- 1Department of Otolaryngology-Head and Neck Surgery, First Affiliated Hospital of Guangxi Medical University, Nanning, China
- 2Department of Pediatric Dentistry, College & Hospital of Stomatology, Guangxi Medical University, Nanning, China
- 3Key Laboratory of Early Prevention and Treatment for Regional High-Frequency Tumor, Guangxi Medical University, Ministry of Education, Nanning, China
Lipid metabolism reprogramming is one of the most prominent metabolic anomalies in cancer, wherein cancer cells undergo dysregulation of lipid metabolism to acquire adequate energy, cell membrane building blocks, as well as signaling molecules essential for cell proliferation, survival, invasion, and metastasis. These adaptations enable cancer cells to effectively respond to challenges posed by the tumor microenvironment, leading to cancer therapy resistance and poor cancer prognosis. Head and neck cancer, ranking as the seventh most prevalent cancer, exhibits numerous abnormalities in lipid metabolism. Nevertheless, the precise role of lipid metabolic rewiring in head and neck cancer remains unclear. In line with the LIPID MAPS Lipid Classification System and cancer risk factors, the present review delves into the dysregulated molecules and pathways participating in the process of lipid uptake, biosynthesis, transportation, and catabolism. We also present an overview of the latest advancements in understanding alterations in lipid metabolism and how they intersect with the carcinogenesis, development, treatment, and prognosis of head and neck cancer. By shedding light on the significance of metabolic therapy, we aspire to improve the overall prognosis and treatment outcomes of head and neck cancer patients.
1 Introduction
Reprogramming of lipid metabolism is a critical hallmark of cancer. In recent years, dysregulated lipid metabolism has emerged as a focal point in cancer research (1). Physiologically, lipids play essential roles as energy sources, vital building blocks for cell membranes, as well as first and second messengers in molecular recognition and signaling processes (2, 3). Pathologically, any disturbance in lipid metabolism would significantly impact serious cellular behaviors, such as cell proliferation, differentiation, apoptosis, and motility, ultimately contributing to carcinogenesis and cancer development (3). In addition, rewiring of lipid metabolism impacts the treatment effects and the outcomes of cancer patients (4). Thus, lipid metabolism-related molecules and pathways have been proposed to be novel targets of anti-cancer treatment.
Head and neck cancer (HNC) comprises a group of tumors that predominantly arise from subsites within the oral cavity, oropharynx, hypopharynx, and larynx (5). It ranks as the seventh most common cancer worldwide, with more than 890,000 new cases and 450,000 deaths each year (6). Head and neck squamous cell carcinoma (HNSCC) accounts for approximately 90% of all HNC cases (7). Infection of human papillomavirus (HPV), tobacco use or smoking, and alcohol consumption are the three well-known risk factors for head and neck cancer (6, 8). Despite notable advancements in diagnosis and treatment, the 5-year overall survival rate for head and neck cancer patients with an advanced stage is 50% to 60%, indicating an urgent demand for further investigation into the underlying mechanisms of head and neck cancer carcinogenesis (9).
Lipid metabolism reprogramming is also linked to the carcinogenesis and development of head and neck cancer. Cancer cells actively promote the lipolysis process. And in turn, the various endpoints of lipid metabolism, including the oxidation of fatty acids, the generation of signaling lipids, epigenetic modifications of proteins, synthesis of cell membrane lipid, and other crucial processes, profoundly affect the malignant characteristics of cancer cells. Accumulated studies have described the dysregulated levels or activities of enzymes and molecules associated with lipid metabolism in head and neck cancer. Alterations of lipid profile in head and neck cancer are evident; for instance, there’s an elevation of fatty acid C16:0 and a reduction in total ceramide (10). In addition, overexpression of crucial components in lipid metabolism, such as cluster of differentiation 36 (CD36), fatty acid-binding protein (FABP), acetyl-CoA carboxylase (ACC), and fatty acid synthase (FASN), have been well-documented in head and neck cancer (11–14). Hence, the molecules involved in the lipid metabolism alteration hold potential as novel biomarkers for the treatment and prognosis of head and neck cancer. Nonetheless, the precise role and underlying mechanisms of lipid metabolism in the context of head and neck cancer remain inadequately understood.
According to the LIPID MAPS Lipid Classification System, lipids are divided into eight categories, known as fatty acids, glycerolipids, glycerophospholipids, sphingolipids, sterol lipids, prenol lipids, saccharolipids, and polyketides (15). In the present review, based on the lipid classification system and cancer risk factors, we focused on the dysregulation of lipid metabolism and its implications on the carcinogenesis, treatment, and prognosis of head and neck cancer. Novel dysregulated molecules and signaling pathways participating in the process of lipid uptake, de novo synthesis, transportation, catabolism, as well as their roles in the composition of lipid profiles, would be involved and discussed, aiming to provide an updated and comprehensive understanding of this field and potential strategies for personalized treatment of head and neck cancer (Figure 1, Table 1).
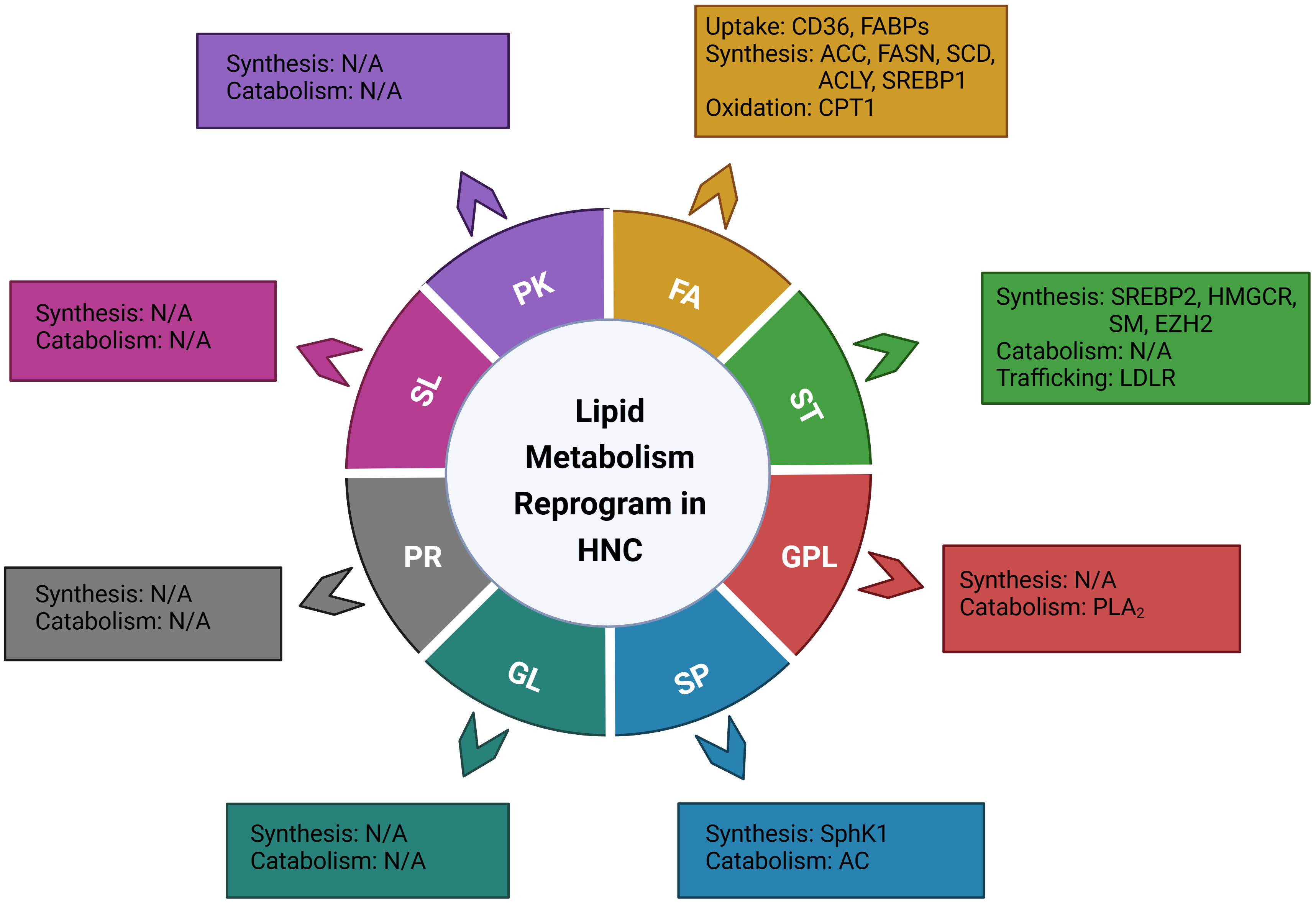
Figure 1 Illustration of the genes or enzymes that have been reported to be involved in the reprograming of lipid metabolism in head and neck cancer, based on the LIPID MAPS Lipid Classification System. FA, fatty Acid; ST, sterol lipids; GPL, glycerophospholipids; SP, sphingolipids; GL, glycerolipids; PR, prenol lipids; SL, saccharolipids; PK, polyketides; CD36, cluster of differentiation 36; FABPs; fatty acid-binding proteins; ACC, acetyl-CoA carboxylase; FASN, fatty acid synthase; SCD, stearoyl-CoA desaturase; ACLY, ATP citrate lyase; SREBP1, sterol regulatory element binding proteins 1; CPT1, Carnitine Palmitoyl Transferase 1; SREBP2, sterol regulatory element-binding protein 2; HMGCR, 3-hydroxy-3-methylglutaryl (HMG)–CoA reductase; SM, Squalene monooxygenase; EZH2, Enhancer of zeste homolog 2; LDLR, low-density lipoprotein receptor; PLA2, phospholipase A2; cPLA2, cytosolic PLA2; sPLA2-IIA, secretory phospholipase A2 IIA; SphK1, Sphingosine kinase 1; AC, Acid ceramidase.
2 Lipid metabolism reprogram in head and neck cancer
2.1 Alteration of fatty acid metabolism in head and neck cancer
Fatty acids are composed of saturated or unsaturated hydrocarbon chains terminating with distinct carboxylic acid groups. Mammals acquire fatty acids from the surrounding environment or by synthesizing de novo (31). The metabolism of fatty acids encompasses two key biological processes: fatty acid synthesis and fatty acid oxidation (FAO) (32).
2.1.1 De novo biosynthesis and catabolism of fatty acids
Fatty acid biosynthesis occurs in the cytosol. The first step involves the conversion of acetyl-CoA into malonyl-CoA through the activation of ACC. This, along with the participation of enzymes such as FASN, leads to the generation of palmitate (FA16:0). Subsequently, elongation processes produce fatty acids of different lengths. These fatty acids can then be esterified with glycerol or sterol skeletons, forming triacylglycerol (TG) or sterol esters, respectively. Finally, they are stored as lipid droplets (LDs) (33). On the other hand, fatty acid catabolism involves the activation of FAs in the cytoplasm to form acyl-CoA. The formed acyl-CoA is then transported to the mitochondrial matrix by carnitine palmitoyltransferase 1 (CPT1) for oxidation (34).
2.1.2 Altered fatty acid profile in head and neck cancer
Dysregulated lipid metabolism in head and neck cancer patients results in a distinctive fatty acid profile. In the context of oral squamous cell carcinoma (OSCC) tissues, it was noted that when compared with healthy controls, the level of fatty acids was frequently elevated, with the most significant increase observed in palmitic acid (C16:0), followed by oleic acid (C18:1) (16). In another study including 30 OSCC patients, Domagala et al. noted a remarkable increase of C16:0 in tumor, adjacent tissue, and blood serum samples. Independent of tumor grade, the levels of oleic acid (C18:1n9), erucic acid (C22:1n13), docosatetraenoic acid (C22:4n6), docosapentaenoic acid (C22:5n3), and nervonic acid (C24:1) were found to be more abundant in tumor-adjacent tissues than in serum, suggesting the potential roles of these fatty acids in promoting tumor progression in OSCC (10). In an explorative study, Laurell et al. compared the circulating lipidomic profile of HNC patients one year before and after treatment. A specific pattern emerged for FA 14:0, 18:3n3, and 20:3n6, showing an early reduction in FA 14:0 and late reductions in FA 18:3n-3 and 20:3n-6. Additionally, FA 14:0 was associated with changes in body weight (17). Taken together, the level of C16:0 is supposed to be increased in head and neck cancer. Nevertheless, a definitive fatty acid profile specific to head and neck cancer remains elusive. To address this, further investigation with a larger sample size and a more rigorous study design is imperative.
2.1.3 Increased fatty acids uptake in head and neck cancer
2.1.3.1 Cluster of differentiation 36
CD36, known as a fatty acid translocase, is responsible for binding and trafficking FAs from the exogenous environment into host cells. Its upregulation has been consistently observed in various human cancers, such as colorectal cancer (35), gastric cancer (36, 37), hepatocellular carcinogenesis (38), and melanoma (39). CD36 plays a vital role in modulating cancer development, metastasis, therapy resistance, and prognosis (40–44). Notably, CD36 is highly expressed in HNC (11, 45–47). Increased expression of CD36 was significantly associated with higher tumor status, tumor grading, and lymph node metastasis rate, exhibiting an over 40-fold increase in oral cancers (45, 46). An intriguing study by Pascual et al. reported that dietary palmitic acid could activate CD36 and accelerate cancer growth in OSCC, suggesting that dietary intake of fatty acids might play a role in CD36 modulation (48). In mice model, inhibition of CD36 has been shown to attenuate the metastasis of several cancers (41, 49, 50), including HNSCC (45). In particular, depletion of CD36 led to a significant inhibition of the lung metastasis ability of OSCC cells (45). Taken together, these findings suggest that CD36 could be a promising therapeutic target for clinical intervention of head and neck cancer.
2.1.3.2 Fatty acid-binding proteins
FABPs are important lipid chaperones, facilitating the transportation of long-chain fatty acids (LCFAs) to specific cell compartments. There are a series of members in the FABP family. The abnormal expression of FABPs in malignant tumors is associated with carcinogenesis, progression, and prognosis (51–55). In the case of HNC, there is notable upregulation of epidermal FABP (E-FABP, also known as FABP5), which might contribute to cancer proliferation and invasiveness (56–58). E-FABP, regulated by the epithelial cell adhesion molecule (EpCAM), is suggested to be a potential target of the oncogene c-Myc, leading to enhanced cell proliferation (57). Interestingly, according to Uma et al., FABP5 exhibited a lower expression in metastatic lymph nodes when compared with the corresponding primary squamous cell carcinoma of the oral tongue tumors (12). Yet, more samples are needed to confirm the conclusion. Furthermore, a study by Ohyama et al. reported abnormal expression of FABP4 and FABP5 in tongue carcinoma. Besides, the cytoplasmic staining for FABP5 was increased in tongue carcinoma patients with advanced T-stage and clinical stage, implicating that FABP5 might be a pathological marker (59). However, the precise mechanisms of FABPs in head and neck cancer need further research and investigation.
