- 1Department of Orthopaedics, the Second Hospital of Shanxi Medical University, Taiyuan, China
- 2Shanxi Key Laboratory of Bone and Soft Tissue Injury Repair, Taiyuan, China
Propose: This meta-analysis aimed to determine whether 3D-printed artificial vertebral bodies (AVBs) have superior clinical efficacy compared to conventional titanium mesh cages (TMCs) for spinal reconstruction after total en bloc spondylectomy (TES) for spinal tumors.
Methods: Electronic databases, including PubMed, OVID, ScienceDirect, Embase, CINAHL, Web of Science, Cochrane Library, WANFANG, and CNKI, were searched to identify clinical trials investigating 3D-printed AVB versus conventional TMC from inception to August 2023. Data on the operation time, intraoperative blood loss, preoperative and postoperative visual analogue scale (VAS) scores, preoperative and postoperative Frankel classification of spinal cord injury, vertebral body subsidence, and early complications were collected from eligible studies for a meta-analysis. Data were analyzed using Review Manager 5.4 and Stata 14.0.
Results: Nine studies assessing 374 patients were included. The results revealed significant differences between the 3D-printed AVB and conventional TMC groups with regard to operation time (P = 0.04), intraoperative blood loss (P = 0.004), postoperative VAS score (P = 0.02), vertebral body subsidence (P < 0.0001), and early complications (P = 0.02). Conversely, the remaining preoperative VAS score and Frankel classifications (pre-and postoperative) did not differ significantly between the groups.
Conclusion: The 3D-printed AVB in spinal reconstruction after TES for spinal tumors has the advantages of a short operative time, little intraoperative blood loss, weak postoperative pain, low occurrence of vertebral body subsidence and early complications, and a significant curative effect. This could provide a strong basis for physicians to make clinical decisions.
Systematic review registration: https://www.crd.york.ac.uk/prospero/display_record.php?ID=CRD42023441521, identifier CRD42023441521.
1 Introduction
In recent years, the incidence of spinal tumors has been rising (1). Regardless of whether primary or metastatic spinal tumors are present, they can progressively develop and compress the spinal cord or cauda equina nerve, leading to neurological dysfunction, which can cause spinal instability, resulting in intractable pain and seriously affecting the quality of life. As the most important surgical method for spinal tumors, the main purpose of total en bloc spondylectomy (TES) is to completely remove the diseased tissues and reconstruct the integrity and stability of the spine (2, 3). In conventional methods, titanium mesh cages (TMCs) composite bone graft materials are commonly used for spinal reconstruction; however, they are prone to poor fusion of vertebral bodies and have a high probability of subsidence, which leads to the failure of internal fixation (4). Both Shinmura et al. (5) and Ji et al. (6) found that due to the cutting effect of the TMC, subsidence was prone to occur, and the incidence rate is as high as 42.5–79.7%, which led to the reconstruction failure. Autologous bone reconstruction has problems such as limited sources and secondary injuries, while allogeneic bone has risks of bone nonunion, infection, rejection, etc. (7). The 3D-printed technology appeared in the mid-1990s and has been widely used in many medical fields (8, 9). In the field of spinal surgery, 3D-printed artificial vertebral bodies (AVBs) have a personalized design that can achieve a complete match and closely fit the upper and lower vertebral bodies, reducing the probability of subsidence (10). Because 3D-printed AVB can simulate the normal human anatomical structure, its reconstruction after spinal tumor resection has more biomechanical characteristics than conventional TMC, and the prosthesis is made of titanium alloy, which has good biocompatibility (11).
Currently, owing to the short clinical application time of 3D-printed AVB, its efficacy and safety lack evidence-based medical evidence. The meta-analysis aimed to compare the clinical efficacy and safety of 3D-printed AVB and conventional TMC in spinal reconstruction after total en bloc spondylectomy for spinal tumors, and to evaluate their advantages. We hypothesized that the clinical efficacy and safety of the 3D-printed AVB were superior to those of the conventional TMC group.
2 Methods
This meta-analysis was conducted in accordance with the Preferred Reporting Items for Systematic Reviews and Meta-Analyses (PRISMA) statement guidelines.
2.1 Search strategy
In accordance with the recommendations of the Cochrane Collaboration, several comprehensive databases were retrieved for studies, including PubMed, OVID, ScienceDirect, Embase, CINAHL, Web of Science, Cochrane Library, WANFANG, and CNKI, were searched from inception to June 2023. Grey literature was identified via manual searches of journal catalogs and references. All relevant studies were retrieved without language restrictions and, if necessary, translated. The search was conducted by using the keywords, “Printing, Three-Dimensional,” “Artificial Vertebral Body,” “Titanium Mesh Cage,” “Spine,” “Neoplasms,” and “Total En Bloc Spondylectomy.” The search strategy was performed by using the all fields, i.e., “((3D-printed artificial vertebral body) OR (Three-Dimensional-printed artificial vertebral body) OR (Titanium Mesh Cage)) AND ((spinal neoplasms) OR (spinal tumors)) AND (total en bloc spondylectomy).” Relevant studies and abstracts were manually searched to identify those that met the inclusion search.
2.2 Study selection
Based on the initially retrieved abstracts, two reviewers independently selected the relevant studies that met the established criteria for a comprehensive review. The full text of the study was reviewed if the abstract did not provide the full information. Studies meeting the established criteria were identified and included.
The inclusion criteria were (1) patients diagnosed with spinal tumors based on pathological results for any age, sex, or race (2); patients undergoing total en bloc spondylectomy (3); implants with 3D-printed AVBs or TMCs (4); controlled trials, cohort studies, prospective studies, and retrospective studies; and (5) results reporting one or more of the operative time, intraoperative blood loss, visual analogue scale (VAS) scores, Frankel classification, vertebral body subsidence, and early complications. The exclusion criteria were as follows (1): cadaveric studies (2); duplicate publications (3); reviews, case reports, letters, commentaries, editorials, or expert opinions (4); studies with missing or incomplete outcome data; and (5) studies not available in the full text.
2.3 Data extraction and quality assessment
Based on a pre-established data extraction form, two investigators (M. D and YJ. G) independently extracted the data from the included studies and resolved any disagreements by consulting a third reviewer (Y. F).
