- 1Unidad de Investigación Médica en Genética Humana, Hospital de Pediatría “Dr. Silvestre Frenk Freund”, Centro Médico Nacional Siglo XXI, Instituto Mexicano del Seguro Social, Ciudad de México, Mexico
- 2Laboratorio de Hematobiología, Escuela Nacional de Medicina y Homeopatía, Instituto Politécnico Nacional, Ciudad de México, Mexico
- 3Servicio de Oncohematología Pediátrica, Hospital Pediátrico Moctezuma, Secretaría de Salud de la Ciudad de México, Ciudad de México, Mexico
- 4Universidad Autónoma Metropolitana, Unidad Xochimilco, Ciudad de México, Mexico
- 5Escuela Superior de Medicina, Instituto Politécnico Nacional, Ciudad de México, Mexico
- 6Laboratorio de Secuenciación, División de Desarrollo de la Investigación, Centro Médico Nacional Siglo XXI, Instituto Mexicano del Seguro Social, Ciudad de México, Mexico
- 7Unidad de Investigación Médica en Epidemiología Clínica, Hospital de Pediatría, Centro Médico Nacional Siglo XXI, Instituto Mexicano del Seguro Social, Ciudad de México, Mexico
- 8Laboratorio de Innovación y Medicina de Precisión, Núcleo A, Instituto Nacional de Medicina Genómica, Ciudad de México, Mexico
- 9Servicio de Oncología, Hospital Pediátrico de Moctezuma, Secretaría de Salud de la Ciudad de México, Ciudad de México, Mexico
- 10Servicio de Hematología Pediátrica, Hospital de Pediatría “Dr. Silvestre Frenk Freund”, Centro Médico Nacional Siglo XXI, Instituto Mexicano del Seguro Social, Ciudad de México, Mexico
- 11Servicio de Hematología Pediátrica, Centro Médico Nacional “20 de Noviembre”, Instituto de Seguridad y Servicios Sociales de los Trabajadores del Estado, Ciudad de México, Mexico
- 12Servicio de Oncología Pediátrica, Hospital de Pediatría “Dr. Silvestre Frenk Freund”, Centro Médico Nacional Siglo XXI, Instituto Mexicano del Seguro Social, Ciudad de México, Mexico
- 13Laboratorio de Genómica del Cáncer, Instituto Nacional de Medicina Genómica, Ciudad de México, Mexico
- 14Facultad de Medicina, Universidad Nacional Autónoma de México, Ciudad de México, Mexico
Background: Advances in the understanding of the pathobiology of childhood B-cell acute lymphoblastic leukemia (B-ALL) have led towards risk-oriented treatment regimens and markedly improved survival rates. However, treatment-related toxicities remain a major cause of mortality in developing countries. One of the most common adverse effects of chemotherapy in B-ALL is the hematologic toxicity, which may be related to genetic variants in membrane transporters that are critical for drug absorption, distribution, and elimination. In this study we detected genetic variants present in a selected group genes of the ABC and SLC families that are associated with the risk of high-grade hematologic adverse events due to chemotherapy treatment in a group of Mexican children with B-ALL.
Methods: Next generation sequencing (NGS) was used to screen six genes of the ABC and seven genes of the SLC transporter families, in a cohort of 96 children with B-ALL. The grade of hematologic toxicity was classified according to the National Cancer Institute’s Common Terminology Criteria for Adverse Events (CTCAE) version 5.0, Subsequently, two groups of patients were formed: the null/low-grade (grades 1 and 2) and the high-grade (grades 3 to 5) adverse events groups. To determine whether there is an association between the genetic variants and high-grade hematologic adverse events, logistic regression analyses were performed using co-dominant, dominant, recessive, overdominant and log-additive inheritance models. Odds ratio (OR) and 95% confidence intervals (95% CI) were calculated.
Results: We found two types of associations among the genetic variants identified as possible predictor factors of hematologic toxicity. One group of variants associated with high-grade toxicity risk: ABCC1 rs129081; ABCC4 rs227409; ABCC5 rs939338, rs1132776, rs3749442, rs4148575, rs4148579 and rs4148580; and another group of protective variants that includes ABCC1 rs212087 and rs212090; SLC22A6 rs4149170, rs4149171 and rs955434.
Conclusion: There are genetic variants in the SLC and ABC transporter families present in Mexican children with B-ALL that can be considered as potential risk markers for hematologic toxicity secondary to chemotherapeutic treatment, as well as other protective variants that may be useful in addition to conventional risk stratification for therapeutic decision making in these highly vulnerable patients.
1 Introduction
Acute Lymphoblastic Leukemia (ALL) is the predominant form of cancer in children (1). According to its cellular lineage, ALL is classified as T-cell ALL (T-ALL) and B-cell ALL (B-ALL); the latter type is the most frequent and comprises 80%-85% of ALL patients (2). Despite the existence of efficient treatment protocols for childhood ALL, 75% of patients experience therapy-related adverse effects, and 5% die from treatment toxicity (3–5). Among the most frequent side effects are hematologic adverse events leading to immunosuppression and myelosuppression and, consequently, an increased risk of infections (6, 7). Moreover, the effects of chemotherapy toxicity lead to treatment modifications or discontinuations which compromise the outcome (8–10).
The processes of drug transport and cellular detoxification involve the so-called ABC transporters, which comprise a superfamily of proteins that use ATP to move substrates across membranes (11). This superfamily consists of 49 members that are divided into 7 subfamilies from A to G according to sequence similarity, domain organization, and by function, whether importing or exporting (12, 13). The transport mechanisms in which these proteins participate are essential for the uptake of drugs through the intestinal wall, their expulsion from the tissues into the systemic circulation and, finally, their elimination through the kidneys and liver. Particularly, within this family of transporters, ABCC1-6 are involved in the transport of chemotherapeutic agents, such as adriamycin, vincristine, etoposide, 6-mercaptopurine, and methotrexate (14–16).
In addition, there are the SLC transporters that represent a superfamily with more than 400 members. Like the ABC family, they are classified into subfamilies according to sequence similarity (17). Their functions include a role in drug absorption and excretion, especially the SLC22 and SLCO subfamilies that are predominantly expressed in kidney, liver, and intestine. There is a broad consensus on the importance of these transporters in the mechanisms concerning the pharmacokinetics and pharmacodynamics of drugs used in chemotherapy, as well as the significance of genetic variants that alter their structure and function. As particular examples, the SLC22A6, SLC22A8 genes have been identified as involved in the methotrexate pathway and some variants in these genes are associated with a delay in their clearance (18, 19).
