- 1Engineering Research Center for New Materials and Precision Treatment Technology of Malignant Tumors Therapy, The Second Affiliated Hospital of Dalian Medical University, Dalian, China
- 2Engineering Technology Research Center for Translational Medicine, The Second Affiliated Hospital of Dalian Medical University, Dalian, China
- 3Division of Hepatobiliary and Pancreatic Surgery, Department of General Surgery, The Second Affiliated Hospital of Dalian Medical University, Dalian, China
- 4Department of Neurosurgery, The Second Affiliated Hospital of Dalian Medical University, Dalian, China
Mesenchymal stem cells (MSCs) have tumor-homing ability and play critical roles in tumor treatment, but their dual influences on tumor progression limit their therapeutic applications. Exosomes derived from MSCs (MSC-exosomes) exhibit great potential in targeted tumor treatment due to their advantages of high stability, low immunogenicity, good biocompatibility, long circulation time and homing characteristics. Furthermore, the artificial modification of MSC-exosomes could amplify their advantages and their inhibitory effect on tumors and could overcome the limit of tumor-promoting effect. In this review, we summarize the latest therapeutic strategies involving artificially modified MSC-exosomes in tumor treatment, including employing these exosomes as nanomaterials to carry noncoding RNAs or their inhibitors and anticancer drugs, and genetic engineering modification of MSC-exosomes. We also discuss the feasibility of utilizing artificially modified MSC-exosomes as an emerging cell-free method for tumor treatment and related challenges.
1 Introduction
Mesenchymal stem cells (MSCs) are well-sourced multipotent stem cells with the capacity to differentiate into osteoblasts, chondrocytes, and adipocytes (1). To unify the standard of MSCs, the Mesenchymal and Tissue Stem Cell Committee of the International Society for Cellular Therapy determined the minimum identification criteria for MSCs. First, MSCs must be plastic-adherent when maintained in standard culture conditions. Second, MSCs must express CD105, CD73 and CD90 and lack expression of CD45, CD34, CD14 or CD11b, CD79α or CD19 and HLA-DR surface molecules. Third, MSCs must differentiate into osteoblasts, adipocytes and chondroblasts in vitro (2). MSCs have unique advantages in tumor treatment. First, MSCs can be ubiquitously found in many tissues, such as bone marrow (3); adipose (4); umbilical vein (5); umbilical cord blood (6); fetal liver (7); synovial membrane (8); amniotic fluid (9); placenta (10); Wharton’s Jelly (11); human umbilical cord perivascular (12); periodontal ligament (13); dental pulp (14); amnion (15); chorion (15); and human levator veli palatini muscle (16), and are not restricted to tissues of mesodermal origin (Table 1). In addition, MSCs can be recruited to injured, inflamed, and hypoxic tissues and the tumor microenvironment, which is termed homing (17–20). Thus, the migratory capacity of MSCs into the tumor microenvironment highlights them as ideal vehicles for tumor-targeted therapy (21). However, we discovered that MSCs have dual tumor-promoting and inhibitory roles in HCC progression (22). Furthermore, according to recent reports, the dual effects of MSCs on tumor progression have been verified in different types of tumors (23–29). In general, MSCs exhibit great potential in tumor treatment, but the dual effects of MSCs on tumor progression have limited their use in tumor therapy. Therefore, exploring a new treatment method for MSCs is of great significance.
Currently, there is a growing consensus regarding the application value of exosomes in tumor-targeted therapy. Exosomes are nanoparticles approximately 40–200 nm in diameter that are saucer shaped under an electron microscope and have special expression patterns represented by markers such as CD9, CD63, CD81 and TSG101 (30, 31). Exosomes released by living cells can carry bioactive substances, such as cytokines, RNA, DNA, and proteins, and are defined as new tools for tumor treatment due to their ability to transfer bioactive substances or anticancer agents into target cells (32–38). Notably, exosomes derived from MSCs (MSC-exosomes) have the advantages of high stability, low immunogenicity, good biocompatibility, and a long period of circulation as well as a homing mechanism similar to MSCs, so MSC-exosomes could be appropriate carriers to transfer bioactive molecules or therapeutic materials to influence the extracellular environment and cancer biology to treat tumors in a safe way (33). In recent studies, MSC-exosomes have been found to have great potential for tumor therapy (39–43). However, the characteristics of MSC-exosomes in promoting the progression of tumors, including promoting tumor growth (44–46), metastasis (45, 47), immunosuppression (48) drug resistance (49) and dormancy in cancer cells (50), limit their application in tumor therapy. We need to explore measures to address restrictions. An increasing number of researchers have proven that modification of MSC-exosomes could overcome these limitations and amplify the advantages of MSC-exosomes in tumor treatment (51–57). Above all, artificially modified MSC-exosomes may eliminate the influence of MSC-exosomes on tumor progression and provide an effective therapy for tumors.
Herein, we provide an overview of recent research progress and describe the application of artificially modified MSC-exosomes in tumor treatment, including carriers of noncoding RNAs or their inhibitors, carriers of anticancer drugs, carriers of nanomaterials and genetic engineering (Figure 1). We also discuss the prospects and challenges regarding the application of MSC-exosomes in tumor treatment to connect the gaps in knowledge and highlight therapeutic opportunities. Furthermore, we also explore the clinical prospects of applying artificially modified MSC-exosomes in tumor treatment and address the feasibility, safety and challenges of artificially modified MSC-exosomes and provide potential solutions.
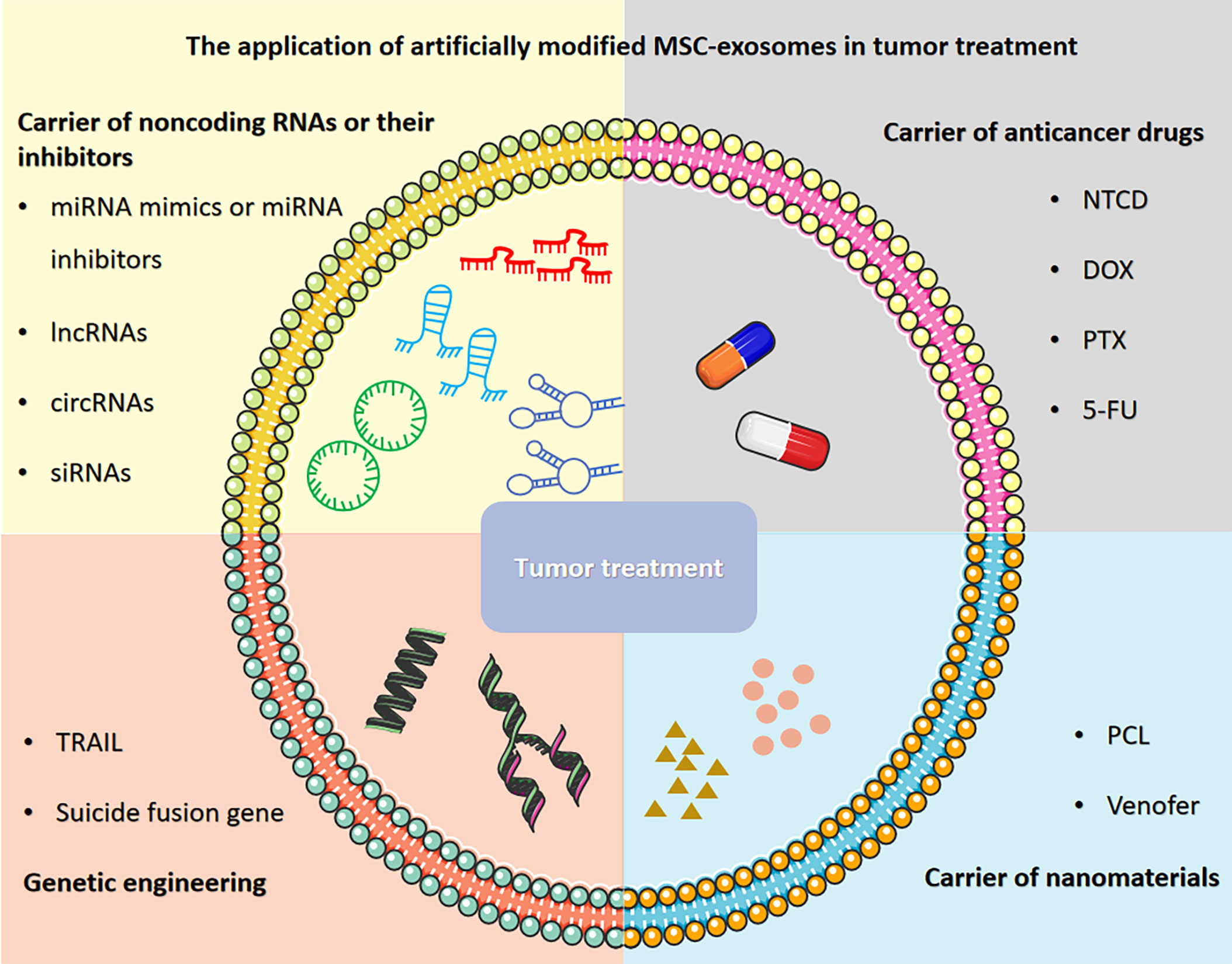
Figure 1 The application of artificially modified MSC-exosomes in tumor treatment includes carriers of noncoding RNAs or their inhibitors, carriers of anticancer drugs, carriers of nanomaterials and genetic engineering. The figure was partly generated using Servier Medical Art, provided by Servier, licensed under a Creative Commons Attribution 3.0 unported license.
2 MSC-exosomes carrying noncoding RNAs or their inhibitors contribute to tumor treatment
Noncoding RNAs (ncRNAs) are genes that are not translated to proteins. They can be divided into different categories according to the size, biogenesis and protein partners of ncRNAs and mainly include microRNAs (miRNAs), small interfering RNAs (siRNAs), PIWI-interacting RNAs (piRNAs), long noncoding RNAs (lncRNAs) and circular RNAs (circRNAs) (58–60). Because ncRNAs cannot code for proteins, it is generally believed that ncRNAs have no function, so ncRNAs are referred to as “junk RNAs” (61). However, recent discoveries have revealed that ncRNAs can affect the expression of other genes through a variety of mechanisms; for example, the binding of miRNAs to the untranslated regions of oligonucleotides could block the effects of oligonucleotides and inhibit gene expression (62, 63). Recent studies have shown that ncRNAs are involved in the progression of different types of diseases, such as cardiac disease (64), neurological diseases (65), bipolar disorders (66), and osteoarthritis (67). Moreover, abundant evidence has shown that ncRNAs are closely related to the occurrence, development, invasion, metastasis and drug resistance of tumors (60). Based on the excellent characteristics of ncRNAs in tumor progression, experiments on the application of ncRNAs in tumor therapy have been widely carried out, which show that changing the expression levels of ncRNAs by transferring mimics and inhibitors of ncRNAs can affect the progression of tumors, thus achieving therapeutic effects (68–72). In recent reports, transferring mimics and inhibitors of ncRNAs into tumor cells through MSC-exosomes has been a very promising strategy in tumor treatment. Here, we summarize the application of MSC-exosomes carrying noncoding RNAs or their inhibitors in tumor treatment.
2.1 Effects of MSC-exosomes with miRNA mimics or miRNA inhibitors on tumor treatment
MiRNAs are a class of 21-23 nucleotide-long, single-stranded noncoding RNAs that regulate posttranscriptional gene expression by inhibiting the process of translation and by promoting mRNA degradation (73–76). It has been reported that miRNAs play essential roles in tumor development by affecting the proliferation, apoptosis, drug resistance, epithelial mesenchymal transition (EMT), cell cycle and metastasis of tumor cells (35, 77–91). Due to the indispensable function of miRNAs in tumor progression, miRNAs could be one of the potentially effective methods for targeted treatment of tumors. MiRNA mimics are synthesized through chemical synthesis methods to simulate endogenous miRNAs in organisms and could enhance the function of endogenous miRNAs (75, 92). In contrast, miRNA inhibitors are a type of inhibitor that are chemically modified and can prevent complementary pairing between miRNAs and their target gene mRNAs, and inhibit the function of miRNAs by strongly competitive binding with mature miRNAs (75). Recent studies reported that MSC-exosomes with miRNA mimics or miRNA inhibitors could be internalized by tumor cells and change the amounts of miRNAs in tumor cells to regulate the corresponding pathways to influence tumor progression and play an important role in tumor therapy (79, 80, 93–95). The loading of MSC-exosomes with miRNA mimics or miRNA inhibitors may be a safe, effective and promising strategy for tumor treatment.
