- 1Department of Obstetrics and Gynecology, The Second Hospital of Jilin University, Changchun, China
- 2Department of Hepato-Biliary-Pancreatic Surgery, The Second Hospital of Jilin University, Changchun, China
The sustenance of human life activities depends on copper, which also serves as a crucial factor for vital enzymes. Under typical circumstances, active homeostatic mechanisms keep the intracellular copper ion concentration low. Excess copper ions cause excessive cellular respiration, which causes cytotoxicity and cell death as levels steadily rise above a threshold. It is a novel cell death that depends on mitochondrial respiration, copper ions, and regulation. Cuproptosis is now understood to play a role in several pathogenic processes, including inflammation, oxidative stress, and apoptosis. Copper death is a type of regulatory cell death(RCD).Numerous diseases are correlated with the development of copper homeostasis imbalances. One of the most popular areas of study in the field of cancer is cuproptosis. It has been discovered that cancer angiogenesis, proliferation, growth, and metastasis are all correlated with accumulation of copper ions. Copper ion concentrations can serve as a crucial marker for cancer development. In order to serve as a reference for clinical research on the product, diagnosis, and treatment of cancer, this paper covers the function of copper ion homeostasis imbalance in malignant cancers and related molecular pathways.
1 Introduction
Cell death plays an important role in maintaining normal body homeostasis by inhibiting the uncontrolled proliferation of tumor cells and other biological processes. Cell death includes RCD and non-RCD (1). RCD is a genetically determined cell-active programmed death, including apoptosis, iron death, pyroptosis, necroptosis, etc., which can be induced by inducing the generation of Reactive Oxygen Species (ROS), regulating protein ubiquitylation, acetylation, and other functionally regulating cell death (2) (Table 1). Tsvetkov et al. reported a new mechanism of cell death different from the known ones and named it cuproptosis. Cuproptosis is closely related to cellular mitochondrial respiration: excess intracellular copper can be transported to the mitochondria via ion carriers and bind directly to the lipoylation component of the mitochondrial respiratory tricarboxylic acid cycle (TCA). Copper ions can interfere with iron-sulfur clusters, which in turn causes lipoylation protein aggregation and iron-sulfur cluster protein loss, inducing proteotoxic stress and ultimately cell death (3, 4). Previous findings have shown that, compared with normal human serum and tissues, copper ions are found at higher levels in the serum and tumor tissues of patients with a variety of human malignant tumors (5). Cuproptosis further reinforces the importance of cell death in tumorigenesis and progression and reveals the mechanisms of malignant tumorigenesis and progression, which provides a more theoretical basis for the search for new therapeutic strategies (6) (Figure 1).
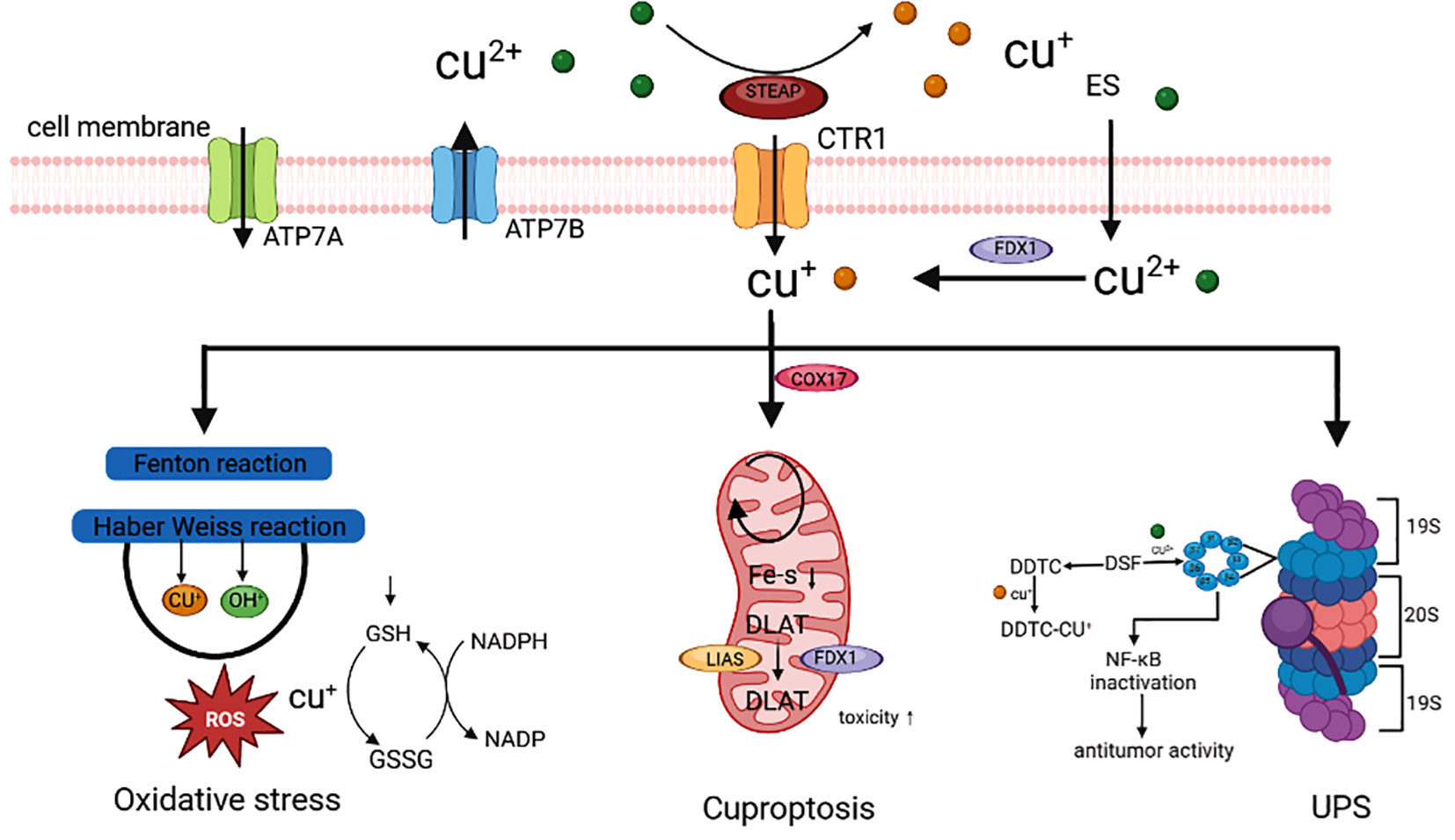
Figure 1 Cuproptosis is involved in the occurrence and development of cancer (3).
2 Copper
One of the most essential heavy metals in the human body is copper (7). Copper is also a significant cofactor in biological redox reactions and is involved in various biosynthetic processes in the body (8). Food is the primary source of copper intake, and both Cu+ and Cu2+ forms of copper can be found in the human body. In the human body, the copper ion is present as Cu2+, which is then converted to Cu+ by reductase after binding to the divalent metal transporter 1 (DMT1), which then enters the cell after binding to the transmembrane copper transporter 1 (CTR1) (9, 10). The Cu+ bound copper proteins enter the bloodstream through particular organelles and are then transported to the tissues and organs where they are required (11). A sufficient amount of copper in cells is essential for life activities as a catalytic cofactor for enzymes involved in energy conversion, iron collection, oxygen transport, and intracellular oxidative metabolism (12). Copper levels in the human body are in dynamic balance under physiological settings. If copper homeostasis is disturbed in an organism, some issues are brought about, including the induction of cell death and the suppression of angiogenesis (13, 14). Iron intake, antioxidant activity, and mitochondrial respiration are all highly dependent on copper (15). Copper plays a crucial role in cell signaling, a biological process that regulates the environment inside the body’s cells (16).
