- 1North America Evidence and Value Development, North America Medical Affairs, EMD Serono, Inc., Rockland, MA, United States, an affiliate of Merck KGaA
- 2Evidence and Access, OPEN Health, Parsippany, NJ, United States
- 3Earle A. Chiles Research Institute, Providence Cancer Institute, Portland, OR, United States
Introduction: Mesenchymal-epidermal transition factor gene amplification (METamp) is being investigated as a therapeutic target in advanced non-small cell lung cancer (NSCLC). We reviewed the epidemiology and disease characteristics associated with primary and secondary METamp, as well as the testing procedures used to identify METamp, in advanced NSCLC. Economic and humanistic burdens, and the practice patterns and treatments under investigation for METamp were also examined.
Methods: Embase and Medline (via ProQuest), ClinicalTrials.gov, and Cochrane Controlled Register of Trials (2015–2022) were systematically searched. Conference abstracts were searched via Embase and conference proceedings websites (2020–2022). The review focused on evidence from the United States; global evidence was included for identified evidence gaps.
Results: The median rate of primary METamp in NSCLC across the references was 4.8% (n=4 studies) and of secondary METamp (epidermal growth factor receptor [EGFR]-mutant NSCLC) was 15% (n=10). Next-generation sequencing (NGS; n=12) and/or fluorescence in situ hybridization (FISH; n=11) were most frequently used in real-world studies and FISH testing most frequently used in clinical trials (n=9/10). METamp definitions varied among clinical trials using ISH/FISH testing (MET to chromosome 7 centromere ratio of ≥1.8 to ≥3.0; or gene copy number [GCN] ≥5 to ≥10) and among trials using NGS (tissue testing: GCN ≥6; liquid biopsy: MET copy number ≥2.1 to >5). Limited to no data were identified on the economic and humanistic burdens, and real-world treatment of METamp NSCLC. Promising preliminary results from trials enrolling patients with EGFR-mutated, METamp advanced NSCLC progressing on an EGFR-tyrosine kinase inhibitor (TKI) were observed with MET-TKIs (i.e., tepotinib, savolitinib, and capmatinib) in combination with EGFR-TKIs (i.e., gefitinib and osimertinib). For metastatic NSCLC and high-level METamp, monotherapy with capmatinib, crizotinib, and tepotinib are recommended in the 2022 published NSCLC NCCN Guidelines.
Conclusion: Primary METamp occurs in approximately 5% of NSCLC cases, and secondary METamp in approximately 15% of cases previously treated with an EGFR inhibitor. Variability in testing methods (including ISH/FISH and NGS) and definitions were observed. Several treatments are promising in treating METamp NSCLC. Additional studies evaluating the clinical, economic, and humanistic burdens are needed.
1 Introduction
Non-small cell lung cancer (NSCLC) is a heterogenous disease that is frequently diagnosed at an advanced stage due to variability of signs and symptoms at diagnosis (1, 2). The treatment of NSCLC is becoming more individualized as broad molecular testing for actionable driver alterations has transformed the diagnosis and treatment of advanced NSCLC (3). As of 2023, both national and international guidelines recommend molecular testing to help guide treatment decisions in advanced NSCLC (4–6).
Alterations in the transmembrane tyrosine kinase mesenchymal-epidermal transition factor (MET) receptor such as MET gene amplification (METamp) have been identified as actionable drivers in NSCLC (7). METamp in NSCLC may be a primary oncogenic driver, or a secondary driver which arises during or following treatment and commonly develops as an adaptive resistance mechanism to epidermal growth factor receptor (EGFR)-tyrosine kinase inhibitor (TKI) treatment (7, 8). Although EGFR-TKIs have significantly improved outcomes in patients with EGFR-mutant advanced NSCLC, the development of METamp-driven resistance to EGFR-TKIs is a key obstacle to long-term disease control (4, 9). In patients with secondary METamp following treatment with an EGFR-TKI, concomitant inhibition of both EGFR and MET is thought to overcome resistance to EGFR inhibitors due to METamp (9).
This targeted review was undertaken to better understand the burden of METamp NSCLC in the United States (US) and to identify the available evidence on the epidemiology and disease characteristics associated with primary and secondary METamp. Other outcomes evaluated included evidence on METamp testing procedures, the economic and humanistic burdens of METamp NSCLC, and practice patterns and treatments under investigation for METamp advanced NSCLC.
2 Methods
A systematic search of studies published as journal articles or conference abstracts written in the English language was performed on June 14, 2022 using predefined search terms in Embase and Medline (via ProQuest; Supplementary Table 1). Journal articles were searched from January 1, 2015 onwards, and conference abstracts were searched from January 1, 2020 onwards. Abstracts from the following 2022 conferences that were not yet indexed in Embase by June 14, 2022 were also searched: the American Association for Cancer Research, Academy of Managed Care Pharmacy, American Society of Clinical Oncology, International Society for Pharmacoeconomics and Outcomes Research, and NCCN. Searches using predefined search terms in ClinicalTrials.gov (Supplementary Table 2) and the Cochrane Controlled Register of Trials (Supplementary Table 3) were performed to identify completed and ongoing clinical trials; searches were performed on June 14, 2022.
The utilized search strategies were global in scope and included terms for NSCLC, METamp, and outcomes of interest. Whereas the focus of this literature review was US-based evidence, global, non-US-based evidence was included when gaps in US-based evidence for outcomes of interest were identified. Publications containing information on the following topics for either primary or secondary METamp were selected for inclusion (Table 1): US-based epidemiology and global evidence for disease characteristics, genomic testing procedures, economic and humanistic burden, real-world treatment patterns, ongoing clinical trials, and clinical trial outcomes. Real-world prospective or retrospective studies, clinical trials, and US-based guidelines were included.
An independent researcher completed title, abstract and full text screening, identified studies to be included based on the Population, Intervention, Comparison, Outcomes, Study Design (PICOS) selection criteria (Table 1), and extracted data. Another independent researcher performed standard quality checks on approximately 10% of randomly selected references during the abstract and full text screening to verify results. Results are descriptive in nature. No formal statistical analyses or comparisons among identified studies were made.
3 Results
A total of 1,004 references were screened; of these, 117 references met the inclusion criteria, including 79 publications from ProQuest and searched conference abstracts, and 38 webpage references from ClinicalTrials.gov (Figure 1). Of the 117 references meeting inclusion criteria, references commonly provided information on more than one topic of interest.
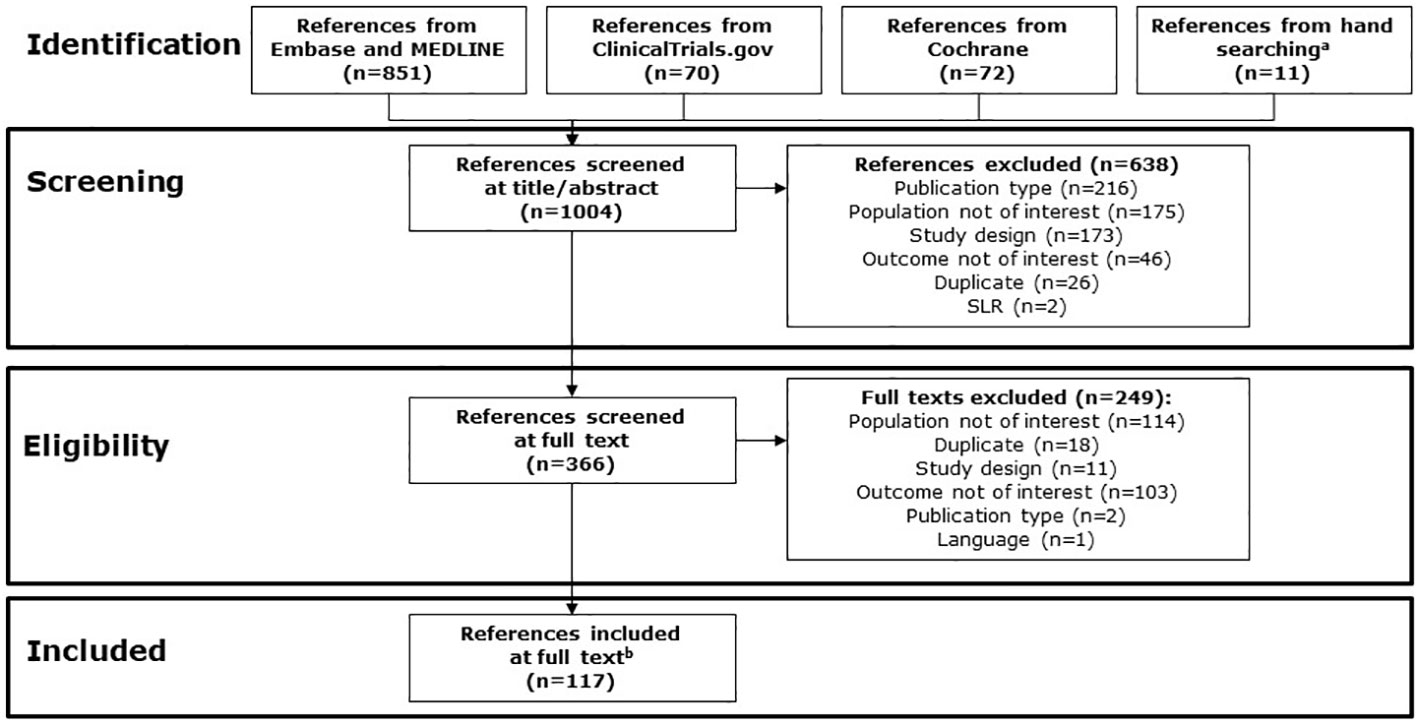
Figure 1 PRISMA study identification flow chart. a Abstracts from the following conferences in 2022 were searched via conference proceedings websites: AACR, AMCP, ASCO, ISPOR, and NCCN. References from relevant SLRs were reviewed. b Includes 79 publications from ProQuest and searching conferences, and 38 references from ClinicalTrials.gov. AACR, American Association for Cancer Research; AMCP, Academy of Managed Care Pharmacy; ASCO, American Society of Clinical Oncology; ISPOR, International Society for Pharmacoeconomics and Outcomes Research; NCCN, National Comprehensive Cancer Network; PRISMA, Preferred Reporting Items for Systematic reviews and Meta-Analyses; SLR, systematic literature review.