2.1.1 Preparation of MSC-exosomes loaded with miRNA mimics or miRNA inhibitors
At present, the preparation of MSC-exosomes loaded with miRNA mimics or miRNA inhibitors involves two main approaches. First, MSC-exosomes can be modified directly, and the methods currently used for directly modifying MSC-exosomes include electroporation (96–98) and transfection through exosome transfection reagents (99). It has been reported that miR-3182 mimics, miR-142-3p mimics and miR-142-3p inhibitors can be transferred into MSC-exosomes by electroporation, and the membrane of MSC-exosomes can form transient pores through brief electrical pulses, which allow them to enter MSC-exosomes (96–98). Furthermore, miRNA mimics or miRNA inhibitors could be loaded into MSC-exosomes via exosome transfection solution. Ding Y et al. obtained MSC-exosomes with miR-145-5p mimics by simply mixing MSC-exosomes, miR-145-5p mimics and exosome transfection solution (99). Indirect modification of MSC-exosomes has also been widely used, the first step of which is to obtain MSCs containing miRNA mimics or miRNA inhibitors through two main transfection methods: lipofectamine transfection (51, 81, 84) and lentivirus transduction (100, 101). Recent reports revealed that miR-424 mimics, miR-424-5p mimics and miR-139-5p mimics could be transferred into MSCs through Lipofectamine transfection, and this method shows high transfection efficiency, but Lipofectamine exhibits certain cytotoxicity (51, 81, 84). Sheykhhasan M and Yan T et al. found that miR-145 mimics and miR-512-5p mimics could be transferred into MSCs through lentivirus transduction, which resulted in better stability; however, the load of lentivirus was limited. Moreover, researchers have also obtained MSCs containing miRNA mimics or miRNA inhibitors by coculturing MSCs with miRNA mimics or miRNA inhibitors. Du L et al. transferred miR-21-5p mimics and miR-21-5p inhibitor into MSCs by coculturing miR-21-5p mimics and miR-21-5p inhibitor with MSCs (102). Then, MSC-exosomes could be isolated from MSCs modified by miRNA mimics or miRNA inhibitors. Although several approaches and methods that produce specific MSC-exosomes have been established, the efficiency of these approaches and methods has not been compared. Further studies are needed to identify the most efficient method of generating specific MSC-exosomes to facilitate the application of these structures in tumor treatment.
2.1.2 Application of MSC-exosomes containing miRNA mimics or miRNA inhibitors in tumor therapy
It is widely known that miRNA expression is altered in tumors, and the differential expression of miRNAs influences their effects on tumor growth, highlighting their potential in tumor therapy (103). Due to the dual effect of miRNAs in tumor progression, two types of approaches can be used to adjust the expression level of miRNAs for tumor therapy: suppressing the expression of miRNAs that promote the biological processes of tumors by transferring miRNA inhibitors to tumor cells and enhancing the expression of miRNAs that inhibit the biological processes of tumors by transferring miRNAs to tumor cells. Compared with transferring miRNA mimics or miRNA inhibitors directly into tumor cells, transferring miRNA mimics or miRNA inhibitors via MSC-exosomes has many advantages. First, MSC-exosomes could enhance the inhibitory effect of miRNA mimics or miRNA inhibitors. Shimbo et al. compared the delivery efficiency of miR-143 in osteosarcoma cells subjected to MSC-exosome treatment or lipofection and found that the efficiency of MSC-exosome delivery was lower than that of lipofection, but the inhibitory effect on cell migration was similar in the migration assay (85). Furthermore, MSC-exosomes have excellent tumor cell-targeting ability and biosafety. Due to the advantages of MSC-exosomes, these structures could be exploited as a potentially efficient tool for transferring miRNA mimics or miRNA inhibitors to facilitate tumor treatment.
Considering that some miRNAs can inhibit the progression of cancers and that the expression of miRNAs decreases in some cancer tissues and cells, artificially increasing the expression of miRNAs in cancer cells through MSC-exosomes has a potential inhibitory effect on tumor progression. Chen Z et al. found that miR-6785-5p was downregulated and inhibin A (INHBA) was upregulated in gastric cancer tissues and cells, and miRNA-6785-5p was targeted by INHBA. The malignant development of gastric cancer cells could be restricted by elevating the expression of miR-6785-5p or inhibiting INHBA. MSCs that transferred miR-6785-5p mimics could release exosomes enriched with miR-6785-5p into gastric cancer cells to increase the expression of miR-6785-5p in gastric cancer cells and then reduce the expression of INHBA to suppress angiogenesis and metastasis in gastric cancer (82). There is a lack of evidence regarding the in vivo effects of this novel treatment modality. However, the hypothesis that MSC-exosomes with miRNA mimics could inhibit the progression of cancer in vivo could be proven through Jia Y’s experiment. They injected MSC-exosomes with and without miR-139-5p mimics into subcutaneous xenograft model mice via the tail vein and found that the presence of miR-139-5p in exosomes reduced the volume and weight of tumors in nude mice. miR-139-5p was downregulated and PCR1 was upregulated in tumor tissues. Overall, MSC-exosomes with miR-139-5p mimics restrain tumorigenesis in bladder cancer by targeting PCR1 in vivo (84). In addition to the above two studies, a variety of studies have reported the effect of MSC-exosomes carrying miRNAs mimics on the progression of tumors; these miRNA mimics cargoes include miR-16-5p (104), miR-4461 (105), miR-7 (106), miR-199a (107), miR-133b (108), miR-29a-3p (35), miR-144 (109), miR-193a (110), miR-143 (85), miR-206 (111), miR-1231 (32), miR-152 (112), miR-205 (113), miR-101-3p (114), miR-124a (115), miR-584-5p (116), miR-512-5p (100), miR-204 (79), miR-424-5p (51), miR-122 (117), miR-199a (118), miR-145 (101), miR-181a (80), miR-375 (86), miR-6785-5p (82), miR-139-5p (84), miR-3940-5p (119), miR-21-5p (102), miR-124 (120), miR-34a (52), miR-3182 (98), miR-145-5p (99), miRNA-222-3p (121) (Table 2).
miRNAs can also promote the progression of cancer; the expression of miRNAs is increased in some cancer tissues and cells, and the progression of cancer could be inhibited by artificially decreasing the expression of miRNAs in cancer cells. Zhang N et al. found that MSC-derived exosomes with a miR-424 inhibitor could reduce the expression of miR-424 in colorectal cancer cells and inhibit the progression of tumors by upregulating TGFBR3 (81); Naseri Z et al. discovered that MSC-derived exosomes carrying a miR-142-3p inhibitor could reduce the tumorigenicity of breast cancer in vitro and in vivo, highlighting the clinical potential of this method for cancer treatment (97). Furthermore, Naseri Z et al. utilized MSC-exosomes to deliver a miR-142-3p inhibitor that was modified by locked nucleic acid molecules into breast cancer stem cells to reduce the tumorigenicity of breast cancer stem cells (96). In general, the processes of proliferation, apoptosis, drug resistance and EMT could be inhibited by reducing the expression of tumor-promoting miRNAs via MSC-exosomes containing miRNA inhibitors to achieve the effect of tumor treatment.
Overall, MSC-exosomes containing miRNA mimics or miRNA inhibitors have great potential in tumor treatment, but the effects of different miRNAs on different tumors are still unclear. Moreover, there are still a series of questions to address regarding the application of MSC-exosomes containing miRNA mimics or miRNA inhibitors in tumor treatment; these questions pertain to the selection of MSC types, the manner of exosome extraction, and the selection of a safe dose of exosomes in vivo.
2.2 Effects of MSC-exosomes containing lncRNAs on tumors
LncRNAs are an important class of noncoding RNA molecules; they are transcripts longer than 200 nucleotides and participate in a range of biological functions by regulating the expression and functions of genes at the transcriptional, translational, and posttranslational levels (126, 127). Although lncRNAs cannot code for proteins, it has been reported that lncRNAs are involved in some aspects of oncology, including tumorigenesis, metastasis, chemoresistance, cancer stem cell function and EMT (127–130).
Because lncRNAs play an important role in tumor formation and progression and dysregulated lncRNAs exhibit distinct biological functions to promote or suppress tumor growth, regulating their expression has become a potential strategy for tumor treatment. Similar to the approach involving miRNAs, lncRNAs could be employed to inhibit the biological processes of tumors by transferring lncRNAs to tumor cells to enhance their expression for tumor treatment. Moreover, because of the advantages of MSC-exosomes over other transfer strategies, MSC-exosomes containing lncRNAs may become a new mode of tumor treatment (Table 3). Hao SC et al. found that the expression of lncRNA PTENP1 was apparently lower in glioma samples than in normal tissues, that lncRNA PTENP1 could inhibit the proliferation of glioma, and that lncRNA PTENP1 could be packaged into MSC-exosomes and transferred to glioma cells and then stabilize PTENP1 by competitively binding miR-10a-5p to improve the antitumor capacity (122).
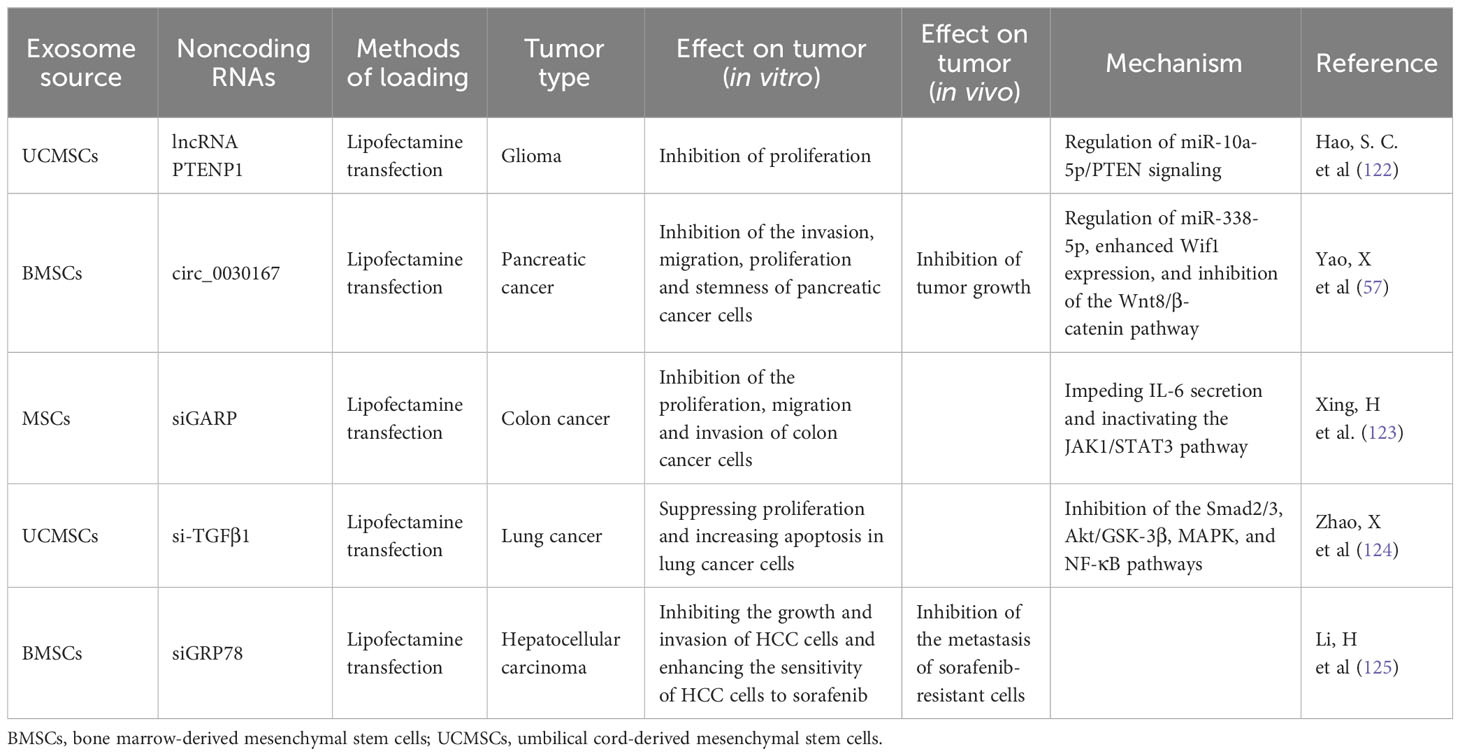
Table 3 Effect of MSC-exosomes enriched with other types of noncoding RNAs (lncRNAs, circRNAs, siRNA) on tumor progression.