3 Homeostatic regulation of copper
The concentration of copper in normal cells is very low. It mainly prevents the accumulation of harmful intracellular free copper through the Dynamic equilibrium mechanism across concentration gradients, thus maintaining the homeostasis of copper in cells (17). In eukaryotic cells, mitochondria are important organelles for copper storage and mobilization; Cytochrome C oxidase copper chaperone protein 17 located in the gap between the cytoplasm and mitochondrial membrane can bind Cu+, carry Cu+ from the cytoplasm to the mitochondrial gap, and transmit it to cytochrome oxidase deficient homolog 1, transfer to the cytochrome C oxidase II and I subunits to activate enzyme activity in the respiratory chain. Regulating the expression of copper Ion channel proteins can affect the transport and metabolism of copper ions (18). Copper signals can be inhibited by copper-selective chelating agents, activated by metal ion carriers to increase copper levels, or redistributed spatially and temporally to cells and subcells (19). Ceruloplasmin (CP) is the main protein carrier for exchangeable copper in mammalian plasma, with the functions of copper translocation and metal chaperone, jointly maintaining appropriate intracellular copper bioavailability and ensuring the metallization of copper-dependent enzymes (20, 21). CP is a multicopper oxidase that initially binds to most of the copper output from liver cells to the systemic circulation, and enters the secretion pathway through copper transport driven by ATP7B. Due to the rapid degradation of ceruloplasmin when not bound to metals, the concentration of ceruloplasmin in plasma can serve as a biomarker for copper deficiency in the body. Considered the primary transporter of copper ions entering cells, solute carrier family 31 member 1 (SLC31A1) is a member of the CTR family with an extracellular copper binding domain. It is a high-affinity transporter protein for reduced Cu+ and has high expression levels in human cancer tissues (22). However, copper’s natural redox properties make it advantageous and potentially harmful to cells (23). Low copper ion concentrations have been linked to albinism, osteoporosis, and other illnesses (24). Because copper is also found in the brain’s copper protein in the central nervous system, a copper deficiency can impact brain development, demonstrating copper’s vital role in the body (25, 26).
4 Cancer prevention and control
Cancer is a major global public health problem, which seriously threatens human health, and incidence rate and mortality are rising. The International Agency for Research on Cancer (IARC) has released a 2020 global cancer statistics report, with approximately 19.3 million new cancer cases worldwide and nearly 10 million deaths from cancer. Female breast cancer has surpassed lung cancer to become the cancer with the highest incidence rate, accounting for 11.7% of all new cancer cases, followed by lung cancer (11.4%), rectal cancer (10.0%), prostate cancer (7.3%) and gastric cancer (5.6%). Lung cancer is the leading cause of death among all cancers, accounting for 18% of all cancer deaths. The second were rectal cancer (9.4%), liver cancer (8.3%), gastric cancer (7.7%) and female breast cancer (6.9%). Compared to 2018, there has been an increase in data, resulting in huge population losses and a heavy economic and medical burden. As the population ages, this trend will become more apparent. It is expected that there will be 28.4 million new cases of cancer in 2040, an increase of 47% compared to 2020. This result suggests that promoting cancer prevention and control measures and conducting early diagnosis and treatment of cancer are crucial for global cancer prevention and control.
5 Correlation between copper and cancers
Direct copper binding to the TCA’s lipoylation component causes proteotoxic stress, which ultimately results in cell death (27). Abnormal accumulation of copper ions can facilitate malignant cell transformation (28). The serum or cancer tissue of patients with various malignancies has been found to contain high copper contents (29). An imbalance in copper alters lipid metabolism, glycolysis, and insulin resistance while affecting mitochondrial respiration. Unbalanced levels of copper activate vascular endothelial growth factor (VEGF), fibroblast Growth Factor2 (FGF2), tumor necrosis factor (TNF), and interleukin (IL) -1, which start ULK1 and ULK2, which regulate autophagy. This promotes angiogenesis, enabling cancer cell proliferation and spread (30). Human angiogenesis is the result of the combined action of multiple small molecules such as VEGF, FGF2, IL-1, IL-6, and IL-8. Research has shown that copper can stimulate angiogenesis by directly binding to angiopoietin or by binding to HIF-1 to activate these small molecules. Abnormally elevated copper within cells can activate autophagy related ULK1 and ULK1 dependent signaling pathways to induce autophagy, thereby inhibiting copper induced cell apoptosis. Both copper ion carriers and copper chelators are regarded as therapeutically beneficial treatment agents for conditions linked to copper homeostasis and are often used. Using biological markers in copper ion carrier clinical trials will be crucial for creating copper-targeted treatment approaches. The Ubiquitin Proteasome System (UPS), copper deficiency-induced angiogenesis inhibition in cancer cells, and cuproptosis, as identified by recent studies, are the primary mechanisms by which copper has been shown to cause cancer cell death (31).
5.1 Catalyzing oxidative stress
The death of normal or cancer cells results from oxidative stress caused by altered oxidative-antioxidant equilibrium in vivo and is primarily characterized by high ROS concentrations (32, 33). The Cu+ in the body maintains the overall copper homeostasis through the absorption and excretion of copper. Excessive copper drives the Fenton reaction, producing a large amount of ROS, causing protein oxidation, DNA damage, nuclear damage, and dysfunction of mitochondria and various enzymes. Oxidative stress caused by copper primarily manifests itself in two ways. ROS can directly oxidize and cleave some copper complexes through the Fenton reaction, leading to necrotic apoptosis and toxic damage to cancer cells (34). Copper also mediates the synthesis of the most active - OH, which causes elevated ROS concentrations in cancer cells and kills cancer cells. Copper can deplete the antioxidant glutathione (GSH) by oxidizing reduced GSH to oxidized glutathione disulfide (GSSG), which disrupts the GSH-related antioxidant defence system and reduces the system’s ability to scavenge highly reactive -OH, which causes cancer cells to die (35). Copper complexes can induce apoptosis and autophagy through mitochondrial malfunction caused by oxidative stress because copper-induced high quantities of ROS are likewise fatal to mitochondria (36, 37). According to studies, the copper chelator elesclomol (ES), a highly lipophilic copper-binding molecule that chelates extracellular Cu2+, forms an ES-Cu2+ complex that transports copper to mitochondria for redox reactions and causes oxidative stress, which causes cancer cells to undergo apoptosis (38).
5.2 UPS
One of the mechanisms for the breakdown of many proteins in the human body is the UPS (39). UPS is essential in cancer cell growth, apoptosis, angiogenesis, and metastasis. Cu2+ can inhibit the proteasome through direct binding, according to studies (40). Disulfiram (DSF) (41) and other copper complexes have been employed as proteasome inhibitors in cancer treatment. A promising anticancer drug, DSF is an acetaldehyde dehydrogenase inhibitor that binds to Cu2+ to kill cancer cells (42). Diethyldithiocarbomate (DDTC) is similarly quickly formed in vivo from DSF (43, 44). It has been discovered that DDTC can form a dinuclear complex with Cu+, or DDTC-Cu+ and that this copper complex causes an accumulation of ubiquitinated proteins, an increase in p27, and inhibition of nuclear factor-kappa B (NF-κB) expression, which inhibits the growth of cancer cells and the activity of the proteasome both in vivo and in vitro. As a crucial transcription factor, NF-κB is vital for cell growth, invasion, metastasis, and angiogenesis (45). Cu2+ dramatically lowers the cancer cells’ bortezomib resistance, making its anticancer activities appear more promising. A new approach to conventional ubiquitin-proteasome inhibitor anticancer treatment is provided by copper complex-targeted UPS (46).
5.3 Inhibiting angiogenesis
Malignant angiogenesis encourages cancer cell growth, invasion, and metastasis since neoangiogenesis is the first stage of cancer proliferation and metastasis (47). The main component in stimulating angiogenesis is copper, which can directly promote endothelial cell migration, expansion, and the production of fibronectin (48). Hypoxia-inducible factor (HIF-1) can bind to copper and activate essential elements that control angiogenesis (49, 50). Angiogenin is a molecule that copper attaches to, stimulating endothelial cells to initiate angiogenesis (51). Copper deficiency will shut off the angiogenic switch, stop endothelial cells from proliferating, and stop the cell cycle. A novel therapeutic approach used in the treatment of cancers is copper depletion to prevent cancer angiogenesis (52). Tetrathiomolybdate (TTM), which inhibits cancer angiogenesis by reacting with copper ions to generate insoluble copper-molybdenum-sulfur clusters, is receiving much attention in cancer therapy (53, 54). TTM has shown good promise when combined with anti-cancer medications as an adjuvant. Since copper-mediated anti-angiogenic effects can block blood vessels required for cancer growth and metastasis and reshape the cancer immune microenvironment, immune checkpoint inhibitors (ICI) can promote vascular normalization, making the combination of immunotherapy with copper complexes a new target for anticancer therapy (55), which is vital in reducing cancer cell proliferation and inducing cell death (56, 57).
