- 1Department of Epidemiology and Biostatistics, School of Public Health, Jilin University, Changchun, China
- 2Department of Radiotherapy, China-Japan Union Hospital, Jilin University, Changchun, China
Introduction: This meta-analysis aims to provide evidence-based medical evidence for formulating rational treatment strategies and evaluating the prognosis of brain metastasis (BM) patients by assessing the effectiveness of the graded prognostic assessment (GPA) model in predicting the survival prognosis of patients with BM after whole-brain radiotherapy (WBRT).
Methods: A comprehensive search was conducted in multiple databases, including the China Biomedical Literature Database (CBM), China National Knowledge Infrastructure (CNKI), PubMed, Wanfang database, Cochrane Library, Web of Science, and Embase. Cohort studies that met the inclusion and exclusion criteria were selected. The quality of the included literature was evaluated using the Newcastle-Ottawa Scale, and all statistical analyses were performed with R version 4.2.2. The effect size (ES) was measured by the hazard ratio (HR) of overall survival (OS). The OS rates at 3, 6, 12, and 24 months of patients with BM were compared between those with GPAs of 1.5–2.5, 3.0, and 3.5–4.0 and those with GPAs of 0–1 after WBRT.
Results: A total of 1,797 participants who underwent WBRT were included in this study. The meta-analysis revealed a significant association between GPA and OS rates after WBRT: compared with BM patients with GPA of 0–1, 3-month OS rates after WBRT were significantly higher in BM patients with GPA of 1.5–2.5 (HR = 0.48; 95% CI: 0.40–0.59), GPA of 3 (HR = 0.38; 95% CI: 0.25–0.57), and GPA of 3.5–4 (HR = 0.28; 95% CI: 0.15–0.52); 6-month OS rates after WBRT were significantly higher in BM patients with GPA of 1.5–2.5 (HR = 0.48; 95% CI: 0.41–0.56), GPA of 3 (HR = 0.33; 95% CI: 0.24–0.45), and GPA of 3.5–4 (HR = 0.24; 95% CI: 0.16–0.35); 12-month OS rates after WBRT were significantly higher in BM patients with GPA of 1.5–2.5 (HR = 0.49; 95% CI: 0.41–0.58), GPA of 3 (HR = 0.48; 95% CI: 0.32–0.73), and GPA of 3.5–4 (HR = 0.31; 95% CI: 0.12–0.79); and 24-month OS rates after WBRT were significantly higher in BM patients with GPA of 1.5–2.5 (HR = 0.49; 95% CI: 0.42–0.58), GPA of 3 (HR = 0.49; 95% CI: 0.32–0.74), and GPA of 3.5–4 (HR = 0.38; 95% CI: 0.15–0.94).
Conclusion: BM patients with higher GPAs generally exhibited better prognoses and survival outcomes after WBRT compared to those with lower GPAs.
Systematic review registration: https://www.crd.york.ac.uk/prospero/, identifier CRD42023422914.
Introduction
In recent years, brain metastasis (BM) has become one of the most common malignant tumors in adults (1). BM occurs when tumor cells from other parts of the body spread to the central nervous system through the bloodstream (2). It has been observed that over 25% of patients with malignant tumors develop metastases in the process of disease progression (3). Due to advancements in clinical imaging techniques, improved tumor treatments, increased patient survival times, and an aging population, the incidence and clinical detection rates of BM are increasing year by year (4–10). Consequently, the number of patients with BM is increasing, and they often experience poor prognosis and high mortality rates (11). Clinically, the emergence of BM has become a new challenge for oncologists (12, 13). The prognosis of BM patients might vary based on the origin of the primary tumor and important prognostic factors, which have attracted more and more attention (14).
Various primary disease types can lead to BM (15). Studies have shown that lung cancer is the most common primary source of BM (16), and more than half of the pathological types are small cell lung cancer (SCLC) (17). Breast cancer, as the second major tissue source of BM (18), is most commonly seen in ER-negative and HER2-positive patients (19). Malignant melanoma is the third most common lesion of BM (20). Additionally, there has been an increasing trend in the incidence of BM originating from kidney and colorectal cancer in recent years (21). Clinically, the presence of BM in the brain can cause compression of brain tissue at the site of tumor formation and an increase in intracranial pressure, leading to various neurological symptoms associated with BM, such as dizziness, headache, nausea, vomiting, epileptic seizures, impaired vision, and language difficulties (22). In severe cases, it can even result in a stroke (23).
Surgery, whole-brain radiotherapy (WBRT), stereotactic radiotherapy (SRT), and chemotherapy are the main therapeutic options currently available for BM patients (24). Surgery is not the treatment of choice for most BM patients due to its high disability rate and risk of recurrence (25–27). However, it is suitable for patients with an unidentified primary tumor, single metastasis, large intracranial lesion, or those experiencing neurological symptoms caused by associated vasogenic edema and mass effect (28). In clinical practice, WBRT and stereotactic radiosurgery (SRS) are the main radiotherapy modalities, and the most basic radiotherapy method is WBRT (27). WBRT is widely used in the treatment of multiple metastases due to its significant palliative effect on tumors (25). Despite its long history, WBRT remains an important treatment option in the current stage of clinical practice (29, 30). In recent years, the combination of WBRT with targeted therapy, or SRS, has also generated substantial interest and discussions in clinical practice (31).
The classification of prognostic assessment of patients with BM has a certain guiding value for clinical management, treatment selection, and stratification of clinical trials (32). Prognostic indices play a crucial role in BM radiotherapy by providing valuable information to guide patient decision-making and clinical trial stratification (33). Over time, various classification models for prognostic assessment have evolved and developed, but there is still no unified standard scheme. These models integrate multiple prognostic factors associated with the survival of BM patients, continuously evolving and developing (34–42). In 2008, a new prognostic prediction model called the graded prognostic assessment (GPA) was proposed (38). Sperduto’s study (43) demonstrated a positive correlation between GPA scores and prognosis. Additionally, it was found that the median survival time of patients with different GPA scores (0–1, 1.5–2.5, 3, and 3.5–4.0) showed a progressive increase, and the median survival time of patients in each GPA group was significantly different. As one of the widely used classification criteria for BM patients undergoing radiotherapy in clinical practice, the GPA model is characterized by its strong quantitative nature and ease of memorization. It serves as the most standardized, objective, and user-friendly indicator for assessing the prognosis of BM patients (44). In a study conducted by Viani et al. (45), different prognostic indices were compared using a neural network-based approach, and it was found that GPA had better predictive performance for the prognosis of BM patients undergoing WBRT (with or without neurosurgery) compared to several other indices.
The purpose of this study is to investigate the effectiveness of the GPA model in predicting the prognosis of BM patients treated with WBRT and explore the relationship between GPA and patients’ prognosis. The ultimate goal is to obtain more objective and reliable findings and provide high-quality evidence-based medical evidence for the prognostic assessment of BM patients undergoing WBRT.
Materials and methods
This meta-analysis was conducted and reported according to the protocol outlined by Preferred Reporting Items for Systematic Reviews and Meta-Analyses (PRISMA) using a research question framed by PICOS methodology (46) and it was registered in the PROSPERO International Prospective Register of Systematic Reviews (CRD42023422914). Ethical approval and informed consent were not required, as this study involved secondary research based on previously published data.
Systematic literature search
Two researchers independently conducted comprehensive searches in the following databases: China National Knowledge Infrastructure (CNKI), PubMed, Wanfang database, Cochrane Library, Web of Science, Embase, and China Biomedical Literature Database (CBM). The search covered the time period from the inception of the databases to 31 December 2022. The following search terms were used in order to find the desired studies: (“metastasis OR metastases OR metastatically OR metastatics OR metastatization OR metastatize OR metastatized OR metastatizing OR secondary OR metastatic” OR “brain metastasis OR brain metastases OR metastatic brain tumor OR cerebral metastases OR Central Nervous System Metastasis OR brain neoplasms”) AND (“GPA OR Graded Prognostic Assessment”) AND (“whole brain radiotherapy OR WBRT”).
