- 1Department of Radiology, University of Florida College of Medicine, Jacksonville, FL, United States
- 2Division of Interventional Radiology, Department of Radiology, Massachusetts General Hospital and Harvard Medical School, Boston, MA, United States
- 3Department of Microbiology and Immunology, College of Arts and Sciences, University of Miami, Coral Gables, FL, United States
- 4Department of Hospital Medicine, Flowers Hospital, Dothan, AL, United States
Colorectal malignancy is the third most common cancer and one of the prevalent causes of death globally. Around 20-25% of patients present with metastases at the time of diagnosis, and 50-60% of patients develop metastases in due course of the disease. Liver, followed by lung and lymph nodes, are the most common sites of colorectal cancer metastases. In such patients, the 5-year survival rate is approximately 19.2%. Although surgical resection is the primary mode of managing colorectal cancer metastases, only 10-25% of patients are competent for curative therapy. Hepatic insufficiency may be the aftermath of extensive surgical hepatectomy. Hence formal assessment of future liver remnant volume (FLR) is imperative prior to surgery to prevent hepatic failure. The evolution of minimally invasive interventional radiological techniques has enhanced the treatment algorithm of patients with colorectal cancer metastases. Studies have demonstrated that these techniques may address the limitations of curative resection, such as insufficient FLR, bi-lobar disease, and patients at higher risk for surgery. This review focuses on curative and palliative role through procedures including portal vein embolization, radioembolization, and ablation. Alongside, we deliberate various studies on conventional chemoembolization and chemoembolization with irinotecan-loaded drug-eluting beads. The radioembolization with Yttrium-90 microspheres has evolved as salvage therapy in surgically unresectable and chemo-resistant metastases.
1 Introduction
Colorectal cancer (CRC) is the third most prevalent malignancy in the United States and the third most common cause of death pertinent to cancer (1). The incidence of CRC has been increasing by approximately 3.2% per year and 2.5 million cases are estimated to be diagnosed in 2035 (2, 3). Around 56% of the patients lose their life from CRC (4). The mortality could be attributed to distant organ metastases noticed in 25% of patients at the time of initial diagnosis and in 50% of patients during disease progression (5). The 5-year survival rate of CRC confined to primary location is 88-91.1%, while the rate falls to 13.3-14% in metastatic CRC (6). Liver (68-75%) followed by lung (21-33%), distant lymph nodes (16-26%), bone (10.7-23.7%), peritoneum (11-15%), brain (0.3-0.6%), adrenal glands and spleen are the most to least common sites of CRC metastases (7, 8).
Synchronous colorectal cancer liver metastases (CRLM) are encountered in 20-25% of CRC patients whereas metachronous CRLM is observed in 20-30% of CRC patients (9, 10). Untreated CRLM has worse prognosis with a median survival of 4.5 to 12 months subject to the extent of disease at diagnosis (10). The intention of any curative treatment is to achieve the R0 resection of both the primary and metastatic tumor. Surgical resection is the potential curative and gold standard treatment for CRLM (11). It has improved the 5-year overall survival (OS) rate to 24-58% and a 10-year survival rate to 28% (10, 12–16). Although 50-60% of patients benefit from curative surgical resection of CRLM, only 10-25% of patients are suitable for surgery owing to tumor anatomy, extrahepatic involvement and general health status (10, 17, 18). Neoadjuvant systemic chemotherapy allows for sufficient tumor shrinkage for resection in merely 10-30% of non-surgical candidates (19). Current chemotherapy regimens include 5-fluorouracil and oxaliplatin (FOLFOX), 5-FU and irinotecan (FOLFIRI), and capecitabine and oxaliplatin (CapOx). These regimens have a response rate of 40% and an OS of 57% at 15-20 months (20). The addition of biologic agents to systemic chemotherapy such as anti-vascular endothelial and anti-epidermal growth factors inhibitors has improved the OS to >24 months (20). However, these systemic therapies are intolerable to a 1/3rd of patients resulting in discontinuation of treatment. A few patients may experience chemotherapy-associated liver injury (CALI) including sinusoidal obstruction syndrome and steatohepatitis (20). Hence, the demand for locoregional therapies has increased to make the tumor amenable to resection in addition to mitigating unwanted side effects of chemotherapy. Minimally invasive interventional therapies such as percutaneous ablation, trans-arterial chemoembolization (TACE), trans-arterial radioembolization (TARE) and portal vein embolization (PVE) have transformed the management algorithms of CRLM. These therapies improve the candidacy for surgical resection, provide curative treatment options for non-surgical candidates, and improve the survival of patients undergoing palliative care (Table 1).
2 Therapies to improve surgical candidacy
2.1 Portal vein embolization
One of the main limitations of curative surgical resection is the presence of low volume of the future liver remnant (FLR), which might lead to hepatic insufficiency following the surgery (21). In the last few decades, various techniques have been introduced to induce hypertrophy of the FLR, thereby preventing the liver failure. In 1980s, Masatoshi Makuuchi introduced PVE of right portal vein to cause hypertrophy of the left lobe of the liver (22). PVE diverts blood flow to the healthy liver through embolization of the portal vein branches of the diseased liver. This results in atrophy of the embolized liver and hypertrophy of the non-embolized liver (Figure 1). The resultant increased FLR makes it possible to resect the large or multiple liver tumors. The exact mechanism of liver atrophy-hypertrophy following PVE remains unclear. However, it is hypothesized to be due to (i) upregulated cytokines and growth factors during liver regeneration, (ii) restituted increase in hepatic arterial perfusion and (iii) cellular host response enhancing the local tumor growth (23).
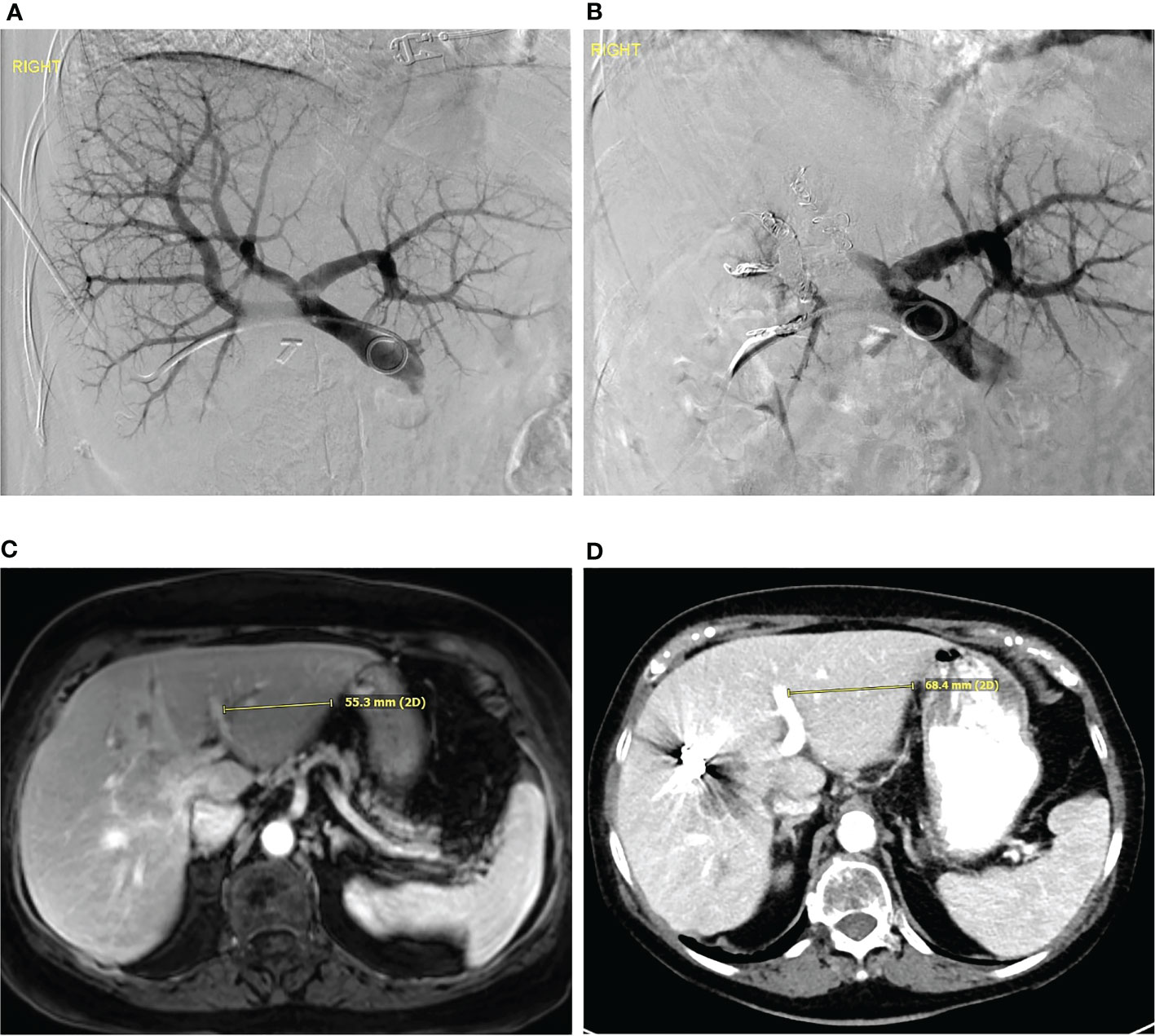
Figure 1 Portal vein embolization (A) Digitally subtracted percutaneous transhepatic portovenogram demonstrates patent main, left and right portal veins. The portal vein was embolized with particles followed by coil embolization. (B) Digitally subtracted portovenogram after portal vein embolization demonstrates flow only to the left hepatic lobe. (C) Pre-procedure MRI and (D) post portal vein embolization CT demonstrates hypertrophy of the left hepatic lobe.
PVE has become the standard of practice for patients with inadequate FLR prior to extensive hepatic resection. The FLR of <20% in the normal liver, <30% in the liver with chemotherapeutic exposure, and <40% in the cirrhotic liver is usually considered an indication of PVE (11, 24–27). The liver regenerates by 20-46% in 6-8 weeks following the procedure (28). The resection rate after PVE is reported to be around 60-80%, 20% of patients may present with insufficient FLR hypertrophy or tumor progression (29–31). Other complications include tumor recurrence and accelerated tumor growth following the procedure (11). Tumor progression is the major concern as it affects the clinical and survival outcomes and may lead to unresectable disease. Pamecha et al. reported increased tumor growth rate among post-PVE cases compared to controls (0.36± 0.68 ml/day vs. 0.05± 0.25 ml/day; P=0.06) (Table 2) (29). For patients with high tumor load, defined as ≥ 9 CRLM or ≥ 5.5 cm diameter for the largest metastatic lesion, a liver transplant may be the preferred management for improved survival (32). Dueland et al. reported a 5-year survival rates of 33.4% and 6.7% in patients who underwent liver transplant and post-PVE liver resection respectively (32).
2.2 Lobar trans-arterial radioembolization
The external beam radiotherapy (EBRT) of the liver exposes the normal hepatic parenchyma to radiation, in addition to the target tumor tissue. Even 35-45Gy, a dose inadequate to induce tumor cell death, can cause radiation-induced liver disease in 50% of the patients due to the low radiation toxicity threshold of normal hepatic parenchyma (40, 41). TARE, also known as selective internal radiation therapy (SIRT) deploys microspheres made of glass or resin and loaded with Yttrium-90 (Y-90) into the hepatic tumor vasculature. The Y-90 TARE emits beta radiation to the selective tumor tissue in contrast to the whole hepatic parenchyma in EBRT. As the radiation is achieved through the infusion of Y-90 microspheres into the hepatic artery, the TARE technique is often referred to as “inside-out radiation” or brachytherapy (42). The Y-90 TARE delivers the radiation with a mean penetration of 2.5 mm, mean energy of 0.94 MeV and targeted radiation dose of 80-150 Gy to the tumors (43).
The concept of lobar TARE as a method to increase the FLR while also controlling the tumor growth in the diseased liver is recently popularized (Table 3). Teo et al. studied seven retrospective clinical studies involving the patients undergoing lobar TARE and reported a FLR hypertrophy of 26-47% within 1.5-9 months of the procedure (47). However, Nebelung et al. reported a significantly greater hypertrophy in patients after PVE than lobar TARE (25.3% vs. 7.4%; P<0.001) (45). However, the post-TARE hypertrophy was substantial with a minimized risk of tumor progression in the embolized lobe (48). Edeline et al. stated that the increase in FLR was similar after TARE as well as PVE procedures (49). Kurilova et al. reported two cases reports in which the patients had insufficient FLR post PVE and underwent lobar TARE. They observed 13% increase in FLR at 4-week follow up in the first patient and 59% increase in FLR at 7-week follow-up in the second patient (50). Liebl et al. studied the FLR hypertrophy in pigs and reported that although PVE induced rapid FLR hypertrophy, it reached a plateau after I month of procedure, whereas, TARE resulted in FLR comparable to PVE within 3-6 months of procedure (51). Vouche et al. studied 83 patients with unilobar disease and observed a reduction in the tumor volume from 134 cc to 56 cc during >9 month follow up period (46). Another study by Edeline et al., including 34 patients, delivered a median lobar dose of 122 Gy and observed a complete response rate of 0%, partial response rate of 26%, stable disease in 63%, and progression of disease in 3% of patients based on RECIST criteria (49). However, CR, PR, SD and PD of 30, 33, 30 and 2% were reported based on mRECIST criteria. Edeline et al. also reported a median OS of 13.5 months and a median time to tumor progression of 21.7 months (49). The lobar TARE has the advantage of tumor control and biological test of time for extrahepatic tumor progression prior to liver resection. Lobar TARE is a well-tolerated procedure with very minimal side effects such as pain and nausea. A few studies reported an increase in Child-Pugh score from 6 to 7 during the first 6 months follow-up which improved later during the >6-9 month follow up period (52). A > 20% increase in the splenic volume was reported without any signs of hypersplenism or additional findings of portal hypertension (52). Serious toxicities including irreversible ascites, temporary and progressive hyperbilirubinemia, and variceal hemorrhage may be observed following the procedure (49).