6 Cuproptosis
6.1 Definition of cuproptosis
Apoptosis, scorch death, autophagy, necroptosis, and metal-induced iron death are the principal types of cell death that are now understood (58, 59). Although the human body needs heavy metal ions, too little or too much can cause cell death (60). Cuproptosis, a recently identified unique form of cell death, is copper-dependent, changeable, and intricately linked to mitochondrial respiration (61). Tsvetkov et al. put out this idea. The researchers first tested 489 distinct cell types utilizing carriers for copper ions and showed that too many accumulated copper ions could result in cell death. The researchers discovered a failure to inhibit ES-induced death of non-small cell lung cancer A549 cells and NCIH2030 cells using all known inhibitors of cell death modalities after targeted knockdown of the BCL2-Associated X (BAX) and Recombinant Bcl2 Antagonist/Killer 1 (BAK1) genes. This suggests that ES-induced cancer cell death differs from known cell death mechanisms. They therefore proposed the name “cuproptosis” for this novel cell death process. Cuproptosis is a unique RCD route with a different lethal tool from oxidative stress-related cell death (62). Research shows that cuproptosis plays an important role in the occurrence and progress of many diseases, including cancer, atherosclerosis, rheumatoid arthritis, acute liver injury, novel coronavirus pneumonia, Wilson disease, Alzheimer’s disease, Parkinson’s disease etc.
6.2 Mechanism of cuproptosis
Tsvetkov et al. discovered that ES-induced cell death might be connected to mitochondrial respiration. Cells relying on glycolysis are around 1000 times more sensitive to copper ion inducers than cells depending on mitochondrial respiration. Conversely, ferredoxin 1 (FDX1) lowers CU2+ to CU+, encouraging lipoylation and aggregation of enzymes responsible for controlling the mitochondrial TCA cycle (63). On the other hand, FDX1 results in the instability of iron-sulfur proteins (Fe-S), which sets off a stress response in the mitochondria and produces cuproptosis (64). In addition to copper ion carriers, copper importers and the ATP-dependent copper transporter 7A (ATP7A) (65) control the intracellular concentration of copper ions to hold the degree of cuproptosis sensitivity (66). The copper chelator GSH, which contains thiols, protects against cuproptosis. The electron transfer chain (ETC) complex inhibitor and the mitochondrial pyruvate carrier (MPC) inhibitor UK5099 prevent ES-induced cuproptosis. cuproptosis brought on by ES (Figure 2).
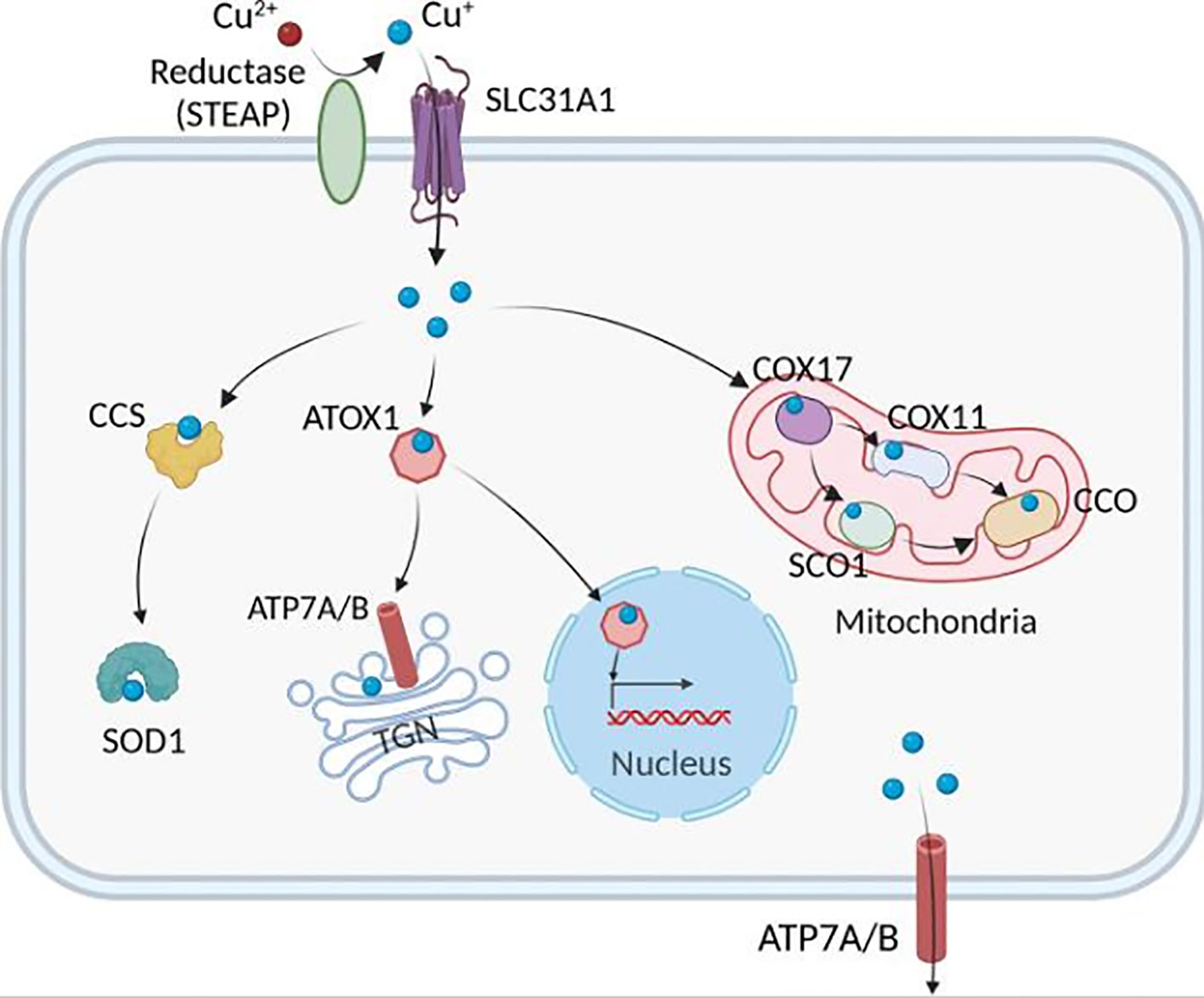
Figure 2 The pathways that mediate cellular Cu metabolism. Extracellular Cu2+ is reduced by the reductase STEAP to Cu+, which is transported into the cell by the Cu transporter CTR1, where it is delivered to cytosolic Cu chaperones such as CCS and SOD1 and then delivered to specific subcellular compartments such as the mitochondria, TGN, and nucleus. In the mitochondria, Cu is involved in the respiratory chain and redox pathways via binding to CCO. In the mitochondrial intermembrane space, COX17 binds to and delivers Cu to either SCO1 or COX11, which transfers Cu to the cytochrome oxidase subunit. In the nucleus, Cu can bind to transcription factors and drive gene expression. Finally, in the TGN, the Cu+-ATPase transporters ATP7A and ATP7B transfer Cu from the cytosol to the TGN lumen, where it activates Cu-dependent enzymes in the secretory pathway. ATOX1, antioxidant 1 copper chaperone; ATP7A and ATP7B, ATPase copper transporter 7A and 7B, respectively; CCO, cytochrome c oxidase; CCS, copper chaperone for superoxide dismutase; COX17, cytochrome c oxidase copper chaperone 17, COX11, cytochrome c oxidase copper chaperone 11, SCO1, synthesis of cytochrome c oxidase 1, SOD1, superoxide dismutase 1, STEAP, the six-transmembrane epithelial antigen of the prostate, SLC31A1, solute carrier family 31 members 1, TGN, trans-Golgi network (17).
6.3 Copper death-related genes
Tsvetkov et al. identified ten copper death-related genes (CRGs) associated explicitly with the cuproptosis metabolic pathway (67), including seven positively regulated genes: FDX1, lipoic acid synthase (LIAS), lipoyl transferase-1 (LIPT1), dihydrolipoamide dehydrogenase (DLD), dihydrolipoamideS-acetyltransferase (DLAT), recombinant Pyruvate dehydrogenase alpha 1 (PDHA1), and pyruvate dehydrogenase (PDHB) (68–70). Metal transcription factor 1 (MTF), glutaminase (GLS), and cyclin-dependent kinase inhibitor 2A (CDKN2A) are three genes that are negatively regulated (71–73). A future study will focus on the precise function of these genes in cuproptosis and the potency of copper toxicity in cancer management (74).
As an ETC carrier, FDX, a member of the small molecular family Fe-S, is frequently utilized in various metabolic activities in living things (75). The decrease of mitochondrial cytochrome and the manufacture of different steroid hormones are both processes that FDX1, an isoform of the FDX family, aids. Cuproptosis, which converts Cu2+ to the more toxic Cu+, causes cytotoxic stress and, as a result, causes cellular cuproptosis, and FDX1 is a co-regulator of this process (76). protein Lysine can be modified post-translationally by a process known as lipoylation, and FDX1 and protein lipoylation share a family relationship (77). A considerable reduction in cellular respiration is caused by the total loss of lipoylation of the DLAT and DLST proteins when FDX1 is knocked down. These findings imply that FDX1, an essential gene in ES-induced copper-dependent cell death and an upstream regulator of protein lipoylation (78), is involved in ES-induced copper-dependent cell death. The lipoylation of mitochondrial proteins was enhanced by copper (79). The degradation of Fe-S cluster proteins by FDX1 may promote copper toxicity. Unrelated to the cuproptosis protein in cancer, FDX1 may act as an oncogene (80). Copper overload causes the aggregation of DLAT, a decrease in Fe-S cluster protein levels, an increase in the number of heat shock protein 70, and protein toxicity stress, leading to the occurrence of cuproptosis.