Inclusion and exclusion criteria
The inclusion criteria for the selection of appropriate studies were defined according to the PI(E)COS approach: Population: patients diagnosed with brain metastatic tumors and treated with WBRT; Exposure: GPA; Controls: patients with the lowest GPA; Outcomes: overall survival (OS) at 3, 6, 12, and 24 months after WBRT for patients with BM, with the effect size reported as hazard ratio (HR); Study design: cohort study.
The exclusion criteria were as follows: Literature such as reviews, surveys, case reports, expert opinions, conference abstracts, and animal studies; Invalid or incomplete article data; Studies with the same patient populations; Studies without a reasonable and appropriate trial design.
Literature screening and quality assessment
The retrieved literature was screened based on the inclusion and exclusion criteria. Relevant information such as authors, country, year, sex ratio, treatment method, 3-, 6-, 12-, and 24-month OS rates, and HRs were extracted and tabulated. In cases where the required data could not be directly obtained from the original text, Tierney’s method was applied to extract the necessary information (47). The process of literature screening, data extraction, and quality assessment was independently conducted by two researchers, and in case of any disagreement, consensus was reached through discussions with experts to minimize potential selection bias and information bias.
The quality assessment of the included literature was conducted using the Newcastle-Ottawa Scale (NOS), which assigned scores in eight areas, including the investigation and assessment of the exposure group. A score of ≥6 was considered indicative of good quality.
Statistical analysis
The data were analyzed using R version 4.2.2. The control group consisted of BM patients with a GPA of 0–1. To combine the HR of each outcome index and its 95% confidence interval (CI), we selected a fixed-effects model or random-effects model based on the results of the I2 test. The random-effects model was chosen when significant heterogeneity was observed among the included studies (I2 ≥ 50%), while the fixed-effects model was used when the heterogeneity was not significant (I2 < 50%). The sensitivity analysis was conducted to assess the stability of the primary combined results and to identify potential sources of bias. The assessment of potential publication bias was conducted by combining funnel plots and Egger’s test (48). An asymmetric funnel plot with a p-value of less than 0.05 was considered to indicate significant publication bias. If potential publication bias is detected, the combined results will be adjusted using the trim and filling method to analyze the impact of publication bias on the merged results.
Results
Literature search and study selection
The detailed process of literature screening is depicted in Figure 1. As shown in the flow chart, 332 duplicates were eliminated using EndNote 20.1 software and manual examination initially. Subsequently, the titles and abstracts of the remaining articles were carefully reviewed to assess their relevance to the topic, leading to the exclusion of 208 studies. Furthermore, a thorough examination of the full text allowed the exclusion of 265 studies based on the predetermined inclusion and exclusion criteria. Ultimately, there were 13 cohort studies included in our study, six of which originated from China, four from Norway, and one each from Sweden, Germany, and France.
Study characteristics
Two researchers independently conducted a detailed review and extracted basic information such as authors, countries, sex ratio, GPA distribution, and so on. The characteristics of the 13 studies, covering 1,797 participants, are summarized in Table 1.
Quality assessment of included literature
The included studies were assessed for quality using the NOS scale, and they all received scores greater than 6, indicating a relatively high level of evidence quality (Table 1).
Results of meta-analysis
Association analysis between GPA and 3-month OS after WBRT in patients with BM
A total of nine studies reported a 3-month OS rate after WBRT in patients with a GPA of 1.5–2.5, compared to those with a GPA of 0–1. There was no significant heterogeneity among them (I2 = 0.0%). The fixed-effects model was selected for the meta-analysis. As shown in Figure 2A, after WBRT, patients with a GPA of 1.5–2.5 had a significantly higher 3-month OS rate than patients in the GPA of 0–1 group (HR = 0.48; 95% CI: 0.40–0.59). Four studies provided a 3-month OS rate after WBRT in patients with a GPA of 3 compared to patients with a GPA of 0–1, and the I2 test showed no significant heterogeneity among them (I2 = 0.0%), so the fixed-effects model was used for the combined analysis. The 3-month OS rate was significantly higher in patients with a GPA of 3 than in patients with a GPA of 0–1 (HR = 0.38; 95% CI: 0.25–0.57, Figure 2B). A total of four studies presented the 3-month OS rate after WBRT in patients with a GPA of 3.5–4 vs. those with a GPA of 0–1. The heterogeneity among these four cohort studies was not significant (I2 = 0.0%). The meta-analysis results indicated that the patients with a GPA of 3.5–4 had a significantly higher 3-month OS rate after WBRT compared to the GPA 0–1 group (Figure 2C: HR = 0.28; 95% CI: 0.15–0.52).
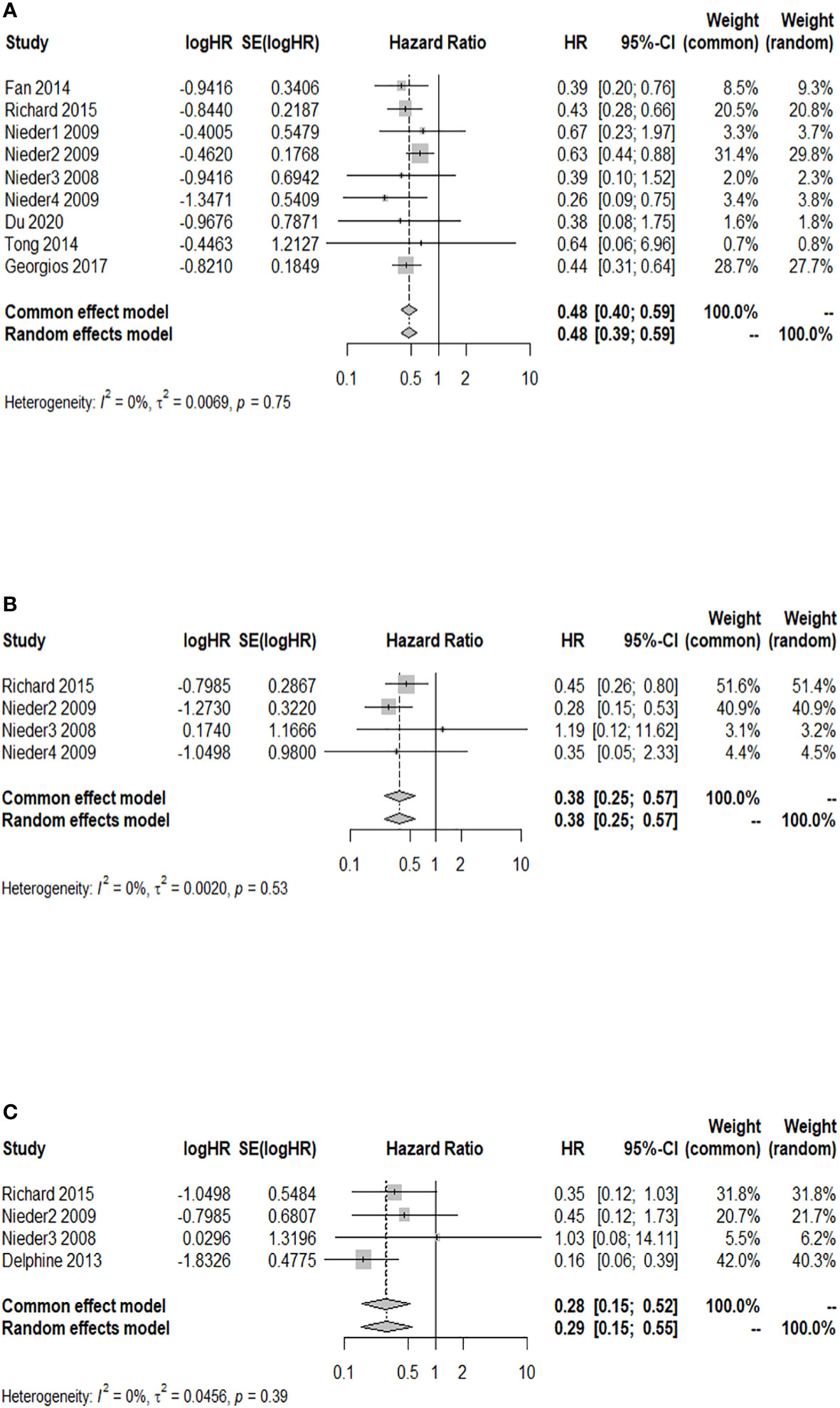
Figure 2 Forest plots of the association analysis between GPA and 3-month OS after WBRT in patients with BM. (A) Comparison of 3-month OS after WBRT in patients with BM of GPA 1.5–2.5 vs. 0–1. (B) Comparison of 3-month OS after WBRT in patients with BM of GPA 3 vs. 0–1. (C) Comparison of 3-month OS after WBRT in patients with BM of GPA 3.5–4 vs. 0–1.