2.3 Combined RFA and surgical resection
A few studies recommend the combination therapy of RFA with surgical resection to slightly improve the survival and recurrence risk compared to RFA alone (Table 4). Mima et al. studied the efficacy of RFA alone and RFA combined with hepatic resection in unresectable CRLM (53). RFA was mainly performed alongside hepatic resection in those patients who had an effective clinical response to preoperative chemotherapy (FOLFOX). Metastatic nodules smaller than 2 cm was the main indication for RFA while the contralateral tumor was for the hepatic resection. The 3-year recurrence free survival was 33.2% in hepatic resection alone group and 18.5% in combined hepatic resection+ RFA group. Although tumor recurrence was reported in both the group of patients, it was not statistically significant (P=0.154). The 3-year PFS was 45.3% in hepatic resection alone group compared to 12.8% in hepatic resection + RFA group (P= 0.472). The 3- and 5-year OS was 70.4% and 62.6% in hepatic resection group and 77.1% and 64.3% in the hepatic resection + RFA group (P= 0.627) (53). Mima et al. concluded that hepatic resection combined with RFA may be a safe and effective alternative after responsive chemotherapy (53) The similar conclusion was observed in a retrospective study by Sasaki et al. (54). They observed improved resection rates in the resection +RFA group compared to resection alone group (15.1% vs. 8.5%; P= 0.071) (54).
3 Therapies with curative intent
3.1 Ablation +/- systemic chemotherapy
Percutaneous thermal ablation is a tumor-destructive technique and is based on exposing the tumor cells to a targeted temperature of > 600 C or < -400 C. Ablation can be accomplished through thermal techniques such as radiofrequency, microwave, cryoablation, laser ablation, and focused ultrasound ablation. The irreversible electroporation (IRE), a nonthermal ablation technique utilizes an electrical field to induce tumor death without damaging the tissue protein architecture of vessels and the bile-ducts (55). Either thermal or non-thermal, ablation techniques have the advantages of being minimally invasive and less morbid than surgical resection and can be delivered as an out-patient treatment. The open or percutaneous approach to thermal ablation has been studied in the literature. Puijk et al., reported significantly improving liver tumor PFS following percutaneous ablation (2010-2013: 37.7%; 2014-2017: 69%; 2018-2021: 86.3%; P< 0.0001) whereas the PFS was stable following open ablations (2010-2013: 87.1%; 2014-2017: 92.7%; 2018-2021: 90.2%; P= 0.12) (56). The complications were less severe in percutaneous rather than open approach (2010-2013: P=0.69; 2014-2017: P= 0.129; 2018-2021: P= 0.02) (56). The tissue damage secondary to ablation is low when compared to surgical resection, which is the most important requisite in patients with underlying liver disease or those who already had extensive liver resection (55).
RFA is a well-studied and most widely used ablative modality in colorectal metastases. The monopolar or bipolar radiofrequency ablation (RFA) systems produce ionic oscillation by a high-frequency alternating current resulting in frictional heating and tissue damage (57). The level of thermal tissue damage varies depending on the achieved temperature. For instance, a 50-550 C for a period of 4-6 minutes induces irreversible cellular damage, 60-1000 C leads to irreversible coagulation of the cells and 100-1100 C results in vaporization and carbonization of tissue (58). The 1, 3, 5,10-year survival rates of CRLM following RFA are 98%, 69%, 48%, and 18% in a study by Solbiati et al. (59). Local tumor progression (LTP) after RFA, seen in 2-60% of cases, is an important factor to consider while ablating the CRLM. There are many factors that attribute to LTP including tumor size, tumor number, ablation zonal geometry, ablative margin, extrahepatic disease, location adjacent to large vessels and subcapsular tumors (60, 61). Radiofrequency ablation (RFA) is usually recommended in patients with ≤ 3-5 metastases of size ≤ 3-3.5 cm, not involving bile ducts or large vessels (≥3 mm), and not located centrally (62, 63).
Tumor size is critical in selecting patients for RFA as the commercially available devices can deliver the ablation to about 4-5 cm in one session and the studies reported high success rates of RFA in tumors ≤ 3-4 cm. In a study by Nielsen et al., the local recurrence after ablation was reported in 9%, 26.5%, and 45% of metastases measuring 0-3 cm, 3-5 cm and > 5 cm respectively (64). Compared to surgical resection, RFA has a lower complication rate (9.5%) and minimal risk of death (10, 65). However, it cannot replace surgical resection, especially in tumors > 3 cm size (57). The number of CRLM is also an important criterion when selecting the patients for RFA. Solitary CRLM is associated with high tumor control and survival rates. Kim et al. reported the 5-year survival and disease-free survival rates as 51% and 34% respectively in patients with solitary CRLM of size < 3 cm (66). Similarly, Gillams et al. studied the 5-year survival rate of solitary CRLM of size 2.3 cm to be 54% with a median survival of 63 months (67). Wang et al. studied the emphasis of ablative and tumor margins and reported that the risk of LTP decreases by 46% for every 5-mm increase in ablative margin size and increases by 22% with every 5 mm increase in tumor size (68). The tumor abutting large vessels causes convective heat loss termed as “heat-sink effect”, hence preventing heat accumulation in the tumor (63). A study by Elias et al. reported that 23% of CRLM, close to the large vessels, recured compared to 3% of CRLM located away from the vessels (69). In such situations, percutaneous balloon occlusion of large vessels during RFA has demonstrated improved tumor progression rates (62). Van Tilborg et al. studied that the centrally located CRLM recur more often compared to peripheral CRLM (21.4% vs. 6.5%; P= 0.009) (10).
Local tumor progression following RFA can be re-treated with repeat RFA, stereotactic body radiation therapy (SBRT), TACE, hepatic resection, and ultimately transplantation; however, with a high failure risk (70). The optimal choice among these techniques is still debatable, and a study by Xie et al. compared the repeat RFA with TACE and transplantation (70). In their study, repeat RFA has comparable outcomes with transplantation; hence the former is the primary choice, while the latter can be performed in patients where RFA failed or is inapplicable (70). Recently, CT-guided I125 brachytherapy has been studied in patients with recurrent HCC after thermal ablation. Its validation in recurrent CRLM is yet to be determined.
Other ablation techniques include microwave ablation (MWA), irreversible electroporation (IRE), and cryoablation. MWA has shown to be effective as an alternative to RFA and in a few cases, it is the preferred modality. The MWA generates heat by utilizing electromagnetic signals. Current machines operate between 900-2450 MHz, a frequency at which the microwaves cause coagulation necrosis by the oscillation of polarized water molecules which produce friction and heat (Figure 2) (57, 71). Compared to RFA, the size and zone of MWA are consistent and less affected by the heat-sink effect, impedance, penetrability, and thermal conductivity (72, 73). Gravante et al. examined the histopathological sample of MWA tissue and found no viable cells 6 cm away from the ablation zone in 93% of cases (74). Ierardi et al. included patients who are unfeasible to RFA such as those with tumors > 3 cm and are abutting larger vessels (> 3 mm) (73). They reported that the local recurrence was observed in 13% of patients with a disease-free OS of 20.5 months. Although no major complications were noticed, approximately 45% of patients had minor complications such as abdominal pain, fever with malaise, nausea, vomiting and elevated serum bilirubin levels (73). Pathak et al. reviewed various studies on RFA and MWA and reported that the local recurrence rates of CRLM after RFA and MWA to be ranging from 10-36% and 5-13% respectively (71). IRE is a non-thermal ablative technique that induces high-voltage electrical pulse waves between the electrodes (75). It is a safer ablation method in case of tumors close to the vascular or biliary structures due to the absence of the heat-sink effect (76, 77). The COLDFIRE-1 is a Phase-I study that demonstrated CRLM death and necrosis when exposed to IRE (78). COLDFIRE-2 is a Phase-II study including the patients with ≤ 5 cm CRLM, and it reported a 1-year PFS of 68%. Around 74% of the patients achieved local tumor control after the repeat IRE procedure (79). In a study by Schicho et al., 67% of patients achieved tumor control after the first IRE and 96% after re-intervention (80). Complications during IRE were reported to be observed in 40% of patients, with the most severe being arrhythmias, portal vein thrombosis, and biliary obstruction (79).
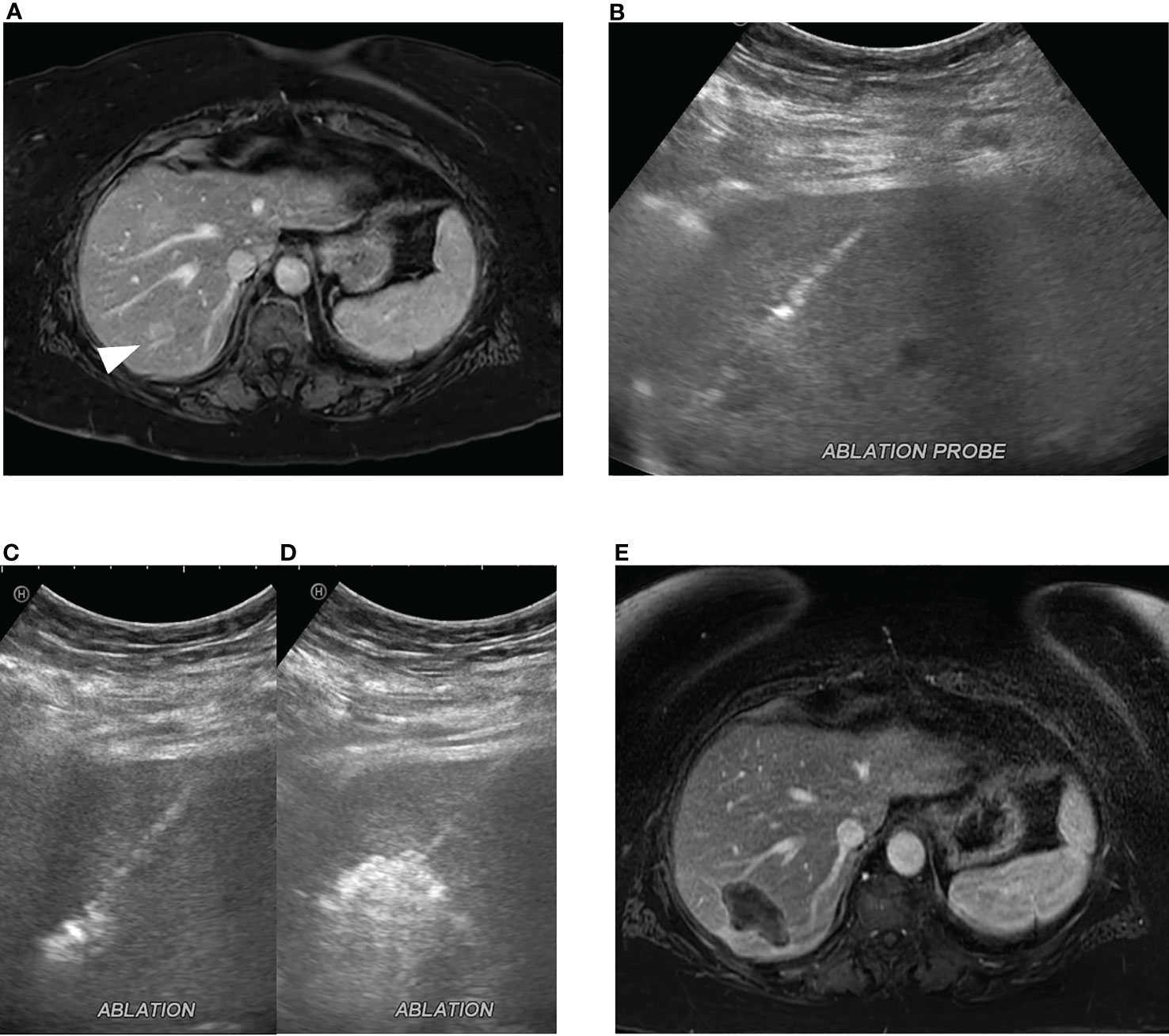
Figure 2 Microwave ablation of colorectal cancer liver metastasis. (A) A 2.0 cm colorectal cancer metastasis in segment 7 (white arrowhead). (B) Ultrasound-guided microwave ablation probe placement in the segment 7 lesion which was confirmed with CT (not shown). Continuous monitoring of ablation was performed with ultrasound with (C) early and (D) late ablation images obtained. (E) Post-ablation MRI, 1 month post procedure, demonstrates ablation zone without evidence of residual disease.
Laser ablation uses micrometer optical fiber to produce heat by transmitting infrared light. The optical fiber is connected to a generator or diode made of neodymium: yttrium aluminum garnet (ND: YAG), which emits a precise wavelength. The size of the fiber, the wavelength used, conduction and penetration of surrounding tissue, and the power and duration of the ablation are the factors that affect the size of the ablation zone (81). The lesions located within 1 cm of the main biliary duct, untreatable coagulopathy, and ascites interposed along the path of the applicator are considered contraindications to the thermal ablation (82). Patients may experience side effects after the procedure including pain, and post-ablation syndrome. Pain is self-limiting and depends on the size of the ablation zone. Post-ablation syndrome presents with flu-like illness with low-grade fever, nausea, vomiting and malaise, and can be managed symptomatically (72). Complications of the ablation procedure can be secondary to the injury to surrounding structures or the ablation itself, such as pneumothorax, intraperitoneal bleeding, hemothorax, portal vein thrombosis, gastrointestinal tract perforation, strictures, bile duct injury, cholecystitis, and liver abscess (72, 82).
