- 1Center for Translational Research, Lady Davis Research Institute-Jewish General Hospital, Montreal, QC, Canada
- 2Pharmacology and Therapeutics, McGill University, Montreal, QC, Canada
- 3Department of Obstetrics and Gynecology, University of Chicago, Chicago, IL, United States
- 4AOA Dx, Boston, MA, United States
- 5Ophthalmology and Vision Science, McGill University, Montreal, QC, Canada
One unexploited family of cancer biomarkers comprise glycoproteins, carbohydrates, and glycolipids (the Tumor Glycocode).A class of glycolipid cancer biomarkers, the tumor-marker gangliosides (TMGs) are presented here as potential diagnostics for detecting cancer, especially at early stages, as the biological function of TMGs makes them etiological. We propose that a quantitative matrix of the Cancer Biomarker Glycocode and artificial intelligence-driven algorithms will expand the menu of validated cancer biomarkers as a step to resolve some of the challenges in cancer diagnosis, and yield a combination that can identify a specific cancer, in a tissue-agnostic manner especially at early stages, to enable early intervention. Diagnosis is critical to reducing cancer mortality but many cancers lack efficient and effective diagnostic tests, especially for early stage disease. Ideal diagnostic biomarkers are etiological, samples are preferably obtained via non-invasive methods (e.g. liquid biopsy of blood or urine), and are quantitated using assays that yield high diagnostic sensitivity and specificity for efficient diagnosis, prognosis, or predicting response to therapy. Validated biomarkers with these features are rare. While the advent of proteomics and genomics has led to the identification of a multitude of proteins and nucleic acid sequences as cancer biomarkers, relatively few have been approved for clinical use. The use of multiplex arrays and artificial intelligence-driven algorithms offer the option of combining data of known biomarkers; however, for most, the sensitivity and the specificity are below acceptable criteria, and clinical validation has proven difficult. One strategic solution to this problem is to expand the biomarker families beyond those currently exploited. One unexploited family of cancer biomarkers comprise glycoproteins, carbohydrates, and glycolipids (the Tumor Glycocode). Here, we focus on a family of glycolipid cancer biomarkers, the tumor-marker gangliosides (TMGs). We discuss the diagnostic potential of TMGs for detecting cancer, especially at early stages. We include prior studies from the literature to summarize findings for ganglioside quantification, expression, detection, and biological function and its role in various cancers. We highlight the examples of TMGs exhibiting ideal properties of cancer diagnostic biomarkers, and the application of GD2 and GD3 for diagnosis of early stage cancers with high sensitivity and specificity. We propose that a quantitative matrix of the Cancer Biomarker Glycocode and artificial intelligence-driven algorithms will expand the menu of validated cancer biomarkers as a step to resolve some of the challenges in cancer diagnosis, and yield a combination that can identify a specific cancer, in a tissue-agnostic manner especially at early stages, to enable early intervention.
1 Introduction
The tumor glycocode includes Tumor-Associated Carbohydrate Antigens (TACAs) that are carbohydrates that are displayed on cancer cell surfaces as glycans, glycoproteins and glycolipids, or can appear in soluble forms in circulation. Gangliosides are a subclass of glycolipids that contain one or more sialic acid residues. The specific TMGs, GD2 and GD3, are part of the tumor glycocode or “sugar code” in cancer (1–6). Gangliosides are the focus of this review, as they exemplify a new class of diagnostic biomarkers for cancer.
Historically, glycolipids in general have not been explored as diagnostic biomarkers because they are difficult to quantify using conventional proteomics or genomics techniques. Moreover, glycolipids have complex pathways for biosynthesis, metabolism, and catabolism. Methods that enable exploitation of these markers are now emerging. Thus, this review focuses on the growing evidence that TMGs represent a novel class of biomarkers with great potential in the early diagnosis of specific types of cancer (1–3).
Cancer is the second leading cause of death in the United States. The American Cancer Society estimates that over 1.9 million individuals will be diagnosed with cancer in 2023 and about 609,820 individuals will die of the disease (7). Efforts to reduce cancer mortality focus on improving cancer prevention, cancer screening (detecting cancer in asymptomatic individuals), early cancer diagnosis (detecting cancer in symptomatic individuals), and cancer treatment. Early cancer diagnosis enables rapid intervention when cancer is at an early stage and treatment outcomes are optimal (8). This has been shown to reduce mortality and morbidity, improve quality of live, and reduce health care costs and utilization (9). Conversely, delays in cancer diagnosis are associated with cancer progression from early stage to late stage, untreatable, disease (10). Thus, the lack of efficient early diagnosis for many types of cancer epitomizes an unmet and urgent medical need.
The identification and implementation of early diagnostic measures has improved outcomes for many cancers, including cancer of the colon (11), lung (12), breast (13), cervical (14) and prostate (15). Conversely, absence of early diagnosis worsens outcomes. Studies showed that a 3-month delay in cancer diagnosis due to the COVID-19 pandemic led to an overall 18% reduction in net survival (10), with breast (22%), lung (20%), ovarian and cervical (50%) and colorectal and stomach (67%) cancers showing the greatest negative impact. The Centers for Disease Control and Prevention supports screening for breast, cervical, colorectal and lung cancers.
However, in cancers which do not have accurate early diagnostic tests [e.g. ovarian and pancreatic (11–14, 16–18)], patient survival rates have remained persistently low with five-year survival of only 50% and 12.5% (9) in spite of introduction of new treatment regimens. Currently available methods for screening of ovarian, pancreatic, prostate, testicular, and thyroid have not reduced cancer deaths. Hypothetical modeling of improved screening and early detection would yield an anticipated potential reduction of five-year mortality of 26-39% (16).
2 Optimal features for cancer biomarkers
An optimal cancer biomarker should be etiological to disease mechanisms, stable, invariant, and with uniform expression in most/all cancer nodules within a patient, should not downregulate in cancer cells, or in the cancer cells that remain or that recur following therapy, and should be accessible or measurable in a patient using non-invasive standard procedures such as liquid biopsy (e.g., a blood sample). Unfortunately, cancer biomarkers that meet all these features are very rare.
2.1 Etiological versus surrogate biomarkers
Biomarkers are defined by the Food and Drug Administration (FDA) as characteristics of the body that you can measure that are a direct measure of how a patient feels, functions, or survives (19). A biomarker is diagnostic of a disease when it is detectable in disease but is low/absent or is not functionally relevant in a healthy cell or healthy individual.
Biomarkers can be surrogate or etiological to the disease. The presence of a surrogate marker may be associated with the presence of a disease, and this is an indirect form of measuring a disease that can lead to poor sensitivity and/or selectivity and unacceptable false positives or false negatives. Thus, surrogate biomarkers are less desirable for diagnostic purposes.
In contrast, etiological biomarkers have a function that is directly relevant or causative of disease or disease progression, and therefore afford a clinically meaningful endpoint. Examples of etiological markers include overexpressed or mutant ERBB2/HER2 (20) in breast cancer, mutant EGFR in lung cancer (21), and mutant NRAS or BRAF in melanoma (22). Etiological biomarkers are expected to be expressed in a stable manner, because a cancer cell cannot readily eliminate or downregulate it without suffering a disadvantage (e.g. loss of the function that the marker provides) although downregulation of an etiological marker may be compensated by mutations or alternative pathways. Moreover, etiological biomarkers are expected to be expressed uniformly within a patient, even though cancer cells are phenotypically and genetically heterogeneous. Paradoxically, however, etiological protein biomarkers such as ERBB2/HER2, mutant EGFR, mutant BRAF and many others are expressed in a heterogeneous manner and often do downregulate expression leading to drug resistance.
2.2 Tissue biopsy versus liquid biopsy
Targets that are exclusively cell-associated (intracellular or membrane-bound in cancer cells) are available for study only through tissue biopsy, which may be invasive and require specialized equipment and procedures. Tissue biopsies are restricted to small sample size, offer a window limited to the tissues collected, and yield limited information regarding genetic heterogeneity within the primary tumor or the metastasized secondary tumors.
However, many cellular targets may also be found in soluble form if they are shed or secreted, or because of a cancer-associated metabolic or physiological process, or after they are proteolytically cleaved, or if they are released by the cancer cell in the form of exosomes or extracellular vesicles (EVs). These soluble markers are available for study using a liquid biopsy such as in blood, urine, tears, saliva, or cerebrospinal (CSF) fluids. Liquid biopsy sample collection is less invasive and more convenient, can be repeated over time, and is independent of tumor location, hence the diagnostic power and coverage of this method is very high (23, 24). Liquid biopsies are emerging as the fastest growing diagnostic tool for cancer and inflammation. Examples include quantification of circulating tumor DNA (ctDNA) (25), RNA, cell-free DNA (cfDNA) (26), DNA methylation and fragmentation (27), and targeted proteomics as approaches for tissue-agnostic biomarkers. Emerging pan-cancer tests are reported to detect up to 3 or 4 tumor types (based on nucleic acids mutations or methylation states), but fall below a threshold diagnostic coverage of over 50% of the patients in the top 30 tumor types (26).
As the FDA issued the first tissue-agnostic (or biomarker-driven) cancer drug approval (28), we anticipate that tissue-agnostic etiological biomarkers will become more interesting clinically and commercially. In this review we put forth the concept that TMGs are biomarkers that meet all the desirable characteristics described above: they are etiological to disease mechanisms, stable, invariant, and with uniform expression in most/all cancer nodules within a patient, do not downregulate following therapy, and are accessible in a liquid biopsy. Despite these favorable characteristics, TMGs remain under-exploited for diagnostics.
2.3 Traditional classes of cancer biomarkers. Proteins and nucleic acids
Cancer biomarkers have traditionally focused on measurements of abnormal proteins that are expressed de novo, or proteins that are over-expressed, mutated, or dysregulated; and on measurements of abnormal nucleic acids (DNA, mutant DNA, mRNA, miRNA), their methylation state, or nuclear structure. Approaches for discovery of novel targets or markers include proteomics using Mass Spectrometry (29) and genomics (30) through Next Generation Sequencing (NGS) and PCR (31), whole genome sequencing, or sequencing mRNA transcripts (32), and metabolomics (33). These “omics” techniques can be applied to tissue biopsies and to liquid biopsies, and have advanced the field by discovering many new biomarkers. The vast array of data obtained is refined qualitatively by incorporating artificial intelligence and bioinformatic platforms to the analysis, to develop potential diagnostic tools.
Most of the FDA approved cancer biomarkers are single glycosylated proteins derived from serum (34). These include Cancer Antigen 125 (CA125) for ovarian cancer (17, 35), cancer antigen 19-9 (CA 19-9) for pancreatic cancer (36), cancer antigen 15-3 (CA 15-3) for breast cancer (37), prostate-specific antigen (PSA) for prostate cancer (15), and carcinoembryonic antigen (CEA) increases in colorectal, bladder, breast, pancreatic and lung cancer (38). These biomarkers are especially useful for monitoring disease in patients who have already been diagnosed, and in practice have had some value as diagnostics.
However, while many potential biomarkers are reported annually through mining of “omics” data, the transition from discovery to actual validation is relatively slow. Moreover, some of these approaches are useful in research but are expensive, require a specialized or centralized facility, and are difficult to translate commercially to provide a qualified large-scale service to populations. These are significant barriers for reliable patient access. Proteins and nucleic acids are not examined in this paper, as they have been the topic of many recent reviews.
3 Novel class of cancer biomarkers. Gangliosides
3.1 The nature of gangliosides
Gangliosides are a family of >40 different sialic acid-containing glycosphingolipids, with an overall organization of two lipid tails with a glycan tree (39). Ganglioside nomenclature is based on the specific glycan sequence and connectivity which is structurally unique, and defines each ganglioside by name in all species (40).
The glycan moiety of the glycolipid is water soluble and extracellular (Figure 1). The sugar head is linked to a ceramide with two lipid tails embedded in the outer leaflet of membranes (41). In that location gangliosides regulate membrane fluidity (42), formation of membrane rafts and size (43–45) and within rafts promote the inclusion or exclusion of signaling proteins.