3.1 Epidemiology and disease characteristics
A total of 13 publications providing information on the epidemiology of primary or secondary METamp in the US were identified. The median incidence of primary METamp in all NSCLC in the US was 4.8% (range: 2.0–5.8%), based on four studies including 3,379 patients (10–13). In these studies, primary METamp was defined as METamp at NSCLC diagnosis and/or before receipt of targeted therapies; METamp was identified either by a single test or by a testing panel that included METamp and other driver alterations (10–13). The median incidence of secondary (acquired) METamp in EGFR-mutant NSCLC previously treated with an EGFR-TKI was 15% (range: 2.9–66.7%), based on a total of 10 studies including 389 patients (13–22).
A total of 29 publications providing information on disease characteristics of METamp NSCLC were identified (23–51). A summary of results from 16 US- or non-US based studies that evaluated demographic and clinical characteristics of patients with NSCLC with or without METamp is found in Supplementary Table 4 (24–27, 29, 32, 33, 35–37, 44–47, 50, 51). Results from the identified studies show that the following characteristics were consistently considered not associated with METamp (either primary, secondary, or not specified): age (11 studies), sex (11 studies), and NSCLC sub-type (adenocarcinoma vs non-adenocarcinoma, squamous cell carcinoma, or other; 6 studies) (24, 26, 27, 32, 33, 36, 44, 45, 47, 50, 51). Results from two studies demonstrated a significant statistical association between METamp and a higher proportion of programmed cell death protein 1 ligand (PD-L1) expression (25, 35). Overall, most studies (8/10) found no statistical association between METamp and a positive smoking history (24, 26, 27, 32, 33, 44, 45, 47, 50, 51). Among two studies that evaluated patients with EGFR-mutant NSCLC who experienced progression on an EGFR-TKI, one study found that history of smoking was associated with a high probability of secondary METamp (p=0.011), and one study found no association between smoking status and presence of METamp (p=0.45) (24, 27). No studies specifically looked at the association between demographics and clinical characteristics in patients with primary or secondary METamp.
Results for patients with stage I–III NSCLC (n=170) show that patients with METamp versus no METamp had a significantly shorter time to development of distant metastasis (11.6 vs 43.8 months; p=0.004), and results of a multivariate analysis confirmed that METamp was highly associated with earlier progression to distant metastases (hazard ratio: 4.86; 95% CI: 1.85, 12.75; p=0.001) (36). All patients in this study received standard of care therapy per their disease stage at diagnosis, such as surgery, chemotherapy, radiation therapy, or EGFR inhibitor therapy when applicable; rates of therapy and primary versus secondary METamp were not reported. A second study found that among primary lung adenocarcinoma and metastatic lung adenocarcinoma tumor samples, the identification of METamp was significantly higher in the metastatic than primary tumor samples (p<0.001) (46). Evidence regarding the association between brain metastases and METamp differed based on the enrolled populations. Results from two different studies evaluating NSCLC tumor samples found that METamp was identified in a significantly higher number of NSCLC brain metastases samples (including lung adenocarcinoma brain metastases) compared with samples from primary tumors (29, 37). Results from one study evaluating patients with advanced NSCLC with EGFR mutations found that rates of brain metastases did not significantly differ among patients with primary METamp versus no METamp (45).
Interpretation of survival results for patients with METamp versus no METamp is limited due to differences in follow-up times, treatments, and patient populations. Among all studies identified, no statistical association between METamp and overall survival for patients with advanced NSCLC was found in six studies (24, 26, 27, 35, 44, 51), and significantly worse overall survival among patients with METamp NSCLC versus non-METamp NSCLC was found in three studies (36, 45, 47). Results from two studies evaluating patients with secondary METamp who had EGFR-mutant NSCLC and progression on an EGFR-TKI found that there was no significant difference in overall survival from the time of initiation of EGFR-TKI therapy according to METamp status (24, 27). Median progression-free survival from the most recent EGFR-TKI treatment was significantly shorter among patients with METamp versus no METamp, based on a multivariate analysis in one study (hazard ratio, 0.898; 95% CI: 0.835, 0.965; p=0.004) (24).
3.2 Testing
A total of 39 publications providing testing data for METamp (including two US-based guidelines providing testing recommendations for METamp NSCLC) were identified. The US-based guidelines included the NCCN Guidelines and the joint guideline by the College of American Pathologists (CAP), the International Association for the Study of Lung Cancer (IASLC), and the Association for Molecular Pathology (AMP) (4, 5).
The NSCLC NCCN Guidelines consider high-level METamp to be an emerging biomarker, which should optimally be identified when broad molecular profiling is performed for the following actionable driver mutations: EGFR, anaplastic lymphoma kinase (ALK), Kristin rat sarcoma virus (KRAS), c-Ros oncogene 1 (ROS1), v-Raf murine sarcoma viral oncogene homolog B (BRAF), neurotrophic tyrosine receptor kinase (NTRK1/2/3), MET exon 14 skipping, RET proto-oncogene (RET), and human epidermal growth factor receptor 2 (ERBB2 [HER2]) (4). The CAP-IASLC-AMP guidelines (published in 2018) recommend that it is appropriate to include MET alterations as part of larger testing panels performed either initially or when routine EGFR, ALK, and ROS1 testing are negative (5). Molecular testing for MET alterations including MET exon 14 mutation and METamp is not indicated as a routine stand-alone assay outside the context of a clinical trial per the CAP-IASLC-AMP guidelines.
The definition of high-level METamp is evolving and may differ according to the assay used for testing (4). Fluorescence in situ hybridization (FISH) is generally considered the gold standard to evaluate MET gene copy number (52, 53). Tissue-based next-generation sequencing (NGS) can also be used to simultaneously test for METamp and other actionable biomarkers (4). There are no clinically defined cut-off values for NGS. While the NCCN Guidelines recognize that the definition of high-level METamp is not established, a copy number greater than 10 is considered consistent with characterizing a result as high-level METamp (4). Of 10 identified clinical trials providing definitions for METamp, FISH testing was the most commonly used testing strategy (nine studies) (30, 54–62). The definition for METamp in clinical trials varied and ranged from a MET to chromosome 7 centromere (MET/CEP7) ratio of ≥1.8 to ≥3.0 (Table 2) (30, 54–62).
Most real-world studies that included data from the US (20 publications from the US and three publications from multi-country assessments including the US) used NGS (12 studies) and/or FISH (11 studies) to test for METamp; among these studies, tissue samples were used in 13 studies, tissue or liquid samples in seven studies, liquid samples in one study, and no sample information was provided for two studies (10–14, 17, 18, 20, 25, 28, 36–38, 40, 49, 63–70). There are limited real-world data comparing the reliability of using NGS versus FISH to identify patients with METamp (52, 53). Results from a study in Germany suggest that there is higher concordance between NGS and FISH when considering highly amplified MET (gene copy number >10); however, NGS may be less able to detect cases harboring lower levels of expression (i.e., low or intermediate METamp) (52).
No studies evaluating the economic impact of specifically testing for METamp were identified in the literature review; however, two studies evaluating the economic impact of using NGS versus single gene testing strategies to identify actionable genomic alterations among patients with advanced NSCLC in the US were identified (71, 72). Both studies demonstrated cost savings with NGS from both a Medicare and US commercial payer’s perspective when testing for actionable genomic alterations included in clinical guideline recommendations (EGFR, ALK, ROS1, BRAF, KRAS, MET, HER2, RET, and NTRK1) compared with single gene testing strategies (71, 72). NGS was associated with a faster mean time to appropriate targeted therapy initiation (2 weeks) compared with other testing strategies, including single gene sequential testing (8–9 weeks) or hotspot panel testing (3 weeks) (71).
3.3 Economic burden and patient-reported outcomes
No information on economic or humanistic burdens in METamp NSCLC was identified in the literature.
