- 1Department of Pathology, Peking Union Medical College Hospital, Chinese Academy of Medical Sciences and Peking Union Medical College, Beijing, China
- 2MRL Global Medical Affairs, MSD China, Shanghai, China
Ovarian cancer (OC) is the eighth most common cancer in women, but the mild, non-specific clinical presentation in early stages often prevents diagnosis until progression to advanced-stage disease, contributing to the high mortality associated with OC. While serum cancer antigen 125 (CA-125) has been successfully used as a blood-borne marker and is routinely monitored in patients with OC, CA-125 testing has limitations in sensitivity and specificity and does not provide direct information on important molecular characteristics that can guide treatment decisions, such as homologous recombination repair deficiency. We comprehensively review the literature surrounding methods based on liquid biopsies, which may provide improvements in sensitivity, specificity, and provide valuable additional information to enable early diagnosis, monitoring of recurrence/progression/therapeutic response, and accurate prognostication for patients with OC, highlighting applications of this research in China.
1 Introduction
Worldwide, ovarian cancer (OC) was the eighth most common cancer and cause of cancer-related death in women in 2020, accounting for 1.6% of all new cancer cases and 2.1% of all cancer-related deaths (1). In China, more than 57,000 new cases of OC were reported in 2020, with over 39,000 deaths (2). More than 75% of OC is diagnosed at an advanced stage because early-stage ovarian tumors often present with mild, non-specific symptoms and minimal physical findings or may be asymptomatic (3, 4). Guidelines from the Society of Gynecologic Oncology (SGO) and the American Society of Clinical Oncology (ASCO) recommend ultrasonography, radiographic imaging, cancer antigen 125 (CA-125) serum level testing, and surgical biopsy (5).
Outcomes for patients with OC are strongly associated with disease stage at diagnosis. The 5-year overall survival (OS) rates are ~80%, ~60%, ~30%, and ~20% among patients with stage I, II, III, and IV OC, respectively (4). OC is also associated with high morbidity and high rates of relapse and metastasis, despite good responses to primary surgery and chemotherapy (6, 7). Therefore, several efforts have been made to establish tools for early diagnosis of OC. Tissue biopsy is considered standard for the histological diagnosis of OC (8), combined with imaging for staging. However, tumor biopsy is invasive and because of the non-specific symptomatology of OC, patients often do not undergo surgery before the disease has already progressed.
In contrast, testing for liquid-based biomarkers is not invasive and can facilitate preoperative diagnosis. While clinically validated tests have been approved as companion diagnostics for poly(ADP)ribose polymerase (PARP) inhibitors in OC and other tumor types in the US (9), currently, CA-125 is the only blood-borne marker recommended for the diagnosis and management of OC, which has been validated in numerous studies (10). Despite this, serum CA-125 levels cannot accurately discriminate benign from malignant ovarian lesions in premenopausal women (11), and CA-125 testing has low sensitivity in early disease stages (12), and does not provide detailed molecular information about the tumor. In addition, previous randomized clinical trials have not indicated a significant reduction in mortality from OC when screening using CA-125 level testing (13, 14). Hence, the US Preventive Services Task Force discourages the use of serum CA-125 levels to screen for OC (15). However, as early detection of OC is potentially cost-effective and may still improve survival (14, 16, 17), novel non-invasive strategies for early detection are in development and are urgently needed.
Broadly, liquid biopsies (LB) involve the analysis of cancer markers released by tumors in easily accessible bodily fluids, such as blood. These markers may include circulating tumor cells (CTCs), circulating tumor DNA (ctDNA), cell-free DNA (cfDNA) and exosome content such as microRNA (miRNA). CTCs have a low concentration in peripheral blood and specialized methods for their isolation and analysis in OC have been developed (18). In contrast, ctDNA/cfDNA has a relatively high concentration in peripheral blood and is detected using techniques such as digital droplet polymerase chain reaction (PCR), quantitative PCR, and next-generation sequencing (NGS) (18). By detection of molecular markers released by or present in cancer cells, LB retains the valuable insights into the molecular profile of the disease (e.g. homologous recombination deficiency [HRD]) afforded by tissue biopsy (19, 20), unlike CA-125 levels. The non-invasive nature of LB means that they are associated with less risk, patient pain, and potentially less cost, while being more easily repeatable than standard tissue biopsy (19, 20). These characteristics of LB may be of particular interest in China, where large regional variances and clusters of incidence and mortality are observed (21).
Here, we comprehensively review the use of LB for the diagnosis of OC, as well as for predicting patient outcomes, response to treatment, and disease progression (Figure 1).
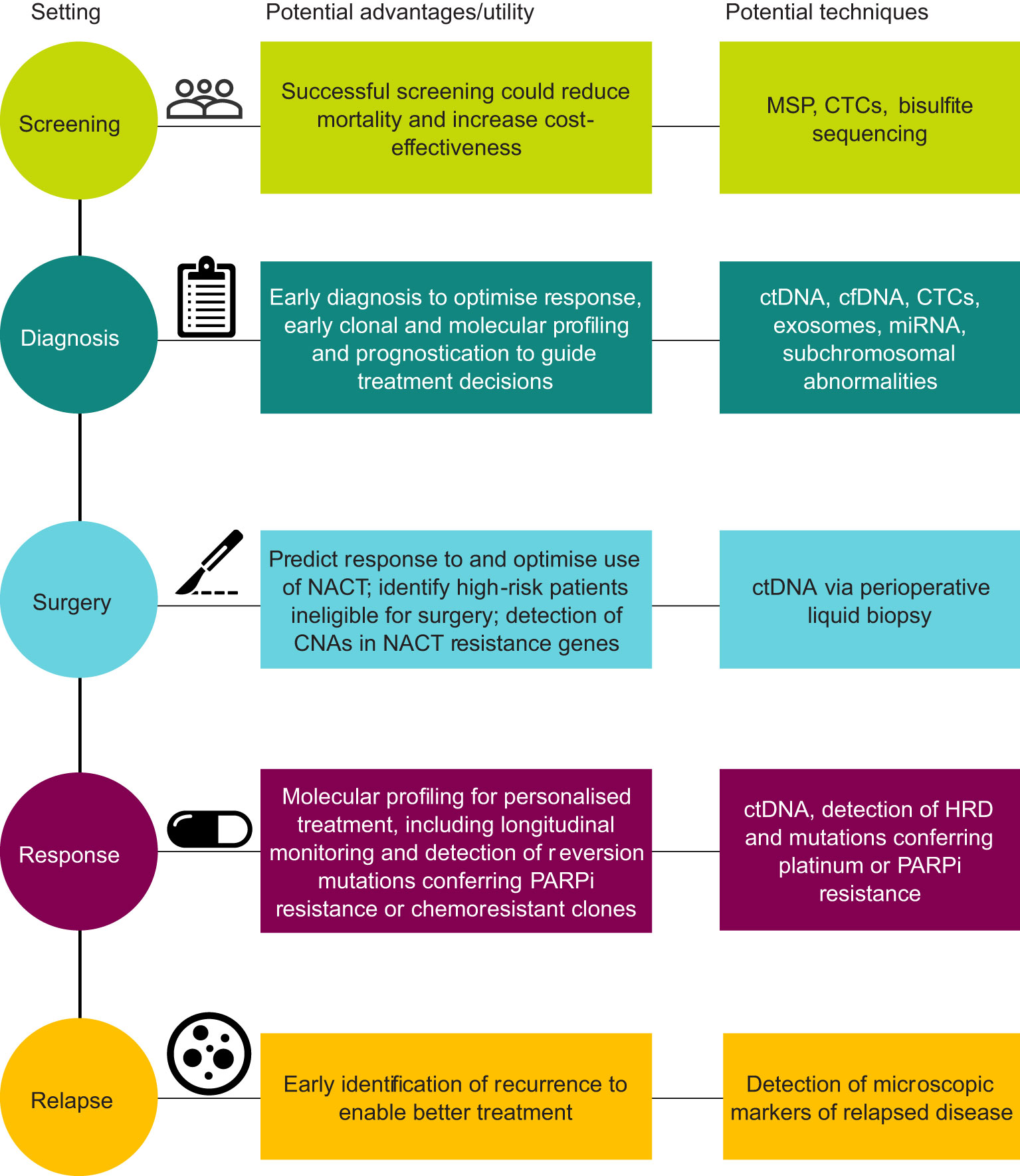
Figure 1 Schematic summary of liquid biopsy in ovarian cancer. CNA, copy-number alteration; CTC, circulating tumor cell; cfDNA, cell-free DNA; ctDNA, circulating tumor DNA; HRD, homologous recombination deficiency; miRNA, microRNA; MSP, methylation-specific PCR; NACT, neoadjuvant chemotherapy; PARPi, poly(ADP-ribose) polymerase inhibitor.
We aim to increase awareness of the clinical relevance of LB in OC and thereby increase clinical adoption to improve early diagnosis and treatment outcomes, and call for future research on the identification of OC biomarkers in LB.
2 Screening
Developing better screening strategies may increase the rates of tumor detection at pre-symptomatic stages and improve outcomes. Ideal screening assays should be specific, sensitive, non-invasive, and cost-effective to enable adoption into routine clinical practice.
Most OC screening strategies using LB in pre-symptomatic individuals are based on cancer-specific epigenetic signatures detected in ctDNA or cfDNA isolated from blood (Table 1).
Several studies conducted in China have attempted to evaluate the utility of LB for the detection of OC. For example, Dong et al. (36) found that the tumor suppressor gene SLIT2 was hypermethylated in 29 of 36 (80.6%) Chinese patients with OC, but not in any of the 25 healthy women evaluated. In 27 of the 29 (93.1%) patients with tumor SLIT2 hypermethylation, SLIT2 was also aberrantly methylated in ctDNA samples. In a similar study in China, Wang et al. (26) used methylation-specific polymerase chain reaction (MSP) to analyze aberrantly methylated genes in cfDNA from 194 patients with OC, and found that OPCML was hypermethylated in patients with early-stage OC but not in healthy donors. Interestingly, serum levels of CA-125 did not differ between patients with OC and healthy donors (26). Aberrant methylation of RASSF2A in cfDNA was also observed in approximately 36% of plasma samples from patients with OC, but was not observed in patients with benign ovarian tumors or healthy volunteers (24).