Association analysis between GPA and 6-month OS after WBRT in patients with BM
A total of 11 cohort studies provided the 6-month OS rate after WBRT in patients with a GPA of 1.5–2.5 compared to a GPA of 0–1. The I2 test revealed no significant heterogeneity among the 11 studies (I2 = 0.0%), and the fixed-effects model was used for the combined analysis. Figure 3A demonstrates a significantly higher 6-month OS rate in patients with a GPA of 1.5–2.5 than in the GPA of 0–1 group after WBRT (HR = 0.48; 95% CI: 0.41–0.56). The results of sensitivity analysis showed that (the combined HR ranged from 0.47 to 0.50), which indicated consistent results with the original results, so the original results were reliable (Supplementary Figure 1). Moreover, the funnel plot distribution appeared to be generally symmetrical (Supplementary Figure 2), and Egger’s test (p = 0.595) suggested the absence of potential publication bias. Six studies reported the 6-month OS rate after WBRT in patients with a GPA of 3 compared to a GPA of 0–1. The fixed-effects model was selected for meta-analysis due to homogeneity among these 6 studies (I2 = 0.0%). The 6-month OS rate was significantly higher in patients with a GPA of 3 than in the GPA of 0–1 group after WBRT (HR = 0.33; 95% CI: 0.24–0.45, Figure 3B). Additionally, a total of five studies reported the 6-month OS rate after WBRT in patients with a GPA of 3.5–4 and GPA of 0–1 (I2 = 33%). As shown in Figure 3C, the 6-month OS rate was significantly higher in patients with a GPA of 3.5–4 than in patients with a GPA of 0–1 after WBRT (HR = 0.24; 95% CI: 0.16–0.35).
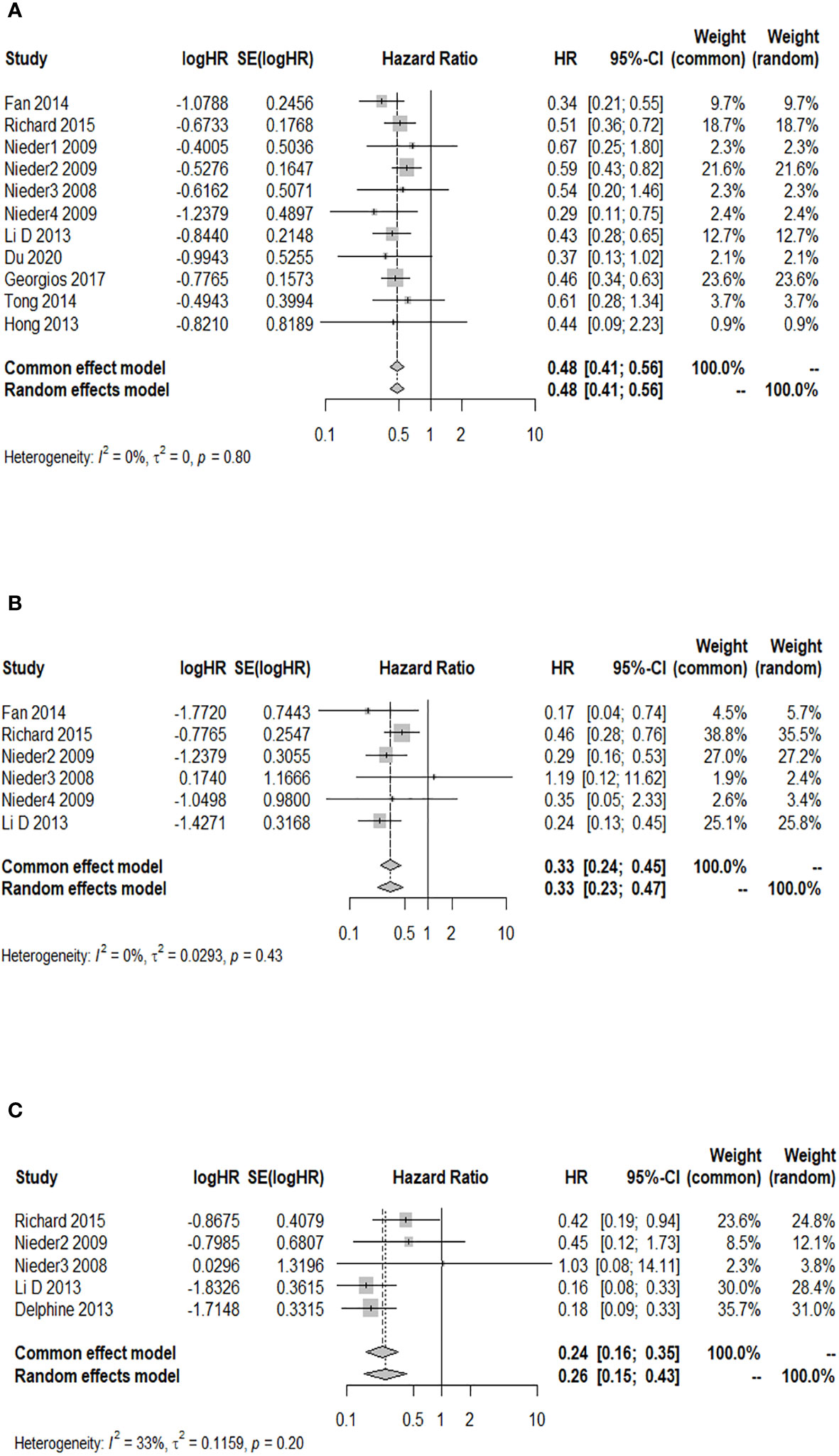
Figure 3 Forest plots of the association analysis between GPA and 6-month OS after WBRT in patients with BM. (A) Comparison of 6-month OS after WBRT in patients with BM of GPA 1.5–2.5 vs. 0–1. (B) Comparison of 6-month OS after WBRT in patients with BM of GPA 3 vs. 0–1. (C) Comparison of 6-month OS after WBRT in patients with BM of GPA 3.5–4 vs. 0–1.
Association analysis between GPA and 12-month OS after WBRT in patients with BM
A total of six studies provided the 12-month OS rate for patients with a GPA of 1.5–2.5 compared to a GPA of 0–1. There was no significant heterogeneity among these studies (p = 0.916, I2 = 0.0%), and the fixed-effects model was used for meta-analysis. Figure 4A illustrates that patients with a GPA of 1.5–2.5 had a significantly higher 12-month OS rate after WBRT than patients with GPA of 0–1 (HR = 0.49; 95% CI: 0.41–0.58). Two studies reported the 12-month OS rate after WBRT in patients with a GPA of 3.0 compared to a GPA of 0–1, and no significant heterogeneity was found (p = 0.714, I2 = 0.0%). The fixed-effects model was selected for the combined analysis. Figure 4B demonstrates that patients with a GPA of 3.0 had a significantly higher 12-month OS rate than those with a GPA of 0–1 (HR = 0.48; 95% CI: 0.32–0.73) after WBRT. Two articles provided the 12-month OS rate after WBRT in BM patients with a GPA of 3.5–4 vs. a GPA of 0-1, showing heterogeneity between the studies (p = 0.022, I2 = 80.9%). A random-effects model was used for the meta-analysis, as shown in Figure 4C. It revealed that patients with a GPA of 3.5–4 had a significantly higher 12-month OS rate after WBRT than patients with a GPA of 0–1 (HR = 0.31; 95% CI: 0.12–0.79).