3.4 Real-world treatment patterns and outcomes data
A total of 18 publications providing information on the real-world treatment patterns and outcomes data for METamp NSCLC were identified. Results from several studies indicated that patients with EGFR-mutant NSCLC who received a variety of first-, second-, or third-generation EGFR-TKIs may have detection of secondary METamp following progression on EGFR-TKI treatment (13, 24, 27, 42, 73, 74). Evidence from real-world studies suggests that there is no association between the type of EGFR-TKI and risk of acquiring METamp (13, 24, 42).
There is a lack of US-based evidence on treatments used in real-world settings following diagnosis of METamp NSCLC. The majority of references evaluating real-world treatment patterns among patients who have secondary METamp NSCLC were from China and assessed crizotinib alone or in combination with EGFR-TKIs (such as gefitinib, erlotinib, osimertinib, and icotinib; Supplementary Table 5) (27, 39, 42, 73–75).
3.5 Investigational treatments in METamp NSCLC
Inhibition of MET signaling is being investigated as a promising therapeutic strategy in patients with METamp NSCLC; a total of 16 publications providing information on clinical trial evidence in METamp NSCLC were identified. Several agents alone or in combination with EGFR-TKIs are currently under investigation for the treatment of METamp NSCLC, including small molecule MET receptor inhibitors (e.g., crizotinib (30, 55), savolitinib (57, 76, 77), tepotinib (61, 62, 78, 79), and capmatinib (56, 60)), monoclonal or bispecific antibodies that can block MET activity (e.g., amivantamab (80)), and an anti-MET antibody–drug conjugate (e.g., telisotuzumab vedotin (81)).
Thirty-eight Phase I–III trials evaluating treatments for METamp NSCLC and registered on ClinicalTrials.gov were identified as of June 14, 2022 (80, 82–118). Many identified trials included patients with several different MET alterations (including MET exon 14 skipping, MET overexpression, and METamp), and some trials included patients with a variety of different actionable genomic alterations. Only results from trials that focus exclusively on METamp or include a subgroup of patients specifically with METamp are included in this review. Studies that only presented combined results for patients with METamp and another MET alteration are not described in this review.
3.5.1 MET tyrosine kinase inhibitors
There are two types of MET-TKIs, including type I MET-TKIs that bind to an active form of MET (e.g., tepotinib, capmatinib, crizotinib, savolitinib, and bozitinib), and type II MET-TKIs that bind to an inactive form of MET (e.g., cabozantinib and glesatinib). The majority of MET-TKIs are orally administered (e.g., tepotinib, capmatinib, savolitinib, crizotinib) (30, 55–57, 60–62, 76, 78, 79).
Published results identified at the time of this literature review from clinical trials evaluating MET-TKIs are summarized in the following sections and in Table 3.
3.5.1.1 MET-TKI combination therapy for the treatment of secondary METamp NSCLC
3.5.1.1.1 Capmatinib
Results from a single arm Phase Ib/II trial evaluating capmatinib plus gefitinib after failure of EGFR inhibitor therapy in patients with EGFR-mutated, METamp NSCLC (n=36) demonstrated that patients treated with capmatinib plus gefitinib had a median (95% CI) progression-free survival of 5.49 (4.21, 7.29) months and an objective response rate of 47% (56).
3.5.1.1.2 Savolitinib
Results from a Phase Ib trial evaluating savolitinib plus gefitinib after progression on an EGFR-TKI in patients with EGFR-mutated, METamp advanced NSCLC (n=51) demonstrated that patients treated with savolitinib plus gefitinib had a median (95% CI) progression-free survival of 4.0 (2.8, 5.5) months and an objective response rate of 31% (76).
Another Phase Ib trial (TATTON) evaluated savolitinib plus gefitinib after progression on an EGFR-TKI in patients with EGFR-mutated, METamp advanced NSCLC in two expansion cohorts (57). The TATTON trial was divided into four parts, A to D, and parts B and D were the two global expansion cohorts evaluating savolitinib plus gefitinib. Part B consisted of three cohorts of patients: those who had been previously treated with a third-generation EGFR-TKI and those who had not been previously treated with a third-generation EGFR-TKI who were either T790M negative or T790M positive; part D enrolled patients who had not previously received a third-generation EGFR-TKI and were T790M negative. Results from this study demonstrated that the median (95% CI) progression-free survival in cohort B (n=138) was 7.6 (5.5, 9.2) months and for cohort D (n=42) was 9.1 (5.4, 12.9) months. The objective response rate was 48% in cohort B and 64% in cohort D.
Savolitinib in combination with osimertinib is being evaluated in several Phase II and III ongoing trials (94, 100–103). Results from the Phase II ORCHARD study evaluating savolitinib plus osimertinib after progression on first-line osimertinib monotherapy among patients with locally advanced/metastatic EGFR-mutant NSCLC demonstrated that patients treated with savolitinib plus osimertinib (n=17) had an overall response rate of 41% after a follow-up of 13 weeks (77). Results for other Phase II and III studies evaluating savolitinib plus osimertinib were not identified at the time of this literature review.
3.5.1.1.3 Tepotinib
A total of 19 patients with EGFR-mutated, METamp advanced NSCLC who progressed on an EGFR-TKI were enrolled in a Phase Ib/II trial (INSIGHT) (61, 78). In this study evaluating tepotinib plus gefitinib (n=12) and chemotherapy (n=7), median (90% CI) progression-free survival was 16.6 (8.3, 22.1) and 4.2 (1.4, 7.0) months (hazard ratio: 0.13; 90% CI: 0.04, 0.43), and median (90% CI) overall survival was 37.3 (21.1, 52.1) and 13.1 (3.3, 22.6) months (hazard ratio: 0.10; 90% CI: 0.02, 0.36). The objective response rate was 67% in patients receiving tepotinib plus gefitinib and 43% in patients receiving chemotherapy (78).
Tepotinib in combination with osimertinib is also being evaluated among patients with EGFR-mutated NSCLC following progression on osimertinib in the Phase II INSIGHT-2 trial (99). Primary analysis results for INSIGHT-2 have been presented at the World Conference on Lung Cancer Congress in September 2023 but were not yet published at the time of this literature review (119).
3.5.1.2 MET-TKI monotherapy
3.5.1.2.1 Bozitinib
Preliminary results from a Phase I, open-label, multicenter study evaluating bozitinib in locally advanced or metastatic NSCLC with MET dysregulation demonstrated that bozitinib had a manageable safety profile at the recommended Phase II dose of 200 mg twice daily (120). Only eight patients had METamp NSCLC in this study, and it was not specified if patients had primary or secondary METamp.
3.5.1.2.2 Capmatinib
Two Phase II trials evaluated the use of capmatinib monotherapy in patients with METamp NSCLC (54, 60). In the first study, a Phase II trial evaluating capmatinib in MET exon 14-mutated or METamp NSCLC, patients did not have an EGFR mutation or ALK fusion (60). Patients with METamp NSCLC and a gene copy number ≥10 had a median (95% CI) progression-free survival of 4.1 (2.9, 4.8) months for patients who had received 1–2 previous lines of therapy (n=69) and 4.2 (1.4, 6.9) months for patients with no previous lines of therapy (n=15) (60). The objective response rate was 29% for patients with 1–2 previous lines of therapy and 40% for patients with no previous lines of therapy. Additional cohorts for patients with a gene copy number less than 10 (gene copy number 6–9, 4–5, or < 4) were initially planned and ultimately closed for futility at an interim analysis (60).
In the second study, a Phase II trial evaluating capmatinib in patients with advanced NSCLC harboring METamp or MET exon 14 skipping alterations, patients must have received treatment with a prior MET-TKI, and there was no restriction on the number of prior treatment regimens (54). Only five patients in this trial had METamp, and none of these five patients achieved an objective response (54). The authors noted that a limitation of this study was the limited number of patients enrolled with METamp NSCLC.
3.5.1.2.3 Crizotinib
In a Phase I study enrolling patients with advanced NSCLC who have not received previous hepatocyte growth factor- or MET-targeted therapy, METamp was defined as a MET/CEP7 ratio >1.8; low levels of METamp were defined as ≥1.8 to ≤2.2; medium levels as >2.2 to < 4.0; and high levels as ≥4.0 (the initial cut-off for the high versus medium threshold was changed from a ≥5 to ≥4 MET/CEP7 ratio based on a preliminary response analysis) (30). Results from this study demonstrated that patients with advanced METamp NSCLC had a median (95% CI) progression-free survival of 5.1 (1.9, 7.0) months for all patients (N=38), 6.7 (3.4, 9.2) months for patients with high METamp (n=21), 1.9 (1.3, 5.6) months for patients with medium METamp (n=14), and 1.8 (0.8, 14.0) months for patients with low METamp (n=3). The objective response rate (95% CI) was 28.9% (15.4, 45.9) for all patients, 38.1% (18.1, 61.6) in the high METamp group, 14.3% (1.8, 42.8) in the medium METamp group, and 33.3% (0.8, 90.6) in the low METamp group. Median overall survival (95% CI) with crizotinib was 11.0 (7.1, 15.9) months for all patients, 11.4 (7.2, 19.3) months for patients with high METamp, 9.2 (2.1, 18.1) months for patients with medium METamp, and 5.6 (1.1, not estimable) months for patients with low METamp.