The following information was extracted (1) the basic characteristics of the included studies, such as the authors, country, publication date, article title, and journal title (2); the methodological characteristics of the studies: randomized, controlled, and blinded (3); demographic characteristics such as race, sex, and age; and (4) other parameters, such as sample size, tumor location, tumor type, pathological diagnosis, segment, surgical approach, follow-up time, and outcomes. We attempted to communicate with the corresponding authors while relevant information about the included studies was unclear or missing and could not be analyzed. The general characteristics of these included nine studies are presented in Table 1. The methodological quality of the included studies was assessed using the risk-of-bias assessment tool outlined in the Cochrane Handbook. Seven domains were evaluated (1): random sequence generation (2); allocation concealment (3); blinding of participants and personnel (4); blinding of outcome assessment (5); incomplete outcome data (6); selective reporting; and (7) other biases.
2.4 Outcome measures
The outcome measures mainly included operation time, intraoperative blood loss, preoperative and postoperative VAS scores, preoperative and postoperative Frankel classifications (grades A–E were scored 1–5 points, respectively), vertebral body subsidence (height > 3 mm), and early complications.
2.5 Statistical analysis
Continuous outcomes in the included studies were expressed as weighted mean differences (WMDs) or standard mean differences (SMDs), and odds ratios (ORs) were used for dichotomous outcomes, all with 95% confidence intervals (CIs). The WMD was mainly used to express the synthetic data of the VAS scores and Frankel classification. The SMD was used to express the operation time and intraoperative blood loss because of the use of different testing methods or too large of these means. Moreover, the OR was used to express vertebral body subsidence and early complications. Heterogeneity was determined by evaluating the proportion of inconsistencies among studies that were caused by actual differences among studies, not random errors or by chance (21). Heterogeneity was detected mainly by I² and chi-square tests in this study. When significant heterogeneity was found (P ≤ 0.10, I² > 50%), a random-effects model was chosen, and when there was no statistical evidence of heterogeneity (P > 0.10, I² ≤ 50%), a fixed-effects model was used (22). Sensitivity and subgroup analyses were performed by excluding individual studies or factors when heterogeneity was found. Moreover, we checked the related factors by meta-regression analysis to search for the source, and by funnel plots and Egger’s test to estimate the publication bias. When heterogeneity was not identified, the results were qualitatively analyzed. The difference was considered statistically significant at P ≤ 0.05. All data were analyzed using Review Manager 5.4 and Stata 14.0 statistical software provided by the Nordic Cochrane Centre and the Stata Corp, respectively.
3 Results
3.1 Search and selection
Through online and manual searches, 538 articles were initially identified. Sixty-six studies remained after removing 472 duplicate studies. Of these, 48 were excluded after reading the abstracts because they were irrelevant to the purpose of this study. The remaining 18 studies were read in full, of which nine were excluded as single-arm clinical trials or animal experiments. Ultimately, nine studies (one in English and eight in Chinese) involving 374 patients were included (12–20). A Flow Diagram of the studies selection process based on the PRISMA 2020 flow diagram is shown in Figure 1 (23). The quality of methodology in the included studies was high, with a low likelihood of bias. A summary and graphs of the risk of bias are shown in Figure 2.
3.2 Operation time
Eight of the included studies (12–19) compared the operation time between 3D-printed AVB (n = 171) and conventional TMC (n = 179) groups. A random-effects model was employed for this parameter because the heterogeneity among the studies was significant (P < 0.00001, I² = 90%). The standard mean difference in the operation time between two groups was -0.78, significantly inclined toward the conventional TMC group (95% CI: -1.53 to -0.03, P = 0.04, Figure 3). No sources of heterogeneity were identified after the sensitivity and meta-regression analyses (Tables 2, 3). Therefore, a qualitative descriptive analysis was performed, and the results showed that four studies (12, 16–18) reported that the operation time of the 3D-printed AVB group was significantly shorter than that of the conventional TMC group, whereas the remaining four studies (13–15, 19) reported no difference between the two groups.
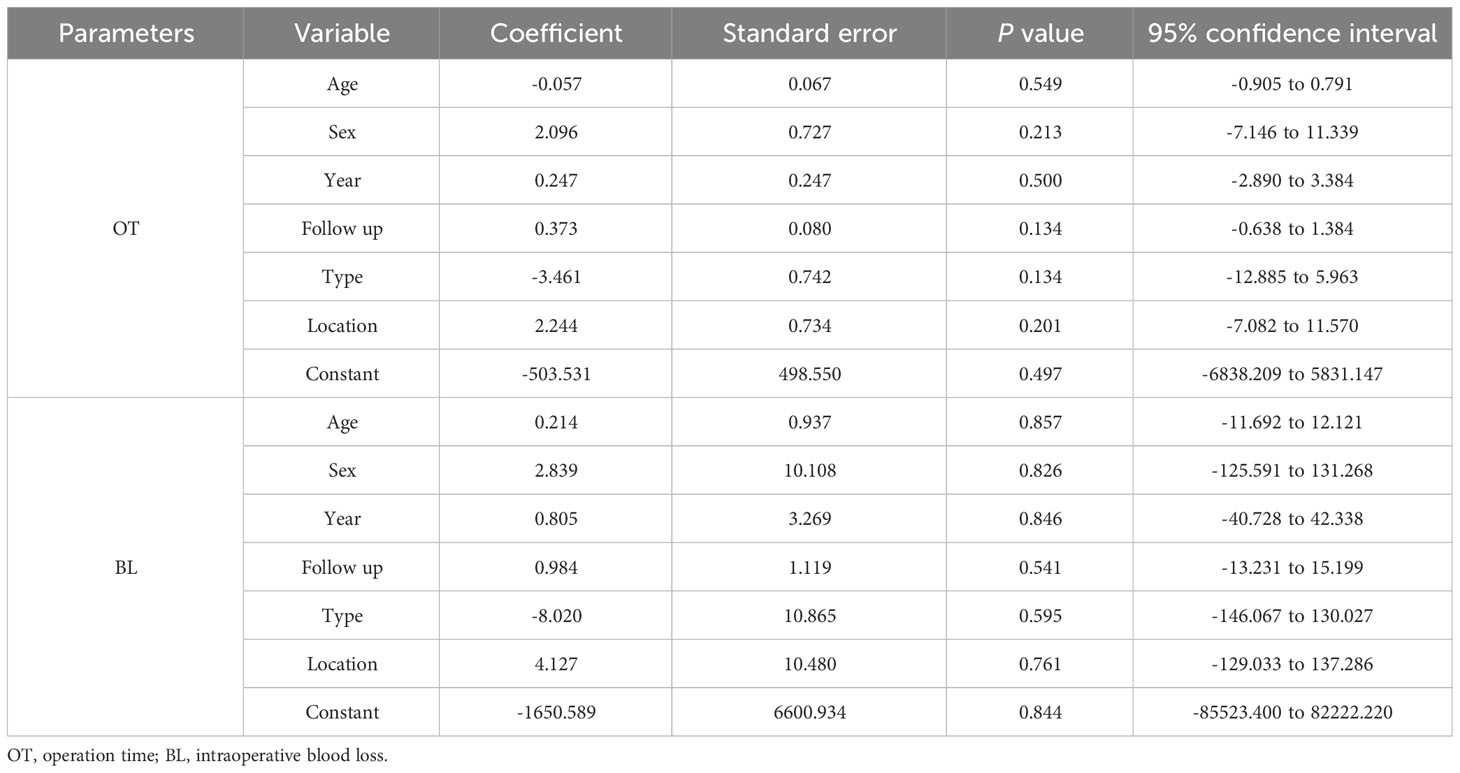
Table 3 Meta-regression analysis in operation time and intraoperative blood loss ratio between two groups.