2.1.4 Dysregulated fatty acids synthesis in head and neck cancer
2.1.4.1 Acetyl-CoA carboxylases
ACCs serve as rate-limiting enzymes in fatty acid synthesis, playing a crucial role in catalyzing the carboxylation of acetyl-CoA to malonyl-CoA (60). The two isoforms of ACC, namely ACC1 (ACCα) and ACC2 (ACCβ), are enriched in lipogenic and oxidative status, respectively (61). ACCs are highly expressed in several cancer types, including prostate cancer, breast cancer, and HNC (62–64). Notably, elevated expression of ACC2 in laryngeal carcinoma correlates with advanced clinical cancer stage, lower cancer differentiation degree, as well as poor survival rates (64). It is known that ACC is regulated by AMP-activated protein kinase (AMPK) via phosphorylation (65). The same modulation also applies within the context of head and neck cancer (66). HNSCC cells with ACC mutations and lacking the AMPK phosphorylation sites, showed resistance to cetuximab treatment (67). With the combination treatment of ACC inhibitor 5-(tetradecyloxy)-2-furoic acid (TOFA) and cetuximab, the growth of cetuximab-resistant HNSCC xenografts was effectively suppressed, suggesting that ACC can rewrite cancer metabolism from glycolysis-dependent to lipogenesis-dependent way (67). However, Chen et al. reported that an enhanced level of pACC was independently associated with poor overall survival of HNSCC patients, particularly in patients with lymph node-metastasis and advanced stage (13). The potential causes of contradiction may stem from various factors, including different ACC subtypes, diverse confounding factors (e.g., TNM stage, primary tumor site), and variation in experimental systems (e.g., in vivo, in vitro, or patient-based studies), among others. Additionally, phosphorylation of ACC is not only governed by AMPK; other kinases such as protein kinase C (PKC) and casein kinase 2 (CK2) also serve as regulators (60) Therefore, further evaluation is warranted to explore the modulation of ACC and its role in head and neck cancer.
2.1.4.2 Fatty acid synthase
FASN is a critical enzyme responsible for generating long-chain fatty acids from acetyl-CoA and malonyl-CoA (68). The level of FASN is naturally low or even undetectable in most human tissues except lactating breasts and cyclical endometrium, as the daily requirements of fatty acids are adequately met by food consumption (68, 69). In contrast, high levels of FASN are observed in various malignancies, which have been linked to increased risks of cancer metastasis, recurrence, and poor survival (70–74). In accordance with this, the expression of FASN is obviously elevated in HNSCC (14, 75–77). Salivary gland carcinoma, including well-differentiated carcinoma (secretory carcinoma, acinic cell carcinoma, etc.), high-grade adenoid cystic carcinoma, and mucoepidermoid carcinoma, also show an increased level of FASN, suggesting its potential role as an indicator of cancer aggressiveness and differentiation (78). Upregulation of FASN is required for the proliferation of oral squamous carcinoma (79). Pathological analysis has indicated that FASN expression was correlated with lymphatic infiltration, perineural infiltration, and regional lymph node metastasis status in OSCC (76). Moreover, high expression of FASN was related to poor prognosis and might be an indicator of pulmonary metastasis in patients with HNSCC (76, 77, 80).
FASN is positively regulated by the cell surface receptor ErbB2 in HNSCC (75, 80, 81), though the precise correlation and underlying mechanisms need further investigation. As a FASN inhibitor, orlistat has demonstrated promising effects in inhibiting the proliferation and metastasis of orthotopic tongue oral squamous cell carcinoma (82). Further, in vivo studies have shown that orlistat reduces the cervical lymph node metastasis rate by 43% (82). Additionally, orlistat or FASN siRNA treatment increased cell cytotoxicity and cell sensitivity to radiotherapy in the radioresistant HNSCC cell line rSCC-61 (83). Orlistat also increased the chemosensitivity of OSCC cells to cisplatin and paclitaxel by downregulating cyclin B1 (84). Moreover, other FASN inhibitors, such as C75 and Tvb-3166, have been shown potential as antineoplastic agents (84, 85). Further research is needed to explore the therapeutic implications of targeting FASN in HNC treatment.
2.1.4.3 Stearoyl-CoA desaturase
SCD is a lipid-modifying enzyme catalyzing the mono-saturation of oleate (18:1) and palmitoleate (16:1) (86). There are two isoforms of SCD (SCD1 and SCD5) required for human lipid metabolism (87). In a series of human malignancies such as lung, breast, colorectal, and bladder cancers, SCD is found to be overexpressed, and its upregulation is associated with tumor aggressiveness, making it a novel prognostic marker (88–90). In OSCC, elevated expression of SCD was negatively correlated with survival (91). Interestingly, overexpression of SCD was detected not only in HNSCC cell lines but also in tobacco-treated normal oral keratinocytes. Inhibition of SCD hampers cell proliferation, invasion, and colony formation, suggesting the potential roles of SCD and tobacco in the carcinogenesis of HNSCC. Thus, SCD has been proposed as a therapeutic target for HNSCC patients, particularly those with a history of tobacco use (92).
2.1.4.4 ATP citrate lyase
ACLY is a cytosolic enzyme which is responsible for acetyl-CoA synthesis during de novo lipogenesis (93). It is frequently upregulated in various malignancies, such as colorectal cancer (94), glioblastoma (95), endometrial cancer (96), and non-small cell lung cancer (97). In HNSCC, ACLY is also upregulated (91, 98) and its heightened expression is associated with an unfavorable prognosis (98). In addition, elevated ACLY expression is associated with the failure of radiotherapy. Notably, treatment of HNSCC cells with the ACLY inhibitor BMS303141 has been demonstrated to facilitate radiosensitivity via disturbing the DNA damage repair process (98), implying the potential role of ACLY inhibitor as a sensitizer for head and neck cancer treatment. Additionally, acetyl-CoA, generated by ACLY, is essential for acetylation reactions, particularly histone acetylation. Thus, ACLY is a critical node which links cellular metabolism to epigenetic modification. In nasopharyngeal carcinoma, ACLY is found to be protected from ubiquitin degradation through its interaction with the long noncoding RNA TINCR, thereby promoting cancer cell proliferation, metastasis, and chemotherapy resistance (99). Nonetheless, there’re limited reports on the role of ACLY in head and neck cancer, warranting further in-depth investigation.
2.1.4.5 Sterol regulatory element binding proteins
SREBPs is a family of transcription factors located in the endoplasmic reticulum. In mammalian cells, three SREBPs (SREBP-1a, -1c, and -2) are expressed, which are encoded by SREBP1 and SREBP2, respectively. Among them, SREBP1 is a major transcriptional regulator of fatty acid synthesis, while SREBP2 is mainly responsible for cholesterol metabolism, which will be discussed later. SREBP1 is highly activated in cancers, and its overexpression is correlated with increased cancer aggressiveness (100–105). It has been reported that overexpression of SREBP1 is necessary for HNSCC cell proliferation and migration (106). Furthermore, SREBP1 is noted to be an important linker between tumor protein p63 (TP63) and fatty acid metabolism, suggesting its potential role as an independent prognostic and therapeutic marker in HNSCC (106). Consistently, Su et al. reported that SREBP1 functions as an oncogene via upregulating steroidogenic acute regulatory protein-related lipid transfer 4 (STARD4) and promoting immune cell infiltration in HNSCC (107). Furthermore, SREBP1-mediated cell survival is disturbed by antioxidant resveratrol by inducing autophagy and subsequently inhibiting lipid metabolism in oral cancer (108). Hence, SREBP1 is postulated to function as an oncogene, suggesting its potential as a viable treatment target of head and neck cancer.
2.1.5 Increased fatty acids oxidation
Physiologically, fatty acids are catabolized via the mitochondrial fatty acid β-oxidation (FAO) pathway to meet the human daily energy requirements (109). In carcinogenesis and tumor progression, FAO plays a vital role in modulating various malignant behaviors, for example, tumor growth, survival, stemness, drug resistance, and metastasis (110).
2.1.5.1 Carnitine palmitoyl transferase 1
The first and rate-limiting step of FAO is catalyzed by CPT1, comprising three isoforms, CPT1A, CPT1B, and CPT1C (111). CPT1 has been extensively studied in a variety of cancers, and overexpression of CPT1A accelerates tumor development by stimulating FAO in prostatic cancer (112), breast cancer (113), gastric cancer (114), and hepatocellular cancer (115). In addition to its role in FAO, CPT1 plays a pivotal role in a series of signaling pathways to modulate the gene expression, apoptosis, and neovascularization (116). Intriguingly, CPT1 is another molecular linking lipid metabolism and epigenetic modification. CPT1 coimmunoprecipitates with histone deacetylase 1 (HDAC1) in the nuclear extracts from MCF-7 cells, promoting tumor cell proliferation (117). Treatment with HDAC inhibitors decreased the nuclear expression of CPT1 (117), indicating the potential role of CPT1 as a therapeutic target for epigenetic and metabolic interventions in cancer. However, research on CPT1 in head and neck cancer remain limited. According to Cao et al., CPT1A was consistently activated in radioresistant nasopharyngeal carcinoma (NPC) cells, and was positively correlated with the poor overall survival of NPC patients undergoing radiotherapy. In oropharyngeal squamous cell carcinoma, Barros-Filho et al. confirmed that elevated CPT1A expression was associated with poor survival (118). However, in HNSCC, Lin et al. observed no statistically significant correlations between CPT1 expression and patient age, as well as no statistically significant differences in CPT expression between early and late tumor stages (T1/T2 stage vs. T3/T4 stage) (119). Thus, it is imperative to conduct further investigation into the role and associated mechanisms of CPT1 in head and neck cancer, and election bias or other confounding factors should be taken in to consideration.
2.2 Alteration of glycerolipid metabolism in head and neck cancer
2.2.1 De novo biosynthesis and catabolism of glycerolipid
The synthesis of glycerolipid utilizes fatty acyl-coenzyme A (FA-CoA) and glycerol-3-phosphate (Gly3P) as substrates. FA-CoA condenses with Gly3P via glycerophosphate acyltransferase, leading to the formation of lysophosphatidic acid (LPA). LPA is subsequently converted to phosphatidic acid by 1-acyl-sn-Gly3P acyltransferase (AGPAT), followed by the production of diacylglycerol (DAG) through the functions of phosphatidic acid phosphatase (PAP) or phospholipids (PLs). DAG, in turn, generates TG by activating DAG acyltransferase (DGAT) (120). During the catabolism of neutral TG, adipose triglyceride lipase (ATGL) first converts TG into diacylglycerols (DGs), and then hormone-sensitive lipase (HSL) hydrolyzes DGs to form monoacylglycerols (MGs). Finally, MG lipase (MGL) hydrolyzes MGs and generates fatty acids and glycerol (121).
2.2.2 Altered glycerolipid profile in head and neck cancer
It has been reported that the plasma level of triglyceride is significantly lower in HNC patients (18, 19). Constantly, a notable decrease of triglyceride was revealed in HNSCC and lymph node metastasis tissues by high-resolution magic-angle spinning (HR-MAS) proton NMR spectroscopy (20). Furthermore, the serum level of triglyceride in precancerous oral lesions as well as oral cancer was found to be reduced when compared with healthy controls. Similarly, the plasma level of triglycerides was also decreased in oral cancer patients when compared to pre-cancerous subjects (21).
2.2.3 Dysregulated glycerolipid biosynthesis and catabolism in head and neck cancer
2.2.3.1 Adipose triglyceride lipase
ATGL, also known as phospholipase A2 (PLA2), or patatin-like phospholipase domain containing 2 (PNPLA2), functions as a key enzyme hydrolyzing TGs to DAGs. Dysregulated expression of ATGL has been noted in a series of human cancers. The expression of ATGL in lung cancer is enhanced, indicating an unfavorable survival (122). Increased ATGL has been shown to promote the tumorigenesis of colon cancer in an obesity-augmented manner (123). In HNSCC, including oral cancer and tongue cancer, Ramamoorthy et al. reported a significantly increased activity of ATGL (124). Conversely, our research showed that ATGL was downregulated, leading to lipid droplets accumulation in NPC. Furthermore, decreased ATGL was associated with poor prognosis of NPC (125, 126). The variation of ATGL expression might be due to different anatomical regions of the HNSCC. Moreover, the mechanisms underlying the role of ATGL in head and neck cancer need further evaluation.
2.3 Alteration of glycerophospholipids metabolism in head and neck cancer
2.3.1 De novo biosynthesis and catabolism of glycerophospholipids
The substrate of glycerophospholipid biosynthesis is fatty acyl-CoA, which is obtained via the activation and conversion of fatty acids by acyl-CoA synthase (ACS). The first step in this process is the formation of lysophosphatidic acid (LPA) through the conversion of glycerol-3-phosphate and fatty acyl CoA, catalyzed by glycerol-3-phosphate acyltransferase (GPAT). The second step follows with the formation of phosphatidic acid, catalyzed by lysophosphatidic acid acyltransferase (LPAAT). Additionally, in certain enzyme reactions, different head groups are attached, generating different glycerophospholipids (127). During catabolism, GPLs are transformed into lysophospholipids (LPLs) and free fatty acids by the action of PLA2 (128).
2.3.2 Altered glycerophospholipids profile in head and neck cancer
In a study conducted by Silen et al., which involved ten cases of OSCC patients, GPL metabolism was found to be mostly dysregulated, with phosphatidylcholine (PC), phosphatidylethanolamine (PE), and phosphatidylinositols (PI) being the most prominent lipid classes (16). However, in another study by Zhang et al., which included fifty cases of OSCC patients and fifty cases of corresponding healthy controls, all glycerophospholipids were found to be decreased, particularly PC and phosphoethanolamine plasmalogens (22). In addition, LysoPC (14:0) exhibited a stepwise decrease with the development and progression of OSCC (22). Considering the discrepancies between the two studies, further studies should be performed to involve larger sample sizes and proper controls. In the study of Jelonek et al., there was first a significant decrease followed by an increase in the levels of several PCs (PC34, PC36, and PC38 variants) and lysophosphatidylcholine (LPC16 and LPC18 variants) in HNC samples after radiotherapy (RT), in a radiation dose positively pattern (129). The precise changes within the glycerophospholipid profile require additional elucidation, and understanding the specific alteration pattern of these molecules could potentially serve as an indicator for radiotherapy effectiveness.
2.3.3 Dysregulated glycerophospholipids biosynthesis and catabolism in head and neck cancer
The PLA2 superfamily, a key group of enzymes involved in glycerophospholipid catabolism, consists of over 30 different types of enzymes. These enzymes are categorized into six subfamilies, including cytosolic PLA2s (cPLA2s), calcium-independent PLA2s (iPLA2s), secreted PLA2s (sPLA2s), lysosomal PLA2s, platelet-activating factor (PAF) acetylhydrolases, and adipose specific PLA2s (130, 131). In general, PLA2s are activated and upregulated in several human cancers (132–135). In a study involving five HNSCC patients, the metabolic profile was investigated using 1H nuclear magnetic resonance (NMR) spectroscopy. The result revealed a significant elevation in the activity of PLA2, especially cPLA2, suggesting that PLA2 may be a potential anti-cancer target of HNSCC (124). Additionally, in plasma level, Menschikowski et al. reported an increase in secretory phospholipase A2 IIA (sPLA2-IIA), which was significantly associated with shorter survival among HNC patients (136). Furthermore, Askari et al. observed constant activation of sPLA2-IIA in OSCC tissues, and its negative correlation with the level of linoleic acid suggested that sPLA2-IIA could serve as a possible indicator of lipid metabolism alteration in OSCC (137). Despite these findings, limited research exists on the regulation of glycerophospholipid synthesis-related enzymes in HNSCC.
2.4 Alteration of sphingolipids metabolism in head and neck cancer
Bioactive sphingolipids encompass important lipid molecules, including sphingosine, ceramide, sphingosine-1-phosphate (S1P), and ceramide-1-phosphate. These sphingolipids play a pivotal role in a multiple of biological processes such as cell mobility, proliferation, and survival (138, 139).
2.4.1 De novo biosynthesis and catabolism of sphingolipids
Ceramide is a central molecule in sphingolipid metabolism and could be formed by the condensation of serine and palmitoyl coenzyme A. The rate-limiting step in this process is catalyzed by the enzyme serine palmitoyltransferase (SPT) (140). Alternatively, ceramide can be generated by hydrolysis of complex sphingolipids via sphingomyelinase (SMases). Moreover, ceramide can be converted into sphingosine-1-phosphate (S1P) through the action of ceramidases (CDases) and sphingosine kinase 1 and 2 (SphK1and SphK2) (139, 140). Sphingomyelin synthase (SMS) takes phosphatidylcholine (PC) as a donor, inserting the choline group into ceramide as a head group, and converting ceramide to SM. Additionally, ceramide can be transformed into ceramide-1-phosphate (C1P) by the enzyme ceramide kinase (CERK). Furthermore, the metabolism of ceramides also gives rise to complex sphingolipids. As for the catabolism, ceramide is hydrolyzed by CDases, releasing free fatty acids and sphingosine (139, 140).