MSC-exosomes containing lncRNAs may represent a new option for treating tumors. However, there are still many challenges to be addressed. First, we need to have a deeper understanding of lncRNA function in tumorigenesis and development, and second, we need to choose suitable lncRNAs to apply to different types of tumor treatments. Finally, we need to explore whether different lncRNAs can be combined to improve the effectiveness of tumor treatment.
2.3 Effects of MSC-exosomes containing circRNAs on tumor treatment
CircRNAs are a new type of noncoding RNA that consists of covalently closed loops formed through backsplicing; these RNAs play key roles in cancer development and progression by acting as miRNA sponges and transcriptional regulators and combining with RNA binding proteins (131–133). In recent reports, the enrichment and stability of circRNAs in exosomes have been assessed (134). Overall, MSC-exosomes with circRNAs have potential applications as a new method of tumor treatment (Table 3). Yao X et al. found that circ_0030167 mainly regulated miR-338-5p, enhanced Wif1 expression, and inhibited the Wnt8/β-catenin pathway, thereby inhibiting pancreatic cancer cell stemness and tumor progression; MSC-exosomes could significantly inhibit the migration, invasion, proliferation, and stemness of pancreatic cancer cells by transferring circ_0030167 (57). However, the mechanism of circRNAs in tumor progression still needs to be explored. In general, MSC-exosomes containing circRNAs may represent a new tool for targeted tumor therapy.
2.4 Effects of exosomes derived from MSCs transfected with siRNAs on tumors
SiRNAs are a class of noncoding RNAs that are generally from 21 to 25 base pairs in length and are produced from longer dsRNA, which is cleaved by the dsRNA-specific endonuclease Dicer (135, 136). siRNAs have shown potential as a new therapeutic reagent in tumor treatment due to their gene-knockdown capability (137).
Because of their advantages in terms of homing and safety, MSC-exosomes have been considered the most promising carrier in tumor treatment. In recent reports, the effect of exosomes derived from MSCs transfected with siRNA in tumor treatment has been noted (Table 3). Xing H et al. found that GARP expression in exosomes derived from MSCs transfected with siGARP was significantly decreased and that siGARP-MSC-derived exosomes could inhibit the proliferation, migration and invasion of colon cancer cells by impeding IL-6 secretion and inactivating the JAK1/STAT3 pathway (123). Zhao X et al. found that MSC-exosomes could inhibit the proliferation and promote the apoptosis of lung cancer cells, yet MSC-exosomes could promote invasion and migration and induce EMT. Furthermore, exosomes derived from MSCs transfected with si-TGFβ1 enhanced the ability to suppress proliferation and increase apoptosis in lung cancer cells and eliminated the induction of EMT in lung cancer cells by inhibiting the Smad2/3, Akt/GSK-3β, MAPK, and NF-κB pathways (124). The above two studies show the ability of MSC-exosomes in tumor treatment, but the expression of genes that siRNAs knock down in tumors has not yet been justified. Exosomes derived from MSCs transfected with siGRP78 have been reported to target GRP78 and inhibit its expression in sorafenib-resistant hepatocellular carcinoma (HCC) cells, inhibit the growth and invasion of HCC cells and enhance the sensitivity of HCC cells to sorafenib (125).
Currently, the application of artificially modified MSC-exosomes in tumor treatment mainly focuses on preclinical studies and clinical trials are also gradually underway. Clinical trial for the treatment of solid tumors with artificially modified MSC-exosomes has been registered in the treatment of metastatic PDAC patients with KrasG12D mutation. M.D. Anderson Cancer Center sponsored a phase 1 clinical trial to explore the best dose and side effects of MSC-exosomes with the KrasG12D siRNA in treating participants with pancreatic cancer with KrasG12D mutation that has spread to other places in the body. Although the treatment results have not been published, we need to pay more attention and have more patience for the clinical transformation of artificially modified MSC-exosomes in tumor therapy. There are many issues, including GMP compliance, regulatory considerations, and safety profiles, that need to be solved before using exosomes in clinical practice. We believe that an increasing number of clinical trials will be applied to different types of solid tumors.
This new approach reveals the potential value of MSC-exosomes in tumor treatment, but researchers have paid attention to the effect of exosomes derived from MSCs transfected with siRNA on tumors, not the application of MSC-exosomes as carriers for tumor treatment. The potential of MSC-exosomes as therapeutic carriers for tumor treatment has not been fully exploited, and the application of MSC-exosomes modified directly with siRNAs in tumor treatment has not been studied. Therefore, this method may be a direction of future research.
3 MSC-exosomes as carriers of anticancer drugs for tumor treatment
A growing body of research suggests that MSCs are potential carriers for delivering anticancer drugs because of their ability to migrate specifically to tumors (138, 139). However, the tumor-promoting ability limits the application of MSCs in the clinical treatment of tumors. With the birth of nanotechnology in the 1990s, nanomedicine has become an important development direction of modern medicine for various disease therapeutics and diagnoses, and exosomes have emerged as promising nanomedicine carriers for tumor treatment due to their advantages (140, 141). It has been reported that MSC-exosomes can be internalized by tumor cells (142), and MSC-exosomes exhibit excellent characteristics in tumor treatment. Compared with traditional chemotherapy modalities, MSC-exosomes carrying anticancer agents can precisely deliver drugs into tumor cells to alleviate the side effects of drugs and greatly enhance tumor inhibition. Wei, H et al. found that MSC-exosomes carrying anticancer drugs could enhance cellular uptake in tumor cells but reduce cytotoxicity to normal cells and repair damaged tissues (34, 143, 144). Overall, MSC-exosomes can serve as carriers of anticancer drugs, bringing new ideas and hopes for the development of new tumor treatments.
3.1 Antitumor effects of MSC-exosomes modified directly with anticancer drugs
Generally, the preparation of MSC-exosomes with anticancer drugs involves two main approaches: direct and indirect modification. First, MSC-exosomes can be modified directly: we can collect MSC-exosomes from the cell supernatant of MSCs, and anticancer drugs can be directly loaded into MSC-exosomes by different loading methods, including electroporation (34), incubation (143), extrusion (145), sonication (146), and freeze−thaw cycles (145) (Figure 2A). Liang, L et al. loaded norcantharidin (NCTD) into MSCs to obtain MSC-exosomes with norcantharidin (MSC-exosome-NCTD) via electroporation, which exhibited positive loading efficiency, but pulses of electroporation may cause damage to anticancer drugs and the membrane integrity of MSC-exosomes. Compared with NCTD treatment alone, MSC-exosomes-NCTD treatment enhanced cellular uptake and apoptosis, induced HCC cell cycle arrest and inhibited HCC cell proliferation. MSC-exosomes-NCTD showed HCC tissue homing ability in vivo and repaired damaged liver tissues (34, 147, 148). Incubation is the most commonly used and easiest method to load drugs, but incubation exhibits low loading efficiency; sonication demonstrates better efficiency than incubation but can destroy MSC-exosome membranes and cause protein aggregation, impacting the efficiency of loading. Chen, M found that 5-fluorouracil (5-FU) could be loaded into MSC-exosomes via incubation and sonication and found that the sonication method had a higher loading efficiency than the incubation method. Moreover, MSC-exosomes-5-FU had good anti-cholangiocarcinoma efficacy and safety (146–148). Furthermore, doxorubicin (DOX) could be loaded into MSC-exosomes via electroporation and incubation. MSC-exosome-DOX exerted a stronger inhibitory effect than DOX alone on colon cancer and osteosarcoma and hindered the progression of tumors (33, 143, 144). In addition, extrusion and freeze−thaw cycles could also be applied as methods for loading anticancer drugs into MSC-exosomes, and uniformly sized MSC-exosomes with a high content of anticancer drugs could be obtained through extrusion, but damage to the MSC-exosome membrane during extrusion and freeze−thaw cycles limits the application of this approach in tumor treatment (145). In recent studies, electroporation and incubation have been the two main ways of loading anticancer drugs into MSC-exosomes directly.
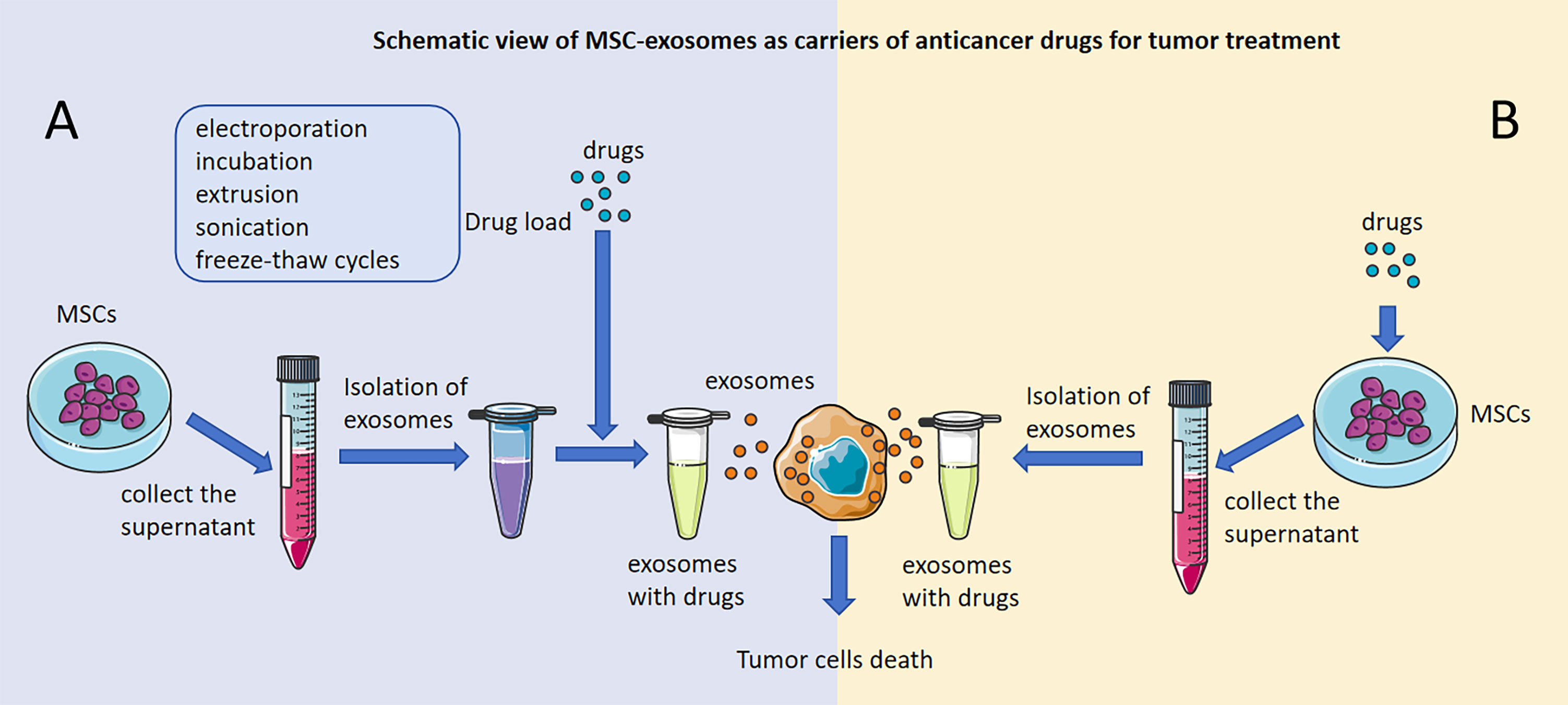
Figure 2 Schematic view of MSC-exosomes as carriers of anticancer drugs for tumor treatment. Antitumor effects of MSC-exosomes modified directly with anticancer drugs. (A) Antitumor effects of drug-loaded MSC-exosomes developed by direct modification of MSCs. (B) The figure was partly generated using Servier Medical Art, provided by Servier, licensed under a Creative Commons Attribution 3.0 unported license.
