correlation was even bigger in the study done by Gargulio et al. (92). The treatment response based on tumor size was 89.5% CR for lesions of 4 cm or smaller and 16.7% for tumors larger than 4 cm. Similar results were demonstrated by Longo et al. (87). This study found that smaller tumors respond better to ECT (>3 cm OR rate 88%, <3 m OR rate 68%) without depending on tumor histology. The negative relationship between tumor response and larger size can be explained both technically (inadequate covering of the cancer by electric fields) and pharmacologically (irregular drug distribution inside the tumor). Another important finding regarding tumor response to the treatment was done by Claussen et al. in 2022. They evaluated tumor response in relation to its ulceration. It proved that non-ulcerated lesions respond to ECT significantly better than ulcerated lesions. This study enrolled a large number of patients (716). The non-ulcerated group had OR of 86% and CR of 65% compared to the ulcerated group’s OR of 79% and CR of 51% (93). It is important to mention that the median lesion size in the non-ulcerated group was twice smaller than in the ulcerated group (15 and 30 mm, respectively), and previous studies demonstrated that smaller tumors respond better to ECT. The relationship between ulceration and tumor response rate may be explained by local hypoxic metabolism or insufficient angiogenesis, resulting in an increase in toxic products (94, 95).
Not all studies were conducted on a palliative treatment basis. A 2015 paper by Landström et al. (96) focused on curative intent for patients with SCC, and 19 patients were included in the study. Patients with tumors deeper than 5 mm received radiotherapy in addition to ECT. The 5-year survival rate was 63.2% (12/19), and there was no local recurrence observed in patients who died during follow-up. It is important to mention that, in this study, they used higher bleomycin doses than in the ESOPE protocol (1,000 IU/cm3, ESOPE 250 IU/cm3). In addition, the calculation of the treatment volume differed from the ESOPE protocol (addition of 1-cm margins compared to ESOPE protocol). This means that high survivability could be linked to higher doses of bleomycin injected intratumorally.
A similar study was done by Bertino et al. in 2016 (89). This study focused on evaluating the efficacy of ECT in local tumor control as a primary treatment (89). They found that primary tumors respond better to ECT than recurrent or metastatic tumors. For the primary tumors (50 patients), CR was 70%, PR 20%, SD 8%, and PD 8%. While for secondary tumors (49 patients), CR was 55%, PR 18.5%, SD 18.5%, and PD 6%. This shows that untreated tumors are more responsive to ECT, and it may be considered as a first-line treatment in some cases. It is imperative to note that recurrent tumors are usually large and irregularly shaped, with deep margins buried under the apparent surface (97). Therefore, covering the whole tumor with a suitable electric field cannot be guaranteed in every situation. Additionally, scar tissue and impaired vascularization from previous treatments may result in reduced bleomycin delivery to tumor cells during electroporation (98).
A study done by Campana et al. in 2014 (99) used cisplatin for treating H&N cancer. It was given intratumorally. It was a small group of two patients, and both were refractory to treatment. In this case, cisplatin showed unsatisfactory results, but previously conducted studies were promising for using cisplatin in ECT (100).
In a 2019 study that was conducted by Jamsek et al. with reduced doses of bleomycin (101), 28 patients, 65 years or older, were treated. The control group consisted of 16 patients who were administrated standard doses of bleomycin (15.000 IU/m2 body surface area). Meanwhile, the experimental group included 12 patients; they received reduced bleomycin dosage (10,000 IU/m2 body surface area). No statistically significant differences were found between the two groups with respect to patient (age, gender) and tumor (diameter, histology, recurrent disease) characteristics (101). This implies that drug dosage, along with its associated toxicity and undesirable effects, could be lessened, potentially resulting in reduced overall toxicity and adverse effects. The study also showed that there was no difference between skin tumors of the head and neck and SCC of the oral cavity or oropharynx or between different electrodes or histological types of tumors, although basal cell carcinoma (BCC) responded better than other types of cancer.
This is evident in a recent comprehensive study involving a large patient cohort (330 patients, 623 tumors) done by Bertino G et al. in 2022 (102) (data was taken from InsPECT database), where OR per tumor was of 93% and CR was 83.1%, though the findings vary when compared to the work of the same author, which concentrated on cutaneous SCC (103). This study compared the data of 162 patients with 342 tumors. OR was 79% and CR was 61%. BCC OR and CR are significantly higher than SCC. This could be due the nature of tumor or tumor extent and, most notably, tumor histology.
Another study focusing on a completely different tumor histology—angiosarcomas—conducted in 2016 by Guida et al. (104) based its design on ESOPE. Its results did not significantly differ from those of other studies predominantly featuring SCC. The tumor response rates were as follows: OR: 85.5%, CR: 66.7%, PR: 18.5%, SD: 9.3%, and PD: 5.6% of the total number of tumors.
Plaschke et al., in 2017, showed that patients who were pretreated with chemo/radiotherapy and or surgery resulted in 56% of OR and 19% of CR. Similar findings were observed in the study done by Di Monta in 2017, in which CR was only 22.7% and OR was 81%. The conclusion drawn was that those studies achieving higher complete response rates are associated with lower tumor stages and a patient cohort displaying less malignant tumor tendencies (105). Meanwhile, other studies demonstrated better results—for instance, in studies conducted by Gargiulio et al. in 2012 and 2018, the tumor responses demonstrated higher rates: achieving an overall response (OR) rate of 100%, a complete response (CR) rate of 72% (92), and an OR rate of 100% with a CR of 71.4% (106), respectively. The cohort group for this research was patients with SCC of the lower lip. Higher response rates were achieved in T1–T2 tumors, and it served as reliable neo-adjuvant therapy for patients with T3 tumors (106).
The most successful study in terms of OR and CR was conducted by Landström et al. in 2015. Both OR and CR were 100% (96). All patients had mucosal primary tumor T1 or T2 (oral cavity or oropharynx). Among the 12 surviving patients, the 5-year local control maintained a rate of 100%, while the 5-year tumor-specific survival stood at 75%. It is important to highlight that 12 out of 19 patients underwent radiotherapy (RT) subsequent to receiving ECT. Additionally, for two patients, neck dissection (ND) was performed concurrently with ECT, and for four patients, it was carried out subsequently. Consequently, this situation cannot be solely attributed to true ECT survivability.
When comparing the treatment response results, we get quite different numbers of OR. Pinpointing an exact reason is challenging due to the inherent heterogeneity within the patient population. Moreover, most of the patients in the studies are pretreated, or all treatment options have been exhausted (72, 89, 90, 107). While the overall response rate might not be particularly high, considering that these are patients who have exhausted all treatment options, it could potentially enhance the overall survival and quality of life—for instance, in a study done by Riva in 2021, all patients were without any other curative treatment options. In this case, OR was 48% (11% CR, 37% PR) (88).
It should be highlighted that, according to current clinical practice, ECT is typically applied for older patients when other treatment options are unsuitable due to age and health risks. To evaluate the efficiency of ECT, the research distinguished two groups: patients older than 90 years old and those younger than 90 years old. The local response of the first group (>90 years old) was similar to that of the group composed of younger patients (<90 years old): OR: 87%, CR: 57% and OR: 88%, CR: 65%, respectively. These findings confirm ECT as a viable treatment choice independent of patient’s age; however, it is indicatory that the response rates could be even better if ECT was used as a primary option for tumor treatment rather than as a last resort.