2.4.2 Altered sphingolipid profile in head and neck cancer
There is limited data available on changes in sphingolipid profiles in HNC. As reported by Ji et al., the concentration levels of sphingolipid 42:2 and 42:3 (SM 42:2 and SM 42:3) were significantly downregulated in laryngeal carcinoma patients when compared to those with laryngeal benign tumors and healthy controls (23).
Ceramide is one of the hub nodes in sphingolipid signaling. As reported by Ogretmen et al., the level of total ceramide was decreased in non-squamous head and neck cancers but increased in HNSCC tumor tissues. Interestingly, the level of C18-ceramide was unexpectedly lower in HNSCC (approximately 50% lower) (24). Increased of C18-ceramide by mammalian upstream regulator of growth and differentiation factor 1 (mUOG1) and mouse homologue of longevity assurance gene 1 (mLAG1), could inhibit the proliferation of HNSCC cells, possibly due to its modulation of telomerase activity and mitochondrial function (24). In accordance with the former study, Senkal et al. observed a low level of C18-ceramide and an upregulated level of C16-ceramide in HNC. Interestingly, C18-ceramide was proposed to have a pro-apoptotic function, whereas C16-ceramide exhibited a protective effect against ER stress and apoptosis (25). Similar decrease in ceramide was observed in laryngeal carcinoma and OSCC when compared with premalignant and normal controls (141–143). Clinically, HNSCC patients with reduced C18-ceramide in tumor tissues tended to have a higher incidence of lymphovascular invasion and lymph node metastasis, which consequently correlated with a higher overall stage of the primary tumor (144). Oppositely, Wang et al. reported that ceramides (d18:1/16:0 and d18:1/18:0) were significantly increased in OSCC patients and positively correlated with pathological stage (22).
Considering the critical functions of ceramide in cancer, more and more research is focusing on its role in cancer therapy. An in vitro study showed that ceramide enhanced paclitaxel-mediated apoptosis. A combination of paclitaxel and ceramide could rewire the cell cycle of HNSCC cells, eliminating cells from S and/or G2-M phases, indicating that this combination may offer an attractive alternative to conventional chemotherapy of HNSCC (145). L-reo-C6-Pyridineium-ceramide-bromide (L-t-C6-Pyr-Cer), a cationic water-soluble ceramide analogue, inhibited the growth of HNSCC cell lines with a low IC50. When combined with gemcitabine (GEM), it significantly prevented tumor growth of HNSCC in vivo. Moreover, the treatment effect of L-t-C6-Pyr-Cer/GMZ was 2.5 times more effective than that of 5-fluorouracil/cisplatin combination (146). Doxorubicin (DOX), an inducer of ceramide production, increased the level of C18-ceramide, inhibited cell growth, and induced cell death in HNSCC patients when combined with GEM (147). In addition, a phase II clinical study demonstrated that GEM/DOX treatment facilitated the chemotherapeutic efficacy in HNSCC patients who failed the first-line platinum therapy (148).
2.4.3 Dysregulated sphingolipids biosynthesis and catabolism in head and neck cancer
2.4.3.1 Sphingosine kinase 1
SphK1, a key enzyme participating in sphingosine-1-phosphate (S1P) synthesis, has been found to be consistently overexpressed in both HNSCC cell lines and primary tumors (149–151). Its expression was higher in HNSCC cells displaying a more invasive phenotype (152). The upregulation of SphK1 has been correlated with advanced tumor stages, lymph node involvement, recurrence of tumor, and poor survival in HNSCC (149, 150). Mechanistically, SphK1 promotes the invasive ability of human tongue squamous cell carcinoma by upregulating EGFR and STAT3 (152). What’s more, inhibition of SphK1 suppressed cell proliferation and enhanced the radiosensitivity of HNSCC cells (151, 153). In summary, SphK1 might function as an oncogene in HNSCC.
2.4.3.2 Acid ceramidase
Acid ceramidase is responsible for the degradation of ceramide within lysosomes (154). Overexpression of AC has been reported in a variety of human cancers, including HNSCC (155, 156). Interestingly, it is higher in HNSCC cell lines generated from metastasis tumor compared to those from primary tumor (157). As reported by Norris et al., overexpression of AC increased the resistance of HNSCC cells to Fas-induced apoptosis (155). Jang et al. found a negative correlation between AC expression and cisplatin sensitivity in head and neck cancer cells. Treatment with AC inhibitor N-oleoyl-ethanolamine (NOE) or genetic silencing of AC might be novel approaches to enhance cisplatin cytotoxicity (156). Moreover, Movila et al. reported that phosphoethanolamine dihydroceramide (PEDHC) derived from P. gingivalis downregulated the expression of AC, promoted the accumulation of ceramidase and inhibited the proliferation and migration of OSCC cell lines (158). Collectively, overexpression of AC facilitates the malignant behaviors of head and neck cancer cells, and it might be a potential target of head and neck cancer treatment.
2.5 Alteration of sterol lipids metabolism in head and neck cancer
Sterol lipids consist of various compounds, including sterols, steroids, secosteroids, bile acids, and others. Among sterols, there are cholesterol, ergosterol, stigmasterol, C24 propylsterol, etc. As the most prominent sterol lipid, cholesterol is widely distributed in mammalian cells. Functionally, sterol lipids are essential for cell membrane formation and participate in a diverse range of physiological and biological processes (159).
2.5.1 De novo biosynthesis and catabolism of sterol lipids
Taking cholesterol as an example, it is mainly synthesized via the mevalonate pathway, starting with acetyl-CoA and processing with the involvement of more than 20 enzymes. The major rate-limiting enzyme, 3-hydroxy-3-methylgrutaryl (HMG)–CoA reductase (HMGCR), transforms 3-hydroxy-3-methylgrutaryl (HMG-CoA) into mevalonate. Mevalonate is converted into farnesyl pyrophosphate (FPP), and two molecules of FPP are condensed to yield squalene. Squalene is then oxidized by squalene epoxidase (SQLE) to generate 2,3-epoxysqualene, lanolin alcohol, and cholesterol subsequently (91). Maintaining cellular cholesterol homeostasis is crucial for normal physiological processes. Excessive intracellular cholesterol is exported from cell by ATP-binding cassette (ABC) transporter to reduce intracellular cholesterol levels (160). As for its circulation, the cholesterol synthesized and obtained exogenously which is stored in the liver, is released into the bloodstream in the form of very-low-density lipoproteins (VLDLs). These VLDLs are converted into low-density lipoproteins (LDLs) and taken up by peripheral cells (161). Excessive circulating cholesterol would be transported to lipid-free or lipid-poor apolipoprotein A-I (apoA-I), leading to the production of high-density lipoproteins (HDLs) (162). Moreover, surplus cholesterol could be esterified by acyl coenzyme A-cholesterol acyltransferase (ACAT) to generate cholesteryl esters, which are stored in lipid droplets or circulated as plasma lipoproteins (163).
2.5.2 Dysregulated sterol lipids profile in head and neck cancer
Studies have shown that the serum level of total cholesterol (TC) in OSCC patients is significantly lower than those in healthy controls (21, 26, 27). In eleven HNC patients, Pereira et al. found a significant positive correlation between baseline LDL-cholesterol levels and changes in radiotherapy-induced carotid intima-media thickness, suggesting that LDL-cholesterol might serve as a predictor for RT-induced carotid atherosclerosis in HNC (28). A retrospective cohort study based on 4,575,818 individuals in Korea revealed that high TC and high LDL-cholesterol levels are protective factors and could reduce the risk of HNC (164). Additionally, a prospective analysis based on 474,929 participants from the UK biobank demonstrated a significant U-shaped association between HDL-C and HNC risk in males (29). Based on the 561,388 individuals of the Swedish AMORIS cohort, we found a positive association between blood levels of TC, apoA-I and the risk of HNC. Furthermore, HNSCC patients showed constantly higher levels of TC and apoA-I during the 30 years before diagnosis (30).
2.5.3 Dysregulated sterol lipids biosynthesis related enzymes in head and neck cancer
2.5.3.1 Sterol regulatory element-binding protein 2
As mentioned previously, SREBP2 plays a critical role in selectively modulating the transcription of genes encoding cholesterologenic enzymes (165). However, there’s limited research on SREBP2 in HNC. According to a study by Yang et al., SREBP2 was significantly downregulated in OSCC tissues and cell lines when compared with normal controls. Restoration of SREBP2 could inhibit cell proliferation, migration, invasion, and induce cell apoptosis in OSCC, suggesting its novel role as a tumor suppressor (166). Nevertheless, further research is needed to fully clarify the role of SREBP2 in HNC.
2.5.3.2 3-hydroxy-3-methylglutaryl–CoA reductase
HMGCR is the rate-limiting enzyme in the mevalonate pathway for cholesterol production. Several studies have found that the downregulation of HMGCR is associated with the progression of various tumors (167–169). Interestingly, the expression of HMGCR was found to be elevated in OSCC (170). Furthermore, additional analysis revealed an increased expression of HMGCR in radiation-resistant HNSCC cells (171).
Statin, an HMGCR inhibitor, is noted not only to decrease the level of cholesterol but also reduce the risk of HNSCC. Moreover, statin use has been linked to improved survival in HNSCC patients, particularly in those with HPV-positive tumors (172). In another study, it was proposed that statin use would facilitate the prognosis of HNC, leading to increased overall survival and cancer-specific survival at 2 years (173). What’s more, the application of atorvastatin reduced the rate of cisplatin-induced hearing loss by 19.7% without compromising the effectiveness of cisplatin treatment in HNC patients (174). However, a Mendelian randomization study proposed that the strategy of cholesterol-lowering in oral cancer and oropharyngeal cancer was confounded, warranting further investigation (175).
2.5.3.3 Squalene monooxygenase
SM, which is encoded by the SQLE gene, is the second rate-limiting enzyme in the process of cholesterol synthesis (3). In HNSCC, an elevated level of SQLE expression and gene amplification has been observed, promoting cell proliferation and correlating with the TNM stage of patients (176, 177). Moreover, a high level of SQLE mRNA expression was negatively associated with the survival of HNSCC patients (178). In addition, SQLE plays a role in the tumor microenvironment in HNSCC. It showed a negative correlation with the infiltration of CD8+ T cells, follicular helper T cells, regulatory T cells, and mast cells, while exhibiting a positive correlation with M0 macrophages and resting mast cells (177). Terbinafine, an inhibitor of SQLE clinically used as an antifungal reagent, has recently gained attention for its anti-cancer effects and is being extensively studied (178). In OSCC, terbinafine has been reported to inhibit the proliferation of cancer cells, possibly by suppressing Raf-MEK-ERK and stimulating the p21(cip1) - and p27(kip1) -associated signaling pathways (179, 180). However, further research is needed to fully understand its potential in treating HNSCC. Taken together, SQLE may serve as a novel biomarker for prognosis and a promising drug target for HNSCC.
2.5.3.4 Enhancer of zeste homolog 2
EZH2, a histone methyltransferase, plays a role in modulating endogenous cholesterol synthesis. It is highly expressed in HNSCC, and its upregulation has been found to be correlated with tumor aggressiveness and poor outcomes in HNSCC patients (181, 182). One of the possible mechanisms behind this correlation might lie in the hypermethylation of tumor suppressor genes induced by EZH2 (182). In addition, EZH2-mediated trimethylation modification of histone H3 lysine 27 directly regulates sterol regulatory element binding transcription factor 2 (SREBF2) and its target gene SQLE, which in turn influences the synthesis of endogenous cholesterol in HNSCC. Inhibition of EZH2 strongly activates genes related to cholesterol synthesis and rewrites cholesterol metabolism. As a result, DZNep, an EZH2 inhibitor, showed an impeded function in the proliferation and survival of the human hypopharynx carcinoma cell line FaDu (182). Furthermore, the effects of EZH2 inhibitors have been found to be enhanced following the inhibition of SQLE (178). Nonetheless, further study is needed to fully elucidate the mechanisms.
2.5.4 Dysregulated sterol lipids trafficking in head and neck cancer
2.5.4.1 Low-density lipoproteins receptor
The LDLR is responsible for trafficking of lipoprotein into cells. Increased level of LDLR has been detected in a variety of tumors, including pancreatic cancer (183), glioblastoma cancer (100), and breast cancer (184). However, the level of LDLR in HNSCC remains unreported. Meanwhile, a Mendelian randomization study suggested that LDLR variants decreased the risk of combined oral and oropharyngeal cancer via heritable reduction of LDL-C (175).
2.6 Alteration of prenol lipids, polyketides, saccharolipids metabolism in head and neck cancer
There have been no reports on the dysregulation of prenol lipids, polyketides, or saccharolipids in HNSCC to date, making them promising areas for further exploration.
3 Relationship between lipid metabolism and risk factors of head and neck cancer
3.1 Tobacco and alcohol consumption
Tobacco consumption is a well-established etiological factor in HNC (185, 186). Studies have consistently shown that HNSCC patients with a history of tobacco consumption have a significantly lower level of TC when compared with healthy individuals, especially those without a tobacco consumption history (18, 27, 187, 188). However, there’s no difference in serum levels of TC, LDL, VLDL, HDL, and triglyceride between oral cancer patients with and without tobacco consumption habit (189, 190). Interestingly, the level of serum HDL in oral cancer and oral precancer patients with habit of tobacco was decreased when compared to healthy controls with habit of tobacco use (189). Thus, tobacco carcinogens may increase the generation of free radicals and reactive oxygen species, leading to elevated oxidation/peroxidation rates of polyunsaturated fatty acids, which in turn affect the basic components of cell membranes and potentially be involved in the process of carcinogenesis (191).
Alcohol consumption is another established risk factor of HNC (192, 193). Prediagnosis alcohol intake has been associated with significantly poor overall survival in HNSCC patients in a dose-dependent pattern (194), which might be modified by the genetic polymorphisms of ADH1B and ALDH2 (195). Chronic excessive alcohol consumption is proposed to impair the effect of PPARα, which is involved in β-oxidation, disrupts the biosynthesis of cholesterol, and induces the accumulation of triglycerides (196).
3.2 Infection of human papillomaviruses
HPV infection has emerged as a hot spot in HNC research. HNSCC patients with different HPV infection status have different clinical outcomes (197, 198). Two virus oncoproteins, E6 and E7, contribute to the tumorigenic potential of HPV by inhibiting and degrading the tumor suppressor p53 and retinoblastoma-associated protein (pRB), respectively (199). What’s more, E6 stimulates hypoxia-inducible factor 1-α (HIF1α), leading to the activation of SREBP1, which, in turn, increases lipid synthesis by stimulating the expression of FASN and ACC. Additionally, HIF1 could promote the lipid uptake via upregulating receptor proteins from the CD36 family and FABPs (200). Moreover, E6 activates PI3K/Akt/mTOR pathways, resulting in the upregulation of downstream SREBP1 to mediate adipogenesis (201). The inactivation of Rb by E7 also activates the PI3K/Akt/mTOR pathway to promote adipogenesis (202). In HPV-positive HNSCC, a series of genes, such as PIK3CA, DDR2 or NF-kB, are found significantly mutated, contributing to the stimulation of glutamine and lipid metabolism (203–205). Meanwhile, HPV-negative HNSCC often exhibits inactivation of tumor suppressors, for example, p53, leading to the promotion of glycolysis (203–205).