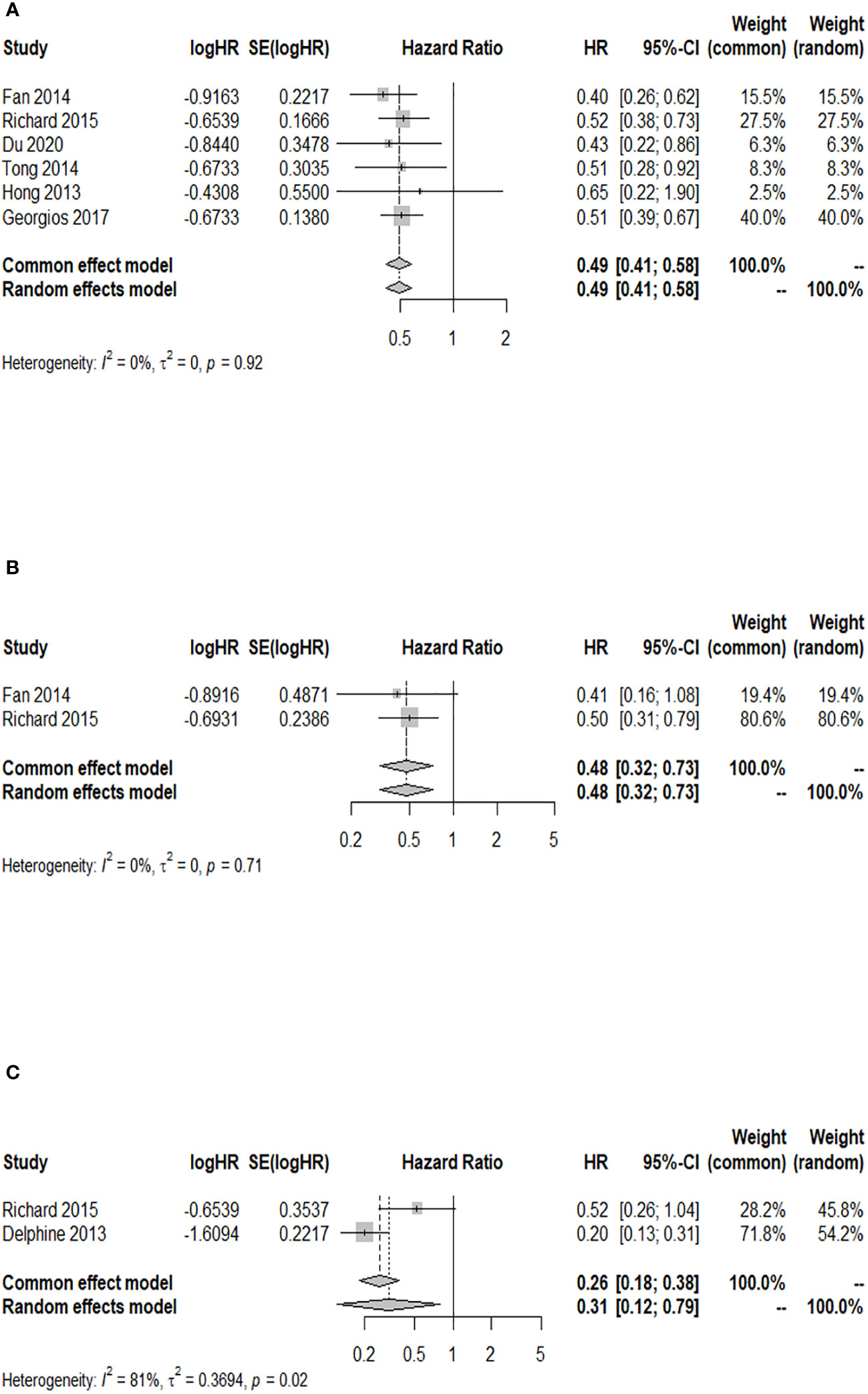
Figure 4 Forest plots of the association analysis between GPA and 12-month OS after WBRT in patients with BM. (A) Comparison of 12-month OS after WBRT in patients with BM of GPA 1.5–2.5 vs. 0–1. (B) Comparison of 12-month OS after WBRT in patients with BM of GPA 3 vs. 0–1. (C) Comparison of 12-month OS after WBRT in patients with BM of GPA 3.5–4 vs. 0–1.
Association analysis between GPA and 24-month OS after WBRT in patients with BM
Among the cohort studies included in the analysis, five studies reported the 24-month OS rate after WBRT in BM patients with a GPA of 1.5–2.5 compared to those with a GPA of 0–1, and there was no heterogeneity detected among the included studies (I2 = 0.0%). The results indicated a significant increase in the 24-month OS rate of BM patients with a GPA of 1.5–2.5 compared to those with a GPA of 0–1 after WBRT (HR = 0.49; 95% CI: 0.42–0.58, Figure 5A). Two of the included cohort studies reported the 24-month OS rate after WBRT in BM patients with a GPA of 3.0 compared to those with a GPA of 0–1. No heterogeneity was observed between these two studies (I2 = 0.0%), so a fixed-effects model was used. The 24-month OS rate for BM patients with a GPA of 3.0 was higher than that for BM patients with a GPA of 0–1 after WBRT, and the difference was statistically significant (HR = 0.49; 95% CI: 0.32–0.74, Figure 5B). Three of the included cohort studies reported the 24-month OS after WBRT in patients with a GPA of 3.5–4 compared to those with a GPA of 0–1, and heterogeneity was identified between these two studies (p = 0.092, I2 = 58.1%), so a random-effects model was chosen for meta-analysis. The results of the meta-analysis showed that at 24 months after WBRT, the OS rate for BM patients with a GPA of 3.5–4 was higher than that of patients with a GPA of 0–1, and the difference was statistically significant (HR = 0.38; 95% CI: 0.15–0.94, Figure 5C).
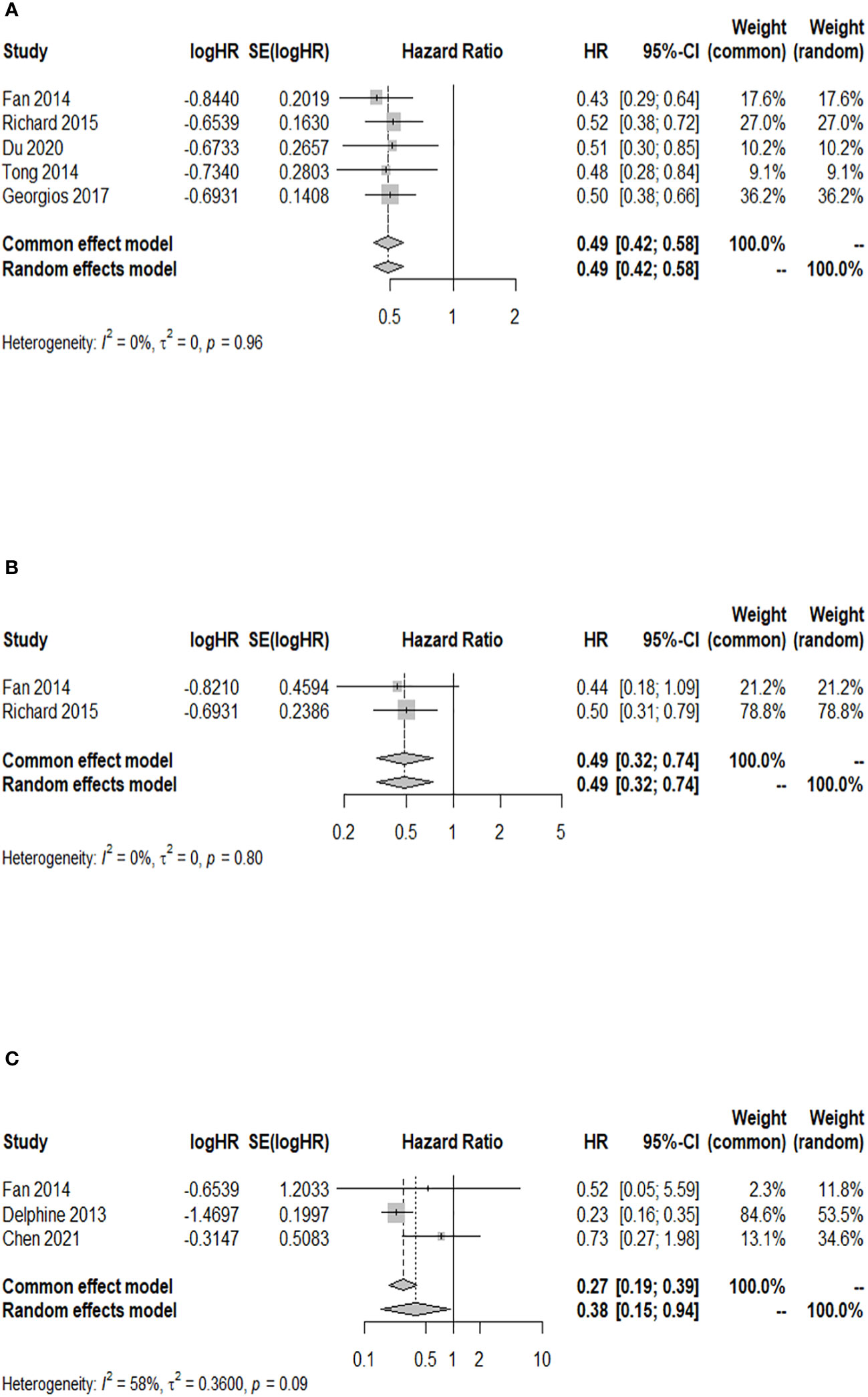
Figure 5 Forest plots of the association analysis between GPA and 24-month OS after WBRT in patients with BM. (A) Comparison of 24-month OS after WBRT in patients with BM of GPA 1.5–2.5 vs. 0–1. (B) Comparison of 24-month OS after WBRT in patients with BM of GPA 3 vs. 0–1. (C) Comparison of 24-month OS after WBRT in patients with BM of GPA 3.5–4 vs. 0–1.