Currently, ECT is frequently used with a palliative intent, while adverse effects, toxicity, and changes in quality of life are also very important. A 2021 systemic review of ECT in mucosal head and neck cancers states that the majority of patients in the included studies had no serious adverse events during and immediately after ECT. At 1 or 2 days later, swelling of the treated site appears, which is followed by necrotic and healing phases. Therefore, a tracheostomy may be needed to avoid upper airway obstruction in some cases. No changes or significant reduction of pain scores and significant improvement in bleeding control were seen in month 1 or 2 post-ECT. No bleomycin toxicity was reported (108).
Nonetheless, the most relevant side effects of ECT-treated H&N cancer were listed by Campana et al. (99). Their research outlined that the effects of electrochemotherapy can cause mild tissue damage or complications, which are not frequent though, local toxicity and tissue swelling, soft tissue necrosis, soft tissue or bone infection, bleeding, pain associated with wound healing, etc.
Quaglino et al. performed a pain assessment before the treatment as well as within 24 h, on day 45, and more than 45 days post-treatment using numeric rating scale (NRS) (109). In total, 121 patients with metastatic melanoma, squamous cell carcinoma, breast cancer, basal cell carcinoma, and other malignancies developing tumors of 0.3 to 40 cm in sizes were under the scope. The occurrence of post-procedure pain exhibited statistically significant associations with the following factors: the presence of moderate or severe pain before treatment, the size of the tumor, formerly irradiation, and the utilization of high PEF values. Most of the patients (60%) did not feel any pain before ECT. After 24 h, the pain perception in these patients increased; however, it decreased again during the subsequent visit. Other researchers associate unpleased sensations with repetitive ECT treatments (110). Thus, the quantity of the procedures should be limited. These factors can be managed through pre-treatment planning, which involves numerical computations, the selection of the most suitable pulsed electric field (PEF) properties, and the use of an appropriate electrode structure.
The biological tissues exposed to the electric field of specific properties experience permanent or transient structural changes in their cell level, recognized as irreversible electroporation (IRE) and reversible electroporation (RE), respectively. As mentioned above, the latest mentioned EP method is successfully employed for ECT or gene delivery (GET), allowing the administration of exogenous molecules, such as anti-cancer drugs or DNA, into the cell nucleus (111). Irreversible electroporation, on the contrary, triggers targeted tissue ablation and death of the cancerous cells without administration of external substances (112). Although both methods found the métier of application in clinical cancer treatment setting, ECT is more favorable in H&N cancer (113). Cell response to EP procedure can be manipulated though pulsed electric field parameters: intensity electric field strength and/or pulse duration (114), shown in Figure 5, and other parameters such as pulse shape (116), number (117), and pulse repetition frequency (118).
Figure 5 Cell response dependency on pulsed electric field strength and pulse duration. The red circle represents standard European Standard Operating Procedures of Electrochemotherapy treatment protocol (eight 100-µs-duration unipolar pulses with 1 Hz repetition frequency and 800–1,400 V/cm electric field strength (115).
The established properties of PEF as defined by ESOPE are typically adopted in clinical ECT treatment. However, due to minimized muscle contractions, pain, or thermal effects (119), there is growing interest in equivalent PEF properties, especially the potential utilization of pulses with reduced duration (nanosecond), which are proven to minimize neuromuscular stimulation (120) and joule heating (121), simultaneously ensuring efficiency-comparable tumor response. Given this perspective, it is advisable to incorporate these new PEF protocols into clinical settings.
Achieving a favorable treatment outcome is hinged on ensuring that the tumor is sufficiently covered by adequate electric field (122). This becomes a challenge due to the significant heterogeneity of the tissue (123). This implies that morphological difference and dielectric properties of the tissue layers (high resistivity cutaneous or high conductivity due to moisture of the mucous membrane with respect to tumor) may distort the spatial distribution of the electric field within the tissue, leading to an either extensively high or an excessively low PEF value within the tumor (124, 125) and, consequently, causing its partial response or the regrowth of the tumor. Another constituent is the electroporation electrodes, which, depending on contact method, utilization method, and composition, induce PEF within the tissue (126). The electrode design must adhere to safety standards, minimize the invasiveness of the procedure, and prevent bacterial infections (127).
During the clinical procedure, it is not feasible to monitor the spatial electric field distribution in real time; however, the first solutions to use electro-acoustic tomography are being proposed (128). Currently, the optimal approach to predetermine the outcome of individual electroporation-based procedure, including ECT, involves pretreatment planning through numerical models (129), though current treatment planning in SOP does not cover simulation of treatment which would be beneficial.
Considering previous clinical settings, the simplified tumor model of HNC located in the oral cavity is shown in Figure 6. The developed model consists of mass tissue representing the tumor and surrounding tissue covered with a thin layer of mucous membrane (0.1 mm in thickness) with 0.04 and 4.61 S/m conductivities, respectively. Two electrode structures typically employed in ECT for head and neck cancer were tested—non-invasive (plate) and invasive (fixed linear needles), with 5- and 4-mm gap size (Figures 6A, D, respectively). Furthermore, 1,000- and 600-V terminal voltages were selected to ensure ~1–1.3 kV/cm PEF inside the tumor depending on the electrode type. When utilizing plate electrodes, ensuring a larger contact area between the electrode and tissue is crucial to adequately envelop the lower part of the tumor with an effective PEF. Forming a lump was deemed beneficial for the studied tumor type and was thus integrated into the finite element model. Conversely, when employing invasive needle array electrodes, compressing the tumor becomes less feasible. To counter this, deeper needle penetration, surpassing the tumor’s depth, was considered for a tumor of equivalent size, as depicted in Figure 6C.
Figure 6 Spatial electric field distribution in the tumor using plate and needle array electrodes: (A) tumor finite element model (FEM) using plate electrodes, (B) typical spatial electric field distribution using plate electrodes (vertical cross-section, ZY axis), (C) typical spatial electric field distribution using plate electrodes (horizontal cross-section, XY axis), (D) tumor FEM model using needle array electrodes, (E) typical spatial electric field distribution using needle electrodes (vertical cross-section, ZY axis), and (F) typical spatial electric field distribution using needle electrodes (horizontal cross-section, XY axis).
Spatial electric field distribution within the tumor and tissues is inhomogeneous, especially in the case of plate electrodes. As per Figures 6B, C, the upper and lower segments of the tumor will experience low electric field values. Simultaneously, the surrounding tissue will receive unnecessarily high PEF, though in the context of oral cancers the thin layer of saliva surrounding the lesion slightly improves the field non-homogeneity at the top part of the tumor. Moisture within the oral cavity functions in a manner similar to that of conductive gel used in cutaneous treatments (130). This similarity lies in its ability to facilitate a more consistent distribution of the electric field. However, it is crucial to regulate the salivation (as well as the amount of conductive gel) during the clinical procedure since it could potentially cause short circuit of the electroporator. Needle electrodes, on the contrary, produce a more uniform electric field. The tumor appears to be enveloped by an electric field of approximately ~1.2 kV/cm, suggesting that employing such a method and electrodes could potentially lead to a more successful treatment outcome. Nevertheless, this type of electrode necessitates physical penetration into the tissue, consequently raising concerns about the invasiveness of the procedure and the potential risk of bacterial contamination.