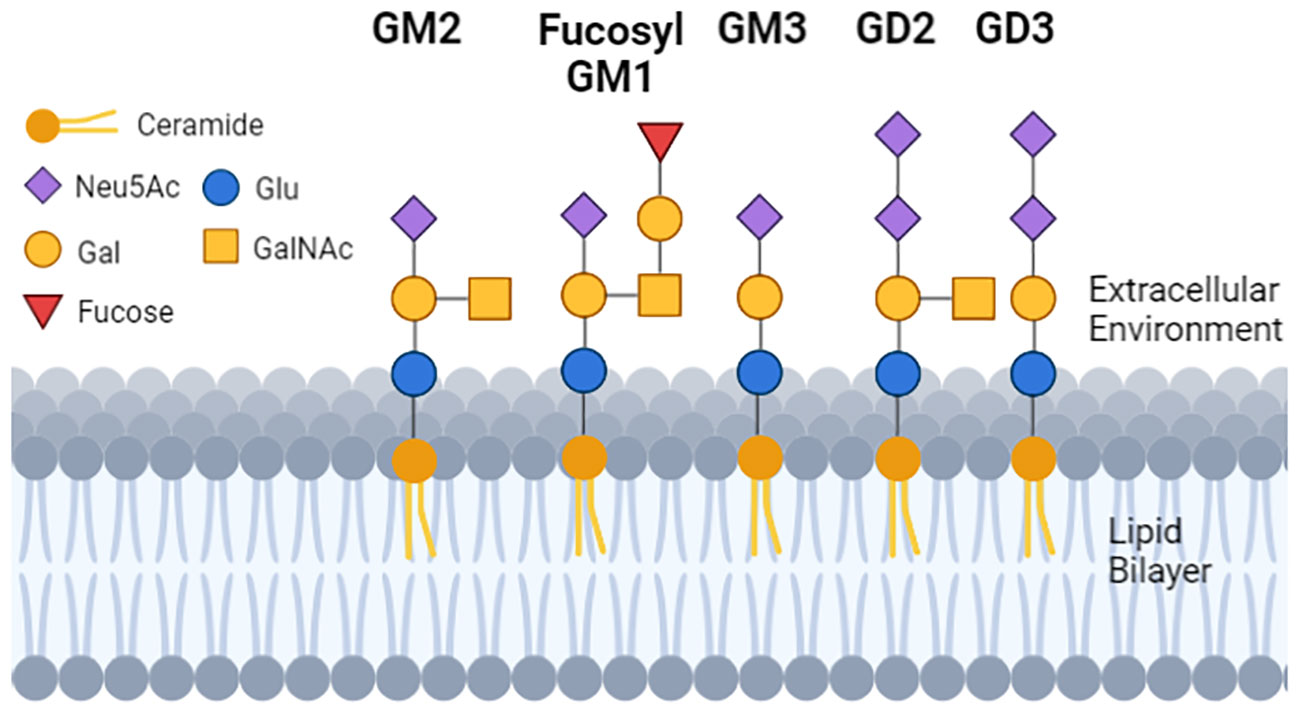
Figure 1 The structure and location of gangliosides in cells. Each geometric shape represents a specific type of sugar. For gangliosides that are on the cell surface, the glycan tree is exposed to the extracellular environment, and is linked to a ceramide (dark orange ball with two lipid tails embedded in the lipid bilayer). The sugar head of normal GM1 and the tumor GD3 differ by 2 sugars, and GD2 and GD3 differ from each other by 1 sugar. GM3 has a single N-Acetylneuraminic acid (mono sialylated) and can be a substrate to make GD3 (di-sialylated). GD3 can be a substrate to make GD2, so GD2 and GD3 can be (but not always) detected on the same cell.
Within a ganglioside, the lipid tails are variable in their physical features such as carbon length and saturation state. The biological significance of the lipid tail heterogeneity is unknown (46). It is likely that the lipid tail variability has biological significance; and we proposed to exploit this heterogeneity diagnostically (47). For example, a cancer may be diagnosed based on a specific ganglioside carbohydrate head as a marker, but additional data mining can be obtained by evaluation of that ganglioside’s lipid tail length (or a range of lengths) (47).
Gangliosides such as GM1 and GM3 are ubiquitous in most cells including healthy cells. Other gangliosides such as GD2 and GD3 are defined as tumor marker gangliosides (TMGs), as they are low/absent in post-embryonic normal cells (46, 48) but are expressed at high levels by tumor cells (39, 49–53). TMGs are similar to carcinoembryonic markers, as they expressed at high levels during embryonic development, and shortly after birth TMGs are mainly absent in healthy cells (other than in a subset of normal adult neurons). TMGs are re-expressed in many types of cancers (39, 50, 51, 53) and cancer stem cells (54, 55) and appear to be implicated in cancer recurrence and cancer resistance to therapy; making them attractive therapeutic targets (56, 57).
3.2 The biosynthesis and location of gangliosides
Synthesis of gangliosides begins in the endoplasmic reticulum and then continues in the Golgi apparatus, where sugars are added or removed by specific glycosyltransferases or glycanases (3, 52, 58). Gangliosides are transported to the outer leaflet of the cell membrane (46). TMGs in cancer cells have been reported to be shed (59) or secreted as micelles or in the form of extracellular vesicles, and may be incorporated within lipoprotein complexes (60–65). The TMGs can therefore be found at the tumor cell surface and also in three forms in bodily fluids: EVs, micelles, and lipoproteins (66). These released TMGs -in whatever form they are present in bodily fluids such as blood- may be potential high value biomarkers for diagnostics, as they are accessible in liquid biopsies (Figure 2).

Figure 2 Graphical summary of the locations and physical forms of TMGs. Tissue biopsies can be evaluated by immunohistochemistry when specific mAbs against a TMG are available. Liquid biopsies can be evaluated by ELISA after extraction of gangliosides from the patient sample (when specific mAbs against a TMG are available), or by assays that quantify the presence of sialic acids. The full set of TMGs (hereafter, the Cancer Gangliosome) can be evaluated by LC-MS without mAbs. Purification of extracellular vesicles or exosomes from liquid biopsies may be an additional purification step to remove interference and to increase sensitivity as the TMGs are present in this compartment.
3.3 Tumor marker gangliosides are present on extracellular vesicles
Cells release large volumes of vesicles from membranes or other organelles, including relatively large (30 to 150 nm) EVs that are shed via the budding of the plasma membrane. EVs are secreted into bodily fluids (e.g. CSF, blood, urine) and are relatively stable in those fluids until they are cleared by the liver or they become lodged in a specific organ. EVs represent an important mode of intercellular communication and a vehicle for transfer of membranes, proteins, mRNA and miRNA between cells (61). Cancer cells use EVs for immune modulation and to remodel the environment of a specific tissue to facilitate tumor cell metastasis (63, 67, 68) thus, EVs play an important role in oncogenesis (69–72).
TMGs are shed or are subject to vesicular release into circulation (43, 60, 64, 73–77). Since is documented that EVs and the cargo within EVs play an important role in oncogenesis (69–71, 78, 79) it is likely that TMGs present in EVs will likely have a role in oncogenesis as well, as discussed next.
3.4 Oncogenic-promoting biological roles of tumor marker gangliosides
Membrane-bound as well as soluble or shed TMGs play many biological functions in cell-cell recognition, cell adhesion, immune inhibition, and signal transduction (41–44, 46, 80). These features make TMGs etiological biomarkers because they are functionally implicated in cancer progression. TMGs provide tumors with advantages in growth/metastasis and immune evasion. The mechanisms described below link functional expression of TMGs with cancer progression.
First, TMGs on the cancer cell membrane activate signals promoting cellular growth [reviewed in (39, 51, 57)]. For example, expression of TMGs can lead to lowering of the threshold for activation of several wild type receptor tyrosine kinases (e.g. platelet-derived growth factor receptor, Trk receptor, and epidermal growth factor receptor) (81–91), and activation of soluble tyrosine kinases (such as p60Src, p56Lck) (81, 85, 92–95). Hence, oncogenic activation by TMGs is independent of growth factors.
This TMG-promoted dysregulated kinase activity is pro-oncogenic. Thus, TMGs may be etiological in tumors where mutations (or mutations of the activated kinases) are not clearly identified or are not a primary event. In addition, VEGF-mediated angiogenesis can be regulated in vivo by a change in TMG ratios. In this manner shed tumor gangliosides may promote tumor progression or facilitate metastasis by enhancing a leaky neo-vasculature and providing the necessary blood supply that enables tumors to extravasate and metastasize (96, 97). Pro-metastatic signals are further enhanced through modulation of adhesion proteins, regulation of membrane rafts, cell-cell and cell-matrix interactions (39, 57, 83, 85, 98–102).
Second, TMGs act functionally as Immune Checkpoint Inhibitors (ICI), to cause local and systemic immunosuppression, allowing the tumor to evade immune surveillance (103). Cancers inhibit or evade the immune system, by coopting a normal ICI process for immune regulation, examples comprising PD-1, CTLA4, and other proteins (104). Indeed, therapeutic strategies that target TMGs [such as anti-GD2 monoclonal antibodies (mAbs)] appear to reverse cancer resistance to therapies blocking an ICI protein PD-1 (1, 105). These data suggest that when a TMG is present, it may replace or potentiate the ICI function of PD-1 (52, 93, 106, 107). Circulating TMGs further suppress antigen presentation and immune activation systemically (i.e., throughout the body and not just in the local environment of the primary tumor) (108).
In sum, TMGs such as GD2 and GD3 suppress antigen presentation (87, 103, 109) and T cell immunity (108) locally and systemically, facilitating metastasis (68, 69, 71). This is advantageous to the tumor because systemic immunosuppression prevents secondary anti-tumor immune reactions against tumor neoantigens. Glycans containing sialic acids are a segment of the glycocode (110–112), and act as part of the glyco-immune-checkpoint, a new category of immune checkpoint blockade.
4 Expression of tumor marker gangliosides in cancer
Table 1 presents publications that have reported expression of specific TMGs in certain cancers.
Tumor Marker Gangliosides have been listed among the most valuable cancer biomarkers (53). TMGs are expressed homogeneously at very high levels in both primary and metastatic nodules, decorate a wide variety of cancers, and are expressed uniformly across nodules within an individual patient (41, 177). Furthermore, in contrast to some other biomarkers, TMGs do not mutate or downregulate, even after chemotherapy (41, 113, 178–181).
5 Historical barriers limiting exploitation of tumor marker gangliosides for cancer diagnosis
To date, there are no approved or standardized diagnostic tests that exploit TMGs, nor has any been published techniques to measure TMGs quantitatively in liquid biopsies. The human cancer phenotypic studies presented in Table 1 predominantly evaluated the expression of 1 or 2 TMGs per study using immunohistochemical (IHC) analysis of expression in tissue. Although IHC is a useful method for evaluating expression of novel markers, it is an inefficient and non-quantitative method. Therefore, there is a paucity of laboratory methods and very few specific antibodies fit for evaluating and quantifying TMGs, limiting their use to develop diagnostic methods (50). Thin Layer Chromatography (TLC) (85, 105, 156, 182) or lipid-associated sialic acids (LASAs) (183) have so far yielded only estimates of expression and often produced contradictory results. Even after 50 years of research worldwide, there are only a handful of monoclonal antibodies against TMGs (81, 146, 157, 184), and many are cross-reactive or poorly characterized (99, 102, 185). Since TMGs are glycolipids that can be generated via multiple biosynthetic pathways and enzymes (39, 51, 55, 147), monitoring mutations or mRNA levels does not appear to be feasible for robust diagnostics. These logistical challenges have limited the evaluation of TMGs for diagnostic purposes in cancer (4). It is noteworthy, however, that TMGs are validated targets for therapy and they represent an important source for developing cancer therapies [recently reviewed in (50, 186)].
5.1 Ovarian cancer
Ovarian cancer is the most fatal gynecological cancer, and lacks reliable diagnostics because there are no methods for early detection that could provide a clinical benefit (7). GD3 has been isolated from the polar lipid fraction of ovarian cancer-associated ascites, and identified to inhibit activation of natural killer (NK) cells. In-vivo, GD3 administration also dose-dependently inhibited natural killer T (NKT)-activation (135). These findings suggest that ovarian cancer tumors use GD3 to inhibit antitumor natural killer T-cell response as a potential mechanism for tumor immune evasion (80).