In a Phase II trial, crizotinib monotherapy was evaluated in patients with pretreated NSCLC with MET dysregulation or evidence of ROS1 rearrangements; patients with EGFR or KRAS mutations were excluded (55). Patients with METamp NSCLC (n=16) had a median (95% CI) progression-free survival of 5.0 (2.7, 7.3) months, a median (95% CI) overall survival of 5.4 (3.4, 7.4) months, and an objective response rate of 31.3% (95% CI: 5.2, 71.4) (55).
3.5.1.2.4 SAR125844
Results from a first-in-human Phase I trial evaluating SAR125844 in advanced solid tumors and MET dysregulation found that patients with advanced METamp NSCLC (n=22) demonstrated a partial response rate of 18.2%; no patients achieved a complete response (58). Patients in this trial were allowed, but not required, to have EGFR mutations or prior EGFR inhibitor therapy.
3.5.1.2.5 Tepotinib
Results from a Phase II trial evaluating tepotinib in EGFR and ALK-wild type NSCLC with high-level METamp (VISION) demonstrated that tepotinib (n=24) showed clinical activity, especially in the first-line setting at the data cut-off (August 20, 2021) (62, 121). Among all patients, the objective response rate was 41.7% (95% CI: 22.1, 63.4) and the median duration of response was 14.3 months (95% CI: 2.8, not estimable). Among patients treated in the first-line setting (n=7), the objective response rate was 71.4% (95% CI: 29.0, 96.3) and the median duration of response was 14.3 months (95% CI: 2.8, not estimable). Results at the August 20, 2021 data cut-off, identified through this literature search, were initially presented as conference proceedings in 2022 (62) and subsequently published in November 2023 (121).
3.5.2 Anti-MET antibodies
3.5.2.1 Amivantamab
Amivantamab has a unique structure in that it is a bispecific antibody that blocks both epidermal growth factor and MET receptors (80). It is being investigated in multiple studies, including in a Phase I trial enrolling patients with metastatic or unresectable NSCLC that has progressed after prior standard of care therapy (CHRYSALIS-1). The objective of this study is to evaluate the safety, pharmacokinetics, and preliminary efficacy of amivantamab (either alone or in combination with lazertinib), and to determine the recommended Phase II doses for expansion of amivantamab monotherapy and combination therapy. One of the cohorts includes patients with primary EGFR-mutated disease and documented METamp or MET mutation after progression on any EGFR-TKI. Published results for patients with METamp NSCLC from this trial were not identified at the time of the literature review.
3.5.2.2 Sym015
Sym015 is a mixture of two humanized antibodies targeting MET (59). Interim safety and efficacy results from a Phase I trial were reported for eight patients with advanced METamp NSCLC (including seven patients who were MET-TKI-naïve, and one patient previously treated with a MET-TKI). The objective response rate was 25%.
3.5.3 Anti-MET antibody–drug conjugates
3.5.3.1 Telisotuzumab vedotin
Telisotuzumab vedotin is a first-in-class antibody–drug conjugate consisting of a humanized MET-targeting antibody, ABT-700, coupled to a cytotoxic microtubule inhibitor, monomethyl auristatin E, through a valine–citrulline linker (81). It is currently being evaluated as monotherapy and in combination with osimertinib, erlotinib, and nivolumab in participants with advanced solid tumors, including METamp NSCLC (98).
4 Discussion
Results from this review indicate that primary METamp occurs in approximately 5% of NSCLC cases. Secondary METamp, which is an established mechanism of resistance for patients with EGFR mutation-positive NSCLC (3), occurs in approximately 15% of advanced NSCLC cases previously treated with an EGFR inhibitor in the US. METamp has also been identified as a mechanism of resistance in patients with NSCLC and other actionable genomic alterations, including ALK fusion, RET fusion, ROS1 fusion, and KRAS G12C mutation (38, 40, 122–127). An overview of the MET pathway and mechanisms of METamp-mediated resistance in NSCLC is not included in the scope of this literature review and has been previously described in several reviews (3, 128–130).
The higher rates of secondary METamp among patients with EGFR-mutant NSCLC compared with primary METamp in NSCLC indicate a need for testing at different time points of the treatment journey, including at diagnosis and upon progression on an EGFR-TKI. In real-world settings, FISH and NGS are both commonly used to test for METamp. Variability exists in how METamp is defined across clinical trials and real-world evidence studies. Further studies are needed to evaluate the use and reliability of different testing strategies (NGS vs FISH; and tumor vs liquid testing) for identification of patients with METamp, with potential advantage to improved standardization of definitions of METamp.
No studies evaluating the economic and humanistic burdens of METamp NSCLC were identified in this literature review, suggesting further studies evaluating these burdens are warranted. Furthermore, there is a lack of US-based evidence on treatments used in real-world settings following diagnosis of METamp NSCLC. Any interpretation of real-world evidence is limited as sample sizes were small in the few studies identified. Studies evaluating real-world practice patterns and outcomes among patients with METamp NSCLC in the US and other countries are needed.
As of March 2023, there were no US Food and Drug Administration approved therapies for METamp NSCLC. The NCCN Guidelines recommend capmatinib, crizotinib, and tepotinib as monotherapy for patients with metastatic NSCLC and high-level METamp (4). The NCCN Guidelines also note that the best management of any patient with cancer is in a clinical trial, and participation in clinical trials is especially encouraged (4). The European Society for Medical Oncology guidelines similarly note that while METamp is a promising therapeutic target, targeting METamp is not currently routinely recommended and recruitment into trials is encouraged (6).
Historically, trials aimed at targeting MET overexpression (e.g., onartuzumab) in NSCLC have failed (6). Onartuzumab (METmab), for example, is a MET-receptor monoclonal antibody that was evaluated in combination with erlotinib for the treatment of advanced NSCLC with MET diagnostic-positive status tested by immunohistochemistry; however, the Phase III trial evaluating onartuzumab combination therapy was stopped prematurely due to lack of clinically meaningful efficacy (131).
Instead of MET overexpression, the focus has shifted to targeting genomic variants, such as MET exon 14 skipping and METamp (6). Several agents alone or in combination with EGFR-TKIs were identified as under investigation for the treatment of METamp NSCLC. Information on ClinicalTrials.gov is evolving and additional trials evaluating therapies for METamp NSCLC have been indexed since the time of our literature search in June 2022, such as the Phase I trial evaluating the antibody-drug conjugate MYTX-011 (132) and a Phase I/II trial evaluating amivantamab and capmatinib combination therapy (133).
Concomitant inhibition of both EGFR and MET is thought to overcome resistance to EGFR inhibitors due to METamp (9, 134), and combination therapy with EGFR inhibitors and several type I MET-TKIs (tepotinib, savolitinib, and capmatinib) have shown promising results among patients with EGFR-mutated, METamp NSCLC who progressed on a prior EGFR-TKI (56, 76, 78, 119, 135). Final analysis of the Phase Ib/II INSIGHT trial demonstrated an objective response rate of 66.7% in patients treated with tepotinib plus gefitinib (78). Primary analysis results from the Phase II INSIGHT-2 trial (data cut-off: March 28, 2023; results were published in 2023 after our literature search was performed) demonstrated that treatment with tepotinib plus osimertinib demonstrated an objective response rate of 50.0% and a median progression-free survival of 5.6 months among patients with EGFR-mutant NSCLC and METamp who progressed on first-line osimertinib (119). Preliminary results from the SAVANNAH Phase II trial published in 2022 showed that savolitinib plus osimertinib demonstrated an objective response rate of 49% in patients with EGFR-mutated NSCLC with high levels of METamp, defined as FISH 10+, whose disease progressed on treatment with osimertinib (135). Results from a Phase Ib/II study showed that patients treated with capmatinib plus gefitinib had an objective response rate of 47% (56).
4.1 Limitations
The scope of the literature review search strategies included terms for NSCLC, METamp, and outcomes of interest. Due to inclusion of METamp terms in the search strategies, references describing rates of secondary METamp as a secondary or exploratory outcome among patients with EGFR-mutant NSCLC, references describing broad molecular testing patterns for all actionable driver mutations in NSCLC, or references describing treatments under investigation for patients with MET alterations generally (and not METamp specifically) may not have been captured. The included data were current as of our search date (June 14, 2022); data published or included in a database after that date were not captured. Treatments under investigation for METamp NSCLC are evolving rapidly, and ClinicalTrials.gov should be checked regularly for an up-to-date list of ongoing trials. Information available on ClinicalTrials.gov, however, is limited and not all actionable genomic alterations being evaluated in a clinical trial and included in a study protocol may be listed on ClinicalTrials.gov.
5 Conclusion
Results from this literature review demonstrate that primary METamp occurs in approximately 5% of NSCLC cases and secondary METamp occurs in approximately 15% of advanced NSCLC cases previously treated with an EGFR inhibitor in the US. NGS and FISH were commonly used to identify METamp in real-world studies; across clinical trials, variability existed in how METamp was defined. Future studies evaluating the economic and humanistic burden, as well as the real-world evidence on treatment for advanced METamp NSCLC are needed.