At the same time, skin electroporation is inherently complex, primarily owing to the distinctive structure and low conductivity of the stratum corneum (131). To minimize the invasiveness of the procedure and mitigate the risk of a bacterial infection, it is advisable to employ non-invasive electrodes, such as plate electrodes. Recent studies suggest a potential solution to address the problem of field non-homogeneity in the tumor when plate electrodes are involved, i.e., delivering short (ns range) electric pulses at high frequencies (>MHz range). This approach is hypothesized to help alleviate the differences in dielectric properties within heterogeneous tissue (132) while ensuring saturated cell permeabilization (124). Other studies suggest to combine IRE and ECT procedures to facilitate the treatment and ensure complete tumor response (133), though in such case there is risk of thermal damage, and therefore the energy input should well controlled.
Patient-specific treatment planning has already been successfully used in radiotherapy, which, like electroporation, is also based on the interaction between a physical agent (radiation in radiotherapy and electric field in electroporation) and biological tissue (134). Radiotherapy consists of the following steps: simulation, treatment planning, setup verification, beam delivery, and response assessment. Simulation is based on the patient’s anatomy; the patient is scanned to obtain medical images [using, e.g., computed tomography (CT) or magnetic resonance imaging (MRI)] in the same position as expected to be when exposed to the radiation beam. Treatment planning starts by using the acquired medical images to generate a three-dimensional model. Similar approaches are being introduced in PEF-based treatments (135). In radiotherapy, the radiation dose must be high enough in the tumor volume to kill all the tumor cells, whereas in electrochemotherapy the electric field in the tumor volume needs to be sufficiently strong and the exposure long enough to cause cell membrane electroporation (33). Similarly to radiotherapy, the electrochemotherapy of deep-seated tumors can also be partitioned into several steps: mathematical modeling using CT and/or MRI images, treatment planning, setup verification, treatment, and response assessment.
At the current state of scientific evidence, electrochemotherapy is a promising approach for various head neck cancers, allowing acceptable tumor response rates to be achieved. Nevertheless, our review revealed that complete or overall tumor response fluctuates across different cases. This can be attributed to various factors, including cancer type and selection of appropriate anti-cancer drug and its concertation as well as insufficient pre-treatment planning. To overcome these obstacles, treatment pre-planning should be better characterized and formalized as a compulsory protocol step. The standards for metrology of the pulses being delivered should also be improved. Finally, it should be highlighted that, currently, ECT in the context of H&N cancers is mostly used as a last resort when other treatment options are not viable. Based on the clinical data, it is indicatory that if ECT was used as a primary option for tumor treatment, the response rates should be significantly higher. The main limitation of ECT lies in the homogeneity of the spatial electric field distribution, which is dependent on electrode type and treated tissue heterogeneity. In case of non-accurate treatment pre-planning, the regrowth of the tumor is inevitable; however, if managed well, the PEF-based modality for cancer treatment offers very good response rates, non-toxic treatment, and fast procedure with little to no side effects. The flexibility to preserve aesthetics without compromises in treatment efficacy following the procedure could be attributed as one of the major motivators to use ECT in the context of head and neck cancers.
AM: Formal analysis, Investigation, Methodology, Visualization, Writing – original draft, Writing – review & editing. VM: Investigation, Software, Validation, Visualization, Writing – original draft, Writing – review & editing. JK: Formal analysis, Investigation, Writing – original draft, Writing – review & editing. JI: Formal analysis, Investigation, Validation, Writing – original draft, Writing – review & editing. TI: Formal analysis, Investigation, Resources, Writing – original draft, Writing – review & editing. VN: Conceptualization, Formal analysis, Investigation, Methodology, Resources, Supervision, Validation, Writing – original draft, Writing – review & editing.
The author(s) declare that no financial support was received for the research, authorship, and/or publication of this article.
The authors declare that the research was conducted in the absence of any commercial or financial relationships that could be construed as a potential conflict of interest.
All claims expressed in this article are solely those of the authors and do not necessarily represent those of their affiliated organizations, or those of the publisher, the editors and the reviewers. Any product that may be evaluated in this article, or claim that may be made by its manufacturer, is not guaranteed or endorsed by the publisher.
The Supplementary Material for this article can be found online at: https://www.frontiersin.org/articles/10.3389/fonc.2024.1353800/full#supplementary-material
1. Cohen N, Fedewa S, Chen AY. Epidemiology and demographics of the head and neck cancer population. Oral Maxillofac Surg Clin North Am. (2018) 30:381–95. doi: 10.1016/J.COMS.2018.06.001
2. Curado MP, Hashibe M. Recent changes in the epidemiology of head and neck cancer. Curr Opin Oncol. (2009) 21:194–200. doi: 10.1097/cco.0b013e32832a68ca
3. Di Credico G, Polesel J, Dal Maso L, Pauli F, Torelli N, Luce D, et al. Alcohol drinking and head and neck cancer risk: the joint effect of intensity and duration. Br J Cancer. (2020) 123:1456–63. doi: 10.1038/S41416-020-01031-Z
5. Yete S, D’Souza W, Saranath D. High-risk human papillomavirus in oral cancer: clinical implications. Oncology. (2017) 94:133–41. doi: 10.1159/000485322