Discussion
The clinical implications of GPA in predicting the prognosis of BM patients after WBRT
The relevant study (33) has demonstrated that utilizing prognostic indices for BM can help identify favorable prognostic groups that may benefit from more intensified therapies while avoiding overtreatment in other groups. This approach facilitates the customization of treatment plans based on individual prognostic factors, thereby optimizing patient outcomes. In our study, we compared the OS rates at different time points after WBRT among patients with BM. We found that a higher GPA score was associated with a better prognosis.
We believe that our findings have the following clinical implications: The application prospects of the GPA model in personalized and precise treatment of BM are promising. By considering various factors such as age, Karnofsky Performance Status (KPS), number of BMs, and the presence of extracranial metastases, the GPA model can assist clinicians in evaluating the prognosis and survival outcomes of individual patients. Furthermore, it helps predict disease progression and survival time for BM patients.
This valuable information allows clinicians to stratify BM patients into different risk groups, enabling tailored treatment options and management strategies based on each patient’s specific needs. As a result, treatment outcomes and quality of life can potentially be improved. Additionally, regular monitoring of the GPA index allows clinicians to assess the effectiveness of treatment and track changes in the patient’s condition. This monitoring facilitates timely adjustments to the treatment plan, leading to better treatment outcomes.
Practicability and limitations of WBRT and GPA model
Upon reviewing the relevant literature, it is apparent that there is limited research on the application of WBRT in the treatment of BM. In contrast, there has been a significant increase in studies investigating alternative treatments of BM such as SRS, targeted pharmaceutical interventions, and immunotherapy (62–67). Moreover, it remains unclear whether WBRT can improve the survival rate of patients (68). On the other hand, SRS not only enhances patients’ quality of life but also reduces the rate of cognitive decline (30). Additionally, targeted therapies have shown good efficacy with a relatively low incidence of side effects (69). The GPA model has demonstrated its effectiveness in predicting the prognosis of BM patients treated with WBRT or surgery, as confirmed by numerous clinical trials (38, 70–72). However, some clinical studies have indicated that the GPA model struggles to predict the changes in physical function and postoperative complications in BM patients treated with surgery. Moreover, the health status of the patient’s body and BM often fluctuate during the treatment process. Consequently, using a GPA model alone may not effectively predict the short-term prognosis of these patients. Evaluating the risks and benefits of surgical treatment for BM using the GPA model alone has yielded unsatisfactory results (71). This meta-analysis found that patients with BM who had a high GPA demonstrated significantly higher OS and a better prognostic status at the same time point. However, the available survival data at certain time points in this study were limited, and further investigation is needed to determine the value of GPA in evaluating the long-term prognostic survival of BM patients treated with WBRT.
Comparison of commonly used prognostic assessment models
In clinical practice, it is crucial to select appropriate prognostic assessment models to prevent the abandonment of treatment due to inaccurate predictions (73). When comparing the more commonly applied prognostic assessment models of GPA, Recursive Partition Analysis (RPA), the Score Index for Radiosurgery in Brain Metastases (SIR), and the Basic Score for Brain Metastases (BSBM), it can be seen that all of these systems incorporate two factors: the Karnofsky score and extracranial metastatic status. Additionally, the control status of the primary tumor is considered in RPA, SIR, and BSBM (34, 36, 37). However, there is a certain subjectivity in the clinical evaluation of whether the primary tumor is controlled. Neil’s study compared the clinical utility of RPA and GPA in predicting the moderate prognosis of BM patients (74). The study revealed that RPA encountered difficulties in further stratifying patients’ prognoses, while GPA overcame this limitation by providing clinicians with a more refined scope for subjective assessment when selecting treatment plans. This could potentially explain why GPA was deemed more beneficial in clinical practice (74). It is worth noting that RPA and BSBM do not include the item of BM status, including both the number and volume, which can significantly influence patients’ prognostic survival time. The RPA, being the earliest prognostic scoring system utilized, has limited applicability in clinical practice due to the imbalanced distribution of patients across its classification levels (75). Moreover, the BSBM does not take into account the status of brain disease, specifically the absence of tumor number and tumor volume, as factors in its assessment (76). The SIR model is rarely used in clinical practice because it incorporates the volume of the largest lesion, which is typically estimated at the time of SRS (77). However, in reality, patients often do not have precise knowledge of the true volume of their lesions when selecting treatment methods. This limitation reduces the practicality of the SIR model. In comparison, the GPA model offers advantages in terms of objectivity, quantification, and practicality of prediction. Looking ahead, it is hoped that there will be simpler and more accurate models for predicting the prognosis of patients with BM to provide more beneficial treatment plans for precise clinical diagnosis and treatment.
Heterogeneity analysis
The heterogeneity observed in this study may be attributed to the individualized treatment principles applied to patients with BM, resulting in variations in treatment approaches. Clinical trials have shown that patients treated with WBRT combined with other therapies had better prognostic survival than those who used WBRT alone (78). Furthermore, the prognosis of patients with BM often varies depending on the location of the primary tumor. Moreover, some studies provided limited survival data for patients, making it impossible to derive meaningful HRs. As a result, only a small number of eligible studies were included in the meta-analysis, leading to potential heterogeneity.
Strengths and limitations of this study
There are some strengths in this meta-analysis: Firstly, the included patients were clearly diagnosed with BM, and their treatment methods were limited to WBRT. Our prognosis survival prediction of patients with BM by GPA was more accurate and reliable because the limitations of treatment minimized their influence on prognosis as a confounding factor. Secondly, the study encompassed a comprehensive range of original literature from multiple databases, which effectively mitigated selection bias, enhancing the robustness of the findings. In our study, we included a total of 1,797 patients with BM, and the evaluation results of the NOS scale indicated that the quality of the included studies was high, thus ensuring the reliability of our findings. Thirdly, there was minimal heterogeneity among the included studies, except for one specific individual outcome (the 12- and 24-month OS rate for patients with BM in GPA 3.5–4 and 0–1 groups). The observed heterogeneity in these studies was probably due to the relatively small number of eligible studies at these two time points. Moreover, the funnel plot and Egger’s test revealed little potential publication bias among the included studies. The results of the sensitivity analysis confirmed the robustness and reliability of the original conclusions, ensuring the validity of the analysis.