Three significant genes that code for the mitochondrial lipoic acid pathway are LIAS, LIPT1, and DLD (81). LIAS and LIPT1 are intricately connected to mitochondrial fatty acid production. An essential gene for mitochondrial protein lipoylation is DLAT. By acetylating 6-glucose-phosphate dehydrogenase (6PGD), DLAT increases enzyme activity, boosting nucleic acid synthesis and encouraging the growth of cancer cell lines (82). The oxidative decarboxylation of pyruvate to acetyl-CoA is made possible by the catalytic action of the pyruvate dehydrogenase complex (PDHC), which connects glycolysis, TCA, and oxidative phosphorylation. PDHA1, the PDHC’s active regulatory site The cuproptosis-related gene PDHA1 is crucial for the metabolic transformation of cancer as it regulates the cuproptosis process (83). The level of PDHA1 expression is significantly higher in cervical squamous cell carcinoma tissues than in paraneoplastic squamous epithelial tissues. It is correlated with patient age, the depth of cancer infiltration, pelvic lymph node metastasis, and International Federation of Gynecology and Obstetrics (FIGO) staging (84, 85). In hepatocellular cancer cell lines, overexpression of PDHA1 controls the activity of the TCA enzyme, prevents aerobic glycolysis, and intensifies mitochondrial-regulated apoptotic signaling pathways (86). According to specific research, CDKN2A changes in melanoma patients with CDKN2A loss may serve as a possible signal for anticipating the effectiveness of melanoma immunotherapy (87).
6.3.1 CRGs regulate metabolism in cancer cells
Pathway Enrichment Analysis revealed that glutamate metabolism, TCA, and p53 signaling pathways were primarily enriched in CRGs (88). The amino acids alanine, glutamate, and aspartate all have metabolic signaling pathways connected to glucose metabolism. Metabolic reprogramming involves the p53 signaling pathway (89). TCA, glycolysis, and other processes are part of the central carbon metabolism in cancer (90). Cellular metabolism is closely tied to each of the signaling pathways mentioned above. The sensitivity of cancer cells to therapeutic drugs can be impacted by cellular metabolism, which is essential for cancer development and metastasis. Through control of cancer cell metabolism, CRGs and their related genes may influence the onset and progression of the disease (91).
6.3.2 CRGs and immune cell infiltration
Analysis of immune cell infiltration revealed a strong correlation between the expression of CRGs and the quantity of various immune cells present (92). The presentation of CRGs was negatively connected with plasma cell-like follicular dendritic cells, while the expression of CRGs was favorably correlated with T helper cells. Major mitochondrial autoantigens known as DLAT-associated complexes increase CD4+ T cell and CD8+ T cell reactivity. The cancer CDKN2A was discovered. CRGs may influence cell metabolism in tumor microenvironment (TMT) by controlling several pathways, including cancer cell growth and apoptosis and the proportion of immune cells and other non-cancer cells. As a part of the TMT, immune cell infiltration may also control the growth and remission of cancers (93).
6.4 Cuproptosis affects mitochondrial function
Mitochondrial respiration and cuproptosis are related (94, 95). Since copper ions are primarily stored in mitochondria, an imbalance in intracellular copper metabolism can have cytotoxic effects and lead to disease onset (96). Cuproptosis may be related to altered mitochondrial activity, as evidenced by a considerable reduction in sensitivity to copper degradation when cells are placed in a hypoxic environment and forced to conduct anaerobic glycolysis (97). Both apoptosis and iron death are caused by mitochondrial stress, which can result in a severe decrease in mitochondrial membrane potential. Inhibiting glucose metabolism reduces the malignant potential of cancer cells and makes them more sensitive to copper ion carrier therapy for the treatment of cancers (98). This is because glycolysis is crucial for the proliferation of cancer cells. According to recent research, antioxidants for the mitochondria can considerably lower cuproptosis levels (99). Cuproptosis, therefore, has a high potential for use in anti-cancer therapy due to its superior anti-cancer mechanism (100).
6.5 Cuproptosis regulates the mechanism of cancer damage
The inflammatory response to cellular damage is impacted by cuproptosis (101). By lowering CD45 levels following cerebral ischemia injury, increasing Iba1 immunoreactivity, and changing the shape of Iba1-positive cells to modify the inflammatory response, cuproptosis can be cerebro protective. Cellular carcinogenesis depends on apoptosis (102). Burgering (103) et al. discovered that Cu, Superoxide Dismutase (SOD1) may be impacted by cuproptosis since protein kinase B (Akt) is important in regulating cell survival and death. In the bad signaling pathway, SOD1 might be necessary (104, 105). Protein lipoylation was found to be closely linked with FDX1 abundance in many cancers, and cell lines with high levels of protein lipoylation were highly susceptible to cuproptosis, indicating that copper ion carrier therapy should focus on malignancies with this metabolic profile.
7 Opportunities for cuproptosis cancer treatment
One of the most promising therapeutic modalities is cancer immunotherapy (106), and research on ICI, in particular, has advanced quickly (107). Current therapeutic approaches aim to selectively promote cancer cell death and prevent harming normal cells because cancer is characterized by dysregulated cell death and altered inflammatory responses (108). The need for copper is greater in cancer cells compared to normal cells. Numerous lipoylated mitochondrial proteins are expressed in high concentrations in some cancers with active respiration (109). Cu2+ in humans through bidirectional regulation has become a new target for cancer therapy since elevated copper ion levels stimulate cancer cell proliferation, metastasis, and angiogenesis (110). The fundamental component of current techniques to combat copper-induced apoptosis is using copper ion chelators and copper ion carriers to control the level of copper in vivo to induce apoptosis in cancer cells and produce anti-cancer effects (111). By chelating copper ions, copper ion carriers DSF and ES influence the breakdown of proteins in cancer cells, leading to copper-dependent cancer cell death (112). As a cofactor of cytochrome c oxidase, ES can control intra-mitochondrial copper ion levels, regulating cytochrome c oxidase activity and treating diseases brought on by abnormalities in human copper metabolism (113). Cytochrome C oxidase is a crucial enzyme in the mitochondrial respiratory chain.
In tumor cells, copper deficiency results in inhibition of angiogenesis, elevated ROS levels, proteasome inhibition, and mitochondrial dysfunction (114). The relationship between cuproptosis and cancer can provide new ideas for tumor salvage, and the effect of cuproptosis on cancer cells can be studied in order to reduce the copper content of cancer cells in order to mitigate the damage. When TTM is used as an antitumor drug with platinum-based chemotherapeutic drugs, the chemotherapeutic drugs become more effective and have positive synergistic effects (115). Some researchers have also discovered that Cu2+ regulates the expression of the immune checkpoint protein PD-L1 and promotes tumor immune evasion, demonstrating the potential of copper chelators as anti-tumor immune enhancers. In some cases, it is necessary to artificially increase the cellular copper content in order to alleviate inflammatory damage, and this balance must be continuously investigated in both fundamental and clinical research (116). In light of the fact that the mechanisms by which copper induces tumor cell demise vary between cancer types, their targeting and specificity must be considered in future research.
8 Conclusion
Copper induces tumor cell death in a variety of ways, and “cuproptosis” is a novel RCD mechanism distinct from apoptosis, iron death, autophagy, and programmed necrosis (117, 118). After a series of safety and efficacy tests, it can help translate basic chemical and biological studies of copper into potential clinical therapies and drug candidates, and it has promising applications in the field of tumor therapy. The exact mechanisms by which cuproptosis works in cancer are currently unknown, and a large number of high-quality basic studies are needed to demonstrate a causal relationship between cuproptosis and tumors. The molecular mechanisms of copper toxicity in tumors and the specific modes of evolution of cuproptosis into definitive cell death need to be further elucidated. As research continues, it is believed that more new and relevant targets and drugs will emerge to establish rational and personalized therapeutic strategies and bring new hope for the treatment of cancer patients.