6. Ferlay J, Soerjomataram I, Dikshit R, Eser S, Mathers C, Rebelo M, et al. Cancer incidence and mortality worldwide: Sources, methods and major patterns in GLOBOCAN 2012. Int J Cancer. (2015) 136:E359–86. doi: 10.1002/ijc.29210
7. Johnson DE, Burtness B, Leemans CR, Lui VWY, Bauman JE, Grandis JR. Head and neck squamous cell carcinoma. Nat Rev Dis Primers. (2020) 6:92. doi: 10.1038/s41572-020-00224-3
8. Gormley M, Creaney G, Schache A, Ingarfield K, Conway DI. Reviewing the epidemiology of head and neck cancer: definitions, trends and risk factors. Br Dent J. (2022) 233:780–6. doi: 10.1038/s41415-022-5166-x
9. Sung H, Ferlay J, Siegel RL, Laversanne M, Soerjomataram I, Jemal A, et al. Global cancer statistics 2020: GLOBOCAN estimates of incidence and mortality worldwide for 36 cancers in 185 countries. CA Cancer J Clin. (2021) 71:209–49. doi: 10.3322/caac.21660
10. Bsoul SA, Huber MA, Terezhalmy GT. Squamous cell carcinoma of the oral tissues: a comprehensive review for oral healthcare providers. J Contemp Dent Pract. (2005) 6:1–16. doi: 10.5005/jcdp-6-4-1
11. Roi A, Roi CI, Andreescu NI, Riviş M, Badea ID, Meszaros N, et al. Oral cancer histopathological subtypes in association with risk factors: a 5-year retrospective study. Romanian J Morphology Embryology. (2021) 61:1213–20. doi: 10.47162/RJME.61.4.22
12. Ciolofan MS, Vlăescu AN, Mogoantă C-A, Ioniță E, Ioniță I, Căpitănescu A-N, et al. Clinical, histological and immunohistochemical evaluation of larynx cancer. Curr Health Sci J. (2017) 43:367–75. doi: 10.12865/CHSJ.43.04.14
13. Issa MR, Samuels SE, Bellile E, Shalabi FL, Eisbruch A, Wolf G. Tumor volumes and prognosis in laryngeal cancer. Cancers (Basel). (2015) 7:2236–61. doi: 10.3390/cancers7040888
14. George M. Cannon DJALRGPMH. Chapter 33 - oropharyngeal cancer. Clin Radiat Oncol (Fourth Edition). (2016), 597–628. doi: 10.1016/B978-0-323-24098-7.00033-2
16. Kwon DI, Miles BA. Hypopharyngeal carcinoma: Do you know your guidelines? Head Neck. (2019) 41:569–76. doi: 10.1002/hed.24752
17. Wycliffe ND, Grover RS, Kim PD, Simental A. Hypopharyngeal cancer. Topics Magnetic Resonance Imaging. (2007) 18:243–58. doi: 10.1097/RMR.0b013e3181570c3f
18. Pan X, Liu Y, Yang W, Chen Y, Tang W, Li C. Histological subtype remains a prognostic factor for survival in nasopharyngeal carcinoma patients. Laryngoscope. (2020) 130:E8–E88. doi: 10.1002/lary.28099
19. Lee AWM, Foo W, Mang O, Sze WM, Chappell R, Lau WH, et al. Changing epidemiology of nasopharyngeal carcinoma in Hong Kong over a 20-year period (1980-99): An encouraging reduction in both incidence and mortality. Int J Cancer. (2003) 103:680–5. doi: 10.1002/ijc.10894
20. Larkins E, Blumenthal GM, Yuan W, He K, Sridhara R, Subramaniam S, et al. FDA approval summary: pembrolizumab for the treatment of recurrent or metastatic head and neck squamous cell carcinoma with disease progression on or after platinum-containing chemotherapy. Oncologist. (2017) 22:873–8. doi: 10.1634/theoncologist.2016-0496
21. Barnes L. Metastases to the head and neck: An overview. Head Neck Pathol. (2009) 3:217–24. doi: 10.1007/s12105-009-0123-4
22. Enokida T, Tahara M. Electrochemotherapy in the treatment of head and neck cancer: current conditions and future directions. Cancers (Basel). (2021) 13:1418. doi: 10.3390/cancers13061418
23. Salcedo-Bellido I, Requena P, Mateos R, Ortega-Rico C, Olmedo-Requena R, Lozano-Lorca M, et al. Factors associated with the development of second primary tumours in head and neck cancer patients. Eur J Cancer Care (Engl). (2022) 31:e13699–9. doi: 10.1111/ecc.13699
24. Baudelet M, Van den Steen L, Tomassen P, Bonte K, Deron P, Huvenne W, et al. Very late xerostomia, dysphagia, and neck fibrosis after head and neck radiotherapy. Head Neck. (2019) 41:3594–603. doi: 10.1002/hed.25880
25. Rajendra A, Noronha V, Joshi A, Patil VM, Menon N, Prabhash K. Palliative chemotherapy in head and neck cancer: balancing between beneficial and adverse effects. Expert Rev Anticancer Ther. (2020) 20:17–29. doi: 10.1080/14737140.2020.1708197
26. Specenier PM, Vermorken JB. Recurrent head and neck cancer: current treatment and future prospects. Expert Rev Anticancer Ther. (2008) 8:375–91. doi: 10.1586/14737140.8.3.375
27. Condello M, D’Avack G, Spugnini EP, Meschini S. Electrochemotherapy: an alternative strategy for improving therapy in drug-resistant SOLID tumors. Cancers (Basel). (2022) 14:4341. doi: 10.3390/cancers14174341
28. Probst U, Fuhrmann I, Beyer L, Wiggermann P. Electrochemotherapy as a new modality in interventional oncology: A review. Technol Cancer Res Treat. (2018) 17:153303381878532. doi: 10.1177/1533033818785329
29. Okino M, Tomie H, Kanesada H, Marumoto M, Esato K, Suzuki H. Optimal electric conditions in electrical impulse chemotherapy. Jpn J Cancer Res. (1992) 83:1095–101. doi: 10.1111/j.1349-7006.1992.tb02727.x
30. Aguado-Romeo MJ, Benot-López S, Romero-Tabares A. Electroquimioterapia para el tratamiento del melanoma cutáneo locorregionalmente avanzado irresecable. Revisión sistemática. Actas Dermosifiliogr. (2017) 108:91–7. doi: 10.1016/j.ad.2016.08.008
31. Jarm T, Cemazar M, Miklavcic D, Sersa G. Antivascular effects of electrochemotherapy: implications in treatment of bleeding metastases. Expert Rev Anticancer Ther. (2010) 10:729–46. doi: 10.1586/era.10.43
32. Mali B, Jarm T, Snoj M, Sersa G, Miklavcic D. Antitumor effectiveness of electrochemotherapy: A systematic review and meta-analysis. Eur J Surg Oncol (EJSO). (2013) 39:4–16. doi: 10.1016/j.ejso.2012.08.016
33. Miklavčič D, Mali B, Kos B, Heller R, Serša G. Electrochemotherapy: from the drawing board into medical practice. BioMed Eng Online. (2014) 13:29. doi: 10.1186/1475-925X-13-29
34. Hecht SM. Bleomycin: new perspectives on the mechanism of action. J Nat Prod. (1999) 63:158–68. doi: 10.1021/np990549f
35. Dasari S, Tchounwou PB. Cisplatin in cancer therapy: molecular mechanisms of action. Eur J Pharmacol. (2014) 740:364–78. doi: 10.1016/j.ejphar.2014.07.025
36. Ghosh S. Cisplatin: The first metal based anticancer drug. Bioorg Chem. (2019) 88. doi: 10.1016/J.BIOORG.2019.102925