3.2 Antitumor effects of drug-loaded MSC-exosomes developed by direct modification of MSCs
Indirect modification of MSC-exosomes has also been applied to load anticancer drugs, and we can collect the cell supernatant of MSCs that are incubated with antitumor drugs and then extract MSC-exosomes with antitumor drugs (Figure 2B). MSC-exosomes that have significant antitumor effects could be isolated from MSCs that were incubated with Taxol, vincristine and paclitaxel (PTX) (55, 56, 142). Pascucci L et al. found that MSC-exosomes could be isolated from MSCs incubated with PTX to form MSC-exosomes-PTX that release PTX into pancreatic cancer cells and have a significant antitumor effect. This was the first time that MSC-exosomes had been proven to be useful as carriers of anticancer agents to treat tumors, and this new strategy was presented as a way to precisely target tumors and release agents for tumor treatment (142). Vincristine could be loaded into MSCs directly via incubation, and then MSC-exosomes-vincristine could be isolated and could increase cytotoxicity for glioblastoma cells (56). However, the method of indirect modification of MSC-exosomes is seldom applied in practice and will be the direction of our future research.
Antitumor drugs have varying degrees of side effects on the human body including myocardial injury, nervous system injury and kidney damage. Reducing the impact of these side effects is a hot topic in tumor treatment for most researchers. Wei H et al. found that MSC-exosome-DOX could reduce the damage of DOX to the myocardium at the cellular and tissue levels (143, 144). Reducing the side effects of antitumor drugs is also our current research focus, and we conjecture whether a series of side effects can be alleviated by enhancing the targeting of MSC-exosomes to tumors.
In general, considering the unique advantages of MSC-exosomes in tumor treatment, MSC-exosomes can play a role as a vehicle for targeted drug delivery. How to improve the drug loading efficiency and the stability of MSC-exosome-antitumor drugs is our next research goal. We can combine two types of drug loading methods to increase drug loading efficiency, and we can utilize new materials such as hydrogels to improve the stability of drug loading systems.
4 MSC-exosomes as carriers of nanomaterials for tumor treatment
With the development of medicine, the combination of drug treatment and other modalities has become a new strategy for tumor treatment. MSC-exosomes are an emerging modality for the treatment of tumors, and their combination with other treatments will have an enormous impact on advancing the treatment of tumors. Due to their ability to home to tumors, MSC-exosomes exhibit a good ability to transport cargo. In recent studies, MSC-exosomes have been applied as tools to transfer new synthetic materials into tumor cells and have demonstrated great potential in tumor therapy. Altanerova, U et al. obtained MSC-exosomes containing Venofer from MSCs incubated with Venofer, an iron oxide magnetic nanoparticle, and found that MSC-exosomes containing Venofer could be efficiently internalized by tumor cells and facilitate targeted tumor cell ablation to achieve tumor therapeutic effects via magnetically induced hyperthermia (149). Furthermore, Rezaie Z et al. found that MSC-exosomes could be incorporated into polycaprolactone (PCL) nanofibers by electrospinning, and PCL-MSC-exosomes exhibited significant effects in inducing the apoptosis of breast cancer cells. PCL-MSC-exosomes could replace traditional antitumor drugs and become a new method of tumor treatment (54). The emerging modalities for the treatment of tumors have considerable advantages and reduce the limitations of traditional methods of tumor treatment, but the emerging modalities require many in vivo experiments to verify their safety. Currently, research on MSC-exosomes as carriers of nanomaterials for tumor treatment is still rare, but nanomedicine is in a rapid development stage, and a growing number of therapeutic nanomaterials are being developed for tumor treatment. We believe this method will be an attractive research hotspot. Faced with this emerging treatment model, we need to further evaluate the safety of nanomaterials considering their effect on protein stability and human metabolism and verify their safety using animal models.
5 Application of MSC-exosomes modified by genetic engineering in tumor treatment
After almost 30 years of development, genetic engineering has rapidly become a critical method of treating a variety of diseases, including blood disorders, genetic diseases and tumors (150–152). In particular, with continuous expansion and adaptation, genetic engineering has emerged as a method for the treatment of different types of tumors, and such strategies could enhance the efficacy and safety of tumor treatments as well as broaden the potential applications for tumor treatments. Furthermore, MSC-exosomes, as an emerging method in tumor treatment, could be combined with genetic engineering to dramatically alter the landscape of tumor treatment. Recent reports revealed that MSCs could be prepared via genetic engineering to express target genes that inhibit the progression of tumors. Engineered MSC-exosomes could also be isolated from engineered MSCs and applied to tumor treatment, which significantly improved the efficiency of tumor treatment (53, 153). Tumor necrosis factor-related apoptosis-inducing ligand (TRAIL) is a potential agent for cancer treatment, and a TRAIL/GFP encoding plasmid could be transfected into MSCs through PEI25Pyr50% (a nonviral vector) to obtain TRAIL-engineered MSCs. TRAIL-engineered MSC-exosomes demonstrated tumor-homing ability and inhibited tumor progression through abundant necrosis in melanoma cells in both in vitro and in vivo models (53). Altanerova U et al. used retrovirus infection to transfer the suicide fusion gene-yeast cytosine deaminase::uracil phosphoribosyl (yCD::UPRT) into MSCs, and yCD::UPRT-MSC-exosomes could be isolated from the conditioned medium (CM) of yCD::UPRT-MSCs and could be internalized by cancer cells. Moreover, yCD::UPRT-MSC-exosomes could convert the prodrug 5-fluorocytosine (5-FC) to 5-FU in cancer cells to efficiently induce tumor cell death (153). You, B et al. extracted MSC-exosomes with L-PGDS (MSC-exosomes- L-PGDS) from MSCs which transferred through adenovirus encoding L-PGDS, and found that MSC-exosomes- L-PGDS could inhibit the colony-forming, migration, and invasion abilities of gastric cancer cells in vitro and inhibit the tumor growth in a nude mouse subcutaneous tumor-bearing model in vivo (154). Genetically engineered MSC-exosomes have been considered a promising tumor treatment strategy, but studies on their application are still in their infancy, and further research is needed. The development of more effective and safer genetic engineering therapy methods for MSCs remains a challenge in tumor treatment.
6 Discussion
As a cell-free system, MSC-exosomes can be employed in various promising approaches for tumor treatment that exhibit safety because of their limited effect on normal cell viability. MSC-exosomes have been used in the clinical treatment of different diseases, including steroid-refractory graft-versus-host disease (155), chronic kidney disease (156), and liver diseases (157), polycystic ovary syndrome (158). However, there are still a few concerns that need to be resolved before applying MSC-derived exosomes in tumor clinical therapy. First, to achieve the large-scale preparation of exosomes, the optimization of methods for isolating exosomes from MSCs is the most important step. At present, the main methods for isolating exosomes include sequential ultracentrifugation, gradient ultracentrifugation, ultrafiltration, size-exclusion chromatography, polymer precipitation, immunoaffinity capture and microfluidics-based techniques. In recent years, although great progress has been made in exosome isolation methods, many problems still need to be solved, such as contamination, low purity, high cost, and low yields (159). The combination of two different types of isolation methods may yield a rapid and cost-effective method for exosome isolation. Concurrently, the number of exosomes produced by cells could be increased. Faezeh Vakhshiteh F et al. found that the abundance of exosomes is related to the source of MSCs. Due to the high proliferation rate and the great capacity of pulp-derived MSCs (DPSCs) to differentiate into different cells, dental pulp has the ability to secrete more exosomes, and DPSCs could be suggested as the ideal cell type for high-yield exosome production (52). Overall, to apply MSC-exosomes for tumor treatment in the clinic, exosome isolation technology should be improved and developed to enable the isolation of high concentrations of exosomes with high efficiency, and suitable sources for isolating MSCs should be assessed. Moreover, the antitumor effect of MSC-exosomes is related to the source of MSCs, processing mode and type of cancer (160). Del Fattore A et al. found that the effects of BMSC-exosomes UCMSC-exosomes and ATMSC-exosomes on the proliferation and apoptosis of glioblastoma cells are different, and different types of MSC-exosomes have various influences on the same tumor (56). In addition to the source of MSCs, exosomes from MSCs treated in different ways exert different effects on tumor cells. Zhang X et al. found that hypoxic MSC-exosomes could promote the invasion of lung cancer cells and EMT by activating STAT3 signaling via the aberrant expression of miRNAs (miR-193a-3p, miR-210-3p and miR-5100) in hypoxic MSC-exosomes (161). Overall, patients with different tumors have different characteristics, and choosing the appropriate MSC source and processing mode is the key to improving the treatment effect.
Notably, the inhibitory effect of MSC-exosomes on tumor progression has been indicated by an increasing number of studies. It has been reported that MSC-exosomes can enhance the cytotoxicity of radiotherapy and chemotherapy in tumors and metastatic tumor foci (1, 39). Combining MSC-exosomes with other treatments, such as radiotherapy and chemotherapy, could greatly improve the efficiency of tumor treatment. Furthermore, MSC-exosomes could be used in tumor treatment directly because MSC-exosomes are rich in miRNAs that could inhibit the progression of tumors. Lee JK et al. found that miR-16 was enriched in MSC-exosomes and could inhibit angiogenesis in vitro and in vivo by significantly downregulating vascular endothelial growth factor (VEGF) in breast cancer cells, and Pakravan K et al. found that MSC-exosomes were rich in miR-100 and could suppress the angiogenesis of breast cancer by modulating the mTOR/HIF-1α/VEGF signaling axis (40, 41). To our surprise, some studies have found that MSC-exosomes exhibit great potential in the treatment of leukemia; it has been reported that MSC-exosomes are rich in miR-124-5p and miR-222-3p and suppress the progression of acute myeloid leukemia by downregulating SMC4C and targeting IRF2/INPP4B (42, 43). The advantage of this characteristic of MSC-exosomes gives us a new idea to explore the potential of MSC-exosomes in tumor treatment. However, there is growing evidence indicating that MSC-exosomes can promote tumor growth (44–46), metastasis (45, 47), immunosuppression (48) drug resistance (49) and dormancy in cancer cells (50). Scientists have recently reported that MSC-exosomes could promote the growth of gastric cancer, colon cancer and osteosarcoma (44–46) and enhance the migration of breast cancer cells and gastric cancer cells (45, 47). Recent reports have shown that immunosuppression is an essential factor affecting tumor development. Wang J et al. found that MSC-derived exosomes could promote the release of NO from myeloid-derived suppressor cells to enhance their inhibitory effects on T cells through the STAT1 and STAT3 pathways to induce immunosuppression and promote the progression of multiple myeloma (48). MSC-exosomes can also activate the CaM-Ks/Raf/MEK/ERK pathway to induce drug resistance in gastric cancer cells, and MSC-exosomes can promote osteosarcoma and gastric cancer cell growth through activation of the hedgehog signaling pathway (45, 46). Deng, M et al. found that lnc00461 is highly expressed in patients with multiple myeloma and that lnc00461 can be transferred into multiple myeloma cells through MSC-derived exosomes and promote multiple myeloma tumorigenesis by regulating miR-15a/16 and BCL-2 (162). It has been reported that miR-23b is enriched in MSC-exosomes and can promote dormancy in metastatic breast cancer cells by decreasing MARCKS expression (50). To address the limitation of the tumor-promoting effect, we chose to artificially modify MSC-exosomes in tumor treatment. Through current research, we determined that artificially modified MSC-exosomes could serve as a potential selection for tumor treatment in future research.
Improving treatment effectiveness is an important indicator of tumor treatment methods. We propose that engineering treatment with MSC-exosomes can improve tumor targeting to improve the therapeutic effect, and we could load modified targeted peptides onto MSC-exosomes membranes to improve tumor targeting. Furthermore, the safety of MSC-exosomes in the treatment of tumors is also an important indicator for preclinical application, although artificially modified MSC-exosomes could significantly reduce the promoting effect of MSC-exosomes on tumor progression, but this not enough. MSC-exosomes mainly exert antitumor effects through contents consisting of miRNAs, proteins, lipids and metabolites, which will be the focus of future research. How to use this characteristic in tumor treatment is worth considering (37). Furthermore, the content of MSC-exosomes also had promoted tumors. To eliminate their impact on tumor progression, whether we could process MSC-exosomes before application to clean up the contents of the MSC-exosomes, we conjectured that reformation of the MSC-exosomes membrane upon sonication resulted in clearance of MSC-exosomes contents. Therefore, we could improve the safety of MSCs-exosomes therapy via this method.