Several promising agents, including MET-TKIs in combination with EGFR-TKIs, are currently under investigation for secondary METamp NSCLC.
Data availability statement
The original contributions presented in the study are included in the article/Supplementary Material. Further inquiries can be directed to the corresponding author.
Author contributions
All authors contributed to the conception or design of the work; the acquisition, analysis, or interpretation of data for the work; drafting the work or revising it critically for important intellectual content; providing final approval of the version to be published; and agree to be accountable for all aspects of the work in ensuring that questions related to the accuracy or integrity of any part of the work are appropriately investigated and resolved. All authors contributed to the article and approved the submitted version.
Funding
The author(s) declare financial support was received for the research and/or publication of this article. This study was funded by EMD Serono, Inc., Rockland, MA, USA, an affiliate of Merck KGaA (CrossRef Funder ID: 10.13039/100004755).
Acknowledgments
Editorial support was provided by Chiara Lee of Syneos Health, UK and funded by EMD Serono, Inc., Rockland, MA, USA, an affiliate of Merck KGaA.
Conflict of interest
Authors MY, FL, and RH were employed by the company EMD Serono, Inc., Rockland, MA, USA, an affiliate of Merck KGaA, at the time of the study. Authors EM and BL were employed by the company OPEN Health at the time of this study, which was the recipient of consulting fees from EMD Serono, Inc., Rockland, MA, USA, an affiliate of Merck KGaA. Author RS received honoraria from AstraZeneca, and Amgen; attended advisory boards and provided consulting for AstraZeneca, EMD Serono, Inc., Rockland, MA, USA, an affiliate of Merck KGaA, Daiichi Sankyo, Lilly, Janssen Oncology, Macrogenics, Sanofi/Aventis, Regeneron, Mirati Therapeutics, and GlaxoSmithKline; received research funding from Merck, AstraZeneca Investigator-sponsored trials, and BMS Institution research funding.
Publisher’s note
All claims expressed in this article are solely those of the authors and do not necessarily represent those of their affiliated organizations, or those of the publisher, the editors and the reviewers. Any product that may be evaluated in this article, or claim that may be made by its manufacturer, is not guaranteed or endorsed by the publisher.
Supplementary material
The Supplementary Material for this article can be found online at: https://www.frontiersin.org/articles/10.3389/fonc.2023.1241402/full#supplementary-material
References
1. Lemjabbar-Alaoui H, Hassan OU, Yang YW, Buchanan P. Lung cancer: Biology and treatment options. Biochim Biophys Acta (2015) 1856(2):189–210. doi: 10.1016/j.bbcan.2015.08.002
2. Ganti AK, Klein AB, Cotarla I, Seal B, Chou E. Update of incidence, prevalence, survival, and initial treatment in patients with non-small cell lung cancer in the US. JAMA Oncol (2021) 7(12):1824–32. doi: 10.1001/jamaoncol.2021.4932
3. Coleman N, Hong L, Zhang J, Heymach J, Hong D, Le X. Beyond epidermal growth factor receptor: MET amplification as a general resistance driver to targeted therapy in oncogene-driven non-small-cell lung cancer. ESMO Open (2021) 6(6):100319. doi: 10.1016/j.esmoop.2021.100319
4. Ettinger DS, Wood DE, Aisner DL, Akerley W, Bauman JR, Bharat A, et al. Non-small cell lung cancer, version 3.2022, NCCN clinical practice guidelines in oncology. J Natl Compr Canc Netw (2022) 20(5):497–530. doi: 10.6004/jnccn.2022.0025
5. Lindeman NI, Cagle PT, Aisner DL, Arcila ME, Beasley MB, Bernicker EH, et al. Updated molecular testing guideline for the selection of lung cancer patients for treatment with targeted tyrosine kinase inhibitors: Guideline from the College of American Pathologists, the International Association for the Study of Lung Cancer, and the Association for Molecular Pathology. J Thorac Oncol (2018) 13(3):323–58. doi: 10.1016/j.jtho.2017.12.001
6. Planchard D, Popat S, Kerr K, Novello S, Smit EF, Faivre-Finn C, et al. Metastatic non-small cell lung cancer: ESMO clinical practice guidelines for diagnosis, treatment and follow-up. Ann Oncol (2018) 29 Suppl 4:iv192–237. doi: 10.1093/annonc/mdy275
7. Guo R, Luo J, Chang J, Rekhtman N, Arcila M, Drilon A. MET-dependent solid tumours - molecular diagnosis and targeted therapy. Nat Rev Clin Oncol (2020) 17(9):569–87. doi: 10.1038/s41571-020-0377-z
8. Peng L-X, Jie G-L, Li A-N, Liu S-Y, Sun H, Zheng M-M, et al. MET amplification identified by next-generation sequencing and its clinical relevance for MET inhibitors. Exp Hematol Oncol (2021) 10(1):52. doi: 10.1186/s40164-021-00245-y
9. Wang Q, Yang S, Wang K, Sun SY. MET inhibitors for targeted therapy of EGFR TKI-resistant lung cancer. J Hematol Oncol (2019) 12(1):63. doi: 10.1186/s13045-019-0759-9
10. Aisner DL, Sholl LM, Berry LD, Rossi MR, Chen H, Fujimoto J, et al. The impact of smoking and tp53 mutations in lung adenocarcinoma patients with targetable mutations—the lung cancer mutation consortium (LCMC2). Clin Cancer Res (2018) 24(5):1038–47. doi: 10.1158/1078-0432.CCR-17-2289
11. Guo R, Berry LD, Aisner DL, Sheren J, Boyle T, Bunn PA Jr., et al. MET IHC is a poor screen for MET amplification or MET exon 14 mutations in lung adenocarcinomas: Data from a tri-institutional cohort of the Lung Cancer Mutation Consortium. J Thorac Oncol (2019) 14(9):1666–71. doi: 10.1016/j.jtho.2019.06.009
12. Yin W, Cheng J, Tang Z, Toruner G, Hu S, Guo M, et al. MET amplification (MET/CEP7 ratio ≥ 1.8) is an independent poor prognostic marker in patients with treatment-naive non-small-cell lung cancer. Clin Lung Cancer (2021) 22(4):e512–e8. doi: 10.1016/j.cllc.2020.11.002
13. Yin W, Liu W, Guo M, Tang Z, Toruner G, Robinson M, et al. Acquired MET amplification in non-small cell lung cancer is highly associated with the exposure of EGFR inhibitors and may not affect patients’ outcome. Exp Mol Pathol (2021) 118:104572. doi: 10.1016/j.yexmp.2020.104572
14. Le X, Puri S, Negrao MV, Nilsson MB, Robichaux J, Boyle T, et al. Landscape of EGFR-dependent and -independent resistance mechanisms to osimertinib and continuation therapy beyond progression in EGFR-mutant NSCLC. Clin Cancer Res (2018) 24(24):6195–203. doi: 10.1158/1078-0432.CCR-18-1542
15. Ramalingam SS, Yang JCH, Lee CK, Kurata T, Kim D-W, John T, et al. Osimertinib as first-line treatment of EGFR mutation-positive advanced non-small-cell lung cancer. J Clin Oncol (2018) 36(9):841–9. doi: 10.1200/JCO.2017.74.7576
16. Roper N, Brown A-L, Wei JS, Pack S, Trindade C, Kim C, et al. Clonal evolution and heterogeneity of osimertinib acquired resistance mechanisms in EGFR mutant lung cancer. Cell Rep Med (2020) 1(1):100007. doi: 10.1016/j.xcrm.2020.100007
17. Schoenfeld AJ, Chan JM, Kubota D, Sato H, Rizvi H, Daneshbod Y, et al. Tumor analyses reveal squamous transformation and off-target alterations as early resistance mechanisms to first-line osimertinib in EGFR-mutant lung cancer. Clin Cancer Res (2020) 26(11):2654–63. doi: 10.1158/1078-0432.CCR-19-3563
18. Seetharamu N, Sullivan K, Carter A, Lee C. P51.02 Stacking on the targets: Secondary resistant, potential targetable genetic alterations in patients with epidermal growth factor receptor NSCLC. J Thorac Oncol (2021) 16(10):S1119. doi: 10.1016/j.jtho.2021.08.539
19. Meador C, Cobb R, Hung Y, Banwait M, Piper-Vallillo A, Muzikansky A, et al. MA14.07 EGFR-mutant NSCLC with de novo or acquired squamous histology: Molecular features and clinical outcomes. J Thorac Oncol (2021) 16(10):S932–S3. doi: 10.1016/j.jtho.2021.08.187
20. Patil T, Mushtaq R, Marsh S, Azelby C, Pujara M, Davies KD, et al. Clinicopathologic characteristics, treatment outcomes, and acquired resistance patterns of atypical EGFR mutations and HER2 alterations in stage IV non–small-cell lung cancer. Clin Lung Cancer (2020) 21(3):e191–204. doi: 10.1016/j.cllc.2019.11.008
21. Yanagita M, Redig AJ, Paweletz CP, Dahlberg SE, O’Connell A, Feeney N, et al. A prospective evaluation of circulating tumor cells and cell-free DNA in EGFR-mutant non-small cell lung cancer patients treated with erlotinib on a phase II trial. Clin Cancer Res (2016) 22(24):6010–20. doi: 10.1158/1078-0432.CCR-16-0909