37. Cemazar M, Sersa G. Recent advances in electrochemotherapy. Bioelectricity. (2019) 1:204–13. doi: 10.1089/bioe.2019.0028
38. Radzevičiūtė-Valčiukė E, Malyško-Ptašinskė V, Mickevičiūtė E, Kulbacka J, Rembiałkowska N, Zinkevičienė A, et al. Calcium electroporation causes ATP depletion in cells and is effective both in microsecond and nanosecond pulse range as a modality of electrochemotherapy. Bioelectrochemistry. (2024) 155:108574. doi: 10.1016/j.bioelechem.2023.108574
39. Frandsen SK, Krüger MB, Mangalanathan UM, Tramm T, Mahmood F, Novak I, et al. Normal and Malignant cells exhibit differential responses to calcium electroporation. Cancer Res. (2017) 77:4389–401. doi: 10.1158/0008-5472.can-16-1611
40. Pinton P, Romagnoli A, Rizzuto R, Giorgi C. Ca2+ Signaling, mitochondria and cell death. Curr Mol Med. (2008) 8:119–30. doi: 10.2174/156652408783769571
41. Cerella C, Diederich M, Ghibelli L. The dual role of calcium as messenger and stressor in cell damage, death, and survival. Int J Cell Biol. (2010) 2010:546163. doi: 10.1155/2010/546163
42. Frandsen SK, Gissel H, Hojman P, Tramm T, Eriksen J, Gehl J. Direct therapeutic applications of calcium electroporation to effectively induce tumor necrosis. Cancer Res. (2012) 72:1336–41. doi: 10.1158/0008-5472.can-11-3782
43. Pisani S, Bertino G, Prina-Mello A, Locati LD, Mauramati S, Genta I, et al. Electroporation in head-and-neck cancer: an innovative approach with immunotherapy and nanotechnology combination. Cancers (Basel). (2022) 14:5363. doi: 10.3390/cancers14215363
44. Plaschke CC, Gehl J, Johannesen HH, Fischer BM, Kjaer A, Lomholt AF, et al. Calcium electroporation for recurrent head and neck cancer: A clinical phase I study. Laryngoscope Investig Otolaryngol. (2019) 4:49–56. doi: 10.1002/lio2.233
45. Wang X, Zhang H, Chen X. Drug resistance and combating drug resistance in cancer. Cancer Drug Resist. (2019) 2:141–60. doi: 10.20517/cdr.2019.10
46. Mansoori B, Mohammadi A, Davudian S, Shirjang S, Baradaran B. The different mechanisms of cancer drug resistance: A brief review. Adv Pharm Bull. (2017) 7:339–48. doi: 10.15171/apb.2017.041
47. Szakács G, Paterson JK, Ludwig JA, Booth-Genthe C, Gottesman MM. Targeting multidrug resistance in cancer. Nat Rev Drug Discovery. (2006) 5:219–34. doi: 10.1038/nrd1984
48. Choi YH, Yu A-M. ABC transporters in multidrug resistance and pharmacokinetics, and strategies for drug development. Curr Pharm Des. (2014) 20:793–807. doi: 10.2174/138161282005140214165212
49. Pao W, Miller VA, Politi KA, Riely GJ, Somwar R, Zakowski MF, et al. Acquired resistance of lung adenocarcinomas to gefitinib or erlotinib is associated with a second mutation in the EGFR kinase domain. PloS Med. (2005) 2:e73–3. doi: 10.1371/journal.pmed.0020073
50. Emran TB, Shahriar A, Mahmud AR, Rahman T, Abir MH, Siddiquee MF-R, et al. Multidrug resistance in cancer: understanding molecular mechanisms, immunoprevention and therapeutic approaches. Front Oncol. (2022) 12:891652. doi: 10.3389/fonc.2022.891652
51. Siddik ZH. Cisplatin: mode of cytotoxic action and molecular basis of resistance. Oncogene. (2003) 22:7265–79. doi: 10.1038/sj.onc.1206933
52. Lord CJ, Ashworth A. PARP inhibitors: Synthetic lethality in the clinic. Science. (2017) 355:1152–8. doi: 10.1126/science.aam7344
53. Huang R, Zhou P-K. DNA damage repair: historical perspectives, mechanistic pathways and clinical translation for targeted cancer therapy. Signal Transduct Target Ther. (2021) 6:254. doi: 10.1038/s41392-021-00648-7
54. Sharma S, Kelly TK, Jones PA. Epigenetics in cancer. Carcinogenesis. (2010) 31:27–36. doi: 10.1093/carcin/bgp220
55. Gote V, Nookala AR, Bolla PK, Pal D. Drug resistance in metastatic breast cancer: tumor targeted nanomedicine to the rescue. Int J Mol Sci. (2021) 22:4673. doi: 10.3390/ijms22094673
56. Galluzzi L, Vitale I. Oncogene-induced senescence and tumour control in complex biological systems. Cell Death Differ. (2018) 25:1005–6. doi: 10.1038/s41418-018-0102-y
57. Strauss J, Figg WD. Epigenetic approaches to overcoming chemotherapy resistance. Lancet Oncol. (2015) 16:1013–5. doi: 10.1016/S1470-2045(15)00231-4
58. Garner IM, Brown R. Is there a role for epigenetic therapies in modulating DNA damage repair pathways to enhance chemotherapy and overcome drug resistance? Cancers (Basel). (2022) 14:1533. doi: 10.3390/cancers14061533
59. Perri F, Pacelli R, Della Vittoria Scarpati G, Cella L, Giuliano M, Caponigro F, et al. Radioresistance in head and neck squamous cell carcinoma: Biological bases and therapeutic implications. Head Neck. (2015) 37:763–70. doi: 10.1002/hed.23837
60. Bouleftour W, Rowinski E, Louati S, Sotton S, Wozny A-S, Moreno-Acosta P, et al. A review of the role of hypoxia in radioresistance in cancer therapy. Med Sci Monit. (2021) 27:e934116–e934116. doi: 10.12659/MSM.934116
61. Overgaard J. Hypoxic modification of radiotherapy in squamous cell carcinoma of the head and neck – A systematic review and meta-analysis. Radiotherapy Oncol. (2011) 100:22–32. doi: 10.1016/j.radonc.2011.03.004
62. Ahmad P, Sana J, Slavik M, Slampa P, Smilek P, Slaby O. MicroRNAs involvement in radioresistance of head and neck cancer. Dis Markers. (2017) 2017:8245345. doi: 10.1155/2017/8245345
63. Yamamoto VN, Thylur DS, Bauschard M, Schmale I, Sinha UK. Overcoming radioresistance in head and neck squamous cell carcinoma. Oral Oncol. (2016) 63:44–51. doi: 10.1016/j.oraloncology.2016.11.002
64. Condello M, D’Avack G, Vona R, Spugnini EP, Scacco L, Meschini S. Electrochemotherapy with mitomycin C potentiates apoptosis death by inhibiting autophagy in squamous carcinoma cells. Cancers (Basel). (2021) 13:3867. doi: 10.3390/cancers13153867
65. Cemazar M, Miklavcic D, Mir LM, Belehradek J, Bonnay M, Fourcault D, et al. Electrochemotherapy of tumours resistant to cisplatin. Eur J Cancer. (2001) 37:1166–72. doi: 10.1016/s0959-8049(01)00091-0
66. Rembiałkowska N, Novickij V, Radzevičiūtė-Valčiukė E, Mickevičiūtė E, Gajewska-Naryniecka A, Kulbacka J. Susceptibility of various human cancer cell lines to nanosecond and microsecond range electrochemotherapy: Feasibility of multi-drug cocktails. Int J Pharm. (2023) 646:123485. doi: 10.1016/j.ijpharm.2023.123485