Recently, our group published an ELISA test that quantifies GD2 and GD3 in blood. We reported detection of 97% of early stage and late-stage ovarian cancer cases including those missed by the current standard clinical test CA125 (4). This was the first demonstration that TMGs can be accessed for quantitative diagnosis in a liquid biopsy. It was first used for ovarian cancer due to a significant unmet clinical need. Current work is expanding the test for diagnosis of other cancer such as melanoma and expanding the scope of the TMGs quantified to comprise the most relevant in Table 1.
5.2 Neuroblastoma
Neuroblastoma is the most common extracranial solid tumor in children. Neuroblastoma tumor tissues express GD2, which appears to be a marker of high-grade malignancy requiring more aggressive therapies. GD2 levels are significantly elevated in the sera of children with neuroblastoma compared healthy children and children with other cancers (59, 65). Furthermore, longitudinal evaluation of GD2 serum levels in patients showed a positive correlation with disease progression (113), indicating the potential for monitoring disease progression or the recurrence of neuroblastoma. However, GD2 levels were not quantified and further study is needed to determine the diagnostic validity. Evaluating combined expression of GD2 and GD3 in pediatric solid tumors, using radiolabeled monoclonal antibodies and tomography, has been suggested as a companion diagnostic to immunotherapy (162). One radiolabeled mAb is used for PET imaging to identify refractory neuroblastoma, but is not approved as standard of care (187).
5.3 Melanoma
GD3 is expressed on human melanoma cells and can modulate immune cell cytotoxicity (182) via siglec-7-dependent and -independent mechanisms (105, 156). Immunosuppressive function in melanoma was reported for GD2 and GD3 (80, 105, 156, 182). TMGs allow for more growth and motility and cellular invasion (99, 102, 157). GD3 is highly expressed in melanoma and regulates Src kinases and Yes kinases (81, 85, 157), likely through rafts, although specific mechanisms remain unidentified.
Given that the majority of human melanoma cases are positive for a TMG (mainly GD3 and GD2 have been evaluated) the possibility of using TMG markers for melanoma diagnosis or prognosis remains of high interest. Given the immunosuppressive role of some TMGs, which exhibit many of the features of immune checkpoint inhibition (ICI) such as PD1/PD1-L, it is also reasonable to hypothesize that TMGs may be at least partially involved in the known lack of response to ICI-blockade therapies in melanoma. This concept has been tested in neuroblastoma (188, 189). Evaluation in melanoma would provide with a diagnostic prediction of likely response to the standard of care ICI-blockade therapies, and this work is ongoing.
5.4 Bladder cancer
Bladder cancer is ranked as the fourth most common cancer in men, with occurrences four times greater than in women. High levels of GM3 have been observed to lower tumor cell motility and invasiveness and lower levels are able to increase cell motility and invasiveness (86). Therefore, in this circumstance, high levels of the specific GM3 ganglioside can be beneficial. Whether the presence of GM3 may related to lower presence of GD3 is an interesting hypothesis.
5.5 Bone cancer- osteosarcoma
GD2 is highly expressed in osteosarcoma tissues. In vitro, osteosarcoma cell lines generally expressed high levels of GD2 and GD3 (83, 164). GD3- and GD2-positive cells showed the most malignant properties. Therefore, GD2 may have a stronger phenotype than GD3 alone. Patient samples obtained during recurrence of cancer showed a higher intensity of GD2 staining compared to samples obtained during the initial biopsy. This indicates a notable increase in GD2 levels from the time of initial diagnosis to the recurrence of the disease (164).
5.6 Glioma and glioblastoma
A high percentage of glioma and glioblastoma tissues express GD2 and/or GD3. In gliomas, a large proportion express 9-O-acetyl GD3, or GD3 with an additional acetyl group on the terminal sialic acid, and the ratio of GD3 to 9-O-acetyl GD3 may be associated with enhanced tumor survival (147) or pro-tumorigenic events in glioblastoma (146).
5.7 Breast cancer
Several studies have suggested that GD2 and GD3 are involved in the development of various breast tumor types but the functional relationships between ganglioside expression and cancer development are not fully understood. GD3 is upregulated in approximately half of all invasive ductal breast carcinoma cases (127). One study showed that GD2 was highly expressed in a cohort of aggressive breast cancer subtypes, such as triple-negative and metaplastic (126), but confirmatory studies are needed. GD2 was also identified as a cancer stem cell-specific marker from human breast cancer cell lines and patient samples (54), and GD3 was associated with activated EGFR signaling in both breast CSCs and breast cancer cell lines (127).
GM3 was reported in the serum of breast cancer patients (190) and GD2/GD2 synthase are reported in breast cancer stem cells (54, 127, 191, 192) that are often responsible for recurrence of the disease. The value of TMGs for breast cancer diagnosis may be important, but it is undefined at this stage and requires further research.
5.8 Head and neck cancer
Head and neck squamous cell carcinoma (HNSCC) is the sixth leading cancer in the world (7). Expression of the glycolipid globotriaosylceramide 3 (GB3) was positively correlated with progression of head and neck cancer (HNC). During malignant transformation of HNC cells, changes in GB3 expression suggests that this marker could be used to identify HNSCC early on (74).
5.9 Lung cancer/small cell lung cancer
In the United States, lung cancer is the third most common cancer, with the annual number of new cases steady over the past 20 years. Expression of glycosyltransferase genes important for biosynthesis of gangliosides is elevated in lung cancer above normal bronchial cells (87). Both non-small cell lung cancer (NSCLC) and small cell lung cancer (SCLC) express mainly GM2 and GM1, whereas only SCLCs express b-series gangliosides GD2, GD1b, and GT1b as well as the expected upregulation of the GD3 synthase gene required for biosynthesis (87, 102), and express fucosylated GM1 (Fuc-GM1) (56). Expression of GD3 synthase or GD2 synthase in SCLCs increased growth rates and invasiveness, and anti-GD2 antibodies induced apoptosis, indicating that GD2 has a functional role in malignant growth.
5.10 Soft tissue sarcomas
GD2 and GD3 expression are present on human soft tissue sarcomas, as assessed in IHC performed on 56 tissue samples, in which 93% of tumors expressed GD2 while 88% expressed GD3 (160). Specifically, GD2 is widely expressed among neuroectodermal tumors as well as adult sarcomas using IHC (168). The intensity of expression and localization varied among sarcomas of different histologic types (160).
5.11 Other cancers
The ganglioside GM2 is highly expressed in pancreatic ductal adenocarcinoma (PDAC) and Cholangiocarcinoma (193). GM2-positive PDAC cells exhibited higher growth rates and invasion and were present in three-dimensional cultures, including cancer stem cell-like cells. GM2 expression was associated with aggressive PDAC characteristics, indicating its potential as a diagnostic target for PDAC. Cholangiocarcinoma, a bile duct epithelium malignancy prevalent in Southeast Asia and a significant health concern due to poor prognosis (194). This may be useful since early detection is challenging as the current serum marker (CA19-9) is insufficient due to low sensitivity/specificity (195).
6 Looking to the future of diagnosis
The data above provides evidence that GD2/GD3 and other TMGs may be present in different cancers at different ratios and with some degree of cancer specificity. Evaluating GD2/GD3 expression has been suggested as a companion diagnostic for utilizing immunotherapy targeting gangliosides for therapy (162). Other studies examined other solid tumors osteosarcoma, rhabdomyosarcoma, Ewing family of tumors, desmoplastic small round cell tumors, and melanoma (117, 160, 168, 184). Further, there were higher GD3 expression levels than GD2 among the other tumors analyzed. Recently, our group quantified GD2 and GD3 in blood as diagnostic of all stages and all forms of epithelial ovarian cancer with detection of 97% of early stage and late-stage ovarian cancer cases including the 40% of cases missed by the current test CA125 (4).
As mentioned above, TMGs are shed into the serum and thus may be utilized for diagnostics using liquid biopsies. However, it is noteworthy expression of one ganglioside does not dictate the fate of having a cancer, but a collection of tumor-gangliosides and their proportions or ratios may have important implications in terms of providing functional advantages for cancer growth or aggressiveness (50) and may be exploited for therapy or for diagnostics. Overall, the data suggests a strong rationale for utilizing a combination of TMGs as biomarkers for early stage detection of cancer and the varying ganglioside patterns/ratios among cancers and cancer types. This is discussed in the next section.
6.1 TMGs as pan-cancer diagnostic markers?
As indicated in Table 1, each tumor may express a limited number of TMGs, but TMGs are markers of a large number of tumor types. This intriguing finding prompted the question of whether it is possible to explore TMGs as a pan-cancer diagnostic. Pan-cancer detection may be a tool that can lead to early suspicion, and then to a genuine diagnosis using tests that detect one tumor-at-a-time. Cancer suspicion and eventual diagnosis often results from patients presenting to a physician with symptoms. When patients present with generic or confounding symptoms (like pelvic pain for ovarian and gastrointestinal cancers) a bona fide diagnosis is more difficult (26, 196). Moreover, there are asymptomatic individuals that do not visit a health care professional. We believe that an affordable pan-cancer diagnostic tool may be of high utility for these populations as well as potentially as a routine screening tool leading to earlier diagnosis.
Many companies are pursuing the goal of attempting to commercialize pan-cancer screens. Emerging tests have been reported to detect up to 3 or 4 tumor types based on nucleic acids mutations or methylation states. So far, tests fall below the expected threshold diagnostic coverage of over 50% of the patients in the top 30 tumor types. Moreover, the efficacy of these methods at detection of early stage cancers (when curative therapy is most likely) is low (26). Current studies utilizing circulating tumor DNA (ctDNA), and circulating miRNA are investigating the rigor and reproducibility of attempts to demonstrate high sensitivity and specificity from innovative diagnostic assays for early stage cancer detection, i.e. CancerSEEK (26). CancerSEEK detected cancer with a sensitivity of 69 to 98% (depending on cancer type) and 99% specificity from a study of 1000 patients previously diagnosed with cancer and 850 healthy control individuals as per their criteria. Although the specificity is high, the sensitivity has a 29% difference from its minimum to maximum percentage detected, suggesting a need for improvement prior to fully distributing and utilizing CancerSEEK among average- to high-risk populations. For circulating miRNA biomarker screening, studies are costly and reported studies are of small sample sizes leading to issues of reproducibility (197). Moreover, unfortunately, both ctDNA and miRNA are unstable and require specialized handling that is not feasible for many healthcare providers (198).
We note that there is still wide debate in the field as to the real clinical usefulness of a pan-cancer diagnostic tool; and the outcome measures, the analytical endpoints, and the preponderance of late stage cancer samples analyzed during the development of these techniques have been criticized (4, 199–202). That being said, identification of pan-cancer biomarkers that have potential to overcome the challenges will resolve an urgent and unmet need (203). This is an evolving field and implementation of pan-cancer tests or screening tools are still at a relatively early phase of development and it remains to be tested in the marketplace (e.g. can they be commercialized successfully over a long period of time).
Given that a large panel of TMGs are accessible in liquid biopsies, and they have ideal features for biomarkers, innovative methods to quantify all TMGs in liquid biopsies may facilitate a pan-cancer method or diagnostic tool. Cancer specificity may arise from the relative ratios of TMGs in a patient. We refer to this diagnostic ratio of TMGs as the Cancer Gangliosome, which may be a novel family for developing a pan-cancer diagnostic. In addition to TMG ratios, several features of the Cancer Gangliosome may be analyzed for developing diagnostic biomarkers. As stated above, the ganglioside lipids are variable in length, saturation, and oxidation states (15). The relevance of ganglioside lipid tail heterogeneity (or changes thereof in disease states) is of interest given that that differences or changes in lipid tail may have biological significance, for example affording different properties to signaling rafts or to the fate of exosomes. Hence, we proposed exploiting lipid heterogeneity diagnostically (47), as it is possible to exploit not only a specific carbohydrate as a diagnostic marker (e.g. GD2) but also to exploit the specific lipid tail length of that GD2 carbohydrate species to enable diagnostic specificity. As quantification of GD2 may be diagnostic of melanoma or glioblastoma or ovarian cancer (see Table 1), quantification of GD2 together with identification of a specific lipid tail length (or range thereof) or other physical features of the tail (such as oxidation or saturation state) can discriminate diagnostically between melanoma or glioblastoma or ovarian cancer.