Some pharmacogenetic tests are now available to help in detecting key variants related to response and side effects to chemotherapy treatment in B-ALL patients. This information represents a valuable tool in making therapeutic decisions aimed at reducing the risk of toxicity before starting chemotherapy. However, the routinely analyzed variants are limited, and a high prevalence of toxicity is still observed in patients with B-ALL (20, 21). Furthermore, it is important to note that the frequency of some of the variants associated with an increased risk of toxicity in Caucasian population may differ in populations of a different genetic background such as the Hispanics. Taking the above into account, the aim of this study was to detect genetic variants present in a selected group genes of the ABC and SLC families that are associated with the risk of high-grade hematologic adverse events due to chemotherapy treatment in a group of Mexican children with B-ALL.
2 Materials and methods
2.1 Patients and samples
The study included ninety-six pediatric patients with B-ALL, diagnosed between 2014 and 2019 in public hospitals, members of the Mexican Interinstitutional Group for the Identification of the Causes of Childhood Leukemia (MIGICCL). These hospitals provide treatment to about 95% of pediatric patients with leukemia in Mexico City. The diagnosis of B-ALL was made at each institution by bone marrow aspirate for histopathological analysis and immunophenotyping. Peripheral blood samples were obtained at the end of remission induction. The pre-analytical sample processing route was performed by trained personnel assigned to each step following official Mexican regulations. The personnel assigned to each hospital attended every day to collect blood samples and/or bone marrow aspirate from the patients; and these were immediately transported to the laboratory where they were processed on the same day of collection, separating the different fractions. For this work, genomic DNA was extracted from the leukocyte fraction and, once the nucleic acids were extracted, the samples were stored properly labeled with a format to preserve patient anonymity in a biobank at -70°C until their use. The sample identification data and clinical characteristics of the patient are integrated in both physical and digital records. Patients were treated using one of two protocols, modified HP09 ALL Berlin-Frankfurt-Münster 95 protocol or St. Jude Total Therapy XIIIB modified (Supplementary Tables S1 , S2), depending on the hospital in which they were assisted. All biological samples and clinical information were obtained under written informed consent signed by parents and informed assent from children aged ≥ 8 years. A structured and exhaustive review of the medical records was performed to capture information related to hematologic toxicity from admission onwards. In case of death, the death certificate was consulted. The correlation between genetic variants and risk of hematologic toxicity was performed in the period of the first 100 days, from the beginning of therapy to the end of the consolidation phase of treatment, since during this period the differences in the physiological response to chemotherapy are clearer because these are the most intense phases of treatment. The grade of severity of each adverse event (AE) was defined according to the Common Terminology Criteria for Adverse Events (CTCAE), version 5, of the National Cancer Institute (22). Five severity event categories were considered: grade 1 (mild), clinical or diagnostic observations, asymptomatic laboratory signs or mild symptoms requiring no intervention; grade 2 (moderate), requiring minimal, local or noninvasive interventions; grade 3 (severe), requiring or prolonging hospitalization or incapacitating; grade 4 (life-threatening), when urgent medical intervention was required; and grade 5 (death), when death was related to the AE. Based on this classification two comparative groups were formed; the first,named as the null/low-grade adverse event group, included patients with no manifestations of hematologic toxicity during the established period as well as patients with adverse events grade 1 or 2. The second, termed high-grade adverse events group, consisted of patients with grade 3 and 4 with episodes of severe and life-threatening hematologic toxicity, respectively; and the only deceased patient in category 5 whose death was due to the hemato-toxic effects of chemotherapy. The total number of events per group is shown in Supplementary Figure S1. This study was approved by the ethics and scientific boards of the Mexican Institute of Social Security (IMSS) (registration number R-2015-785-121).
2.2 DNA extraction
DNA was extracted from peripheral blood samples using Maxwell® 16 Blood DNA Purification Kit (Promega, Madison, WI, USA) according to manufacturer’s recommendations. The purity and concentration of the DNA samples were measured using a Qubit 4 fluorometer (Thermo Fisher Scientific, Waltham, MA, USA).
2.3 Data collection
Information regarding sex, age at diagnosis, white blood cells (WBC) count in peripheral blood at diagnosis confirmation, immunophenotype, gene rearrangement characterization, and chemotherapeutic scheme was collected from patient records by personnel with prior standardized training. The four most common gene rearrangements with a known impact on the prognosis of children with leukemia (ETV6::RUNX1, TCF3::PBX1, BCR::ABL1, and KMT2A::AFF1), were examined by RT-PCR. Risk classification was determined at the time of diagnosis according to the NCI criteria: standard risk (ages from 1-9.99 years; WBC count <50,000/μl) or as high risk (age <1 or ≥10 years or WBC ≥50,000/μl).
2.4 Next-generation sequencing
Variants of the genes of interest were detected by next-generation sequencing (NGS) with a custom panel (Agilent, Santa Clara, CA, USA) to target coding regions, UTRs and some intronic variants of the transporters of the ABC and SLC family that had been previously reported as risk variants for hematologic toxicity: ABCC1, ABCB1, ABCC2, ABCC4, ABCC5, ABCG2, SLC19A1, SLC29A1, SLC22A1, SLC28A3, SLC22A8, SLC22A6 and SLC25A37. Capture-based enriched libraries were pooled (12 indexed libraries per pool) and sequenced on a Nextseq 500 System (Illumina, San Diego, CA, USA). Sequencing depth coverage was 60 to 100 X for all target regions. Genetic variant detection was analyzed with the Genome Analysis Toolkit (GATK, Broad Institute, Cambridge, UK).
2.5 Statistical analysis
The clinical characteristics of the two groups, with null/low or high toxicity, were compared either by chi square test (two-sided) or Fisher’s Exact Test when expected values were less than five, using SPSS Statistics 24.0 (IBM Canada Ltd.). P-values ≤0.05 were considered statistically significant. Deviation from Hardy–Weinberg equilibrium (HWE) of genetic variants was calculated based on chi-square test. Association analysis between single nucleotide variants (SNVs) and hematologic adverse events grade under five models of inheritance (codominant, dominant, recessive, overdominant, and log-additive model) were performed using online SNPStats program (http://bioinfo.iconcologia.net/SNPStats). The strength of association was measured by odds ratio (OR) with a 95% confidence interval (CI). The best model of inheritance for each SNV was selected based on Akaike (AIC) and Bayessian (BIC) information criteria. Linkage disequilibrium (LD) and haplotypes were analyzed using the online program SNPStats, and the LD plot was generated in Haploview version 4.1 software.
3 Results
3.1 Patients general characteristics
A total of 96 patients with B-ALL aged less than 16 years of age were included in the present study (Table 1). Of these, 44 were female and 52 were male. In terms of child´s sex, age, leukocyte count at baseline, chemotherapy protocol and risk classification, no statistically significant differences were found between groups. Fusion transcripts were not detected in most patients, and in 11 cases these data were not available. Most of the adverse events of chemotherapy were detected in the consolidation phase.