67. Lee NCJ, Kelly JR, Park HS, An Y, Judson BL, Burtness BA, et al. Patterns of failure in high-metastatic node number human papillomavirus-positive oropharyngeal carcinoma. Oral Oncol. (2018) 85:35–9. doi: 10.1016/j.oraloncology.2018.08.001
68. Mendenhall WM, Hinerman RW, Amdur RJ, Malyapa RS, Lansford CD, Werning JW, et al. Postoperative radiotherapy for squamous cell carcinoma of the head and neck. Clin Med Res. (2006) 4:200–8. doi: 10.3121/cmr.4.3.200
69. Anderson G, Ebadi M, Vo K, Novak J, Govindarajan A, Amini A. An updated review on head and neck cancer treatment with radiation therapy. Cancers (Basel). (2021) 13:4912. doi: 10.3390/cancers13194912
70. Geoffrois L, Martin L, Garaud P, De Raucourt D, Miny J, Maingon P, et al. Induction docetaxel platinum 5-FU (TPF) followed by cetuximab-radiotherapy (cetux-RT) versus concurrent chemo-radiotherapy (CT/RT) in patients with N2b/c-N3 non operated stage III-IV squamous cell cancer of the head and neck (SCCHN): Results of the GORTEC 2007-02 phase III randomized trial. J Clin Oncol. (2016) 34:6000–0. doi: 10.1200/JCO.2016.34.15_suppl.6000
71. Bernier J, Domenge C, Ozsahin M, Matuszewska K, Lefèbvre J-L, Greiner RH, et al. Postoperative irradiation with or without concomitant chemotherapy for locally advanced head and neck cancer. New Engl J Med. (2004) 350:1945–52. doi: 10.1056/NEJMoa032641
72. Pichi B, Pellini R, Spriano G. Electrochemotherapy – A locoregional therapy with well-established palliative effect in patient with large recurrent lesion of head and neck. J Cranio-Maxillofacial Surg. (2019) 47:41–6. doi: 10.1016/j.jcms.2018.10.014
73. Wilberg P, Hjermstad MJ, Ottesen S, Herlofson BB. Chemotherapy-associated oral sequelae in patients with cancers outside the head and neck region. J Pain Symptom Manage. (2014) 48:1060–9. doi: 10.1016/j.jpainsymman.2014.02.009
74. Plaschke CC, Johannesen HH, Hansen RH, Hendel HW, Kiss K, Gehl J, et al. The DAHANCA 32 study: Electrochemotherapy for recurrent mucosal head and neck cancer. Head Neck. (2019) 41:329–39. doi: 10.1002/hed.25454
75. Burke G, Faithfull S, Probst H. Radiation induced skin reactions during and following radiotherapy: A systematic review of interventions. Radiography. (2022) 28:232–9. doi: 10.1016/j.radi.2021.09.006
76. Wyatt AJ, Leonard GD, Sachs DL. Cutaneous reactions to chemotherapy and their management. Am J Clin Dermatol. (2006) 7:45–63. doi: 10.2165/00128071-200607010-00005
77. Strojan P, Hutcheson KA, Eisbruch A, Beitler JJ, Langendijk JA, Lee AWM, et al. Treatment of late sequelae after radiotherapy for head and neck cancer. Cancer Treat Rev. (2017) 59:79–92. doi: 10.1016/j.ctrv.2017.07.003
78. Fayette J, Fontaine-Delaruelle C, Ambrun A, Daveau C, Poupart M, Ramade A, et al. (docetaxel, cisplatin, fluorouracil) for patients unfit to standard TPF in locally advanced head and neck squamous cell carcinoma: a study of 48 patients. Oncotarget. (2016) 7:37297–304. doi: 10.18632/oncotarget.8934
79. Demoor-Goldschmidt C, de Vathaire F. Review of risk factors of secondary cancers among cancer survivors. Br J Radiol. (2018) 92:20180390. doi: 10.1259/bjr.20180390
80. Goldust M, Giulini M, Weidenthaler-Barth B, Gupta M, Grabbe S, Schepler H. Increased risk of angiosarcoma secondary to cancer radiotherapy: Case series and review of the treatment options. Dermatol Ther. (2020) 33:e13234–e13239. doi: 10.1111/dth.13234
81. Nugent TS, Low EZ, Fahy MR, Donlon NE, McCormick PH, Mehigan BJ, et al. Prostate radiotherapy and the risk of secondary rectal cancer—a meta-analysis. Int J Colorectal Dis. (2022) 37:437–47. doi: 10.1007/s00384-021-04075-6
82. Min SS, Wierzbicki AS. Radiotherapy, chemotherapy and atherosclerosis. Curr Opin Cardiol. (2017) 32:441–7. doi: 10.1097/HCO.0000000000000404
83. Mir LM, Gehl J, Sersa G, Collins CG, Garbay J-R, Billard V, et al. Standard operating procedures of the electrochemotherapy: Instructions for the use of bleomycin or cisplatin administered either systemically or locally and electric pulses delivered by the CliniporatorTM by means of invasive or non-invasive electrodes. Eur J Cancer Suppl. (2006) 4:14–25. doi: 10.1016/j.ejcsup.2006.08.003
84. Gehl J, Sersa G, Matthiessen LW, Muir T, Soden D, Occhini A, et al. Updated standard operating procedures for electrochemotherapy of cutaneous tumours and skin metastases. Acta Oncol (Madr). (2018) 57:874–82. doi: 10.1080/0284186X.2018.1454602
85. Plaschke CC, Johannesen HH, Hansen RH, Hendel HW, Kiss K, Gehl J, et al. The DAHANCA 32 study: Electrochemotherapy for recurrent mucosal head and neck cancer. Head Neck. (2018) 41(2):329–39. doi: 10.1002/hed.25454
86. Plaschke CC, Bertino G, McCaul JA, Grau JJ, de Bree R, Sersa G, et al. European Research on Electrochemotherapy in Head and Neck Cancer (EURECA) project: Results from the treatment of mucosal cancers. Eur J Cancer. (2017) 87:172–81. doi: 10.1016/j.ejca.2017.10.008
87. Longo F, Perri F, Pavone E, Aversa C, Maglione MG, Guida A, et al. Electrochemotherapy as palliative treatment in patients with advanced head and neck tumours: Outcome analysis in 93 patients treated in a single institution. Oral Oncol. (2019) 92:77–84. doi: 10.1016/j.oraloncology.2019.03.016
88. Riva G, Salonia L, Fassone E, Sapino S, Piano F, Pecorari G. Quality of life in electrochemotherapy for cutaneous and mucosal head and neck tumors. J Clin Med. (2021) 10:4366. doi: 10.3390/jcm10194366
89. Bertino G, Sersa G, De Terlizzi F, Occhini A, Plaschke CC, Groselj A, et al. European Research on Electrochemotherapy in Head and Neck Cancer (EURECA) project: Results of the treatment of skin cancer. Eur J Cancer. (2016) 63:41–52. doi: 10.1016/j.ejca.2016.05.001
90. Matthiessen LW, Chalmers RL, Sainsbury DCG, Veeramani S, Kessell G, Humphreys AC, et al. Management of cutaneous metastases using electrochemotherapy. Acta Oncol (Madr). (2011) 50:621–9. doi: 10.3109/0284186X.2011.573626