3.3 High-fat diet and obesity
Unhealthy diet pattern disturbs the lipid metabolism and plays an important role in HNSCC. The consumption of fried meals, high-fat and processed meats, and sweets has been linked to an increased risk of laryngeal cancer (206–208). Specifically, the consumption of “unsaturated fats” and “animal unsaturated fatty acids” has been identified as risk factors for laryngeal carcinoma but protective factors for oral and pharyngeal cancer, as supported by references (209, 210). Intriguingly, different fat subtypes have been associated with the prognosis of HNSCC patients, impacting outcome factors such as recurrence and mortality. It was shown that high long-chain fatty acid (LCFA), unsaturated fatty acid, ω-3 PUFAs, and ω-6 PUFAs diet was significantly associated with a reduced risk of all-cause mortality, respectively (211). Additionally, a high intake of unsaturated fatty acid has been shown to reduce the specific mortality risk associated with HNSCC (211). Interestingly, it has been observed that a diet pattern with a low ω-6/ω-3 fatty acid ratio (ω-6/ω-3 = 2) suppressed carcinogenesis in the DMBA/BQE-induced hamster oral cancer model via inhibiting the expression of NF-κB p65, PCNA, and cyclin D1 (212).
Obesity, as one of the consequences of high-fat diet, is associated with an elevated incidence and impacts the overall survival of many human cancer types (213–216). In the complicated crosstalk between adipose tissue and cancer cells, mature adipocytes provide adipokines and lipids to cancer cells, while stromal and immune cells within adipose tissue release paracrine factors into the tumor microenvironment. Concurrently, the proliferation of cancer cells drives lipolysis in adipocytes (217). The possible mechanisms underpinning in the obesity-cancer link are proposed as: chronic inflammation in adipose tissue, oxidative stress, interplay between cancer cells and neighboring adipocytes, obesity-induced hypoxia, genetic susceptibility, immune response, and more (215).
However, the impact of obesity on head and neck cancer remains a subject of debate. There are some studies reported no significant association between body mass index (BMI) and the incidence of head and neck cancer (218, 219). In contrast, Dannenberg et al. reported that obesity was an independent risk factor for T1/2N0M0 OSCC patients, leading to poorer progression survival and disease-specific survival (220). In an in silico study, a combination of lipid metabolism-related genes, including TGFB1, SPP1, and SERPINE1, was proposed to be potential prognosis markers of OSCC patients (220, 221). Conversely, there are other studies showing that obesity was more likely to be a protective factor against the development of head and neck cancer (222–224). In a retrospective study, Gupta et al. brought out that being obese at the time of diagnosis of HNSCC (including oropharynx cancer, laryngeal carcinoma, and oral cancer patients; 72% of the patients were identified as stage IVA/B, and 28% identified as stage I-III), was an independent prognostic factor conferring better survival, suggesting extended time to recurrence, and better improvement of distant control (223). In a multinational case-control study conducted across nine countries, a low BMI was found to be associated with an increased risk of oral cancer. This conclusion remained consistent even after stratified analyses were performed (224). The underlying factors contributing to this contradiction might lie in the confounding variables such as age, alcohol and cigarette consumption, cancer stage, and treatment characteristics. A systemic review has summarized that obesity mechanically influences the levels and activities of lipid metabolism-related molecules (e.g., FFA, FAS, sPLA2, FABP4, and FABP5), thereby contributing to the carcinogenesis and progression of head and neck cancer (225). Nonetheless, the precise mechanism through which obesity may reduce the risk of head and neck cancer remains elusive. In conclusion, further research is required to elucidate the relationship between obesity and head and neck cancer. And of importance, promoting a healthy diet and lifestyle should be emphasized in public health education for cancer prevention.
4 Conclusions
Lipid metabolism reprogram plays a critical role in the carcinogenesis and progression of head and neck cancer. In the present review, we have summarized the current understanding and advantages regarding the abnormal lipid metabolism profile, novel biomarkers, and possible mechanisms in head and neck cancer, according to the LIPID MAPS Lipid Classification System and cancer risk factors (Figure 2). We also discuss and emphasize their potential applications as biomarkers in the diagnosis, treatment and prognosis of head and neck cancer (Table 2).
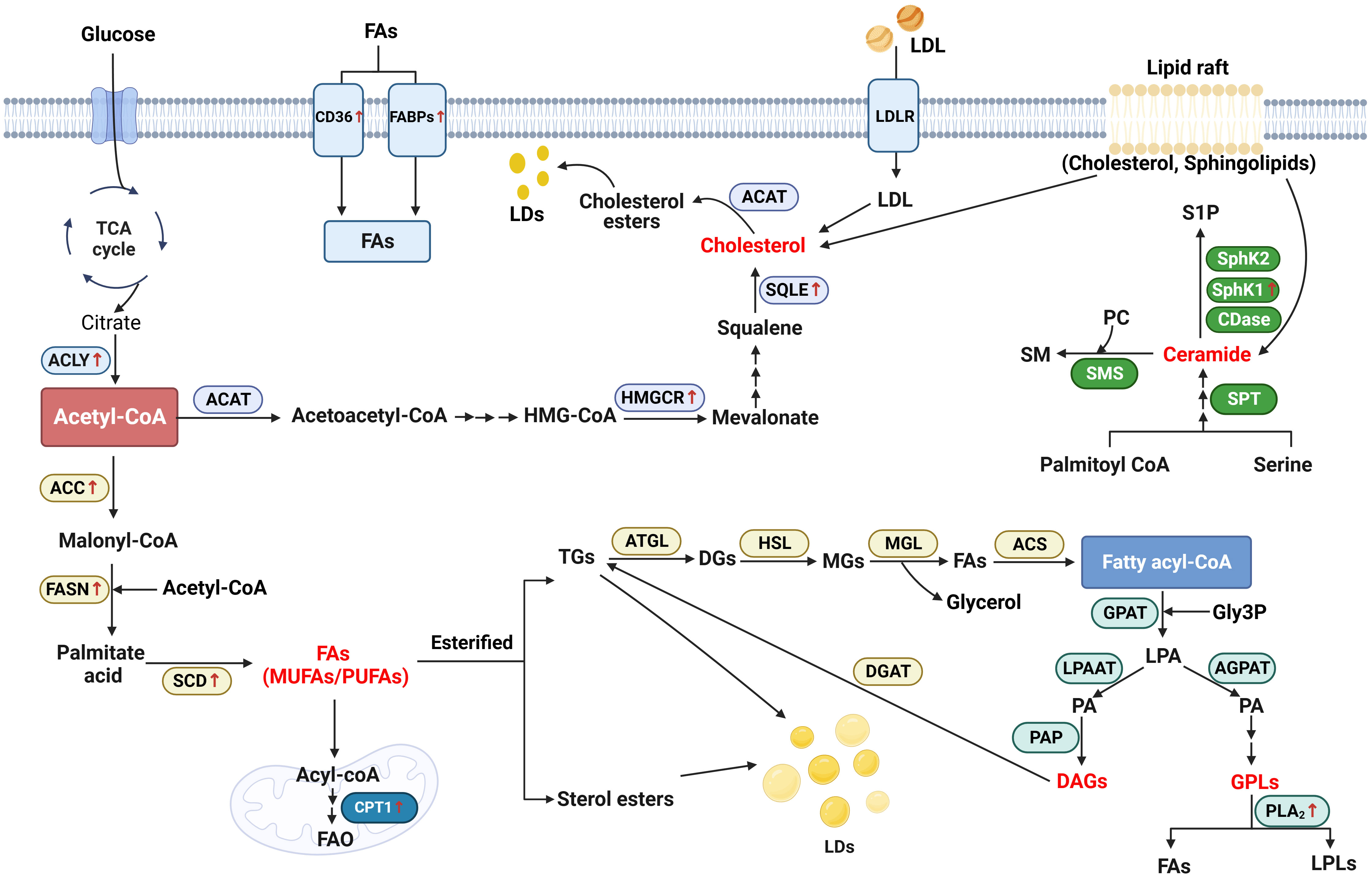
Figure 2 Schematic representation of lipid metabolism reprogramming implicated in head and neck cancer. Generally, in the biosynthesis of fatty acids (FAs), acetyl-CoA generated from citrate is converted to malonyl-CoA by the upregulated acetyl-CoA carboxylase (ACC). Malonyl-CoA combines with acetyl-CoA to generate palmitate acid via activated FASN. The palmitate acid is elongated to form monounsaturated fatty acids (MUFAs) or polyunsaturated fatty acids (PUFAs) by the activated stearoyl-CoA desaturase (SCD). In the catabolism, FAs are transported into the mitochondrial matrix for oxidation by the activated carnitine palmitoyltransferase 1 (CPT1). Additionally, cluster of differentiation 36 (CD36) and fatty acid-binding proteins (FABPs) which are responsible for the trafficking of FAs are notably upregulated in head and neck cancer (HNC). As an important substrate, acetyl-CoA is also converted to acetoacetyl-CoA and HMG-CoA after a serious of reactions. 3-hydroxy-3-methylgrutaryl (HMG-CoA) is transformed to mevalonate by activated 3-hydroxy-3-methylgrutaryl (HMG)–CoA reductase (HMGCR). Squalene is converted by the upregulated squalene epoxidase (SQLE) to form cholesterol, which is another important lipid category. As for the biosynthesis of glycerolipid, fatty acyl-coenzyme A (FA-CoA) condenses with glycerol-3-phosphate (Gly3P) to generate lysophosphatidic acid (LPA) via glycerol-3-phosphate acyltransferase (GPAT). With the function of 1-acyl-sn-Gly3P acyltransferase (AGPAT) and lysophosphatidic acid acyltransferase (LPAAT), LPA is then transformed into phosphatidic acid (PA). Using phosphatidic as the source, glycerophospholipids (GPLs) is generated from after attached with different head groups and it is degraded by phospholipase A2 (PLA2) to generate FAs and lysophospholipids (LPLs). On the other hand, diacylglycerol (DAG) is generated by the function of phosphatidic acid phosphatase (PAP). DAG, in turn, generates triacylglycerol (TG) by activating diglyceride acyltransferase (DGAT). During the catabolism of neutral TG, adipose triglyceride lipase (ATGL) first converts TGs into diacylglycerol (DGs), and then hormone-sensitive lipase (HSL) hydrolyzes DGs to form monoacylglycerols (MGs). Finally, MAG lipase (MGL) hydrolyzes MGs and generates FAs and glycerol. Ceramide is the main type of sphingolipid. It is generated by the condensation of serine and palmitoyl-CoA via the function of serine palmitoyltransferase (SPT). Ceramide is degraded into sphingosine-1-phosphate (S1P), with the function of Ceramidases (CDases), sphingosine kinase 1 (SphK1) and Sphingosine kinase 2 (SphK2).
Although significant advances have been made, as underscored in our review, there are still many unresolved scientific gaps demanding to be addressed. For instance, the roles of HSL, MCL, prenol lipids, polyketides, and saccharolipids in head and neck cancer need to be clarified. A more in-depth exploration of the related mechanisms is essential to shed light on the signaling crosstalk responsible for the alteration of lipid metabolism. The controversial roles of phosphatidylcholine, acetyl-CoA carboxylase, and especially obesity, remain to be elucidated. What’s more, there’s still an urgent need for the identification of novel therapeutic targets related to lipid metabolism.
In conclusion, a more profound understanding of lipid metabolism alterations and the intricacies of associated mechanisms will improve the accuracy of diagnosis, enable the customization of personalized treatments, and fine-tune prognosis strategies for individuals facing head and neck cancer.
Author contributions
JL: Conceptualization, Writing – original draft. LL: Writing – original draft. LML: Writing – original draft. XZ: Writing – review & editing. ZZ: Writing – review & editing. YH: Writing – review & editing. XX: Conceptualization, Writing – review & editing.
Funding
The author(s) declare financial support was received for the research, authorship, and/or publication of this article. This work was supported by grants from the National Natural Scientific Foundation of China (82060511), “Medical Excellence Award” funded by the Creative Research Development Grant from the First Affiliated Hospital of Guangxi Medical University, and Key Laboratory of Early Prevention and Treatment for Regional High Frequency Tumor (Guangxi Medial University), Ministry of Education (GKE-ZZ-202228/GKE-ZZ-202229).
Conflict of interest
The authors declare that the research was conducted in the absence of any commercial or financial relationships that could be construed as a potential conflict of interest.
Publisher’s note
All claims expressed in this article are solely those of the authors and do not necessarily represent those of their affiliated organizations, or those of the publisher, the editors and the reviewers. Any product that may be evaluated in this article, or claim that may be made by its manufacturer, is not guaranteed or endorsed by the publisher.
References
1. Beloribi-Djefaflia S, Vasseur S, Guillaumond F. Lipid metabolic reprogramming in cancer cells. Oncogenesis (2016) 5(1):e189. doi: 10.1038/oncsis.2015.49
2. van Meer G, Voelker DR, Feigenson GW. Membrane lipids: where they are and how they behave. Nat Rev Mol Cell Biol (2008) 9(2):112–24. doi: 10.1038/nrm2330
3. Bian X, Liu R, Meng Y, Xing D, Xu D, Lu Z. Lipid metabolism and cancer. J Exp Med (2021) 218(1):e20201606. doi: 10.1084/jem.20201606
4. Santos CR, Schulze A. Lipid metabolism in cancer. FEBS J (2012) 279(15):2610–23. doi: 10.1111/j.1742-4658.2012.08644.x
5. Johnson DE, Burtness B, Leemans CR, Lui VWY, Bauman JE, Grandis JR. Head and neck squamous cell carcinoma. Nat Rev Dis Primers (2020) 6(1):92. doi: 10.1038/s41572-020-00224-3
7. Wise-Draper TM, Draper DJ, Gutkind JS, Molinolo AA, Wikenheiser-Brokamp KA, Wells SI. Future directions and treatment strategies for head and neck squamous cell carcinomas. Trans Res J Lab Clin Med (2012) 160(3):167–77. doi: 10.1016/j.trsl.2012.02.002
8. Muzaffar J, Bari S, Kirtane K, Chung CH. Recent advances and future directions in clinical management of head and neck squamous cell carcinoma. Cancers (2021) 13(2):338. doi: 10.3390/cancers13020338
9. van Harten AM, Brakenhoff RH. Targeted treatment of head and neck (Pre)Cancer: preclinical target identification and development of novel therapeutic applications. Cancers (2021) 13(11):2774. doi: 10.3390/cancers13112774
10. Halczy-Kowalik L, Drozd A, Stachowska E, Drozd R, Żabski T, Domagała W. Fatty acids distribution and content in oral squamous cell carcinoma tissue and its adjacent microenvironment. PloS One (2019) 14(6):e0218246. doi: 10.1371/journal.pone.0218246
11. Sakurai K, Tomihara K, Yamazaki M, Heshiki W, Moniruzzaman R, Sekido K, et al. Cd36 expression on oral squamous cell carcinoma cells correlates with enhanced proliferation and migratory activity. Oral Dis (2020) 26(4):745–55. doi: 10.1111/odi.13210
12. Uma RS, Naresh KN, D'Cruz AK, Mulherkar R, Borges AM. Metastasis of squamous cell carcinoma of the oral tongue is associated with down-regulation of epidermal fatty acid binding protein (E-fabp). Oral Oncol (2007) 43(1):27–32. doi: 10.1016/j.oraloncology.2005.12.024
13. Su YW, Lin YH, Pai MH, Lo AC, Lee YC, Fang IC, et al. Association between phosphorylated amp-activated protein kinase and acetyl-coa carboxylase expression and outcome in patients with squamous cell carcinoma of the head and neck. PloS One (2014) 9(4):e96183. doi: 10.1371/journal.pone.0096183
14. Krontiras H, Roye GD, Beenken SE, Myers RB, Mayo MS, Peters GE, et al. Fatty acid synthase expression is increased in neoplastic lesions of the oral tongue. Head Neck (1999) 21(4):325–9. doi: 10.1002/(sici)1097-0347(199907)21:4<325::aid-hed6>3.0.co;2-p
15. Fahy E, Subramaniam S, Murphy RC, Nishijima M, Raetz CR, Shimizu T, et al. Update of the lipid maps comprehensive classification system for lipids. J Lipid Res (2009) 50 Suppl(Suppl):S9–14. doi: 10.1194/jlr.R800095-JLR200
16. Dickinson A, Saraswat M, Joenväärä S, Agarwal R, Jyllikoski D, Wilkman T, et al. Mass spectrometry-based lipidomics of oral squamous cell carcinoma tissue reveals aberrant cholesterol and glycerophospholipid metabolism - a pilot study. Trans Oncol (2020) 13(10):100807. doi: 10.1016/j.tranon.2020.100807
17. Christou CN, Tiblom Ehrsson Y, Lampa E, Risérus U, Laurell G. Circulating fatty acids in patients with head and neck cancer after treatment: an explorative study with a one-year perspective. Acta oto-laryngologica (2021) 141(9):878–84. doi: 10.1080/00016489.2021.1959950
18. Poorey VK, Thakur P. Alteration of lipid profile in patients with head and neck Malignancy. Indian J Otolaryngol Head Neck Surg Off Publ Assoc Otolaryngologists India (2016) 68(2):135–40. doi: 10.1007/s12070-015-0829-4
19. Patel PS, Shah MH, Jha FP, Raval GN, Rawal RM, Patel MM, et al. Alterations in plasma lipid profile patterns in head and neck cancer and oral precancerous conditions. Indian J Cancer (2004) 41(1):25–31. doi: 10.4103/0019-509X.12341
20. Somashekar BS, Kamarajan P, Danciu T, Kapila YL, Chinnaiyan AM, Rajendiran TM, et al. Magic angle spinning nmr-based metabolic profiling of head and neck squamous cell carcinoma tissues. J Proteome Res (2011) 10(11):5232–41. doi: 10.1021/pr200800w
21. Garg D, Sunil MK, Singh PP, Singla N, Rani SR, Kaur B. Serum lipid profile in oral precancer and cancer: A diagnostic or prognostic marker? J Int Oral Health JIOH (2014) 6(2):33–9.