7 Conclusions and perspectives
We highlighted the great potential of MSC-exosomes in tumor treatment compared with traditional methods of tumor treatment, but the dual effects of exosomes from MSCs on tumors limit their application. We propose the feasibility of using artificially modified MSC-exosomes to treat tumors. There are still considerable challenges regarding the preparation of high-concentration exosomes with high efficiency and the feasibility of clinical application. While studies on the effects of artificially modified MSC-exosomes in tumor treatment are still in the early development period, research progress in the field provides hope that artificially modified MSC-exosomes could be regarded as an efficient, safe and targeted therapy for tumors with minor side effects.
Author contributions
YS: Writing – original draft, Writing – review & editing. QS: Writing – review & editing. DH: Writing – review & editing. BS: Writing – review & editing. MG: Writing – review & editing. XL: Writing – review & editing. BQ: Writing – review & editing. LS: Writing – review & editing. ZY: Writing – original draft, Writing – review & editing. LW: Writing – original draft, Writing – review & editing.
Funding
The author(s) declare financial support was received for the research, authorship, and/or publication of this article. This work was supported by the National Natural Science Foundation of China [81972749, 82203888]; Science and Technology Project of Liaoning [2021JH2/10300020, 2021-BS-204]; Innovative Teams Project in Key Areas of Dalian [2021RT01]; Dalian Science and technology talents plan [2022RQ044]. The funding body played no role in the design of the study and collection, analysis, and interpretation of data and in writing the manuscript.
Conflict of interest
The authors declare that the research was conducted in the absence of any commercial or financial relationships that could be construed as a potential conflict of interest.
Publisher’s note
All claims expressed in this article are solely those of the authors and do not necessarily represent those of their affiliated organizations, or those of the publisher, the editors and the reviewers. Any product that may be evaluated in this article, or claim that may be made by its manufacturer, is not guaranteed or endorsed by the publisher.
References
1. Liu Y, Song B, Wei Y, Chen F, Chi Y, Fan H, et al. Exosomes from mesenchymal stromal cells enhance imatinib-induced apoptosis in human leukemia cells via activation of caspase signaling pathway. Cytotherapy (2018) 20:181–8. doi: 10.1016/j.jcyt.2017.11.006
2. Dominici M, Le Blanc K, Mueller I, Slaper-Cortenbach I, Marini F, Krause D, et al. Minimal criteria for defining multipotent mesenchymal stromal cells. The International Society for Cellular Therapy position statement. Cytotherapy (2006) 8:315–7. doi: 10.1080/14653240600855905
3. Pittenger MF, Mackay AM, Beck SC, Jaiswal RK, Douglas R, Mosca JD, et al. Multilineage potential of adult human mesenchymal stem cells. Science (1999) 284:143–7. doi: 10.1126/science.284.5411.143
4. Zuk PA, Zhu M, Mizuno H, Huang J, Futrell JW, Katz AJ, et al. Multilineage cells from human adipose tissue: implications for cell-based therapies. Tissue Eng (2001) 7:211–28. doi: 10.1089/107632701300062859
5. Covas DT, Siufi JL, Silva AR, Orellana MD. Isolation and culture of umbilical vein mesenchymal stem cells. Braz J Med Biol Res (2003) 36:1179–83. doi: 10.1590/s0100-879x2003000900006
6. Lee OK, Kuo TK, Chen WM, Lee KD, Hsieh SL, Chen TH. Isolation of multipotent mesenchymal stem cells from umbilical cord blood. Blood (2004) 103:1669–75. doi: 10.1182/blood-2003-05-1670
7. Gotherstrom C, Ringden O, Westgren M, Tammik C, Le Blanc K. Immunomodulatory effects of human foetal liver-derived mesenchymal stem cells. Bone Marrow Transplant (2003) 32:265–72. doi: 10.1038/sj.bmt.1704111
8. De Bari C, Dell'Accio F, Tylzanowski P, Luyten FP. Multipotent mesenchymal stem cells from adult human synovial membrane. Arthritis Rheum (2001) 44:1928–42. doi: 10.1002/1529-0131(200108)44:8<1928::aid-art331>3.0.co;2-p
9. Tsai MS, Lee JL, Chang YJ, Hwang SM. Isolation of human multipotent mesenchymal stem cells from second-trimester amniotic fluid using a novel two-stage culture protocol. Hum Reprod (2004) 19:1450–6. doi: 10.1093/humrep/deh279
10. Fukuchi Y, Nakajima H, Sugiyama D, Hirose I, Kitamura T, Tsuji K. Human placenta-derived cells have mesenchymal stem/progenitor cell potential. Stem Cells (2004) 22:649–58. doi: 10.1634/stemcells.22-5-649
11. Wang HS, Hung SC, Peng ST, Huang CC, Wei HM, Guo YJ, et al. Mesenchymal stem cells in the Wharton's jelly of the human umbilical cord. Stem Cells (2004) 22:1330–7. doi: 10.1634/stemcells.2004-0013
12. Sarugaser R, Lickorish D, Baksh D, Hosseini MM, Davies JE. Human umbilical cord perivascular (HUCPV) cells: a source of mesenchymal progenitors. Stem Cells (2005) 23:220–9. doi: 10.1634/stemcells.2004-0166
13. Seo BM, Miura M, Gronthos S, Bartold PM, Batouli S, Brahim J, et al. Investigation of multipotent postnatal stem cells from human periodontal ligament. Lancet (2004) 364:149–55. doi: 10.1016/s0140-6736(04)16627-0
14. Pierdomenico L, Bonsi L, Calvitti M, Rondelli D, Arpinati M, Chirumbolo G, et al. Multipotent mesenchymal stem cells with immunosuppressive activity can be easily isolated from dental pulp. Transplantation (2005) 80:836–42. doi: 10.1097/01.tp.0000173794.72151.8
15. Kwon A, Kim Y, Kim M, Kim J, Choi H, Jekarl DW, et al. Tissue-specific differentiation potency of mesenchymal stromal cells from perinatal tissues. Sci Rep (2016) 6:23544. doi: 10.1038/srep23544
16. Bueno DF, Kabayashi GS, Pinheiro CCG, Tanikawa DYS, Raposo-Amaral CE, Rocha DL, et al. Human levator veli palatini muscle: a novel source of mesenchymal stromal cells for use in the rehabilitation of patients with congenital craniofacial malformations. Stem Cell Res Ther (2020) 11:501. doi: 10.1186/s13287-020-02017-7
17. Nakamizo A, Marini F, Amano T, Khan A, Studeny M, Gumin J, et al. Human bone marrow-derived mesenchymal stem cells in the treatment of gliomas. Cancer Res (2005) 65:3307–18. doi: 10.1158/0008-5472.Can-04-1874
18. Zong C, Meng Y, Ye F, Yang X, Li R, Jiang J, et al. AIF1(+) CSF1R(+) MSCs, induced by TNF-α, act to generate an inflammatory microenvironment and promote hepatocarcinogenesis. Hepatology (2022) 78:434–51. doi: 10.1002/hep.32738
19. Zhuang WZ, Lin YH, Su LJ, Wu MS, Jeng HY, Chang HC, et al. Mesenchymal stem/stromal cell-based therapy: mechanism, systemic safety and biodistribution for precision clinical applications. J BioMed Sci (2021) 28:28. doi: 10.1186/s12929-021-00725-7
20. Chen J, Fujita N, Takeda T, Hanyu W, Takatani H, Nakagawa T, et al. Canine bone marrow peri-adipocyte cells could therapeutically benefit acute spinal cord injury through migration and secretion of hepatocyte growth factor to inflammatory milieu. Exp Anim (2022) 72:19–29. doi: 10.1538/expanim.22-0026
21. Studeny M, Marini FC, Dembinski JL, Zompetta C, Cabreira-Hansen M, Bekele BN, et al. Mesenchymal stem cells: potential precursors for tumor stroma and targeted-delivery vehicles for anticancer agents. J Natl Cancer Inst (2004) 96:1593–603. doi: 10.1093/jnci/djh299
22. Yin Z, Jiang K, Li R, Dong C, Wang L. Multipotent mesenchymal stromal cells play critical roles in hepatocellular carcinoma initiation, progression and therapy. Mol Cancer (2018) 17:178. doi: 10.1186/s12943-018-0926-6
23. Qiao L, Xu Z, Zhao T, Zhao Z, Shi M, Zhao RC, et al. Suppression of tumorigenesis by human mesenchymal stem cells in a hepatoma model. Cell Res (2008) 18:500–7. doi: 10.1038/cr.2008.40
24. Lu L, Chen G, Yang J, Ma Z, Yang Y, Hu Y, et al. Bone marrow mesenchymal stem cells suppress growth and promote the apoptosis of glioma U251 cells through downregulation of the PI3K/AKT signaling pathway. BioMed Pharmacother (2019) 112:108625. doi: 10.1016/j.biopha.2019.108625
25. Ma F, Chen D, Chen F, Chi Y, Han Z, Feng X, et al. Human umbilical cord mesenchymal stem cells promote breast cancer metastasis by interleukin-8- and interleukin-6-dependent induction of CD44(+)/CD24(-) cells. Cell Transplant (2015) 24:2585–99. doi: 10.3727/096368915x687462
26. Bian ZY, Fan QM, Li G, Xu WT, Tang TT. Human mesenchymal stem cells promote growth of osteosarcoma: involvement of interleukin-6 in the interaction between human mesenchymal stem cells and Saos-2. Cancer Sci (2010) 101:2554–60. doi: 10.1111/j.1349-7006.2010.01731.x
27. Xue C, Gao Y, Sun Z, Li X, Zhang M, Yang Y, et al. Mesenchymal stem cells derived from adipose tissue accelerate the progression of colon cancer by inducing a MTCAF phenotype via ICAM1/STAT3/AKT axis. Front Oncol (2022) 12:837781. doi: 10.3389/fonc.2022.837781
28. Xue C, Gao Y, Li X, Zhang M, Yang Y, Han Q, et al. Mesenchymal stem cells derived from adipose accelerate the progression of colon cancer by inducing a MT-CAFs phenotype via TRPC3/NF-KB axis. Stem Cell Res Ther (2022) 13:335. doi: 10.1186/s13287-022-03017-5
29. Babajani A, Soltani P, Jamshidi E, Farjoo MH, Niknejad H. Recent advances on drug-loaded mesenchymal stem cells with anti-neoplastic agents for targeted treatment of cancer. Front Bioeng Biotechnol (2020) 8:748. doi: 10.3389/fbioe.2020.00748
30. Shao H, Im H, Castro CM, Breakefield X, Weissleder R, Lee H. New technologies for analysis of extracellular vesicles. Chem Rev (2018) 118:1917–50. doi: 10.1021/acs.chemrev.7b00534
31. van Niel G, D'Angelo G, Raposo G. Shedding light on the cell biology of extracellular vesicles. Nat Rev Mol Cell Biol (2018) 19:213–28. doi: 10.1038/nrm.2017.125
32. Shang S, Wang J, Chen S, Tian R, Zeng H, Wang L, et al. Exosomal miRNA-1231 derived from bone marrow mesenchymal stem cells inhibits the activity of pancreatic cancer. Cancer Med (2019) 8:7728–40. doi: 10.1002/cam4.2633
33. Bagheri E, Abnous K, Farzad SA, Taghdisi SM, Ramezani M, Alibolandi M. Targeted doxorubicin-loaded mesenchymal stem cells-derived exosomes as a versatile platform for fighting against colorectal cancer. Life Sci (2020) 261:118369. doi: 10.1016/j.lfs.2020.118369