91. Maglitto F, Salzano G, Longo F, Di Bernardo E, D’alessio V, Fusco R, et al. Electrochemotherapy as palliative treatment in patients with recurrent and/or metastatic head and neck tumours: features analysis for an early determination of the partial responsive patients. Eur Rev Med Pharmacol Sci (2022) 26(4):1350–63. doi: 10.26355/eurrev_202202_28128
92. Gargiulo M, Papa A, Capasso P, Moio M, Cubicciotti E, Parascandolo S. Electrochemotherapy for non-melanoma head and neck cancers: Clinical outcomes in 25 patients. Ann Surg. (2012) 255:1158–64. doi: 10.1097/SLA.0b013e31824f68b2
93. Claussen CS, Moir G, Bechara FG, Orlando A, Matteucci P, Mowatt D, et al. Prospective cohort study by InspECT on safety and efficacy of electrochemotherapy for cutaneous tumors and metastases depending on ulceration. JDDG - J German Soc Dermatol. (2022) 20:470–81. doi: 10.1111/ddg.14699
94. O’Byrne KJ, Dalgleish AG, Browning MJ, Steward WP, Harris AL. The relationship between angiogenesis and the immune response in carcinogenesis and the progression of Malignant disease. Eur J Cancer. (2000) 36:151–69. doi: 10.1016/S0959-8049(99)00241-5
95. Gualdi G, Panarese F, Meogrossi G, Marchioni M, De Tursi M, Di Marino P, et al. Speed rate (SR) as a new dynamic index of melanoma behavior. Pigment Cell Melanoma Res. (2020) 33:709–18. doi: 10.1111/pcmr.12890
96. Landström FJ, Reizenstein J, Adamsson GB, Von Beckerath M, Möller C. Long-term follow-up in patients treated with curative electrochemotherapy for cancer in the oral cavity and oropharynx. Acta Otolaryngol. (2015) 135:1070–8. doi: 10.3109/00016489.2015.1049663
97. Nissi L, Suilamo S, Kytö E, Vaittinen S, Irjala H, Minn H. Recurrence of head and neck squamous cell carcinoma in relation to high-risk treatment volume. Clin Transl Radiat Oncol. (2021) 27:139–46. doi: 10.1016/j.ctro.2021.01.013
98. Vivod G, Jesenko T, Gasljevic G, Kovacevic N, Bosnjak M, Sersa G, et al. Treatment of vulvar cancer recurrences with electrochemotherapy – a detailed analysis of possible causes for unsuccessful treatment. Radiol Oncol. (2023) 57:121–6. doi: 10.2478/raon-2023-0010
99. Campana LG, Mali B, Sersa G, Valpione S, Giorgi CA, Strojan P, et al. Electrochemotherapy in non-melanoma head and neck cancers: A retrospective analysis of the treated cases. Br J Oral Maxillofac Surg. (2014) 52:957–64. doi: 10.1016/j.bjoms.2014.08.004
100. Raeisi E, Aghamiri S, Bandi A, Rahmatpour N, Firoozabadi S, Kafi-Abad S, et al. The antitumor efficiency of combined electrochemotherapy and single dose irradiation on a breast cancer tumor model. Radiol Oncol. (2012) 46:226–32. doi: 10.2478/v10019-012-0035-x
101. Jamsek C, Sersa G, Bosnjak M, Groselj A. Long term response of electrochemotherapy with reduced dose of bleomycin in elderly patients with head and neck non-melanoma skin cancer. Radiol Oncol. (2020) 54:79–85. doi: 10.2478/raon-2020-0009
102. Bertino G, Muir T, Odili J, Groselj A, Marconato R, Curatolo P, et al. Treatment of basal cell carcinoma with electrochemotherapy: insights from the inspECT registry (2008–2019). Curr Oncol. (2022) 29:5324–37. doi: 10.3390/curroncol29080423
103. Bertino G, Groselj A, Campana LG, Kunte C, Schepler H, Gehl J, et al. Electrochemotherapy for the treatment of cutaneous squamous cell carcinoma: The INSPECT experience (2008-2020). Front Oncol. (2022) 12:951662. doi: 10.3389/fonc.2022.951662
104. Guida M, Campana LG, Curatolo P, Strippoli S, Bonadies A, Grilz G, et al. Local treatment with electrochemotherapy of superficial angiosarcomas: Efficacy and safety results from a multi-institutional retrospective study. J Surg Oncol. (2016) 114:246–53. doi: 10.1002/jso.24287
105. Di Monta G, Caracò C, Simeone E, Grimaldi AM, Marone U, Di Marzo M, et al. Electrochemotherapy efficacy evaluation for treatment of locally advanced stage III cutaneous squamous cell carcinoma: A 22-cases retrospective analysis. J Transl Med. (2017) 15. doi: 10.1186/s12967-017-1186-8
106. Gargiulo M, Serra Mestre JM, Cortese A, Murphy DC, Parascandolo S, Razzano S. Long term effectiveness of electrochemotherapy for the treatment of lower lip squamous cell carcinoma. J Cranio-Maxillofacial Surg. (2018) 46:1968–74. doi: 10.1016/j.jcms.2018.08.013
107. Mevio N, Bertino G, Occhini A, Scelsi D, Tagliabue M, Mura F, et al. Electrochemotherapy for the treatment of recurrent head and neck cancers: preliminary results. J Craniomaxillofac Surg 46(11):1968–74. doi: 10.1177/030089161209800305
108. Strojan P, Grošelj A, Serša G, Plaschke CC, Vermorken JB, Nuyts S, et al. Electrochemotherapy in mucosal cancer of the head and neck: A systematic review. Cancers (Basel). (2021) 13:1254. doi: 10.3390/cancers13061254
109. Quaglino P, Matthiessen LW, Curatolo P, Muir T, Bertino G, Kunte C, et al. Predicting patients at risk for pain associated with electrochemotherapy. Acta Oncol (Madr). (2015) 54:298–306. doi: 10.3109/0284186X.2014.992546
110. Campana LG, Valpione S, Falci C, Mocellin S, Basso M, Corti L, et al. The activity and safety of electrochemotherapy in persistent chest wall recurrence from breast cancer after mastectomy: a phase-II study. Breast Cancer Res Treat. (2012) 134:1169–78. doi: 10.1007/s10549-012-2095-4
111. Yarmush ML, Golberg A, Serša G, Kotnik T, Miklavčič D. Electroporation-based technologies for medicine: principles, applications, and challenges. Annu Rev BioMed Eng. (2014) 16:295–320. doi: 10.1146/annurev-bioeng-071813-104622
112. Aycock KN, Davalos RV. Irreversible electroporation: background, theory, and review of recent developments in clinical oncology. Bioelectricity. (2019) 1:214–34. doi: 10.1089/bioe.2019.0029
113. Tian L, Wang L, Qiao Y, Lu L, Lee P, Chang A, et al. Antitumor efficacy of liposome-encapsulated NVP-BEZ235 combined with irreversible electroporation for head and neck cancer. Molecules. (2019) 24:3560. doi: 10.3390/molecules24193560
114. Vizintin A, Markovic S, Scancar J, Kladnik J, Turel I, Miklavcic D. Nanosecond electric pulses are equally effective in electrochemotherapy with cisplatin as microsecond pulses. Radiol Oncol. (2022) 56:326–35. doi: 10.2478/raon-2022-0028
115. Bendix MB, Houston A, Forde PF, Brint E. Defining optimal parameters to maximize the effect of electrochemotherapy on lung cancer cells whilst preserving the integrity of immune cells. Bioelectrochemistry. (2022) 148:108257. doi: 10.1016/j.bioelechem.2022.108257