Author contributions
MW: Conceptualization, Data curation, Resources, Software, Writing – original draft, Writing – review & editing. LZ: Investigation, Software, Supervision, Validation, Writing – original draft, Writing – review & editing. SM: Conceptualization, Formal Analysis, Methodology, Project administration, Resources, Writing – original draft, Writing – review & editing. RL: Conceptualization, Data curation, Investigation, Writing – original draft, Writing – review & editing. JL: Methodology, Project administration, Supervision, Validation, Visualization, Writing – review & editing. SY: Formal Analysis, Funding acquisition, Supervision, Validation, Visualization, Writing – original draft.
Funding
The author(s) declare financial support was received for the research, authorship, and/or publication of this article. Funding for this work was provided by the Jilin Provincial Department of Finance Project and Jilin Provincial Development and Reform Commission Health Special Fund (No. 2020SCZT078 and No. 3D5204901429).
Conflict of interest
The authors declare that the research was conducted in the absence of any commercial or financial relationships that could be construed as a potential conflict of interest.
Publisher’s note
All claims expressed in this article are solely those of the authors and do not necessarily represent those of their affiliated organizations, or those of the publisher, the editors and the reviewers. Any product that may be evaluated in this article, or claim that may be made by its manufacturer, is not guaranteed or endorsed by the publisher.
Glossary
References
1. Bao JH, Lu WC, Duan H, Ye YQ, Li JB, Liao WT, et al. Identification of a novel cuproptosis-related gene signature and integrative analyses in patients with lower-grade gliomas. Front Immunol (2022) 13. doi: 10.3389/fimmu.2022.933973
2. Zirngibl M, Assinck P, Sizov A, Caprariello AV, Plemel JR. Oligodendrocyte death and myelin loss in the cuprizone model: an updated overview of the intrinsic and extrinsic causes of cuprizone demyelination. Mol Neurodegeneration (2022) 17(1):34. doi: 10.1186/s13024-022-00538-8
3. Huang YL, Li JZ. Mechanism of copper induced tumor cell death and its research progress in tumor treatment. J Otolaryngology and Ophthalmology, Shandong University (2023), 1–23.
4. Tsvetkov P, Coy S, Petrova B, Dreishpoon M, Verma A, Abdusamad M, et al. Copper induces cell death by targeting lipoylated TCA cycle proteins. Science (2022) 375(6586):1254–+. doi: 10.1126/science.abf0529
5. Wang Z, Jin DK, Zhou SS, Dong NJ, Ji YT, An P, et al. Regulatory roles of copper metabolism and cuproptosis in human cancers. Front Oncol (2023) 13. doi: 10.3389/fonc.2023.1123420
6. Aishajiang R, Liu ZS, Wang TJ, Zhou L, Yu D. Recent advances in cancer therapeutic copper-based nanomaterials for anticancer therapy. Molecules (2023) 28(5):2303. doi: 10.3390/molecules28052303
7. Teschke R. Aluminum, arsenic, beryllium, cadmium, chromium, cobalt, copper, iron, lead, mercury, molybdenum, nickel, platinum, thallium, titanium, vanadium, and zinc: molecular aspects in experimental liver injury. Int J Mol Sci (2022) 23(20):12213. doi: 10.3390/ijms232012213
8. Yuan HJ, Xue YT, Liu Y. Cuproptosis, the novel therapeutic mechanism for heart failure: a narrative review. Cardiovasc Diagnosis Ther (2022) 12(5):681–92. doi: 10.21037/cdt-22-214
9. Zhao QW, Qi TG. The implications and prospect of cuproptosis-related genes and copper transporters in cancer progression. Front Oncol (2023) 13. doi: 10.3389/fonc.2023.1117164
10. Nose Y, Wood LK, Kim BE, Prohaska JR, Fry RS, Spears JW, et al. Ctr1 is an apical copper transporter in mammalian intestinal epithelial cells in vivo that is controlled at the level of protein stability. J Biol Chem (2010) 285(42):32385–92. doi: 10.1074/jbc.M110.143826
11. Xu JH, Hu ZA, Cao H, Zhang H, Luo P, Zhang J, et al. Multi-omics pan-cancer study of cuproptosis core gene FDX1 and its role in kidney renal clear cell carcinoma. Front Immunol (2022) 13. doi: 10.3389/fimmu.2022.981764
12. Chakraborty J, Pakrashi S, Sarbajna A, Dutta M, Bandyopadhyay J. Quercetin attenuates copper-induced apoptotic cell death and endoplasmic reticulum stress in SH-SY5Y cells by autophagic modulation. Biol Trace Element Res (2022) 200(12):5022–41. doi: 10.1007/s12011-022-03093-x
13. Li YQ, Huang RX, Li XL, Li XR, Yu DD, Zhang MZ, et al. Decreased expression of pyruvate dehydrogenase A1 predicts an unfavorable prognosis in ovarian carcinoma. Am J Cancer Res (2016) 6(9):2076–87.
14. Qi XC, Wang J, Che XY, Li QL, Li XW, Wang QF, et al. The potential value of cuproptosis (copper-induced cell death) in the therapy of clear cell renal cell carcinoma. Am J Cancer Res (2022) 12(8):3947–66.
15. Guthrie LM, Soma S, Yuan S, Silva A, Zulkifli M, Snavely TC, et al. Elesclomol alleviates Menkes pathology and mortality by escorting Cu to cuproenzymes in mice. Science (2020) 368(6491):620–+. doi: 10.1126/science.aaz8899
16. Agarwal P, Ayton S, Agrawal S, Dhana K, Bennett DA, Barnes LL, et al. Brain copper may protect from cognitive decline and Alzheimer's disease pathology: a community-based study. Mol Psychiatry (2022) 27(10):4307–13. doi: 10.1038/s41380-022-01802-5
17. Chen LY, Min JX, Wang FD. Copper homeostasis and cuproptosis in health and disease. Signal Transduction Targeted Ther (2022) 7(1):378. doi: 10.1038/s41392-022-01229-y
18. Zhu XY, Boulet A, Buckley KM, Phillips CB, Gammon MG, Oldfather LE, et al. Mitochondrial copper and phosphate transporter specificity was defined early in the evolution of eukaryotes. Elife (2021) 10:e64690. doi: 10.7554/eLife.64690
19. Xie JM, Yang YN, Gao YB, He J. Cuproptosis: mechanisms and links with cancers. Mol Cancer (2023) 22(1):46. doi: 10.1186/s12943-023-01732-y
20. Palumbo CS, Schilsky ML. Clinical practice guidelines in Wilson disease. Ann Trans Med (2019) 7(Suppl 2):S65. doi: 10.21037/atm.2018.12.53
21. Shanbhag V, Jasmer-McDonald K, Zhu S, Martin AL, Gudekar N, Khan A, et al. ATP7A delivers copper to the lysyl oxidase family of enzymes and promotes cancerogenesis and metastasis. Proc Natl Acad Sci USA (2019) 116(14):6836–41. doi: 10.1073/pnas.1817473116
22. Long SC, Wang Y, Chen YQ, Fang TS, Yao YB, Fu K. Pan-cancer analysis of cuproptosis regulation patterns and identification of mTOR-target responder in clear cell renal cell carcinoma. Biol Direct (2022) 17(1):28. doi: 10.1186/s13062-022-00340-y
23. Babak MV, Ahn D. Modulation of intracellular copper levels as the mechanism of action of anticancer copper complexes: clinical relevance. Biomedicines (2021) 9(8):852. doi: 10.3390/biomedicines9080852
24. Borobia M, Villanueva-Saz S, de Arcaute MR, Fernandez A, Verde MT, Gonzalez JM, et al. Copper poisoning, a deadly hazard for sheep. Animals (2022) 12(18):2388. doi: 10.3390/ani12182388
25. Stelmashook EV, Alexandrova OP, Genrikhs EE, Novikova SV, Salmina AB, Isaev NK. Effect of zinc and copper ions on cadmium-induced toxicity in rat cultured cortical neurons. J Trace Elements Med Biol (2022) 73:127012. doi: 10.1016/j.jtemb.2022.127012