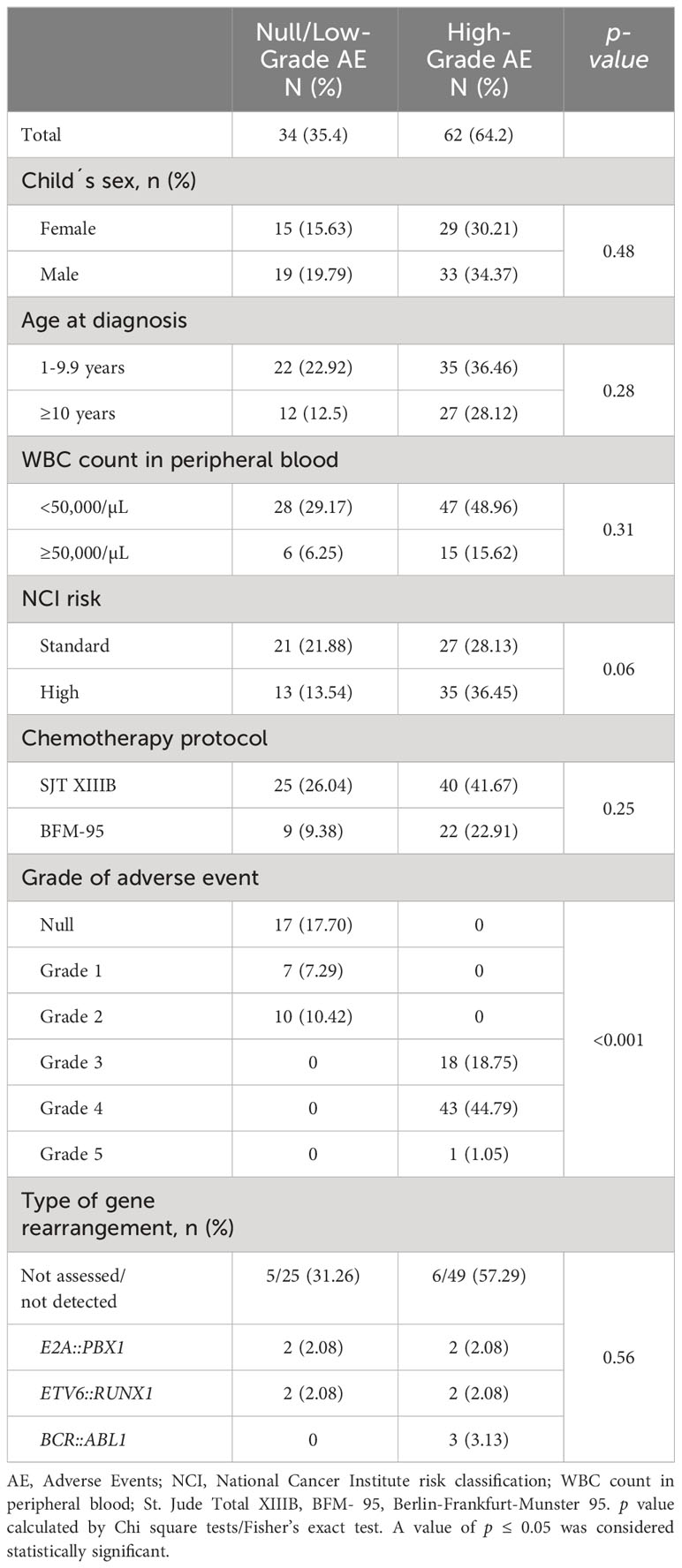
Table 1 Clinical features of the study population according to hematologic adverse events grade classification in patients B- ALL.
3.2 Genetic variants associated with risk of high-grade hematologic adverse events
Through NGS screening, some variants were detected in the ABC and SLC gene families that were associated with the risk of developing hematologic toxicity events of high grade. This association was analyzed using codominant, dominant, recessive, overdominant and log-additive models of inheritance (Supplementary Table S3). Selection of the inheritance model was based on Akaike and Bayesian informative criteria (data not shown). All genotype frequencies were in accordance with the Hardy-Weinberg equation. Table 2 shows the frequencies of variants detected with a statistically significant association with an increased risk of high-grade adverse events. The adjustment of our correlation analysis was performed considering the effect of homocedasticity and the effect of gender, age at diagnosis, white blood cell count at baseline, and genetic rearrangements. All detected risk variants belong to the ABCC gene family (Supplementary Table S4); two of them under the dominant model: ABCC1 rs129081, OR=2.58, 95% CI=1.01-6.57, p=0.047; and ABCC4 rs2274409, OR=2.9, 95% CI=1.16-7.27, p=0.014 (Table 2). Additionally, a risk association was found with a group of ABCC5 variants (r3749442; rs4148580; rs4148579; rs4148575 and rs939338), all of them under the recessive model. Among these ABCC5 variants it is noteworthy that genotypes rs3749442 AA (p=0.0066) and rs4148580 TT (p=0.019) are present only in individuals in the high-grade adverse events group. We then analyzed the linkage disequilibrium (LD) of the ABCC5 variants group using Haploview 4.1. The pairwise D’ values obtained are higher than 0.9 (p<0.001), so we can confirm that these nine variants of the ABCC5 gene are commonly inherited as a block (Figure 1). On the other hand, in this same gene we found four variants with OR values of 7.12 p=0.021, but broad confidence intervals (CI) ranging from 0.88 to 57.70 (Supplementary Table S5); therefore, although a trend is observed as risk variants, statistical significance was not obtained. For the SLC transporter family, no statistically significant association was found between any of its variants and the risk of high-grade hematologic adverse events.