Zhang et al. (23) developed a multiplex MSP assay for the detection of early-stage OC using serum cfDNA in China. The assay was based on seven genes that are frequently hypermethylated in OC: APC, CDH1, OPCML, RASSF1A, RUNX3, SFRP5, and TFPI2. Using preoperative cfDNA samples from 87 patients with OC (stage I, n = 41; stage II–IV, n = 46), 53 with benign ovarian tumors, and 62 healthy donors, the high specificity (90.5%) and sensitivity (85.3%) of this assay was notably higher than the respective values for CA-125 in this cohort (64.2% and 56.1%, respectively) (23).
More recently, results from the US/Canada-based Circulating Cell-free Genome Atlas study (CCGA) have been reported, which used a methylation-based cfDNA approach combined with machine learning to screen for multiple tumor types (27). With a high specificity of 99.5%, the test had an overall sensitivity of 51.5% across tumor types. Among patients with OC, the test had a sensitivity of 80.0–94.7% in patients with stage II–IV disease and 50.0% in patients with stage I disease (27).
While these early results from methylation-based screening are promising, further study is needed to further characterize and refine screening methods and drive more widespread and standard selection of genes of interest. Because of the relatively low prevalence of OC, screening assays need to demonstrate a high predictive value; hence, larger studies are needed to confirm that LB-based assays exhibit high specificity (>99.7%) and sensitivity (>75%) before adoption into routine clinical practice (49).
3 Early diagnosis
3.1 ctDNA and cfDNA
Tumor-specific genetic alterations can be detected by cfDNA and ctDNA, which are small DNA fragments released by apoptotic or tumor cells that circulate through the bloodstream (Table 1).
In one of the first studies involving sequencing of entire genes to detect cancer mutations in cfDNA, Forshew et al. (30) used tagged-amplicon deep sequencing (Tam-Seq) to screen nearly 6000 genomic regions for mutations in the plasma of patients with advanced (stage III–IV) OC. This non-invasive method allowed the identification of cancer mutations with frequencies as low as 2%, providing a sensitivity and specificity of 97.5%. This method also allowed the monitoring of the evolution of tumors over time and identification of the source of metastatic relapse in patients with multiple primary tumors (30).
A NGS analysis of tumor and plasma samples from 96 patients with OC showed that tumor somatic variants in at least one of 27 cancer-related genes were present in the serum of 83.3% of patients with stage IV OC; however, the sensitivity of this test was lower for early-stage disease (32). Mutations in TP53 and BRCA1 in ctDNA or cfDNA have also been shown to have diagnostic utility in OC (31), and analysis of Chinese patients has shown that mutation frequency in ctDNA using hybrid capture-based genomic profiling were generally similar between tissue biopsies and LB (50).
Multiple studies have shown that testing cfDNA or ctDNA samples for methylation of various genes, including RASSF1A, CALCA, EP300, APC, CDH1, OPCML, RUNX3, SFRP5, COL23A1, C2CD4D, WNT6, TFPI2, HOXA9, and PAX1, may help detect early-stage OC (22, 23, 25, 26, 37, 38).
Testing for chromosomal instability in cfDNA or ctDNA may help identify patients with early-stage ovarian tumors. In a proof-of-concept study, Vanderstichele et al. (29) conducted low-coverage whole-genome sequencing of plasma cfDNA from 68 patients with an adnexal mass, 57 of whom were diagnosed with OC. Chromosomal instability levels in cfDNA matched those in tissue biopsies and were significantly higher in patients with OC than in those with benign tumors or healthy individuals. Chromosomal instability testing in cfDNA detected OC with area under the curve (AUC) values of 0.89 in the entire cohort and 0.94 in patients with high-grade serous OC. These AUC values were higher than those of serum CA-125 (AUC=0.78) (29).
A prospective study involving low-coverage sequencing of preoperative samples of circulating DNA from 32 women with OC (16 stage I–II, 16 stage III–IV) and 32 women with benign tumors supports the potential utility of genomic aberrations in cfDNA to detect malignant tumors (28). Subchromosomal abnormalities in cfDNA were present in 13 of 32 (41%) patients with OC, compared with 2 of 32 women with benign neoplasms, leading to a specificity of 93.8% but sensitivity of 40.6%, suggesting that further refinement of these methods is required to improve their performance.
Liang et al. investigated differentially methylated regions in OC ctDNA from the Chinese Academy of Medical Sciences Hospital, and developed two models: one for detection and one for prognostication of OC (51). The detection model was superior to CA-125-based detection (AUC, 0.987 [95% CI, 0.971−1.00] vs. 0.940 [95% CI: 0.895−0.985]), and the prognostic model for risk stratification also outperformed CA-125 (AUC, 0.949 [95% CI: 0.85−1.00] vs AUC, 0.659 [95% CI: 0.44−0.87]). These encouraging improvements over CA-125-based detection and prognostication warrant further investigation.
3.2 CTCs
CTCs are tumor cells that have entered the peripheral blood from the original tumor. As such, CTCs may provide information on multiple facets of OC, such as molecular classification to enable risk stratification (52–54). However, the concentration of CTCs in peripheral blood in early stages of OC is low, necessitating specialized techniques for enrichment and detection (42, 54–56). These techniques may include immunoaffinity and immunomagnetic techniques (54), dialectrophoresis and other microfluidic techniques (44, 57), as well as others for enrichment and detection.
Despite requirement of these specialized techniques, CTCs have shown promise as diagnostic biomarkers for OC (Table 1), as highlighted by multiple Chinese studies. Zhang et al. (41) used immunomagnetic detection of epithelial antigens (EpCAM, HER2, and MUC1) for enrichment combined with multiplex reverse transcriptase-polymerase chain reaction (RT-PCR) to detect CTCs in serum samples from 109 patients with OC; CTCs were found in 98 (90%) patients.
In a prospective analysis of samples from 61 women with suspected OC in China, Guo et al. (42) used size-based microfluidic separation and immunocytochemical detection and found that the counts of CTCs expressing HE4 and epithelial-to-mesenchymal transition (EMT) markers without CD45 were significantly higher in patients diagnosed with OC than in those with benign lesions, providing 86.7% specificity in patients with CA-125 ≥35 U/mL (42). The sensitivity of CTCs for detecting OC was higher than that of plasma CA-125 levels (73.3% vs. 56.7%).
To further improve the diagnostic utility of CTCs in ovarian cancer, Wang et al. (43) developed an optimized detection method based on EpCAM, MUC1, and WT1. This method was highly specific (92.2%) and had 79.4% sensitivity. Notably, the detection rate of CTCs was higher than that of CA-125 for early-stage (stage I/II) tumors (74.5% vs. 58.2%, P = 0.069).
While these findings are promising, a key challenge limiting the clinical utility of CTC-based diagnostics in early stages of OC is the low number of CTCs in the early stages of the disease (42, 55, 56), as well as reported detection rates varying from 12 to 90% across different platforms (42). In contrast, CTCs can be found in higher numbers in the circulation of patients with advanced disease (stages III and IV), with diagnostic sensitivity and specificity reaching 76%–83% and 55%–97%, respectively (39–41, 58, 59).
3.3 Exosomes and miRNAs
Exosomes are small (30–100 nm) vesicles released by cells that regulate cellular communication and transfer of molecules, including RNA, DNA, and proteins. Exosomes released by cancer cells can be used as diagnostic markers (Table 1).
Zhang et al. found that exosomes from Chinese patients with OC were enriched in proteins involved in tumorigenesis and metastasis (46). Exosomal FGA and GSN levels were significantly elevated, whereas FGG and LBP levels were significantly downregulated in exosomes from Chinese patients with OC compared with those from healthy donors (46), providing proof-of-concept evidence that proteomic profiling of exosomes can be used to diagnose OC in Chinese patients.
Emerging evidence suggests that circulating miRNAs may serve as diagnostic markers for OC (Table 1). Todeschini et al. (48) analyzed serum samples from 168 patients with stage III–IV OC and 65 healthy volunteers. They found that the levels of miR-1246, miR-595, and miR-2278 were significantly higher in serum samples from patients with OC than those from healthy controls. Receiver operating characteristic curve analysis revealed that among these miRNAs, miR-1246 had the highest diagnostic utility, and had an AUC of 0.89, sensitivity of 87%, specificity of 77%, and diagnostic accuracy of 84%. In a similar study, Liu et al. (47) seven exosome-derived miRNAs (miR-4732-5p, miR-877-5p, miR-574-3p, let-7a-5p, let-7b-5p, let-7c-5p, and let-7f-5p) were up-regulated and two down-regulated (miR-1273f and miR-342-3p) in patients with EOC; miR-4732-5p had an AUC of 0.889, with 85.7% sensitivity and 82.4% specificity in diagnosis of EOC. Another exploratory study in Chinese patients found that exosomal miRNA-205 expression was significantly associated with OC, and had elevated levels during metastasis (60), and exploratory analysis of circular RNAs in Chinese patients found that such RNAs may have diagnostic utility in combination with CA-125 (61). While a range of miRNAs have been identified as potential OC biomarkers (62), the heterogeneity of OC means that more studies are needed to assess the diagnostic utility of circulating and exosomal non-coding RNAs in patients with OC so that clearer and more consistent miRNA signatures and profiles can be developed and allow more routine early diagnosis using LB.
4 Surgery/perioperative liquid biopsy
Following diagnosis of OC, an early treatment decision is whether initial cytoreductive surgery should be primary (upfront) or interval (i.e. following neoadjuvant chemotherapy [NACT]). While large randomized trials have generally not found significant differences in survival outcomes between the two approaches (63–66), SGO/ASCO guidelines recommend that this decision is made according to clinical risk to avoid unnecessary exposure to platinum-based chemotherapy (67). In this way, LB represent a valuable tool in risk stratification by providing a non-invasive method that enables early identification of factors before surgery that may predict response to NACT such as platinum resistance, prognostic factors following surgery such as microscopic residual disease, and monitor response to treatment to guide treatment decisions.