26. Chen XY, Cai Q, Liang RK, Zhang DJ, Liu X, Zhang MY, et al. Copper homeostasis and copper-induced cell death in the pathogenesis of cardiovascular disease and therapeutic strategies. Cell Death Dis (2023) 14(2):105. doi: 10.1038/s41419-023-05639-w
27. Jiang YC, Huo ZY, Qi XL, Zuo TM, Wu ZH. Copper-induced cancer cell death mechanisms and anticancer theragnostic applications of copper complexes. Nanomedicine (2022) 17(5):303–24. doi: 10.2217/nnm-2021-0374
28. Cobine PA, Moore SA, Leary SC. Getting out what you put in Copper in mitochondria and its impacts on human disease. Biochim Et Biophys Acta-Molecular Cell Res (2021) 1868(1):118867. doi: 10.1016/j.bbamcr.2020.118867
29. Song Q, Zhou R, Shu FP, Fu W. Cuproptosis scoring system to predict the clinical outcome and immune response in bladder cancer. Front Immunol (2022) 13. doi: 10.3389/fimmu.2022.958368
30. Chen X, Kang R, Kroemer G, Tang DL. Broadening horizons: the role of ferroptosis in cancer. Nat Rev Clin Oncol (2021) 18(5):280–96. doi: 10.1038/s41571-020-00462-0
31. Farhan M, Rizvi A. Understanding the prooxidant action of plant polyphenols in the cellular microenvironment of Malignant cells: role of copper and therapeutic implications. Front Pharmacol (2022) 13. doi: 10.3389/fphar.2022.929853
32. Alhasawi MAI, Aatif M, Muteeb G, Alam MW, El Oirdi M, Farhan M. Curcumin and its derivatives induce apoptosis in human cancer cells by mobilizing and redox cycling genomic copper ions. Molecules (2022) 27(21):7410. doi: 10.3390/molecules27217410
33. Farshori NN, Siddiqui MA, Al-Oqail MM, Al-Sheddi ES, Al-Massarani SM, Ahamed M, et al. Copper oxide nanoparticles exhibit cell death through oxidative stress responses in human airway epithelial cells: a mechanistic study. Biol Trace Element Res (2022) 200(12):5042–51. doi: 10.1007/s12011-022-03107-8
34. Gupte A, Mumper RJ. Elevated copper and oxidative stress in cancer cells as a target for cancer treatment. Cancer Treat Rev (2009) 35(1):32–46. doi: 10.1016/j.ctrv.2008.07.004
35. Yip NC, Fombon IS, Liu P, Brown S, Kannappan V, Armesilla AL, et al. Disulfiram modulated ROS-MAPK and NFκB pathways and targeted breast cancer cells with cancer stem cell-like properties. Br J Cancer (2011) 104(10):1564–74. doi: 10.1038/bjc.2011.126
36. Yang D, Xiao PY, Qiu BT, Yu HF, Teng CB. Copper chaperone antioxidant 1: multiple roles and a potential therapeutic target. J Mol Medicine-Jmm (2023) 101(5):527–42. doi: 10.1007/s00109-023-02311-w
37. Nagai M, Vo NH, Ogawa LS, Chimmanamada D, Inoue T, Chu J, et al. The oncology drug elesclomol selectively transports copper to the mitochondria to induce oxidative stress in cancer cells. Free Radical Biol Med (2012) 52(10):2142–50. doi: 10.1016/j.freeradbiomed.2012.03.017
38. Li X, Ma ZS, Mei LH. Cuproptosis-related gene SLC31A1 is a potential predictor for diagnosis, prognosis and therapeutic response of breast cancer. Am J Cancer Res (2022) 12(8):3561–+.
39. Narayanan S, Cai CY, Assaraf YG, Guo HQ, Cui QB, Wei LY, et al. Targeting the ubiquitin-proteasome pathway to overcome anti-cancer drug resistance. Drug Resistance Updates (2020) 48:100663. doi: 10.1016/j.drup.2019.100663
40. Cobine PA, Brady DC. Cuproptosis: Cellular and molecular mechanisms underlying copper-induced cell death. Mol Cell (2022) 82(10):1786–7. doi: 10.1016/j.molcel.2022.05.001
41. Chen D, Cui QZC, Yang HJ, Dou QP. Disulfiram, a clinically used anti-alcoholism drug and copper-binding agent, induces apoptotic cell death in breast cancer cultures and xenografts via inhibition of the proteasome activity. Cancer Res (2006) 66(21):10425–33. doi: 10.1158/0008-5472.CAN-06-2126
42. Oliveri V, Lanza V, Milardi D, Viale M, Maric I, Sgarlata C, et al. Amino- and chloro-8-hydroxyquinolines and their copper complexes as proteasome inhibitors and antiproliferative agents. Metallomics (2017) 9(10):1439–46. doi: 10.1039/C7MT00156H
43. Michalczyk K, Cymbaluk-Ploska A. The role of zinc and copper in gynecological Malignancies. Nutrients (2020) 12(12):3732. doi: 10.3390/nu12123732
44. Skrott Z, Mistrik M, Andersen KK, Friis S, Majera D, Gursky J, et al. Alcohol-abuse drug disulfiram targets cancer via p97 segregate adaptor NPL4. Nature (2017) 552(7684):194. doi: 10.1038/nature25016
45. Han J, Liu L, Yue X, Chang J, Shi W, Hua Y. A binuclear complex constituted by diethyldithiocarbamate and copper(I) functions as a proteasome activity inhibitor in pancreatic cancer cultures and xenografts. Toxicol Appl Pharmacol (2013) 273(3):477–83. doi: 10.1016/j.taap.2013.09.009
46. Hughes RE, Elliott RJR, Li XD, Munro AF, Makda A, Carter RN, et al. Multiparametric high-content cell painting identifies copper ionophores as selective modulators of esophageal cancer phenotypes. ACS Chem Biol (2022) 17(7):1876–89. doi: 10.1021/acschembio.2c00301
47. Yin R, Wang H, Li C, Wang L, Lai S, Yang X, et al. Induction of apoptosis and autosis in cardiomyocytes by the combination of homocysteine and copper via NOX-mediated p62 expression. Cell Death discovery (2022) 8(1):75. doi: 10.1038/s41420-022-00870-4
48. Caro-Ramírez JY, Rivas MG, Gonzalez PJ, Williams PAM, Naso LG, Ferrer EG. Copper(II) cation and bathophenanthroline coordination enhance the therapeutic effects of naringenin against lung cancer cells. BioMetals (2022) 35(5):1059–76. doi: 10.1007/s10534-022-00422-4
49. Agnieszka Z, Maciej K. Hypoxia-inducible factor-1 in physiological and pathophysiological angiogenesis: applications and therapies. BioMed Res Int (2015) 2015:549412. doi: 10.1155/2015/549412
50. Wang J, Luo C, Shan CL, You QC, Lu JY, Elf SN, et al. Inhibition of human copper trafficking by a small molecule significantly attenuates cancer cell proliferation. Nat Chem (2015) 7(12):968–79. doi: 10.1038/nchem.2381
51. Chi H, Peng GG, Wang R, Yang FY, Xie XX, Zhang JH, et al. Cuproptosis programmed-cell-death-related lncRNA signature predicts prognosis and immune landscape in PAAD patients. Cells (2022) 11(21):3436. doi: 10.3390/cells11213436
52. Ge EJ, Bush AI, Casini A, Cobine PA, Cross JR, DeNicola GM, et al. Connecting copper and cancer: from transition metal signalling to metaplasia. Nat Rev Cancer (2022) 22(2):102–13. doi: 10.1038/s41568-021-00417-2
53. Brewer GJ. Copper-lowering therapy with tetrathiomolybdate for cancer and diseases of fibrosis and inflammation. J Trace Elements Exp Med (2003) 16(4):191–9. doi: 10.1002/jtra.10045
54. Czlonkowska A, Litwin T, Dusek P, Ferenci P, Lutsenko S, Medici V, et al. Wilson disease. Nat Rev Dis Primers (2018) 4(1):21. doi: 10.1038/s41572-018-0018-3
55. Morisawa A, Okui T, Shimo T, Ibaragi S, Okusha Y, Ono M, et al. Ammonium tetrathiomolybdate enhances the anticancer effects of cetuximab via the suppression of osteoclastogenesis in head and neck squamous carcinoma. Int J Oncol (2018) 52(3):989–99. doi: 10.3892/ijo.2018.4242
56. Lan QH, Du CC, Yu RJ, Zhai JY, Shi YN, Kou LF, et al. Disulfiram-loaded copper sulfide nanoparticles for potential anti-glioma therapy. Int J Pharmaceutics (2021) 607:120978. doi: 10.1016/j.ijpharm.2021.120978
57. Cao HZ, Yang WT, Zheng PS. Cytotoxic effect of disulfiram/copper on human cervical cancer cell lines and LGR5-positive cancer stem-like cells. BMC Cancer (2022) 22(1):1–13. doi: 10.1186/s12885-022-09574-5