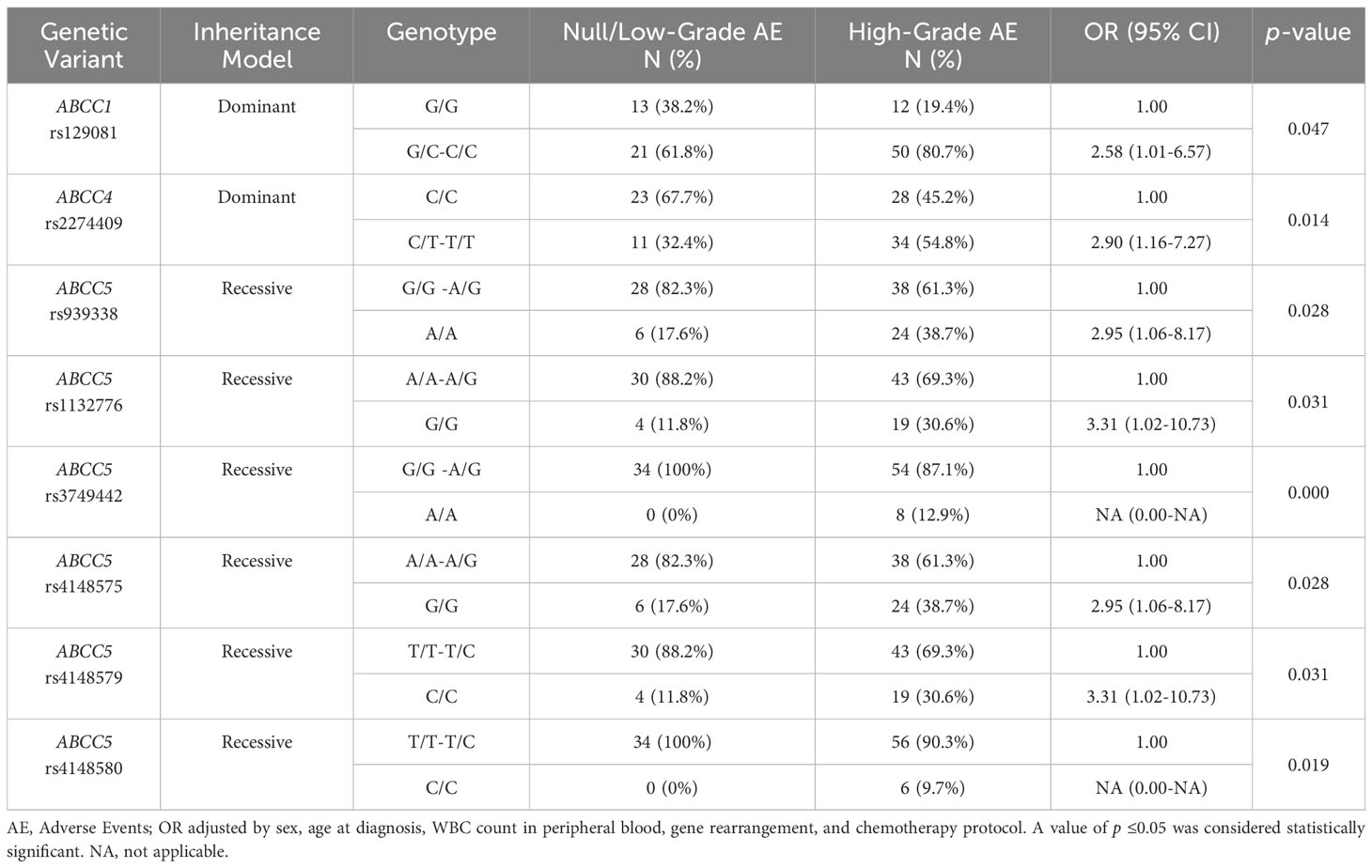
Table 2 Association of high-grade hematologic adverse events risk with genetic variants in ABC and SLC genes.
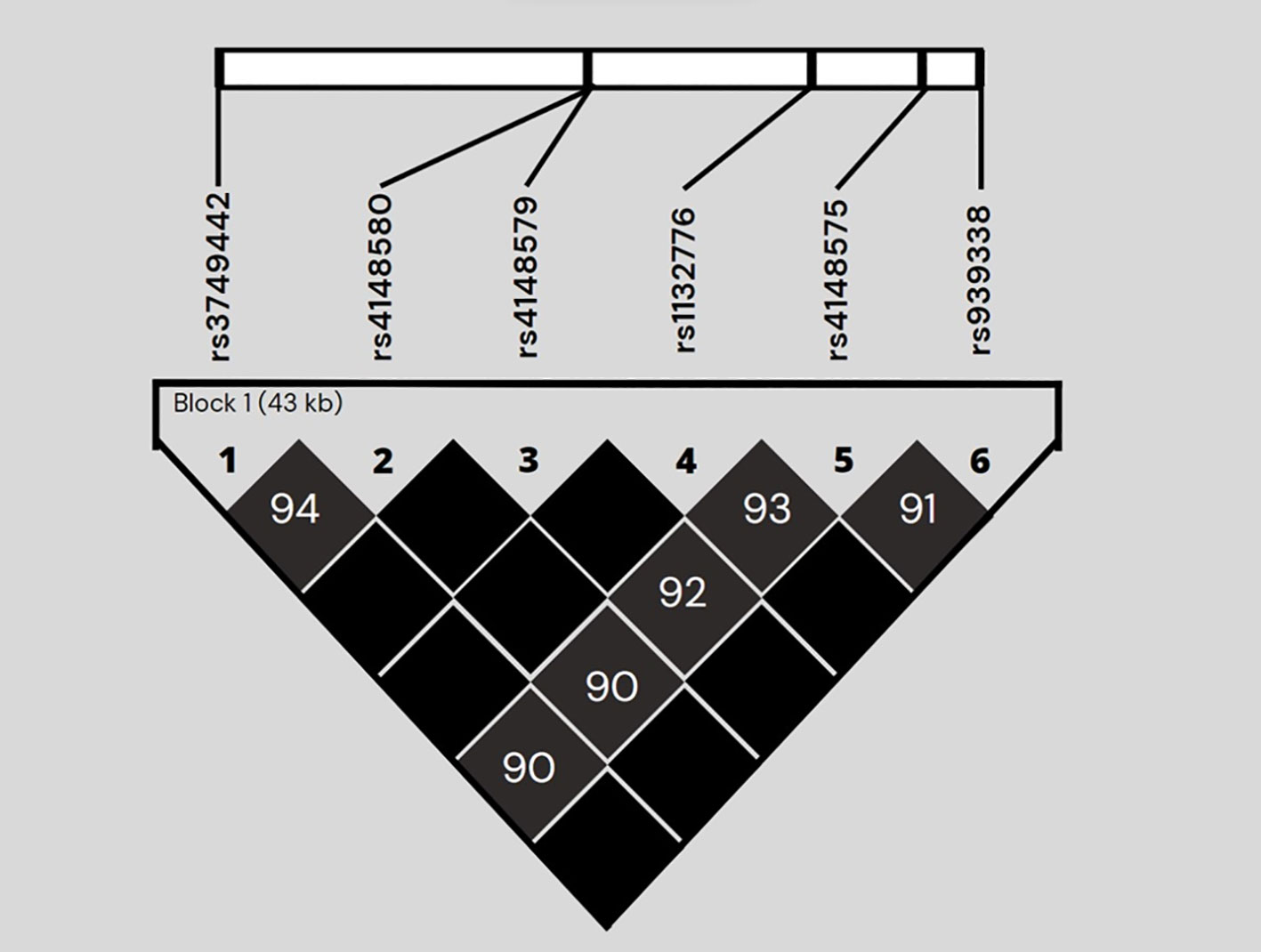
Figure 1 Linkage disequilibrium plot generated by Haploview software of the genetic risk variants of the ABCC5 gene. Linkage disequilibrium (LD) is shown as pairwise D' values. The unnumbered black diamonds correspond to D' values of 1.0.