The EORTC-CLOCC trial was a phase-II clinical trial that studied the efficacy of systemic chemotherapy with or without RFA in 119 patients diagnosed with unresectable CRLM (83). The trial randomized patients to receive systemic treatment alone or in combination with RFA. A significant improvement in OS and PFS was reported in the combined modality group rather than the systemic chemotherapy alone group (83). Improved OS in the combined modality group compared to the systemic treatment alone group (HR: 0.58; P=0.01) was observed. The 3-, 5- and 8-year OS rates were 56.9%, 43.1%, and 35.9% respectively in the combined modality group, and 55.2%, 30.3%, and 8.9% respectively in the systemic chemotherapy alone group. The median OS was 45.6 months in the combined modality group and 40.5 months in the systemic chemotherapy alone group. There was a prolonged PFS in the combined modality group (HR: 0.57; P=0.05). The liver as the first site of recurrence was noticed in 46.7% of the combined modality group and 78% of the systemic chemotherapy alone group (83). Another study, the ARF2003, included 52 unresectable CRLM treated with neoadjuvant chemotherapy and RFA. The study reported complete hepatic response in 75% of patients at their 3-month follow-up. The OS at 1-, and 5-years was 94% and 43% respectively, whereas PFS was 46% and 19% (84). Furthermore, another study reported that the combination of RFA and systemic chemotherapy has shown improved 3-year progression-free survival in comparison to systemic chemotherapy alone (27.6% vs. 10.6%; hazard ratio= 0.63; CI: 0.42-0.95; P=0.025) (85).
SBRT delivers the radiation to a specified region of interest with millimetric accuracy and reduces the irradiation to surrounding parenchyma. Unlike RFA and MWA, the SBRT is the better technique to access the perihilar, periampullary, or subcapsular lesions (86). SBRT can be considered, in combination with surgical resection, for oligometastatic liver disease that failed local therapies (87). Candidates with ≤ 5 CRLM involving <700 cc of the liver, an expected survival of > 3 months, curative extrahepatic disease, no chemotherapy received before two weeks of planned SBRT, and ≤ 2 Eastern Cooperative Oncology Group performance status are suitable for SBRT (87). A radiotherapy dose of ≥ 100-110 Gy can achieve local tumor control in 80-90% of the patients, while a higher dose may be required in case of larger tumors to attain similar outcomes (86–88). A study by Petrelli et al. included 656 patients and reported that the SBRT provides an overall survival of 67% and 57% and local tumor control of 67% and 59% at 1 and 2 years, respectively (89, 90). Compared to RFA, SBRT achieves greater 2-year local tumor control (84% vs. 60%); however, both the techniques had similar OS rates (91). The OLIVER trial compares the SBRT and chemotherapy alone and may provide further validations for its application (NCT03296839).
3.2 Radiation segmentectomy
Radiation segmentectomy (RS) delivers a very high ablative radiation dose (>190 Gray) confined to one or two liver segments, thus limiting the radiation-related complications (92, 93). The dose is based on the available literature for RS in patients with HCC which demonstrated a correlation between the level of tumor necrosis and the radiation exposure (93). The major intent of RS is to achieve cure in patients with CRLM, similar to the ablation or ablative external radiation therapy (94, 95). Diagnostic and therapeutic advancements through proper patient selection, imaging and radiation dosimetry allowed transition of lobar salvage to segmental curative radioembolization, especially in patients with features including (i) solitary tumor of size ≤ 5 cm (ii) primary or secondary liver tumor without other organ involvement and (iii) a tumor that can be targeted angiographically such that ≤ 2 liver segments receive the ablative dose of radiation (92, 96).
RECIST criteria have been widely employed to evaluate the response to TARE, however, PRECIST has proved to be more accurate in CRLM (97–99). Among all the parameters included in PRECIST, metabolic tumor volume and total lesional glycolysis are observed to be the significant predictors of OS (100). Recently, Choi criteria based on tumoral attenuation and diameter on CT imaging was identified to be a reliable criterion in CRLM to predict the PFS (101). Kurilova et al. observed that the RS of ≤ 3 hepatic segments can provide a 2-year tumor control rate of 83% in patients with limited therapeutic options and limited metastatic disease (Table 5) (102). They also reported that the tumor progression occurred in 21% of their study population which is similar to the study by Padia et al. (103) who reported tumor progression in 28% (102). In a study by Meiers et al, the authors included 10 patients of which 7 patients had inoperable CRLM confined to ≤ 2 liver segments (104). The procedure was unsuccessful in one among 7 patients due to attenuated hepatic vasculature. Of the remaining 6 patients with CRLM, four had a complete response or stable disease at their follow-up evaluation ranging from 1-14 months. Two of six patients had progressive disease after 7- and 18-months period. There were no reported adverse events. The mean PFS was 7.1 months for the entire cohort (92, 104).
Although RS has a promising role in the treatment of HCC that cannot be resected or ablated, the literature on CRLM is limited (93, 105–107). In addition, as the most of the CRLM patients may have been pre-treated with chemotherapeutic regimens, the hepatic vasculature can be altered limiting the ability to perform the super-selective RS. Furthermore, the hypovascular nature of CRLM results in difficulty targeting the tumor. Based on the available data, RS appears to provide local tumor control with acceptable toxicity in patients with CRLM. Further studies on patient selection and tumor response are required to emphasize the application of RS in patients with CRLM.
3.3 Firstline chemotherapy plus TARE
Combined therapy with radioembolization and systemic chemotherapy has been studied in the literature. Haber et al. reported 38-month and 25-month median survival of CRLM patients treated with combined systemic chemotherapy plus TARE and systemic chemotherapy alone groups, respectively from the date of primary diagnosis (108). Three phase-III clinical trials, SIRFLOX, FOXFIRE and FOXFIRE-Global, studied the efficacy of combined chemotherapy with Y90 TARE over chemotherapy alone among 1103 patients in total (109–111). SIRFLOX trial by Van Hazel et al. concluded that the addition of TARE to the chemotherapy did not improve the PFS, however delayed the tumor progression significantly (Table 6) (110). A combined analysis of FOXFIRE, SIRFLOX and FOXFIRE-Global was performed by Wasan et al. with a total of 1103 patients (113). The patients were randomized to receive FOLFOX alone (549) or in combination with single cycle of TARE (554). Higher overall response rate was reported in the combined group (72% vs. 63%) however no differences were identified in median OS (22.6 months vs. 23.3 months; P=0.61). Radiological progression of the tumor was observed in 49% of FOLFOX alone group and 31% of the combined group. The cumulative incidence of tumor progression in the first 12 months follow up period was 22% in the combined group compared to 39% in FOLFOX alone group. An objective response rate was reported in 72% of the combined group and 63% of FOLFOX alone group (P= 0.0012). The study also reported high odds of grade 3 or worse adverse events in the combined group (74%) than the FOLFOX alone group (67%) (OR: 1.42; P= 0.008) (113). Wasan et al. reported 17% resectablity rate in TARE + chemotherapy group and 16% in chemotherapy alone group (P=0.67) (113). Garlipp et al. reported an improved resectability rate of the lesions after TARE+ chemotherapy compared to chemotherapy alone (38.1% vs. 28.9%; P<0.001) (115). The subgroup analyses of the FOXFIRE, SIRFLOX and FOXFIRE-Global trials reported no significant difference in OS between the combined and FOLFOX alone group (112, 114). However, when tumors are stratified based on location, the addition of SIRT improved the OS in right-sided but not left-sided primary CRC (Table 6) (112, 114).
4 Therapies with palliative intent
4.1 TACE
Approximately 80% of blood supply to CRLM is derived from the hepatic artery while it is from the portal vein to the normal liver parenchyma (42, 116). Transarterial therapies utilize the advantage of dual blood supply of the liver and hence the cytotoxic agents infused through the hepatic artery selectively target tumor over normal cells. In addition, the first pass metabolism of the chemotherapeutic agents can be bypassed in the intra-arterial therapies. TACE is a catheter-based infusion of one or more chemotherapeutic medications and embolizing material into the hepatic artery. Embolizing material can be either temporary or permanent. The former includes collagen, gelatin sponge and degradable starch microspheres, while the latter include polyvinyl alcohol particles. Lipiodol has both the vaso-occlusive effect and the ability to enhance the effect of cytotoxic agents (117). TACE procedure was first introduced by Yamada et al. in late 1970s (118). In general, TACE is indicated as a second-line modality of treatment in patients who are refractory to systemic chemotherapy or in inoperable CRLM (119). Conventional TACE (cTACE) represents the injection of lipiodol + chemotherapy and embolizing agents. Recently, the drug-eluting beads are being used as embolic materials termed as DEB-TACE. The efficacy of cTACE and DEB-TACE have been extensively studied in the management of CRLM.
4.1.1 Conventional TACE
The chemotherapeutic regimen and embolic materials are variable in the published studies. Albert et al. studied the efficacy of TACE with doxorubicin, cisplatin, mitomycin C and lipiodol mixture followed by embolization material- polyvinyl alcohol particles, in 245 unresectable CRLM in 121 patients who were refractory to systemic chemotherapy (120). Median survival from initial CRLM diagnosis and TACE was 27 months and 9 months, respectively. The study described that the OS was better with TACE after first- or second-line systemic chemotherapy than after three to five lines of systemic chemotherapy (11-12 months vs. 6 months; P= 0.03) (120). Vogl et al. studied 463 patients with unresectable CRLM (117). Patients were divided into three groups with each receiving mitomycin C alone, mitomycin C plus gemcitabine, or mitomycin C plus irinotecan and followed by embolization with starch microspheres. The authors reported that 1-year and 2-year survival rates were 62% and 28% respectively with no significant difference among the patient groups (117). A study by Gruber-Rouh et al. involved 564 patients who were infused with mitomycin C, gemcitabine, irinotecan or cisplatin depending on the prior systemic chemotherapy regimen (Table 7) (123). For instance, patients treated with systemic FOLFOX or FOLFIRI were treated with mitomycin alone. Embolization was performed with iodized oil and starch microspheres. The study reported survival of 14.3 months from the start of first cTACE (123).
Vogl et al. studied on patients treated with cTACE as a palliative or a neoadjuvant option (Table 7) (122). The cTACE was followed by ablation in the neoadjuvant group. All the patients were refractory to prior systemic chemotherapy. Vogl et al. reported significant improvement in OS and PFS in palliative (12.6 and 5.9 months, respectively) and neoadjuvant (25.8 and 10.8 months, respectively) groups (122). The presence of extrahepatic metastases was described as the significant factor for OS and PFS in both palliative and neoadjuvant groups (122). Vogl et al. concluded that cTACE was effective in unresectable advanced CRLM and further improves survival, if followed by ablation (122). Nishiofuku et al. studied the efficacy of TACE with cisplatin powder and degradable starch microspheres (DSM) and a reported tumor response rate in 61.1% of patients (124). They also reported the median OS, PFS, and hepatic-PFS as 21.1 months, 5.8 months, and 8.8 months (124). However, majority of patients became eligible for surgical resection post-TACE, which might overestimate the OS benefit of TACE. The authors studied the tumor response rate in wild-type and mutated KRAS tumors to be around 75% and 66.7%, respectively (124). The study concluded that cisplatin, at a dose of 80 mg/m2 with the DSM, can provide a high tumor response rate and prolonged survival time for patients with unresectable CRLM refractory to FOLFOX systemic chemotherapy (124). Short embolization effect and good tumor response are the two main advantages of DSM-TACE over conventional TACE (127). In summary, all the described studies demonstrate that cTACE is a feasible treatment modality in patients who are unresponsive to conventional therapy.
The TACE in combination with RFA is studied to improve the survival and outcomes in single HCC lesion >5 cm and multiple HCC lesions >3 cm (128). The same has also been applied in CRLM by Faiella et al., who discovered a positive impact on the patient survival (129). However, the data is limited as the protocol for TACE is quite different from RFA. Regular TACE protocol is for widespread CRLM, while targeted TACE, along with RFA, can be used for focal metastases (128).
4.1.2 DEBIRI-TACE
A current area of research involves the use of irinotecan drug-eluting beads (DEBIRI-TACE) to treat CRLM. The initial results of a Phase II clinical trial comprising 20 patients reported an 80% response rate with reduction of contrast enhancement of treated tumors following treatment with irinotecan drug-eluting beads [37]. Similarly, Aliberti et al. reported 78% tumor response rate at three months in a phase II study comprising 82 patients (130). All the patients had at least two failed systemic chemotherapy lines. The study also described the OS and PFS as 25 months and 8 months respectively (130). Martin et al. studied the efficacy of DEBIRI in patients refractory to oxaliplatin- and irinotecan-based systemic chemotherapy. The study concluded that DEBIRI was safe with minimal complications and 75% tumor response rate (131). This promising treatment for patients with colorectal metastases merits further study both as a salvage agent and potentially in combination with systemic chemotherapy. Fiorentini et al. compared the efficacy of FOLFIRI and DEBIRI-TACE (132). Median OS was longer for DEBIRI-TACE group (22 vs. 15 months). In addition, DEBIRI-TACE group had better quality of life (8 vs. 3 months) and objective tumor response (69% vs. 20%) (132). However, the study was limited by the omission of bevacizumab, oxaliplatin, panitumumab or cetuximab in the standard care of treatment (132). Martin et al. overcame this limitation by comparing DEBIRI plus systemic FOLFOX and bevacizumab with systemic FOLFOX plus bevacizumab alone (133). The study observed a significantly greater response rate in DEBIRI-FOLFOX arm compared to FOLFOX/bevacizumab arm at the end of 2 months (78% vs. 54%) and 6 months (76% vs. 60%) (133). Th significant tumor downsizing was observed in DEBIRI-FOLFOX arm than the comparison arm (35% vs. 16%) (133). The median PFS of 15.3 months was reported in DEBIRI-FOLFOX arm and 7.6 months in FOLFOX/bevacizumab arm (133). Nonetheless, the study by Martin et al. did not demonstrate improvement in OS compared to cTACE studies that excluded systemic chemotherapy (Table 8) (133). Recently, a systematic review by Akinwande et al. included 13 studies comprising a total of 850 patients (135). The weighted average PFS and OS were 8.1 months and 16.8 months respectively (135).