22. Hartmaier RJ, Markovets A, Cho BC, De Langen AJ, Goldberg SB, Goldman J, et al. Tumor genomics in patients (pts) with advanced epidermal growth factor receptor mutant (EGFRm) non-small cell lung cancer (NSCLC) whose disease has progressed on first-line (1L) osimertinib therapy in the Phase II ORCHARD study. Cancer Res (2022) 82(12_Supplement):LB078. doi: 10.1158/1538-7445.AM2022-LB078
23. Aguado C, Teixido C, Román R, Reyes R, Giménez-Capitán A, Marin E, et al. Multiplex RNA-based detection of clinically relevant MET alterations in advanced non-small cell lung cancer. Mol Oncol (2021) 15(2):350–63. doi: 10.1002/1878-0261.12861
24. Ahn B-C, Lee JH, Kim MH, Pyo K-H, Lee C-K, Lim SM, et al. Distinct characteristics and clinical outcomes to predict the emergence of met amplification in patients with non-small cell lung cancer who developed resistance after treatment with epidermal growth factor receptor tyrosine kinase inhibitors. Cancers (2021) 13(12):3096. doi: 10.3390/cancers13123096
25. Albitar M, Sudarsanam S, Ma W, Jiang S, Chen W, Funari V, et al. Correlation of MET gene amplification and TP53 mutation with PD-L1 expression in non-small cell lung cancer. Oncotarget (2018) 9(17):13682–93. doi: 10.18632/oncotarget.24455
26. Ando R, Fujino M, Kominami-Kiriyama A, Ito A, Koide T, Ito M. Mesenchymal–epithelial transition gene amplification and protein overexpression in stage IV pulmonary adenocarcinoma. Jpn J Clin Oncol (2019) 49(8):755–61. doi: 10.1093/jjco/hyz060
27. Baldacci S, Mazieres J, Tomasini P, Girard N, Guisier F, Audigier-Valette C, et al. Outcome of EGFR-mutated NSCLC patients with MET-driven resistance to EGFR tyrosine kinase inhibitors. Oncotarget (2017) 8(62):105103–14. doi: 10.18632/oncotarget.21707
28. Bittoni M, Yang JC-H, Shih J-Y, Peled N, Smit EF, Camidge DR, et al. Real-world insights into patients with advanced NSCLC and MET alterations. Lung Cancer (2021) 159:96–106. doi: 10.1016/j.lungcan.2021.06.015
29. Burns TF, Dacic S, Velez MA, Somasundaram A, Bhattacharya S, Chakka A, et al. MET alterations are enriched in lung adenocarcinoma brain metastases and define a distinct molecular and transcriptomic subtype. Cancer Res (2021) 81(13_Supplement):2218. doi: 10.1158/1538-7445.AM2021-2218
30. Camidge DR, Otterson GA, Clark JW, Ignatius Ou S-H, Weiss J, Ades S, et al. Crizotinib in patients with MET-amplified NSCLC. J Thorac Oncol (2021) 16(6):1017–29. doi: 10.1016/j.jtho.2021.02.010
31. Castiglione R, Alidousty C, Holz B, Wagener S, Baar T, Heydt C, et al. Comparison of the genomic background of MET-altered carcinomas of the lung: biological differences and analogies. Mod Pathol (2019) 32(5):627–38. doi: 10.1038/s41379-018-0182-8
32. Chan KI, Vong HT, Sin LF, Yip YC, Zhong XY, Wen JM. Relationship between driver gene mutations, their relative protein expressions and survival in non-small cell lung carcinoma in Macao. Clin Respir J (2018) 12(4):1416–23. doi: 10.1111/crj.12670
33. Chen D, Xu C, Wu J, Zhang Y, Fang M. A comparison of consistency of detecting c-MET gene amplification in peripheral blood and tumor tissue of nonsmall cell lung cancer patients. J Cancer Res Ther (2015) 11(5):C63–C7. doi: 10.4103/0973-1482.163843
34. Cui W, Milner-Watts C, Faull I, Nagy RJ, Scott S, Minchom A, et al. Circulating tumour (ct) DNA next generation sequencing (NGS) in advanced non-small cell lung cancer (mNSCLC): A UK single institution experience. Ann Oncol (2020) 31:S867. doi: 10.1016/j.annonc.2020.08.1666
35. Domènech M, Muñoz Marmol AM, Mate JL, Estival A, Moran T, Cucurull M, et al. Correlation between PD-L1 expression and MET gene amplification in patients with advanced non-small cell lung cancer and no other actionable oncogenic driver. Oncotarget (2021) 12(18):1802–10. doi: 10.18632/oncotarget.28045
36. Fang L, Chen H, Tang Z, Kalhor N, Liu C-H, Yao H, et al. MET amplification assessed using optimized FISH reporting criteria predicts early distant metastasis in patients with nonsmall cell lung cancer. Oncotarget (2018) 9(16):12959–70. doi: 10.18632/oncotarget.24430
37. Huang RSP, Harries L, Decker B, Hiemenz MC, Murugesan K, Creeden J, et al. Clinicopathologic and genomic landscape of non-small cell lung cancer brain metastases. Oncologist (2022) 27(10):839–48. doi: 10.1093/oncolo/oyac094
38. Lee J, Rosenzweig M, Piper-Vallillo A, Vanderlaan P, Tolba K, Li T, et al. MA02.03 MET-driven acquired resistance (AR) in fusion-positive non-small cell lung cancer (NSCLC). J Thorac Oncol (2021) 16(10):S890. doi: 10.1016/j.jtho.2021.08.113
39. Li J, Wang Y, Zhang B, Xu J, Cao S, Zhong H. Characteristics and response to crizotinib in lung cancer patients with MET amplification detected by next-generation sequencing. Lung Cancer (2020) 149:17–22. doi: 10.1016/j.lungcan.2020.08.021
40. Lin JJ, Liu SV, McCoach CE, Zhu VW, Tan AC, Yoda S, et al. Mechanisms of resistance to selective RET tyrosine kinase inhibitors in RET fusion-positive non-small-cell lung cancer. Ann Oncol (2020) 31(12):1725–33. doi: 10.1016/j.annonc.2020.09.015
41. Liu L, Kalyani FS, Yang H, Zhou C, Xiong Y, Zhu S, et al. Prognosis and concurrent genomic alterations in patients with advanced NSCLC harboring MET amplification or MET exon 14 skipping mutation treated with MET inhibitor: A retrospective study. Front Oncol (2021) 11:649766. doi: 10.3389/fonc.2021.649766
42. Liu L, Qu J, Heng J, Zhou C, Xiong Y, Yang H, et al. A large real-world study on the effectiveness of the combined inhibition of EGFR and MET in EGFR-mutant non-small-cell lung cancer after development of EGFR-TKI resistance. Front Oncol (2021) 11:722039. doi: 10.3389/fonc.2021.722039
43. Otsubo K, Sakai K, Takeshita M, Harada D, Azuma K, Ota K, et al. Genetic profiling of non-small cell lung cancer at development of resistance to first- or second-generation EGFR-TKIs by CAPP-Seq analysis of circulating tumor DNA. Oncologist (2019) 24(8):1022–6. doi: 10.1634/theoncologist.2019-0101
44. Park S, Koh J, Kim D-W, Kim M, Keam B, Kim TM, et al. MET amplification, protein expression, and mutations in pulmonary adenocarcinoma. Lung Cancer (2015) 90(3):381–7. doi: 10.1016/j.lungcan.2015.10.022
45. Peng K-C, Su J-W, Xie Z, Wang H-M, Fang M-M, Li W-F, et al. Clinical outcomes of EGFR+/METamp+ vs. EGFR+/METamp- untreated patients with advanced non-small cell lung cancer. Thorac Cancer (2022) 13(11):1619–30. doi: 10.1111/1759-7714.14429
46. Shi H, Seegobin K, Heng F, Zhou K, Zhao Y, Manochakian R, et al. Genomic characterization of primary versus metastatic lung adenocarcinoma. J Thorac Oncol (2021) 16(10):S973. doi: 10.1016/j.jtho.2021.08.258
47. Song Z, Wang X, Zheng Y, Su H, Zhang Y. MET gene amplification and overexpression in Chinese non–small-cell lung cancer patients without EGFR mutations. Clin Lung Cancer (2017) 18(2):213–9.e2. doi: 10.1016/j.cllc.2016.09.011
48. Suryavanshi M, Shah A, Kumar D, Panigrahi MK, Metha A, Batra U. MET amplification and response to MET inhibitors in stage IV lung adenocarcinoma. Oncol Res Treat (2017) 40(4):198–202. doi: 10.1159/000457801
49. Tsui DCC, Lee JK, Frampton GM, Tolba K, Oxnard GR, Camidge DR, et al. Real-world (rw) analysis of quantitative MET copy number (CN) as a biomarker in NSCLC. J Clin Oncol (2022) 40(16_suppl):9123. doi: 10.1200/JCO.2022.40.16_suppl.9123
50. Xu C-W, Wang W-X, Wu M-J, Zhu Y-C, Zhuang W, Lin G, et al. Comparison of the c-MET gene amplification between primary tumor and metastatic lymph nodes in non-small cell lung cancer. Thorac Cancer (2017) 8(5):417–22. doi: 10.1111/1759-7714.12455
51. Yoshimura K, Inoue Y, Inui N, Karayama M, Yasui H, Hozumi H, et al. MET amplification and efficacy of nivolumab in patients with NSCLC. JTO Clin Res Rep (2021) 2(11):100239. doi: 10.1016/j.jtocrr.2021.100239
52. Schubart C, Stöhr R, Tögel L, Fuchs F, Sirbu H, Seitz G, et al. MET amplification in non-small cell lung cancer (NSCLC)— a consecutive evaluation using next-generation sequencing (NGS) in a real-world setting. Cancers (2021) 13(19):5023. doi: 10.3390/cancers13195023