7 Conclusions
TMG glycan trees provide for stable, invariant, and non-mutating cell surface markers, their expression is uniform in cancer nodules (41), does not downregulate in the surviving tumor cells after chemotherapy, and the biomarkers are functionally etiological to cancer (52, 163, 179, 204). The glycan markers are detectable in tissues (50, 205) and also in liquid biopsies which provides advantages for sampling (4). In addition, the TMG lipid tail lengths or other physical features may provide for additional parameters to develop diagnostic tools (47).
Early stage detection of cancers is essential to improving patient survival and quality of life. Exploiting a new family of tumor gangliosides is a promising addition to the menu of markers for diagnosing early stage cancers using a simple non-invasive blood test. In patients presenting to a healthcare provider with symptoms, an analysis of relevant TMGs can be used to aid diagnosis. Future work should be focused on the quantification of glycolipids and ganglioside presence (or relative ratios) in blood for potentially diagnosing patients at early and late-stages of cancer.
Here we propose that the Cancer Biomarker Glycocode family and the Cancer Gangliosome as a class which is poorly exploited in diagnosis. These markers are complementary to the more commonly exploited families of protein or nucleic acid markers, and can add to the repertoire of available markers, leading to innovation in cancer diagnosis and screening. Having available a diversity of biomarkers will help to alleviate the serious unmet clinical diagnostic needs, potentially accelerate treatments, lower morbidity, lower mortality, and reduced healthcare costs.
Author contributions
AN: Conceptualization, Writing – original draft, Writing – review & editing. SY: Writing – original draft. AJ: Conceptualization, Writing – review & editing. HS: Conceptualization, Writing – review & editing, Funding acquisition, Writing – original draft.
Funding
The author(s) declare financial support was received for the research, authorship, and/or publication of this article. HS was funded by the Reseau Quebecois pour le Medicines (RQRM) and the Canadian Institutes of Health Research (CIHR, 202104PQQ).
Acknowledgments
Mysha Ibnat proofread and helped edit the references of this manuscript.
Conflict of interest
Author HS discloses patent filings protecting claims of intellectual property, under License to AOA Dx where author AJ works and where HS serves as consultant.
The remaining authors declare that the research was conducted in the absence of any commercial or financial relationships that could be construed as a potential conflict of interest.
The author(s) declared that they were an editorial board member of Frontiers, at the time of submission. This had no impact on the peer review process and the final decision.
Publisher’s note
All claims expressed in this article are solely those of the authors and do not necessarily represent those of their affiliated organizations, or those of the publisher, the editors and the reviewers. Any product that may be evaluated in this article, or claim that may be made by its manufacturer, is not guaranteed or endorsed by the publisher.
References
1. Cao S, Hu X, Ren S, Wang Y, Shao Y, Wu K, et al. The biological role and immunotherapy of gangliosides and GD3 synthase in cancers. Front Cell Dev Biol (2023) 11:1076862. doi: 10.3389/fcell.2023.1076862
2. Liu J, Zheng X, Pang X, Li L, Wang J, Yang C, et al. Ganglioside GD3 synthase (GD3S), a novel cancer drug target. Acta Pharm Sin B (2018) 8:713–20. doi: 10.1016/j.apsb.2018.07.009
3. Groux-Degroote S, Guerardel Y, Delannoy P. Gangliosides: structures, biosynthesis, analysis, and roles in cancer. Chembiochem (2017) 18:1146–54. doi: 10.1002/cbic.201600705
4. Galan A, Papaluca A, Nejatie A, Matanes E, Brahimi F, Tong W, et al. GD2 and GD3 gangliosides as diagnostic biomarkers for all stages and subtypes of epithelial ovarian cancer. Front Oncol (2023) 13:1134763. doi: 10.3389/fonc.2023.1134763
5. Cerliani JP, Blidner AG, Toscano MA, Croci DO, Rabinovich GA. Translating the 'Sugar Code' into immune and vascular signaling programs. Trends Biochem Sci (2017) 42:255–73. doi: 10.1016/j.tibs.2016.11.003
6. Cagnoni AJ, Perez Saez JM, Rabinovich GA, Marino KV. Turning-off signaling by Siglecs, Selectins, and Galectins: chemical inhibition of glycan-dependent interactions in cancer. Front Oncol (2016) 6:109. doi: 10.3389/fonc.2016.00109
7. Siegel RL, Miller KD, Wagle NS, Jemal A. Cancer statistics, 2023. CA Cancer J Clin (2023) 73:17–48. doi: 10.3322/caac.21763
8. Koo MM, Swann R, McPhail S, Abel GA, Elliss-Brookes L, Rubin GP, et al. Presenting symptoms of cancer and stage at diagnosis: evidence from a cross-sectional, population-based study. Lancet Oncol (2020) 21:73–9. doi: 10.1016/S1470-2045(19)30595-9
9. WHO- NCD Management-Screening, Diagnosis and Treatment Team. Guide to Cancer: Early Diagnosis. World Health Organization, Elsevier (ScienceDirect) (2017).
10. Sud A, Torr B, Jones ME, Broggio J, Scott S, Loveday C, et al. Effect of delays in the 2-week-wait cancer referral pathway during the COVID-19 pandemic on cancer survival in the UK: a modelling study. Lancet Oncol (2020) 21:1035–44. doi: 10.1016/S1470-2045(20)30392-2
11. Doubeni CA, Corley DA, Quinn VP, Jensen CD, Zauber AG, Goodman M, et al. Effectiveness of screening colonoscopy in reducing the risk of death from right and left colon cancer: a large community-based study. Gut (2018) 67:291–8. doi: 10.1136/gutjnl-2016-312712
12. Squires BS, Levitin R, Grills IS. The US preventive services task force recommendation on lung cancer screening. JAMA (2021) 326:440–1. doi: 10.1001/jama.2021.8240
13. Nelson HD, Fu R, Cantor A, Pappas M, Daeges M, Humphrey L. Effectiveness of breast cancer screening: systematic review and meta-analysis to update the 2009 U.S. preventive services task force recommendation. Ann Intern Med (2016) 164:244–55. doi: 10.7326/M15-0969
14. U.S.P.S.T. Force, Curry SJ, Krist AH, Owens DK, Barry MJ, Caughey AB, et al. Screening for cervical cancer: US preventive services task force recommendation statement. JAMA (2018) 320:674–86. doi: 10.1001/jama.2018.10897
15. Fenton JJ, Weyrich MS, Durbin S, Liu Y, Bang H, Melnikow J. Prostate-specific antigen-based screening for prostate cancer: evidence report and systematic review for the US preventive services task force. JAMA (2018) 319:1914–31. doi: 10.1001/jama.2018.3712
16. Hubbell E, Clarke CA, Aravanis AM, Berg CD. Modeled reductions in late-stage cancer with a multi-cancer early detection test. Cancer Epidemiol Biomarkers Prev (2021) 30:460–8. doi: 10.1158/1055-9965.EPI-20-1134
17. Jacobs IJ, Menon U, Ryan A, Gentry-Maharaj A, Burnell M, Kalsi JK, et al. Ovarian cancer screening and mortality in the UK Collaborative Trial of Ovarian Cancer Screening (UKCTOCS): a randomised controlled trial. Lancet (2016) 387:945–56. doi: 10.1016/S0140-6736(15)01224-6
18. Buys SS, Partridge E, Black A, Johnson CC, Lamerato L, Isaacs C, et al. Effect of screening on ovarian cancer mortality: the Prostate, Lung, Colorectal and Ovarian (PLCO) Cancer Screening Randomized Controlled Trial. JAMA (2011) 305:2295–303. doi: 10.1001/jama.2011.766
19. Bravo-Merodio L, Acharjee A, Russ D, Bisht V, Williams JA, Tsaprouni LG, et al. Translational biomarkers in the era of precision medicine. Adv Clin Chem (2021) 102:191–232. doi: 10.1016/bs.acc.2020.08.002
20. Loibl S, Poortmans P, Morrow M, Denkert C, Curigliano G. Breast cancer. Lancet (2021) 397:1750–69. doi: 10.1016/S0140-6736(20)32381-3
21. da Cunha Santos G, Shepherd FA, Tsao MS. EGFR mutations and lung cancer. Annu Rev Pathol (2011) 6:49–69. doi: 10.1146/annurev-pathol-011110-130206
22. Platz A, Egyhazi S, Ringborg U, Hansson J. Human cutaneous melanoma; a review of NRAS and BRAF mutation frequencies in relation to histogenetic subclass and body site. Mol Oncol (2008) 1:395–405. doi: 10.1016/j.molonc.2007.12.003
23. Lone SN, Nisar S, Masoodi T, Singh M, Rizwan A, Hashem S, et al. Liquid biopsy: a step closer to transform diagnosis, prognosis and future of cancer treatments. Mol Cancer (2022) 21:79. doi: 10.1186/s12943-022-01543-7
24. Connal S, Cameron JM, Sala A, Brennan PM, Palmer DS, Palmer JD, et al. Liquid biopsies: the future of cancer early detection. J Transl Med (2023) 21:118. doi: 10.1186/s12967-023-03960-8
25. Sivapalan L, Murray JC, Canzoniero JV, Landon B, Jackson J, Scott S, et al. Liquid biopsy approaches to capture tumor evolution and clinical outcomes during cancer immunotherapy. J Immunother Cancer (2023) 11. doi: 10.1136/jitc-2022-005924
26. Cohen JD, Li L, Wang Y, Thoburn C, Afsari B, Danilova L, et al. Detection and localization of surgically resectable cancers with a multi-analyte blood test. Science (2018) 359:926–30. doi: 10.1126/science.aar3247
27. Mathios D, Johansen JS, Cristiano S, Medina JE, Phallen J, Larsen KR, et al. Detection and characterization of lung cancer using cell-free DNA fragmentomes. Nat Commun (2021) 12:5060. doi: 10.1038/s41467-021-24994-w
28. Poh A. First tissue-agnostic drug approval issued. Cancer Discovery (2017) 7:656. doi: 10.1158/2159-8290.CD-NB2017-078
29. Marimpietri D, Petretto A, Raffaghello L, Pezzolo A, Gagliani C, Tacchetti C, et al. Proteome profiling of neuroblastoma-derived exosomes reveal the expression of proteins potentially involved in tumor progression. PloS One (2013) 8:e75054. doi: 10.1371/journal.pone.0075054
30. Ahmad E, Ali A, Nimisha A, Ahmed F, Mehdi Dar G, Mohan Singh A, et al. Molecular approaches in cancer. Clin Chim Acta (2022) 537:60–73. doi: 10.1016/j.cca.2022.09.027
31. Zhao YY, Cheng XL, Lin RC. Lipidomics applications for discovering biomarkers of diseases in clinical chemistry. Int Rev Cell Mol Biol (2014) 313:1–26. doi: 10.1016/B978-0-12-800177-6.00001-3
32. Ouyang Y, Lu W, Wang Y, Wang B, Li F, Li X, et al. Integrated analysis of mRNA and extrachromosomal circular DNA profiles to identify the potential mRNA biomarkers in breast cancer. Gene (2023) 857:147174. doi: 10.1016/j.gene.2023.147174
33. Calapre L, Giardina T, Beasley AB, Reid AL, Stewart C, Amanuel B, et al. Identification of TP53 mutations in circulating tumour DNA in high grade serous ovarian carcinoma using next generation sequencing technologies. Sci Rep (2023) 13:278. doi: 10.1038/s41598-023-27445-2
34. Ludwig JA, Weinstein JN. Biomarkers in cancer staging, prognosis and treatment selection. Nat Rev Cancer (2005) 5:845–56. doi: 10.1038/nrc1739
35. Jacobs I, Bast RC Jr. The CA 125 tumour-associated antigen: a review of the literature. Hum Reprod (1989) 4:1–12. doi: 10.1093/oxfordjournals.humrep.a136832
36. Zhao B, Zhao B, Chen F. Diagnostic value of serum carbohydrate antigen 19-9 in pancreatic cancer: a systematic review and meta-analysis. Eur J Gastroenterol Hepatol (2022) 34:891–904. doi: 10.1097/MEG.0000000000002415
37. Duffy MJ, Evoy D, McDermott EW. CA 15-3: uses and limitation as a biomarker for breast cancer. Clin Chim Acta (2010) 411:1869–74. doi: 10.1016/j.cca.2010.08.039
38. Hammarstrom S. The carcinoembryonic antigen (CEA) family: structures, suggested functions and expression in normal and Malignant tissues. Semin Cancer Biol (1999) 9:67–81. doi: 10.1006/scbi.1998.0119
39. Gagnon M, Saragovi HU. Gangliosides: therapeutic agents or therapeutic targets? Expert Opin Ther Patents (2002) 12:1215–23. doi: 10.1517/13543776.12.8.1215
40. Svennerholm L. CHROMATOGRAPHlC SEPARATION OF HUMAN BRAIN GANGLIOSIDES*. J Neurochem (1963) 10:613–23. doi: 10.1111/j.1471-4159.1963.tb08933.x
41. Doronin II, Vishnyakova PA, Kholodenko IV, Ponomarev ED, Ryazantsev DY, Molotkovskaya IM, et al. Ganglioside GD2 in reception and transduction of cell death signal in tumor cells. BMC Cancer (2014) 14:295. doi: 10.1186/1471-2407-14-295
42. Chiantia S, Kahya N, Schwille P. Raft domain reorganization driven by short- and long-chain ceramide: a combined AFM and FCS study. Langmuir (2007) 23:7659–65. doi: 10.1021/la7010919
43. Logozzi M, De Milito A, Lugini L, Borghi M, Calabro L, Spada M, et al. High levels of exosomes expressing CD63 and caveolin-1 in plasma of melanoma patients. PloS One (2009) 4:e5219. doi: 10.1371/journal.pone.0005219
44. Khatun Z, Bhat A, Sharma S, Sharma A. Elucidating diversity of exosomes: biophysical and molecular characterization methods. Nanomed (Lond) (2016) 11:2359–77. doi: 10.2217/nnm-2016-0192
45. Lobb RJ, Becker M, Wen SW, Wong CS, Wiegmans AP, Leimgruber A, et al. Optimized exosome isolation protocol for cell culture supernatant and human plasma. J Extracell Vesicles (2015) 4:27031. doi: 10.3402/jev.v4.27031
46. Yu RK, Tsai YT, Ariga T, Yanagisawa M. Structures, biosynthesis, and functions of gangliosides–an overview. J Oleo Sci (2011) 60:537–44. doi: 10.5650/jos.60.537