However, this study may have the following limitations: First, all 13 included studies were retrospective cohort studies, and the information bias might be due to the fact that patients with BM had deviations in the memory of their own conditions. Additionally, the samples of the included studies were sourced from various countries in Europe and Asia, which inevitably caused regional limitations. The differences in regional, cultural, and environmental factors among different countries can lead to variations in research results. Therefore, the regional limitations may introduce biases in the representativeness of our research samples and impact the external validity of the findings. Moreover, the number of patients meeting our requirements for individual outcome indicators was limited, and some individual research studies did not provide sufficient valid data.
Conclusion
The prognosis of BM patients with high GPAs after WBRT was generally better than that of patients with low GPAs. The GPA model can be utilized to predict the prognosis of patients with BM after WBRT.
Data availability statement
The datasets presented in this study can be found in online repositories. The names of the repository/repositories and accession number(s) can be found in the article/Supplementary Material.
Author contributions
XG: Data curation, Formal analysis, Investigation, Methodology, Writing – original draft. CK: Conceptualization, Supervision, Validation, Writing – review & editing. JW: Conceptualization, Supervision, Validation, Writing – review & editing.
Funding
The author(s) declare that no financial support was received for the research, authorship, and/or publication of this article.
Conflict of interest
The authors declare that the research was conducted in the absence of any commercial or financial relationships that could be construed as a potential conflict of interest.
Publisher’s note
All claims expressed in this article are solely those of the authors and do not necessarily represent those of their affiliated organizations, or those of the publisher, the editors and the reviewers. Any product that may be evaluated in this article, or claim that may be made by its manufacturer, is not guaranteed or endorsed by the publisher.
Supplementary material
The Supplementary Material for this article can be found online at: https://www.frontiersin.org/articles/10.3389/fonc.2023.1322262/full#supplementary-material
References
1. Hao P, Chen L, Ge Y, You Y, Yan G, Jin ZW. Time taken for a primary tumor to metastasize to the brain and the overall survival of patients with brain metastasis: An analysis of outcomes and factors affecting survival. Technol Health Care (2022) 30:259–70. doi: 10.3233/thc-228025
2. Verhaak E, Gehring K, Hanssens PEJ, Aaronson NK, Sitskoorn MM. Health-related quality of life in adult patients with brain metastases after stereotactic radiosurgery: a systematic, narrative review. Support Care Cancer. (2020) 28:473–84. doi: 10.1007/s00520-019-05136-x
3. Cao J, Yang YP, Wang G. Progress in radiotherapy for brain metastases. J Modern Oncol (2020) 28:5. doi: 10.3969/j.issn.1672-4992.2020.11.041
4. Davis FG, Dolecek TA, McCarthy BJ, Villano JL. Toward determining the lifetime occurrence of metastatic brain tumors estimated from 2007 United States cancer incidence data. Neuro Oncol (2012) 14:1171–7. doi: 10.1093/neuonc/nos152
5. Tabouret E, Bauchet L, Carpentier AF. [Brain metastases epidemiology and biology]. Bull Cancer. (2013) 100:57–62. doi: 10.1684/bdc.2012.1681
6. Soffietti R, Rudà R, Trevisan E. Brain metastases: current management and new developments. Curr Opin Oncol (2008) 20:676–84. doi: 10.1097/CCO.0b013e32831186fe
7. Nayak L, Lee EQ, Wen PY. Epidemiology of brain metastases. Curr Oncol Rep (2012) 14:48–54. doi: 10.1007/s11912-011-0203-y
8. Hanssens P, Karlsson B, Yeo TT, Chou N, Beute G. Detection of brain micrometastases by high-resolution stereotactic magnetic resonance imaging and its impact on the timing of and risk for distant recurrences. J Neurosurg (2011) 115:499–504. doi: 10.3171/2011.4.Jns101832
9. Gerosa M, Nicolato A, Foroni R. The role of gamma knife radiosurgery in the treatment of primary and metastatic brain tumors. Curr Opin Oncol (2003) 15:188–96. doi: 10.1097/00001622-200305000-00002
10. Arvold ND, Lee EQ, Mehta MP, Margolin K, Alexander BM, Lin NU, et al. Updates in the management of brain metastases. Neuro Oncol (2016) 18:1043–65. doi: 10.1093/neuonc/now127
11. Yuan JZ. Analysis efficacy and safety of whole brain radiotherapy combined with temozolomide chemotherapy in treatment of brain metastases. China Foreign Med Treat (2016) 35:115–7. doi: 10.16662/j.cnki.1674-0742.2016.28.115
12. Franchino F, Rudà R, Soffietti R. Mechanisms and therapy for cancer metastasis to the brain. Front Oncol (2018) 8:161. doi: 10.3389/fonc.2018.00161
13. Boire A, Brastianos PK, Garzia L, Valiente M. Brain metastasis. Nat Rev Cancer. (2020) 20:4–11. doi: 10.1038/s41568-019-0220-y
14. Valiente M, Ahluwalia MS, Boire A, Brastianos PK, Goldberg SB, Lee EQ, et al. The evolving landscape of brain metastasis. Trends Cancer (2018) 4:176–96. doi: 10.1016/j.trecan.2018.01.003
15. Kaal EC, Niël CG, Vecht CJ. Therapeutic management of brain metastasis. Lancet Neurol (2005) 4:289–98. doi: 10.1016/s1474-4422(05)70072-7
16. Nieder C, Haukland E, Mannsåker B, Pawinski AR, Yobuta R, Dalhaug A. Presence of brain metastases at initial diagnosis of cancer: patient characteristics and outcome. Cureus (2019) 11:e4113. doi: 10.7759/cureus.4113
17. Rudin CM, Brambilla E, Faivre-Finn C, Sage J. Small-cell lung cancer. Nat Rev Dis Primers. (2021) 7:3. doi: 10.1038/s41572-020-00235-0
18. Fecci PE, Champion CD, Hoj J, McKernan CM, Goodwin CR, Kirkpatrick JP, et al. The evolving modern management of brain metastasis. Clin Cancer Res (2019) 25:6570–80. doi: 10.1158/1078-0432.Ccr-18-1624
19. Bailleux C, Eberst L, Bachelot T. Treatment strategies for breast cancer brain metastases. Br J Cancer. (2021) 124:142–55. doi: 10.1038/s41416-020-01175-y
20. Bottoni U, Clerico R, Paolino G, Ambrifi M, Corsetti P, Calvieri S. Predictors and survival in patients with melanoma brain metastases. Med Oncol (2013) 30:466. doi: 10.1007/s12032-013-0466-2
21. Berghoff AS, Preusser M. Anti-angiogenic therapies in brain metastases. Memo (2018) 11:14–7. doi: 10.1007/s12254-018-0384-2
22. Hosonaga M, Saya H, Arima Y. Molecular and cellular mechanisms underlying brain metastasis of breast cancer. Cancer Metastasis Rev (2020) 39:711–20. doi: 10.1007/s10555-020-09881-y
23. Hubbs JL, Boyd JA, Hollis D, Chino JP, Saynak M, Kelsey CR. Factors associated with the development of brain metastases: analysis of 975 patients with early stage nonsmall cell lung cancer. Cancer (2010) 116:5038–46. doi: 10.1002/cncr.25254
24. Soffietti R, Ahluwalia M, Lin N, Rudà R. Management of brain metastases according to molecular subtypes. Nat Rev Neurol (2020) 16:557–74. doi: 10.1038/s41582-020-0391-x
25. Brown PD, Ballman KV, Cerhan JH, Anderson SK, Carrero XW, Whitton AC, et al. Postoperative stereotactic radiosurgery compared with whole brain radiotherapy for resected metastatic brain disease (NCCTG N107C/CEC·3): a multicentre, randomised, controlled, phase 3 trial. Lancet Oncol (2017) 18:1049–60. doi: 10.1016/s1470-2045(17)30441-2