53. Schmitt C, Schulz A-A, Winkelmann R, Smith K, Wild PJ, Demes M. Comparison of MET gene amplification analysis by next-generation sequencing and fluorescence in situ hybridization. Oncotarget (2021) 12(22):2273–82. doi: 10.18632/ONCOTARGET.28092
54. Dagogo-Jack I, Moonsamy P, Gainor JF, Lennerz JK, Piotrowska Z, Lin JJ, et al. A phase 2 study of capmatinib in patients with MET-altered lung cancer previously treated with a MET inhibitor. J Thorac Oncol (2021) 16(5):850–9. doi: 10.1016/j.jtho.2021.01.1605
55. Landi L, Chiari R, Tiseo M, D’Inca F, Dazzi C, Chella A, et al. Crizotinib in MET-deregulated or ROS1-rearranged pretreated non–small cell lung cancer (METROS): A phase II, prospective, multicenter, two-arms trial. Clin Cancer Res (2019) 25(24):7312–9. doi: 10.1158/1078-0432.CCR-19-0994
56. Wu Y-L, Zhang L, Kim D-W, Liu X, Lee DH, Yang JC-H, et al. Phase Ib/II study of capmatinib (INC280) plus gefitinib after failure of epidermal growth factor receptor (EGFR) inhibitor therapy in patients with EGFR-mutated, MET factor-dysregulated non-small-cell lung cancer. J Clin Oncol (2018) 36(31):3101–9. doi: 10.1200/JCO.2018.77.7326
57. Sequist LV, Han J-Y, Ahn M-J, Cho BC, Yu H, Kim S-W, et al. Osimertinib plus savolitinib in patients with EGFR mutation-positive, MET-amplified, non-small-cell lung cancer after progression on EGFR tyrosine kinase inhibitors: Interim results from a multicentre, open-label, phase 1b study. Lancet Oncol (2020) 21(3):373–86. doi: 10.1016/S1470-2045(19)30785-5
58. Angevin E, Spitaleri G, Rodon J, Dotti K, Isambert N, Salvagni S, et al. A first-in-human phase I study of SAR125844, a selective MET tyrosine kinase inhibitor, in patients with advanced solid tumours with MET amplification. Eur J Cancer (2017) 87:131–9. doi: 10.1016/j.ejca.2017.10.016
59. Camidge DR, Janku F, Martinez-Bueno A, Catenacci DVT, Lee J, Lee S-H, et al. Safety and preliminary clinical activity of the MET antibody mixture, Sym015 in advanced non-small cell lung cancer (NSCLC) patients with MET amplification/exon 14 deletion (METAmp/Ex14Δ). J Clin Oncol (2020) 38(15_suppl):9510. doi: 10.1200/JCO.2020.38.15-suppl.9510
60. Wolf J, Seto T, Han J-Y, Reguart N, Garon EB, Groen HJM, et al. Capmatinib in MET exon 14-mutated or MET-amplified non-small-cell lung cancer. N Engl J Med (2020) 383(10):944–57. doi: 10.1056/NEJMoa2002787
61. Wu Y-L, Cheng Y, Zhou J, Lu S, Zhang Y, Zhao J, et al. Tepotinib plus gefitinib in patients with EGFR-mutant non-small-cell lung cancer with MET overexpression or MET amplification and acquired resistance to previous EGFR inhibitor (INSIGHT study): An open-label, phase 1b/2, multicentre, randomised trial. Lancet Respir Med (2020) 8(11):1132–43. doi: 10.1016/S2213-2600(20)30154-5
62. Le X, Paz-Ares L, Meerbeeck JV, Ramirez SV, Galvez CC, Baz DV, et al. Clinical response to tepotinib according to circulating tumor (ct) DNA biomarkers in patients with advanced NSCLC with high-level MET amplification (METamp) detected by liquid biopsy (LBx). J Clin Oncol (2022) 40(16_suppl):9121. doi: 10.1200/JCO.2022.40.16_suppl.9121
63. Bustamante Alvarez JG, Janse S, Owen DH, Kiourtsis S, Bertino EM, He K, et al. Treatment of non-small-cell lung cancer based on circulating cell-free DNA and impact of variation allele frequency. Clin Lung Cancer (2021) 22(4):e519–e27. doi: 10.1016/j.cllc.2020.11.007
64. Gutierrez ME, Choi K, Lanman RB, Licitra EJ, Skrzypczak SM, Pe Benito R, et al. Genomic profiling of advanced non–small cell lung cancer in community settings: Gaps and opportunities. Clin Lung Cancer (2017) 18(6):651–9. doi: 10.1016/j.cllc.2017.04.004
65. Steuer CE, Behera M, Berry L, Kim S, Rossi M, Sica G, et al. Role of race in oncogenic driver prevalence and outcomes in lung adenocarcinoma: Results from the Lung Cancer Mutation Consortium. Cancer (2016) 122(5):766–72. doi: 10.1002/cncr.29812
66. Lam VK, Tran HT, Banks KC, Lanman RB, Rinsurongkawong W, Peled N, et al. Targeted tissue and cell-free tumor DNA sequencing of advanced lung squamous-cell carcinoma reveals clinically significant prevalence of actionable alterations. Clin Lung Cancer (2019) 20(1):30–6.e3. doi: 10.1016/j.cllc.2018.08.020
67. Press RH, Zhang C, Cassidy RJ, Ferris MJ, Zhong J, Steuer CE, et al. Targeted sequencing and intracranial outcomes of patients with lung adenocarcinoma brain metastases treated with radiotherapy. Cancer (2018) 124(17):3586–95. doi: 10.1002/cncr.31589
68. Tang Z, Zhang J, Lu X, Wang W, Chen H, Robinson MK, et al. Coexistent genetic alterations involving ALK, RET, ROS1 or MET in 15 cases of lung adenocarcinoma. Mod Pathol (2018) 31(2):307–12. doi: 10.1038/modpathol.2017.109
69. Yin W, Guo M, Tang Z, Toruner GA, Cheng J, Medeiros LJ, et al. MET expression level in lung adenocarcinoma loosely correlates with MET copy number gain/amplification and is a poor predictor of patient outcome. Cancers (2022) 14(10):2433. doi: 10.3390/cancers14102433
70. Yio J, Wolf B, Yang A, Bayewitz AY, Xu Y. Analysis of correlations between driver mutations and biomarkers with response to immunotherapy in patients with metastatic nonsmall cell lung cancer (NSCLC). J Clin Oncol (2021) 39(15_suppl):e21022. doi: 10.1200/JCO.2021.39.15_suppl.e21022
71. Pennell NA, Mutebi A, Zhou Z-Y, Ricculli ML, Tang W, Wang H, et al. Economic impact of next-generation sequencing versus single-gene testing to detect genomic alterations in metastatic non–small-cell lung cancer using a decision analytic model. JCO Precis Oncol (2019) 3:1–9. doi: 10.1200/PO.18.00356
72. Vanderpoel J, Stevens AL, Emond B, Lafeuille M-H, Hilts A, Lefebvre P, et al. Total cost of testing for genomic alterations associated with next-generation sequencing versus polymerase chain reaction testing strategies among patients with metastatic non-small cell lung cancer. J Med Econ (2022) 25(1):457–68. doi: 10.1080/13696998.2022.2053403
73. Wang Y, Tian P, Xia L, Li L, Han R, Zhu M, et al. The clinical efficacy of combinatorial therapy of EGFR-TKI and crizotinib in overcoming MET amplification-mediated resistance from prior EGFR-TKI therapy. Lung Cancer (2020) 146:165–73. doi: 10.1016/j.lungcan.2020.06.003
74. Wang W, Wang H, Lu P, Yu Z, Xu C, Zhuang W, et al. Crizotinib with or without an EGFR-TKI in treating EGFR-mutant NSCLC patients with acquired MET amplification after failure of EGFR-TKI therapy: A multicenter retrospective study. J Transl Med (2019) 17(1):52. doi: 10.1186/s12967-019-1803-9
75. Fuchs V, Roisman L, Kian W, Daniel L, Dudnik J, Nechushtan H, et al. The impact of osimertinib’ line on clonal evolution in EGFRm NSCLC through NGS-based liquid biopsy and overcoming strategies for resistance. Lung Cancer (2021) 153:126–33. doi: 10.1016/j.lungcan.2020.12.039
76. Yang J-J, Fang J, Shu Y-Q, Chang J-H, Chen G-Y, He JX, et al. A phase Ib study of the highly selective MET-TKI savolitinib plus gefitinib in patients with EGFR-mutated, MET-amplified advanced non-small-cell lung cancer. Invest New Drugs (2021) 39(2):477–87. doi: 10.1007/s10637-020-01010-4
77. Yu HA, Ambrose H, Baik C, Cho BC, Cocco E, Goldberg SB, et al. ORCHARD osimertinib + savolitinib interim analysis: A biomarker-directed phase II platform study in patients (pts) with advanced non-small cell lung cancer (NSCLC) whose disease has progressed on first-line (1L) osimertinib. Ann Oncol (2021) 32:S978–S9. doi: 10.1016/j.annonc.2021.08.1844
78. Liam CK, Ahmad AR, Hsia T-C, Zhou J, Kim D-W, Soo RA, et al. Tepotinib + gefitinib in patients with EGFRmutant NSCLC with MET amplification: Final analysis of INSIGHT. Cancer Res (2022) 82(12_Supplement):CT538. doi: 10.1158/1538-7445.AM2022-CT538
79. Le X, Paz-Ares LG, Van Meerbeeck J, Viteri S, Galvez CC, Baz DV, et al. Tepotinib in patients (pts) with advanced non-small cell lung cancer (NSCLC) with MET amplification (METamp). J Clin Oncol (2021) 39(15_suppl):9021. doi: 10.1200/JCO.2021.39.15-suppl.9021
80. ClinicalTrials.gov. Study of amivantamab, a human bispecific EGFR and cMet antibody, in participants with advanced non-small cell lung cancer (CHRYSALIS)(2022). Available at: https://clinicaltrials.gov/ct2/show/NCT02609776 (Accessed June 14, 2022).