Mutations in post-surgical ctDNA have been associated with inferior survival outcomes (68), detection of post-surgical ctDNA outperforms CA-125 monitoring as a predictor for mortality (69, 70), may be predictive of complete resection following NACT or following surgery (70, 71), and copy number alterations in MROH1, TMEM249, and HSF1 in ctDNA of patients with OC resistant to NACT were significantly associated with worse OS and high expression levels compared with patients with NACT-sensitive disease, suggesting that specific ctDNA mutations could be useful in LB for response monitoring and prediction (72). Larger, prospective studies of risk stratification and biomarker identification using perioperative LB are warranted to enable routine clinical adoption.
5 Treatment response and monitoring progression
5.1 Predicting and monitoring response to PARP inhibition
PARP (poly-ADP-ribose polymerase) inhibitors prevent repair of single-stranded breaks in DNA, generating double-stranded breaks that cannot be accurately repaired in tumors with HRD (73). HRD is typically caused by germline or somatic BRCA1/BRCA2 mutations, epigenetic factors such as BRCA1/BRCA2 silencing via promoter methylation, or potentially other genetic or genomic causes of genomic instability such as telomeric allelic imbalance, loss of heterozygosity, or large-scale state transitions in OC and other tumor types (74–77).
The efficacy of PARP inhibition (with or without bevacizumab) for OC has been demonstrated in global clinical trials (78–82), particularly as first-line maintenance therapy. Several PARP inhibitors have been approved in China for the treatment of newly diagnosed advanced HRD-positive, or platinum-sensitive relapsed OC and emerging real-world evidence highlights the importance of HRD as a biomarker to predict response to PARP inhibition in China (83–85). Based on results from the global phase III PRIMA trial, the PARP inhibitor niraparib was approved in China for patients with newly diagnosed advanced HRD-positive or HRD-negative tumors (86), though the benefit in PFS was most pronounced among patients who had HRD-positive tumors (median PFS for niraparib vs placebo among patients with HRD-positive tumors, 24.5 vs 11.2 months; hazard ratio [HR], 0.52 [95% CI, 0.40–0.68] and for patients with HRD-negative tumors 8.4 vs 5.4 months; HR, 0.65 [95% CI, 0.49–0.87]) and the higher, 300 mg, starting dose (78, 87, 88). Multiple LB have been approved as companion diagnostics for PARP inhibitors in various indications, including to detect HRD in OC and prostate cancer (9). Therefore, various studies have been conducted to assess the value of LB as a non-invasive method to assess HRD status and predict response to PARP inhibition (Table 2).
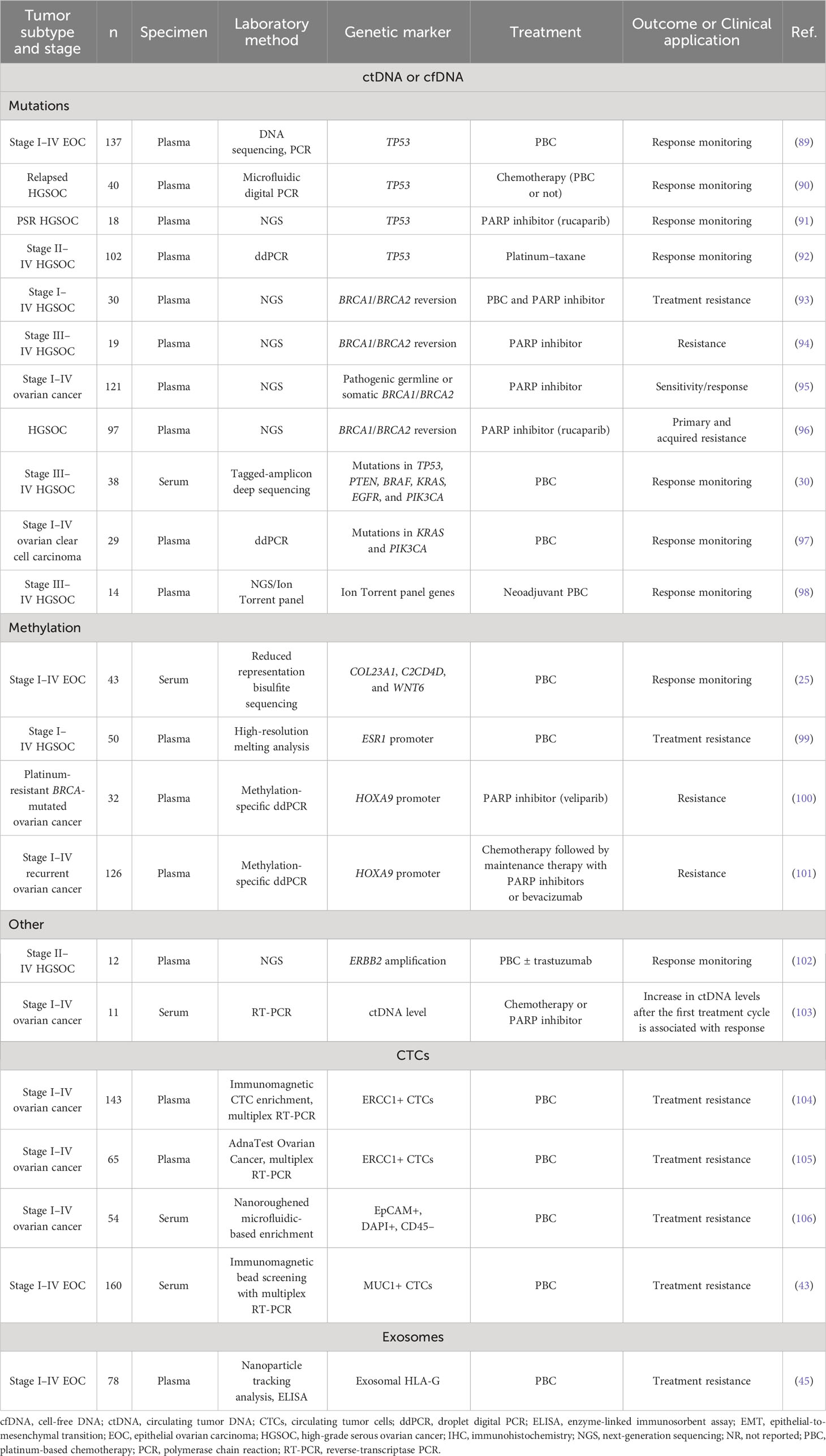
Table 2 Summary of studies using liquid biopsy to predict or monitor response to treatment in patients with ovarian cancer (n≥10).
5.1.1 cfDNA and ctDNA
Ratajska et al. (95) used NGS to analyze ctDNA samples from 121 patients with stage I–IV OC, demonstrating that 30 of the 121 (24.8%) patients had ctDNA with pathogenic germline or somatic BRCA1/BRCA2 mutations, comparable to reported germline and somatic BRCA mutation prevalences in China (107–109) and providing proof-of-concept evidence that BRCA1/BRCA2 mutation testing using ctDNA samples from patients with OC could be used in the clinic to identify patients best suited for PARP inhibition and to monitor response.
Reversion mutations in BRCA1/BRCA2 that restore protein function have been associated with the development of resistance to PARP inhibitors. In an NGS analysis of pretreatment ctDNA samples from 96 patients with OC, Lin et al. (96) found that BRCA1/BRCA2 reversion mutations in ctDNA were associated with primary and acquired resistance to rucaparib. NGS studies of preoperative cfDNA samples from patients with OC harboring germline BRCA1/BRCA2 mutations showed that reversion alterations restoring the BRCA1/BRCA2 open reading frame (ORF) were associated with resistance to PARP inhibition in patients with recurrent disease (93, 94), and that these reversion mutations may be caused by the microhomology-mediated end joining pathway (110).
Rusan et al. (100) found that the methylation levels of HOXA9 in ctDNA during treatment were associated with poor response to PARP inhibition in patients with platinum-resistant, BRCA1/BRCA2-mutated OC. Survival outcomes were significantly inferior in patients with detectable HOXA9 methylation in ctDNA than in those without HOXA9 methylation (median progression-free survival [PFS]: 5.1 vs. 8.3 months, P < 0.0001; median OS: 9.5 vs. 19.4 months, P < 0.002) (100). Faaborg et al. reported similar findings in a study of 126 patients (38.9% platinum sensitive and 81.7% with recurrent OC undergoing treatment with chemotherapy followed by maintenance therapy with PARP inhibitors or bevacizumab (101); with the increasing use of PARP inhibition in earlier lines of therapy, validation of these biomarkers in first line will be increasingly important.
TP53 mutations are one of the most prevalent genetic alterations in OC. In the Phase II ARIEL2 study of rucaparib in platinum-sensitive relapsed OC, targeted amplicon deep sequencing to detect low-frequency mutations in TP53 in ctDNA suggested that reduction in the frequency of TP53 mutations in ctDNA during treatment was associated with response to rucaparib (91).
5.2 Identifying platinum resistance and monitoring progression
5.2.1 cfDNA and ctDNA
Evaluating genetic markers of response to chemotherapy using LB is emerging as a promising approach for molecular profiling in patients with OC. As LB are easy to obtain and repeatable, they may be used for longitudinal monitoring of treatment response and disease progression (Table 2).
To evaluate the clinical utility of cfDNA analysis to monitor response to chemotherapy and disease progression, Arend et al. (98) conducted NGS analysis of cfDNA samples, which provided proof-of-concept evidence that cfDNA analysis before and after treatment can be used to monitor disease progression and the genetic evolution of tumors during chemotherapy.
In an analysis of pretreatment ctDNA samples from patients with OC, Lin et al. (96) found that BRCA1/BRCA2 reversion mutations were significantly more frequent in patients with platinum-refractory (18%; 2/11) or platinum-resistant (13%; 5/38) disease compared with platinum-sensitive disease (2%; 1/48). Another analysis of preoperative cfDNA samples from 30 patients with OC harboring germline BRCA1 or BRCA2 mutations showed that reversion alterations restoring the BRCA1/BRCA2 ORF were associated with resistance to platinum-based chemotherapy in patients with recurrent disease (93).