22. Wang L, Wang X, Li Y, Hou Y, Sun F, Zhou S, et al. Plasma lipid profiling and diagnostic biomarkers for oral squamous cell carcinoma. Oncotarget (2017) 8(54):92324–32. doi: 10.18632/oncotarget.21289
23. Wang H, Luo Y, Chen H, Hou H, Hu Q, Ji M. Non-targeted serum lipidomics analysis and potential biomarkers of laryngeal cancer based on uhplc-qtof-ms. Metabolites (2022) 12(11):1087. doi: 10.3390/metabo12111087
24. Koybasi S, Senkal CE, Sundararaj K, Spassieva S, Bielawski J, Osta W, et al. Defects in cell growth regulation by C18:0-ceramide and longevity assurance gene 1 in human head and neck squamous cell carcinomas. J Biol Chem (2004) 279(43):44311–9. doi: 10.1074/jbc.M406920200
25. Senkal CE, Ponnusamy S, Bielawski J, Hannun YA, Ogretmen B. Antiapoptotic roles of ceramide-synthase-6-generated C16-ceramide via selective regulation of the atf6/chop arm of er-stress-response pathways. FASEB J Off Publ Fed Am Societies Exp Biol (2010) 24(1):296–308. doi: 10.1096/fj.09-135087
26. Sherubin EJ, Kannan KS, Kumar DN, Joseph I. Estimation of plasma lipids and its significance on histopathological grades in oral cancer: prognostic significance an original research. J Oral Maxillofac Pathol JOMFP (2013) 17(1):4–9. doi: 10.4103/0973-029x.110685
27. Acharya S, Rai P, Hallikeri K, Anehosur V, Kale J. Serum lipid profile in oral squamous cell carcinoma: alterations and association with some clinicopathological parameters and tobacco use. Int J Oral Maxillofac Surg (2016) 45(6):713–20. doi: 10.1016/j.ijom.2016.01.015
28. Pereira EB, Gemignani T, Sposito AC, Matos-Souza JR, Nadruz W Jr. Low-density lipoprotein cholesterol and radiotherapy-induced carotid atherosclerosis in subjects with head and neck cancer. Radiat Oncol (London England) (2014) 9:134. doi: 10.1186/1748-717x-9-134
29. Jiang H, Zhou L, He Q, Jiang K, Yuan J, Huang X. The effect of metabolic syndrome on head and neck cancer incidence risk: A population-based prospective cohort study. Cancer Metab (2021) 9(1):25. doi: 10.1186/s40170-021-00261-w
30. Huang Y, Xiao X, Sadeghi F, Feychting M, Hammar N, Fang F, et al. Blood metabolic biomarkers and the risk of head and neck cancer: an epidemiological study in the swedish amoris cohort. Cancer Lett (2023) 557:216091. doi: 10.1016/j.canlet.2023.216091
31. Koundouros N, Poulogiannis G. Reprogramming of fatty acid metabolism in cancer. Br J Cancer (2020) 122(1):4–22. doi: 10.1038/s41416-019-0650-z
32. Currie E, Schulze A, Zechner R, Walther TC, Farese RV Jr. Cellular fatty acid metabolism and cancer. Cell Metab (2013) 18(2):153–61. doi: 10.1016/j.cmet.2013.05.017
33. Klapan I, Katić V, Culo F, Cuk V. Prognostic significance of plasma prostaglandin E concentration in patients with head and neck cancer. J Cancer Res Clin Oncol (1992) 118(4):308–13. doi: 10.1007/bf01208621
34. Ma Y, Temkin SM, Hawkridge AM, Guo C, Wang W, Wang XY, et al. Fatty acid oxidation: an emerging facet of metabolic transformation in cancer. Cancer Lett (2018) 435:92–100. doi: 10.1016/j.canlet.2018.08.006
35. Drury J, Rychahou PG, Kelson CO, Geisen ME, Wu Y, He D, et al. Upregulation of cd36, a fatty acid translocase, promotes colorectal cancer metastasis by increasing mmp28 and decreasing E-cadherin expression. Cancers (2022) 14(1):252. doi: 10.3390/cancers14010252
36. Wang J, Wen T, Li Z, Che X, Gong L, Jiao Z, et al. Cd36 upregulates dek transcription and promotes cell migration and invasion via gsk-3β/Β-catenin-mediated epithelial-to-mesenchymal transition in gastric cancer. Aging (2020) 13(2):1883–97. doi: 10.18632/aging.103985
37. Jiang M, Wu N, Xu B, Chu Y, Li X, Su S, et al. Fatty acid-induced cd36 expression via O-glcnacylation drives gastric cancer metastasis. Theranostics (2019) 9(18):5359–73. doi: 10.7150/thno.34024
38. Luo X, Zheng E, Wei L, Zeng H, Qin H, Zhang X, et al. The fatty acid receptor cd36 promotes hcc progression through activating src/pi3k/akt axis-dependent aerobic glycolysis. Cell Death Dis (2021) 12(4):328. doi: 10.1038/s41419-021-03596-w
39. Aloia A, Müllhaupt D, Chabbert CD, Eberhart T, Flückiger-Mangual S, Vukolic A, et al. A fatty acid oxidation-dependent metabolic shift regulates the adaptation of braf-mutated melanoma to mapk inhibitors. Clin Cancer Res (2019) 25(22):6852–67. doi: 10.1158/1078-0432.Ccr-19-0253
40. Wang J, Li Y. Cd36 tango in cancer: signaling pathways and functions. Theranostics (2019) 9(17):4893–908. doi: 10.7150/thno.36037
41. Yang P, Qin H, Li Y, Xiao A, Zheng E, Zeng H, et al. Cd36-mediated metabolic crosstalk between tumor cells and macrophages affects liver metastasis. Nat Commun (2022) 13(1):5782. doi: 10.1038/s41467-022-33349-y
42. Aoki T, Kinoshita J, Munesue S, Hamabe-Horiike T, Yamaguchi T, Nakamura Y, et al. Hypoxia-induced cd36 expression in gastric cancer cells promotes peritoneal metastasis via fatty acid uptake. Ann Surg Oncol (2023) 30(5):3125–36. doi: 10.1245/s10434-022-12465-5
43. Ligorio F, Di Cosimo S, Verderio P, Ciniselli CM, Pizzamiglio S, Castagnoli L, et al. Predictive role of cd36 expression in her2-positive breast cancer patients receiving neoadjuvant trastuzumab. J Natl Cancer Institute (2022) 114(12):1720–7. doi: 10.1093/jnci/djac126
44. Feng WW, Wilkins O, Bang S, Ung M, Li J, An J, et al. Cd36-mediated metabolic rewiring of breast cancer cells promotes resistance to her2-targeted therapies. Cell Rep (2019) 29(11):3405–20.e5. doi: 10.1016/j.celrep.2019.11.008
45. Pascual G, Avgustinova A, Mejetta S, Martín M, Castellanos A, Attolini CS, et al. Targeting metastasis-initiating cells through the fatty acid receptor cd36. Nature (2017) 541(7635):41–5. doi: 10.1038/nature20791
46. Haidari S, Tröltzsch M, Knösel T, Liokatis P, Kasintsova A, Eberl M, et al. Fatty acid receptor cd36 functions as a surrogate parameter for lymph node metastasis in oral squamous cell carcinoma. Cancers (2021) 13(16):4125. doi: 10.3390/cancers13164125
47. Scalia A, Kindt N, Trelcat A, Nachtergael A, Duez P, Journé F, et al. Development of a method for producing oxldl: characterization of their effects on hpv-positive head and neck cancer cells. Int J Mol Sci (2022) 23(20):12552. doi: 10.3390/ijms232012552
48. Pascual G, Domínguez D, Elosúa-Bayes M, Beckedorff F, Laudanna C, Bigas C, et al. Dietary palmitic acid promotes a prometastatic memory via schwann cells. Nature (2021) 599(7885):485–90. doi: 10.1038/s41586-021-04075-0
49. Tao L, Ding X, Yan L, Xu G, Zhang P, Ji A, et al. Cd36 accelerates the progression of hepatocellular carcinoma by promoting fas absorption. Med Oncol (Northwood London England) (2022) 39(12):202. doi: 10.1007/s12032-022-01808-7
50. Pan J, Fan Z, Wang Z, Dai Q, Xiang Z, Yuan F, et al. Cd36 mediates palmitate acid-induced metastasis of gastric cancer via akt/gsk-3β/Β-catenin pathway. J Exp Clin Cancer Res CR (2019) 38(1):52. doi: 10.1186/s13046-019-1049-7
51. O'Sullivan SE, Kaczocha M. Fabp5 as a novel molecular target in prostate cancer. Drug Discovery Today (2020) 25(11):2056–61. doi: 10.1016/j.drudis.2020.09.018
52. Liu RZ, Godbout R. An amplified fatty acid-binding protein gene cluster in prostate cancer: emerging roles in lipid metabolism and metastasis. Cancers (2020) 12(12):3823. doi: 10.3390/cancers12123823
53. Dum D, Ocokoljic A, Lennartz M, Hube-Magg C, Reiswich V, Höflmayer D, et al. Fabp1 expression in human tumors: A tissue microarray study on 17,071 tumors. Virchows Archiv an Int J Pathol (2022) 481(6):945–61. doi: 10.1007/s00428-022-03394-5
54. Prayugo FB, Kao TJ, Anuraga G, Ta HDK, Chuang JY, Lin LC, et al. Expression profiles and prognostic value of fabps in colorectal adenocarcinomas. Biomedicines (2021) 9(10):1460. doi: 10.3390/biomedicines9101460
55. Wu G, Xu Y, Wang Q, Li J, Li L, Han C, et al. Fabp5 is correlated with poor prognosis and promotes tumour cell growth and metastasis in clear cell renal cell carcinoma. Eur J Pharmacol (2019) 862:172637. doi: 10.1016/j.ejphar.2019.172637
56. Rauch J, Ahlemann M, Schaffrik M, Mack B, Ertongur S, Andratschke M, et al. Allogenic antibody-mediated identification of head and neck cancer antigens. Biochem Biophys Res Commun (2004) 323(1):156–62. doi: 10.1016/j.bbrc.2004.08.071
57. Münz M, Zeidler R, Gires O. The tumour-associated antigen epcam upregulates the fatty acid binding protein E-fabp. Cancer Lett (2005) 225(1):151–7. doi: 10.1016/j.canlet.2004.11.048
58. Fang LY, Wong TY, Chiang WF, Chen YL. Fatty-acid-binding protein 5 promotes cell proliferation and invasion in oral squamous cell carcinoma. J Oral Pathol Med Off Publ Int Assoc Oral Pathologists Am Acad Oral Pathol (2010) 39(4):342–8. doi: 10.1111/j.1600-0714.2009.00836.x
59. Ohyama Y, Kawamoto Y, Chiba T, Kikuchi K, Sakashita H, Imai K. Differential expression of fatty acid-binding proteins and pathological implications in the progression of tongue carcinoma. Mol Clin Oncol (2014) 2(1):19–25. doi: 10.3892/mco.2013.198
60. Kim KH. Regulation of mammalian acetyl-coenzyme a carboxylase. Annu Rev Nutr (1997) 17:77–99. doi: 10.1146/annurev.nutr.17.1.77
61. Zu X, Zhong J, Luo D, Tan J, Zhang Q, Wu Y, et al. Chemical genetics of acetyl-coa carboxylases. Molecules (Basel Switzerland) (2013) 18(2):1704–19. doi: 10.3390/molecules18021704
62. Brusselmans K, De Schrijver E, Verhoeven G, Swinnen JV. Rna interference-mediated silencing of the acetyl-coa-carboxylase-alpha gene induces growth inhibition and apoptosis of prostate cancer cells. Cancer Res (2005) 65(15):6719–25. doi: 10.1158/0008-5472.Can-05-0571
63. Chajès V, Cambot M, Moreau K, Lenoir GM, Joulin V. Acetyl-coa carboxylase alpha is essential to breast cancer cell survival. Cancer Res (2006) 66(10):5287–94. doi: 10.1158/0008-5472.Can-05-1489
64. Li K, Zhang C, Chen L, Wang P, Fang Y, Zhu J, et al. The role of acetyl-coa carboxylase2 in head and neck squamous cell carcinoma. PeerJ (2019) 7:e7037. doi: 10.7717/peerj.7037
65. Luo Z, Saha AK, Xiang X, Ruderman NB. Ampk, the metabolic syndrome and cancer. Trends Pharmacol Sci (2005) 26(2):69–76. doi: 10.1016/j.tips.2004.12.011
66. Li K, Chen L, Lin Z, Zhu J, Fang Y, Du J, et al. Role of the ampk/acc signaling pathway in trpp2-mediated head and neck cancer cell proliferation. BioMed Res Int (2020) 2020:4375075. doi: 10.1155/2020/4375075
67. Luo J, Hong Y, Lu Y, Qiu S, Chaganty BK, Zhang L, et al. Acetyl-coa carboxylase rewires cancer metabolism to allow cancer cells to survive inhibition of the warburg effect by cetuximab. Cancer Lett (2017) 384:39–49. doi: 10.1016/j.canlet.2016.09.020
68. Menendez JA, Lupu R. Fatty acid synthase and the lipogenic phenotype in cancer pathogenesis. Nat Rev Cancer (2007) 7(10):763–77. doi: 10.1038/nrc2222
69. Bandyopadhyay S, Pai SK, Watabe M, Gross SC, Hirota S, Hosobe S, et al. Fas expression inversely correlates with pten level in prostate cancer and a pi 3-kinase inhibitor synergizes with fas sirna to induce apoptosis. Oncogene (2005) 24(34):5389–95. doi: 10.1038/sj.onc.1208555
70. Ogino S, Nosho K, Meyerhardt JA, Kirkner GJ, Chan AT, Kawasaki T, et al. Cohort study of fatty acid synthase expression and patient survival in colon cancer. J Clin Oncol (2008) 26(35):5713–20. doi: 10.1200/jco.2008.18.2675
71. Horiguchi A, Asano T, Asano T, Ito K, Sumitomo M, Hayakawa M. Fatty acid synthase over expression is an indicator of tumor aggressiveness and poor prognosis in renal cell carcinoma. J Urol (2008) 180(3):1137–40. doi: 10.1016/j.juro.2008.04.135
72. Takahiro T, Shinichi K, Toshimitsu S. Expression of fatty acid synthase as a prognostic indicator in soft tissue sarcomas. Clin Cancer Res (2003) 9(6):2204–12.