3.3 Intraoperative blood loss
Eight studies compared the intraoperative blood loss between the 3D-printed AVB (n = 171) and conventional TMC (n = 179) groups (12–19). A random-effects model was employed for this parameter because the heterogeneity among the studies was significant (P < 0.00001, I² = 96%). The standard mean difference in blood loss between two groups was -1.87, significantly inclined toward the conventional TMC group (95% CI: -3.14 to -0.60, P = 0.004, Figure 4). No sources of heterogeneity were identified after the sensitivity and meta-regression analyses (Tables 2, 3). Therefore, a qualitative descriptive analysis was performed, and the results showed that five studies (12, 13, 16–18) reported significantly less intraoperative blood loss in the 3D-printed AVB group than in the conventional TMC group, whereas the remaining three studies (14, 15, 19) reported no significant difference between the two groups.
3.4 VAS scores
The VAS scores were subclassified according to follow-up time: preoperative and postoperative scores. The preoperative VAS score between 3D-printed AVB (n = 115) and conventional TMC (n = 126) groups was compared in five studies (14, 16–19). The results showed that the 3D-printed AVB group had a higher preoperative VAS score than that in the conventional TMC group, but the difference was also not statistically significant (WMD = 0.06, 95% CI: -0.26 to 0.38, P = 0.73; I² = 0%, fixed-effects model; Figure 5). Six studies (12, 14, 16–19) compared the postoperative VAS score between the 3D-printed AVB (n = 145) and conventional TMC (n = 156) groups. However, the results showed that the 3D-printed AVB group achieved a significantly lower postoperative VAS score than that achieved by the conventional TMC group (WMD = -0.21, 95% CI: -0.39 to -0.04, P = 0.02; I² = 0%, fixed-effects model; Figure 5).
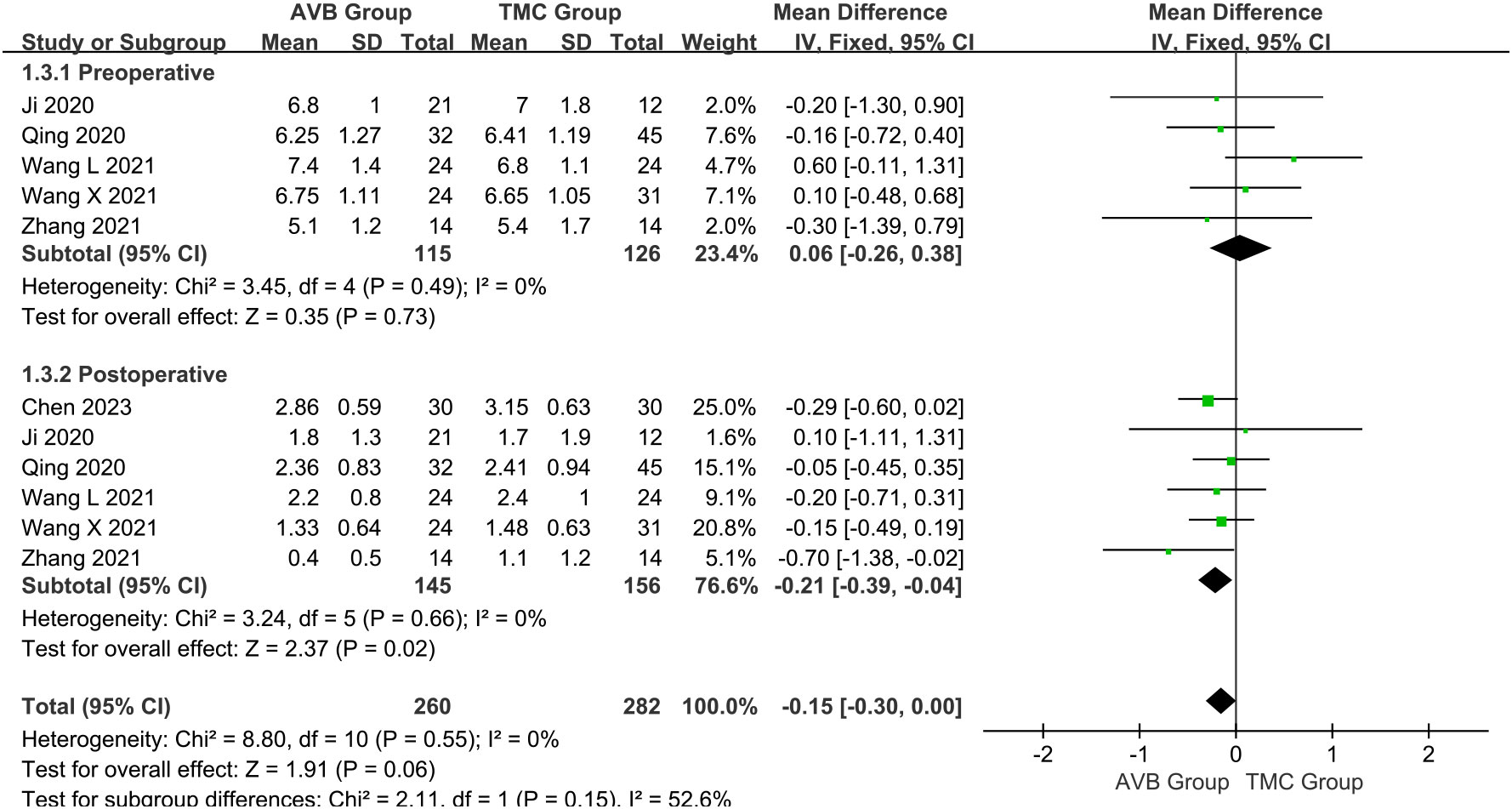
Figure 5 Forest plot of the preoperative and postoperative visual analogue scale scores in the two groups.