116. Scuderi M, Rebersek M, Miklavcic D, Dermol-Cerne J. The use of high-frequency short bipolar pulses in cisplatin electrochemotherapy in vitro. Radiol Oncol. (2019) 53:194–205. doi: 10.2478/raon-2019-0025
117. Romeo S, Sannino A, Scarfì MR, Vernier PT, Cadossi R, Gehl J, et al. ESOPE-equivalent pulsing protocols for calcium electroporation: an in vitro optimization study on 2 cancer cell models. Technol Cancer Res Treat. (2018) 17:153303381878807. doi: 10.1177/1533033818788072
118. Shankayi Z, Firoozabadi SMP, Hassan ZS. Optimization of electric pulse amplitude and frequency in vitro for low voltage and high frequency electrochemotherapy. J Membr Biol. (2014) 247:147–54. doi: 10.1007/s00232-013-9617-9
119. Fusco R, Di Bernardo E, D’Alessio V, Salati S, Cadossi M. Reduction of muscle contraction and pain in electroporation-based treatments: An overview. World J Clin Oncol. (2021) 12:367–81. doi: 10.5306/wjco.v12.i5.367
120. Gudvangen E, Kim V, Novickij V, Battista F, Pakhomov AG. Electroporation and cell killing by milli- to nanosecond pulses and avoiding neuromuscular stimulation in cancer ablation. Sci Rep. (2022) 12:1763. doi: 10.1038/s41598-022-04868-x
121. Youssef HM, El-Bary AA. Voltage and Time Required for Irreversible Thermal Damage of Tumor Tissues during Electrochemotherapy under Thomson Effect. Mathematics. (2020) 8:1488. doi: 10.3390/math8091488
122. Miklavčič D, Beravs K, Šemrov D, Čemažar M, Demšar F, Serša G. The importance of electric field distribution for effective in vivo electroporation of tissues. Biophys J. (1998) 74:2152–8. doi: 10.1016/S0006-3495(98)77924-X
123. Treuting PM, Morton TH. Oral cavity and teeth. In: Comparative Anatomy and Histology. United States: Elsevier (2012). p. 95–110. doi: 10.1016/B978-0-12-381361-9.00007-X
124. Novickij V, Baleviciute A, Malysko V, Zelvys A, Radzeviciute E, Kos B, et al. Effects of time delay between unipolar pulses in high frequency nano-electrochemotherapy. IEEE Trans BioMed Eng. (2022) 69:1726–32. doi: 10.1109/TBME.2021.3129176
125. Suzuki DOH, Berkenbrock JA, Frederico MJS, Silva FRMB, Rangel MMM. Oral mucosa model for electrochemotherapy treatment of dog mouth cancer: ex vivo, in silico, and in vivo experiments. Artif Organs. (2018) 42:297–304. doi: 10.1111/aor.13003
126. Malyško-Ptašinskė V, Staigvila G, Novickij V. Invasive and non-invasive electrodes for successful drug and gene delivery in electroporation-based treatments. Front Bioeng Biotechnol. (2023) 10:1094968. doi: 10.3389/fbioe.2022.1094968
127. Cvetkoska A, Pirc E, Rebersek M, Magjarevic R, Miklavcic D. Towards standardization of electroporation devices and protocols. IEEE Instrum Meas Mag. (2020) 23:74–81. doi: 10.1109/MIM.2020.9062692
128. Xu Y, Sun L, Wang S, Yan Y, Pandey P, Novickij V, et al. Electroacoustic tomography for real-time visualization of electrical field dynamics in deep tissue during electroporation. Commun Eng. (2023) 2:75. doi: 10.1038/s44172-023-00125-9
129. Corovic S, Zupanic A, Miklavcic D. Numerical modeling and optimization of electric field distribution in subcutaneous tumor treated with electrochemotherapy using needle electrodes. IEEE Trans Plasma Sci. (2008) 36:1665–72. doi: 10.1109/TPS.2008.2000996
130. Ivorra A, Al-Sakere B, Rubinsky B, Mir LM. Use of conductive gels for electric field homogenization increases the antitumor efficacy of electroporation therapies. Phys Med Biol. (2008) 53:6605–18. doi: 10.1088/0031-9155/53/22/020
131. Gelker M, Müller-Goymann CC, Viöl W. Permeabilization of human stratum corneum and full-thickness skin samples by a direct dielectric barrier discharge. Clin Plasma Med. (2018) 9:34–40. doi: 10.1016/j.cpme.2018.02.001
132. Lorenzo MF, Bhonsle SP, Arena CB, Davalos RV. Rapid impedance spectroscopy for monitoring tissue impedance, temperature, and treatment outcome during electroporation-based therapies. IEEE Trans BioMed Eng. (2021) 68:1536–46. doi: 10.1109/TBME.2020.3036535
133. Chen Y, Moser MAJ, Luo Y, Zhang W, Zhang B. Chemical enhancement of irreversible electroporation: A review and future suggestions. Technol Cancer Res Treat. (2019) 18:153303381987412. doi: 10.1177/1533033819874128
134. Lecchi M, Fossati P, Elisei F, Orecchia R, Lucignani G. Current concepts on imaging in radiotherapy. Eur J Nucl Med Mol Imaging. (2008) 35:821–37. doi: 10.1007/s00259-007-0631-y
Keywords: head and neck cancer, electrochemotherapy, tumors, pulsed electric fields, FEM analysis
Citation: Morozas A, Malyško-Ptašinskė V, Kulbacka J, Ivaška J, Ivaškienė T and Novickij V (2024) Electrochemotherapy for head and neck cancers: possibilities and limitations. Front. Oncol. 14:1353800. doi: 10.3389/fonc.2024.1353800
Received: 11 December 2023; Accepted: 29 January 2024;
Published: 15 February 2024.
Edited by:
Remco De Bree, University Medical Center Utrecht, NetherlandsReviewed by:
Giulia Bertino, San Matteo Hospital Foundation (IRCCS), ItalyCopyright © 2024 Morozas, Malyško-Ptašinskė, Kulbacka, Ivaška, Ivaškienė and Novickij. This is an open-access article distributed under the terms of the Creative Commons Attribution License (CC BY). The use, distribution or reproduction in other forums is permitted, provided the original author(s) and the copyright owner(s) are credited and that the original publication in this journal is cited, in accordance with accepted academic practice. No use, distribution or reproduction is permitted which does not comply with these terms.
*Correspondence: Vitalij Novickij, dml0YWxpai5ub3ZpY2tpakB2aWxuaXVzdGVjaC5sdA==
†These authors have contributed equally to this work
Disclaimer: All claims expressed in this article are solely those of the authors and do not necessarily represent those of their affiliated organizations, or those of the publisher, the editors and the reviewers. Any product that may be evaluated in this article or claim that may be made by its manufacturer is not guaranteed or endorsed by the publisher.
Research integrity at Frontiers
Learn more about the work of our research integrity team to safeguard the quality of each article we publish.