34. Liang L, Zhao L, Wang Y, Wang Y. Treatment for hepatocellular carcinoma is enhanced when norcantharidin is encapsulated in exosomes derived from bone marrow mesenchymal stem cells. Mol Pharm (2021) 18:1003–13. doi: 10.1021/acs.molpharmaceut.0c00976
35. Zhang Z, Guo X, Guo X, Yu R, Qian M, Wang S, et al. MicroRNA-29a-3p delivery via exosomes derived from engineered human mesenchymal stem cells exerts tumour suppressive effects by inhibiting migration and vasculogenic mimicry in glioma. Aging (Albany NY) (2021) 13:5055–68. doi: 10.18632/aging.202424
36. Massey AE, Malik S, Sikander M, Doxtater KA, Tripathi MK, Khan S, et al. Clinical implications of exosomes: targeted drug delivery for cancer treatment. Int J Mol Sci (2021) 22. doi: 10.3390/ijms22105278
37. Jafari A, Babajani A, Abdollahpour-Alitappeh M, Ahmadi N, Rezaei-Tavirani M. Exosomes and cancer: from molecular mechanisms to clinical applications. Med Oncol (2021) 38:45. doi: 10.1007/s12032-021-01491-0
38. Tai YL, Chen KC, Hsieh JT, Shen TL. Exosomes in cancer development and clinical applications. Cancer Sci (2018) 109:2364–74. doi: 10.1111/cas.13697
39. de Araujo Farias V, O'Valle F, Serrano-Saenz S, Anderson P, Andrés E, López-Peñalver J, et al. Exosomes derived from mesenchymal stem cells enhance radiotherapy-induced cell death in tumor and metastatic tumor foci. Mol Cancer (2018) 17:122. doi: 10.1186/s12943-018-0867-0
40. Lee JK, Park SR, Jung BK, Jeon YK, Lee YS, Kim MK, et al. Exosomes derived from mesenchymal stem cells suppress angiogenesis by down-regulating VEGF expression in breast cancer cells. PLoS One (2013) 8:e84256. doi: 10.1371/journal.pone.0084256
41. Pakravan K, Babashah S, Sadeghizadeh M, Mowla SJ, Mossahebi-Mohammadi M, Ataei F, et al. MicroRNA-100 shuttled by mesenchymal stem cell-derived exosomes suppresses in vitro angiogenesis through modulating the mTOR/HIF-1α/VEGF signaling axis in breast cancer cells. Cell Oncol (Dordr) (2017) 40:457–70. doi: 10.1007/s13402-017-0335-7
42. Zhang F, Lu Y, Wang M, Zhu J, Li J, Zhang P, et al. Exosomes derived from human bone marrow mesenchymal stem cells transfer miR-222-3p to suppress acute myeloid leukemia cell proliferation by targeting IRF2/INPP4B. Mol Cell Probes (2020) 51:101513. doi: 10.1016/j.mcp.2020.101513
43. Xu YC, Lin YS, Zhang L, Lu Y, Sun YL, Fang ZG, et al. MicroRNAs of bone marrow mesenchymal stem cell-derived exosomes regulate acute myeloid leukemia cell proliferation and apoptosis. Chin Med J (Engl) (2020) 133:2829–39. doi: 10.1097/cm9.0000000000001138
44. Zhu W, Huang L, Li Y, Zhang X, Gu J, Yan Y, et al. Exosomes derived from human bone marrow mesenchymal stem cells promote tumor growth in vivo. Cancer Lett (2012) 315:28–37. doi: 10.1016/j.canlet.2011.10.002
45. Gu H, Ji R, Zhang X, Wang M, Zhu W, Qian H, et al. Exosomes derived from human mesenchymal stem cells promote gastric cancer cell growth and migration via the activation of the Akt pathway. Mol Med Rep (2016) 14:3452–8. doi: 10.3892/mmr.2016.5625
46. Qi J, Zhou Y, Jiao Z, Wang X, Zhao Y, Li Y, et al. Exosomes derived from human bone marrow mesenchymal stem cells promote tumor growth through hedgehog signaling pathway. Cell Physiol Biochem (2017) 42:2242–54. doi: 10.1159/000479998
47. Lin R, Wang S, Zhao RC. Exosomes from human adipose-derived mesenchymal stem cells promote migration through Wnt signaling pathway in a breast cancer cell model. Mol Cell Biochem (2013) 383:13–20. doi: 10.1007/s11010-013-1746-z
48. Wang J, De Veirman K, De Beule N, Maes K, De Bruyne E, Van Valckenborgh E, et al. The bone marrow microenvironment enhances multiple myeloma progression by exosome-mediated activation of myeloid-derived suppressor cells. Oncotarget (2015) 6:43992–4004. doi: 10.18632/oncotarget.6083
49. Ji R, Zhang B, Zhang X, Xue J, Yuan X, Yan Y, et al. Exosomes derived from human mesenchymal stem cells confer drug resistance in gastric cancer. Cell Cycle (2015) 14:2473–83. doi: 10.1080/15384101.2015.1005530
50. Ono M, Kosaka N, Tominaga N, Yoshioka Y, Takeshita F, Takahashi RU, et al. Exosomes from bone marrow mesenchymal stem cells contain a microRNA that promotes dormancy in metastatic breast cancer cells. Sci Signal (2014) 7:ra63. doi: 10.1126/scisignal.2005231
51. Zhou Y, Yamamoto Y, Takeshita F, Yamamoto T, Xiao Z, Ochiya T. Delivery of miR-424-5p via Extracellular Vesicles Promotes the Apoptosis of MDA-MB-231 TNBC Cells in the Tumor Microenvironment. Int J Mol Sci (2021) 22. doi: 10.3390/ijms22020844
52. Vakhshiteh F, Rahmani S, Ostad SN, Madjd Z, Dinarvand R, Atyabi F. Exosomes derived from miR-34a-overexpressing mesenchymal stem cells inhibit in vitro tumor growth: A new approach for drug delivery. Life Sci (2021) 266:118871. doi: 10.1016/j.lfs.2020.118871
53. Shamili FH, Bayegi HR, Salmasi Z, Sadri K, Mahmoudi M, Kalantari M, et al. Exosomes derived from TRAIL-engineered mesenchymal stem cells with effective anti-tumor activity in a mouse melanoma model. Int J Pharm (2018) 549:218–29. doi: 10.1016/j.ijpharm.2018.07.067
54. Rezaie Z, Ardeshirylajimi A, Ashkezari MD. Improved anticancer properties of stem cells derived exosomes by prolonged release from PCL nanofibrous structure. Gene (2018) 665:105–10. doi: 10.1016/j.gene.2018.05.003
55. Melzer C, Ohe JV, Hass R. Anti-tumor effects of exosomes derived from drug-incubated permanently growing human MSC. Int J Mol Sci (2020) 21. doi: 10.3390/ijms21197311
56. Del Fattore A, Luciano R, Saracino R, Battafarano G, Rizzo C, Pascucci L, et al. Differential effects of extracellular vesicles secreted by mesenchymal stem cells from different sources on glioblastoma cells. Expert Opin Biol Ther (2015) 15:495–504. doi: 10.1517/14712598.2015.997706
57. Yao X, Mao Y, Wu D, Zhu Y, Lu J, Huang Y, et al. Exosomal circ_0030167 derived from BM-MSCs inhibits the invasion, migration, proliferation and stemness of pancreatic cancer cells by sponging miR-338-5p and targeting the Wif1/Wnt8/β-catenin axis. Cancer Lett (2021) 512:38–50. doi: 10.1016/j.canlet.2021.04.030
58. Beermann J, Piccoli MT, Viereck J, Thum T. Non-coding RNAs in development and disease: background, mechanisms, and therapeutic approaches. Physiol Rev (2016) 96:1297–325. doi: 10.1152/physrev.00041.2015
59. Esteller M. Non-coding RNAs in human disease. Nat Rev Genet (2011) 12:861–74. doi: 10.1038/nrg3074
60. Yan H, Bu P. Non-coding RNA in cancer. Essays Biochem (2021) 65:625–39. doi: 10.1042/ebc20200032
61. Saw PE, Xu X, Chen J, Song EW. Non-coding RNAs: the new central dogma of cancer biology. Sci China Life Sci (2021) 64:22–50. doi: 10.1007/s11427-020-1700-9
62. van Rooij E, Kauppinen S. Development of microRNA therapeutics is coming of age. EMBO Mol Med (2014) 6:851–64. doi: 10.15252/emmm.201100899
63. Matsui M, Corey DR. Non-coding RNAs as drug targets. Nat Rev Drug Discovery (2017) 16:167–79. doi: 10.1038/nrd.2016.117
64. Kreutzer FP, Fiedler J, Thum T. Non-coding RNAs: key players in cardiac disease. J Physiol (2020) 598:2995–3003. doi: 10.1113/jp278131
65. Das T, Das TK, Khodarkovskaya A, Dash S. Non-coding RNAs and their bioengineering applications for neurological diseases. Bioengineered (2021) 12:11675–98. doi: 10.1080/21655979.2021.2003667
66. Bella F, Campo S. Long non-coding RNAs and their involvement in bipolar disorders. Gene (2021) 796-797:145803. doi: 10.1016/j.gene.2021.145803
67. Ghafouri-Fard S, Poulet C, Malaise M, Abak A, Mahmud Hussen B, Taheriazam A, et al. The emerging role of non-coding RNAs in osteoarthritis. Front Immunol (2021) 12:773171. doi: 10.3389/fimmu.2021.773171
68. Yan T, Wang X, Zhou D, Qiu H, Zhang J, Yang W. Circ_0003489 facilitates multiple myeloma progression by targeting miR-433-3p/PBX3 axis. Hematology (2022) 27:951–9. doi: 10.1080/16078454.2022.2109554
69. Zhang J, Li S, Zhang J, Zhang W, Jiang J, Wu H, et al. Docetaxel resistance-derived LINC01085 contributes to the immunotherapy of hormone-independent prostate cancer by activating the STING/MAVS signaling pathway. Cancer Lett (2022) 545:215829. doi: 10.1016/j.canlet.2022.215829
70. Chen LJ, Wu L, Wang W, Zhai LL, Xiang F, Li WB, et al. Long non−coding RNA 01614 hyperactivates WNT/β−catenin signaling to promote pancreatic cancer progression by suppressing GSK−3β. Int J Oncol (2022) 61. doi: 10.3892/ijo.2022.5406
71. Yang X, Shen Z, Tian M, Lin Y, Li L, Chai T, et al. LncRNA C9orf139 can regulate the progression of esophageal squamous carcinoma by mediating the miR-661/HDAC11 axis. Transl Oncol (2022) 24:101487. doi: 10.1016/j.tranon.2022.101487
72. Taghehchian N, Alemohammad R, Farshchian M, Asoodeh A, Abbaszadegan MR. Inhibitory role of LINC00332 in gastric cancer progression through regulating cell EMT and stemness. Life Sci (2022) 305:120759. doi: 10.1016/j.lfs.2022.120759
73. Bushati N, Cohen SM. microRNA functions. Annu Rev Cell Dev Biol (2007) 23:175–205. doi: 10.1146/annurev.cellbio.23.090506.123406
74. Paul S, Reyes PR, Garza BS, Sharma A. MicroRNAs and child neuropsychiatric disorders: A brief review. Neurochem Res (2020) 45:232–40. doi: 10.1007/s11064-019-02917-y
75. Hammond SM. An overview of microRNAs. Adv Drug Delivery Rev (2015) 87:3–14. doi: 10.1016/j.addr.2015.05.001
76. Alahdal M, Elkord E. Non-coding RNAs in cancer immunotherapy: Predictive biomarkers and targets. Clin Transl Med (2023) 13:e1425. doi: 10.1002/ctm2.1425
77. Deng Y, Julaiti A, Ran W, He Y. Bone marrow mesenchymal stem cells-derived exosomal microRNA-19b-3p targets SOCS1 to facilitate progression of esophageal cancer. Life Sci (2021) 278:119491. doi: 10.1016/j.lfs.2021.119491
78. Xing L, Tang X, Wu K, Huang X, Yi Y, Huan J. LncRNA HAND2-AS1 suppressed the growth of triple negative breast cancer via reducing secretion of MSCs derived exosomal miR-106a-5p. Aging (Albany NY) (2020) 13:424–36. doi: 10.18632/aging.202148
79. Liu XN, Zhang CB, Lin H, Tang XY, Zhou R, Wen HL, et al. microRNA-204 shuttled by mesenchymal stem cell-derived exosomes inhibits the migration and invasion of non-small-cell lung cancer cells via the KLF7/AKT/HIF-1α axis. Neoplasma (2021) 68:719–31. doi: 10.4149/neo_2021_201208N1328