73. Sebastiani V, Visca P, Botti C, Santeusanio G, Galati GM, Piccini V, et al. Fatty acid synthase is a marker of increased risk of recurrence in endometrial carcinoma. Gynecologic Oncol (2004) 92(1):101–5. doi: 10.1016/j.ygyno.2003.10.027
74. Alo PL, Visca P, Marci A, Mangoni A, Botti C, Di Tondo U. Expression of fatty acid synthase (Fas) as a predictor of recurrence in stage I breast carcinoma patients. Cancer (1996) 77(3):474–82. doi: 10.1002/(sici)1097-0142(19960201)77:3<474::Aid-cncr8>3.0.Co;2-k
75. Silva SD, Agostini M, Nishimoto IN, Coletta RD, Alves FA, Lopes MA, et al. Expression of fatty acid synthase, erbb2 and ki-67 in head and neck squamous cell carcinoma. A clinicopathological study. Oral Oncol (2004) 40(7):688–96. doi: 10.1016/j.oraloncology.2004.01.004
76. Silva SD, Perez DE, Nishimoto IN, Alves FA, Pinto CA, Kowalski LP, et al. Fatty acid synthase expression in squamous cell carcinoma of the tongue: clinicopathological findings. Oral Dis (2008) 14(4):376–82. doi: 10.1111/j.1601-0825.2007.01395.x
77. da Silva SD, Cunha IW, Nishimoto IN, Soares FA, Carraro DM, Kowalski LP, et al. Clinicopathological significance of ubiquitin-specific protease 2a (Usp2a), fatty acid synthase (Fasn), and erbb2 expression in oral squamous cell carcinomas. Oral Oncol (2009) 45(10):e134–9. doi: 10.1016/j.oraloncology.2009.02.004
78. de Angelis CM, de Lima-Souza RA, Scarini JF, Egal ESA, do Amaral-Silva GK, de Oliveira Gondak R, et al. Immunohistochemical expression of fatty acid synthase (Fasn) is correlated to tumor aggressiveness and cellular differentiation in salivary gland carcinomas. Head Neck Pathol (2021) 15(4):1119–26. doi: 10.1007/s12105-021-01319-3
79. Agostini M, Silva SD, Zecchin KG, Coletta RD, Jorge J, Loda M, et al. Fatty acid synthase is required for the proliferation of human oral squamous carcinoma cells. Oral Oncol (2004) 40(7):728–35. doi: 10.1016/j.oraloncology.2004.01.011
80. Silva SD, Cunha IW, Younes RN, Soares FA, Kowalski LP, Graner E. Erbb receptors and fatty acid synthase expression in aggressive head and neck squamous cell carcinomas. Oral Dis (2010) 16(8):774–80. doi: 10.1111/j.1601-0825.2010.01687.x
81. Silva SD, Cunha IW, Rangel AL, Jorge J, Zecchin KG, Agostini M, et al. Differential expression of fatty acid synthase (Fas) and erbb2 in nonmalignant and Malignant oral keratinocytes. Virchows Archiv an Int J Pathol (2008) 453(1):57–67. doi: 10.1007/s00428-008-0626-5
82. Agostini M, Almeida LY, Bastos DC, Ortega RM, Moreira FS, Seguin F, et al. The fatty acid synthase inhibitor orlistat reduces the growth and metastasis of orthotopic tongue oral squamous cell carcinomas. Mol Cancer Ther (2014) 13(3):585–95. doi: 10.1158/1535-7163.Mct-12-1136
83. Mims J, Bansal N, Bharadwaj MS, Chen X, Molina AJ, Tsang AW, et al. Energy metabolism in a matched model of radiation resistance for head and neck squamous cell cancer. Radiat Res (2015) 183(3):291–304. doi: 10.1667/rr13828.1
84. Boelcke WP, Teixeira IF, Aquino IG, Mazzaro AR, Cuadra-Zelaya FJM, de Souza AP, et al. Pharmacological fatty acid synthase inhibitors differently affect the Malignant phenotype of oral cancer cells. Arch Oral Biol (2022) 135:105343. doi: 10.1016/j.archoralbio.2021.105343
85. Aquino IG, Bastos DC, Cuadra-Zelaya FJM, Teixeira IF, Salo T, Coletta RD, et al. Anticancer properties of the fatty acid synthase inhibitor tvb-3166 on oral squamous cell carcinoma cell lines. Arch Oral Biol (2020) 113:104707. doi: 10.1016/j.archoralbio.2020.104707
86. Tesfay L, Paul BT, Konstorum A, Deng Z, Cox AO, Lee J, et al. Stearoyl-coa desaturase 1 protects ovarian cancer cells from ferroptotic cell death. Cancer Res (2019) 79(20):5355–66. doi: 10.1158/0008-5472.Can-19-0369
87. Miyazaki M, Dobrzyn A, Elias PM, Ntambi JM. Stearoyl-coa desaturase-2 gene expression is required for lipid synthesis during early skin and liver development. Proc Natl Acad Sci United States America (2005) 102(35):12501–6. doi: 10.1073/pnas.0503132102
88. Wang C, Shi M, Ji J, Cai Q, Zhao Q, Jiang J, et al. Stearoyl-coa desaturase 1 (Scd1) facilitates the growth and anti-ferroptosis of gastric cancer cells and predicts poor prognosis of gastric cancer. Aging (2020) 12(15):15374–91. doi: 10.18632/aging.103598
89. Ma XL, Sun YF, Wang BL, Shen MN, Zhou Y, Chen JW, et al. Sphere-forming culture enriches liver cancer stem cells and reveals stearoyl-coa desaturase 1 as a potential therapeutic target. BMC Cancer (2019) 19(1):760. doi: 10.1186/s12885-019-5963-z
90. Liao C, Li M, Li X, Li N, Zhao X, Wang X, et al. Trichothecin inhibits invasion and metastasis of colon carcinoma associating with scd-1-mediated metabolite alteration. Biochim Biophys Acta Mol Cell Biol Lipids (2020) 1865(2):158540. doi: 10.1016/j.bbalip.2019.158540
91. Abraham M, Sowmya SV, Rao RS, Haragannavar VC, Patil S, Augustine D, et al. Stearoyl coenzyme A desaturase: A diagnostic and prognostic biomarker in patients with oral epithelial dysplasia and oral squamous cell carcinoma. Trans Res Oral Oncol (2018) 2057178X18782512. doi: 10.1177/2057178x18782512
92. Nanjappa V, Renuse S, Sathe GJ, Raja R, Syed N, Radhakrishnan A, et al. Chronic exposure to chewing tobacco selects for overexpression of stearoyl-coa desaturase in normal oral keratinocytes. Cancer Biol Ther (2015) 16(11):1593–603. doi: 10.1080/15384047.2015.1078022
93. Zu XY, Zhang QH, Liu JH, Cao RX, Zhong J, Yi GH, et al. Atp citrate lyase inhibitors as novel cancer therapeutic agents. Recent patents anti-cancer Drug Discovery (2012) 7(2):154–67. doi: 10.2174/157489212799972954
94. Zhou Y, Bollu LR, Tozzi F, Ye X, Bhattacharya R, Gao G, et al. Atp citrate lyase mediates resistance of colorectal cancer cells to sn38. Mol Cancer Ther (2013) 12(12):2782–91. doi: 10.1158/1535-7163.Mct-13-0098
95. Beckner ME, Fellows-Mayle W, Zhang Z, Agostino NR, Kant JA, Day BW, et al. Identification of atp citrate lyase as a positive regulator of glycolytic function in glioblastomas. Int J Cancer (2010) 126(10):2282–95. doi: 10.1002/ijc.24918
96. Dai M, Yang B, Chen J, Liu F, Zhou Y, Zhou Y, et al. Nuclear-translocation of acly induced by obesity-related factors enhances pyrimidine metabolism through regulating histone acetylation in endometrial cancer. Cancer Lett (2021) 513:36–49. doi: 10.1016/j.canlet.2021.04.024
97. Migita T, Narita T, Nomura K, Miyagi E, Inazuka F, Matsuura M, et al. Atp citrate lyase: activation and therapeutic implications in non-small cell lung cancer. Cancer Res (2008) 68(20):8547–54. doi: 10.1158/0008-5472.Can-08-1235
98. Göttgens EL, van den Heuvel CN, de Jong MC, Kaanders JH, Leenders WP, Ansems M, et al. Acly (Atp citrate lyase) mediates radioresistance in head and neck squamous cell carcinomas and is a novel predictive radiotherapy biomarker. Cancers (2019) 11(12):1971. doi: 10.3390/cancers11121971
99. Zheng ZQ, Li ZX, Guan JL, Liu X, Li JY, Chen Y, et al. Long noncoding rna tincr-mediated regulation of acetyl-coa metabolism promotes nasopharyngeal carcinoma progression and chemoresistance. Cancer Res (2020) 80(23):5174–88. doi: 10.1158/0008-5472.Can-19-3626
100. Guo D, Reinitz F, Youssef M, Hong C, Nathanson D, Akhavan D, et al. An lxr agonist promotes glioblastoma cell death through inhibition of an egfr/akt/srebp-1/ldlr-dependent pathway. Cancer Discovery (2011) 1(5):442–56. doi: 10.1158/2159-8290.Cd-11-0102
101. Ettinger SL, Sobel R, Whitmore TG, Akbari M, Bradley DR, Gleave ME, et al. Dysregulation of sterol response element-binding proteins and downstream effectors in prostate cancer during progression to androgen independence. Cancer Res (2004) 64(6):2212–21. doi: 10.1158/0008-5472.can-2148-2
102. Bao J, Zhu L, Zhu Q, Su J, Liu M, Huang W. Srebp-1 is an independent prognostic marker and promotes invasion and migration in breast cancer. Oncol Lett (2016) 12(4):2409–16. doi: 10.3892/ol.2016.4988
103. Li C, Yang W, Zhang J, Zheng X, Yao Y, Tu K, et al. Srebp-1 has a prognostic role and contributes to invasion and metastasis in human hepatocellular carcinoma. Int J Mol Sci (2014) 15(5):7124–38. doi: 10.3390/ijms15057124
104. Tiong TY, Weng PW, Wang CH, Setiawan SA, Yadav VK, Pikatan NW, et al. Targeting the srebp-1/hsa-mir-497/scap/fasn oncometabolic axis inhibits the cancer stem-like and chemoresistant phenotype of non-small cell lung carcinoma cells. Int J Mol Sci (2022) 23(13):7283. doi: 10.3390/ijms23137283
105. Li X, Chen YT, Josson S, Mukhopadhyay NK, Kim J, Freeman MR, et al. Microrna-185 and 342 inhibit tumorigenicity and induce apoptosis through blockade of the srebp metabolic pathway in prostate cancer cells. PloS One (2013) 8(8):e70987. doi: 10.1371/journal.pone.0070987
106. Li LY, Yang Q, Jiang YY, Yang W, Jiang Y, Li X, et al. Interplay and cooperation between srebf1 and master transcription factors regulate lipid metabolism and tumor-promoting pathways in squamous cancer. Nat Commun (2021) 12(1):4362. doi: 10.1038/s41467-021-24656-x
107. Tan M, Lin X, Chen H, Ye W, Yi J, Li C, et al. Sterol regulatory element binding transcription factor 1 promotes proliferation and migration in head and neck squamous cell carcinoma. PeerJ (2023) 11:e15203. doi: 10.7717/peerj.15203
108. Fukuda M, Ogasawara Y, Hayashi H, Inoue K, Sakashita H. Resveratrol inhibits proliferation and induces autophagy by blocking srebp1 expression in oral cancer cells. Molecules (Basel Switzerland) (2022) 27(23):8250. doi: 10.3390/molecules27238250
109. Carracedo A, Cantley LC, Pandolfi PP. Cancer metabolism: fatty acid oxidation in the limelight. Nat Rev Cancer (2013) 13(4):227–32. doi: 10.1038/nrc3483
110. Li M, Xian HC, Tang YJ, Liang XH, Tang YL. Fatty acid oxidation: driver of lymph node metastasis. Cancer Cell Int (2021) 21(1):339. doi: 10.1186/s12935-021-02057-w
111. Luo X, Cheng C, Tan Z, Li N, Tang M, Yang L, et al. Emerging roles of lipid metabolism in cancer metastasis. Mol Cancer (2017) 16(1):76. doi: 10.1186/s12943-017-0646-3
112. Schlaepfer IR, Rider L, Rodrigues LU, Gijón MA, Pac CT, Romero L, et al. Lipid catabolism via cpt1 as a therapeutic target for prostate cancer. Mol Cancer Ther (2014) 13(10):2361–71. doi: 10.1158/1535-7163.Mct-14-0183
113. Tan Z, Zou Y, Zhu M, Luo Z, Wu T, Zheng C, et al. Carnitine palmitoyl transferase 1a is a novel diagnostic and predictive biomarker for breast cancer. BMC Cancer (2021) 21(1):409. doi: 10.1186/s12885-021-08134-7
114. Wang L, Li C, Song Y, Yan Z. Inhibition of carnitine palmitoyl transferase 1a-induced fatty acid oxidation suppresses cell progression in gastric cancer. Arch Biochem biophysics (2020) 696:108664. doi: 10.1016/j.abb.2020.108664
115. Xu A, Wang B, Fu J, Qin W, Yu T, Yang Z, et al. Diet-induced hepatic steatosis activates ras to promote hepatocarcinogenesis via cpt1α. Cancer Lett (2019) 442:40–52. doi: 10.1016/j.canlet.2018.10.024
116. Qu Q, Zeng F, Liu X, Wang QJ, Deng F. Fatty acid oxidation and carnitine palmitoyltransferase I: emerging therapeutic targets in cancer. Cell Death Dis (2016) 7(5):e2226. doi: 10.1038/cddis.2016.132
117. Mazzarelli P, Pucci S, Bonanno E, Sesti F, Calvani M, Spagnoli LG. Carnitine palmitoyltransferase I in human carcinomas: A novel role in histone deacetylation? Cancer Biol Ther (2007) 6(10):1606–13. doi: 10.4161/cbt.6.10.4742