26. Patchell RA, Tibbs PA, Regine WF, Dempsey RJ, Mohiuddin M, Kryscio RJ, et al. Postoperative radiotherapy in the treatment of single metastases to the brain: a randomized trial. Jama (1998) 280:1485–9. doi: 10.1001/jama.280.17.1485
27. Mahajan A, Ahmed S, McAleer MF, Weinberg JS, Li J, Brown P, et al. Post-operative stereotactic radiosurgery versus observation for completely resected brain metastases: a single-centre, randomised, controlled, phase 3 trial. Lancet Oncol (2017) 18:1040–8. doi: 10.1016/s1470-2045(17)30414-x
28. Kotecha R, Ahluwalia MS, Siomin V, McDermott MW. Surgery, stereotactic radiosurgery, and systemic therapy in the management of operable brain metastasis. Neurol Clin (2022) 40:421–36. doi: 10.1016/j.ncl.2021.11.002
29. Crockett C, Belderbos J, Levy A, McDonald F, Le Péchoux C, Faivre-Finn C. Prophylactic cranial irradiation (PCI), hippocampal avoidance (HA) whole brain radiotherapy (WBRT) and stereotactic radiosurgery (SRS) in small cell lung cancer (SCLC): Where do we stand? Lung Cancer (2021) 162:96–105. doi: 10.1016/j.lungcan.2021.10.016
30. Kotecha R, Gondi V, Ahluwalia MS, Brastianos PK, Mehta MP. Recent advances in managing brain metastasis. F1000Res (2018) 7:1772. doi: 10.12688/f1000research.15903.1
31. Soffietti R, Abacioglu U, Baumert B, Combs SE, Kinhult S, Kros JM, et al. Diagnosis and treatment of brain metastases from solid tumors: guidelines from the European Association of Neuro-Oncology (EANO). Neuro Oncol (2017) 19:162–74. doi: 10.1093/neuonc/now241
32. Rodrigues G, Bauman G, Palma D, Louie AV, Mocanu J, Senan S, et al. Systematic review of brain metastases prognostic indices. Pract Radiat Oncol (2013) 3:101–6. doi: 10.1016/j.prro.2012.04.001
33. Rodrigues G, Gonzalez-Maldonado S, Bauman G, Senan S, Lagerwaard F. A statistical comparison of prognostic index systems for brain metastases after stereotactic radiosurgery or fractionated stereotactic radiation therapy. Clin Oncol (R Coll Radiol). (2013) 25:227–35. doi: 10.1016/j.clon.2012.11.006
34. Gaspar L, Scott C, Rotman M, Asbell S, Phillips T, Wasserman T, et al. Recursive partitioning analysis (RPA) of prognostic factors in three Radiation Therapy Oncology Group (RTOG) brain metastases trials. Int J Radiat Oncol Biol Phys (1997) 37:745–51. doi: 10.1016/s0360-3016(96)00619-0
35. Lagerwaard FJ, Levendag PC, Nowak PJ, Eijkenboom WM, Hanssens PE, Schmitz PI. Identification of prognostic factors in patients with brain metastases: a review of 1292 patients. Int J Radiat Oncol Biol Phys (1999) 43:795–803. doi: 10.1016/s0360-3016(98)00442-8
36. Weltman E, Salvajoli JV, Brandt RA, de Morais Hanriot R, Prisco FE, Cruz JC, et al. Radiosurgery for brain metastases: a score index for predicting prognosis. Int J Radiat Oncol Biol Phys (2000) 46:1155–61. doi: 10.1016/s0360-3016(99)00549-0
37. Lorenzoni J, Devriendt D, Massager N, David P, Ruíz S, Vanderlinden B, et al. Radiosurgery for treatment of brain metastases: estimation of patient eligibility using three stratification systems. Int J Radiat Oncol Biol Phys (2004) 60:218–24. doi: 10.1016/j.ijrobp.2004.02.017
38. Sperduto PW, Berkey B, Gaspar LE, Mehta M, Curran W. A new prognostic index and comparison to three other indices for patients with brain metastases: an analysis of 1,960 patients in the RTOG database. Int J Radiat Oncol Biol Phys (2008) 70:510–4. doi: 10.1016/j.ijrobp.2007.06.074
39. Rades D, Dunst J, Schild SE. A new scoring system to predicting the survival of patients treated with whole-brain radiotherapy for brain metastases. Strahlenther Onkol. (2008) 184:251–5. doi: 10.1007/s00066-008-1831-5
40. Struffert T, Roth C, Romeike B, Grunwald IO, Reith W. Onyx in an experimental aneurysm model: histological and angiographic results. J Neurosurg (2008) 109:77–82. doi: 10.3171/jns/2008/109/7/0077
41. Sperduto PW, Kased N, Roberge D, Xu Z, Shanley R, Luo X, et al. Summary report on the graded prognostic assessment: an accurate and facile diagnosis-specific tool to estimate survival for patients with brain metastases. J Clin Oncol (2012) 30:419–25. doi: 10.1200/jco.2011.38.0527
42. Rades D, Dziggel L, Haatanen T, Veninga T, Lohynska R, Dunst J, et al. Scoring systems to estimate intracerebral control and survival rates of patients irradiated for brain metastases. Int J Radiat Oncol Biol Phys (2011) 80:1122–7. doi: 10.1016/j.ijrobp.2010.03.031
43. Sperduto CM, Watanabe Y, Mullan J, Hood T, Dyste G, Watts C, et al. A validation study of a new prognostic index for patients with brain metastases: the Graded Prognostic Assessment. J Neurosurg (2008) 109 Suppl:87–9. doi: 10.3171/jns/2008/109/12/s14
44. Ribeiro LM, Bomtempo FF, Rocha RB, Telles JPM, Neto EB, Figueiredo EG. Development and adaptations of the Graded Prognostic Assessment (GPA) scale: a systematic review. Clin Exp Metastasis. (2023) 40:445–63. doi: 10.1007/s10585-023-10237-3
45. Viani GA, da Silva LG, Stefano EJ. Prognostic indexes for brain metastases: which is the most powerful? Int J Radiat Oncol Biol Phys (2012) 83:e325–30. doi: 10.1016/j.ijrobp.2011.12.082
46. Shamseer L, Moher D, Clarke M, Ghersi D, Liberati A, Petticrew M, et al. Preferred reporting items for systematic review and meta-analysis protocols (PRISMA-P) 2015: elaboration and explanation. BMJ (2015) 350:g7647. doi: 10.1136/bmj.g7647
47. Tierney JF, Stewart LA, Ghersi D, Burdett S, Sydes MR. Practical methods for incorporating summary time-to-event data into meta-analysis. Trials (2007) 8:16. doi: 10.1186/1745-6215-8-16
48. Egger M, Davey Smith G, Schneider M, Minder C. Bias in meta-analysis detected by a simple, graphical test. Bmj (1997) 315:629–34. doi: 10.1136/bmj.315.7109.629
49. Fan Y. A study on the efficacy and treatment model of EGFR-TKIs for non-small cell lung cancer with brain metastases [dissertation]. Wuhan: Wuhan University (2014).
50. Li DJ, Duan SM, Li HW, Zhang YJ, Peng KG, Jiang H, et al. Clinical analysis of 186 cases of brain metastases treated with radiotherapy. Chin J Gerontology. (2013) 33:937–8. doi: 10.3969/j.issn.1005-9202.2013.04.097
51. Nieder C, Pawiniski A, Dalhaug A. Presentation and outcome in cancer patients with extensive spread to the brain. BMC Res Notes. (2009) 2:247. doi: 10.1186/1756-0500-2-247
52. Partl R, Fastner G, Kaiser J, Kronhuber E, Cetin-Strohmer K, Steffal C, et al. KPS/LDH index: a simple tool for identifying patients with metastatic melanoma who are unlikely to benefit from palliative whole brain radiotherapy. Support Care Cancer. (2016) 24:523–8. doi: 10.1007/s00520-015-2793-7
53. Tsakonas G, Hellman F, Gubanski M, Friesland S, Tendler S, Lewensohn R, et al. Prognostic factors affecting survival after whole brain radiotherapy in patients with brain metastasized lung cancer. Acta Oncol (2018) 57:231–8. doi: 10.1080/0284186x.2017.1386799
54. Nieder C, Bremnes RM, Andratschke NH. Prognostic scores in patients with brain metastases from non-small cell lung cancer. J Thorac Oncol (2009) 4:1337–41. doi: 10.1097/JTO.0b013e3181b6b6f4
55. Hong FR. Analysis on the effects and prognostic of simultaneous integrated boost with intensity-modulated radiation therapy in lung cancer with brain metastases [master's thesis]. Fujian: Fujian Med University (2014).