80. Liu J, Zhu M, Tang Q. Human umbilical cord mesenchymal stem cells-derived exosomal microRNA-181a retards nasopharyngeal carcinoma development by mediating KDM5C. J Cancer Res Clin Oncol (2021) 147:2867–77. doi: 10.1007/s00432-021-03684-6
81. Zhang N, Li L, Luo J, Tan J, Hu W, Li Z, et al. Inhibiting microRNA-424 in bone marrow mesenchymal stem cells-derived exosomes suppresses tumor growth in colorectal cancer by upregulating TGFBR3. Arch Biochem Biophys (2021) 709:108965. doi: 10.1016/j.abb.2021.108965
82. Chen Z, Xie Y, Chen W, Li T, Chen X, Liu B. microRNA-6785-5p-loaded human umbilical cord mesenchymal stem cells-derived exosomes suppress angiogenesis and metastasis in gastric cancer via INHBA. Life Sci (2021) 284:119222. doi: 10.1016/j.lfs.2021.119222
83. Li YH, Lv MF, Lu MS, Bi JP. Bone marrow mesenchymal stem cell-derived exosomal MiR-338-3p represses progression of hepatocellular carcinoma by targeting ETS1. J Biol Regul Homeost Agents (2021) 35:617–27. doi: 10.23812/20-638-a
84. Jia Y, Ding X, Zhou L, Zhang L, Yang X. Mesenchymal stem cells-derived exosomal microRNA-139-5p restrains tumorigenesis in bladder cancer by targeting PRC1. Oncogene (2021) 40:246–61. doi: 10.1038/s41388-020-01486-7
85. Shimbo K, Miyaki S, Ishitobi H, Kato Y, Kubo T, Shimose S, et al. Exosome-formed synthetic microRNA-143 is transferred to osteosarcoma cells and inhibits their migration. Biochem Biophys Res Commun (2014) 445:381–7. doi: 10.1016/j.bbrc.2014.02.007
86. He Z, Li W, Zheng T, Liu D, Zhao S. Human umbilical cord mesenchymal stem cells-derived exosomes deliver microRNA-375 to downregulate ENAH and thus retard esophageal squamous cell carcinoma progression. J Exp Clin Cancer Res (2020) 39:140. doi: 10.1186/s13046-020-01631-w
87. Mishra S, Yadav T, Rani V. Exploring miRNA based approaches in cancer diagnostics and therapeutics. Crit Rev Oncol Hematol (2016) 98:12–23. doi: 10.1016/j.critrevonc.2015.10.003
88. Hill M, Tran N. miRNA interplay: mechanisms and consequences in cancer. Dis Model Mech (2021) 14. doi: 10.1242/dmm.047662
89. Ferragut Cardoso AP, Banerjee M, Nail AN, Lykoudi A, States JC. miRNA dysregulation is an emerging modulator of genomic instability. Semin Cancer Biol (2021) 76:120–31. doi: 10.1016/j.semcancer.2021.05.004
90. Ao YQ, Gao J, Jiang JH, Wang HK, Wang S, Ding JY. Comprehensive landscape and future perspective of long noncoding RNAs in non-small cell lung cancer: it takes a village. Mol Ther (2023) 31:3389–413. doi: 10.1016/j.ymthe.2023.09.015
91. Nail HM, Chiu CC, Leung CH, Ahmed MMM, Wang HD. Exosomal miRNA-mediated intercellular communications and immunomodulatory effects in tumor microenvironments. J BioMed Sci (2023) 30:69. doi: 10.1186/s12929-023-00964-w
92. Søkilde R, Newie I, Persson H, Borg Å, Rovira C. Passenger strand loading in overexpression experiments using microRNA mimics. RNA Biol (2015) 12:787–91. doi: 10.1080/15476286.2015.1020270
93. Oveili E, Vafaei S, Bazavar H, Eslami Y, Mamaghanizadeh E, Yasamineh S, et al. The potential use of mesenchymal stem cells-derived exosomes as microRNAs delivery systems in different diseases. Cell Commun Signal (2023) 21:20. doi: 10.1186/s12964-022-01017-9
94. Lu X, Guo H, Wei X, Lu D, Shu W, Song Y, et al. Current status and prospect of delivery vehicle based on mesenchymal stem cell-derived exosomes in liver diseases. Int J Nanomedicine (2023) 18:2873–90. doi: 10.2147/ijn.S404925
95. Dalmizrak A, Dalmizrak O. Mesenchymal stem cell-derived exosomes as new tools for delivery of miRNAs in the treatment of cancer. Front Bioeng Biotechnol (2022) 10:956563. doi: 10.3389/fbioe.2022.956563
96. Naseri Z, Oskuee RK, Forouzandeh-Moghadam M, Jaafari MR. Delivery of LNA-antimiR-142-3p by mesenchymal stem cells-derived exosomes to breast cancer stem cells reduces tumorigenicity. Stem Cell Rev Rep (2020) 16:541–56. doi: 10.1007/s12015-019-09944-w
97. Naseri Z, Oskuee RK, Jaafari MR, Forouzandeh Moghadam M. Exosome-mediated delivery of functionally active miRNA-142-3p inhibitor reduces tumorigenicity of breast cancer in vitro and in vivo. Int J Nanomedicine (2018) 13:7727–47. doi: 10.2147/ijn.S182384
98. Khazaei-Poul Y, Shojaei S, Koochaki A, Ghanbarian H, Mohammadi-Yeganeh S. Evaluating the influence of Human Umbilical Cord Mesenchymal Stem Cells-derived exosomes loaded with miR-3182 on metastatic performance of Triple Negative Breast Cancer cells. Life Sci (2021) 286:120015. doi: 10.1016/j.lfs.2021.120015
99. Ding Y, Cao F, Sun H, Wang Y, Liu S, Wu Y, et al. Exosomes derived from human umbilical cord mesenchymal stromal cells deliver exogenous miR-145-5p to inhibit pancreatic ductal adenocarcinoma progression. Cancer Lett (2019) 442:351–61. doi: 10.1016/j.canlet.2018.10.039
100. Yan T, Wu M, Lv S, Hu Q, Xu W, Zeng A, et al. Exosomes derived from microRNA-512-5p-transfected bone mesenchymal stem cells inhibit glioblastoma progression by targeting JAG1. Aging (Albany NY) (2021) 13:9911–26. doi: 10.18632/aging.202747
101. Sheykhhasan M, Kalhor N, Sheikholeslami A, Dolati M, Amini E, Fazaeli H. Exosomes of Mesenchymal Stem Cells as a Proper Vehicle for Transfecting miR-145 into the Breast Cancer Cell Line and Its Effect on Metastasis. BioMed Res Int (2021) 2021:5516078. doi: 10.1155/2021/5516078
102. Du L, Tao X, Shen X. Human umbilical cord mesenchymal stem cell-derived exosomes inhibit migration and invasion of breast cancer cells via miR-21-5p/ZNF367 pathway. Breast Cancer (2021) 28:829–37. doi: 10.1007/s12282-021-01218-z
103. Peng Y, Croce CM. The role of MicroRNAs in human cancer. Signal Transduct Target Ther (2016) 1:15004. doi: 10.1038/sigtrans.2015.4
104. Xu Y, Shen L, Li F, Yang J, Wan X, Ouyang M. microRNA-16-5p-containing exosomes derived from bone marrow-derived mesenchymal stem cells inhibit proliferation, migration, and invasion, while promoting apoptosis of colorectal cancer cells by downregulating ITGA2. J Cell Physiol (2019) 234:21380–94. doi: 10.1002/jcp.28747
105. Chen HL, Li JJ, Jiang F, Shi WJ, Chang GY. MicroRNA-4461 derived from bone marrow mesenchymal stem cell exosomes inhibits tumorigenesis by downregulating COPB2 expression in colorectal cancer. Biosci Biotechnol Biochem (2020) 84:338–46. doi: 10.1080/09168451.2019.1677452
106. Zhang X, Zhang X, Hu S, Zheng M, Zhang J, Zhao J, et al. Identification of miRNA-7 by genome-wide analysis as a critical sensitizer for TRAIL-induced apoptosis in glioblastoma cells. Nucleic Acids Res (2017) 45:5930–44. doi: 10.1093/nar/gkx317
107. Yu L, Gui S, Liu Y, Qiu X, Zhang G, Zhang X, et al. Exosomes derived from microRNA-199a-overexpressing mesenchymal stem cells inhibit glioma progression by down-regulating AGAP2. Aging (Albany NY) (2019) 11:5300–18. doi: 10.18632/aging.102092
108. Xu H, Zhao G, Zhang Y, Jiang H, Wang W, Zhao D, et al. Mesenchymal stem cell-derived exosomal microRNA-133b suppresses glioma progression via Wnt/β-catenin signaling pathway by targeting EZH2. Stem Cell Res Ther (2019) 10:381. doi: 10.1186/s13287-019-1446-z
109. Liang Y, Zhang D, Li L, Xin T, Zhao Y, Ma R, et al. Exosomal microRNA-144 from bone marrow-derived mesenchymal stem cells inhibits the progression of non-small cell lung cancer by targeting CCNE1 and CCNE2. Stem Cell Res Ther (2020) 11:87. doi: 10.1186/s13287-020-1580-7
110. Wu H, Mu X, Liu L, Wu H, Hu X, Chen L, et al. Bone marrow mesenchymal stem cells-derived exosomal microRNA-193a reduces cisplatin resistance of non-small cell lung cancer cells via targeting LRRC1. Cell Death Dis (2020) 11:801. doi: 10.1038/s41419-020-02962-4
111. Zhang H, Wang J, Ren T, Huang Y, Liang X, Yu Y, et al. Bone marrow mesenchymal stem cell-derived exosomal miR-206 inhibits osteosarcoma progression by targeting TRA2B. Cancer Lett (2020) 490:54–65. doi: 10.1016/j.canlet.2020.07.008
112. Tang M, Wang Q, Wang K, Wang F. Mesenchymal stem cells-originated exosomal microRNA-152 impairs proliferation, invasion and migration of thyroid carcinoma cells by interacting with DPP4. J Endocrinol Invest (2020) 43:1787–96. doi: 10.1007/s40618-020-01406-2
113. Jiang S, Mo C, Guo S, Zhuang J, Huang B, Mao X. Human bone marrow mesenchymal stem cells-derived microRNA-205-containing exosomes impede the progression of prostate cancer through suppression of RHPN2. J Exp Clin Cancer Res (2019) 38:495. doi: 10.1186/s13046-019-1488-1
114. Xie C, Du LY, Guo F, Li X, Cheng B. Exosomes derived from microRNA-101-3p-overexpressing human bone marrow mesenchymal stem cells suppress oral cancer cell proliferation, invasion, and migration. Mol Cell Biochem (2019) 458:11–26. doi: 10.1007/s11010-019-03526-7
115. Lang FM, Hossain A, Gumin J, Momin EN, Shimizu Y, Ledbetter D, et al. Mesenchymal stem cells as natural biofactories for exosomes carrying miR-124a in the treatment of gliomas. Neuro Oncol (2018) 20:380–90. doi: 10.1093/neuonc/nox152
116. Kim R, Lee S, Lee J, Kim M, Kim WJ, Lee HW, et al. Exosomes derived from microRNA-584 transfected mesenchymal stem cells: novel alternative therapeutic vehicles for cancer therapy. BMB Rep (2018) 51:406–11. doi: 10.5483/bmbrep.2018.51.8.105
117. Lou G, Song X, Yang F, Wu S, Wang J, Chen Z, et al. Exosomes derived from miR-122-modified adipose tissue-derived MSCs increase chemosensitivity of hepatocellular carcinoma. J Hematol Oncol (2015) 8:122. doi: 10.1186/s13045-015-0220-7
118. Lou G, Chen L, Xia C, Wang W, Qi J, Li A, et al. MiR-199a-modified exosomes from adipose tissue-derived mesenchymal stem cells improve hepatocellular carcinoma chemosensitivity through mTOR pathway. J Exp Clin Cancer Res (2020) 39:4. doi: 10.1186/s13046-019-1512-5
119. Li T, Wan Y, Su Z, Li J, Han M, Zhou C. Mesenchymal stem cell-derived exosomal microRNA-3940-5p inhibits colorectal cancer metastasis by targeting integrin α6. Dig Dis Sci (2021) 66:1916–27. doi: 10.1007/s10620-020-06458-1
120. Sharif S, Ghahremani MH, Soleimani M. Delivery of exogenous miR-124 to glioblastoma multiform cells by Wharton's Jelly mesenchymal stem cells decreases cell proliferation and migration, and confers chemosensitivity. Stem Cell Rev Rep (2018) 14:236–46. doi: 10.1007/s12015-017-9788-3