3.3 Genetic variants protective of high-grade hematologic adverse events
Along with the risk variants, we found protective variants in genes of both gene families under a dominant model of inheritance (Table 3, Supplementary Table S4). The rs212087 and rs212090 variants of the ABCC1 gene, showed a protective association (OR=0.32, 95% CI=0.14-0.93, p=0.03; and OR=0.36, 95% CI=0.15-0.99, p=0.044, respectively). For the SLC family, a protective effect was observed from the rs4149170 and rs4149171 variants of the SLC22A6 gene in linkage disequilibrium (pairwise D’ value 1), and a value of OR=0.32, 95% CI=0.12-0.91, p=0.030 in both cases. In the same gene, the rs955434 variant was detected as protective for high-grade hematologic adverse events (OR=0.37, 95% CI=0.14, p=0.040) in the study population.
4 Discussion
Childhood type B acute lymphoblastic leukemia is the main type of childhood cancer in our country and, although survival rates have increased significantly in developed countries, in Mexico there is still a high mortality rate, part of which is associated with the adverse effects of chemotherapeutic treatment (23, 24). The individual’s ability to metabolize chemotherapeutic drugs depends on numerous factors including environment, gender, age, nutritional status, and genetic profile (25). The latter is of great relevance as it can cause changes in the structure of enzymes involved in drug metabolism and transport. These interindividual differences are the cause of treatment-related toxicities in a fraction of the pediatric ALL population, which can affect different organs and can range from mild to life-threatening (26). Currently, the application of FDA-approved pharmacogenetic testing has yielded promising results and encourages physicians to prescribe individualized medications (27). Nevertheless, a high percentage of patients continue to experience the effects of toxicity associated with chemotherapy treatment, the most frequent being hematologic toxicity (26, 27). Currently, the most successful example of pharmacogenetics in ALL in a clinical setting is the detection of genetic variants of genes encoding TPMT and NUDT enzymes prior to the prescription of thiopurine drugs and, although it has been implemented in different parts of our country, hematologic toxicity still occurs with high frequency (28). Since most of the studies related to this topic have been performed in Caucasian populations with different genetic backgrounds, it is essential to carry out pharmacogenomic studies in other populations. Therefore, in the present work, the main interest was to describe the association of genetic variants present in genes encoding drug transporters with high-grade hematologic adverse events caused by chemotherapeutic treatment in Mexican children diagnosed with B-ALL.
The uptake of chemotherapeutic treatment drugs used by blast cells may be affected by different transporters, including upstream and downstream transporters, especially solute transporters (SLC) and ATP-binding cassette (ABC) family pumps, respectively. Previous pharmacogenetic studies have suggested that single nucleotide variants of SLC and ABC transporters may play a promising role in drug exposure and have been associated with clinical response and toxicity. ABC transporters harness energy from ATP hydrolysis and function as efflux transporters, whereas SLC transporters are mainly involved in small molecule uptake into cells (29). In this study, we analyzed, by NGS, thirteen genes from these families selected based on the background of their association with risk of drug toxicity in the treatment of B-ALL. From this analysis we detected this type of association between ABC family variants and hematologic toxicity as risk factors in our group of B-ALL patients, particularly in ABCC1, ABCC4 and ABCC5.
ABCC1 transports a wide range of antineoplastic agents, including methotrexate, anthracyclines, vinca alkaloids, and numerous glucuronidated, glutathionylated, and sulfated derivative agents. ABCC1 gene sequence is highly conserved, however, genetic variants in its sequence have been associated with neurotoxicity, cardiotoxicity, and hematologic toxicity (30, 31). Vulsteke et al. (2013), reported the association of three variants in the ABCC1 gene with hematologic toxicity during chemotherapy in a Belgian population of women with breast cancer (32). In this study, we found that the ABCC1 rs129081 variant is associated with risk of high-grade hematologic toxicity. On the other hand, we observed that the ABCC1 rs212087 and rs212090 variants are protective against high-grade adverse events. When contrasting these results with other populations, we found data confirming the presence of risk or protective variants in ABCC1. In this sense, Gutiérrez-Camino et al. (2018) reported that the rs3743527 variant was associated with protection against anthracycline cardiotoxicity in Mexican pediatric patients with ALL treated with a traditional chemotherapeutic regimen (33). On the other hand, Kunadt et al. (2020) analyzed the presence of ABCC1 rs129081, rs212090 and 212091 variants located in the 3′ UTR region, finding an association with overall survival and disease-free survival in patients with AML. Although they did not conduct functional analyses, they propose that the change of nucleotides in these non-coding regions modifies the binding capacity of regulatory miRNAs with consequences on mRNA and protein expression (34). Thus, we can observe the duality between the presence of different variants in the same gene associated alternatively with risk or protection against high-grade hematological adverse events, which seem to influence the different responses to chemotherapeutic treatment.
Regarding the pharmacogenomics of the ABCC4 gene, the association of certain variants with 6-mercaptopurine intolerance has been described in Chinese children with ALL, while an association with hematologic toxicity was found in an Egyptian population with ALL (35, 36). Similarly, we found a risk association between high-grade hematologic adverse events and the ABCC4 rs2274409 variant. Besides, Krishnamurthy et al. (2008) observed that the rs3765534 missense variant reduces the function of the transporter by preventing its membrane localization, causing an accumulation of 6-thioguanine nucleotides in myeloid precursor cells (37). Although a possible negative dominant effect of this variant was ruled out, it was shown in a subsequent study in a Japanese population that the combination of rs3765534 with other NUDT15 intermediate activity variants in ALL patients caused intolerance to 6-mercaptopurine and marked leukopenia (38). Therefore, in future studies it will be important to intentionally search for combinations of risk variants that may be particularly affecting our population.
As for ABCC5, it is an efflux transporter for cyclic nucleotides and nucleotide analogues (39). Some of its genetic variants have previously been associated with doxorubicin cardiotoxicity in survivors of childhood ALL in a cohort of Canadian patients (40). In this study, we observed a clear association between high-grade hematologic adverse events and six ABCC5 gene variants, all in linkage disequilibrium. In addition, we found four more variants with a trend as risk factors, however, the confidence intervals are outside the limits of what can be considered a statistically significant association. In particular, the rs939336 variant stands out, which generates a stop codon that translates into a truncated protein that could affect its function in the transport of antineoplastic drugs (Supplementary Table S4). We consider that with a larger sample size it is very likely that this association between ABCC5 variants and hematologic toxicity will be confirmed; in any case, the results obtained already reflect the importance of this gene in the pharmacogenomics of B-ALL in Mexican pediatric population.
Regarding the SLC family, it has been demonstrated in other populations that certain variants are associated with the risk of different manifestations of toxicity (41). Although several genes of the family were considered within the sequencing strategy of this work, we only observed a protective association against high-grade hematologic adverse events in the SLC22A6 rs4149170, rs4149171 and rs955434 variants. To our knowledge, this is the first report of such an association with these specific variants. These results are in addition to those described by Visscher et al. (42), who demonstrated an association between the genetic variant rs7853758 of the SLC28A3 gene as a protective factor against anthracycline-induced cardiotoxicity in the pediatric population. Although the most clinically relevant approach in the study of genetic variants usually focuses on the description of risk variants, it is also important to have information on protective variants.