A longitudinal analysis of ctDNA samples to assess for mutations in more than 500 cancer-related genes revealed good concordance of genetic alterations in ctDNA and tumor samples from 12 patients with OC (102). The study also showed that testing for ERBB2 amplification in ctDNA from relapsed OC patients could identify patients who may benefit from ERBB2/HER2 inhibitors, such as trastuzumab (102).
In addition to mutations and structural aberrations in cfDNA or ctDNA, methylation of various genes, including COL23A1, C2CD4D, WNT6, ESR1, and HOXA9, has been shown to be associated with resistance to chemotherapy and could be used to predict or monitor treatment response (25, 99–101), suggesting that LB-based molecular testing may be useful in this setting, particularly in patients ineligible for tissue biopsy or for whom archival tissue is not available.
5.2.2 CTCs
Data from various studies suggest that CTCs could be used as markers of response or platinum resistance in patients with OC. For example, the numbers of EpCAM-positive CTCs and MUC1-positive CTCs were significantly higher in chemoresistant patients than in patients who responded to chemotherapy (26.3% vs. 11.9%, P < 0.05; 26.4% vs. 13.4%, P < 0.05; Table 2) (43).
The number of ERCC1-positive CTCs has also been associated with chemotherapy resistance. CTC enrichment analyses in patients with OC showed that the presence of ERCC1-positive CTCs at diagnosis was a significant predictor of resistance to platinum-based chemotherapy (104, 105). Despite the predictive role of ERCC1-positive CTCs, ERCC1 expression levels in primary tumor tissues and circulating ERCC1 mRNA levels did not predict resistance to chemotherapy, suggesting a particularly important role for LB in this setting.
Additionally, enrichment of CTCs (EpCAM-positive, DAPI-positive, and CD45-negative) using a nanoroughened microfluidic device showed that in 54 patients with stage I–IV OC, the number of CTCs was significantly associated with platinum resistance (106).
5.2.3 Exosomes
Exosomes have been implicated in metastasis and treatment resistance in patients with OC. Au Yeung et al. (111) conducted preclinical NGS analysis of exosomes from cancer-associated adipocytes (CAAs), cancer-associated fibroblasts (CAFs), and OC cells. Interestingly, they found that the levels of miR21 were significantly higher in exosomes from CAAs and CAFs than in those from OC cells. They also found that miR-21 transfer from CAAs and CAFs to ovarian cancer cells resulted in APAF1 silencing, thereby promoting chemoresistance and suppressing apoptosis (111). These findings suggest that levels of miR21 in exosomes may predict the risk of metastasis and chemoresistance in patients with OC, although clinical validation is required (Table 2).
Similar mechanistic studies have shown that exosomal miR-1246, miR-223, miR-183-5p, miR-130a, and miR-374a promote chemoresistance in OC (112–115). Additionally, Schwich et al. (45) showed that exosomal HLA-G levels were associated with platinum resistance. The clinical utility of these exosomal markers in OC requires further evaluation in clinical studies.
6 Prognostication and monitoring progression
6.1 cfDNA and ctDNA
Detection of cfDNA or ctDNA levels as well as examination of genetic and epigenetic characteristics are areas of great interest and have been well studied in the context of prognostication and monitoring of disease progression in OC (Table 3).
In analysis of pre-surgical cfDNA from patients with OC, Kamat et al. (118) found that higher levels of cfDNA (≥22,000 IU/mL) were significantly associated with worse survival, with multivariate analysis indicating that higher cfDNA levels were independently associated with worse disease-specific survival.
Hou et al. (69) found that when examining pre-surgical samples, ctDNA was more frequently detected and its levels significantly elevated in patients who subsequently experienced disease progression and died, with numerically higher ctDNA positivity and levels found in patients with high-grade OC. Following surgery, the presence of ctDNA was significantly associated with poor RFS, and all patients with ctDNA following surgery experienced disease progression; moreover, ctDNA-based methods detected recurrence 10 months before CT imaging (69). Similarly, Minato et al. (116) developed a droplet digital PCR-based assay to detect tumor-specific mutations in cfDNA in plasma, which was able to detect disease progression in all six patients who experienced disease recurrence. ctDNA levels were associated with increased tumor volume after recurrence. Notably, in both of these studies, analysis of ctDNA was able to detect disease recurrence earlier than CA-125 (69, 116).
Beyond cfDNA levels, genetic or epigenetic alterations in cfDNA or ctDNA associated with poor outcomes in patients with OC who receive PARP inhibitors or chemotherapy include RAB25 downregulation [associated with poor OS in patients before surgery (119)], loss of heterozygosity at 6q and 10q [associated with poor OS in patients before surgery (120)], HOXA9 promoter methylation [associated with poor OS and PFS in patients who received veliparib (100, 101)], RASSF1A promoter methylation [associated with poor OS following chemotherapy (117)], and BRCA1/BRCA2 reversion mutations [associated with poor PFS in patients receiving rucaparib (96)]. Several studies have also shown that TP53 mutations in plasma DNA were associated with shorter time to progression and poor OS (Table 3) (89, 90, 92).
6.2 CTCs and exosomes
The clinical significance of CTCs in OC is controversial (Table 3). Early studies showed no association between CTCs and survival outcomes (121, 122). However, Fan et al. defined invasive CTCs as those expressing CAM molecules and epithelial markers; the presence of these invasive CTCs in 71 patients with suspected OC was significantly associated with poor DFS (123), but had no significant impact on OS. In contrast, a similar study involving the detection of CTCs before surgery and after chemotherapy in 122 patients found that the presence of CTCs (based on EpCAM, MUC-1, and HER-2 expression) was associated with poor OS, but not DFS or PFS (124). Further research is needed to clarify the role of CTCs and the impact of specific molecular markers on outcomes in OC.
ERCC1 has also been proposed to predict poor outcomes among patients with OC; in 143 patients the presence of ERCC1-positive CTCs at diagnosis was a significant predictor of poor OS (HR, 2.5 [95% CI, 1.1–5.5]) and PFS (HR, 3.4 [95% CI, 1.4–8.3]) (104), with similar findings in another subsequent study (105).
In another study of patients with newly diagnosed OC, CTCs were detected in 98 of 109 (90%) patients (41). In this cohort, the number of CTCs was significantly associated with tumor stage (P = 0.034), and the expression of EpCAM and HER2 in CTCs was associated with chemoresistance (P=0.003 and P=0.035, respectively). The number of EpCAM-positive CTCs was significantly associated with poor OS (P=0.041).
Lee et al. (106) developed a nanoroughened microfluidic device that facilitates the enrichment of CTCs as EpCAM-positive, DAPI-positive, and CD45-negative circulating cells, and found that the number of CTCs was associated with worse PFS and platinum resistance.
Another study in patients with stage I–IV EOC showed that the presence of MUC1-positive CTCs was associated with poor OS; however, similar to previous reports, PFS was unaffected (43). A meta-analysis of data from two clinical trials and 13 retrospective studies involving 1285 patients found that the presence of CTCs was significantly associated with poor OS (HR: 1.77 [95% CI, 1.42–2.21], P <0.00001) and PFS (HR: 1.53 [95% CI, 1.26–1.86], P <0.0001) (127). A significant role of CTCs was observed across different clinical settings, including pre-treatment patients and patients undergoing debulking surgery. Notably, the predictive role of CTCs seemed to vary depending on the CTC enrichment method, which might explain the contradictory findings regarding the significance of CTCs in patients with OC.
Schwich et al. (45) found that exosomal HLA-G levels were significantly higher in patients with OC than in healthy donors. Although the total number of exosomes was not associated with outcomes, increased levels of exosomal HLA-G were associated with aggressive tumor features and poor outcomes, including residual tumor burden, high numbers of CTCs, and poor PFS.
6.3 Circulating miRNAs
Circulating miRNAs may be associated with outcomes in patients with OC (Table 3). Zuberi et al. (128) analyzed the expression levels of miR-200a, miR-200b, and miR-200c in the serum of patients with stage I–IV EOC, and found that the expression of miR-200a and miR-200c appeared to be associated with advanced disease stage and presence of metastasis. Similarly, Halvorsen et al. (129) assessed the levels of miR-1274B, miR-200b, and miR-141 in the serum of patients with OC and found that low levels of these miRNAs were associated with improved OS.
7 Conclusions
Accumulating evidence supports the diagnostic, predictive, and prognostic utility of multiple markers present in LB for OC, suggesting that LB potentially offers non-invasive, easily repeatable, accurate tools that may allow for early detection of OC and improve response prediction and early molecular profiling. Longer-term prospective studies, including cost-effectiveness analyses, are needed to assess the impact on patient outcomes. Such tools may be particularly useful among patients ineligible for surgery, who represent a notable proportion of patients with OC. However, widespread clinical implementation still faces many challenges. A key challenge is assay sensitivity and specificity for analysis of minute amounts of tumor-derived material. The accuracy of current diagnostic tests still needs to be improved. Another main challenge for current LB assays is the need for specialized equipment and technical expertise, which leads to long turnover time and high cost, making these assays inappropriate for routine clinical applications. The improvements to standardized and automated processing and analysis methods will help streamline workflow, ensure reliability and reproducibility, and reduce turnover time and cost. Moreover, many previous studies were limited by their sample sizes and designs, making their results difficult to interpret or reproduce. Longer-term prospective studies with appropriate designs, including cost-effectiveness analyses, are needed to assess the impact of LB on the outcomes of patients with OC. It is also noteworthy that most data exploring the clinical utility of LB for OC have focused on ctDNA and cfDNA; further research in larger, prospective studies regarding the clinical utility of other markers within LB for OC, such as exosomes, circulating non-coding RNAs, or to identify other markers is needed to further refine LB for clinical adoption.
Author contributions
HZ: Conceptualization, Writing – review & editing. LW: Conceptualization, Writing – original draft. HW: Conceptualization, Writing – review & editing.
Funding
The author(s) declare financial support was received for the research, authorship, and/or publication of this article. This manuscript was funded by MSD China.
Acknowledgments
Editorial assistance for this review article was provided by Christos Evangelou, PhD (Rude Health Consulting). This assistance was funded by MSD China.
Conflict of interest
LW is an employee of MSD China.
The remaining authors declare that the research was conducted in the absence of any commercial or financial relationships that could be construed as a potential conflict of interest.