3.5 Frankel classification
The Frankel classification was divided into: before and after surgery. Three studies (16, 18, 19) compared the preoperative Frankel classification between the 3D-printed AVB (n = 70) and conventional TMC (n = 90) groups. The results showed that the preoperative Frankel classification in the 3D-printed AVB group was the same as that in the conventional TMC group (WMD = 0, 95% CI: -0.24 to 0.23, P = 0.97; I² = 0%, fixed-effects model; Figure 6). The postoperative Frankel classification between the 3D-printed AVB (n = 78) and conventional TMC (n = 100) groups was compared in four studies (15, 16, 18, 19). The weighted mean difference in the postoperative Frankel classification between the two groups was -0.08, which non-significantly inclined toward the conventional TMC group (95% CI: -0.38 to 0.22, P = 0.60; I² = 0%, fixed-effects model; Figure 6).
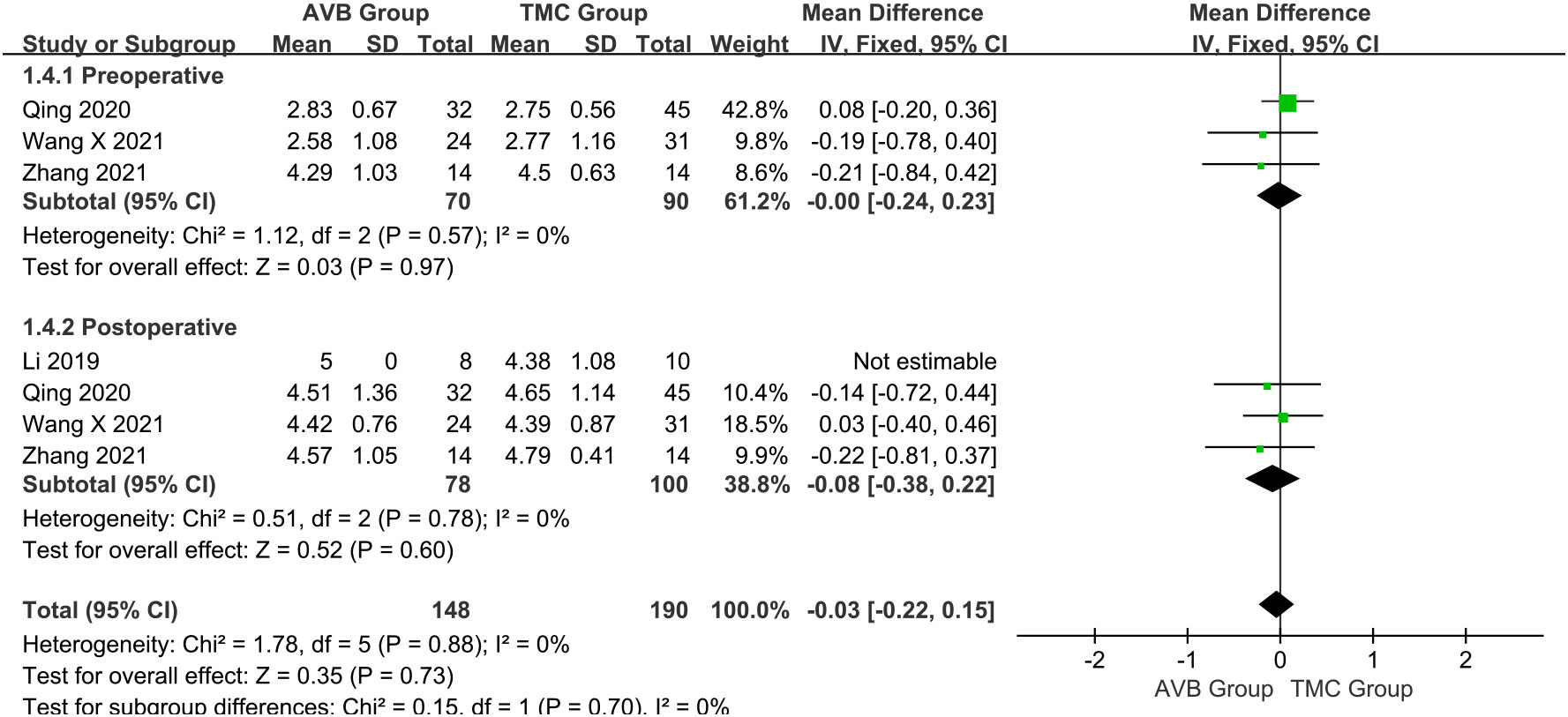
Figure 6 Forest plot of the preoperative and postoperative Frankel classification in the two groups.
3.6 Vertebral body subsidence
The occurrence of vertebral body subsidence between 3D-printed AVB (n = 68) and conventional TMC (n = 72) groups was compared in four studies (14, 18–20). The OR in vertebral body subsidence between two groups was 0.08, significantly inclined toward conventional TMC group (95% CI: 0.03 to 0.27, P < 0.0001; I² = 0%, fixed-effects model; Figure 7).
3.7 Early complications
Early complications were mainly those that occurred during the surgery or during the recovery period after the surgery. Early complications mainly included cerebrospinal fluid leakage, pleural effusion or lung infection due to pleural rupture, poor healing of infected wounds, and neurological complications. The occurrence of early complications between 3D-printed AVB (n = 150) and conventional TMC (n = 167) groups was compared in seven studies (12, 13, 15–19). The OR in early complications between two groups was 0.52, significantly inclined toward the conventional TMC group (95% CI: 0.29 to 0.90, P = 0.02; I² = 40%, fixed-effects model; Figure 8).
3.8 Sensitivity and subgroup analyses
The results showed that the heterogeneity of the operation time and intraoperative blood loss was high. A random-effects model was employed to partially eliminate the effect of heterogeneity, but it was still high. After removing each included article separately for operation time and intraoperative blood loss (Table 2), we found that heterogeneity did not decrease significantly, but it did not affect the results, which shows that the results of this meta-analysis are relatively reliable. And we conducted a qualitative descriptive analysis of operation time and intraoperative blood loss.