58. Zhang SP, Xin W, Anderson GJ, Li RB, Gao L, Chen SG, et al. Double-edge sword roles of iron in driving energy production versus instigating ferroptosis. Cell Death Dis (2022) 13(1):40. doi: 10.1038/s41419-021-04490-1
59. Bock FJ, Tait SWG. Mitochondria as multifaceted regulators of cell death. Nat Rev Mol Cell Biol (2020) 21(2):85–100. doi: 10.1038/s41580-019-0173-8
60. Tong XH, Tang R, Xiao MM, Xu J, Wang W, Zhang B, et al. Targeting cell death pathways for cancer therapy: recent developments in necroptosis, pyroptosis, ferroptosis, and cuproptosis research. J Hematol Oncol (2022) 15(1):174. doi: 10.1186/s13045-022-01392-3
61. Litwin T, Bembenek J, Antos A, Kurkowska-Jastrzebska I, Przybylkowski A, Skowronska M, et al. The maternal and fetal outcomes of pregnancy in wilson's disease: A systematic literature review and meta-analysis. Biomedicines (2022) 10(9):2072. doi: 10.3390/biomedicines10092072
62. Cui XN, Wang Y, Liu H, Shi MJ, Wang JW, Wang YF. The molecular mechanisms of defective copper metabolism in diabetic cardiomyopathy. Oxid Med Cell Longevity (2022) 2022:5418376. doi: 10.1155/2022/5418376
63. Zeng R, Peng B, Peng EM. Downregulated copper homeostasis-related gene FOXO1 as a novel indicator for the prognosis and immune response of breast cancer. J Immunol Res (2022) 2022:9140461. doi: 10.1155/2022/9140461
64. Wang L, Cao Y, Guo W, Xu J. High expression of cuproptosis-related gene FDX1 in relation to good prognosis and immune cells infiltration in colon adenocarcinoma (COAD). J Cancer Res Clin Oncol (2023) 149(1):15–24. doi: 10.1007/s00432-022-04382-7
65. da Silva DA, De Luca A, Squitti R, Rongioletti M, Rossi L, MaChado CML, et al. Copper in cancers and the use of copper-based compounds in cancer treatment. J Inorganic Biochem (2022) 226:111634. doi: 10.1016/j.jinorgbio.2021.111634
66. Qasem Z, Pavlin M, Ritacco I, Avivi MY, Meron S, Hirsch M, et al. Disrupting Cu trafficking as a potential therapy for cancer. Front Mol Biosci (2022) 9. doi: 10.3389/fmolb.2022.1011294
67. Duan WJ, He RR. Cuproptosis: copper-induced regulated cell death. Sci China-Life Sci (2022) 65(8):1680–2. doi: 10.1007/s11427-022-2106-6
68. Dorsam B, Fahrer J. The disulfide compound α-lipoic acid and its derivatives: A novel class of anticancer agents targeting mitochondria. Cancer Letters (2016) 371(1):12–9. doi: 10.1016/j.canlet.2015.11.019
69. Sha SN, Si LY, Wu XR, Chen YB, Xiong H, Xu Y, et al. Prognostic analysis of cuproptosis-related gene in triple-negative breast cancer. Front Immunol (2022) 13. doi: 10.3389/fimmu.2022.922780
70. Liu HR. Pan-cancer profiles of the cuproptosis gene set. Am J Cancer Res (2022) 12(8):4074–+. doi: 10.3389/fonc.2022.952290
71. Sharma P, Goyal D, Chudasama B. Antibacterial Activity of Colloidal Copper Nanoparticles against Gram-negative (Escherichia coli and Proteus vulgaris) Bacteria. Lett Appl Microbiol (2022) 74(5):695–706. doi: 10.1111/lam.13655
72. Rayess H, Wang MB, Srivatsan ES. Cellular senescence and cancer suppressor gene p16. Int J Cancer (2012) 130(8):1715–25. doi: 10.1002/ijc.27316
73. Cen D, Brayton D, Shahandeh B, Meyskens Jr Farmer FL PJ. Disulfiram facilitates intracellular cu uptake and induces apoptosis in human melanoma cells. J Medicinal Chem (2004) 47(27):6914–20. doi: 10.1021/jm049568z
74. Jiang ZT, Sha GY, Zhang WJ, Zhang ZL, Liu T, Wang DR, et al. The vast potential of targeting copper status in the treatment of colorectal cancer. Clin Trans Oncol (2023) 25(7):1977–90. doi: 10.1007/s12094-023-03107-7
75. Baszuk P, Marciniak W, Derkacz R, Jakubowska A, Cybulski C, Gronwald J, et al. Blood copper levels and the occurrence of colorectal cancer in Poland. Biomedicines (2021) 9(11):1628. doi: 10.3390/biomedicines9111628
76. Hu Q, Wang RT, Ma HY, Zhang ZW, Xue Q. Cuproptosis predicts the risk and clinical outcomes of lung adenocarcinoma. Front Oncol (2022) 12. doi: 10.3389/fonc.2022.922332
77. Wang T, Liu YF, Li Q, Luo Y, Liu DW, Li B. Cuproptosis-related gene FDX1 expression correlates with the prognosis and cancer immune microenvironment in clear cell renal cell carcinoma. Front Immunol (2022) 13. doi: 10.3389/fimmu.2022.999823
78. Diz M, Duran-Carril ML, Castro J, Alvo S, Bada L, Vina D, et al. Anticancer activity of copper(II) complexes with Schiff bases derived from N' -tosyl benzene-1,2-diamine. J Inorganic Biochem (2022) 236:111975. doi: 10.1016/j.jinorgbio.2022.111975
79. Jiapaer Z, Zhang L, Ma W, Liu H, Li C, Huang W, et al. Disulfiram-loaded hollow copper sulfide nanoparticles show anti-cancer effects in preclinical models of colorectal cancer. Biochem Biophys Res Commun (2022) 635:291–8. doi: 10.1016/j.bbrc.2022.10.027
80. Yun YH, Wang Y, Yang ED, Jing X. Cuproptosis-related gene-SLC31A1, FDX1 and ATP7B-polymorphisms are associated with risk of lung cancer. Pharmacogenomics Personalized Med (2022) 15:733–42. doi: 10.2147/PGPM.S372824
81. Tang DL, Chen X, Kroemer G. Cuproptosis: a copper-triggered modality of mitochondrial cell death. Cell Res (2022) 32(5):417–8. doi: 10.1038/s41422-022-00653-7
82. Davis CI, Gu XX, Kiefer RM, Ralle M, Gade TP, Brady DC. Altered copper homeostasis underlies the sensitivity of hepatocellular carcinoma to copper chelation. Metallomics (2020) 12(12):1995–2008. doi: 10.1039/d0mt00156b
83. Li J, Wu F, Li CF, Sun SY, Feng C, Wu HZ, et al. The cuproptosis-related signature predicts prognosis and indicates the immune microenvironment in breast cancer. Front Genet (2022) 13. doi: 10.3389/fgene.2022.977322
84. Lei L, Tan L, Sui L. A novel cuproptosis-related gene signature for predicting prognosis in cervical cancer. Front Genet (2022) 13. doi: 10.3389/fgene.2022.957744
85. Zhang M, Shi M, Zhao Y. Association between serum copper levels and cervical cancer risk: a meta-analysis. Bioscience Rep (2018) 38(4):BSR20180161. doi: 10.1042/BSR20180161
86. Wang YX, Zhang YF, Wang LR, Zhang N, Xu WQ, Zhou JM, et al. Development and experimental verification of a prognosis model for cuproptosis-related subtypes in HCC. Hepatol Int (2022) 16(6):1435–47. doi: 10.1007/s12072-022-10381-0
87. Lv HZ, Liu X, Zeng XH, Liu YT, Zhang CJ, Zhang Q, et al. Comprehensive analysis of cuproptosis-related genes in immune infiltration and prognosis in melanoma. Front Pharmacol (2022) 13. doi: 10.3389/fphar.2022.930041
88. Yang LF, Zhang YW, Wang Y, Jiang P, Liu FP, Feng NH. Ferredoxin 1 is a cuproptosis-key gene responsible for cancer immunity and drug sensitivity: A pan-cancer analysis. Front Pharmacol (2022) 13. doi: 10.3389/fphar.2022.938134
89. Xiong C, Ling H, Hao Q, Zhou X. Cuproptosis: p53-regulated metabolic cell death? Cell Death Differ (2023) 30(4):876–84. doi: 10.1038/s41418-023-01125-0
90. Peng XY, Zhu JF, Liu SC, Luo C, Wu X, Liu ZT, et al. Signature construction and molecular subtype identification based on cuproptosis-related genes to predict the prognosis and immune activity of patients with hepatocellular carcinoma. Front Immunol (2022) 13. doi: 10.3389/fimmu.2022.990790
91. Zhang Z, Zeng XY, Wu YH, Liu Y, Zhang X, Song ZW. Cuproptosis-related risk score predicts prognosis and characterizes the cancer microenvironment in hepatocellular carcinoma. Front Immunol (2022) 13. doi: 10.3389/fimmu.2022.925618