56. Tong HG, Xie CY, Lin QB. Application of prognostic index graded prognostic assessment (GPA) in lung cancer with brain metastases. J Chin Oncol (2014) 20:221–5. doi: 10.11735/j.issn.1671-170X.2014.03.B011
57. Nieder C, Geinitz H, Molls M. Validation of the graded prognostic assessment index for surgically treated patients with brain metastases. Anticancer Res (2008) 28:3015–7.
58. Chen GY, Cheng JC, Chen YF, Yang JC, Hsu FM. Circulating exosomal integrin β3 is associated with intracranial failure and survival in lung cancer patients receiving cranial irradiation for brain metastases: A prospective observational study. Cancers (Basel) (2021) 13:380. doi: 10.3390/cancers13030380
59. Antoni D, Clavier JB, Pop M, Schumacher C, Lefebvre F, Noël G. Institutional, retrospective analysis of 777 patients with brain metastases: treatment outcomes and diagnosis-specific prognostic factors. Int J Radiat Oncol Biol Phys (2013) 86:630–7. doi: 10.1016/j.ijrobp.2013.02.027
60. Nieder C, Pawinski A, Balteskard L. Colorectal cancer metastatic to the brain: time trends in presentation and outcome. Oncology (2009) 76:369–74. doi: 10.1159/000210026
61. Du TQ. Prognostic significance of whole brain radiotherapy, whole brain radiotherapy with consecutive boost, and simultaneous integrated boost in brain metastases of lung cancer [master's thesis]. Shenyang: China Med University (2020).
62. Cho A, Untersteiner H, Fitschek F, Khalaveh F, Pruckner P, Pavo N, et al. The clinical relevance of laboratory prognostic scores for patients with radiosurgically treated brain metastases of non-pulmonary primary tumor. J Neurooncol. (2021) 153:497–505. doi: 10.1007/s11060-021-03788-6
63. Cabanie C, Biau J, Durando X, Mansard S, Molnar I, Chassin V, et al. Toxicity and time lapse between immunotherapy and stereotactic radiotherapy of brain metastases. Cancer Radiother. (2021) 25:432–40. doi: 10.1016/j.canrad.2021.01.007
64. Wang X, Haaland B, Hu-Lieskovan S, Colman H, Holmen SL. First line immunotherapy extends brain metastasis free survival, improves overall survival, and reduces the incidence of brain metastasis in patients with advanced melanoma. Cancer Rep (Hoboken). (2021) 4:e1419. doi: 10.1002/cnr2.1419
65. Gore ME, Hariharan S, Porta C, Bracarda S, Hawkins R, Bjarnason GA, et al. Sunitinib in metastatic renal cell carcinoma patients with brain metastases. Cancer (2011) 117:501–9. doi: 10.1002/cncr.25452
66. Margolin K, Ernstoff MS, Hamid O, Lawrence D, McDermott D, Puzanov I, et al. Ipilimumab in patients with melanoma and brain metastases: an open-label, phase 2 trial. Lancet Oncol (2012) 13:459–65. doi: 10.1016/s1470-2045(12)70090-6
67. Massard C, Zonierek J, Gross-Goupil M, Fizazi K, Szczylik C, Escudier B. Incidence of brain metastases in renal cell carcinoma treated with sorafenib. Ann Oncol (2010) 21:1027–31. doi: 10.1093/annonc/mdp411
68. Thiel E, Korfel A, Martus P, Kanz L, Griesinger F, Rauch M, et al. High-dose methotrexate with or without whole brain radiotherapy for primary CNS lymphoma (G-PCNSL-SG-1): a phase 3, randomised, non-inferiority trial. Lancet Oncol (2010) 11:1036–47. doi: 10.1016/s1470-2045(10)70229-1
69. Huang X, Zhang JY, Wang SF. Study on the clinical effect of targeted therapy combined with radiotherapy and chemotherapy on lung cancer patients with brain metastasis. Pract J Cancer (2020) 35:967–70. doi: 10.3969/j.issn.1001-5930.2020.06.027
70. Nieder C, Marienhagen K, Geinitz H, Molls M. Validation of the graded prognostic assessment index for patients with brain metastases. Acta Oncol (2009) 48:457–9. doi: 10.1080/02841860802342390
71. Jakola AS, Gulati S, Nerland US, Solheim O. Surgical resection of brain metastases: the prognostic value of the graded prognostic assessment score. J Neurooncol. (2011) 105:573–81. doi: 10.1007/s11060-011-0623-4
72. Golden DW, Lamborn KR, McDermott MW, Kunwar S, Wara WM, Nakamura JL, et al. Prognostic factors and grading systems for overall survival in patients treated with radiosurgery for brain metastases: variation by primary site. J Neurosurg (2008) 109 Suppl:77–86. doi: 10.3171/jns/2008/109/12/s13
73. Nieder C, Mehta MP. Prognostic indices for brain metastases–usefulness and challenges. Radiat Oncol (2009) 4:10. doi: 10.1186/1748-717x-4-10
74. Estabrook NC, Lutz ST, Johnson CS, Lo SS, Henderson MA. Does Graded Prognostic Assessment outperform Recursive Partitioning Analysis in patients with moderate prognosis brain metastases? CNS Oncol (2016) 5:69–76. doi: 10.2217/cns.15.45
75. Zindler JD, Rodrigues G, Haasbeek CJ, De Haan PF, Meijer OW, Slotman BJ, et al. The clinical utility of prognostic scoring systems in patients with brain metastases treated with radiosurgery. Radiother Oncol (2013) 106:370–4. doi: 10.1016/j.radonc.2013.01.015
76. Serizawa T, Higuchi Y, Nagano O, Hirai T, Ono J, Saeki N, et al. Testing different brain metastasis grading systems in stereotactic radiosurgery: Radiation Therapy Oncology Group's RPA, SIR, BSBM, GPA, and modified RPA. J Neurosurg (2012) 117 Suppl:31–7. doi: 10.3171/2012.8.Gks12710
77. Yamamoto M, Kawabe T, Higuchi Y, Sato Y, Nariai T, Watanabe S, et al. Validity of prognostic grading indices for brain metastasis patients undergoing repeat radiosurgery. World Neurosurg (2014) 82:1242–9. doi: 10.1016/j.wneu.2014.08.008
78. Buchsbaum JC, Suh JH, Lee SY, Chidel MA, Greskovich JF, Barnett GH. Survival by radiation therapy oncology group recursive partitioning analysis class and treatment modality in patients with brain metastases from Malignant melanoma: a retrospective study. Cancer (2002) 94:2265–72. doi: 10.1002/cncr.10426
Keywords: brain metastasis, whole brain radiotherapy, graded prognostic assessment, prognosis, meta-analysis
Citation: Geng X, Kou C and Wang J (2024) The association between graded prognostic assessment and the prognosis of brain metastases after whole brain radiotherapy: a meta-analysis. Front. Oncol. 13:1322262. doi: 10.3389/fonc.2023.1322262
Received: 16 October 2023; Accepted: 11 December 2023;
Published: 09 January 2024.
Edited by:
Manuela Caroli, IRCCS Ca ‘Granda Foundation Maggiore Policlinico Hospital, ItalyReviewed by:
Raees Tonse, Baptist Hospital of Miami, United StatesChunzhi Zhang, Tianjin Hospital, China
Copyright © 2024 Geng, Kou and Wang. This is an open-access article distributed under the terms of the Creative Commons Attribution License (CC BY). The use, distribution or reproduction in other forums is permitted, provided the original author(s) and the copyright owner(s) are credited and that the original publication in this journal is cited, in accordance with accepted academic practice. No use, distribution or reproduction is permitted which does not comply with these terms.
*Correspondence: Changgui Kou, a2NnbmJ1bXNAMTYzLmNvbQ==; Jianfeng Wang, amZ3YW5nQGpsdS5lZHUuY24=