In addition, the tumor pathologies and types in the nine included studies are presented in Supplementary Table 1. Based on the pathological tumor diagnosis, we divided these studies into Type 1 (primary), Type 2 (primary and metastatic), and Type 3 (metastatic) for subgroup analysis to eliminate heterogeneity.
For the operation time Type 2 subgroup, a random-effects model was employed because the heterogeneity among the studies was still significant (P < 0.00001, I² = 94%). The standard mean difference in this subgroup between the two groups was -0.78, which non-significantly inclined toward the conventional TMC group (95% CI: -2.10 to 0.54, P = 0.25; Figure 9). For the intraoperative blood loss Type 2 subgroup, a random-effects model was employed because the heterogeneity among the studies was still significant (P < 0.00001, I² = 97%). The standard mean difference in this subgroup between the two groups was -2.82, significantly inclined toward the conventional TMC group (95% CI: -5.29 to -0.35, P = 0.03; Figure 10). The subgroup analysis did not eliminate heterogeneity. Qualitative descriptive analysis showed that two studies (16, 18) reported significantly shorter operation time and less intraoperative blood loss in the 3D-printed AVB group than in the conventional TMC group, whereas the remaining three studies (14, 15, 19) reported no difference between the two groups.
3.9 Meta-regression analysis
Table 3 shows the results of the meta-regression analysis of the operation time and intraoperative blood loss between the 3D-printed AVB and conventional TMC groups in terms of age, sex, publication year, follow-up time, tumor type, and location. The results showed that these variables did not significantly affect the mean difference in operation time or intraoperative blood loss between the two groups (all P > 0.05).
3.10 Publication bias analysis
The results of the publication bias analysis are shown in Table 4 and Supplementary Figures 1–8. We constructed funnel plots to evaluate publication bias (Supplementary Figures 1–8), and the results were largely symmetrical except for intraoperative blood loss, indicating that publication bias was acceptable in our analysis. The Egger’s test was performed for further evaluation (Table 4). The results revealed that publication bias existed in terms of the intraoperative blood loss between the two groups (P = 0.032).

Table 4 Publication bias analysis (Egger’s test) in operation time and intraoperative blood loss between two groups.
4 Discussion
With the in-depth studies of spinal tumors and the development of adjuvant therapy, the survival time of patients has been significantly prolonged, greatly increasing the chances of surgical intervention. Two main points must be considered in the surgical treatment of spinal tumors: total en bloc resection of the tumors, which can reduce local recurrence, and spinal reconstruction. Tomita et al. (24) proposed TES as an important surgical method for the en bloc resection of spinal tumors. The Weinstein-Boriani-Biagini (WBB) classification can describe the anatomical location of spinal tumors, growth depth of tumors around the spinal cord, and invasion of soft tissue around the spine, as well as personalized surgical resection guidance for spinal tumors of different shapes (25). Compared with the TES, en bloc resection under the guidance of the WBB classification increases the difficulty of the surgical operation; however, it can formulate a more stringent tumor-free osteotomy plan (26). Because few studies on this procedure met the inclusion criteria, our results focused mainly on patients undergoing TES.
The most widely used reconstruction method is TMC combined with autologous or allograft bone (27). As emerging implants for spinal reconstruction, 3D-printed AVB offer advantages in conformal matching and osseointegration (28). After including nine studies, our results confirmed that 3D-printed AVB was superior to conventional TMC in terms of reducing operation time and intraoperative blood loss, reducing postoperative VAS score, and reducing the occurrence of vertebral body subsidence and early complications, which supports the hypothesis that the clinical efficacy and safety in the 3D-printed AVB group were superior to those in the conventional TMC group.
The Ti6Al4V is an important material for 3D-printed AVB because of its excellent mechanical properties and tissue biocompatibility (29). By the microporous structure loading nano-zinc oxide composite hydroxyapatite, antibiotics, and anti-tumor drugs, it can elevate osseointegration between the host bone and implants and prevent infection and tumor recurrence (30, 31). The 3D-printed AVBs in the nine included studies were fabricated from Ti6Al4V. Recently, Wang et al. (32) and Guo et al. (33) found that compared with Ti6Al4V implants, porous tantalum implants with a pore size of 400 μm had better cell adhesion and proliferation; radiological analyses showed that the new bone formation rate of tantalum implants was significantly higher; mechanical analyses showed that tantalum implants had controllable elastic modulus and compressive strength. Tantalum is expected to become the focus for 3D-printed AVB metals in the future. Although 3D-printed AVB have been widely used clinically, most available studies involved early efficacy evaluations and long-term follow-up studies of large-scale cases are lacking.
The spinal tumor type, location, size, number of vertebral bodies involved, surgical approach, and operator experience all impact the operation time and intraoperative blood loss (34). The richness of the tumor blood supply and whether preoperative vascular embolization therapy can also cause changes in intraoperative blood loss. Zhou et al. (35) believed that in traditional surgery, operators repeatedly endeavored to select suitable interbody fusion cages, which increased the operation time and intraoperative blood loss. In 3D-printed AVB surgery, the entire vertebral body is scanned and modeled by CT or MRI before surgery, thus avoiding the repeated selection of suitable interbody fusion cages, shortening the operation time, and reducing the blood loss (36). Our results showed that both operation time and blood loss were significantly different between the two groups; however, the reliability of the results was questionable because of the high heterogeneity among the studies. Therefore, whether implanting a 3D-printed AVB for spinal reconstruction during TES can significantly shorten the operation time and reduce intraoperative blood loss requires further verification.
The subsidence of implants can easily lead to the loss of spinal height and physiological curvature, adjacent vertebral fracture, or nail-rods fracture, etc., resulting in severe instability of the spine. Therefore, reducing the occurrence of implants subsidence is key to maintaining the long-term stability of spinal reconstruction. When the subsidence height is greater than 5 mm, the fixation system is prone to fracture due to overstress, resulting in the failure of vertebral body reconstruction (27). The main reason for this phenomenon is that the elastic moduli of conventional TMC are not consistent with those of normal human bones, leading to a stress shielding effect. Zhang et al. (19) noticed that the stress shielding effect could cause the loss of adjacent vertebral bone tissue and osteoporosis, resulting in the displacement of implants, formation of an angle with the endplate of the adjacent vertebral body, and even fracture of implants. Excessive stress and sharp edges can also cause adjacent vertebral fractures, directly destroying spinal stability (27). Our study found that the incidence of vertebral body subsidence was lower in the 3D-printed AVB group than that in the conventional TMC group. The 3D-printed AVB was designed according to the location of the tumor and physiological curvature of the spine. The large contact area is conducive to the adhesion of bone tissue cells and provides good stability (37). Its three-dimensional microporous structure renders it high in strength and low in modulus, and it is less prone to the stress shielding effect. Some studies have confirmed that the microporous design of 3D-printed AVB can create an osteogenic environment suitable for bone morphogenetic protein 2 (BMP2), BMP4, and BMP7; promote the differentiation and maturation of osteoblasts; and facilitate earlier completion of osseous fusion (38, 39).