The major limitation of this study was the small sample size. In a next phase, we plan to increase the number of patients and, additionally, to analyze the ancestry of the participants from different regions of the country. Equally important, it will be to complement this information with the detection of genetic variants clearly associated with hematological toxicity such as those of the TPMT and NUDT15 genes. The objective is to have enough information to integrate it into a risk stratification algorithm according to the genetic background of each population and finally to be able to offer a personalized treatment with the lowest possible risk of side effects.
5 Conclusions
In summary, we found genetic variants within genes of the SLC and ABC transporter families as risk markers for high-grade hematologic adverse events risk from chemotherapeutic treatment of ALL in Mexican children, as well as other protective variants. These results show us that interindividual patient variability in these drug transporter coding genes should be considered in the management of their treatment as part of a personalized medicine strategy to improve the likelihood of a successful outcome in ALL patients.
Data availability statement
The datasets presented in this study can be found in online repositories. The names of the repository/repositories and accession number(s) can be found below: https://www.ncbi.nlm.nih.gov/, PRJNA1004576.
Ethics statement
The studies involving humans were approved by Comité Nacional de Investigación Científica del Instituto Mexicano del Seguro Social. The studies were conducted in accordance with the local legislation and institutional requirements. Written informed consent for participation in this study was provided by the participants’ legal guardians/next of kin.
Author contributions
DE-B: Writing – original draft, Data curation, Formal analysis, Investigation, Methodology. DC: Formal analysis, Writing – original draft, Conceptualization, Supervision, Writing – review & editing. EJ-H: Conceptualization, Writing – review & editing, Funding acquisition. CG-T: Conceptualization, Writing – review & editing, Data curation, Formal analysis, Investigation, Methodology. JG-C: Data curation, Formal analysis, Investigation, Methodology, Writing – review & editing, Conceptualization. JN-E: Conceptualization, Data curation, Formal analysis, Investigation, Methodology, Writing – review & editing, Funding acquisition, Project administration, Supervision, Writing – original draft. OS-R: Formal analysis, Supervision, Writing – review & editing. MI: Formal analysis, Supervision, Writing – review & editing. SJ-M: Formal analysis, Supervision, Writing – review & editing. JS-L: Formal analysis, Supervision, Writing – review & editing. MM-R: Writing – review & editing, Conceptualization, Investigation, Methodology. JT-N: Investigation, Writing – review & editing. JM-T: Investigation, Writing – review & editing. LF-V: Investigation, Writing – review & editing. MG-R: Investigation, Writing – review & editing. LM-P: Investigation, Writing – review & editing. KS-L: Investigation, Writing – review & editing. MM-M: Investigation, Writing – review & editing. GH-E: Investigation, Writing – review & editing. DO-R: Investigation, Writing – review & editing. JF-L: Investigation, Writing – review & editing, Data curation. MP-S: Investigation, Writing – review & editing. JM-A: Writing – review & editing, Conceptualization, Formal analysis, Funding acquisition, Methodology, Project administration, Supervision, Writing – original draft. HR-V: Conceptualization, Formal analysis, Funding acquisition, Methodology, Project administration, Supervision, Writing – original draft, Writing – review & editing.
Funding
The author(s) declare financial support was received for the research, authorship, and/or publication of this article. This research was funded by the Instituto Mexicano Seguro Social [Grant Numbers: FIS/IMSS/PRIO/15/048 and FIS/IMSS/PROT/PRIO/18/080]; by the Consejo Nacional Humanidades, Ciencias y Tecnologías Tecnologı́as (CONAHCYT) [Grant Numbers: FORDECYT PRONACES: 303019; FORDECYT PRONACES-377883-2020, as well as CF-2023-G-1399 to JM-A] and Instituto Nacional de Medicina Genómica (2023-01) to JM-A.
Acknowledgments
The authors would like to thank the contributors from the following participating hospitals and institutions: UMAE Hospital de Pediatría Centro Médico Nacional Siglo XXI ‘‘Dr. Silvestre Frenk Freund’’; Secretaría de Salud de la Ciudad de México (Hospital Pediátrico Moctezuma), and the Instituto de Seguridad Social al Servicio de los Trabajadores del Estado, Centro Médico Nacional “20 de Noviembre”.
Conflict of interest
The authors declare that the research was conducted in the absence of any commercial or financial relationships that could be construed as a potential conflict of interest.
The author(s) declared that author JN-E was a review editor and JM-A was an associate editor, review editor and a guest associate editor. Both authors were an editorial board member of Frontiers, at the time of submission. This had no impact on the peer review process and the final decision.
Publisher’s note
All claims expressed in this article are solely those of the authors and do not necessarily represent those of their affiliated organizations, or those of the publisher, the editors and the reviewers. Any product that may be evaluated in this article, or claim that may be made by its manufacturer, is not guaranteed or endorsed by the publisher.
Supplementary material
The Supplementary Material for this article can be found online at: https://www.frontiersin.org/articles/10.3389/fonc.2023.1276352/full#supplementary-material
Supplementary Figure 1 | Total number of hematologic adverse events recorded by group. (A) High-grade adverse events group, (B) Low-grade or no adverse events group. The overall number of events by grade and type according to CTCAE v5.0 is shown.