Publisher’s note
All claims expressed in this article are solely those of the authors and do not necessarily represent those of their affiliated organizations, or those of the publisher, the editors and the reviewers. Any product that may be evaluated in this article, or claim that may be made by its manufacturer, is not guaranteed or endorsed by the publisher.
References
1. Sung H, Ferlay J, Siegel RL, Laversanne M, Soerjomataram I, Jemal A, et al. Global cancer statistics 2020: GLOBOCAN estimates of incidence and mortality worldwide for 36 cancers in 185 countries. CA Cancer J Clin (2021) 71(3):209–49. doi: 10.3322/caac.21660
2. Xia C, Dong X, Li H, Cao M, Sun D, He S, et al. Cancer statistics in China and United States, 2022: profiles, trends, and determinants. Chin Med J (Engl) (2022) 135(5):584–90. doi: 10.1097/CM9.0000000000002108
3. Lheureux S, Gourley C, Vergote I, Oza AM. Epithelial ovarian cancer. Lancet (2019) 393(10177):1240–53. doi: 10.1016/S0140-6736(18)32552-2
4. Lheureux S, Braunstein M, Oza AM. Epithelial ovarian cancer: Evolution of management in the era of precision medicine. CA Cancer J Clin (2019) 69(4):280–304. doi: 10.3322/caac.21559
5. Vanderpuye VD, Clemenceau JRV, Temin S, Aziz Z, Burke WM, Cevallos NL, et al. Assessment of adult women with ovarian masses and treatment of epithelial ovarian cancer: ASCO resource-stratified guideline. JCO Glob Oncol (2021) 7:1032–66. doi: 10.1200/GO.21.00085
6. Azais H, Estevez JP, Foucher P, Kerbage Y, Mordon S, Collinet P. Dealing with microscopic peritoneal metastases of epithelial ovarian cancer. A surgical challenge. Surg Oncol (2017) 26(1):46–52. doi: 10.1016/j.suronc.2017.01.001
7. Chandra A, Pius C, Nabeel M, Nair M, Vishwanatha JK, Ahmad S, et al. Ovarian cancer: Current status and strategies for improving therapeutic outcomes. Cancer Med (2019) 8(16):7018–31. doi: 10.1002/cam4.2560
8. NCCN. Clinical Practice Guidelines in Oncology version 2.2023. Available from: https://www.nccn.org/professionals/physician_gls/pdf/ovarian.pdf. (Accessed 22 Nov 2023)
9. FDA. List of cleared or approved companion diagnostic devices. In Vitro and Imaging Tools (2023). Available at: https://www.fda.gov/media/119249/download.
10. Charkhchi P, Cybulski C, Gronwald J, Wong FO, Narod SA, Akbari MR. CA125 and ovarian cancer: A comprehensive review. Cancers (Basel) (2020) 12(12). doi: 10.3390/cancers12123730
11. Van Calster B, Timmerman D, Bourne T, Testa AC, Van Holsbeke C, Domali E, et al. Discrimination between benign and Malignant adnexal masses by specialist ultrasound examination versus serum CA-125. J Natl Cancer Inst (2007) 99(22):1706–14. doi: 10.1093/jnci/djm199
12. Urban N, McIntosh MW, Andersen M, Karlan BY. Ovarian cancer screening. Hematol Oncol Clin North Am (2003) 17(4):989–1005, ix. doi: 10.1016/S0889-8588(03)00063-7
13. Buys SS, Partridge E, Black A, Johnson CC, Lamerato L, Isaacs C, et al. Effect of screening on ovarian cancer mortality: the Prostate, Lung, Colorectal and Ovarian (PLCO) Cancer Screening Randomized Controlled Trial. JAMA (2011) 305(22):2295–303. doi: 10.1001/jama.2011.766
14. Jacobs IJ, Menon U, Ryan A, Gentry-Maharaj A, Burnell M, Kalsi JK, et al. Ovarian cancer screening and mortality in the UK Collaborative Trial of Ovarian Cancer Screening (UKCTOCS): a randomised controlled trial. Lancet (2016) 387(10022):945–56. doi: 10.1016/S0140-6736(15)01224-6
15. Henderson JT, Webber EM, Sawaya GF. Screening for ovarian cancer: updated evidence report and systematic review for the US preventive services task force. JAMA (2018) 319(6):595–606. doi: 10.1001/jama.2017.21421
16. Moss HA, Berchuck A, Neely ML, Myers ER, Havrilesky LJ. Estimating cost-effectiveness of a multimodal ovarian cancer screening program in the United States: secondary analysis of the UK collaborative trial of ovarian cancer screening (UKCTOCS). JAMA Oncol (2018) 4(2):190–5. doi: 10.1001/jamaoncol.2017.4211
17. Skates SJ, Singer DE. Quantifying the potential benefit of CA 125 screening for ovarian cancer. J Clin Epidemiol (1991) 44(4-5):365–80. doi: 10.1016/0895-4356(91)90075-K
18. Zhu JW, Charkhchi P, Akbari MR. Potential clinical utility of liquid biopsies in ovarian cancer. Mol Cancer (2022) 21(1):114. doi: 10.1186/s12943-022-01588-8
19. Marrugo-Ramirez J, Mir M, Samitier J. Blood-based cancer biomarkers in liquid biopsy: A promising non-invasive alternative to tissue biopsy. Int J Mol Sci (2018) 19(10):2877. doi: 10.3390/ijms19102877
20. Michela B. Liquid biopsy: A family of possible diagnostic tools. Diagnostics (Basel) (2021) 11(8):1391. doi: 10.3390/diagnostics11081391
21. He R, Zhu B, Liu J, Zhang N, Zhang WH, Mao Y. Women's cancers in China: a spatio-temporal epidemiology analysis. BMC Womens Health (2021) 21(1):116. doi: 10.1186/s12905-021-01260-1
22. Liggett TE, Melnikov A, Yi Q, Replogle C, Hu W, Rotmensch J, et al. Distinctive DNA methylation patterns of cell-free plasma DNA in women with Malignant ovarian tumors. Gynecol Oncol (2011) 120(1):113–20. doi: 10.1016/j.ygyno.2010.09.019
23. Zhang Q, Hu G, Yang Q, Dong R, Xie X, Ma D, et al. A multiplex methylation-specific PCR assay for the detection of early-stage ovarian cancer using cell-free serum DNA. Gynecol Oncol (2013) 130(1):132–9. doi: 10.1016/j.ygyno.2013.04.048
24. Wu Y, Zhang X, Lin L, Ma XP, Ma YC, Liu PS. Aberrant methylation of RASSF2A in tumors and plasma of patients with epithelial ovarian cancer. Asian Pac J Cancer Prev (2014) 15(3):1171–6. doi: 10.7314/APJCP.2014.15.3.1171
25. Widschwendter M, Zikan M, Wahl B, Lempiainen H, Paprotka T, Evans I, et al. The potential of circulating tumor DNA methylation analysis for the early detection and management of ovarian cancer. Genome Med (2017) 9(1):116. doi: 10.1186/s13073-017-0500-7
26. Wang B, Yu L, Luo X, Huang L, Li QS, Shao XS, et al. Detection of OPCML methylation, a possible epigenetic marker, from free serum circulating DNA to improve the diagnosis of early-stage ovarian epithelial cancer. Oncol Lett (2017) 14(1):217–23. doi: 10.3892/ol.2017.6111
27. Klein EA, Richards D, Cohn A, Tummala M, Lapham R, Cosgrove D, et al. Clinical validation of a targeted methylation-based multi-cancer early detection test using an independent validation set. Ann Oncol (2021) 32(9):1167–77. doi: 10.1016/j.annonc.2021.05.806
28. Cohen PA, Flowers N, Tong S, Hannan N, Pertile MD, Hui L. Abnormal plasma DNA profiles in early ovarian cancer using a non-invasive prenatal testing platform: implications for cancer screening. BMC Med (2016) 14(1):126. doi: 10.1186/s12916-016-0667-6
29. Vanderstichele A, Busschaert P, Smeets D, Landolfo C, Van Nieuwenhuysen E, Leunen K, et al. Chromosomal instability in cell-free DNA as a highly specific biomarker for detection of ovarian cancer in women with adnexal masses. Clin Cancer Res (2017) 23(9):2223–31. doi: 10.1158/1078-0432.CCR-16-1078
30. Forshew T, Murtaza M, Parkinson C, Gale D, Tsui DW, Kaper F, et al. Noninvasive identification and monitoring of cancer mutations by targeted deep sequencing of plasma DNA. Sci Transl Med (2012) 4(136):136ra68. doi: 10.1126/scitranslmed.3003726
31. Du ZH, Bi FF, Wang L, Yang Q. Next-generation sequencing unravels extensive genetic alteration in recurrent ovarian cancer and unique genetic changes in drug-resistant recurrent ovarian cancer. Mol Genet Genomic Med (2018) 6(4):638–647. doi: 10.1002/mgg3.414
32. Barbosa A, Pinto P, Peixoto A, Guerra J, Pinheiro M, Santos C, et al. Next generation sequencing of tumor and matched plasma samples: identification of somatic variants in ctDNA from ovarian cancer patients. Front Oncol (2021) 11:754094. doi: 10.3389/fonc.2021.754094
33. Su HY, Lai HC, Lin YW, Chou YC, Liu CY, Yu MH. An epigenetic marker panel for screening and prognostic prediction of ovarian cancer. Int J Cancer (2009) 124(2):387–93. doi: 10.1002/ijc.23957
34. Melnikov A, Scholtens D, Godwin A, Levenson V. Differential methylation profile of ovarian cancer in tissues and plasma. J Mol Diagn (2009) 11(1):60–5. doi: 10.2353/jmoldx.2009.080072
35. Bondurant AE, Huang Z, Whitaker RS, Simel LR, Berchuck A, Murphy SK. Quantitative detection of RASSF1A DNA promoter methylation in tumors and serum of patients with serous epithelial ovarian cancer. Gynecol Oncol (2011) 123(3):581–7. doi: 10.1016/j.ygyno.2011.08.029