The most common complications following TACE procedure include post-embolization syndrome (PES) (15-90%), cholecystitis, and hepatic insufficiency (134, 136). Complications such as segmental biliary dilatation, thrombocytopenia, leukopenia, hepatic artery thrombosis, embolus migration are less common (134). The etiology of PES is not entirely determined but several theories have been proposed including hepatic capsular distention, tumor necrosis, hepatic ischemia, anti-inflammatory response to chemotherapeutic medications and gallbladder infarction (136, 137). Paye et al. studied that the PES following TACE is due to injury to the non-tumoral hepatic cells (138). Risk factors for the adverse effects include complete flow stasis during embolization, lack of pre-treatment with lidocaine, infusion of > 100 mg of DEBIRI, bilirubin > 2 ug/dl, with > 50% liver involvement, and achievement of complete stasis (131). Hence, patients with extrahepatic metastases, tumor burden of >70% liver parenchyma, increased bilirubin levels (> 3mg/dl), renal dysfunction (serum creatinine, > 2 mg/dl), and complete portal venous thrombosis are usually excluded from TACE (123).
DEB-TACE has certain limitations including (i) inability to identify the beads in real-time which in turn prevents the visualization of intraoperative precise delivery and post-operative effects (ii) as the DEBs load only positively charged chemotherapeutic medications, the options of drugs are restricted (139). Hence, new drug carriers are being studied to overcome the limitations. Iodine-containing and superparamagnetic iron oxide- containing microspheres are studied to visualize on the X-ray and MR imaging respectively.
4.2 TARE
Guidelines support TARE as a treatment option in patients with CRLM who are refractory to ≥ 2 lines of systemic chemotherapy (Figure 3) (category 2A and Grade B recommendation as per European Society for Medical Oncology and National Comprehensive Cancer Network, respectively) (57, 140, 141). The application of TARE as a second-line therapy in unresectable CRLM refractory to first-line systemic chemotherapy require endorsement from further studies. Ideal candidates for Y90-TARE shall be ≥ 18 years old, Eastern Cooperative Oncology group (ECOG) score ≤ 2, serum bilirubin < 3 mg/dl, serum creatinine < 2 mg/dl, and with adequate lung function (140). Mulcahy et al. reported tumor response rate of 40.3% in unresectable CRLM when exposed to a median dose of 118Gy (Table 9) (148). The MORE study included 606 patients with CRLM who had two lines of prior systemic chemotherapy. The study reported OS of 9.6 months (144). Hickey et al. reported OS of 10.6 months in their study which involved 531 patients who were refractory to prior systemic chemotherapy or locoregional therapies (143). Absence of extrahepatic metastases, <25% tumor burden, albumin > 3 g/dl, good performance status and receipt of < 2 chemotherapeutic medications are the independent predictors of survival (143). In a prospective study by Helmberger et al. involving 1027 patients who underwent Y90-TARE for primary or metastatic hepatic tumors, the authors reported the OS of 9.8 months in CRLM (150). Wu et al. compared the survival outcomes with Y90-TARE in right versus left sided primary tumor location. They observed that patients with right sided primary tumors had decreased OS compared to left sided primary tumors (5.4 vs. 6.2 months; P=0.03) (151). However, no significant difference in hepatic PFS, tumor response and disease progression were observed (151). Lahti et al. studied the KRAS status as the prognostic factor in unresectable CRLM who underwent Y-90 TARE. They reported that median OS was greater in patients with KRAS wild-type genes than mutant genes (9.5 months vs. 4.8 months; P= 0.04) (152). The KRAS status, carcinoembryonic antigen levels, and Child-Pugh class were found to be the prognostic factors for OS (152). Narsinh et al. described the importance of hepatopulmonary shunting as a prognostic indicator of survival in their study of 606 patients who underwent Y90-TARE for CRLM. They reported that increased liver shunt fraction (LSF) indicated worse prognosis in CRLM. The LSF > 10% was associated with reduced survival rate compared to LSF < 10% (6.9 months vs. 10 months; HR: 1.60; P<0.001) (153).
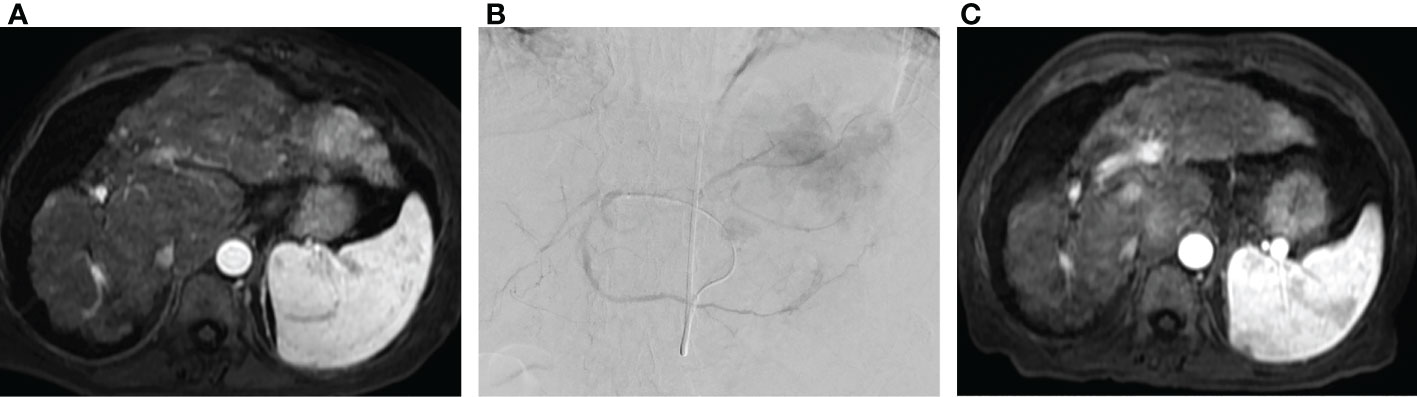
Figure 3 Radioembolization as salvage therapy. (A) Pre-procedure MRI in patient with metastatic colorectal cancer to the liver following three lines of chemotherapy demonstrating multifocal metastatic disease involving the left hepatic lobe. (B) Transradial radioembolization of the left hepatic lobe with Yttrium-90 resin microspheres via a replaced left hepatic artery arising from a left gastric artery. (C) Post procedure MRI with interval reduction in size and enhancement of left hepatic lobe tumor.
Dendy et al. studied the survival predictive biomarkers in patients who underwent Y90-TARE for CRLM (140). They described that low tumor burden, sufficient calculated Y90 dose, increased albumin, and low ECOG score are the pre-interventional biomarkers which indicate favorable outcome (140). Likewise, after the procedure, decreased tumor burden, reduced tumor glycolysis, radiological tumor response and reduced expression of surviving, p53, Bcl-2 are indicative of favorable outcome (140). Irrespective of timing of biomarker evaluation, the increased HMGB1(High mobility group box 1), nucleosome expression, increased carcinoembryonic antigen, CA 19-9, CYFRA 21-1 (Cytokeratin 19 fragment), lactate dehydrogenase, aspartate transaminase, choline esterase, gamma glutamyl transferase, alkaline phosphatase, amylase are the indicators of unfavorable response (140). Usually, Y90 radioembolization is safe with minor complications and post-embolization syndrome. Gastric ulceration (<5%), portal hypertension (<1%), radiation induced liver fibrosis (<4%), pancreatitis (<1%), biloma (<1%), cholecystitis (<1%), abscess formation (<1%), and radiation induced pneumonitis (<1%) are the few of reported complications secondary to radioembolization (154). The post-embolization syndrome can be observed in 50% of the patients within 2 weeks of the procedure. In contrast to post-embolic syndrome, it rarely requires patient hospitalization.
5 Other hepatic metastases
Very few studies have been performed on the interventional management of non-CRLM. In liver metastases secondary to gastric tumors, RFA is proven beneficial only in cases of single metastases limited to a single lobe and without extrahepatic disease (155). Combined systemic chemotherapy is also recommended in addition to RFA to prolong the OS (155). RFA in liver metastases secondary to breast cancer has also been studied to improve OS; however, the extrahepatic metastases (P=0.013) and age >60 years (P=0.025) are considered worse prognostic factors for OS (156). MWA has equal benefits to RFA and can be an alternate therapy in patients with liver metastases originating from ovarian, pancreatic, esophageal, and neuroendocrine neoplasms (157). Further broad studies are required for more data on patient outcomes and efficacy. Arterial interventions such as TACE with raltitrexed eluting beads are studied to be safe and efficient in hepatic metastases due to gastric adenocarcinoma (158). In contrast to CRLM, the focus of arterial interventions in neuroendocrine liver metastases (NELM) is on the controlling the endocrine secretions (159). NELM are hypervascular tumors, and the studies show that the embolization alone has good efficacy on patient outcomes, unlike colorectal metastases, requiring chemotherapeutic embolization (160, 161). Elf et al. demonstrated that the NELM has optimal response rates to embolization therapies compared to SIRT (162). Other than CRLM and NELM, the literature is limited to other hepatic metastases. Saxena et al. studied that SIRT in chemoresistant hepatic metastases due to breast cancer has improved 24-month survival rates to 39% (163). Despite this, prospective trials on optimal patient selection and survival data are necessary for further validation.
6 Future directions
The combination of immunotherapy and targeted ablation is a new revolutionizing concept based on enhanced exposure of the tumor antigen. Ablated and dead tumor cells release tumor antigens into the bloodstream which augments the T-cell response, enhancing the efficacy of immunotherapy (164). Both the pro-inflammatory cytokines such as IL-6, and the anti-inflammatory cytokines, such as IL-10, get elevated after the ablation procedure. So far, cryoablation has been proven to induce a higher (4.6 fold) IL-10 release compared to heat-based techniques such as RFA (1,7 fold) and MWA (1.2 fold) (165, 166). Shi et al. reported that the PD-L1-PD-1 axis inhibits the T-cell response; hence monoclonal antibodies against the PD-1 are used to increase the feasibility of an anti-tumor immune response (167). The stronger T-cell response, robust anti-tumor immunity, and improved survival rates were observed in mice after combining anti-PD1 monoclonal antibodies with an ablation procedure (167). Likely, the TACE procedure triggers tissue hypoxia and the release of vascular endothelial growth factor, which could be used as the target for bevacizumab and tyrosine kinase inhibitors. Tumor-associated macrophages (TAMs) are responsible for tumor nurture and metastasization by inducing the epithelial-to-mesenchymal transition (EMT) and vascular disruption. Current studies are targeting TGF-beta signaling pathway, which is responsible for the EMT. The collagen triple helix repeat containing 1 (CTHRC1) is secreted by the colorectal cancer cells, stabilizing the TGF-beta signaling and activation. Studies show that the monoclonal antibodies against CTHRC1 combined with PD-1/PD-L1 blockade have led to the shrinkage of CRLM (168). Similarly, strategies targeting the TAMs reprogramming, depletion, and inhibition were studied (169). However, stronger validations are not yet provided due to the heterogenous behavior of the TAMs.
7 Conclusion
Tremendous evolution has occurred over the last two decades in the locoregional interventional therapies for CRLM. Surgical resection is the curative treatment for patients with CRLM. In case of unresectable tumors or non-surgical candidates, evaluation for ablation is recommended. Transarterial therapies are indicated as a salvage therapy and Y90-TARE is the FDA approved therapy for CRLM. DEBIRI-TACE or cTACE is considered in patients with progressive liver disease after Y90-TARE.
Author contributions
SV, PS, SK, NO and SPK have contributed equally to this work. All authors contributed to the article and approved the submitted version.
Conflict of interest
SPK reports grants from NIH, BD, Black Swan, and Trisalus for Institution; reports royalties from Elsevier, Springer, and Thieme for himself; reports consulting fees from Penumbra, Okami Medical, Boston Scientific, Medtronic, Covidien, US Vascular, Dova Pharmaceuticals, Instylla, and BD for himself; reports payment from Stony Brook University, American Institute of Biology, UT Houston, and NACCME for himself; reports payment for expert testimony from Southern Institute for Medical and Legal Affairs LLC for himself; reports participation from NIH for institution; reports leadership from Chief, Interventional Radiology, Massachusetts General Hospital, Boston, MA; Chair, Vascular Panel, ACR Appropriateness Criteria; International Editor, Journal of Clinical Interventional Radiology ISVIR; Assistant Editor, Radiology – Cardiothoracic, RSNA; reports stock from Biogen Inc, Clover Health Investments Corp, Inovio Pharmaceuticals, Moderna Inc, Pfizer Inc, Novavax Inc, Orphazyme, Cassava Sciences Inc, Vivos Therapeutics Inc,Ardelyx Inc, Althea Health, Sarepta Therapeutics, Clover Health Investments Corp, CureVac BV, Immunoprecise Antibodies Ltd, Infinity Pharmaceuticals Inc, Zymergen Inc, BioNTech SE, Trillium Therapeutics Inc, Theravance Biopharma Inc, Doximity Inc, Eargo Inc, Allogent Therapeutics Inc, NRx Pharmaceuticals Inc, Atea pharmaceuticals Inc, for himself and spouse; and reports financial or nonfinancial interests as Adjunct Associate Professor from University of Texas Southwestern Medical Center and Professor of Radiology at Harvard medical school.