References
1. Inaba H, Mullighan CG. Pediatric acute lymphoblastic leukemia. Haematologica (2020) 105(11):2524–39. doi: 10.3324/haematol.2020.247031
2. Bernaldez-Rios R, Ortega-Alvarez MC, Perez-Saldivar ML, Alatoma-Medina NE, Del Campo-Martinez M de L, Rodriguez-Zepeda M del C, et al. The age incidence of childhood B-cell precursor acute lymphoblastic leukemia in Mexico City. J Pediatr Hematol Oncol (2008) 30(3):199–203. doi: 10.1097/MPH.0b013e318162bcdc
3. Thomas A. How can we improve on the already impressive results in pediatric ALL? Hematol Am Soc Hematol Educ Progr (2015) 2015(1):414–9. doi: 10.1182/asheducation-2015.1.414
4. Mlakar V, Huezo-Diaz Curtis P, Satyanarayana Uppugunduri CR, Krajinovic M, Ansari M. Pharmacogenomics in pediatric oncology: review of gene drug associations for clinical use. Int J Mol Sci (2016) 17(9):1502. doi: 10.3390/ijms17091502
5. Muñoz-Aguirre P, Huerta-Gutierrez R, Zamora S, Mohar A, Vega-Vega L, Hernández-Ávila JE, et al. Acute lymphoblastic leukaemia survival in children covered by seguro popular in Mexico: A national comprehensive analysis 2005-2017. Health Syst Reform (2021) 7(1):e1914897. doi: 10.1080/23288604.2021.1914897
6. Song Z, Hu Y, Liu S, Jiang D, Yi Z, Benjamin MM, et al. The role of genetic polymorphisms in high-dose methotrexate toxicity and response in hematologic Malignancies: A systematic review and meta-analysis. Front Pharmacol (2021) 12:757464. doi: 10.3389/fphar.2021.757464
7. Ali SS, Raj R, Kaur T, Weadick B, Nayak D, No M, et al. Solute carrier nucleoside transporters in hematopoiesis and hematologic drug toxicities: a perspective. Cancers (Basel) (2022) 14(13):3113. doi: 10.3390/cancers14133113
8. Yeoh A, Collins A, Fox K, Shields S, Ritchie P, Kirby M, et al. Treatment delay and the risk of relapse in pediatric acute lymphoblastic leukemia. Pediatr Hematol Oncol (2017) 34(1):38–42. doi: 10.1080/08880018.2016.1276235
9. Schmiegelow K, Müller K, Mogensen SS, Mogensen PR, Wolthers BO, Stoltze UK, et al. Non-infectious chemotherapy-associated acute toxicities during childhood acute lymphoblastic leukemia therapy. F1000Research (2017) 6:444. doi: 10.12688/f1000research.10768.1
10. Hough R, Vora A. Crisis management in the treatment of childhood acute lymphoblastic leukemia: Putting right what can go wrong (emergency complications of disease and treatment). Hematol Am Soc Hematol Educ Program (2017) 2017(1):251–8. doi: 10.1182/asheducation-2017.1.251
11. Gu RX, Corradi V, Singh G, Choudhury HG, Beis K, Tieleman DP. Conformational changes of the antibacterial peptide ATP binding cassette transporter mcjD revealed by molecular dynamics simulations. Biochemistry (2015) 54(38):5989–98. doi: 10.1021/acs.biochem.5b00753
12. Taylor NMI, Manolaridis I, Jackson SM, Kowal J, Stahlberg H, Locher KP. Structure of the human multidrug transporter ABCG2. Nature (2017) 546:504–9. doi: 10.1038/nature22345
13. Xiong J, Feng J, Yuan D, Zhou J, Miao W. Tracing the structural evolution of eukaryotic ATP binding cassette transporter superfamily. Sci Rep (2015) 5:16724. doi: 10.1038/srep16724
14. Iram SH, Gruber SJ, Raguimova ON, Thomas DD, Robia SL. ATP binding cassette transporter structure changes detected by intramolecular fluorescence energy transfer for high-throughput screening. Mol Pharmacol (2015) 88(1):84–94. doi: 10.1124/mol.114.096792
15. Mehrvar N, Abolghasemi H, Rezvany MR, Esmaeil Akbari M, Saberynejad J, Mehrvar A, et al. Pattern of ABCC transporter gene expression in pediatric patients with relapsed acute lymphoblastic leukemia. Rep Biochem Mol Biol (2019) 8(2):184–93.
16. Clifton MC, Simon MJ, Erramilli SK, Zhang H, Zaitseva J, Hermodson MA, et al. In vitro reassembly of the ribose ATP-binding cassette transporter reveals a distinct set of transport complexes. J Biol Chem (2015) 290(9):5555–65. doi: 10.1074/jbc.M114.621573
17. Geier EG, Chen EC, Webb A, Papp AC, Yee SW, Sadee W, et al. Profiling solute carrier transporters in the human blood-brain barrier. Clin Pharmacol Ther (2013) 96(6):636–9. doi: 10.1038/clpt.2013.175
18. Mikkelsen TS, Thorn CF, Yang JJ, Ulrich CM, French D, Zaza G, et al. PharmGKB summary: methotrexate pathway. Pharmacogenet Genomics (2011) 21(10):679–86. doi: 10.1097/FPC.0b013e328343dd93
19. Nigam SK. What do drug transporters really do? Nat Rev Drug Discov (2015) 14(1):29–44. doi: 10.1038/nrd4461
20. Lennard L. Implementation of TPMT testing. Br J Clin Pharmacol (2014) 77(4):704–14. doi: 10.1111/bcp.12226
21. Koutsilieri S, Caudle KE, Khaled S, Monte AA, Relling MV, Patrinos GP. Optimizing thiopurine dosing based on TPMT and NUDT15 genotypes: It takes two to tango. Am J Hematol (2019) 94(7):737–40. doi: 10.1002/ajh.25485
22. Common Terminology Criteria for Adverse Events (CTCAE) Version 5.0. Available at: https://ctep.cancer.gov/protocoldevelopment/electronic_applications/docs/CTCAE_v5_Quick_Reference_5x7.pdf (Accessed 09 May 2020).
23. Rivera-Luna R, Zapata-Tarres M, Shalkow-Klincovstein J, Velasco-Hidalgo L, Olaya-Vargas A, Finkelstein-Mizrahi N, et al. The burden of childhood cancer in Mexico: Implications for low- and middle-income countries. Pediatr Blood Cancer (2017) 64(6):e26366. doi: 10.1002/pbc.26366
24. Zapata-Tarrés M, Balandrán JC, Rivera-Luna R, Pelayo R. Childhood acute leukemias in developing nations: successes and challenges. Curr Oncol Rep (2021) 23(5):56. doi: 10.1007/s11912-021-01043-9
25. Kahn JM, Cole PD, Blonquist TM, Stevenson K, Jin Z, Barrera S, et al. An investigation of toxicities and survival in Hispanic children and adolescents with ALL: Results from the Dana-Farber Cancer Institute ALL Consortium protocol 05-001. Pediatr Blood Cancer (2018) 65(3):1–22. doi: 10.1002/pbc.26871
26. Maxwell RR, Cole PD. Pharmacogenetic predictors of treatment-related toxicity among children with acute lymphoblastic leukemia. Curr Hematol Malig Rep (2017) 12(3):176–86. doi: 10.1007/s11899-017-0376-z
27. Dean L, Kane M. Mercaptopurine Therapy and TPMT and NUDT15 Genotype. In: Pratt VM, Scott SA, Pirmohamed M, Esquivel B, Kattman BL, Malheiro AJ, editors. Medical Genetics Summaries. Bethesda (MD: National Center for Biotechnology Information (US (2012).