47. Saragovi HU. MASS SPECTROMETRY METHODS FOR CANCER DIAGNOSIS. In: USPTO. USA: AOA Dx Inc. (2022).
48. Tsuchida T, Ravindranath MH, Saxton RE, Irie RF. Gangliosides of human melanoma: altered expression in vivo and in vitro. Cancer Res (1987) 47:1278–81.
49. Mitra I, Snyder CM, Zhou X, Campos MI, Alley WR Jr., Novotny MV, et al. Structural characterization of serum N-glycans by methylamidation, fluorescent labeling, and analysis by microchip electrophoresis. Anal Chem (2016) 88:8965–71. doi: 10.1021/acs.analchem.6b00882
50. Bartish M, Del Rincon SV, Rudd CE, Saragovi HU. Aiming for the sweet spot: Glyco-immune checkpoints and gammadelta T cells in targeted immunotherapy. Front Immunol (2020) 11:564499. doi: 10.3389/fimmu.2020.564499
51. Daniotti JL, Vilcaes AA, Torres Demichelis V, Ruggiero FM, Rodriguez-Walker M. Glycosylation of glycolipids in cancer: basis for development of novel therapeutic approaches. Front Oncol (2013) 3:306. doi: 10.3389/fonc.2013.00306
52. Zhang X, Kiechle FL. Review: Glycosphingolipids in health and disease. Ann Clin Lab Sci (2004) 34:3–13.
53. Cheever MA, Allison JP, Ferris AS, Finn OJ, Hastings BM, Hecht TT, et al. The prioritization of cancer antigens: a national cancer institute pilot project for the acceleration of translational research. Clin Cancer Res (2009) 15:5323–37. doi: 10.1158/1078-0432.CCR-09-0737
54. Battula VL, Shi Y, Evans KW, Wang RY, Spaeth EL, Jacamo RO, et al. Ganglioside GD2 identifies breast cancer stem cells and promotes tumorigenesis. J Clin Invest (2012) 122:2066–78. doi: 10.1172/JCI59735
55. Sarkar TR, Battula VL, Werden SJ, Vijay GV, Ramirez-Pena EQ, Taube JH, et al. GD3 synthase regulates epithelial-mesenchymal transition and metastasis in breast cancer. Oncogene (2015) 34:2958–67. doi: 10.1038/onc.2014.245
56. Heimburg-Molinaro J, Lum M, Vijay G, Jain M, Almogren A, Rittenhouse-Olson K. Cancer vaccines and carbohydrate epitopes. Vaccine (2011) 29:8802–26. doi: 10.1016/j.vaccine.2011.09.009
57. Regina Todeschini A, Hakomori SI. Functional role of glycosphingolipids and gangliosides in control of cell adhesion, motility, and growth, through glycosynaptic microdomains. Biochim Biophys Acta (2008) 1780:421–33. doi: 10.1016/j.bbagen.2007.10.008
58. Breiden B, Sandhoff K. Ganglioside metabolism and its inherited diseases. Methods Mol Biol (2018) 1804:97–141. doi: 10.1007/978-1-4939-8552-4_5
59. Ladisch S, Wu ZL, Feig S, Ulsh L, Schwartz E, Floutsis G, et al. Shedding of GD2 ganglioside by human neuroblastoma. Int J Cancer (1987) 39:73–6. doi: 10.1002/ijc.2910390113
60. Haber DA, Velculescu VE. Blood-based analyses of cancer: circulating tumor cells and circulating tumor DNA. Cancer Discovery (2014) 4:650–61. doi: 10.1158/2159-8290.CD-13-1014
61. Abels ER, Breakefield XO. Introduction to extracellular vesicles: biogenesis, RNA cargo selection, content, release, and uptake. Cell Mol Neurobiol (2016) 36:301–12. doi: 10.1007/s10571-016-0366-z
62. Raposo G, Stoorvogel W. Extracellular vesicles: exosomes, microvesicles, and friends. J Cell Biol (2013) 200:373–83. doi: 10.1083/jcb.201211138
63. Bobrie A, Colombo M, Raposo G, Thery C. Exosome secretion: molecular mechanisms and roles in immune responses. Traffic (2011) 12:1659–68. doi: 10.1111/j.1600-0854.2011.01225.x
64. Trajkovic K, Hsu C, Chiantia S, Rajendran L, Wenzel D, Wieland F, et al. Ceramide triggers budding of exosome vesicles into multivesicular endosomes. Science (2008) 319:1244–7. doi: 10.1126/science.1153124
65. Balis FM, Busch CM, Desai AV, Hibbitts E, Naranjo A, Bagatell R, et al. The ganglioside GD2 as a circulating tumor biomarker for neuroblastoma. Pediatr Blood Cancer (2020) 67:e28031. doi: 10.1002/pbc.28031
66. Kong Y, Li R, Ladisch S. Natural forms of shed tumor gangliosides. Biochim Biophys Acta (1998) 1394:43–56. doi: 10.1016/S0005-2760(98)00096-4
67. Ridder K, Sevko A, Heide J, Dams M, Rupp AK, Macas J, et al. Extracellular vesicle-mediated transfer of functional RNA in the tumor microenvironment. Oncoimmunology (2015) 4:e1008371. doi: 10.1080/2162402X.2015.1008371
68. Filipazzi P, Burdek M, Villa A, Rivoltini L, Huber V. Recent advances on the role of tumor exosomes in immunosuppression and disease progression. Semin Cancer Biol (2012) 22:342–9. doi: 10.1016/j.semcancer.2012.02.005
69. Hood JL, San RS, Wickline SA. Exosomes released by melanoma cells prepare sentinel lymph nodes for tumor metastasis. Cancer Res (2011) 71:3792–801. doi: 10.1158/0008-5472.CAN-10-4455
70. Luga V, Zhang L, Viloria-Petit AM, Ogunjimi AA, Inanlou MR, Chiu E, et al. Exosomes mediate stromal mobilization of autocrine Wnt-PCP signaling in breast cancer cell migration. Cell (2012) 151:1542–56. doi: 10.1016/j.cell.2012.11.024
71. Zomer A, Maynard C, Verweij FJ, Kamermans A, Schafer R, Beerling E, et al. In Vivo imaging reveals extracellular vesicle-mediated phenocopying of metastatic behavior. Cell (2015) 161:1046–57. doi: 10.1016/j.cell.2015.04.042
72. Peinado H, Alec kovic M, Lavotshkin S, Matei I, Costa-Silva B, Moreno-Bueno G, et al. Corrigendum: Melanoma exosomes educate bone marrow progenitor cells toward a pro-metastatic phenotype through MET. Nat Med (2016) 22:1502. doi: 10.1038/nm1216-1502b
73. Nazha B, Inal C, Owonikoko TK. Disialoganglioside GD2 expression in solid tumors and role as a target for cancer therapy. Front Oncol (2020) 10:1000. doi: 10.3389/fonc.2020.01000
74. Garcia-Hevia L, Munoz-Guerra D, Casafont I, Morales-Angulo C, Ovejero VJ, Lobo D, et al. Gb3/cd77 is a predictive marker and promising therapeutic target for head and neck cancer. Biomedicines (2022) 10. doi: 10.3390/biomedicines10040732
75. Cavdarli S, Groux-Degroote S, Delannoy P. Gangliosides: the double-edge sword of neuro-ectodermal derived tumors. Biomolecules (2019) 9. doi: 10.3390/biom9080311
76. Llorente A, Skotland T, Sylvanne T, Kauhanen D, Rog T, Orlowski A, et al. Molecular lipidomics of exosomes released by PC-3 prostate cancer cells. Biochim Biophys Acta (2013) 1831:1302–9. doi: 10.1016/j.bbalip.2013.04.011
77. Wubbolts R, Leckie RS, Veenhuizen PT, Schwarzmann G, Mobius W, Hoernschemeyer J, et al. Proteomic and biochemical analyses of human B cell-derived exosomes. Potential implications for their function and multivesicular body formation. J Biol Chem (2003) 278:10963–72. doi: 10.1074/jbc.M207550200
78. Peinado H, Aleckovic M, Lavotshkin S, Matei I, Costa-Silva B, Moreno-Bueno G, et al. Melanoma exosomes educate bone marrow progenitor cells toward a pro-metastatic phenotype through MET. Nat Med (2012) 18:883–91. doi: 10.1038/nm.2753
79. Garcia-Silva S, Benito-Martin A, Sanchez-Redondo S, Hernandez-Barranco A, Ximenez-Embun P, Nogues L, et al. Use of extracellular vesicles from lymphatic drainage as surrogate markers of melanoma progression and BRAF (V600E) mutation. J Exp Med (2019) 216:1061–70. doi: 10.1084/jem.20181522
80. Potapenko M, Shurin GV, de Leon J. Gangliosides as immunomodulators. Adv Exp Med Biol (2007) 601:195–203. doi: 10.1007/978-0-387-72005-0_20
81. Tong W, Maira M, Gagnon M, Saragovi HU. Ligands binding to cell surface ganglioside GD2 cause src-dependent activation of N-methyl-D-aspartate receptor signaling and changes in cellular morphology. PloS One (2015) 10:e0134255. doi: 10.1371/journal.pone.0134255
82. Chen YX, Chen XW, Li CG, Yue LJ, Mai HR, Wen FQ. Effect of tumor gangliosides on tyrosine phosphorylation of p125FAK in platelet adhesion to collagen. Oncol Rep (2013) 29:343–8. doi: 10.3892/or.2012.2092
83. Shibuya H, Hamamura K, Hotta H, Matsumoto Y, Nishida Y, Hattori H, et al. Enhancement of Malignant properties of human osteosarcoma cells with disialyl gangliosides GD2/GD3. Cancer Sci (2012) 103:1656–64. doi: 10.1111/j.1349-7006.2012.02344.x
84. Furukawa K, Hamamura K, Ohkawa Y, Ohmi Y, Furukawa K. Disialyl gangliosides enhance tumor phenotypes with differential modalities. Glycoconj J (2012) 29:579–84. doi: 10.1007/s10719-012-9423-0
85. Hamamura K, Tsuji M, Hotta H, Ohkawa Y, Takahashi M, Shibuya H, et al. Functional activation of Src family kinase yes protein is essential for the enhanced Malignant properties of human melanoma cells expressing ganglioside GD3. J Biol Chem (2011) 286:18526–37. doi: 10.1074/jbc.M110.164798
86. Mitsuzuka K, Handa K, Satoh M, Arai Y, Hakomori S. A specific microdomain ("glycosynapse 3") controls phenotypic conversion and reversion of bladder cancer cells through GM3-mediated interaction of alpha3beta1 integrin with CD9. J Biol Chem (2005) 280:35545–53. doi: 10.1074/jbc.M505630200
87. Yoshida S, Fukumoto S, Kawaguchi H, Sato S, Ueda R, Furukawa K. Ganglioside G(D2) in small cell lung cancer cell lines: enhancement of cell proliferation and mediation of apoptosis. Cancer Res (2001) 61:4244–52.