118. Barros-Filho MC, Reis-Rosa LA, Hatakeyama M, Marchi FA, Chulam T, Scapulatempo-Neto C, et al. Oncogenic drivers in 11q13 associated with prognosis and response to therapy in advanced oropharyngeal carcinomas. Oral Oncol (2018) 83:81–90. doi: 10.1016/j.oraloncology.2018.06.010
119. Su YW, Wu PS, Lin SH, Huang WY, Kuo YS, Lin HP. Prognostic value of the overexpression of fatty acid metabolism-related enzymes in squamous cell carcinoma of the head and neck. Int J Mol Sci (2020) 21(18):6851. doi: 10.3390/ijms21186851
120. Prentki M, Madiraju SR. Glycerolipid metabolism and signaling in health and disease. Endocrine Rev (2008) 29(6):647–76. doi: 10.1210/er.2008-0007
121. Zechner R, Zimmermann R, Eichmann TO, Kohlwein SD, Haemmerle G, Lass A, et al. Fat signals–lipases and lipolysis in lipid metabolism and signaling. Cell Metab (2012) 15(3):279–91. doi: 10.1016/j.cmet.2011.12.018
122. Liu X, Lu Y, Chen Z, Liu X, Hu W, Zheng L, et al. The ubiquitin-specific peptidase usp18 promotes lipolysis, fatty acid oxidation, and lung cancer growth. Mol Cancer Res MCR (2021) 19(4):667–77. doi: 10.1158/1541-7786.Mcr-20-0579
123. Iftikhar R, Penrose HM, King AN, Samudre JS, Collins ME, Hartono AB, et al. Elevated atgl in colon cancer cells and cancer stem cells promotes metabolic and tumorigenic reprogramming reinforced by obesity. Oncogenesis (2021) 10(11):82. doi: 10.1038/s41389-021-00373-4
124. Tripathi P, Kamarajan P, Somashekar BS, MacKinnon N, Chinnaiyan AM, Kapila YL, et al. Delineating metabolic signatures of head and neck squamous cell carcinoma: phospholipase A2, a potential therapeutic target. Int J Biochem Cell Biol (2012) 44(11):1852–61. doi: 10.1016/j.biocel.2012.06.025
125. Zhou X, Wei J, Chen F, Xiao X, Huang T, He Q, et al. Epigenetic downregulation of the isg15-conjugating enzyme ubch8 impairs lipolysis and correlates with poor prognosis in nasopharyngeal carcinoma. Oncotarget (2015) 6(38):41077–91. doi: 10.18632/oncotarget.6218
126. Zheng S, Matskova L, Zhou X, Xiao X, Huang G, Zhang Z, et al. Downregulation of adipose triglyceride lipase by eb viral-encoded lmp2a links lipid accumulation to increased migration in nasopharyngeal carcinoma. Mol Oncol (2020) 14(12):3234–52. doi: 10.1002/1878-0261.12824
127. Dolce V, Cappello AR, Lappano R, Maggiolini M. Glycerophospholipid synthesis as a novel drug target against cancer. Curr Mol Pharmacol (2011) 4(3):167–75. doi: 10.2174/1874467211104030167
128. Murakami M, Sato H, Taketomi Y. Updating phospholipase a(2) biology. Biomolecules (2020) 10(10):1457. doi: 10.3390/biom10101457
129. Jelonek K, Pietrowska M, Ros M, Zagdanski A, Suchwalko A, Polanska J, et al. Radiation-induced changes in serum lipidome of head and neck cancer patients. Int J Mol Sci (2014) 15(4):6609–24. doi: 10.3390/ijms15046609
130. Kita Y, Shindou H, Shimizu T. Cytosolic phospholipase a(2) and lysophospholipid acyltransferases. Biochim Biophys Acta Mol Cell Biol Lipids (2019) 1864(6):838–45. doi: 10.1016/j.bbalip.2018.08.006
131. Peng Z, Chang Y, Fan J, Ji W, Su C. Phospholipase A2 superfamily in cancer. Cancer Lett (2021) 497:165–77. doi: 10.1016/j.canlet.2020.10.021
132. Dong Q, Patel M, Scott KF, Graham GG, Russell PJ, Sved P. Oncogenic action of phospholipase A2 in prostate cancer. Cancer Lett (2006) 240(1):9–16. doi: 10.1016/j.canlet.2005.08.012
133. Denizot Y, Chianéa T, Labrousse F, Truffinet V, Delage M, Mathonnet M. Platelet-activating factor and human thyroid cancer. Eur J Endocrinol (2005) 153(1):31–40. doi: 10.1530/eje.1.01947
134. Yamashita S, Yamashita J, Ogawa M. Overexpression of group ii phospholipase A2 in human breast cancer tissues is closely associated with their Malignant potency. Br J Cancer (1994) 69(6):1166–70. doi: 10.1038/bjc.1994.229
135. Cai Q, Zhao Z, Antalis C, Yan L, Del Priore G, Hamed AH, et al. Elevated and secreted phospholipase a2 Activities as new potential therapeutic targets in human epithelial ovarian cancer. FASEB J Off Publ Fed Am Societies Exp Biol (2012) 26(8):3306–20. doi: 10.1096/fj.12-207597
136. Menschikowski M, Hagelgans A, Schuler U, Froeschke S, Rosner A, Siegert G. Plasma levels of phospholipase A2-iia in patients with different types of Malignancies: prognosis and association with inflammatory and coagulation biomarkers. Pathol Oncol Res POR (2013) 19(4):839–46. doi: 10.1007/s12253-013-9652-y
137. Askari M, Darabi M, Zare Mahmudabadi R, Oboodiat M, Fayezi S, Mostakhdemin Hosseini Z, et al. Tissue fatty acid composition and secretory phospholipase-A2 activity in oral squamous cell carcinoma. Clin Trans Oncol Off Publ Fed Spanish Oncol Societies Natl Cancer Institute Mexico (2015) 17(5):378–83. doi: 10.1007/s12094-014-1242-2
138. Hannun YA, Obeid LM. Sphingolipids and their metabolism in physiology and disease. Nat Rev Mol Cell Biol (2018) 19(3):175–91. doi: 10.1038/nrm.2017.107
139. Ogretmen B. Sphingolipid metabolism in cancer signalling and therapy. Nat Rev Cancer (2018) 18(1):33–50. doi: 10.1038/nrc.2017.96
140. van Kruining D, Luo Q, van Echten-Deckert G, Mielke MM, Bowman A, Ellis S, et al. Sphingolipids as prognostic biomarkers of neurodegeneration, neuroinflammation, and psychiatric diseases and their emerging role in lipidomic investigation methods. Advanced Drug delivery Rev (2020) 159:232–44. doi: 10.1016/j.addr.2020.04.009
141. Yuan Y, Chi F, Wang S, Wang Z. Significance of ceramide and DNA ploidy in laryngeal carcinogenesis. ORL; J oto-rhino-laryngology its related specialties (2007) 69(5):283–8. doi: 10.1159/000103872
142. Chi FL, Yuan YS, Wang SY, Wang ZM. Study on ceramide expression and DNA content in patients with healthy mucosa, leukoplakia, and carcinoma of the larynx. Arch otolaryngology–head Neck Surg (2004) 130(3):307–10. doi: 10.1001/archotol.130.3.307
143. Young MR, Neville BW, Chi AC, Lathers DM, Gillespie MB, Day TA. Autocrine motility-stimulatory pathways of oral premalignant lesion cells. Clin Exp metastasis (2007) 24(2):131–9. doi: 10.1007/s10585-007-9063-0
144. Karahatay S, Thomas K, Koybasi S, Senkal CE, Elojeimy S, Liu X, et al. Clinical relevance of ceramide metabolism in the pathogenesis of human head and neck squamous cell carcinoma (Hnscc): attenuation of C(18)-ceramide in hnscc tumors correlates with lymphovascular invasion and nodal metastasis. Cancer Lett (2007) 256(1):101–11. doi: 10.1016/j.canlet.2007.06.003
145. Mehta S, Blackinton D, Omar I, Kouttab N, Myrick D, Klostergaard J, et al. Combined cytotoxic action of paclitaxel and ceramide against the human tu138 head and neck squamous carcinoma cell line. Cancer chemotherapy Pharmacol (2000) 46(2):85–92. doi: 10.1007/s002800000140
146. Senkal CE, Ponnusamy S, Rossi MJ, Sundararaj K, Szulc Z, Bielawski J, et al. Potent antitumor activity of a novel cationic pyridinium-ceramide alone or in combination with gemcitabine against human head and neck squamous cell carcinomas in vitro and in vivo. J Pharmacol Exp Ther (2006) 317(3):1188–99. doi: 10.1124/jpet.106.101949
147. Senkal CE, Ponnusamy S, Rossi MJ, Bialewski J, Sinha D, Jiang JC, et al. Role of human longevity assurance gene 1 and C18-ceramide in chemotherapy-induced cell death in human head and neck squamous cell carcinomas. Mol Cancer Ther (2007) 6(2):712–22. doi: 10.1158/1535-7163.Mct-06-0558
148. Saddoughi SA, Garrett-Mayer E, Chaudhary U, O'Brien PE, Afrin LB, Day TA, et al. Results of a phase ii trial of gemcitabine plus doxorubicin in patients with recurrent head and neck cancers: serum C18-ceramide as a novel biomarker for monitoring response. Clin Cancer Res (2011) 17(18):6097–105. doi: 10.1158/1078-0432.Ccr-11-0930
149. Facchinetti MM, Gandini NA, Fermento ME, Sterin-Speziale NB, Ji Y, Patel V, et al. The expression of sphingosine kinase-1 in head and neck carcinoma. Cells tissues organs (2010) 192(5):314–24. doi: 10.1159/000318173
150. Shirai K, Kaneshiro T, Wada M, Furuya H, Bielawski J, Hannun YA, et al. A role of sphingosine kinase 1 in head and neck carcinogenesis. Cancer Prev Res (Philadelphia Pa) (2011) 4(3):454–62. doi: 10.1158/1940-6207.Capr-10-0299
151. Sinha UK, Schorn VJ, Hochstim C, Chinn SB, Zhu S, Masood R. Increased radiation sensitivity of head and neck squamous cell carcinoma with sphingosine kinase 1 inhibition. Head Neck (2011) 33(2):178–88. doi: 10.1002/hed.21418
152. Tamashiro PM, Furuya H, Shimizu Y, Kawamori T. Sphingosine kinase 1 mediates head & Neck squamous cell carcinoma invasion through sphingosine 1-phosphate receptor 1. Cancer Cell Int (2014) 14(1):76. doi: 10.1186/s12935-014-0076-x
153. Tamashiro PM, Furuya H, Shimizu Y, Iino K, Kawamori T. The impact of sphingosine kinase-1 in head and neck cancer. Biomolecules (2013) 3(3):481–513. doi: 10.3390/biom3030481
154. Coant N, Sakamoto W, Mao C, Hannun YA. Ceramidases, roles in sphingolipid metabolism and in health and disease. Adv Biol Regul (2017) 63:122–31. doi: 10.1016/j.jbior.2016.10.002
155. Elojeimy S, Liu X, McKillop JC, El-Zawahry AM, Holman DH, Cheng JY, et al. Role of acid ceramidase in resistance to fasl: therapeutic approaches based on acid ceramidase inhibitors and fasl gene therapy. Mol Ther J Am Soc Gene Ther (2007) 15(7):1259–63. doi: 10.1038/sj.mt.6300167
156. Roh JL, Park JY, Kim EH, Jang HJ. Targeting acid ceramidase sensitises head and neck cancer to cisplatin. Eur J Cancer (Oxford Engl 1990) (2016) 52:163–72. doi: 10.1016/j.ejca.2015.10.056
157. Beckham TH, Elojeimy S, Cheng JC, Turner LS, Hoffman SR, Norris JS, et al. Targeting sphingolipid metabolism in head and neck cancer: rational therapeutic potentials. Expert Opin Ther Targets (2010) 14(5):529–39. doi: 10.1517/14728221003752768
158. Yamada C, Ho A, Nusbaum A, Xu R, Davey ME, Nichols F, et al. Inhibitory effect of porphyromonas gingivalis-derived phosphoethanolamine dihydroceramide on acid ceramidase expression in oral squamous cells. J Cell Mol Med (2023) 27(9):1290–5. doi: 10.1111/jcmm.17722
159. Wollam J, Antebi A. Sterol regulation of metabolism, homeostasis, and development. Annu Rev Biochem (2011) 80:885–916. doi: 10.1146/annurev-biochem-081308-165917
160. Huang B, Song BL, Xu C. Cholesterol metabolism in cancer: mechanisms and therapeutic opportunities. Nat Metab (2020) 2(2):132–41. doi: 10.1038/s42255-020-0174-0
161. Goldstein JL, Brown MS. The ldl receptor. Arteriosclerosis thrombosis Vasc Biol (2009) 29(4):431–8. doi: 10.1161/atvbaha.108.179564
162. Phillips MC. Molecular mechanisms of cellular cholesterol efflux. J Biol Chem (2014) 289(35):24020–9. doi: 10.1074/jbc.R114.583658
163. Chang TY, Li BL, Chang CC, Urano Y. Acyl-coenzyme A:Cholesterol acyltransferases. Am J Physiol Endocrinol Metab (2009) 297(1):E1–9. doi: 10.1152/ajpendo.90926.2008
164. Choi SY, Cheong HK, Lee MK, Kang JW, Lee YC, Oh IH, et al. Metabolic diseases and risk of head and neck cancer: A cohort study analyzing nationwide population-based data. Cancers (2022) 14(13):3277. doi: 10.3390/cancers14133277
165. Brown MS, Goldstein JL. The srebp pathway: regulation of cholesterol metabolism by proteolysis of a membrane-bound transcription factor. Cell (1997) 89(3):331–40. doi: 10.1016/s0092-8674(00)80213-5
166. Ming G, Li W, Bi-ru Z, Yue-hong S, Hong-yu Y. Effect of srebp2 on proliferation and migration of oral squamous cell carcinoma and its regulatory mechanism. China J Oral Maxillofac Surg (2021) 19(4):295.