36. Dong R, Yu J, Pu H, Zhang Z, Xu X. Frequent SLIT2 promoter methylation in the serum of patients with ovarian cancer. J Int Med Res (2012) 40(2):681–6. doi: 10.1177/147323001204000231
37. Dvorska D, Brany D, Nagy B, Grendar M, Poka R, Soltesz B, et al. Aberrant methylation status of tumour suppressor genes in ovarian cancer tissue and paired plasma samples. Int J Mol Sci (2019) 20(17):4119. doi: 10.3390/ijms20174119
38. Singh A, Gupta S, Badarukhiya JA, Sachan M. Detection of aberrant methylation of HOXA9 and HIC1 through multiplex MethyLight assay in serum DNA for the early detection of epithelial ovarian cancer. Int J Cancer (2020) 147(6):1740–52. doi: 10.1002/ijc.32984
39. Pearl ML, Zhao Q, Yang J, Dong H, Tulley S, Zhang Q, et al. Prognostic analysis of invasive circulating tumor cells (iCTCs) in epithelial ovarian cancer. Gynecol Oncol (2014) 134(3):581–90. doi: 10.1016/j.ygyno.2014.06.013
40. Pearl ML, Dong H, Tulley S, Zhao Q, Golightly M, Zucker S, et al. Treatment monitoring of patients with epithelial ovarian cancer using invasive circulating tumor cells (iCTCs). Gynecol Oncol (2015) 137(2):229–38. doi: 10.1016/j.ygyno.2015.03.002
41. Zhang X, Li H, Yu X, Li S, Lei Z, Li C, et al. Analysis of circulating tumor cells in ovarian cancer and their clinical value as a biomarker. Cell Physiol Biochem (2018) 48(5):1983–94. doi: 10.1159/000492521
42. Guo YX, Neoh KH, Chang XH, Sun Y, Cheng HY, Ye X, et al. Diagnostic value of HE4+ circulating tumor cells in patients with suspicious ovarian cancer. Oncotarget (2018) 9(7):7522–33. doi: 10.18632/oncotarget.23943
43. Wang T, Gao Y, Wang X, Tian J, Li Y, Yu B, et al. Establishment of an optimized CTC detection model consisting of EpCAM, MUC1 and WT1 in epithelial ovarian cancer and its correlation with clinical characteristics. Chin J Cancer Res (2022) 34(2):95–108. doi: 10.21147/j.issn.1000-9604.2022.02.04
44. Jie XX, Zhang M, Du M, Cai QQ, Cong Q, Xu CJ, et al. Detection of circulating tumor cells and evaluation of epithelial-mesenchymal transition patterns of circulating tumor cells in ovarian cancer. Transl Cancer Res (2022) 11(8):2636–46. doi: 10.21037/tcr-22-529
45. Schwich E, Rebmann V, Horn PA, Celik AA, Bade-Doding C, Kimmig R, et al. Vesicular-bound HLA-G as a predictive marker for disease progression in epithelial ovarian cancer. Cancers (Basel) (2019) 11(8):1106. doi: 10.3390/cancers11081106
46. Zhang W, Ou X, Wu X. Proteomics profiling of plasma exosomes in epithelial ovarian cancer: A potential role in the coagulation cascade, diagnosis and prognosis. Int J Oncol (2019) 54(5):1719–33. doi: 10.3892/ijo.2019.4742
47. Liu J, Yoo J, Ho JY, Jung Y, Lee S, Hur SY, et al. Plasma-derived exosomal miR-4732-5p is a promising noninvasive diagnostic biomarker for epithelial ovarian cancer. J Ovarian Res (2021) 14(1):59. doi: 10.1186/s13048-021-00814-z
48. Todeschini P, Salviato E, Paracchini L, Ferracin M, Petrillo M, Zanotti L, et al. Circulating miRNA landscape identifies miR-1246 as promising diagnostic biomarker in high-grade serous ovarian carcinoma: A validation across two independent cohorts. Cancer Lett (2017) 388:320–7. doi: 10.1016/j.canlet.2016.12.017
49. Elias KM, Guo J, Bast RC Jr. Early detection of ovarian cancer. Hematol Oncol Clin North Am (2018) 32(6):903–14. doi: 10.1016/j.hoc.2018.07.003
50. Shen W, Shan B, Liang S, Zhang J, Yu Y, Zhang Y, et al. Hybrid capture-based genomic profiling of circulating tumor DNA from patients with advanced ovarian cancer. Pathol Oncol Res (2021) 27:581534. doi: 10.3389/pore.2021.581534
51. Liang L, Zhang Y, Li C, Liao Y, Wang G, Xu J, et al. Plasma cfDNA methylation markers for the detection and prognosis of ovarian cancer. EBioMedicine (2022) 83:104222. doi: 10.1016/j.ebiom.2022.104222
52. Yap TA, Lorente D, Omlin A, Olmos D, Bono JS. Circulating tumor cells: a multifunctional biomarker. Clin Cancer Res (2014) 20(10):2553–68. doi: 10.1158/1078-0432.CCR-13-2664
53. Attard G, de Bono JS. Utilizing circulating tumor cells: challenges and pitfalls. Curr Opin Genet Dev (2011) 21(1):50–8. doi: 10.1016/j.gde.2010.10.010
54. Jou HJ, Ling PY, Hsu HT. Circulating tumor cells as a "real-time liquid biopsy": Recent advances and the application in ovarian cancer. Taiwan J Obstet Gynecol (2022) 61(1):34–9. doi: 10.1016/j.tjog.2021.11.008
55. Poveda A, Kaye SB, McCormack R, Wang S, Parekh T, Ricci D, et al. Circulating tumor cells predict progression free survival and overall survival in patients with relapsed/recurrent advanced ovarian cancer. Gynecol Oncol (2011) 122(3):567–72. doi: 10.1016/j.ygyno.2011.05.028
56. Liu JF, Kindelberger D, Doyle C, Lowe A, Barry WT, Matulonis UA. Predictive value of circulating tumor cells (CTCs) in newly-diagnosed and recurrent ovarian cancer patients. Gynecol Oncol (2013) 131(2):352–6. doi: 10.1016/j.ygyno.2013.08.006
57. Cheng J, Liu Y, Zhao Y, Zhang L, Zhang L, Mao H, et al. Nanotechnology-assisted isolation and analysis of circulating tumor cells on microfluidic devices. Micromachines (Basel) (2020) 11(8):774. doi: 10.3390/mi11080774
58. Obermayr E, Castillo-Tong DC, Pils D, Speiser P, Braicu I, Van Gorp T, et al. Molecular characterization of circulating tumor cells in patients with ovarian cancer improves their prognostic significance – a study of the OVCAD consortium. Gynecol Oncol (2013) 128(1):15–21. doi: 10.1016/j.ygyno.2012.09.021
59. Kim M, Suh DH, Choi JY, Bu J, Kang YT, Kim K, et al. Post-debulking circulating tumor cell as a poor prognostic marker in advanced stage ovarian cancer: A prospective observational study. Med (Baltimore) (2019) 98(20):e15354. doi: 10.1097/MD.0000000000015354
60. Zhu Z, Chen Z, Wang M, Zhang M, Chen Y, Yang X, et al. Detection of plasma exosomal miRNA-205 as a biomarker for early diagnosis and an adjuvant indicator of ovarian cancer staging. J Ovarian Res (2022) 15(1):27. doi: 10.1186/s13048-022-00961-x
61. Ge L, Sun Y, Shi Y, Liu G, Teng F, Geng Z, et al. Plasma circRNA microarray profiling identifies novel circRNA biomarkers for the diagnosis of ovarian cancer. J Ovarian Res (2022) 15(1):58. doi: 10.1186/s13048-022-00988-0
62. Wang KH, Ding DC. The role and applications of exosomes in gynecological cancer: A review. Cell Transplant (2023) 32:9636897231195240. doi: 10.1177/09636897231195240
63. Vergote I, Coens C, Nankivell M, Kristensen GB, Parmar MKB, Ehlen T, et al. Neoadjuvant chemotherapy versus debulking surgery in advanced tubo-ovarian cancers: pooled analysis of individual patient data from the EORTC 55971 and CHORUS trials. Lancet Oncol (2018) 19(12):1680–7. doi: 10.1016/S1470-2045(18)30566-7
64. Kehoe S, Hook J, Nankivell M, Jayson GC, Kitchener H, Lopes T, et al. Primary chemotherapy versus primary surgery for newly diagnosed advanced ovarian cancer (CHORUS): an open-label, randomised, controlled, non-inferiority trial. Lancet (2015) 386(9990):249–57. doi: 10.1016/S0140-6736(14)62223-6
65. Onda T, Satoh T, Ogawa G, Saito T, Kasamatsu T, Nakanishi T, et al. Comparison of survival between primary debulking surgery and neoadjuvant chemotherapy for stage III/IV ovarian, tubal and peritoneal cancers in phase III randomised trial. Eur J Cancer (2020) 130:114–25. doi: 10.1016/j.ejca.2020.02.020
66. Fagotti A, Ferrandina MG, Vizzielli G, Pasciuto T, Fanfani F, Gallotta V, et al. Randomized trial of primary debulking surgery versus neoadjuvant chemotherapy for advanced epithelial ovarian cancer (SCORPION-NCT01461850). Int J Gynecol Cancer (2020) 30(11):1657–64. doi: 10.1136/ijgc-2020-001640
67. Wright AA, Bohlke K, Armstrong DK, Bookman MA, Cliby WA, Coleman RL, et al. Neoadjuvant chemotherapy for newly diagnosed, advanced ovarian cancer: society of gynecologic oncology and american society of clinical oncology clinical practice guideline. J Clin Oncol (2016) 34(28):3460–73. doi: 10.1200/JCO.2016.68.6907
68. Chao A, Chen SJ, Chen HC, Tan KT, Hsiao W, Jung SM, et al. Mutations in circulating tumor DNA detected in the postoperative period predict poor survival in patients with ovarian cancer. BioMed J (2022) 46(5):100563. doi: 10.1016/j.bj.2022.09.004
69. Hou JY, Chapman JS, Kalashnikova E, Pierson W, Smith-McCune K, Pineda G, et al. Circulating tumor DNA monitoring for early recurrence detection in epithelial ovarian cancer. Gynecol Oncol (2022) 167:334–341. doi: 10.1016/j.ygyno.2022.09.004