In addition, implant subsidence is also related to many other factors. Bone density is positively correlated with the maximum load on the vertebral endplate; therefore, patients with osteoporosis are more likely to experience subsidence (6). Bao et al. (40) found that preoperative or postoperative radiotherapy and resection of more than 2 vertebral body segments were independent risk factors for implants failure. Radiotherapy does not cause fracture of bone collagen but changes the biomechanical characteristics of the bone matrix, resulting in reduced fatigue resistance (41). Multi-segment resection can lead to insufficient blood supply to the surrounding tissues, which prolongs bone fusion time and affects spinal stability. Weber et al. (42) also noticed that excessive intervertebral space distraction increased the load between the implants and vertebral bodies, and the distraction height was positively correlated with the subsidence rate. Moreover, some studies have reported that the subsidence of implants may be related to the patient’s age, sex, and smoking status (6, 42). Since the included studies’ data are incomplete and have a low level of evidence, we are conducting a prospective and large-scale cases study to explore factors influencing implants subsidence after total en bloc spondylectomy and spinal reconstruction of spinal tumors.
When TES is performed for spinal tumors, normal tissue structures such as blood vessels and nerves are inevitably damaged during the operation, leading to severe trauma, prolonged operation, and multiple complications (43). Early complications mainly include pleural effusion, cerebrospinal fluid leakage, and infection, whereas late complications mainly include the failure of internal fixation systems, such as broken nails and broken rods. Based on our results, the incidence of early complications was significantly lower in the 3D-printed AVB group than in the conventional TMC group. We believe that this difference is not only related to the type of implants, but also to the experience of the surgeon and the preoperative patient’s physical condition. If not treated on time, early complications will affect the stability of implants fusion and spinal reconstruction and even cause late complications.
Although this study revealed some important discoveries, some limitations should be acknowledged. First, this was a systematic review and meta-analysis, and all nine studies included were non-randomized controlled trials with a low level of evidence. Second, only nine studies were included, all of which were from China; therefore, a certain publication bias existed. We performed sensitivity, subgroup, and publication bias analyses for the operation time and intraoperative blood loss, but sensitivity and subgroup analyses could not eliminate heterogeneity, and publication bias of intraoperative blood loss was found. Third, the results have a selection bias due to the differences in tumor type, location, size, number of vertebral bodies involved, and surgical approach. However, due to the insufficient number of included studies and missing data, we could only perform meta-regression analysis for the tumor type and location, but not the rest. Therefore, these limitations should be noted when generalizing the results. The results require further validation in the future as more high-quality studies become available.
5 Conclusion
As the first systematic review and meta-analysis comparing the efficacy and safety of 3D-printed vertebral bodies and conventional TMC in spinal reconstruction after total en bloc spondylectomy for spinal tumors, our findings demonstrate that 3D-printed AVB is superior to conventional TMC in reducing operation time, intraoperative blood loss, postoperative VAS score, and occurrence of vertebral body subsidence and early complications.
Data availability statement
The original contributions presented in the study are included in the article/Supplementary Material. Further inquiries can be directed to the corresponding author.
Author contributions
MD: Conceptualization, Investigation, Methodology, Writing – original draft, Writing – review & editing. YJG: Data curation, Writing – original draft. HF: Supervision, Writing – original draft. YW: Visualization, Writing – review & editing. JL: Formal analysis, Writing – original draft. JB: Supervision, Writing – original draft. PS: Methodology, Supervision, Writing – original draft. YG: Data curation, Methodology, Writing – original draft. ZL: Supervision, Validation, Writing – original draft. YF: Resources, Supervision, Validation, Visualization, Writing – original draft, Writing – review & editing.
Funding
The author(s) declare that no financial support was received for the research, authorship, and/or publication of this article.
Conflict of interest
The authors declare that the research was conducted in the absence of any commercial or financial relationships that could be construed as a potential conflict of interest.
Publisher’s note
All claims expressed in this article are solely those of the authors and do not necessarily represent those of their affiliated organizations, or those of the publisher, the editors and the reviewers. Any product that may be evaluated in this article, or claim that may be made by its manufacturer, is not guaranteed or endorsed by the publisher.