167. Huang J, Zhao X, Li X, Peng J, Yang W, Mi S. Hmgcr inhibition stabilizes the glycolytic enzyme pkm2 to support the growth of renal cell carcinoma. PloS Biol (2021) 19(4):e3001197. doi: 10.1371/journal.pbio.3001197
168. Bengtsson E, Nerjovaj P, Wangefjord S, Nodin B, Eberhard J, Uhlén M, et al. Hmg-coa reductase expression in primary colorectal cancer correlates with favourable clinicopathological characteristics and an improved clinical outcome. Diagn Pathol (2014) 9:78. doi: 10.1186/1746-1596-9-78
169. Brennan DJ, Brändstedt J, Rexhepaj E, Foley M, Pontén F, Uhlén M, et al. Tumour-specific hmg-coar is an independent predictor of recurrence free survival in epithelial ovarian cancer. BMC Cancer (2010) 10:125. doi: 10.1186/1471-2407-10-125
170. Chang JT, Wang HM, Chang KW, Chen WH, Wen MC, Hsu YM, et al. Identification of differentially expressed genes in oral squamous cell carcinoma (Oscc): overexpression of npm, cdk1 and ndrg1 and underexpression of ches1. Int J Cancer (2005) 114(6):942–9. doi: 10.1002/ijc.20663
171. Ricco N, Flor A, Wolfgeher D, Efimova EV, Ramamurthy A, Appelbe OK, et al. Mevalonate pathway activity as a determinant of radiation sensitivity in head and neck cancer. Mol Oncol (2019) 13(9):1927–43. doi: 10.1002/1878-0261.12535
172. Getz KR, Bellile E, Zarins KR, Rullman C, Chinn SB, Taylor JMG, et al. Statin use and head and neck squamous cell carcinoma outcomes. Int J Cancer (2020) 148(10):2440–8. doi: 10.1002/ijc.33441
173. Gupta A, Stokes W, Eguchi M, Hararah M, Amini A, Mueller A, et al. Statin use associated with improved overall and cancer specific survival in patients with head and neck cancer. Oral Oncol (2019) 90:54–66. doi: 10.1016/j.oraloncology.2019.01.019
174. Fernandez KA, Allen P, Campbell M, Page B, Townes T, Li CM, et al. Atorvastatin is associated with reduced cisplatin-induced hearing loss. J Clin Invest (2021) 131(1):e142616. doi: 10.1172/jci142616
175. Gormley M, Yarmolinsky J, Dudding T, Burrows K, Martin RM, Thomas S, et al. Using genetic variants to evaluate the causal effect of cholesterol lowering on head and neck cancer risk: A mendelian randomization study. PloS Genet (2021) 17(4):e1009525. doi: 10.1371/journal.pgen.1009525
176. Li J, Yang T, Wang Q, Li Y, Wu H, Zhang M, et al. Upregulation of sqle contributes to poor survival in head and neck squamous cell carcinoma. Int J Biol Sci (2022) 18(9):3576–91. doi: 10.7150/ijbs.68216
177. Liu Y, Fang L, Liu W. High sqle expression and gene amplification correlates with poor prognosis in head and neck squamous cell carcinoma. Cancer Manage Res (2021) 13:4709–23. doi: 10.2147/cmar.S305719
178. Xu X, Chen J, Li Y, Yang X, Wang Q, Wen Y, et al. Targeting epigenetic modulation of cholesterol synthesis as a therapeutic strategy for head and neck squamous cell carcinoma. Cell Death Dis (2021) 12(5):482. doi: 10.1038/s41419-021-03760-2
179. Chien MH, Lee TS, Kao C, Yang SF, Lee WS. Terbinafine inhibits oral squamous cell carcinoma growth through anti-cancer cell proliferation and anti-angiogenesis. Mol carcinogenesis (2012) 51(5):389–99. doi: 10.1002/mc.20800
180. Li B, Lu L, Zhong M, Tan XX, Liu CY, Guo Y, et al. Terbinafine inhibits ksr1 and suppresses raf-mek-erk signaling in oral squamous cell carcinoma cells. Neoplasma (2013) 60(4):406–12. doi: 10.4149/neo_2013_052
181. Nienstedt JC, Schroeder C, Clauditz T, Simon R, Sauter G, Muenscher A, et al. Ezh2 overexpression in head and neck cancer is related to lymph node metastasis. J Oral Pathol Med (2018) 47(3):240–5. doi: 10.1111/jop.12673
182. Mochizuki D, Misawa Y, Kawasaki H, Imai A, Endo S, Mima M, et al. Aberrant epigenetic regulation in head and neck cancer due to distinct ezh2 overexpression and DNA hypermethylation. Int J Mol Sci (2018) 19(12):3707. doi: 10.3390/ijms19123707
183. Guillaumond F, Bidaut G, Ouaissi M, Servais S, Gouirand V, Olivares O, et al. Cholesterol uptake disruption, in association with chemotherapy, is a promising combined metabolic therapy for pancreatic adenocarcinoma. Proc Natl Acad Sci United States America (2015) 112(8):2473–8. doi: 10.1073/pnas.1421601112
184. de Gonzalo-Calvo D, López-Vilaró L, Nasarre L, Perez-Olabarria M, Vázquez T, Escuin D, et al. Intratumor cholesteryl ester accumulation is associated with human breast cancer proliferation and aggressive potential: A molecular and clinicopathological study. BMC Cancer (2015) 15:460. doi: 10.1186/s12885-015-1469-5
185. Mirbod SM, Ahing SI. Tobacco-associated lesions of the oral cavity: part I. Nonmalignant lesions. J (Canadian Dental Association) (2000) 66(5):252–6.
186. Mirbod SM, Ahing SI. Tobacco-associated lesions of the oral cavity: part ii. Malignant lesions. . J (Canadian Dental Association) (2000) 66(6):308–11.
187. Kumar P, Augustine J, Urs AB, Arora S, Gupta S, Mohanty VR. Serum lipid profile in oral cancer and leukoplakia: correlation with tobacco abuse and histological grading. J Cancer Res Ther (2012) 8(3):384–8. doi: 10.4103/0973-1482.103517
188. Mohan SLC, Satyanarayana D. Altered serum lipids in the cases of head and neck cancer associated with the habit of tobacco consumption. Int J Otolaryngol Head Neck Surg (2017) 6(3):28–37. doi: 10.4236/ijohns.2017.63006
189. Lohe VK, Degwekar SS, Bhowate RR, Kadu RP, Dangore SB. Evaluation of correlation of serum lipid profile in patients with oral cancer and precancer and its association with tobacco abuse. J Oral Pathol Med (2010) 39(2):141–8. doi: 10.1111/j.1600-0714.2009.00828.x
190. Banseria N, Malik R, Nigam RK, Trichal V, Shrivastava A, Jain R, et al. Correlation of serum lipid profile, serum calcium, alkaline phosphatase and serum protein with histopathological grading and staging in head and neck cancer. J Evol Med Dent Sci (2014) 3:1978–86. doi: 10.14260/jemds/2014/2090
191. Ames BN. Dietary carcinogens and anticarcinogens. Oxygen radicals and degenerative diseases. Sci (New York NY) (1983) 221(4617):1256–64. doi: 10.1126/science.6351251
192. Di Credico G, Polesel J, Dal Maso L, Pauli F, Torelli N, Luce D, et al. Alcohol drinking and head and neck cancer risk: the joint effect of intensity and duration. Br J Cancer (2020) 123(9):1456–63. doi: 10.1038/s41416-020-01031-z
193. Koo HY, Han K, Shin DW, Yoo JE, Cho MH, Jeon KH, et al. Alcohol drinking pattern and risk of head and neck cancer: A nationwide cohort study. Int J Environ Res Public Health (2021) 18(21);11204. doi: 10.3390/ijerph182111204
194. Lee WT, Hsiao JR, Ou CY, Huang CC, Chang CC, Tsai ST, et al. The influence of prediagnosis alcohol consumption and the polymorphisms of ethanol-metabolizing genes on the survival of head and neck cancer patients. Cancer epidemiology Biomarkers Prev Publ Am Assoc Cancer Research cosponsored by Am Soc Prev Oncol (2019) 28(2):248–57. doi: 10.1158/1055-9965.Epi-18-0425
195. Tsai ST, Wong TY, Ou CY, Fang SY, Chen KC, Hsiao JR, et al. The interplay between alcohol consumption, oral hygiene, aldh2 and adh1b in the risk of head and neck cancer. Int J Cancer (2014) 135(10):2424–36. doi: 10.1002/ijc.28885
196. Tomita K, Azuma T, Kitamura N, Nishida J, Tamiya G, Oka A, et al. Pioglitazone Prevents Alcohol-Induced Fatty Liver in Rats through up-Regulation of C-Met. Gastroenterology (2004) 126(3):873–85. doi: 10.1053/j.gastro.2003.12.008
197. Grønhøj C, Jakobsen KK, Jensen DH, Rasmussen J, Andersen E, Friborg J, et al. Pattern of and survival following loco-regional and distant recurrence in patients with hpv+ and hpv- oropharyngeal squamous cell carcinoma: A population-based study. Oral Oncol (2018) 83:127–33. doi: 10.1016/j.oraloncology.2018.06.012
198. Ang KK, Harris J, Wheeler R, Weber R, Rosenthal DI, Nguyen-Tân PF, et al. Human papillomavirus and survival of patients with oropharyngeal cancer. New Engl J Med (2010) 363(1):24–35. doi: 10.1056/NEJMoa0912217
199. Leemans CR, Snijders PJF, Brakenhoff RH. The molecular landscape of head and neck cancer. Nat Rev Cancer (2018) 18(5):269–82. doi: 10.1038/nrc.2018.11
200. Sitarz K, Czamara K, Szostek S, Kaczor A. The impact of hpv infection on human glycogen and lipid metabolism - a review. Biochim Biophys Acta Rev Cancer (2022) 1877(1):188646. doi: 10.1016/j.bbcan.2021.188646
201. Yi J, Zhu J, Wu J, Thompson CB, Jiang X. Oncogenic activation of pi3k-akt-mtor signaling suppresses ferroptosis via srebp-mediated lipogenesis. Proc Natl Acad Sci United States America (2020) 117(49):31189–97. doi: 10.1073/pnas.2017152117
202. Menges CW, Baglia LA, Lapoint R, McCance DJ. Human Papillomavirus Type 16 E7 up-Regulates Akt Activity through the Retinoblastoma Protein. Cancer Res (2006) 66(11):5555–9. doi: 10.1158/0008-5472.Can-06-0499
203. Mauro C, Leow SC, Anso E, Rocha S, Thotakura AK, Tornatore L, et al. Nf-Κb controls energy homeostasis and metabolic adaptation by upregulating mitochondrial respiration. Nat Cell Biol (2011) 13(10):1272–9. doi: 10.1038/ncb2324
204. Kawai I, Matsumura H, Fujii W, Naito K, Kusakabe K, Kiso Y, et al. Discoidin domain receptor 2 (Ddr2) regulates body size and fat metabolism in mice. Transgenic Res (2014) 23(1):165–75. doi: 10.1007/s11248-013-9751-2
205. Hao Y, Samuels Y, Li Q, Krokowski D, Guan BJ, Wang C, et al. Oncogenic pik3ca mutations reprogram glutamine metabolism in colorectal cancer. Nat Commun (2016) 7:11971. doi: 10.1038/ncomms11971
206. Bradshaw PT, Siega-Riz AM, Campbell M, Weissler MC, Funkhouser WK, Olshan AF. Associations between dietary patterns and head and neck cancer: the carolina head and neck cancer epidemiology study. Am J Epidemiol (2012) 175(12):1225–33. doi: 10.1093/aje/kwr468
207. Edefonti V, Hashibe M, Ambrogi F, Parpinel M, Bravi F, Talamini R, et al. Nutrient-based dietary patterns and the risk of head and neck cancer: A pooled analysis in the international head and neck cancer epidemiology consortium. Ann Oncol (2012) 23(7):1869–80. doi: 10.1093/annonc/mdr548
208. De Vito R, Lee YCA, Parpinel M, Serraino D, Olshan AF, Zevallos JP, et al. Shared and study-specific dietary patterns and head and neck cancer risk in an international consortium. Epidemiol (Cambridge Mass) (2019) 30(1):93–102. doi: 10.1097/ede.0000000000000902
209. Edefonti V, Bravi F, La Vecchia C, Randi G, Ferraroni M, Garavello W, et al. Nutrient-based dietary patterns and the risk of oral and pharyngeal cancer. Oral Oncol (2010) 46(5):343–8. doi: 10.1016/j.oraloncology.2009.11.017
210. Edefonti V, Bravi F, Garavello W, La Vecchia C, Parpinel M, Franceschi S, et al. Nutrient-based dietary patterns and laryngeal cancer: evidence from an exploratory factor analysis. Cancer epidemiology Biomarkers Prev Publ Am Assoc Cancer Research cosponsored by Am Soc Prev Oncol (2010) 19(1):18–27. doi: 10.1158/1055-9965.Epi-09-0900
211. Taha HM, Rozek LS, Chen X, Li Z, Zarins KR, Slade AN, et al. Risk of disease recurrence and mortality varies by type of fat consumed before cancer treatment in a longitudinal cohort of head and neck squamous cell carcinoma patients. J Nutr (2022) 152(5):1298–305. doi: 10.1093/jn/nxac032
212. Lee HC, Liang A, Lin YH, Guo YR, Huang SY. Low dietary N-6/N-3 polyunsaturated fatty acid ratio prevents induced oral carcinoma in a hamster pouch model. Prostaglandins leukotrienes essential Fatty Acids (2018) 136:67–75. doi: 10.1016/j.plefa.2017.03.003
213. Calle EE, Rodriguez C, Walker-Thurmond K, Thun MJ. Overweight, obesity, and mortality from cancer in a prospectively studied cohort of U.S. Adults. New Engl J Med (2003) 348(17):1625–38. doi: 10.1056/NEJMoa021423
214. Harvey AE, Lashinger LM, Hursting SD. The growing challenge of obesity and cancer: an inflammatory issue. Ann New York Acad Sci (2011) 1229:45–52. doi: 10.1111/j.1749-6632.2011.06096.x
215. De Pergola G, Silvestris F. Obesity as a major risk factor for cancer. J Obes (2013) 2013:291546. doi: 10.1155/2013/291546
216. He Q, Xia B, Liu A, Li M, Zhou Z, Cheung EC, et al. Association of body composition with risk of overall and site-specific cancers: A population-based prospective cohort study. Int J Cancer (2021) 149(7):1435–47. doi: 10.1002/ijc.33697
217. Lengyel E, Makowski L, DiGiovanni J, Kolonin MG. Cancer as a matter of fat: the crosstalk between adipose tissue and tumors. Trends Cancer (2018) 4(5):374–84. doi: 10.1016/j.trecan.2018.03.004
218. Gaudet MM, Patel AV, Sun J, Hildebrand JS, McCullough ML, Chen AY, et al. Prospective studies of body mass index with head and neck cancer incidence and mortality. Cancer epidemiology Biomarkers Prev Publ Am Assoc Cancer Research cosponsored by Am Soc Prev Oncol (2012) 21(3):497–503. doi: 10.1158/1055-9965.Epi-11-0935
219. Hashibe M, Hunt J, Wei M, Buys S, Gren L, Lee YC. Tobacco, alcohol, body mass index, physical activity, and the risk of head and neck cancer in the prostate, lung, colorectal, and ovarian (Plco) cohort. Head Neck (2013) 35(7):914–22. doi: 10.1002/hed.23052
220. Iyengar NM, Kochhar A, Morris PG, Morris LG, Zhou XK, Ghossein RA, et al. Impact of obesity on the survival of patients with early-stage squamous cell carcinoma of the oral tongue. Cancer (2014) 120(7):983–91. doi: 10.1002/cncr.28532
221. Hu Q, Peng J, Chen X, Li H, Song M, Cheng B, et al. Obesity and genes related to lipid metabolism predict poor survival in oral squamous cell carcinoma. Oral Oncol (2019) 89:14–22. doi: 10.1016/j.oraloncology.2018.12.006
222. Franceschi S, Dal Maso L, Levi F, Conti E, Talamini R, La Vecchia C. Leanness as early marker of cancer of the oral cavity and pharynx. Ann Oncol (2001) 12(3):331–6. doi: 10.1023/a:1011191809335
223. Hicks DF, Bakst R, Doucette J, Kann BH, Miles B, Genden E, et al. Impact of obesity on outcomes for patients with head and neck cancer. Oral Oncol (2018) 83:11–7. doi: 10.1016/j.oraloncology.2018.05.027
224. Kreimer AR, Randi G, Herrero R, Castellsagué X, La Vecchia C, Franceschi S. Diet and body mass, and oral and oropharyngeal squamous cell carcinomas: analysis from the iarc multinational case-control study. Int J Cancer (2006) 118(9):2293–7. doi: 10.1002/ijc.21577
Keywords: lipid metabolism, reprogramming, head and neck cancer, biomarker, prognosis
Citation: Liang J, Li L, Li L, Zhou X, Zhang Z, Huang Y and Xiao X (2023) Lipid metabolism reprogramming in head and neck cancer. Front. Oncol. 13:1271505. doi: 10.3389/fonc.2023.1271505
Received: 02 August 2023; Accepted: 09 October 2023;
Published: 20 October 2023.
Edited by:
Bela Ozsvari, University of Salford, United KingdomReviewed by:
Pritam Sadhukhan, Johns Hopkins University, United StatesZhengrui Li, Shanghai Jiao Tong University, China
Balkrishna Chaube, Yale University, United States
Copyright © 2023 Liang, Li, Li, Zhou, Zhang, Huang and Xiao. This is an open-access article distributed under the terms of the Creative Commons Attribution License (CC BY). The use, distribution or reproduction in other forums is permitted, provided the original author(s) and the copyright owner(s) are credited and that the original publication in this journal is cited, in accordance with accepted academic practice. No use, distribution or reproduction is permitted which does not comply with these terms.
*Correspondence: Xue Xiao, eGlhb3h1ZUBneG11LmVkdS5jbg==; Yi Huang, eWkuaHVhbmdAc3IuZ3htdS5lZHUuY24=
†These authors have contributed equally to this work