The remaining authors declare that the research was conducted in the absence of any commercial or financial relationships that could be construed as a potential conflict of interest.
Publisher’s note
All claims expressed in this article are solely those of the authors and do not necessarily represent those of their affiliated organizations, or those of the publisher, the editors and the reviewers. Any product that may be evaluated in this article, or claim that may be made by its manufacturer, is not guaranteed or endorsed by the publisher.
References
1. Piawah S, Venook AP. Targeted therapy for colorectal cancer metastases: a review of current methods of molecularly targeted therapy and the use of tumor biomarkers in the treatment of metastatic colorectal cancer. Cancer (2019) 125(23):4139–47. doi: 10.1002/cncr.32163
2. Zhou H, Liu Z, Wang Y, Wen X, Amador EH, Yuan L, et al. Colorectal liver metastasis: molecular mechanism and interventional therapy. Signal Transduct Target Ther (2022) 7(1):70. doi: 10.1038/s41392-022-00922-2
3. Dekker E, Tanis PJ, Vleugels JLA, Kasi PM, Wallace MB. Colorectal cancer. Lancet (2019) 394(10207):1467–80. doi: 10.1016/S0140-6736(19)32319-0
4. Riihimäki M, Hemminki A, Sundquist J, Hemminki K. Patterns of metastasis in colon and rectal cancer. Sci Rep (2016) 6(1):1–9. doi: 10.1038/srep29765
5. Van Cutsem E, Oliveira J. Advanced colorectal cancer: ESMO clinical recommendations for diagnosis, treatment and follow-up. Ann Oncol (2009) 20:iv61–iv3. doi: 10.1093/annonc/mdp130
6. Siegel RL, Miller KD, Fedewa SA, Ahnen DJ, Meester RGS, Barzi A, et al. Colorectal cancer statistics, 2017. CA Cancer J Clin (2017) 67(3):177–93. doi: 10.3322/caac.21395
7. Vatandoust S, Price TJ, Karapetis CS. Colorectal cancer: metastases to a single organ. World J Gastroenterol (2015) 21(41):11767. doi: 10.3748/wjg.v21.i41.11767
8. Osterlund P, Salminen T, Soveri LM, Kallio R, Kellokumpu I, Lamminmäki A, et al. Repeated centralized multidisciplinary team assessment of resectability, clinical behavior, and outcomes in 1086 Finnish metastatic colorectal cancer patients (RAXO): a nationwide prospective intervention study. Lancet Reg Health Eur (2021) 3:100049. doi: 10.1016/j.lanepe.2021.100049
9. Leporrier J, Maurel J, Chiche L, Bara S, Segol P, Launoy G. A population-based study of the incidence, management and prognosis of hepatic metastases from colorectal cancer. J Br Surg (2006) 93(4):465–74. doi: 10.1002/bjs.5278
10. Van Tilborg A, Meijerink M, Sietses C, Van Waesberghe J, Mackintosh M, Meijer S, et al. Long-term results of radiofrequency ablation for unresectable colorectal liver metastases: a potentially curative intervention. Br J Radiol (2011) 84(1002):556–65. doi: 10.1259/bjr/78268814
11. Al-Sharif E, Simoneau E, Hassanain M. Portal vein embolization effect on colorectal cancer liver metastasis progression: lessons learned. World J Clin Oncol (2015) 6(5):142–6. doi: 10.5306/wjco.v6.i5.142
12. Andreou A, Aloia TA, Brouquet A, Vauthey J-N. Recent advances in the curative treatment of colorectal liver metastases. Gastrointest Cancer Res: GCR (2011) 4(4 Suppl 1):S2.
13. Fernandez FG, Drebin JA, Linehan DC, Dehdashti F, Siegel BA, Strasberg SM. Five-year survival after resection of hepatic metastases from colorectal cancer in patients screened by positron emission tomography with f-18 fluorodeoxyglucose (FDG-PET). Ann Surg (2004) 240(3):438. doi: 10.1097/01.sla.0000138076.72547.b1
14. Abdalla EK, Vauthey J-N, Ellis LM, Ellis V, Pollock R, Broglio KR, et al. Recurrence and outcomes following hepatic resection, radiofrequency ablation, and combined resection/ablation for colorectal liver metastases. Ann Surg (2004) 239(6):818. doi: 10.1097/01.sla.0000128305.90650.71
15. Wei AC, Greig PD, Grant D, Taylor B, Langer B, Gallinger S. Survival after hepatic resection for colorectal metastases: a 10-year experience. Ann Surg Oncol (2006) 13(5):668–76. doi: 10.1245/ASO.2006.05.039
16. Adam R, Avisar E, Ariche A, Giachetti S, Azoulay D, Castaing D, et al. Five-year survival following hepatic resection after neoadjuvant therapy for nonresectable colorectal [liver] metastases. Ann Surg Oncol (2001) 8(4):347–53. doi: 10.1007/s10434-001-0347-3
17. Deschamps F, Ronot M, Gelli M, Durand-Labrunie J, Tazdait M, Hollebecque A, et al. Interventional radiology for colorectal liver metastases. Curr Colorectal Cancer Rep (2020) 16(2):29–37. doi: 10.1007/s11888-020-00449-0
18. Raoux L, Maulat C, Mokrane F-Z, Fares N, Suc B, Muscari F. Impact of the strategy for curative treatment of synchronous colorectal cancer liver metastases. J Visceral Surg (2020) 157(4):289–99. doi: 10.1016/j.jviscsurg.2019.10.007
19. Xing M, Kooby DA, El-Rayes BF, Kokabi N, Camacho JC, Kim HS. Locoregional therapies for metastatic colorectal carcinoma to the liver–an evidence-based review. J Surg Oncol (2014) 110(2):182–96. doi: 10.1002/jso.23619
20. Fairchild AH, White SB. Decision making in interventional oncology: intra-arterial therapies for metastatic colorectal cancer-Y90 and chemoembolization. Semin Intervent Radiol (2017) 34(2):87–91. doi: 10.1055/s-0037-1601854
21. Mahnken AH, Pereira PL, de Baère T. Interventional oncologic approaches to liver metastases. Radiology (2013) 266(2):407–30. doi: 10.1148/radiol.12112544
22. Makuuchi M, Thai BL, Takayasu K, Takayama T, Kosuge T, Gunvén P, et al. Preoperative portal embolization to increase safety of major hepatectomy for hilar bile duct carcinoma: a preliminary report. Surgery (1990) 107(5):521–7.
23. de Graaf W, van den Esschert JW, van Lienden KP, van Gulik TM. Induction of tumor growth after preoperative portal vein embolization: is it a real problem? Ann Surg Oncol (2009) 16(2):423–30. doi: 10.1245/s10434-008-0222-6
24. Mueller L, Hillert C, Möller L, Krupski-Berdien G, Rogiers X, Broering DC. Major hepatectomy for colorectal metastases: is preoperative portal occlusion an oncological risk factor? Ann Surg Oncol (2008) 15(7):1908–17. doi: 10.1245/s10434-008-9925-y
25. Shindoh J, Tzeng CW, Aloia TA, Curley SA, Zimmitti G, Wei SH, et al. Optimal future liver remnant in patients treated with extensive preoperative chemotherapy for colorectal liver metastases. Ann Surg Oncol (2013) 20(8):2493–500. doi: 10.1245/s10434-012-2864-7
26. Ribero D, Abdalla EK, Madoff DC, Donadon M, Loyer EM, Vauthey JN. Portal vein embolization before major hepatectomy and its effects on regeneration, resectability and outcome. Br J Surg (2007) 94(11):1386–94. doi: 10.1002/bjs.5836
27. Abdalla EK, Barnett CC, Doherty D, Curley SA, Vauthey JN. Extended hepatectomy in patients with hepatobiliary malignancies with and without preoperative portal vein embolization. Arch Surg (2002) 137(6):675–80. doi: 10.1001/archsurg.137.6.675
28. Wen XD, Xiao L. Associating liver partition and portal vein ligation for staged hepatectomy in the treatment of colorectal cancer liver metastases. World J Gastrointest Surg (2021) 13(8):814–21. doi: 10.4240/wjgs.v13.i8.814
29. Pamecha V, Levene A, Grillo F, Woodward N, Dhillon A, Davidson BR. Effect of portal vein embolisation on the growth rate of colorectal liver metastases. Br J Cancer (2009) 100(4):617–22. doi: 10.1038/sj.bjc.6604872
30. Collin Y, Paré A, Belblidia A, Létourneau R, Plasse M, Dagenais M, et al. Portal vein embolization does not affect the long-term survival and risk of cancer recurrence among colorectal liver metastases patients: a prospective cohort study. Int J Surg (2019) 61:42–7. doi: 10.1016/j.ijsu.2018.11.029
31. Coco D, Leanza S. Associating liver partition and portal vein ligation for staged hepatectomy (ALPPS) in colorectal liver metastases: review of the literature. Clin Exp Hepatol (2021) 7(2):125–33. doi: 10.5114/ceh.2021.106521
32. Dueland S, Yaqub S, Syversveen T, Carling U, Hagness M, Brudvik KW, et al. Survival outcomes after portal vein embolization and liver resection compared with liver transplant for patients with extensive colorectal cancer liver metastases. JAMA Surg (2021) 156(6):550–7. doi: 10.1001/jamasurg.2021.0267
33. Huiskens J, Olthof PB, van der Stok EP, Bais T, van Lienden KP, Moelker A, et al. Does portal vein embolization prior to liver resection influence the oncological outcomes - a propensity score matched comparison. Eur J Surg Oncol (2018) 44(1):108–14. doi: 10.1016/j.ejso.2017.09.017
34. Ironside N, Bell R, Bartlett A, McCall J, Powell J, Pandanaboyana S. Systematic review of perioperative and survival outcomes of liver resections with and without preoperative portal vein embolization for colorectal metastases. HPB (Oxford) (2017) 19(7):559–66. doi: 10.1016/j.hpb.2017.03.003
35. Giglio MC, Giakoustidis A, Draz A, Jawad ZAR, Pai M, Habib NA, et al. Oncological outcomes of major liver resection following portal vein embolization: a systematic review and meta-analysis. Ann Surg Oncol (2016) 23(11):3709–17. doi: 10.1245/s10434-016-5264-6
36. Hoekstra LT, van Lienden KP, Doets A, Busch OR, Gouma DJ, van Gulik TM. Tumor progression after preoperative portal vein embolization. Ann Surg (2012) 256(5):812–7. doi: 10.1097/SLA.0b013e3182733f09
37. Simoneau E, Aljiffry M, Salman A, Abualhassan N, Cabrera T, Valenti D, et al. Portal vein embolization stimulates tumour growth in patients with colorectal cancer liver metastases. HPB (Oxford) (2012) 14(7):461–8. doi: 10.1111/j.1477-2574.2012.00476.x
38. Pamecha V, Glantzounis G, Davies N, Fusai G, Sharma D, Davidson B. Long-term survival and disease recurrence following portal vein embolisation prior to major hepatectomy for colorectal metastases. Ann Surg Oncol (2009) 16(5):1202–7. doi: 10.1245/s10434-008-0269-4
39. Kokudo N, Tada K, Seki M, Ohta H, Azekura K, Ueno M, et al. Proliferative activity of intrahepatic colorectal metastases after preoperative hemihepatic portal vein embolization. Hepatology (2001) 34(2):267–72. doi: 10.1053/jhep.2001.26513
40. Geschwind JFH, Salem R, Carr BI, Soulen MC, Thurston KG, Goin KA, et al. Yttrium-90 microspheres for the treatment of hepatocellular carcinoma. Gastroenterology (2004) 127(5):S194–205. doi: 10.1053/j.gastro.2004.09.034
41. Dawson LA, Normolle D, Balter JM, McGinn CJ, Lawrence TS, Ten Haken RK. Analysis of radiation-induced liver disease using the Lyman NTCP model. Int J Radiat Oncol Biol Physics (2002) 53(4):810–21. doi: 10.1016/S0360-3016(02)02846-8
42. Raval M, Bande D, Pillai AK, Blaszkowsky LS, Ganguli S, Beg MS, et al. Yttrium-90 radioembolization of hepatic metastases from colorectal cancer. Front Oncol (2014) 4:120. doi: 10.3389/fonc.2014.00120
43. Kalva SP, Thabet A, Wicky S. Recent advances in transarterial therapy of primary and secondary liver malignancies. Radiographics (2008) 28(1):101–17. doi: 10.1148/rg.281075115
44. Chiu AM, Savoor R, Gordon AC, Riaz A, Sato KT, Hohlastos E, et al. Yttrium-90 radiation segmentectomy in oligometastatic secondary hepatic malignancies. J Vasc Interv Radiol (2023) 34(3):362–8. doi: 10.1016/j.jvir.2022.12.021
45. Nebelung H, Wolf T, Bund S, Radosa CG, Plodeck V, Grosche-Schlee S, et al. Radioembolization versus portal vein embolization for contralateral liver lobe hypertrophy: effect of cirrhosis. Abdom Radiol (NY) (2021) 46(8):4046–55. doi: 10.1007/s00261-021-03048-1
46. Vouche M, Lewandowski RJ, Atassi R, Memon K, Gates VL, Ryu RK, et al. Radiation lobectomy: time-dependent analysis of future liver remnant volume in unresectable liver cancer as a bridge to resection. J Hepatol (2013) 59(5):1029–36. doi: 10.1016/j.jhep.2013.06.015