Supplementary material
The Supplementary Material for this article can be found online at: https://www.frontiersin.org/articles/10.3389/fonc.2024.1327319/full#supplementary-material
References
1. Bell JS, Koffie RM, Rattani A, Dewan MC, Baticulon RE, Qureshi MM, et al. Global incidence of brain and spinal tumors by geographic region and income level based on cancer registry data. J Clin Neurosci: Off J Neurosurgical Soc Australasia (2019) 66:121–7. doi: 10.1016/j.jocn.2019.05.003
2. Chatain GP, Finn M. Compassionate use of a custom 3D-printed sacral implant for revision of failing sacrectomy: case report. J Neurosurg Spine (2020) 33:1–6. doi: 10.3171/2020.3.SPINE191497
3. Jones M, Holton J, Hughes S, Czyz M. Total en bloc spondylectomy. J Spine Surg (Hong Kong) (2018) 4(3):663–5. doi: 10.21037/jss.2018.06.12
4. Chen Z, Lü G, Wang X, He H, Yuan H, Pan C, et al. Is 3D-printed prosthesis stable and economic enough for anterior spinal column reconstruction after spinal tumor resection? A retrospective comparative study between 3D-printed off-the-shelf prosthesis and titanium mesh cage. Eur Spine J (2023) 32(1):261–70. doi: 10.1007/s00586-022-07480-9
5. Shinmura K, Demura S, Kato S, Yokogawa N, Handa M, Annen R, et al. A modified spinal reconstruction method reduces instrumentation failure in total en bloc spondylectomy for spinal tumors. Spine Surg related Res (2023) 7(1):60–5. doi: 10.22603/ssrr.2022-0111
6. Ji C, Yu S, Yan N, Wang J, Hou F, Hou T, et al. Risk factors for subsidence of titanium mesh cage following single-level anterior cervical corpectomy and fusion. BMC Musculoskelet Disord (2020) 21(1):32. doi: 10.1186/s12891-019-3036-8
7. Othman S, Bricker JT, Azoury SC, Elfanagely O, Weber KL, Kovach SJ. Allograft alone vs. Allograft with intramedullary vascularized fibular graft for lower extremity bone cancer: A systematic review and meta-analysis. J plastic reconstructive aesthetic surgery: JPRAS (2020) 73(7):1221–31. doi: 10.1016/j.bjps.2020.02.030
8. Kessler A, Hickel R, Reymus M. 3D printing in dentistry-state of the art. Oper Dent (2020) 45(1):30–40. doi: 10.2341/18-229-L
9. Maroulakos M, Kamperos G, Tayebi L, Halazonetis D, Ren Y. Applications of 3D printing on craniofacial bone repair: A systematic review. J Dent (2019) 80:1–14. doi: 10.1016/j.jdent.2018.11.004
10. Li Y, Wang H, Cui W, Li C. Comparison of 3D printed cervical spine with titanium mesh cage for fractures of lower cervical spine (in Chinese). Chin J Orthop Trauma (2018) 20(8):705–11. doi: 10.3760/cma.j.issn.1671-7600.2018.08.013
11. Chen J, Zhai S, Zhou H, Hu P, Liu X, Liu Z, et al. Implant materials for anterior column reconstruction of cervical spine tumor. Orthop Surg (2023) 15(5):1219–27. doi: 10.1111/os.13702
12. Chen Z, Guan K, Sun Y, Wang J, Huo H, Jin X. Clinical value of 3D printed personalized artificial vertebral weight construction for spinal tumor surgery (in Chinese). Guangdong Med J (2023) 44(1):96–101. doi: 10.13820/j.cnki.gdyx.20222191
13. Hu P, Du S, Wei F, Zhai S, Zhou H, Liu X, et al. Reconstruction after resection of C2 vertebral tumors: A comparative study of 3D-printed vertebral body versus titanium mesh. Front Oncol (2022) 12:1065303. doi: 10.3389/fonc.2022.1065303
14. Ji J, Hu Y, Miao J. Application of 3D printed porous artificial vertebra in reconstruction of thoracolumbar tumor (in Chinese). Chin J Orthops. (2020) 40(4):208–16. doi: 10.3760/cma.j.issn.0253-2352.2020.04.003
15. Li Z, Wei F, Liu Z, Liu X, Jiang L, Wu F, et al. Safety and mid-term follow-up results of en bloc resection for primary and metastatic spine tumors based on Weinstein-Boriani-Biagini classification (in Chinese). J Clin Orthopedics Res (2019) 4(5):261–7, 80. doi: 10.19548/j.2096-269x.2019.05.002
16. Qing P, Cao Y, Xu J, Deng H. Effectiveness analysis of using 3D printing technique in spinal reconstruction post excision of spinal tumors (in Chinese). China Med Equipment (2020) 17(1):109–12. doi: 10.3969/J.ISSN.1672-8270.2020.01.029
17. Wang L, Gao S, Liu J, Luo J, Zhang J, Zhong C, et al. Effect of 3D printing artificial vertebral body in reconstruction of spinal stability after total resection of spi-nal metastases (in Chinese). J Pract Med (2021) 37(23):3008–13. doi: 10.3969/j.issn.1006-5725.2021.23.010
18. Wang X, Ji Y, Leng Z, Shang G, Kou H, Song Z, et al. New 3D printed individualized artificial vertebral body for spinal reconstruction after spinal tumor resection (in Chinese). Chin J Exp Surgery. (2021) 38(6):1155–8. doi: 10.3760/cma.j.cn421213-20201023-01382
19. Zhang Y, Sun Y, Xiong W, Fang Z, Wang W, Shi Z, et al. Application of 3D-printed individual artificial vertebral body in reconstruction of thoracolumbar tumor after tobal en-bloc spondylectomy (in Chinese). Orthop Biomechanics Materials Clin Study (2021) 18(1):17–21, 6. doi: 10.3969/j.issn.1672-5972.2021.01.005
20. Zhou H, Wang R, Liu Z, Liu X, Wu F, Dang L, et al. 3D-printed vertebral body in anterior spinal reconstruction after total spondylectomy for patients with cervical chordoma (in Chinese). J Peking Univ (Health Sciences) (2023) 55(1):144–8. doi: 10.19723/j.issn.1671-167X.2023.01.022
21. Nha KW, Shon OJ, Kong BS, Shin YS. Gait comparison of unicompartmental knee arthroplasty and total knee arthroplasty during level walking. PloS One (2018) 13(8):e0203310. doi: 10.1371/journal.pone.0203310
22. Dong M, Fan H, Yang D, Sun X, Yan C, Feng Y. Comparison of spatiotemporal, kinematic, and kinetic gait characteristics in total and unicompartmental knee arthroplasty during level walking: A systematic review and meta-analysis. Gait posture (2023) 104:58–69. doi: 10.1016/j.gaitpost.2023.06.005
23. Page MJ, McKenzie JE, Bossuyt PM, Boutron I, Hoffmann TC, Mulrow CD, et al. The PRISMA 2020 statement: an updated guideline for reporting systematic reviews. BMJ (2021) 372:n71. doi: 10.1016/j.jclinepi.2021.03.001
24. Tomita K, Kawahara N, Baba H, Tsuchiya H, Fujita T, Toribatake Y. Total en bloc spondylectomy. A new surgical technique for primary Malignant vertebral tumors. Spine (1997) 22(3):324–33. doi: 10.1097/00007632-199702010-00018