88. Daniotti JL, Crespo PM, Yamashita T. In vivo modulation of epidermal growth factor receptor phosphorylation in mice expressing different gangliosides. J Cell Biochem (2006) 99:1442–51. doi: 10.1002/jcb.21034
89. Iglesias-Bartolome R, Crespo PM, Gomez GA, Daniotti JL. The antibody to GD3 ganglioside, R24, is rapidly endocytosed and recycled to the plasma membrane via the endocytic recycling compartment. Inhibitory effect of brefeldin A and monensin. FEBS J (2006) 273:1744–58. doi: 10.1111/j.1742-4658.2006.05194.x
90. Ohkawa Y, Momota H, Kato A, Hashimoto N, Tsuda Y, Kotani N, et al. Ganglioside GD3 enhances invasiveness of gliomas by forming a complex with platelet-derived growth factor receptor alpha and yes kinase. J Biol Chem (2015) 290:16043–58. doi: 10.1074/jbc.M114.635755
91. Rampersaud AA, Oblinger JL, Ponnappan RK, Burry RW, Yates AJ. Gangliosides and growth factor receptor regulation. Biochem Soc Trans (1999) 27:415–22. doi: 10.1042/bst0270415
92. Tong W, Sprules T, Gehring K, Saragovi HU. Rational design of peptide ligands against a glycolipid by NMR studies. Methods Mol Biol (2012) 928:39–52. doi: 10.1007/978-1-62703-008-3_4
93. Sorice M, Longo A, Garofalo T, Mattei V, Misasi R, Pavan A. Role of GM3-enriched microdomains in signal transduction regulation in T lymphocytes. Glycoconjugate J (2004) 20:63–70. doi: 10.1023/B:GLYC.0000018018.29488.c6
94. Kasahara K, Watanabe Y, Yamamoto T, Sanai Y. Association of Src family tyrosine kinase Lyn with ganglioside GD3 in rat brain. Possible regulation of Lyn by glycosphingolipid in caveolae-like domains. J Biol Chem (1997) 272:29947–53. doi: 10.1074/jbc.272.47.29947
95. Yates AJ, Rampersaud A. Sphingolipids as receptor modulators. An overview. Ann N Y Acad Sci (1998) 845:57–71. doi: 10.1111/j.1749-6632.1998.tb09662.x
96. Ziche M, Morbidelli L, Alessandri G, Gullino PM. Angiogenesis can be stimulated or repressed in vivo by a change in GM3:GD3 ganglioside ratio. Lab Invest (1992) 67:711–5.
97. Liu Y, McCarthy J, Ladisch S. Membrane ganglioside enrichment lowers the threshold for vascular endothelial cell angiogenic signaling. Cancer Res (2006) 66:10408–14. doi: 10.1158/0008-5472.CAN-06-1572
98. Iwabuchi K, Handa K, Hakomori S. Separation of "glycosphingolipid signaling domain" from caveolin-containing membrane fraction in mouse melanoma B16 cells and its role in cell adhesion coupled with signaling. J Biol Chem (1998) 273:33766–73. doi: 10.1074/jbc.273.50.33766
99. Nakashima H, Hamamura K, Houjou T, Taguchi R, Yamamoto N, Mitsudo K, et al. Overexpression of caveolin-1 in a human melanoma cell line results in dispersion of ganglioside GD3 from lipid rafts and alteration of leading edges, leading to attenuation of Malignant properties. Cancer Sci (2007) 98:512–20. doi: 10.1111/j.1349-7006.2007.00419.x
100. Aixinjueluo W, Furukawa K, Zhang Q, Hamamura K, Tokuda N, Yoshida S, et al. Mechanisms for the apoptosis of small cell lung cancer cells induced by anti-GD2 monoclonal antibodies: roles of anoikis. J Biol Chem (2005) 280:29828–36. doi: 10.1074/jbc.M414041200
101. Yoshida S, Kawaguchi H, Sato S, Ueda R, Furukawa K. An anti-GD2 monoclonal antibody enhances apoptotic effects of anti-cancer drugs against small cell lung cancer cells via JNK (c-Jun terminal kinase) activation. Jpn J Cancer Res (2002) 93:816–24. doi: 10.1111/j.1349-7006.2002.tb01324.x
102. Furukawa K, Hamamura K, Aixinjueluo W, Furukawa K. Biosignals modulated by tumor-associated carbohydrate antigens: novel targets for cancer therapy. Ann New York Acad Sci (2006) 1086:185–98. doi: 10.1196/annals.1377.017
103. Caldwell S, Heitger A, Shen W, Liu Y, Taylor B, Ladisch S. Mechanisms of ganglioside inhibition of APC function. J Immunol (2003) 171:1676–83. doi: 10.4049/jimmunol.171.4.1676
104. Gaillard SL, Secord AA, Monk B. The role of immune checkpoint inhibition in the treatment of ovarian cancer. Gynecol Oncol Res Pract (2016) 3:11. doi: 10.1186/s40661-016-0033-6
105. Wu DY, Segal NH, Sidobre S, Kronenberg M, Chapman PB. Cross-presentation of disialoganglioside GD3 to natural killer T cells. J Exp Med (2003) 198:173–81. doi: 10.1084/jem.20030446
106. Rabu C, McIntosh R, Jurasova Z, Durrant L. Glycans as targets for therapeutic antitumor antibodies. Future Oncol (2012) 8:943–60. doi: 10.2217/fon.12.88
107. Rashidijahanabad Z, Huang X. Recent advances in tumor associated carbohydrate antigen based chimeric antigen receptor T cells and bispecific antibodies for anti-cancer immunotherapy. Semin Immunol (2020) 47:101390. doi: 10.1016/j.smim.2020.101390
108. Grayson G, Ladisch S. Immunosuppression by human gangliosides. II. Carbohydrate structure and inhibition of human NK activity. Cell Immunol (1992) 139:18–29. doi: 10.1016/0008-8749(92)90096-8
109. Shurin GV, Shurin MR, Bykovskaia S, Shogan J, Lotze MT, Barksdale EM Jr. Neuroblastoma-derived gangliosides inhibit dendritic cell generation and function. Cancer Res (2001) 61:363–9.
110. Dube DH, Bertozzi CR. Glycans in cancer and inflammation–potential for therapeutics and diagnostics. Nat Rev Drug Discovery (2005) 4:477–88. doi: 10.1038/nrd1751
111. RodrIguez E, Schetters STT, van Kooyk Y. The tumour glyco-code as a novel immune checkpoint for immunotherapy. Nat Rev Immunol (2018) 18:204–11. doi: 10.1038/nri.2018.3
112. Astronomo RD, Burton DR. Carbohydrate vaccines: developing sweet solutions to sticky situations? Nat Rev Drug Discovery (2010) 9:308–24. doi: 10.1038/nrd3012
113. Schulz G, Cheresh DA, Varki NM, Yu A, Staffileno LK, Reisfeld RA. Detection of ganglioside GD2 in tumor tissues and sera of neuroblastoma patients. Cancer Res (1984) 44:5914–20.
114. Zhang S, Cordon-Cardo C, Zhang HS, Reuter VE, Adluri S, Hamilton WB, et al. Selection of tumor antigens as targets for immune attack using immunohistochemistry: I. Focus on gangliosides. Int J Cancer (1997) 73:42–9. doi: 10.1002/(SICI)1097-0215(19970926)73:1<42::AID-IJC8>3.0.CO;2-1
115. Mujoo K, Cheresh DA, Yang HM, Reisfeld RA. Disialoganglioside GD2 on human neuroblastoma cells: target antigen for monoclonal antibody-mediated cytolysis and suppression of tumor growth. Cancer Res (1987) 47:1098–104.
116. Hettmer S, Ladisch S, Kaucic K. Low complex ganglioside expression characterizes human neuroblastoma cell lines. Cancer Lett (2005) 225:141–9. doi: 10.1016/j.canlet.2004.11.036
117. Cheresh DA, Pierschbacher MD, Herzig MA, Mujoo K. Disialogangliosides GD2 and GD3 are involved in the attachment of human melanoma and neuroblastoma cells to extracellular matrix proteins. J Cell Biol (1986) 102:688–96. doi: 10.1083/jcb.102.3.688
118. Liu X, Wills CA, Chen L, Zhang J, Zhao Y, Zhou M, et al. Small extracellular vesicles induce resistance to anti-GD2 immunotherapy unveiling tipifarnib as an adjunct to neuroblastoma immunotherapy. J Immunother Cancer (2022) 10. doi: 10.1136/jitc-2021-004399
119. Kozireski-Chuback D, Wu G, Ledeen RW. Developmental appearance of nuclear GM1 in neurons of the central and peripheral nervous systems. Brain Res Dev Brain Res (1999) 115:201–8. doi: 10.1016/S0165-3806(99)00062-0
120. Cavanna B, Carpo M, Pedotti R, Scarpini E, Meucci N, Allaria S, et al. Anti-GM2 IgM antibodies: clinical correlates and reactivity with a human neuroblastoma cell line. J Neuroimmunol (1999) 94:157–64. doi: 10.1016/S0165-5728(98)00245-8
121. Vrionis FD, Wikstrand CJ, Fredman P, Mansson JE, Svennerholm L, Bigner DD. Five new epitope-defined monoclonal antibodies reactive with GM2 and human glioma and medulloblastoma cell lines. Cancer Res (1989) 49:6645–51.
122. Mirkin BL, Clark SH, Zhang C. Inhibition of human neuroblastoma cell proliferation and EGF receptor phosphorylation by gangliosides GM1, GM3, GD1A and GT1B. Cell Prolif (2002) 35:105–15. doi: 10.1046/j.1365-2184.2002.00228.x
123. Hildebrandt H, Becker C, Gluer S, Rosner H, Gerardy-Schahn R, Rahmann H. Polysialic acid on the neural cell adhesion molecule correlates with expression of polysialyltransferases and promotes neuroblastoma cell growth. Cancer Res (1998) 58:779–84.
124. Valentiner U, Muhlenhoff M, Lehmann U, Hildebrandt H, Schumacher U. Expression of the neural cell adhesion molecule and polysialic acid in human neuroblastoma cell lines. Int J Oncol (2011) 39:417–24. doi: 10.3892/ijo.2011.1038
125. Liang YJ, Ding Y, Levery SB, Lobaton M, Handa K, Hakomori SI. Differential expression profiles of glycosphingolipids in human breast cancer stem cells vs. cancer non-stem cells. Proc Natl Acad Sci U.S.A. (2013) 110:4968–73.