121. Yuan Y, Tan S, Wang H, Zhu J, Li J, Zhang P, et al. Mesenchymal stem cell-derived exosomal miRNA-222-3p increases Th1/Th2 ratio and promotes apoptosis of acute myeloid leukemia cells. Anal Cell Pathol (Amst) (2023) 2023:4024887. doi: 10.1155/2023/4024887
122. Hao SC, Ma H, Niu ZF, Sun SY, Zou YR, Xia HC. hUC-MSCs secreted exosomes inhibit the glioma cell progression through PTENP1/miR-10a-5p/PTEN pathway. Eur Rev Med Pharmacol Sci (2019) 23:10013–23. doi: 10.26355/eurrev_201911_19568
123. Xing H, Liang C, Xu X, Sun H, Ma X, Jiang Z. Mesenchymal stroma/stem-like cells of GARP knockdown inhibits cell proliferation and invasion of mouse colon cancer cells (MC38) through exosomes. J Cell Mol Med (2020) 24:13984–90. doi: 10.1111/jcmm.16008
124. Zhao X, Wu X, Qian M, Song Y, Wu D, Zhang W. Knockdown of TGF-β1 expression in human umbilical cord mesenchymal stem cells reverts their exosome-mediated EMT promoting effect on lung cancer cells. Cancer Lett (2018) 428:34–44. doi: 10.1016/j.canlet.2018.04.026
125. Li H, Yang C, Shi Y, Zhao L. Exosomes derived from siRNA against GRP78 modified bone-marrow-derived mesenchymal stem cells suppress Sorafenib resistance in hepatocellular carcinoma. J Nanobiotechnology (2018) 16:103. doi: 10.1186/s12951-018-0429-z
126. TaNiue K, Akimitsu N. The functions and unique features of lncRNAs in cancer development and tumorigenesis. Int J Mol Sci (2021) 22. doi: 10.3390/ijms22020632
127. Chi Y, Wang D, Wang J, Yu W, Yang J. Long non-coding RNA in the pathogenesis of cancers. Cells (2019) 8. doi: 10.3390/cells8091015
128. McCabe EM, Rasmussen TP. lncRNA involvement in cancer stem cell function and epithelial-mesenchymal transitions. Semin Cancer Biol (2021) 75:38–48. doi: 10.1016/j.semcancer.2020.12.012
129. Raei N, Safaralizadeh R, Hesseinpourfeizi M, Yazdanbod A, Pourfarzi F, Latifi-Navid S. Crosstalk between lncRNAs and miRNAs in gastrointestinal cancer drug resistance. Life Sci (2021) 284:119933. doi: 10.1016/j.lfs.2021.119933
130. Fang Y, Fullwood MJ. Roles, functions, and mechanisms of long non-coding RNAs in cancer. Genomics Proteomics Bioinf (2016) 14:42–54. doi: 10.1016/j.gpb.2015.09.006
131. Lei M, Zheng G, Ning Q, Zheng J, Dong D. Translation and functional roles of circular RNAs in human cancer. Mol Cancer (2020) 19:30. doi: 10.1186/s12943-020-1135-7
132. Yu T, Wang Y, Fan Y, Fang N, Wang T, Xu T, et al. CircRNAs in cancer metabolism: a review. J Hematol Oncol (2019) 12:90. doi: 10.1186/s13045-019-0776-8
133. Kristensen LS, Jakobsen T, Hager H, Kjems J. The emerging roles of circRNAs in cancer and oncology. Nat Rev Clin Oncol (2022) 19:188–206. doi: 10.1038/s41571-021-00585-y
134. Wang Y, Liu J, Ma J, Sun T, Zhou Q, Wang W, et al. Exosomal circRNAs: biogenesis, effect and application in human diseases. Mol Cancer (2019) 18:116. doi: 10.1186/s12943-019-1041-z
135. Jain S, Pathak K, Vaidya A. Molecular therapy using siRNA: Recent trends and advances of multi target inhibition of cancer growth. Int J Biol Macromol (2018) 116:880–92. doi: 10.1016/j.ijbiomac.2018.05.077
136. Pushparaj PN, Aarthi JJ, Manikandan J, Kumar SD. siRNA, miRNA, and shRNA: in vivo applications. J Dent Res (2008) 87:992–1003. doi: 10.1177/154405910808701109
137. Lee SJ, Kim MJ, Kwon IC, Roberts TM. Delivery strategies and potential targets for siRNA in major cancer types. Adv Drug Delivery Rev (2016) 104:2–15. doi: 10.1016/j.addr.2016.05.010
138. Loebinger MR, Janes SM. Stem cells as vectors for antitumour therapy. Thorax (2010) 65:362–9. doi: 10.1136/thx.2009.128025
139. Shams F, Pourjabbar B, Hashemi N, Farahmandian N, Golchin A, Nuoroozi G, et al. Current progress in engineered and nano-engineered mesenchymal stem cells for cancer: From mechanisms to therapy. BioMed Pharmacother (2023) 167:115505. doi: 10.1016/j.biopha.2023.115505
140. Rehman FU, Liu Y, Zheng M, Shi B. Exosomes based strategies for brain drug delivery. Biomaterials (2022) 293:121949. doi: 10.1016/j.biomaterials.2022.121949
141. Tan A, de la Peña H, Seifalian AM. The application of exosomes as a nanoscale cancer vaccine. Int J Nanomedicine (2010) 5:889–900. doi: 10.2147/ijn.S13402
142. Pascucci L, Coccè V, Bonomi A, Ami D, Ceccarelli P, Ciusani E, et al. Paclitaxel is incorporated by mesenchymal stromal cells and released in exosomes that inhibit in vitro tumor growth: a new approach for drug delivery. J Control Release (2014) 192:262–70. doi: 10.1016/j.jconrel.2014.07.042
143. Wei H, Chen J, Wang S, Fu F, Zhu X, Wu C, et al. A nanodrug consisting of doxorubicin and exosome derived from mesenchymal stem cells for osteosarcoma treatment in vitro. Int J Nanomedicine (2019) 14:8603–10. doi: 10.2147/ijn.S218988
144. Wei H, Chen F, Chen J, Lin H, Wang S, Wang Y, et al. Mesenchymal stem cell derived exosomes as nanodrug carrier of doxorubicin for targeted osteosarcoma therapy via SDF1-CXCR4 axis. Int J Nanomedicine (2022) 17:3483–95. doi: 10.2147/ijn.S372851
145. Le Saux S, Aarrass H, Lai-Kee-Him J, Bron P, Armengaud J, Miotello G, et al. Post-production modifications of murine mesenchymal stem cell (mMSC) derived extracellular vesicles (EVs) and impact on their cellular interaction. Biomaterials (2020) 231:119675. doi: 10.1016/j.biomaterials.2019.119675
146. Chen M, Li Y, Ma N, Zang J. Mesenchymal stem cell−derived exosomes loaded with 5−Fu against cholangiocarcinoma in vitro. Mol Med Rep (2022) 25. doi: 10.3892/mmr.2022.12729
147. Jafari D, Shajari S, Jafari R, Mardi N, Gomari H, Ganji F, et al. Designer exosomes: A new platform for biotechnology therapeutics. BioDrugs (2020) 34:567–86. doi: 10.1007/s40259-020-00434-x
148. Haney MJ, Klyachko NL, Zhao Y, Gupta R, Plotnikova EG, He Z, et al. Exosomes as drug delivery vehicles for Parkinson's disease therapy. J Control Release (2015) 207:18–30. doi: 10.1016/j.jconrel.2015.03.033
149. Altanerova U, Babincova M, Babinec P, Benejova K, Jakubechova J, Altanerova V, et al. Human mesenchymal stem cell-derived iron oxide exosomes allow targeted ablation of tumor cells via magnetic hyperthermia. Int J Nanomedicine (2017) 12:7923–36. doi: 10.2147/ijn.S145096
150. Doering CB, Archer D, Spencer HT. Delivery of nucleic acid therapeutics by genetically engineered hematopoietic stem cells. Adv Drug Delivery Rev (2010) 62:1204–12. doi: 10.1016/j.addr.2010.09.005
151. Dunbar CE, High KA, Joung JK, Kohn DB, Ozawa K, Sadelain M. Gene therapy comes of age. Science (2018) 359. doi: 10.1126/science.aan4672
152. Hu X, Zhou W, Pi R, Zhao X, Wang W. Genetically modified cancer vaccines: Current status and future prospects. Med Res Rev (2022) 42:1492–517. doi: 10.1002/med.21882
153. Altanerova U, Jakubechova J, Benejova K, Priscakova P, Pesta M, Pitule P, et al. Prodrug suicide gene therapy for cancer targeted intracellular by mesenchymal stem cell exosomes. Int J Cancer (2019) 144:897–908. doi: 10.1002/ijc.31792
154. You B, Jin C, Zhang J, Xu M, Xu W, Sun Z, et al. MSC-derived extracellular vesicle-delivered L-PGDS inhibit gastric cancer progression by suppressing cancer cell stemness and STAT3 phosphorylation. Stem Cells Int (2022) 2022:9668239. doi: 10.1155/2022/9668239
155. Kordelas L, Rebmann V, Ludwig AK, Radtke S, Ruesing J, Doeppner TR, et al. MSC-derived exosomes: a novel tool to treat therapy-refractory graft-versus-host disease. Leukemia (2014) 28:970–3. doi: 10.1038/leu.2014.41
156. Nassar W, El-Ansary M, Sabry D, Mostafa MA, Fayad T, Kotb E, et al. Umbilical cord mesenchymal stem cells derived extracellular vesicles can safely ameliorate the progression of chronic kidney diseases. Biomater Res (2016) 20:21. doi: 10.1186/s40824-016-0068-0
157. Lou G, Chen Z, Zheng M, Liu Y. Mesenchymal stem cell-derived exosomes as a new therapeutic strategy for liver diseases. Exp Mol Med (2017) 49:e346. doi: 10.1038/emm.2017.63
158. Park HS, Cetin E, Siblini H, Seok J, Alkelani H, Alkhrait S, et al. Therapeutic potential of mesenchymal stem cell-derived extracellular vesicles to treat PCOS. Int J Mol Sci (2023) 24. doi: 10.3390/ijms241311151
159. Yang D, Zhang W, Zhang H, Zhang F, Chen L, Ma L, et al. Progress, opportunity, and perspective on exosome isolation - efforts for efficient exosome-based theranostics. Theranostics (2020) 10:3684–707. doi: 10.7150/thno.41580
160. Reza A, Choi YJ, Yasuda H, Kim JH. Human adipose mesenchymal stem cell-derived exosomal-miRNAs are critical factors for inducing anti-proliferation signalling to A2780 and SKOV-3 ovarian cancer cells. Sci Rep (2016) 6:38498. doi: 10.1038/srep38498
161. Zhang X, Sai B, Wang F, Wang L, Wang Y, Zheng L, et al. Hypoxic BMSC-derived exosomal miRNAs promote metastasis of lung cancer cells via STAT3-induced EMT. Mol Cancer (2019) 18:40. doi: 10.1186/s12943-019-0959-5
Keywords: mesenchymal stem cells, exosomes, tumor-targeted therapy, anti-cancer, drug delivery
Citation: Song Y, Song Q, Hu D, Sun B, Gao M, Liang X, Qu B, Suo L, Yin Z and Wang L (2024) The potential applications of artificially modified exosomes derived from mesenchymal stem cells in tumor therapy. Front. Oncol. 13:1299384. doi: 10.3389/fonc.2023.1299384
Received: 22 September 2023; Accepted: 15 December 2023;
Published: 04 January 2024.
Edited by:
Yong Sang Song, Seoul National University, Republic of KoreaReviewed by:
Zoi Piperigkou, University of Patras, GreeceKanagaraj Palaniyandi, SRM Institute of Science and Technology, India
Amirhesam Babajani, Iran University of Medical Sciences, Iran
Majid Mossahebi, Wenzhou Medical University, China
Copyright © 2024 Song, Song, Hu, Sun, Gao, Liang, Qu, Suo, Yin and Wang. This is an open-access article distributed under the terms of the Creative Commons Attribution License (CC BY). The use, distribution or reproduction in other forums is permitted, provided the original author(s) and the copyright owner(s) are credited and that the original publication in this journal is cited, in accordance with accepted academic practice. No use, distribution or reproduction is permitted which does not comply with these terms.
*Correspondence: Liming Wang, d2FuZ2JjYzI1OUAxNjMuY29t; Zeli Yin, eWluemVsaTE5OTNAMTYzLmNvbQ==
†These authors have contributed equally to this work and share first authorship