25. Boriani S, Gasbarrini A, Bandiera S, Ghermandi R, Lador R. En bloc resections in the spine: the experience of 220 patients during 25 years. World Neurosurg (2017) 98:217–29. doi: 10.1016/j.wneu.2016.10.086
26. Shah AA, Paulino Pereira NR, Pedlow FX, Wain JC, Yoon SS, Hornicek FJ, et al. Modified en bloc spondylectomy for tumors of the thoracic and lumbar spine: surgical technique and outcomes. J Bone Joint Surg Am volume (2017) 99(17):1476–84. doi: 10.2106/JBJS.17.00141
27. Li Z, Wei F, Liu Z, Liu X, Jiang L, Yu M, et al. Risk factors for instrumentation failure after total en bloc spondylectomy of thoracic and lumbar spine tumors using titanium mesh cage for anterior reconstruction. World Neurosurg (2020) 135:e106–e15. doi: 10.1016/j.wneu.2019.11.057
28. Zhou H, Liu S, Li Z, Liu X, Dang L, Li Y, et al. 3D-printed vertebral body for anterior spinal reconstruction in patients with thoracolumbar spinal tumors. J Neurosurg Spine (2022) 37:1–9. doi: 10.3171/2022.1.SPINE21900
29. Attarilar S, Salehi MT, Al-Fadhalah KJ, Djavanroodi F, Mozafari M. Functionally graded titanium implants: Characteristic enhancement induced by combined severe plastic deformation. PloS One (2019) 14(8):1:e022149. doi: 10.1371/journal.pone.0221491
30. Zhang Y, Liu X, Li Z, Zhu S, Yuan X, Cui Z, et al. Nano Ag/ZnO-Incorporated hydroxyapatite composite coatings: highly effective infection prevention and excellent osteointegration. ACS Appl materials interfaces (2018) 10(1):1266–77. doi: 10.1021/acsami.7b17351
31. Zhang T, Wei Q, Zhou H, Jing Z, Liu X, Zheng Y, et al. Three-dimensional-printed individualized porous implants: A new “implant-bone” interface fusion concept for large bone defect treatment. Bioact Mater (2021) 6(11):3659–70. doi: 10.1016/j.bioactmat.2021.03.030
32. Wang H, Su K, Su L, Liang P, Ji P, Wang C. Comparison of 3D-printed porous tantalum and titanium scaffolds on osteointegration and osteogenesis. Materials Sci Eng C Materials Biol applications. (2019) 104:109908. doi: 10.1016/j.msec.2019.109908
33. Guo Y, Xie K, Jiang W, Wang L, Li G, Zhao S, et al. In vitro and in vivo study of 3D-printed porous tantalum scaffolds for repairing bone defects. ACS Biomater Sci Eng (2019) 5(2):1123–33. doi: 10.1021/acsBiomater.8b01094
34. Cheng H, Luo G, Xu D, Li Y, Wang Z, Yang H, et al. Comparison of radiological and clinical outcomes of 3D-printed artificial vertebral body with Titanium mesh cage in single-level anterior cervical corpectomy and fusion: A meta-analysis. Front Surg (2022) 9:1077551. doi: 10.3389/fsurg.2022.1077551
35. Zhou E, Huang H, Zhao Y, Wang L, Fan Y. The effects of titanium mesh cage size on the biomechanical responses of cervical spine after anterior cervical corpectomy and fusion: A finite element study. Clin biomechanics (Bristol Avon) (2022) 91:105547. doi: 10.1016/j.clinbiomech.2021.105547
36. Jin YZ, Zhao B, Lu XD, Zhao YB, Zhao XF, Wang XN, et al. Mid- and long-term follow-up efficacy analysis of 3D-printed interbody fusion cages for anterior cervical discectomy and fusion. Orthop Surg (2021) 13(7):1969–78. doi: 10.1111/os.13005
37. Dong C, Wei H, Zhu Y, Zhou J, Ma H. Application of titanium alloy 3D-printed artificial vertebral body for stage III kümmell’s disease complicated by neurological deficits. Clin Interventions aging (2020) 15:2265–76. doi: 10.2147/CIA.S283809
38. Fang T, Zhang M, Yan J, Zhao J, Pan W, Wang X, et al. Comparative analysis of 3D-printed artificial vertebral body versus titanium mesh cage in repairing bone defects following single-level anterior cervical corpectomy and fusion. Med Sci monitor (2021) 27:e928022. doi: 10.12659/MSM.928022
39. Liu Z, Xu Z, Wang X, Zhang Y, Wu Y, Jiang D, et al. Construction and osteogenic effects of 3D-printed porous titanium alloy loaded with VEGF/BMP-2 shell-core microspheres in a sustained-release system. Front Bioeng Biotechnol (2022) 10:1028278. doi: 10.3389/fbioe.2022.1028278
40. Bao WD, Jia Q, Wang T, Lou Y, Jiang DJ, Yang C, et al. Factors related to instrumentation failure in titanium mesh reconstruction for thoracic and lumbar tumors: retrospective analysis of 178 patients. Cancer Manage Res (2021) 13:3345–55. doi: 10.2147/CMAR.S294616
41. Bartlow CM, Mann KA, Damron TA, Oest ME. Altered mechanical behavior of demineralized bone following therapeutic radiation. J orthopaedic Res (2021) 39(4):750–60. doi: 10.1002/jor.24868
42. Weber MH, Fortin M, Shen J, Tay B, Hu SS, Berven S, et al. Graft subsidence and revision rates following anterior cervical corpectomy: A clinical study comparing different interbody cages. Clin Spine Surg (2017) 30(9):E1239–e45. doi: 10.1097/BSD.0000000000000428
Keywords: 3D-printed artificial vertebral body, titanium mesh cage, spinal tumor, total en bloc spondylectomy, meta-analysis
Citation: Dong M, Gao Y, Fan H, Wang Y, Lv J, Bai J, Shao P, Gao Y, Lv Z and Feng Y (2024) Comparison of clinical efficacy of 3D-printed artificial vertebral body and conventional titanium mesh cage in spinal reconstruction after total en bloc spondylectomy for spinal tumors: a systematic review and meta-analysis. Front. Oncol. 14:1327319. doi: 10.3389/fonc.2024.1327319
Received: 24 October 2023; Accepted: 22 January 2024;
Published: 06 February 2024.
Edited by:
Patricia Sullivan, Rhode Island Hospital, United StatesReviewed by:
Johannes Wach, University Hospital Leipzig, GermanyEdvin Zekaj, Galeazzi Orthopedic Institute (IRCCS), Italy
Copyright © 2024 Dong, Gao, Fan, Wang, Lv, Bai, Shao, Gao, Lv and Feng. This is an open-access article distributed under the terms of the Creative Commons Attribution License (CC BY). The use, distribution or reproduction in other forums is permitted, provided the original author(s) and the copyright owner(s) are credited and that the original publication in this journal is cited, in accordance with accepted academic practice. No use, distribution or reproduction is permitted which does not comply with these terms.
*Correspondence: Yi Feng, ZmVuZ3lpMTYwQDEyNi5jb20=