28. Texis T, Guzmán-Cruz C, Rodríguez-Dorantes M, Sánchez-García S, Mino-León D, Gonzalez-Covarrubias V. Genotyping NUDT15*3 rs1166855232 reveals higher frequency of potential adverse effects of thiopurines in Natives and Mestizos from Mexico. Pharmacol Rep (2022) 74(1):257–62. doi: 10.1007/s43440-021-00287-3
29. Franke RM, Gardner ER, Sparreboom A. Pharmacogenetics of drug transporters. Curr Pharm Des (2010) 16(2):220–30. doi: 10.2174/138161210790112683
30. Franca R, Rebora P, Bertorello N, Fagioli F, Conter V, Biondi A, et al. Pharmacogenetics and induction/consolidation therapy toxicities in acute lymphoblastic leukemia patients treated with AIEOP-BFM ALL 2000 protocol. Pharmacogenomics J (2017) 17(1):4–10. doi: 10.1038/tpj.2015.83
31. Gradhand U, Kim RB. Pharmacogenomics of MRP transporters (ABCC1-5) and BCRP (ABCG2). Drug Metab Rev (2008) 40(2):317–54. doi: 10.1080/03602530801952617
32. Vulsteke C, Lambrechts D, Dieudonné A, Hatse S, Brouwers B, van Brussel T, et al. Genetic variability in the multidrug resistance associated protein-1 (ABCC1/MRP1) predicts hematologic toxicity in breast cancer patients receiving (neo-)adjuvant chemotherapy with 5-fluorouracil, epirubicin and cyclophosphamide (FEC). Ann Oncol (2013) 24(6):1513–25. doi: 10.1093/annonc/mdt008
33. Gándara-Mireles JA, Lares-Asseff I, Reyes Espinoza EA, Blanco JG, González Font AE, Córdova Hurtado LP, et al. Association of genetic polymorphisms NCF4 rs1883112, CBR3 rs1056892, and ABCC1 rs3743527 with the cardiotoxic effects of doxorubicin in children with acute lymphoblastic leukemia. Pharmacogenet Genomics (2021) 31(5):108–15. doi: 10.1097/FPC.0000000000000428
34. Kunadt D, Dransfeld C, Dill C, Schmiedgen M, Kramer M, Altmann H, et al. Multidrug-related protein 1 (MRP1) polymorphisms rs129081, rs212090, and rs212091 predict survival in normal karyotype acute myeloid leukemia. Ann Hematol (2020) 99(9):2173–80. doi: 10.1007/s00277-020-04163-7
35. Fan POL, Leung KT, Chan KYY, Leung AWK, Lam GKS, Chow TTW, et al. ABCC4, ITPA, NUDT15, TPMT and their interaction as genetic predictors of 6-mercaptopurine intolerance in chinese patients with acute lymphoblastic leukemia. Pediatr Hematol Oncol (2022) 39(3):254–66. doi: 10.1080/08880018.2021.1973628
36. Hareedy MS, El Desoky ES, Woillard JB, Thabet RH, Ali AM, Marquet P, et al. Genetic variants in 6- mercaptopurine pathway as potential factors of hematologic toxicity in acute lymphoblastic leukemia patients. Pharmacogenomics (2015) 16(10):1119–34. doi: 10.2217/PGS.15.62
37. Krishnamurthy P, Schwab M, Takenaka K, Nachagari D, Morgan J, Leslie M, et al. Transporter-mediated protection against thiopurine-induced hematopoietic toxicity. Cancer Res (2008) 68(13):4983–9. doi: 10.1158/0008-5472.CAN-07-6790
38. Tanaka Y, Nakadate H, Kondoh K, Nakamura K, Koh K, Manabe A. Interaction between NUDT15 and ABCC4 variants enhances intolerability of 6-mercaptopurine in Japanese patients with childhood acute lymphoblastic leukemia. Pharmacogenomics J (2018) 8(2):275–80. doi: 10.1038/tpj.2017.12
39. Hu YH, Zhou L, Wang SS, Jing X, Guo HL, Sun F, et al. Methotrexate disposition in pediatric patients with acute lymphoblastic leukemia: what have we learnt from the genetic variants of drug transporters. Curr Pharm Des (2019) 25(6):627–34. doi: 10.2174/1381612825666190329141003
40. Krajinovic M, Elbared J, Drouin S, Bertout L, Rezgui A, Ansari M, et al. Polymorphisms of ABCC5 and NOS3 genes influence doxorubicin cardiotoxicitym in survivors of childhood acute lymphoblastic leukemia. Pharmacogenomics J (2017) 17(1):107. doi: 10.1038/tpj.2015.63
41. Maamari D, El-Khoury H, Saifi O, Muwakkit SA, Zgheib NK. Implementation of pharmacogenetics to individualize treatment regimens for children with acute lymphoblastic leukemia. Pharmgenomics Pers Med (2020) 13:295–317. doi: 10.2147/PGPM.S239602
Keywords: pharmacogenomics, membrane transporters, toxicity, acute lymphoblastic leukemia, ATP-binding cassette transporter, solute carrier family, hematologic adverse event
Citation: Escalante-Bautista D, Cerecedo D, Jiménez-Hernández E, González-Torres C, Gaytán-Cervantes J, Núñez-Enríquez JC, Sepúlveda-Robles OA, De Ita M, Jiménez-Morales S, Sánchez-López JM, Mata-Rocha M, Torres-Nava JR, Martín-Trejo JA, Flores-Villegas LV, Gutiérrez-Rivera MdL, Merino-Pasaye LE, Solís-Labastida KA, Miranda-Madrazo MR, Hernández-Echáurregui GA, Orozco-Ruíz D, Flores-Lujano J, Pérez-Saldívar ML, Mejía-Aranguré JM and Rosas-Vargas H (2024) Association between genetic variants of membrane transporters and the risk of high-grade hematologic adverse events in a cohort of Mexican children with B-cell acute lymphoblastic leukemia. Front. Oncol. 13:1276352. doi: 10.3389/fonc.2023.1276352
Received: 11 August 2023; Accepted: 28 November 2023;
Published: 10 January 2024.
Edited by:
Rozangela Curi Pedrosa, Federal University of Santa Catarina, BrazilReviewed by:
Elisa Dorantes, Federico Gómez Children’s Hospital, MexicoZeina Nizar Al-Mahayri, United Arab Emirates University, United Arab Emirates
Copyright © 2024 Escalante-Bautista, Cerecedo, Jiménez-Hernández, González-Torres, Gaytán-Cervantes, Núñez-Enríquez, Sepúlveda-Robles, De Ita, Jiménez-Morales, Sánchez-López, Mata-Rocha, Torres-Nava, Martín-Trejo, Flores-Villegas, Gutiérrez-Rivera, Merino-Pasaye, Solís-Labastida, Miranda-Madrazo, Hernández-Echáurregui, Orozco-Ruíz, Flores-Lujano, Pérez-Saldívar, Mejía-Aranguré and Rosas-Vargas. This is an open-access article distributed under the terms of the Creative Commons Attribution License (CC BY). The use, distribution or reproduction in other forums is permitted, provided the original author(s) and the copyright owner(s) are credited and that the original publication in this journal is cited, in accordance with accepted academic practice. No use, distribution or reproduction is permitted which does not comply with these terms.
*Correspondence: Haydeé Rosas-Vargas, aGF5cm92QGdtYWlsLmNvbQ==; Juan Manuel Mejía-Aranguré, am1lamlhQGlubWVnZW4uZ29iLm14
†These authors share first authorship