70. Pereira E, Camacho-Vanegas O, Anand S, Sebra R, Catalina Camacho S, Garnar-Wortzel L, et al. Personalized circulating tumor DNA biomarkers dynamically predict treatment response and survival in gynecologic cancers. PloS One (2015) 10(12):e0145754. doi: 10.1371/journal.pone.0145754
71. Roxane Mari CP, Colombo P-E, Narducci F, Garnier Séverine, Carbuccia N, Guille A, et al. Circulating tumor DNA as a noninvasive marker of resectability in ovarian carcinomas, in ASCO. J Clin Oncol (2021) 39(15_suppl):5548. doi: 10.1200/JCO.2021.39.15_suppl.5548
72. Sharbatoghli M, Fattahi F, Aboulkheyr Es H, Akbari A, Akhavan S, Ebrahimi M, et al. Copy number variation of circulating tumor DNA (ctDNA) detected using NIPT in neoadjuvant chemotherapy-treated ovarian cancer patients. Front Genet (2022) 13:938985. doi: 10.3389/fgene.2022.938985
73. Morales J, Li L, Fattah FJ, Dong Y, Bey EA, Patel M, et al. Review of poly (ADP-ribose) polymerase (PARP) mechanisms of action and rationale for targeting in cancer and other diseases. Crit Rev Eukaryot Gene Expr (2014) 24(1):15–28. doi: 10.1615/CritRevEukaryotGeneExpr.2013006875
74. Moschetta M, George A, Kaye SB, Banerjee S. BRCA somatic mutations and epigenetic BRCA modifications in serous ovarian cancer. Ann Oncol (2016) 27(8):1449–55. doi: 10.1093/annonc/mdw142
75. Telli ML, Timms KM, Reid J, Hennessy B, Mills GB, Jensen KC, et al. Homologous recombination deficiency (HRD) score predicts response to platinum-containing neoadjuvant chemotherapy in patients with triple-negative breast cancer. Clin Cancer Res (2016) 22(15):3764–73. doi: 10.1158/1078-0432.CCR-15-2477
76. Takaya H, Nakai H, Takamatsu S, Mandai M, Matsumura N. Homologous recombination deficiency status-based classification of high-grade serous ovarian carcinoma. Sci Rep (2020) 10(1):2757. doi: 10.1038/s41598-020-59671-3
77. Takamatsu S, Brown JB, Yamaguchi K, Hamanishi J, Yamanoi K, Takaya H, et al. Utility of homologous recombination deficiency biomarkers across cancer types. JCO Precis Oncol (2022) 6:e2200085. doi: 10.1200/PO.22.00085
78. Gonzalez-Martin A, Pothuri B, Vergote I, Christensen DePont R, Graybill W, Mirza MR, et al. Niraparib in patients with newly diagnosed advanced ovarian cancer. N Engl J Med (2019) 381(25):2391–402. doi: 10.1056/NEJMoa1910962
79. Coleman RL, Fleming GF, Brady MF, Swisher EM, Steffensen KD, Friedlander M, et al. Veliparib with first-line chemotherapy and as maintenance therapy in ovarian cancer. N Engl J Med (2019) 381(25):2403–15. doi: 10.1056/NEJMoa1909707
80. Ray-Coquard I, Pautier P, Pignata S, Perol D, Gonzalez-Martin A, Berger R, et al. Olaparib plus bevacizumab as first-line maintenance in ovarian cancer. N Engl J Med (2019) 381(25):2416–28. doi: 10.1056/NEJMoa1911361
81. Barretina-Ginesta MP, Monk BJ, Han S, Pothuri B, Auranen A, Chase DM, et al. Quality-adjusted time without symptoms of disease or toxicity and quality-adjusted progression-free survival with niraparib maintenance in first-line ovarian cancer in the PRIMA trial. Ther Adv Med Oncol (2022) 14:17588359221126149. doi: 10.1177/17588359221126149
82. Ray-Coquard I, Leary A, Pignata S, Cropet C, González-Martín A, Marth C, et al. Olaparib plus bevacizumab first-line maintenance in ovarian cancer: final overall survival results from the PAOLA-1/ENGOT-ov25 trial. Ann Oncol (2023) 34(8):681–92. doi: 10.1016/j.annonc.2023.05.005
83. Kamel D, et al. PARP inhibitor drugs in the treatment of breast, ovarian, prostate and pancreatic cancers: an update of clinical trials. Curr Drug Targets (2018) 19(1):21–37. doi: 10.2174/1389450118666170711151518
84. Ni J, Guo W, Zhao Q, Cheng X, Xu X, Zhou R, et al. Homologous recombination deficiency associated with response to poly (ADP-ribose) polymerase inhibitors in ovarian cancer patients: the first real-world evidence from China. Front Oncol (2021) 11:746571. doi: 10.3389/fonc.2021.746571
85. Yabing Cao HC, Huang Y, Hu H. Real-world clinical outcomes of olaparib therapy in Chinese patients with advanced serous ovarian cancer treated in Macau. Cancer Reports (2019) 2(5). doi: 10.1002/cnr2.1180
86. Zai Lab Announces NMPA Approval of ZEJULA® (Niraparib) for First-Line Maintenance Treatment of Ovarian Cancer in CHINA. (2020). Available at: https://ir.zailaboratory.com/zh-hant/node/8371/pdf.
87. Gonzalez Martin AJ, Pothuri B, Vergote IB, Graybill W, Mirza MR, Mccormick C, et al. PRIMA/ENGOT-OV26/GOG-3012 study: Updated long-term PFS and safety, in ESMO. Ann Oncol (2022) 33(Supplement 7):S789. doi: 10.1016/j.annonc.2022.07.658
88. EPAR—Assessment Report—Variation (Zejula-H-C-003943-II-0019—EMA/531223/2020). (2022). Available at: https://www.ema.europa.eu/documents/variation-report/zejula-h-c-003943-ii-0019-epar-assessment-report-variation_en.pdf.
89. Swisher EM, Wollan M, Mahtani SM, Willner JB, Garcia R, Goff BA, et al. Tumor-specific p53 sequences in blood and peritoneal fluid of women with epithelial ovarian cancer. Am J Obstet Gynecol (2005) 193(3 Pt 1):662–7. doi: 10.1016/j.ajog.2005.01.054
90. Parkinson CA, Gale D, Piskorz AM, Biggs H, Hodgkin C, Addley H, et al. Exploratory analysis of TP53 mutations in circulating tumour DNA as biomarkers of treatment response for patients with relapsed high-grade serous ovarian carcinoma: A retrospective study. PloS Med (2016) 13(12):e1002198. doi: 10.1371/journal.pmed.1002198
91. Piskorz A, Lin KK, Morris JA, Mann E, Oza AM, Coleman RL, et al. Feasibility of monitoring response to the PARP inhibitor rucaparib with targeted deep sequencing of circulating tumor DNA (ctDNA) in women with high-grade serous carcinoma on the ARIEL2 trial. J Clin Oncol (2016) 34(15_suppl):5549–9. doi: 10.1200/JCO.2016.34.15_suppl.5549
92. Kim YM, Lee SW, Lee YJ, Lee HY, Lee JE, Choi EK. Prospective study of the efficacy and utility of TP53 mutations in circulating tumor DNA as a non-invasive biomarker of treatment response monitoring in patients with high-grade serous ovarian carcinoma. J Gynecol Oncol (2019) 30(3):e32. doi: 10.1016/j.ygyno.2019.01.008
93. Christie EL, Fereday S, Doig K, Pattnaik S, Dawson SJ, Bowtell DDL. Reversion of BRCA1/2 germline mutations detected in circulating tumor DNA from patients with high-grade serous ovarian cancer. J Clin Oncol (2017) 35(12):1274–80. doi: 10.1200/JCO.2016.70.4627
94. Weigelt B, Comino-Mendez I, Bruijn I, Tian L, Meisel JL, Garcia-Murillas I, et al. Diverse BRCA1 and BRCA2 reversion mutations in circulating cell-free DNA of therapy-resistant breast or ovarian cancer. Clin Cancer Res (2017) 23(21):6708–20. doi: 10.1158/1078-0432.CCR-17-0544
95. Ratajska M, Koczkowska M, Zuk M, Gorczynski A, Kuzniacka A, Stukan M, et al. Detection of BRCA1/2 mutations in circulating tumor DNA from patients with ovarian cancer. Oncotarget (2017) 8(60):101325–32. doi: 10.18632/oncotarget.20722
96. Lin KK, Harrell MI, Oza AM, Oaknin A, Ray-Coquard I, Tinker AV, et al. BRCA reversion mutations in circulating tumor DNA predict primary and acquired resistance to the PARP inhibitor rucaparib in high-grade ovarian carcinoma. Cancer Discovery (2019) 9(2):210–9. doi: 10.1158/2159-8290.CD-18-0715
97. Morikawa A, Hayashi T, Shimizu N, Kobayashi M, Taniue K, Takahashi A, et al. PIK3CA and KRAS mutations in cell free circulating DNA are useful markers for monitoring ovarian clear cell carcinoma. Oncotarget (2018) 9(20):15266–74. doi: 10.18632/oncotarget.24555
98. Arend RC, Londono AI, Montgomery AM, Smith HJ, Dobbin ZC, Katre AA, et al. Molecular response to neoadjuvant chemotherapy in high-grade serous ovarian carcinoma. Mol Cancer Res (2018) 16(5):813–24. doi: 10.1158/1541-7786.MCR-17-0594
99. Giannopoulou L, Mastoraki S, Buderath P, Strati A, Pavlakis K, Kasimir-Bauer S, et al. ESR1 methylation in primary tumors and paired circulating tumor DNA of patients with high-grade serous ovarian cancer. Gynecol Oncol (2018) 150(2):355–60. doi: 10.1016/j.ygyno.2018.05.026
100. Rusan M, et al. Circulating HOXA9-methylated tumour DNA: A novel biomarker of response to poly (ADP-ribose) polymerase inhibition in BRCA-mutated epithelial ovarian cancer. Eur J Cancer (2020) 125:121–9. doi: 10.1016/j.ejca.2019.11.012
101. Faaborg L, Andersen RF, Waldstrom M, Henriksen JR, Adimi P, Jakobsen A, et al. Prognostic impact of circulating methylated homeobox A9 DNA in patients undergoing treatment for recurrent ovarian cancer. Cancers (Basel) (2022) 14(7):1766. doi: 10.3390/cancers14071766