126. Orsi G, Barbolini M, Ficarra G, Tazzioli G, Manni P, Petrachi T, et al. GD2 expression in breast cancer. Oncotarget (2017) 8:31592–600. doi: 10.18632/oncotarget.16363
127. Liang YJ, Wang CY, Wang IA, Chen YW, Li LT, Lin CY, et al. Interaction of glycosphingolipids GD3 and GD2 with growth factor receptors maintains breast cancer stem cell phenotype. Oncotarget (2017) 8:47454–73. doi: 10.18632/oncotarget.17665
128. Steenackers A, Vanbeselaere J, Cazet A, Bobowski M, Rombouts Y, Colomb F, et al. Accumulation of unusual gangliosides G(Q3) and G(P3) in breast cancer cells expressing the G(D3) synthase. Molecules (2012) 17:9559–72. doi: 10.3390/molecules17089559
129. Bobowski M, Vincent A, Steenackers A, Colomb F, Van Seuningen I, Julien S, et al. Estradiol represses the G(D3) synthase gene ST8SIA1 expression in human breast cancer cells by preventing NFkappaB binding to ST8SIA1 promoter. PloS One (2013) 8:e62559.
130. Ma Q, Zhuo D, Guan F, Li X, Yang X, Tan Z. Vesicular ganglioside GM1 from breast tumor cells stimulated epithelial-to-mesenchymal transition of recipient MCF-10A cells. Front Oncol (2022) 12:837930. doi: 10.3389/fonc.2022.837930
131. Wang X, Li X, Zeng YN, He F, Yang XM, Guan F. Enhanced expression of polysialic acid correlates with Malignant phenotype in breast cancer cell lines and clinical tissue samples. Int J Mol Med (2016) 37:197–206. doi: 10.3892/ijmm.2015.2395
132. Liang JX, Liang Y, Gao W. Clinicopathological and prognostic significance of sialyl Lewis X overexpression in patients with cancer: a meta-analysis. Onco Targets Ther (2016) 9:3113–25.
133. Natoni A, Macauley MS, O'Dwyer ME. Targeting selectins and their ligands in cancer. Front Oncol (2016) 6:93. doi: 10.3389/fonc.2016.00093
134. Ravindranath MH, Muthugounder S, Presser N, Selvan SR, Santin AD, Bellone S, et al. Immunogenic gangliosides in human ovarian carcinoma. Biochem Biophys Res Commun (2007) 353:251–8. doi: 10.1016/j.bbrc.2006.12.001
135. Webb TJ, Li X, Giuntoli RL 2nd, Lopez PH, Heuser C, Schnaar RL, et al. Molecular identification of GD3 as a suppressor of the innate immune response in ovarian cancer. Cancer Res (2012) 72:3744–52. doi: 10.1158/0008-5472.CAN-11-2695
136. Ravindranath MH, Muthugounder S, Hannah MR, Morton DL. Significance of endogenous augmentation of antiganglioside IgM in cancer patients: potential tool for early detection and management of cancer therapy. Ann New York Acad Sci (2007) 1107:212–22. doi: 10.1196/annals.1381.023
137. Prinetti A, Aureli M, Illuzzi G, Prioni S, Nocco V, Scandroglio F, et al. GM3 synthase overexpression results in reduced cell motility and in caveolin-1 upregulation in human ovarian carcinoma cells. Glycobiology (2010) 20:62–77. doi: 10.1093/glycob/cwp143
138. Brezicka FT, Olling S, Nilsson O, Bergh J, Holmgren J, Sorenson S, et al. Immunohistological detection of fucosyl-GM1 ganglioside in human lung cancer and normal tissues with monoclonal antibodies. Cancer Res (1989) 49:1300–5.
139. Yamada T, Bando H, Takeuchi S, Kita K, Li Q, Wang W, et al. Genetically engineered humanized anti-ganglioside GM2 antibody against multiple organ metastasis produced by GM2-expressing small-cell lung cancer cells. Cancer Sci (2011) 102:2157–63. doi: 10.1111/j.1349-7006.2011.02093.x
140. Blanco R, Dominguez E, Morales O, Blanco D, Martinez D, Rengifo CE, et al. Prognostic significance of N-glycolyl GM3 ganglioside expression in non-small cell lung carcinoma patients: new evidences. Patholog Res Int (2015) 2015:132326. doi: 10.1155/2015/132326
141. Hayashi N, Chiba H, Kuronuma K, Go S, Hasegawa Y, Takahashi M, et al. Detection of N-glycolyated gangliosides in non-small-cell lung cancer using GMR8 monoclonal antibody. Cancer Sci (2013) 104:43–7. doi: 10.1111/cas.12027
142. Tanaka F, Otake Y, Nakagawa T, Kawano Y, Miyahara R, Li M, et al. Prognostic significance of polysialic acid expression in resected non-small cell lung cancer. Cancer Res (2001) 61:1666–70.
143. Tanaka F, Otake Y, Nakagawa T, Kawano Y, Miyahara R, Li M, et al. Expression of polysialic acid and STX, a human polysialyltransferase, is correlated with tumor progression in non-small cell lung cancer. Cancer Res (2000) 60:3072–80.
144. Watarai S, Kiura K, Shigeto R, Shibayama T, Kimura I, Yasuda T. Establishment of monoclonal antibodies specific for ganglioside GM1: detection of ganglioside GM1 in small cell lung carcinoma cell lines and tissues. J Biochem (1994) 116:948–54. doi: 10.1093/oxfordjournals.jbchem.a124651
145. Mennel HD, Bosslet K, Wiegandt H, Sedlacek HH, Bauer BL, Rodden AF. Expression of GD2-epitopes in human intracranial tumors and normal brain. Exp Toxicol Pathol (1992) 44:317–24. doi: 10.1016/S0940-2993(11)80218-6
146. Lama G, Mangiola A, Proietti G, Colabianchi A, Angelucci C, D' Alessio A, et al. Progenitor/stem cell markers in brain adjacent to glioblastoma: GD3 ganglioside and NG2 proteoglycan expression. J Neuropathol Exp Neurol (2016) 75:134–47. doi: 10.1093/jnen/nlv012
147. Birks SM, Danquah JO, King L, Vlasak R, Gorecki DC, Pilkington GJ. Targeting the GD3 acetylation pathway selectively induces apoptosis in glioblastoma. Neuro Oncol (2011) 13:950–60. doi: 10.1093/neuonc/nor108
148. Elkashef SM, Allison SJ, Sadiq M, Basheer HA, Ribeiro Morais G, Loadman PM, et al. Polysialic acid sustains cancer cell survival and migratory capacity in a hypoxic environment. Sci Rep (2016) 6:33026. doi: 10.1038/srep33026
149. Hedberg KM, Mahesparan R, Read TA, Tysnes BB, Thorsen F, Visted T, et al. The glioma-associated gangliosides 3'-isoLM1, GD3 and GM2 show selective area expression in human glioblastoma xenografts in nude rat brains. Neuropathol Appl Neurobiol (2001) 27:451–64. doi: 10.1046/j.1365-2990.2001.00353.x
150. Fujimoto Y, Izumoto S, Suzuki T, Kinoshita M, Kagawa N, Wada K, et al. Ganglioside GM3 inhibits proliferation and invasion of glioma. J Neurooncol (2005) 71:99–106. doi: 10.1007/s11060-004-9602-3
151. Noll EN, Lin J, Nakatsuji Y, Miller RH, Black PM. GM3 as a novel growth regulator for human gliomas. Exp Neurol (2001) 168:300–9. doi: 10.1006/exnr.2000.7603
152. Bassi R, Viani P, Giussani P, Riboni L, Tettamanti G. GM3 ganglioside inhibits endothelin-1-mediated signal transduction in C6 glioma cells. FEBS Lett (2001) 507:101–4. doi: 10.1016/S0014-5793(01)02966-0
153. Trouillas J, Daniel L, Guigard MP, Tong S, Gouvernet J, Jouanneau E, et al. Polysialylated neural cell adhesion molecules expressed in human pituitary tumors and related to extrasellar invasion. J Neurosurg (2003) 98:1084–93. doi: 10.3171/jns.2003.98.5.1084
154. Sasaki N, Hirabayashi K, Michishita M, Takahashi K, Hasegawa F, Gomi F, et al. Ganglioside GM2, highly expressed in the MIA PaCa-2 pancreatic ductal adenocarcinoma cell line, is correlated with growth, invasion, and advanced stage. Sci Rep (2019) 9:19369. doi: 10.1038/s41598-019-55867-4
155. Pukel CS, Lloyd KO, Travassos LR, Dippold WG, Oettgen HF, Old LJ. GD3, a prominent ganglioside of human melanoma. Detection and characterisation by mouse monoclonal antibody. J Exp Med (1982) 155:1133–47. doi: 10.1084/jem.155.4.1133
156. Nicoll G, Avril T, Lock K, Furukawa K, Bovin N, Crocker PR. Ganglioside GD3 expression on target cells can modulate NK cell cytotoxicity via siglec-7-dependent and -independent mechanisms. Eur J Immunol (2003) 33:1642–8. doi: 10.1002/eji.200323693
157. Hamamura K, Furukawa K, Hayashi T, Hattori T, Nakano J, Nakashima H, et al. Ganglioside GD3 promotes cell growth and invasion through p130Cas and paxillin in Malignant melanoma cells. Proc Natl Acad Sci U.S.A. (2005) 102:11041–6.
158. Nicolae CD, Nicolae I. Antibodies against GM1 gangliosides associated with metastatic melanoma. Acta Dermatovenerol Croat (2013) 21:86–92.
159. Hoon DS, Ando I, Sviland G, Tsuchida T, Okun E, Morton DL, et al. Ganglioside GM2 expression on human melanoma cells correlates with sensitivity to lymphokine-activated killer cells. Int J Cancer (1989) 43:857–62. doi: 10.1002/ijc.2910430520
160. Chang HR, Cordon-Cardo C, Houghton AN, Cheung NK, Brennan MF. Expression of disialogangliosides GD2 and GD3 on human soft tissue sarcomas. Cancer (1992) 70:633–8. doi: 10.1002/1097-0142(19920801)70:3<633::AID-CNCR2820700315>3.0.CO;2-F
161. Heiner JP, Miraldi F, Kallick S, Makley J, Neely J, Smith-Mensah WH, et al. Localization of GD2-specific monoclonal antibody 3F8 in human osteosarcoma. Cancer Res (1987) 47:5377–81.
162. Dobrenkov K, Ostrovnaya I, Gu J, Cheung IY, Cheung NK. Oncotargets GD2 and GD3 are highly expressed in sarcomas of children, adolescents, and young adults. Pediatr Blood Cancer (2016) 63:1780–5. doi: 10.1002/pbc.26097
163. Poon VI, Roth M, Piperdi S, Geller D, Gill J, Rudzinski ER, et al. Ganglioside GD2 expression is maintained upon recurrence in patients with osteosarcoma. Clin Sarcoma Res (2015) 5:4. doi: 10.1186/s13569-014-0020-9
164. Roth M, Linkowski M, Tarim J, Piperdi S, Sowers R, Geller D, et al. Ganglioside GD2 as a therapeutic target for antibody-mediated therapy in patients with osteosarcoma. Cancer (2014) 120:548–54. doi: 10.1002/cncr.28461
165. Lipinski M, Braham K, Philip I, Wiels J, Philip T, Goridis C, et al. Neuroectoderm-associated antigens on Ewing's sarcoma cell lines. Cancer Res (1987) 47:183–7.