47. Teo JY, Allen JC Jr., Ng DC, Choo SP, Tai DW, Chang JP, et al. A systematic review of contralateral liver lobe hypertrophy after unilobar selective internal radiation therapy with Y90. HPB (Oxford) (2016) 18(1):7–12. doi: 10.1016/j.hpb.2015.07.002
48. Garlipp B, de Baere T, Damm R, Irmscher R, van Buskirk M, Stübs P, et al. Left-liver hypertrophy after therapeutic right-liver radioembolization is substantial but less than after portal vein embolization. Hepatology (2014) 59(5):1864–73. doi: 10.1002/hep.26947
49. Edeline J, Lenoir L, Boudjema K, Rolland Y, Boulic A, Le Du F, et al. Volumetric changes after (90)y radioembolization for hepatocellular carcinoma in cirrhosis: an option to portal vein embolization in a preoperative setting? Ann Surg Oncol (2013) 20(8):2518–25. doi: 10.1245/s10434-013-2906-9
50. Kurilova I, Pompa V, Guerrero R, Tapias MA, Calatayud MD, Fondevila C, et al. (90)Y-radioembolization after failed portal vein embolization for colorectal liver metastases: a case report. Cardiovasc Intervent Radiol (2020) 43(8):1232–6. doi: 10.1007/s00270-020-02537-y
51. Liebl M, Pedersoli F, Zimmermann M, Schulze-Hagen M, Truhn D, Sieben P, et al. Induction of contralateral hepatic hypertrophy by unilobar yttrium-90 transarterial radioembolization versus portal vein embolization: an animal study. J Vasc Interv Radiol (2021) 32(6):836–42.e2. doi: 10.1016/j.jvir.2021.01.281
52. Fernández-Ros N, Silva N, Bilbao JI, Iñarrairaegui M, Benito A, D'Avola D, et al. Partial liver volume radioembolization induces hypertrophy in the spared hemiliver and no major signs of portal hypertension. HPB (Oxford) (2014) 16(3):243–9. doi: 10.1111/hpb.12095
53. Mima K, Beppu T, Chikamoto A, Miyamoto Y, Nakagawa S, Kuroki H, et al. Hepatic resection combined with radiofrequency ablation for initially unresectable colorectal liver metastases after effective chemotherapy is a safe procedure with a low incidence of local recurrence. Int J Clin Oncol (2013) 18(5):847–55. doi: 10.1007/s10147-012-0471-z
54. Sasaki K, Margonis GA, Andreatos N, Kim Y, Wilson A, Gani F, et al. Combined resection and RFA in colorectal liver metastases: stratification of long-term outcomes. J Surg Res (2016) 206(1):182–9. doi: 10.1016/j.jss.2016.06.098
55. Petre EN, Sofocleous CT, Solomon SB. Ablative and catheter-directed therapies for colorectal liver and lung metastases. Hematol Oncol Clin North Am (2015) 29(1):117–33. doi: 10.1016/j.hoc.2014.09.007
56. Puijk RS, Dijkstra M, van den Bemd BAT, Ruarus AH, Nieuwenhuizen S, Geboers B, et al. Improved outcomes of thermal ablation for colorectal liver metastases: a 10-year analysis from the prospective Amsterdam CORE registry (AmCORE). Cardiovasc Intervent Radiol (2022) 45:1074–89. doi: 10.1007/s00270-022-03152-9
57. Tsitskari M, Filippiadis D, Kostantos C, Palialexis K, Zavridis P, Kelekis N, et al. The role of interventional oncology in the treatment of colorectal cancer liver metastases. Ann Gastroenterol (2019) 32(2):147. doi: 10.20524/aog.2019.0338
58. Rhim H, Goldberg SN, Dodd GD, Solbiati L, Lim HK, Tonolini M, et al. Essential techniques for successful radio-frequency thermal ablation of malignant hepatic tumors. Radiographics (2001) 21(suppl_1):S17–35. doi: 10.1148/radiographics.21.suppl_1.g01oc11s17
59. Solbiati L, Ahmed M, Cova L, Ierace T, Brioschi M, Goldberg SN. Small liver colorectal metastases treated with percutaneous radiofrequency ablation: local response rate and long-term survival with up to 10-year follow-up. Radiology (2012) 265(3):958–68. doi: 10.1148/radiol.12111851
60. Kei SK, Rhim H, Choi D, Lee WJ, Lim HK, Kim Y-s. Local tumor progression after radiofrequency ablation of liver tumors: analysis of morphologic pattern and site of recurrence. Am J Roentgenol (2008) 190(6):1544–51. doi: 10.2214/AJR.07.2798
61. Wang C-Z, Yan G-X, Xin H, Liu Z-Y. Oncological outcomes and predictors of radiofrequency ablation of colorectal cancer liver metastases. World J Gastrointest Oncol (2020) 12(9):1044. doi: 10.4251/wjgo.v12.i9.1044
62. de Baere T, Deschamps F, Briggs P, Dromain C, Boige V, Hechelhammer L, et al. Hepatic malignancies: percutaneous radiofrequency ablation during percutaneous portal or hepatic vein occlusion. Radiology (2008) 248(3):1056–66. doi: 10.1148/radiol.2483070222
63. Lu DS, Raman SS, Limanond P, Aziz D, Economou J, Busuttil R, et al. Influence of large peritumoral vessels on outcome of radiofrequency ablation of liver tumors. J Vasc Interv Radiol (2003) 14(10):1267–74. doi: 10.1097/01.RVI.0000092666.72261.6B
64. Nielsen K, van Tilborg AA, Meijerink MR, Macintosh MO, Zonderhuis BM, de Lange ES, et al. Incidence and treatment of local site recurrences following RFA of colorectal liver metastases. World J Surg (2013) 37(6):1340–7. doi: 10.1007/s00268-013-1997-6
65. Curley SA, Marra P, Beaty K, Ellis LM, Vauthey JN, Abdalla EK, et al. Early and late complications after radiofrequency ablation of malignant liver tumors in 608 patients. Ann Surg (2004) 239(4):450. doi: 10.1097/01.sla.0000118373.31781.f2
66. Kim KH, Yoon YS, Yu CS, Kim TW, Kim HJ, Kim PN, et al. Comparative analysis of radiofrequency ablation and surgical resection for colorectal liver metastases. J Korean Surg Society (2011) 81(1):25–34. doi: 10.4174/jkss.2011.81.1.25
67. Gillams AR, Lees WR. Five-year survival following radiofrequency ablation of small, solitary, hepatic colorectal metastases. J Vasc Interv Radiol (2008) 19(5):712–7. doi: 10.1016/j.jvir.2008.01.016
68. Wang X, Sofocleous CT, Erinjeri JP, Petre EN, Gonen M, Do KG, et al. Margin size is an independent predictor of local tumor progression after ablation of colon cancer liver metastases. Cardiovasc Interv Radiol (2013) 36(1):166–75. doi: 10.1007/s00270-012-0377-1
69. Elias D, Baton O, Sideris L, Matsuhisa T, Pocard M, Lasser P. Local recurrences after intraoperative radiofrequency ablation of liver metastases: a comparative study with anatomic and wedge resections. Ann Surg Oncol (2004) 11(5):500–5. doi: 10.1245/ASO.2004.08.019
70. Xie X, Jiang C, Peng Z, Liu B, Hu W, Wang Y, et al. Local recurrence after radiofrequency ablation of hepatocellular carcinoma: treatment choice and outcome. J Gastrointest Surg (2015) 19(8):1466–75. doi: 10.1007/s11605-015-2850-z
71. Pathak S, Jones R, Tang J, Parmar C, Fenwick S, Malik H, et al. Ablative therapies for colorectal liver metastases: a systematic review. Colorectal Dis (2011) 13(9):e252–e65. doi: 10.1111/j.1463-1318.2011.02695.x
72. Filippiadis DK, Velonakis G, Kelekis A, Sofocleous CT. The role of percutaneous ablation in the management of colorectal cancer liver metastatic disease. Diagnost (Basel) (2021) 11(2):308. doi: 10.3390/diagnostics11020308
73. Ierardi AM, Floridi C, Fontana F, Chini C, Giorlando F, Piacentino F, et al. Microwave ablation of liver metastases to overcome the limitations of radiofrequency ablation. La Radiol Medica (2013) 118(6):949–61. doi: 10.1007/s11547-013-0968-1
74. Gravante G, Ong SL, Metcalfe MS, Strickland A, Dennison AR, Lloyd DM. Hepatic microwave ablation: a review of the histological changes following thermal damage. Liver Int (2008) 28(7):911–21. doi: 10.1111/j.1478-3231.2008.01810.x
75. Spiers HV, Lancellotti F, de Liguori Carino N, Pandanaboyana S, Frampton AE, Jegatheeswaran S, et al. Irreversible electroporation for liver metastases from colorectal cancer: a systematic review. Cancers (2023) 15(9):2428. doi: 10.3390/cancers15092428
76. Nieuwenhuizen S, Dijkstra M, Puijk RS, Geboers B, Ruarus AH, Schouten EA, et al. Microwave ablation, radiofrequency ablation, irreversible electroporation, and stereotactic ablative body radiotherapy for intermediate size (3-5 cm) unresectable colorectal liver metastases: a systematic review and meta-analysis. Curr Oncol Rep (2022) 24(6):793–808. doi: 10.1007/s11912-022-01248-6
77. Torres-Jiménez J, Esteban-Villarrubia J, Ferreiro-Monteagudo R, Carrato A. Local treatments in the unresectable patient with colorectal cancer metastasis: a review from the point of view of the medical oncologist. Cancers (Basel) (2021) 13(23):5938. doi: 10.3390/cancers13235938
78. Scheffer HJ, Nielsen K, van Tilborg AA, Vieveen JM, Bouwman RA, Kazemier G, et al. Ablation of colorectal liver metastases by irreversible electroporation: results of the COLDFIRE-I ablate-and-resect study. Eur Radiol (2014) 24(10):2467–75. doi: 10.1007/s00330-014-3259-x
79. Meijerink MR, Ruarus AH, Vroomen LGPH, Puijk RS, Geboers B, Nieuwenhuizen S, et al. Irreversible electroporation to treat unresectable colorectal liver metastases (COLDFIRE-2): a phase II, two-center, single-arm clinical trial. Radiology (2021) 299(2):470–80. doi: 10.1148/radiol.2021203089
80. Schicho A, Niessen C, Haimerl M, Wiesinger I, Stroszczynski C, Beyer LP, et al. Long-term survival after percutaneous irreversible electroporation of inoperable colorectal liver metastases. Cancer Manag Res (2019) 11:317–22. doi: 10.2147/CMAR.S182091
81. Filippiadis D, Mauri G, Marra P, Charalampopoulos G, Gennaro N, De Cobelli F. Percutaneous ablation techniques for renal cell carcinoma: current status and future trends. Int J Hyperthermia (2019) 36(2):21–30. doi: 10.1080/02656736.2019.1647352
82. Crocetti L, de Baére T, Pereira PL, Tarantino FP. CIRSE standards of practice on thermal ablation of liver tumours. Cardiovasc Intervent Radiol (2020) 43(7):951–62. doi: 10.1007/s00270-020-02471-z
83. Ruers T, Van Coevorden F, Punt CJ, Pierie J-PE, Borel-Rinkes I, Ledermann JA, et al. Local treatment of unresectable colorectal liver metastases: results of a randomized phase II trial. JNCI: J Natl Cancer Institute (2017) 109(9):djx015. doi: 10.1093/jnci/djx015
84. Kazemier G. Unresectable colorectal cancer liver metastases treated by intraoperative radiofrequency ablation with or without resection (Br J surg 2012; 99: 558–565). Br J Surg (2012) 99(4):566. doi: 10.1002/bjs.8724
85. Ruers T, Punt C, Van Coevorden F, Pierie J, Borel-Rinkes I, Ledermann J, et al. Radiofrequency ablation combined with systemic treatment versus systemic treatment alone in patients with non-resectable colorectal liver metastases: a randomized EORTC intergroup phase II study (EORTC 40004). Ann Oncol (2012) 23(10):2619–26. doi: 10.1093/annonc/mds053
86. Lee SL, Bassetti MF, Rusthoven CG. The role of stereotactic body radiation therapy in the management of liver metastases. Semin Radiat Oncol (2023) 33(2):181–92. doi: 10.1016/j.semradonc.2022.11.008
87. Mohamad I, Barry A, Dawson L, Hosni A. Stereotactic body radiation therapy for colorectal liver metastases. Int J Hyperthermia (2022) 39(1):611–9. doi: 10.1080/02656736.2021.1923836
88. Tubin S, Gupta S, Grusch M, Popper HH, Brcic L, Ashdown ML, et al. Shifting the immune-suppressive to predominant immune-stimulatory radiation effects by SBRT-PArtial tumor irradiation targeting HYpoxic segment (SBRT-PATHY). Cancers (Basel) (2020) 13(1):50. doi: 10.3390/cancers13010050
89. Morris VK, Kennedy EB, Baxter NN, Benson AB, Cercek A, Cho M, et al. Treatment of metastatic colorectal cancer: ASCO guideline. J Clin Oncol (2023) 41(3):678–700. doi: 10.1200/JCO.22.01690
90. Petrelli F, Comito T, Barni S, Pancera G, Scorsetti M, Ghidini A, et al. Stereotactic body radiotherapy for colorectal cancer liver metastases: a systematic review. Radiother Oncol (2018) 129(3):427–34. doi: 10.1016/j.radonc.2018.06.035