102. Oikkonen J, Zhang K, Salminen L, Schulman I, Lavikka K, Andersson N, et al. Prospective longitudinal ctDNA workflow reveals clinically actionable alterations in ovarian cancer. JCO Precis Oncol (2019) 3. doi: 10.1200/PO.18.00343
103. Alves MC, Fonseca FLA, Yamada A, Barros L, Lopes A, Silva L, et al. Increased circulating tumor DNA as a noninvasive biomarker of early treatment response in patients with metastatic ovarian carcinoma: A pilot study. Tumour Biol (2020) 42(5):1010428320919198. doi: 10.1177/1010428320919198
104. Kuhlmann JD, Wimberger P, Bankfalvi A, Keller T, Scholer S, Aktas B, et al. ERCC1-positive circulating tumor cells in the blood of ovarian cancer patients as a predictive biomarker for platinum resistance. Clin Chem (2014) 60(10):1282–9. doi: 10.1373/clinchem.2014.224808
105. Chebouti I, Kuhlmann JD, Buderath P, Weber S, Wimberger P, Bokeloh Y, et al. ERCC1-expressing circulating tumor cells as a potential diagnostic tool for monitoring response to platinum-based chemotherapy and for predicting post-therapeutic outcome of ovarian cancer. Oncotarget (2017) 8(15):24303–13. doi: 10.18632/oncotarget.13286
106. Lee M, Kim EJ, Cho Y, Kim S, Chung HH, Park NH, et al. Predictive value of circulating tumor cells (CTCs) captured by microfluidic device in patients with epithelial ovarian cancer. Gynecol Oncol (2017) 145(2):361–5. doi: 10.1016/j.ygyno.2017.02.042
107. You Y, Li L, Lu J, Wu H, Wang J, Gao J, et al. Germline and Somatic BRCA1/2 Mutations in 172 chinese women with epithelial ovarian cancer. Front Oncol (2020) 10:295. doi: 10.3389/fonc.2020.00295
108. Bu H, Chen J, Li Q, Hou J, Wei Y, Yang X, et al. BRCA mutation frequency and clinical features of ovarian cancer patients: A report from a Chinese study group. J Obstet Gynaecol Res (2019) 45(11):2267–74. doi: 10.1111/jog.14090
109. Shi T, Wang P, Xie C, Yin S, Shi D, Wei C, et al. BRCA1 and BRCA2 mutations in ovarian cancer patients from China: ethnic-related mutations in BRCA1 associated with an increased risk of ovarian cancer. Int J Cancer (2017) 140(9):2051–9. doi: 10.1002/ijc.30633
110. Lukashchuk N, Armenia J, Tobalina L, Carr TH, Milenkova T, Liu YL, et al. BRCA reversion mutations mediated by microhomology-mediated end joining (MMEJ) as a mechanism of resistance to PARP inhibitors in ovarian and breast cancer., in ASCO. J Clin Oncol (2022) 40(16_suppl):5559. doi: 10.1200/JCO.2022.40.16_suppl.5559
111. Au Yeung CL, Co NN, Tsuruga T, Yeung TL, Kwan SY, Leung CS, et al. Exosomal transfer of stroma-derived miR21 confers paclitaxel resistance in ovarian cancer cells through targeting APAF1. Nat Commun (2016) 7:11150. doi: 10.1038/ncomms11150
112. Kanlikilicer P, Bayraktar R, Denizli M, Rashed MH, Ivan C, Aslan B, et al. Exosomal miRNA confers chemo resistance via targeting Cav1/p-gp/M2-type macrophage axis in ovarian cancer. EBioMedicine (2018) 38:100–12. doi: 10.1016/j.ebiom.2018.11.004
113. Zhu X, Shen H, Yin X, Yang M, Wei H, Chen Q, et al. Macrophages derived exosomes deliver miR-223 to epithelial ovarian cancer cells to elicit a chemoresistant phenotype. J Exp Clin Cancer Res (2019) 38(1):81. doi: 10.1186/s13046-019-1095-1
114. Feng Y, Hang W, Sang Z, Li S, Xu W, Miao Y, et al. Identification of exosomal and nonexosomal microRNAs associated with the drug resistance of ovarian cancer. Mol Med Rep (2019) 19(5):3376–92. doi: 10.3892/mmr.2019.10008
115. Li N, Yang L, Wang H, Yi T, Jia X, Chen C, et al. MiR-130a and miR-374a function as novel regulators of cisplatin resistance in human ovarian cancer A2780 cells. PloS One (2015) 10(6):e0128886. doi: 10.1371/journal.pone.0128886
116. Minato T, Ito S, Li B, Fujimori H, Mochizuki M, Yamaguchi K, et al. Liquid biopsy with droplet digital PCR targeted to specific mutations in plasma cell-free tumor DNA can detect ovarian cancer recurrence earlier than CA125. Gynecol Oncol Rep (2021) 38:100847. doi: 10.1016/j.gore.2021.100847
117. Giannopoulou L, Chebouti I, Pavlakis K, Kasimir-Bauer S, Lianidou ES. RASSF1A promoter methylation in high-grade serous ovarian cancer: A direct comparison study in primary tumors, adjacent morphologically tumor cell-free tissues and paired circulating tumor DNA. Oncotarget (2017) 8(13):21429–43. doi: 10.18632/oncotarget.15249
118. Kamat AA, Baldwin M, Urbauer D, Dang D, Han LY, Godwin A, et al. Plasma cell-free DNA in ovarian cancer: an independent prognostic biomarker. Cancer (2010) 116(8):1918–25. doi: 10.1002/cncr.24997
119. No JH, Kim K, Park KH, Kim YB. Cell-free DNA level as a prognostic biomarker for epithelial ovarian cancer. Anticancer Res (2012) 32(8):3467–71.
120. Kuhlmann JD, Schwarzenbach H, Wimberger P, Poetsch M, Kimmig R, Kasimir-Bauer S. LOH at 6q and 10q in fractionated circulating DNA of ovarian cancer patients is predictive for tumor cell spread and overall survival. BMC Cancer (2012) 12:325. doi: 10.1186/1471-2407-12-325
121. Marth C, Kisic J, Kaern J, Trope C, Fodstad O. Circulating tumor cells in the peripheral blood and bone marrow of patients with ovarian carcinoma do not predict prognosis. Cancer (2002) 94(3):707–12. doi: 10.1002/cncr.10250
122. Judson PL, Geller MA, Bliss RL, Boente MP, Downs LS Jr, Argenta PA, et al. Preoperative detection of peripherally circulating cancer cells and its prognostic significance in ovarian cancer. Gynecol Oncol (2003) 91(2):389–94. doi: 10.1016/j.ygyno.2003.08.004
123. Fan T, Zhao Q, Chen JJ, Chen WT, Pearl ML. Clinical significance of circulating tumor cells detected by an invasion assay in peripheral blood of patients with ovarian cancer. Gynecol Oncol (2009) 112(1):185–91. doi: 10.1016/j.ygyno.2008.09.021
124. Aktas B, Kasimir-Bauer S, Heubner M, Kimmig R, Wimberger P. Molecular profiling and prognostic relevance of circulating tumor cells in the blood of ovarian cancer patients at primary diagnosis and after platinum-based chemotherapy. Int J Gynecol Cancer (2011) 21(5):822–30. doi: 10.1097/IGC.0b013e318216cb91
125. Obermayr E, Bednarz-Knoll N, Orsetti B, Weier HU, Lambrechts S, Castillo-Tong DC, et al. Circulating tumor cells: potential markers of minimal residual disease in ovarian cancer? a study of the OVCAD consortium. Oncotarget (2017) 8(63):106415–28. doi: 10.18632/oncotarget.22468
126. Paracchini L, Beltrame L, Grassi T, Inglesi A, Fruscio R, Landoni F, et al. Genome-wide copy-number alterations in circulating tumor DNA as a novel biomarker for patients with high-grade serous ovarian cancer. Clin Cancer Res (2021) 27(9):2549–59. doi: 10.1158/1078-0432.CCR-20-3345
127. Huang C, Lin X, He J, Liu N. Enrichment and detection method for the prognostic value of circulating tumor cells in ovarian cancer: A meta-analysis. Gynecol Oncol (2021) 161(2):613–20. doi: 10.1016/j.ygyno.2021.02.024
128. Zuberi M, Mir R, Das J, Ahmad I, Javid J, Yadav P, et al. Expression of serum miR-200a, miR-200b, and miR-200c as candidate biomarkers in epithelial ovarian cancer and their association with clinicopathological features. Clin Transl Oncol (2015) 17(10):779–87. doi: 10.1007/s12094-015-1303-1
Keywords: ovarian cancer, liquid biopsy, circulating tumor DNA, cell-free DNA, poly(ADP)ribose polymerase inhibitors, China
Citation: Zhang H, Wang L and Wu H (2023) Liquid biopsy in ovarian cancer in China and the world: current status and future perspectives. Front. Oncol. 13:1276085. doi: 10.3389/fonc.2023.1276085
Received: 11 August 2023; Accepted: 17 November 2023;
Published: 19 December 2023.
Edited by:
Deyin Xing, Johns Hopkins University, United StatesReviewed by:
Yan Shen, Tianjin Central Hospital of Gynecology Obstetrics, ChinaKaushlendra Tripathi, University of Alabama at Birmingham, United States
Copyright © 2023 Zhang, Wang and Wu. This is an open-access article distributed under the terms of the Creative Commons Attribution License (CC BY). The use, distribution or reproduction in other forums is permitted, provided the original author(s) and the copyright owner(s) are credited and that the original publication in this journal is cited, in accordance with accepted academic practice. No use, distribution or reproduction is permitted which does not comply with these terms.
*Correspondence: Huanwen Wu, d3VodWFud2VuMTA3MDBAcHVtY2guY24=