166. Kailayangiri S, Altvater B, Meltzer J, Pscherer S, Luecke A, Dierkes C, et al. The ganglioside antigen G(D2) is surface-expressed in Ewing sarcoma and allows for MHC-independent immune targeting. Br J Cancer (2012) 106:1123–33. doi: 10.1038/bjc.2012.57
167. Scursoni AM, Galluzzo L, Camarero S, Lopez J, Lubieniecki F, Sampor C, et al. Detection of N-glycolyl GM3 ganglioside in neuroectodermal tumors by immunohistochemistry: an attractive vaccine target for aggressive pediatric cancer. Clin Dev Immunol (2011) 2011:245181. doi: 10.1155/2011/245181
168. Modak S, Gerald W, Cheung N-KV. Disialoganglioside GD2 and a novel tumor antigen: potential targets for immunotherapy of desmoplastic small round cell tumor. Med Pediatr Oncol (2002) 39:547–51. doi: 10.1002/mpo.10151
169. Portoukalian J, David MJ, Gain P, Richard M. Shedding of GD2 ganglioside in patients with retinoblastoma. Int J Cancer (1993) 53:948–51. doi: 10.1002/ijc.2910530614
170. Torbidoni AV, Scursoni A, Camarero S, Segatori V, Gabri M, Alonso D, et al. Immunoreactivity of the 14F7 Mab raised against N-Glycolyl GM3 Ganglioside in retinoblastoma tumours. Acta Ophthalmol (2015) 93:e294–300. doi: 10.1111/aos.12578
171. Sakakibara N, Gasa S, Kamio K, Makita A, Nonomura K, Togashi M, et al. Distinctive glycolipid patterns in Wilms' tumor and renal cell carcinoma. Cancer Lett (1991) 57:187–92. doi: 10.1016/0304-3835(91)90155-B
172. Roth J, Zuber C, Wagner P, Blaha I, Bitter-Suermann D, Heitz PU. Presence of the long chain form of polysialic acid of the neural cell adhesion molecule in Wilms' tumor. Identification of a cell adhesion molecule as an oncodevelopmental antigen and implications for tumor histogenesis. Am J Pathol (1988) 133:227–40.
173. Komminoth P, Roth J, Saremaslani P, Matias-Guiu X, Wolfe HJ, Heitz PU. Polysialic acid of the neural cell adhesion molecule in the human thyroid: a marker for medullary thyroid carcinoma and primary C-cell hyperplasia. An immunohistochemical study on 79 thyroid lesions. Am J Surg Pathol (1994) 18:399–411. doi: 10.1097/00000478-199404000-00008
174. Das T, Sa G, Hilston C, Kudo D, Rayman P, Biswas K, et al. GM1 and tumor necrosis factor-alpha, overexpressed in renal cell carcinoma, synergize to induce T-cell apoptosis. Cancer Res (2008) 68:2014–23. doi: 10.1158/0008-5472.CAN-07-6037
175. Biswas K, Richmond A, Rayman P, Biswas S, Thornton M, Sa G, et al. GM2 expression in renal cell carcinoma: potential role in tumor-induced T-cell dysfunction. Cancer Res (2006) 66:6816–25. doi: 10.1158/0008-5472.CAN-06-0250
176. Borzym-Kluczyk M, Radziejewska I, Cechowska-Pasko M. Increased expression of MUC1 and sialyl Lewis antigens in different areas of clear renal cell carcinoma. Clin Exp Nephrol (2015) 19:732–7. doi: 10.1007/s10157-014-1013-y
177. Zhuo D, Li X, Guan F. Biological roles of aberrantly expressed glycosphingolipids and related enzymes in human cancer development and progression. Front Physiol (2018) 9:466. doi: 10.3389/fphys.2018.00466
178. Weiner LM, Surana R, Wang S. Monoclonal antibodies: versatile platforms for cancer immunotherapy. Nat Rev Immunol (2010) 10:317–27. doi: 10.1038/nri2744
179. Kramer G, Suss W, Kohler H, Schneider B, Hopf HC. [Ganglioside therapy in uremic polyneuropathy]. Med Klin (Munich) (1988) 83:7–11.
180. Svennerholm L, Bostrom K, Fredman P, Jungbjer B, Lekman A, Mansson JE, et al. Gangliosides and allied glycosphingolipids in human peripheral nerve and spinal cord. Biochim Biophys Acta (1994) 1214:115–23. doi: 10.1016/0005-2760(94)90034-5
181. Wu ZL, Schwartz E, Seeger R, Ladisch S. Expression of GD2 ganglioside by untreated primary human neuroblastomas. Cancer Res (1986) 46:440–3.
182. Park J-E, Wu DY, Prendes M, Lu SX, Ragupathi G, Schrantz N, et al. Fine specificity of natural killer T cells against GD3 ganglioside and identification of GM3 as an inhibitory natural killer T-cell ligand. Immunology (2008) 123:145–55. doi: 10.1111/j.1365-2567.2007.02760.x
183. Xu J, Fan W, Tu XX, Zhang T, Hou ZJ, Guo T, et al. Neural ganglioside GD2(+) cells define a subpopulation of mesenchymal stem cells in adult murine bone marrow. Cell Physiol Biochem (2013) 32:889–98. doi: 10.1159/000354492
184. Cheresh DA, Rosenberg J, Mujoo K, Hirschowitz L, Reisfeld RA. Biosynthesis and expression of the disialoganglioside GD2, a relevant target antigen on small cell lung carcinoma for monoclonal antibody-mediated cytolysis. Cancer Res (1986) 46:5112–8.
185. Park JE, Lu SX, Wu DY, Prendes M, Chapman PB. Antibody response to GD3 ganglioside is independent of NKT cells. Cytotherapy (2008) 10:38–44. doi: 10.1080/14653240701762380
186. Machy P, Mortier E, Birkle S. Biology of GD2 ganglioside: implications for cancer immunotherapy. Front Pharmacol (2023) 14:1249929. doi: 10.3389/fphar.2023.1249929
187. Cheung NK, Saarinen U, Neely J, Miraldi F, Strandjord S, Warkentin P, et al. Development of neuroblastoma monoclonal antibodies for potential utilization in diagnosis and therapy. Prog Clin Biol Res (1985) 175:501–5.
188. Ivasko SM, Anders K, Grunewald L, Launspach M, Klaus A, Schwiebert S, et al. Combination of GD2-directed bispecific trifunctional antibody therapy with Pd-1 immune checkpoint blockade induces anti-neuroblastoma immunity in a syngeneic mouse model. Front Immunol (2022) 13:1023206. doi: 10.3389/fimmu.2022.1023206
189. Siebert N, Zumpe M, Schwencke CH, Biskupski S, Troschke-Meurer S, Leopold J, et al. Combined blockade of TIGIT and PD-L1 enhances anti-neuroblastoma efficacy of GD2-directed immunotherapy with dinutuximab beta. Cancers (Basel) (2023) 15. doi: 10.3390/cancers15133317
190. Li Q, Sun M, Yu M, Fu Q, Jiang H, Yu G, et al. Gangliosides profiling in serum of breast cancer patient: GM3 as a potential diagnostic biomarker. Glycoconj J (2019) 36:419–28. doi: 10.1007/s10719-019-09885-z
191. Jaggupilli A, Ly S, Nguyen K, Anand V, Yuan B, El-Dana F, et al. Metabolic stress induces GD2(+) cancer stem cell-like phenotype in triple-negative breast cancer. Br J Cancer (2022) 126:615–27. doi: 10.1038/s41416-021-01636-y
192. Cheng JY, Hung JT, Lin J, Lo FY, Huang JR, Chiou SP, et al. O-acetyl-GD2 as a therapeutic target for breast cancer stem cells. Front Immunol (2021) 12:791551. doi: 10.3389/fimmu.2021.791551
193. Talabnin K, Talabnin C, Kumagai T, Sutatum N, Khiaowichit J, Dechsukhum C, et al. Ganglioside GM2: a potential biomarker for cholangiocarcinoma. J Int Med Res (2020) 48:300060520903216. doi: 10.1177/0300060520903216
194. Mao LM, Wang JQ. Dopaminergic and cholinergic regulation of Fyn tyrosine kinase phosphorylation in the rat striatum in vivo. Neuropharmacology (2015) 99:491–9. doi: 10.1016/j.neuropharm.2015.08.017
195. Tshering G, Dorji PW, Chaijaroenkul W, Na-Bangchang K. Biomarkers for the diagnosis of cholangiocarcinoma: A systematic review. Am J Trop Med Hyg (2018) 98:1788–97. doi: 10.4269/ajtmh.17-0879
196. Liu MC, Oxnard GR, Klein EA, Swanton C, Seiden MV, Consortium C. Sensitive and specific multi-cancer detection and localization using methylation signatures in cell-free DNA. Ann Oncol (2020) 31:745–59. doi: 10.1016/j.annonc.2020.02.011
197. Kok MGM, de Ronde MWJ, Moerland PD, Ruijter JM, Creemers EE, Pinto-Sietsma SJ. Small sample sizes in high-throughput miRNA screens: A common pitfall for the identification of miRNA biomarkers. Biomol Detect Quantif (2018) 15:1–5. doi: 10.1016/j.bdq.2017.11.002
198. Kang Q, Henry NL, Paoletti C, Jiang H, Vats P, Chinnaiyan AM, et al. Comparative analysis of circulating tumor DNA stability In K(3)EDTA, Streck, and CellSave blood collection tubes. Clin Biochem (2016) 49:1354–60. doi: 10.1016/j.clinbiochem.2016.03.012
199. Pons-Belda OD, Fernandez-Uriarte A, Diamandis EP. Multi Cancer Early Detection by Using Circulating Tumor DNA-The Galleri Test. Reply to Klein et al. The Promise of Multicancer Early Detection. Comment on "Pons-Belda et al. Can Circulating Tumor DNA Support a Successful Screening Test for Early Cancer Detection? The Grail Paradigm. Diagnostics 2021, 11, 2171". Diagnost (Basel) (2022) 12.
200. Fiala C, Diamandis EP. Can a broad molecular screen based on circulating tumor DNA aid in early cancer detection? J Appl Lab Med (2020) 5:1372–7. doi: 10.1093/jalm/jfaa138
201. Fiala C, Diamandis EP. Circulating tumor DNA (ctDNA) is not a good proxy for liquid biopsies of tumor tissues for early detection. Clin Chem Lab Med (2020) 58:1651–3. doi: 10.1515/cclm-2020-0083
202. Chernicky CL, Yi L, Tan H, Gan SU, Ilan J. Treatment of human breast cancer cells with antisense RNA to the type I insulin-like growth factor receptor inhibits cell growth, suppresses tumorigenesis, alters the metastatic potential, and prolongs survival in vivo. Cancer Gene Ther (2000) 7:384–95. doi: 10.1038/sj.cgt.7700126
203. Lennon AM, Buchanan AH, Kinde I, Warren A, Honushefsky A, Cohain AT, et al. Feasibility of blood testing combined with PET-CT to screen for cancer and guide intervention. Science (2020) 369. doi: 10.1126/science.abb9601
204. Brignole C, Pastorino F, Marimpietri D, Pagnan G, Pistorio A, Allen TM, et al. Immune cell-mediated antitumor activities of GD2-targeted liposomal c-myb antisense oligonucleotides containing CpG motifs. J Natl Cancer Institute (2004) 96:1171–80. doi: 10.1093/jnci/djh221
Keywords: biomarker, diagnosis, cancer, prognosis, early stage, screening, ganglioside
Citation: Nejatie A, Yee SS, Jeter A and Saragovi HU (2023) The cancer glycocode as a family of diagnostic biomarkers, exemplified by tumor-associated gangliosides. Front. Oncol. 13:1261090. doi: 10.3389/fonc.2023.1261090
Received: 18 July 2023; Accepted: 13 October 2023;
Published: 26 October 2023.
Edited by:
Laura Gatti, IRCCS Carlo Besta Neurological Institute Foundation, ItalyReviewed by:
Yuh-Jin Liang, Taipei Veterans General Hospital, TaiwanJaume Mora, Sant Joan de Déu Hospital, Spain
Copyright © 2023 Nejatie, Yee, Jeter and Saragovi. This is an open-access article distributed under the terms of the Creative Commons Attribution License (CC BY). The use, distribution or reproduction in other forums is permitted, provided the original author(s) and the copyright owner(s) are credited and that the original publication in this journal is cited, in accordance with accepted academic practice. No use, distribution or reproduction is permitted which does not comply with these terms.
*Correspondence: Horacio Uri Saragovi, VXJpLnNhcmFnb3ZpQG1jZ2lsbC5jYQ==
†ORCID: Ali Nejatie, orcid.org/0000-0001-8187-4831
Samantha S. Yee, orcid.org/0000-0001-7953-0183
Anna Jeter, orcid.org/0009-0002-9757-3536
Horacio Uri Saragovi, orcid.org/0000-0001-6884-8815