91. Lee J, Shin IS, Yoon WS, Koom WS, Rim CH. Comparisons between radiofrequency ablation and stereotactic body radiotherapy for liver malignancies: meta-analyses and a systematic review. Radiother Oncol (2020) 145:63–70. doi: 10.1016/j.radonc.2019.12.004
92. Entezari P, Gabr A, Salem R, Lewandowski RJ. Yttrium-90 for colorectal liver metastasis - the promising role of radiation segmentectomy as an alternative local cure. Int J Hyperthermia (2022) 39(1):620–6. doi: 10.1080/02656736.2021.1933215
93. Vouche M, Habib A, Ward TJ, Kim E, Kulik L, Ganger D, et al. Unresectable solitary hepatocellular carcinoma not amenable to radiofrequency ablation: multicenter radiology-pathology correlation and survival of radiation segmentectomy. Hepatology (2014) 60(1):192–201. doi: 10.1002/hep.27057
94. Dupré A, Jones RP, Diaz-Nieto R, Fenwick SW, Poston GJ, Malik HZ. Curative-intent treatment of recurrent colorectal liver metastases: a comparison between ablation and resection. Eur J Surg Oncol (2017) 43(10):1901–7. doi: 10.1016/j.ejso.2017.08.008
95. He X, Zhang P, Li Z, Bi F, Xu F, Wang X, et al. Curative-intent radiotherapy in patients with oligometastatic lesions from colorectal cancer: a single-center study. Med (Baltimore) (2018) 97(40):e12601. doi: 10.1097/MD.0000000000012601
96. Jia Z, Wang C, Paz-Fumagalli R, Wang W. Radiation segmentectomy for hepatic malignancies: indications, devices, dosimetry, procedure, clinical outcomes, and toxicity of yttrium-90 microspheres. J Interv Med (2019) 2(1):1–4. doi: 10.1016/j.jimed.2019.05.001
97. Zerizer I, Al-Nahhas A, Towey D, Tait P, Ariff B, Wasan H, et al. The role of early 18F-FDG PET/CT in prediction of progression-free survival after 90Y radioembolization: comparison with RECIST and tumour density criteria. Eur J Nucl Med Mol Imag (2012) 39(9):1391–9. doi: 10.1007/s00259-012-2149-1
98. Jongen JMJ, Rosenbaum C, Braat M, van den Bosch M, Sze DY, Kranenburg O, et al. Anatomic versus metabolic tumor response assessment after radioembolization treatment. J Vasc Interv Radiol (2018) 29(2):244–53.e2. doi: 10.1016/j.jvir.2017.09.024
99. Sager S, Akgün E, Uslu-Beşli L, Asa S, Akovali B, Sahin O, et al. Comparison of PERCIST and RECIST criteria for evaluation of therapy response after yttrium-90 microsphere therapy in patients with hepatocellular carcinoma and those with metastatic colorectal carcinoma. Nucl Med Commun (2019) 40(5):461–8. doi: 10.1097/MNM.0000000000001014
100. Shady W, Kishore S, Gavane S, Do RK, Osborne JR, Ulaner GA, et al. Metabolic tumor volume and total lesion glycolysis on FDG-PET/CT can predict overall survival after (90)Y radioembolization of colorectal liver metastases: a comparison with SUVmax, SUVpeak, and RECIST 1.0. Eur J Radiol (2016) 85(6):1224–31. doi: 10.1016/j.ejrad.2016.03.029
101. Shady W, Sotirchos VS, Do RK, Pandit-Taskar N, Carrasquillo JA, Gonen M, et al. Surrogate imaging biomarkers of response of colorectal liver metastases after salvage radioembolization using 90Y-loaded resin microspheres. AJR Am J Roentgenol (2016) 207(3):661–70. doi: 10.2214/AJR.15.15202
102. Kurilova I, Bendet A, Fung EK, Petre EN, Humm JL, Boas FE, et al. Radiation segmentectomy of hepatic metastases with y-90 glass microspheres. Abdom Radiol (NY) (2021) 46(7):3428–36. doi: 10.1007/s00261-021-02956-6
103. Padia SA, Johnson GE, Agopian VG, DiNorcia J, Srinivasa RN, Sayre J, et al. Yttrium-90 radiation segmentectomy for hepatic metastases: a multi-institutional study of safety and efficacy. J Surg Oncol (2021) 123(1):172–8. doi: 10.1002/jso.26223
104. Meiers C, Taylor A, Geller B, Toskich B. Safety and initial efficacy of radiation segmentectomy for the treatment of hepatic metastases. J Gastrointest Oncol (2018) 9(2):311–5. doi: 10.21037/jgo.2017.11.02
105. Padia SA, Kwan SW, Roudsari B, Monsky WL, Coveler A, Harris WP. Superselective yttrium-90 radioembolization for hepatocellular carcinoma yields high response rates with minimal toxicity. J Vasc Interv Radiol (2014) 25(7):1067–73. doi: 10.1016/j.jvir.2014.03.030
106. Biederman DM, Titano JJ, Korff RA, Fischman AM, Patel RS, Nowakowski FS, et al. Radiation segmentectomy versus selective chemoembolization in the treatment of early-stage hepatocellular carcinoma. J Vasc Interv Radiol (2018) 29(1):30–7.e2. doi: 10.1016/j.jvir.2017.08.026
107. Lewandowski RJ, Gabr A, Abouchaleh N, Ali R, Al Asadi A, Mora RA, et al. Radiation segmentectomy: potential curative therapy for early hepatocellular carcinoma. Radiology (2018) 287(3):1050–8. doi: 10.1148/radiol.2018171768
108. Haber Z, Lee EW, Price M, Wainberg Z, Hecht JR, Sayre J, et al. Survival advantage of yttrium-90 radioembolization to systemic therapy in patients with hepatic metastases from colorectal cancer in the salvage setting: results of a matched pair study. Acad Radiol (2021) 28:S210–S7. doi: 10.1016/j.acra.2021.03.033
109. Gibbs P, Gebski V, Van Buskirk M, Thurston K, Cade DN, Van Hazel GA. Selective internal radiation therapy (SIRT) with yttrium-90 resin microspheres plus standard systemic chemotherapy regimen of FOLFOX versus FOLFOX alone as first-line treatment of non-resectable liver metastases from colorectal cancer: the SIRFLOX study. BMC Cancer (2014) 14:897. doi: 10.1186/1471-2407-14-897
110. Van Hazel GA, Heinemann V, Sharma NK, Peeters M. SIRFLOX: randomized phase III trial comparing first-line mFOLFOX6 (plus or minus bevacizumab) versus mFOLFOX6 (plus or minus bevacizumab) plus selective internal radiation therapy in patients with metastatic colorectal cancer. J Clin Oncol -New York (2016) 34(15):1723–31. doi: 10.1200/JCO.2015.66.1181
111. Dutton SJ, Kenealy N, Love SB, Wasan HS, Sharma RA. FOXFIRE protocol: an open-label, randomised, phase III trial of 5-fluorouracil, oxaliplatin and folinic acid (OxMdG) with or without interventional selective internal radiation therapy (SIRT) as first-line treatment for patients with unresectable liver-only or liver-dominant metastatic colorectal cancer. BMC Cancer (2014) 14:497. doi: 10.1186/1471-2407-14-497
112. Gibbs P, Heinemann V, Sharma NK, Taieb J, Ricke J, Peeters M, et al. Effect of primary tumor side on survival outcomes in untreated patients with metastatic colorectal cancer when selective internal radiation therapy is added to chemotherapy: combined analysis of two randomized controlled studies. Clin Colorectal Cancer (2018) 17(4):e617–e29. doi: 10.1016/j.clcc.2018.06.001
113. Wasan HS, Gibbs P, Sharma NK, Taieb J, Heinemann V, Ricke J, et al. First-line selective internal radiotherapy plus chemotherapy versus chemotherapy alone in patients with liver metastases from colorectal cancer (FOXFIRE, SIRFLOX, and FOXFIRE-global): a combined analysis of three multicentre, randomised, phase 3 trials. Lancet Oncol (2017) 18(9):1159–71. doi: 10.1016/S1470-2045(17)30457-6
114. van Hazel G, Heinemann V, Sharma N, Taieb J, Ricke J, Peeters M, et al. Impact of primary tumour location on survival in patients with metastatic colorectal cancer receiving selective internal radiation therapy and chemotherapy as first-line therapy. Ann Oncol (2017) 28:iii152. doi: 10.1093/annonc/mdx302.005
115. Garlipp B, Gibbs P, Van Hazel GA, Jeyarajah R, Martin RCG, Bruns CJ, et al. Secondary technical resectability of colorectal cancer liver metastases after chemotherapy with or without selective internal radiotherapy in the randomized SIRFLOX trial. Br J Surg (2019) 106(13):1837–46. doi: 10.1002/bjs.11283
117. Vogl TJ, Zangos S, Eichler K, Yakoub D, Nabil M. Colorectal liver metastases: regional chemotherapy via transarterial chemoembolization (TACE) and hepatic chemoperfusion: an update. Eur Radiol (2007) 17(4):1025–34. doi: 10.1007/s00330-006-0372-5
118. Yamada R, Sato M, Kawabata M, Nakatsuka H, Nakamura K, Takashima S. Hepatic artery embolization in 120 patients with unresectable hepatoma. Radiology (1983) 148(2):397–401. doi: 10.1148/radiology.148.2.6306721
119. Gruber-Rouh T, Marko C, Thalhammer A, Nour-Eldin NE, Langenbach M, Beeres M, et al. Current strategies in interventional oncology of colorectal liver metastases. Br J Radiol (2016) 89(1064):20151060. doi: 10.1259/bjr.20151060
120. Albert M, Kiefer MV, Sun W, Haller D, Fraker DL, Tuite CM, et al. Chemoembolization of colorectal liver metastases with cisplatin, doxorubicin, mitomycin c, ethiodol, and polyvinyl alcohol. Cancer (2011) 117(2):343–52. doi: 10.1002/cncr.25387
121. Maraj T, Mirrahimi A, Dey C. Survival of patients with colorectal liver metastases after transarterial chemoembolization using irinotecan-eluting microspheres: a single-center retrospective analysis comparing RECIST 1.1 and choi criteria. J Vasc Interv Radiol (2023). doi: 10.1016/j.jvir.2023.02.005
122. Vogl TJ, Lahrsow M, Albrecht MH, Hammerstingl R, Thompson ZM, Gruber-Rouh T. Survival of patients with non-resectable, chemotherapy-resistant colorectal cancer liver metastases undergoing conventional lipiodol-based transarterial chemoembolization (cTACE) palliatively versus neoadjuvantly prior to percutaneous thermal ablation. Eur J Radiol (2018) 102:138–45. doi: 10.1016/j.ejrad.2018.03.015
123. Gruber-Rouh T, Naguib NN, Eichler K, Ackermann H, Zangos S, Trojan J, et al. Transarterial chemoembolization of unresectable systemic chemotherapy-refractory liver metastases from colorectal cancer: long-term results over a 10-year period. Int J Cancer (2014) 134(5):1225–31. doi: 10.1002/ijc.28443
124. Nishiofuku H, Tanaka T, Matsuoka M, Otsuji T, Anai H, Sueyoshi S, et al. Transcatheter arterial chemoembolization using cisplatin powder mixed with degradable starch microspheres for colorectal liver metastases after FOLFOX failure: results of a phase I/II study. J Vasc Interv Radiol (2013) 24(1):56–65. doi: 10.1016/j.jvir.2012.09.010
125. Müller H, Nakchbandi V, Chatzisavvidis I, von Voigt C. Repetitive chemoembolization with melphalan plus intra-arterial immuno-chemotherapy within 5-fluorouracil and granulocyte-macrophage colony-stimulating factor (GM-CSF) as effective first- and second-line treatment of disseminated colorectal liver metastases. Hepatogastroenterology (2003) 50(54):1919–26.
126. Wasser K, Giebel F, Fischbach R, Tesch H, Landwehr P. [Transarterial chemoembolization of liver metastases of colorectal carcinoma using degradable starch microspheres (Spherex): personal investigations and review of the literature]. Radiologe (2005) 45(7):633–43. doi: 10.1007/s00117-004-1061-5
127. Vogl TJ, Marko C, Langenbach MC, Naguib NNN, Filmann N, Hammerstingl R, et al. Transarterial chemoembolization of colorectal cancer liver metastasis: improved tumor response by DSM-TACE versus conventional TACE, a prospective, randomized, single-center trial. Eur Radiol (2021) 31(4):2242–51. doi: 10.1007/s00330-020-07253-2
128. Seager MJ, Jakobs TF, Sharma RA, Bandula S. Combination of ablation and embolization for intermediate-sized liver metastases from colorectal cancer: what can we learn from treating primary liver cancer? Diagn Interv Radiol (2021) 27(5):677–83. doi: 10.5152/dir.2021.20520
129. Faiella E, Calabrese A, Santucci D, de Felice C, Pusceddu C, Fior D, et al. Combined trans-arterial embolization and ablation for the treatment of Large (>3 cm) liver metastases: review of the literature. J Clin Med (2022) 11(19):5576. doi: 10.3390/jcm11195576
130. Aliberti C, Fiorentini G, Muzzio PC, Pomerri F, Tilli M, Dallara S, et al. Trans-arterial chemoembolization of metastatic colorectal carcinoma to the liver adopting DC bead®, drug-eluting bead loaded with irinotecan: results of a phase II clinical study. Anticancer Res (2011) 31(12):4581–7.