92. Ding FX, Li F, Tang DS, Wang B, Liu JY, Mao XY, et al. Restoration of the immunogenicity of cancer cells for enhanced cancer therapy via nanoparticle-mediated copper chaperone inhibition. Angewandte Chemie-International Edition (2022) 61(31):e202203546. doi: 10.1002/anie.202203546
93. Engin AB, Engin ED, Engin A. Can iron, zinc, copper and selenium status be a prognostic determinant in COVID-19 patients? Environ Toxicol Pharmacol (2022) 95:14. doi: 10.1016/j.etap.2022.103937
94. Bian ZL, Fan R, Xie LM. A novel cuproptosis-related prognostic gene signature and validation of differential expression in clear cell renal cell carcinoma. Genes (2022) 13(5):851. doi: 10.3390/genes13050851
95. Zhao JA, Guo SC, Schrodi SJ, He DY. Cuproptosis and cuproptosis-related genes in rheumatoid arthritis: Implication, prospects, and perspectives. Front Immunol (2022) 13. doi: 10.3389/fimmu.2022.930278
96. Coelho FC, Cerchiaro G, Santo Araujo SE, Daher JPL, Cardoso SA, Coelho GF, et al. Is there a connection between the metabolism of copper, sulfur, and molybdenum in alzheimer's disease? New insights on disease etiology. Int J Mol Sci (2022) 23(14):7935. doi: 10.3390/ijms23147935
97. Malik M, Switlicka A, Bienko A, Komarnicka UK, Bienko DC, Koziel S, et al. Copper(II) complexes with 2-ethyl pyridine and related hydroxyl pyridine derivatives: structural, spectroscopic, magnetic and anticancer in vitro studies. RSC Advances (2022) 12(42):27648–65. doi: 10.1039/D2RA05133H
98. Shan JS, Geng R, Zhang Y, Wei JT, Liu JH, Bai JL. Identification of cuproptosis-related subtypes, the establishment of a prognostic model and cancer immune landscape in endometrial carcinoma. Comput Biol Med (2022) 149:105988. doi: 10.1016/j.compbiomed.2022.105988
99. Liu J, Liu Y, Wang Y, Kang R, Tang DL. HMGB1 is a mediator of cuproptosis-related sterile inflammation. Front Cell Dev Biol (2022) 10. doi: 10.3389/fcell.2022.996307
100. Feng AQ, He LN, Chen T, Xu MD. A novel cuproptosis-related lncRNA nomogram to improve the prognosis prediction of gastric cancer. Front Oncol (2022) 12. doi: 10.3389/fonc.2022.957966
101. Kahlson MA, Dixon SJ. Copper-induced cell death. Sci (New York NY) (2022) 375(6586):1231–2. doi: 10.1126/science.abo3959
102. Li GX, Peng LH, Wu MJ, Zhao YP, Cheng Z, Li G. Appropriate level of cuproptosis may be involved in alleviating pulmonary fibrosis. Front Immunol (2022) 13. doi: 10.3389/fimmu.2022.1039510
103. Burgering BM, Coffer PJ. Protein kinase B (c-Akt) in phosphatidylinositol-3-OH kinase signal transduction. Nature (1995) 376(6541):599–602. doi: 10.1038/376599a0
104. McAlary L, Shephard VK, Wright GSA, Yerbury JJ. A copper chaperone-mimetic polytherapy for SOD1-associated amyotrophic lateral sclerosis. J Biol Chem (2022) 298(3):101612. doi: 10.1016/j.jbc.2022.101612
105. Saito A, Hayashi T, Okuno S, Ferranddrake M, Chan PH. Overexpression of Copper/Zinc Superoxide Dismutase in Transgenic Mice Protects against Neuronal Cell Death after Transient Focal Ischemia by Blocking Activation of the Bad Cell Death Signaling Pathway. J Neurosci Off J Soc Neurosci (2003) 23(5):1710–8. doi: 10.1523/JNEUROSCI.23-05-01710.2003
106. Bao XZ, Wang Q, Ren XR, Dai F, Zhou B. A hydrogen peroxide-activated Cu(II) pro-ionophore strategy for modifying naphthazarin as a promising anticancer agent with high selectivity for generating ROS in HepG2 cells over in L02 cells. Free Radical Biol Med (2020) 152:597–608. doi: 10.1016/j.freeradbiomed.2019.12.001
107. Liu JS, Lu YY, Dai YY, Shen Y, Zeng C, Liu XL, et al. A comprehensive analysis and validation of cuproptosis-associated genes across cancers: Overall survival, the cancer microenvironment, stemness scores, and drug sensitivity. Front Genet (2022) 13. doi: 10.3389/fgene.2022.939956
108. Xia SY, Jia HX, Qian ZP, Xiu YC. Role of copper ionophore-induced death in the immune microenvironment and clinical prognosis of ccRCC: An integrated analysis. Front Genet (2022) 13. doi: 10.3389/fgene.2022.994999
109. Oliveri V. Selective targeting of cancer cells by copper ionophores: an overview. Front Mol Biosci (2022) 9. doi: 10.3389/fmolb.2022.841814
110. Tsymbal S, Li G, Agadzhanian N, Sun YH, Zhang JZN, Dukhinova M, et al. Recent advances in copper-based organic complexes and nanoparticles for cancer theranostics. Molecules (2022) 27(20):7066. doi: 10.3390/molecules27207066
111. Tsymbal SA, Refeld AG, Kuchur OA. The p53 Cancer Suppressor and Copper Metabolism: An Unrevealed but Important Link. Mol Biol (2022) 56(6):979–92. doi: 10.1134/S0026893322060188
112. Tsvetkov P, Detappe A, Cai K, Keys HR, Brune Z, Ying WW, et al. Mitochondrial metabolism promotes adaptation to proteotoxic stress. Nat Chem Biol (2019) 15(7):681–+. doi: 10.1038/s41589-019-0291-9
113. Tsang T, Posimo JM, Gudiel AA, Cicchini M, Brady DC. Copper is an essential regulator of the autophagic kinases ULK1/2 to drive lung adenocarcinoma. Nat Cell Biol (2019) 22(4):412–24. doi: 10.1038/s41556-020-0481-4
114. Farhan M, Rizvi A, Ali F, Ahmad A, Aatif M, Malik A, et al. Pomegranate juice anthocyanidins induce cell death in human cancer cells by mobilizing intracellular copper ions and producing reactive oxygen species. Front Oncol (2022) 12. doi: 10.3389/fonc.2022.998346
115. Gao XX, Huang HC, Pan CX, Mei ZB, Yin SY, Zhou L, et al. Disulfiram/copper induces immunogenic cell death and enhances CD47 blockade in hepatocellular carcinoma. Cancers (2022) 14(19):4715. doi: 10.3390/cancers14194715
116. Agarwal A, Khandelwal A, Pal K, Khare NK, Jadhav V, Gurjar M, et al. A novel pro-oxidant combination of resveratrol and copper reduce transplant-related toxicities in patients receiving high dose melphalan for multiple myeloma (RESCU 001). PloS One (2022) 17(2):e0262212. doi: 10.1371/journal.pone.0262212
117. Aschner M, Skalny AV, Martins AC, Sinitskii AI, Farina M, Lu RZ, et al. Ferroptosis as a mechanism of non-ferrous metal toxicity. Arch Toxicology (2022) 96(9):2391–417. doi: 10.1007/s00204-022-03317-y
Keywords: copper, cuproptosis, cell death, cancer, metabolism, immunotherapy, molecular targeted therapy
Citation: Wang M, Zheng L, Ma S, Lin R, Li J and Yang S (2023) Cuproptosis: emerging biomarkers and potential therapeutics in cancers. Front. Oncol. 13:1288504. doi: 10.3389/fonc.2023.1288504
Received: 04 September 2023; Accepted: 23 October 2023;
Published: 07 November 2023.
Edited by:
Peng Su, Shandong University, ChinaReviewed by:
Michael Petronek, The University of Iowa, United StatesArchita Venugopal Menon, Northeastern University, United States
Copyright © 2023 Wang, Zheng, Ma, Lin, Li and Yang. This is an open-access article distributed under the terms of the Creative Commons Attribution License (CC BY). The use, distribution or reproduction in other forums is permitted, provided the original author(s) and the copyright owner(s) are credited and that the original publication in this journal is cited, in accordance with accepted academic practice. No use, distribution or reproduction is permitted which does not comply with these terms.
*Correspondence: Shuli Yang, yangsl@jlu.edu.cn