131. Martin RC, Robbins K, Tomalty D, O'Hara R, Bosnjakovic P, Padr R, et al. Transarterial chemoembolisation (TACE) using irinotecan-loaded beads for the treatment of unresectable metastases to the liver in patients with colorectal cancer: an interim report. World J Surg Oncol (2009) 7:80. doi: 10.1186/1477-7819-7-80
132. Fiorentini G, Aliberti C, Tilli M, Mulazzani L, Graziano F, Giordani P, et al. Intra-arterial infusion of irinotecan-loaded drug-eluting beads (DEBIRI) versus intravenous therapy (FOLFIRI) for hepatic metastases from colorectal cancer: final results of a phase III study. Anticancer Res (2012) 32(4):1387–95.
133. Martin RC 2nd, Scoggins CR, Schreeder M, Rilling WS, Laing CJ, Tatum CM, et al. Randomized controlled trial of irinotecan drug-eluting beads with simultaneous FOLFOX and bevacizumab for patients with unresectable colorectal liver-limited metastasis. Cancer (2015) 121(20):3649–58. doi: 10.1002/cncr.29534
134. Szemitko M, Golubinska-Szemitko E, Sienko J, Falkowski A. Complications following irinotecan-loaded microsphere chemoembolization of colorectal metastatic liver lesions associated with hepatic-artery branch temporary stasis. Curr Oncol (2021) 28(3):2296–307. doi: 10.3390/curroncol28030211
135. Akinwande O, Dendy M, Ludwig JM, Kim HS. Hepatic intra-arterial injection of irinotecan drug eluting beads (DEBIRI) for patients with unresectable colorectal liver metastases: a systematic review. Surg Oncol (2017) 26(3):268–75. doi: 10.1016/j.suronc.2017.05.003
136. Dhand S, Gupta R. Hepatic transcatheter arterial chemoembolization complicated by postembolization syndrome. Semin Intervent Radiol (2011) 28(2):207–11. doi: 10.1055/s-0031-1280666
137. Gonsalves CF, Brown DB. Chemoembolization of hepatic malignancy. Abdom Imag (2009) 34(5):557–65. doi: 10.1007/s00261-008-9446-y
138. Paye F, Farges O, Dahmane M, Vilgrain V, Flejou JF, Belghiti J. Cytolysis following chemoembolization for hepatocellular carcinoma. Br J Surg (1999) 86(2):176–80. doi: 10.1046/j.1365-2168.1999.01014.x
139. He Y, Zhang Y, Gong Y, Zhang Z, Xu T, Tian L, et al. Multimodal imaging of nano-assembled microspheres loaded with doxorubicin and cisplatin for liver tumor therapy. Front Bioeng Biotechnol (2022) 10:1024174. doi: 10.3389/fbioe.2022.1024174
140. Dendy MS, Ludwig JM, Kim HS. Predictors and prognosticators for survival with yttrium-90 radioembolization therapy for unresectable colorectal cancer liver metastasis. Oncotarget (2017) 8(23):37912–22. doi: 10.18632/oncotarget.16007
141. Wang DS, Louie JD, Sze DY. Evidence-based integration of yttrium-90 radioembolization in the contemporary management of hepatic metastases from colorectal cancer. Tech Vasc Interv Radiol (2019) 22(2):74–80. doi: 10.1053/j.tvir.2019.02.007
142. Kalva SP, Rana RS, Liu R, Rachamreddy N, Dave B, Sharma A, et al. Yttrium-90 radioembolization as salvage therapy for liver metastases from colorectal cancer. Am J Clin Oncol (2017) 40(3):288–93. doi: 10.1097/COC.0000000000000151
143. Hickey R, Lewandowski RJ, Prudhomme T, Ehrenwald E, Baigorri B, Critchfield J, et al. 90Y radioembolization of colorectal hepatic metastases using glass microspheres: safety and survival outcomes from a 531-patient multicenter study. J Nucl Med (2016) 57(5):665–71. doi: 10.2967/jnumed.115.166082
144. Kennedy AS, Ball D, Cohen SJ, Cohn M, Coldwell DM, Drooz A, et al. Multicenter evaluation of the safety and efficacy of radioembolization in patients with unresectable colorectal liver metastases selected as candidates for (90)Y resin microspheres. J Gastrointest Oncol (2015) 6(2):134–42. doi: 10.3978/j.issn.2078-6891.2014.109
145. Saxena A, Bester L, Shan L, Perera M, Gibbs P, Meteling B, et al. A systematic review on the safety and efficacy of yttrium-90 radioembolization for unresectable, chemorefractory colorectal cancer liver metastases. J Cancer Res Clin Oncol (2014) 140(4):537–47. doi: 10.1007/s00432-013-1564-4
146. Evans KA, Richardson MG, Pavlakis N, Morris DL, Liauw W, Bester L. Survival outcomes of a salvage patient population after radioembolization of hepatic metastases with yttrium-90 microspheres. J Vasc Interv Radiol (2010) 21(10):1521–6. doi: 10.1016/j.jvir.2010.06.018
147. Cianni R, Urigo C, Notarianni E, Saltarelli A, Salvatori R, Pasqualini V, et al. Selective internal radiation therapy with SIR-spheres for the treatment of unresectable colorectal hepatic metastases. Cardiovasc Intervent Radiol (2009) 32(6):1179–86. doi: 10.1007/s00270-009-9658-8
148. Mulcahy MF, Lewandowski RJ, Ibrahim SM, Sato KT, Ryu RK, Atassi B, et al. Radioembolization of colorectal hepatic metastases using yttrium-90 microspheres. Cancer (2009) 115(9):1849–58. doi: 10.1002/cncr.24224
149. Kennedy AS, Coldwell D, Nutting C, Murthy R, Wertman DE Jr., Loehr SP, et al. Resin 90Y-microsphere brachytherapy for unresectable colorectal liver metastases: modern USA experience. Int J Radiat Oncol Biol Phys (2006) 65(2):412–25. doi: 10.1016/j.ijrobp.2005.12.051
150. Helmberger T, Golfieri R, Pech M, Pfammatter T, Arnold D, Cianni R, et al. Clinical application of trans-arterial radioembolization in hepatic malignancies in Europe: first results from the prospective multicentre observational study CIRSE registry for SIR-spheres therapy (CIRT). Cardiovasc Intervent Radiol (2021) 44(1):21–35. doi: 10.1007/s00270-020-02642-y
151. Wu V, Li MD, Goodwin JS, Wehrenberg-Klee EP, Zurkiya O, Kalva SP, et al. Yttrium-90 hepatic radioembolization for advanced chemorefractory metastatic colorectal cancer: survival outcomes based on right- versus left-sided primary tumor location. AJR Am J Roentgenol (2021) 217(5):1141–52. doi: 10.2214/AJR.20.25315
152. Lahti SJ, Xing M, Zhang D, Lee JJ, Magnetta MJ, Kim HS. KRAS status as an independent prognostic factor for survival after yttrium-90 radioembolization therapy for unresectable colorectal cancer liver metastases. J Vasc Interv Radiol (2015) 26(8):1102–11. doi: 10.1016/j.jvir.2015.05.032
153. Narsinh KH, Van Buskirk M, Kennedy AS, Suhail M, Alsaikhan N, Hoh CK, et al. Hepatopulmonary shunting: a prognostic indicator of survival in patients with metastatic colorectal adenocarcinoma treated with (90)Y radioembolization. Radiology (2017) 282(1):281–8. doi: 10.1148/radiol.2016152100
154. Riaz A, Lewandowski RJ, Kulik LM, Mulcahy MF, Sato KT, Ryu RK, et al. Complications following radioembolization with yttrium-90 microspheres: a comprehensive literature review. J Vasc Interv Radiol (2009) 20(9):1121–30. doi: 10.1016/j.jvir.2009.05.030
155. Hwang JE, Kim SH, Jin J, Hong JY, Kim MJ, Jung SH, et al. Combination of percutaneous radiofrequency ablation and systemic chemotherapy are effective treatment modalities for metachronous liver metastases from gastric cancer. Clin Exp Metastasis (2014) 31(1):25–32. doi: 10.1007/s10585-013-9606-5
156. Schullian P, Johnston E, Laimer G, Putzer D, Eberle G, Scharll Y, et al. Stereotactic radiofrequency ablation of breast cancer liver metastases: short- and long-term results with predicting factors for survival. Cardiovasc Intervent Radiol (2021) 44(8):1184–93. doi: 10.1007/s00270-021-02820-6
157. Vogl TJ, Jaraysa Y, Martin SS, Gruber-Rouh T, Savage RH, Nour-Eldin NA, et al. A prospective randomized trial comparing microwave and radiofrequency ablation for the treatment of liver metastases using a dual ablation system ─ the Mira study. Eur J Radiol Open (2022) 9:100399. doi: 10.1016/j.ejro.2022.100399
158. Bi Y, Jiao D, Wang Y, Han X, Ren J. Preliminary outcomes of raltitrexed eluting bead-transarterial chemoembolization using callispheres® beads for gastrointestinal adenocarcinoma liver metastasis. World J Surg Oncol (2022) 20(1):229. doi: 10.1186/s12957-022-02696-x
159. Barat M, Cottereau AS, Kedra A, Dermine S, Palmieri LJ, Coriat R, et al. The role of interventional radiology for the treatment of hepatic metastases from neuroendocrine tumor: an updated review. J Clin Med (2020) 9(7):2302. doi: 10.3390/jcm9072302
160. Fiorentini G, Sarti D, Nani R, Aliberti C, Fiorentini C, Guadagni S. Updates of colorectal cancer liver metastases therapy: review on DEBIRI. Hepat Oncol (2020) 7(1):HEP16. doi: 10.2217/hep-2019-0010
161. Maire F, Lombard-Bohas C, O'Toole D, Vullierme MP, Rebours V, Couvelard A, et al. Hepatic arterial embolization versus chemoembolization in the treatment of liver metastases from well-differentiated midgut endocrine tumors: a prospective randomized study. Neuroendocrinology (2012) 96(4):294–300. doi: 10.1159/000336941
162. Elf AK, Andersson M, Henrikson O, Jalnefjord O, Ljungberg M, Svensson J, et al. Radioembolization versus bland embolization for hepatic metastases from small intestinal neuroendocrine tumors: short-term results of a randomized clinical trial. World J Surg (2018) 42(2):506–13. doi: 10.1007/s00268-017-4324-9
163. Saxena A, Kapoor J, Meteling B, Morris DL, Bester L. Yttrium-90 radioembolization for unresectable, chemoresistant breast cancer liver metastases: a large single-center experience of 40 patients. Ann Surg Oncol (2014) 21(4):1296–303. doi: 10.1245/s10434-013-3436-1
164. Dai Y, Zhao W, Yue L, Dai X, Rong D, Wu F, et al. Perspectives on immunotherapy of metastatic colorectal cancer. Front Oncol (2021) 11:659964. doi: 10.3389/fonc.2021.659964
165. Erinjeri JP, Fine GC, Adema GJ, Ahmed M, Chapiro J, den Brok M, et al. Immunotherapy and the interventional oncologist: challenges and opportunities-a society of interventional oncology white paper. Radiology (2019) 292(1):25–34. doi: 10.1148/radiol.2019182326
166. Adnan A, Sheth RA, Tam A. Oligometastatic disease in the liver: the role of interventional oncology. Br J Radiol (2022) 95(1138):20211350. doi: 10.1259/bjr.20211350
167. Shi L, Chen L, Wu C, Zhu Y, Xu B, Zheng X, et al. PD-1 blockade boosts radiofrequency ablation-elicited adaptive immune responses against tumor. Clin Cancer Res (2016) 22(5):1173–84. doi: 10.1158/1078-0432.CCR-15-1352
168. Zhang XL, Hu LP, Yang Q, Qin WT, Wang X, Xu CJ, et al. CTHRC1 promotes liver metastasis by reshaping infiltrated macrophages through physical interactions with TGF-β receptors in colorectal cancer. Oncogene (2021) 40(23):3959–73. doi: 10.1038/s41388-021-01827-0
Keywords: colorectal metastases, hepatic colorectal metastases, interventional oncology, interventions in colorectal metastases, TARE, TACE, percutaneous ablation, DEBIRI-TACE
Citation: Vulasala SSR, Sutphin PD, Kethu S, Onteddu NK and Kalva SP (2023) Interventional radiological therapies in colorectal hepatic metastases. Front. Oncol. 13:963966. doi: 10.3389/fonc.2023.963966
Received: 08 June 2022; Accepted: 19 May 2023;
Published: 30 May 2023.
Edited by:
Xiankai Sun, University of Texas Southwestern Medical Center, United StatesReviewed by:
Vlastimil Válek, University Hospital Brno, CzechiaAimin Jiang, The First Affiliated Hospital of Xi’an Jiaotong University, China
Hridayesh Prakash, Amity University, India
Copyright © 2023 Vulasala, Sutphin, Kethu, Onteddu and Kalva. This is an open-access article distributed under the terms of the Creative Commons Attribution License (CC BY). The use, distribution or reproduction in other forums is permitted, provided the original author(s) and the copyright owner(s) are credited and that the original publication in this journal is cited, in accordance with accepted academic practice. No use, distribution or reproduction is permitted which does not comply with these terms.
*Correspondence: Sanjeeva P. Kalva, skalva@partners.org