81. Camidge DR, Morgensztern D, Heist RS, Barve M, Vokes E, Goldman JW, et al. Phase I study of 2- or 3-week dosing of telisotuzumab vedotin, an antibody-drug conjugate targeting c-met, monotherapy in patients with advanced non-small cell lung carcinoma. Clin Cancer Res (2021) 27(21):5781–92. doi: 10.1158/1078-0432.CCR-21-0765
82. ClinicalTrials.gov. Study of SAR125844 single agent administered as slow intravenous infusion in adult patients with advanced Malignant solid tumors (SARMET) (2016). Available at: https://clinicaltrials.gov/study/NCT01391533 (Accessed June 14, 2022).
83. ClinicalTrials.gov. A study assessing efficacy and safety of SAR125844 in NSCLC patients with MET amplification (2016). Available at: https://clinicaltrials.gov/study/NCT02435121 (Accessed June 14, 2022).
84. ClinicalTrials.gov. Crizotinib in pretreated metastatic non-small-cell lung cancer with MET amplification or ROS1 translocation (METROS) (2017). Available at: https://clinicaltrials.gov/study/NCT02499614 (Accessed June 14, 2022).
85. ClinicalTrials.gov. Study of metatinib tromethamine tablet (2017). Available at: https://clinicaltrials.gov/study/NCT02650375 (Accessed June 14, 2022).
86. ClinicalTrials.gov. CABozantinib in non-small cell lung cancer (NSCLC) patients with MET deregulation (CABinMET) (2019). Available at: https://clinicaltrials.gov/study/NCT03911193 (Accessed June 14, 2022).
87. ClinicalTrials.gov. First-in-human phase I study of a selective c-Met inhibitor PLB1001 (2019). Available at: https://clinicaltrials.gov/study/NCT02896231 (Accessed June 14, 2022).
88. ClinicalTrials.gov. Phase 2 study of MGCD265 in patients with non-small cell lung cancer with activating genetic alterations in MET (2020). Available at: https://clinicaltrials.gov/study/NCT02544633 (Accessed June 14, 2022).
89. ClinicalTrials.gov. Evaluate safety, tolerability and PK of HLX55 in patients with advanced solid tumors with different cMET status (2020). Available at: https://clinicaltrials.gov/study/NCT04169178 (Accessed June 14, 2022).
90. ClinicalTrials.gov. Glumetinib combined with osimertinib in the treatment of relapsed and metastatic non-small cell lung cancer patients who failed to receive EGFR inhibitors (2020). Available at: https://clinicaltrials.gov/study/NCT04338243 (Accessed June 14, 2022).
91. ClinicalTrials.gov. Tepotinib in solid tumors harboring MET alterations (2020). Available at: https://clinicaltrials.gov/study/NCT04647838 (Accessed June 14, 2022).
92. ClinicalTrials.gov. A phase I study of safety and pharmacokinetics of volitinib in combination with gefitinib in EGFR(+) NSCLC (2020). Available at: https://clinicaltrials.gov/study/NCT02374645 (Accessed June 14, 2022).
93. ClinicalTrials.gov. Study to evaluate the safety and anti-tumor activity of SCC244 (2020). Available at: https://clinicaltrials.gov/study/NCT03466268 (Accessed June 14, 2022).
94. ClinicalTrials.gov. Study on savolitinib combined with osimertinib in treatment of advanced NSCLC with MET amplification (SACHI) (2021). Available at: https://clinicaltrials.gov/ct2/show/NCT05015608 (Accessed June 14, 2022).
95. ClinicalTrials.gov. A safety and efficacy study of INC280 and gefitinib in patients with EGFR mutated, c-MET-amplified NSCLC who have progressed after EGFRi treatment (2021). Available at: https://clinicaltrials.gov/study/NCT01610336 (Accessed June 14, 2022).
96. ClinicalTrials.gov. Study of crizotinib for ROS1 and MET activated lung cancer (2021). Available at: https://clinicaltrials.gov/study/NCT04084717 (Accessed June 14, 2022).
97. ClinicalTrials.gov. Osimertinib with or without savolitinib as 1L in de novo MET+, EGFR+ NSCLC (FLOWERS) (2021). Available at: https://clinicaltrials.gov/study/NCT05163249 (Accessed June 14, 2022).
98. ClinicalTrials.gov. A study evaluating the safety, pharmacokinetics (PK), and preliminary efficacy of ABBV-399 in participants with advanced solid tumors (2022). Available at: https://clinicaltrials.gov/ct2/show/NCT02099058 (Accessed June 14, 2022).
99. ClinicalTrials.gov. A study of tepotinib plus osimertinib in osimertinib relapsed MET amplified NSCLC (INSIGHT 2) (2022). Available at: https://clinicaltrials.gov/ct2/show/NCT03940703 (Accessed June 14, 2022).
100. ClinicalTrials.gov. Phase 2 platform study in patients with advanced non-small lung cancer who progressed on first-line osimertinib therapy (ORCHARD) (2022). Available at: https://clinicaltrials.gov/ct2/show/NCT03944772 (Accessed June 14, 2022).
101. ClinicalTrials.gov. A study comparing savolitinib plus osimertinib vs savolitinib plus placebo in patients with EGFRm+ and MET amplified advanced NSCLC (CoC) (2022). Available at: https://clinicaltrials.gov/ct2/show/NCT04606771 (Accessed June 14, 2022).
102. ClinicalTrials.gov. Osimertinib plus savolitinib in EGFRm+/MET+ NSCLC following prior osimertinib (SAVANNAH) (2022). Available at: https://clinicaltrials.gov/ct2/show/NCT03778229 (Accessed June 14, 2022).
103. ClinicalTrials.gov. Savolitinib plus osimertinib versus platinum-based doublet chemotherapy in participants with non-small cell lung cancer who have progressed on osimertinib treatment (SAFFRON) (2022). Available at: https://clinicaltrials.gov/ct2/show/NCT05261399 (Accessed June 14, 2022).
104. ClinicalTrials.gov. A study of oral PF-02341066, a c-Met/hepatocyte growth factor tyrosine kinase inhibitor, in patients with advanced cancer (2022). Available at: https://clinicaltrials.gov/study/NCT00585195 (Accessed June 14, 2022).
105. ClinicalTrials.gov. Cabozantinib in patients with RET fusion-positive advanced non-small cell lung cancer and those with other genotypes: ROS1 or NTRK fusions or increased MET or AXL activity (2022). Available at: https://clinicaltrials.gov/study/NCT01639508 (Accessed June 14, 2022).
106. ClinicalTrials.gov. Clinical study of oral cMET inhibitor INC280 in adult patients with EGFR wild-type advanced non-small cell lung cancer (Geometry Mono-1) (2022). Available at: https://clinicaltrials.gov/study/NCT02414139 (Accessed June 14, 2022).
107. ClinicalTrials.gov. Sym015 (Anti-MET) in patients with advanced solid tumor Malignancies (2022). Available at: https://clinicaltrials.gov/study/NCT02648724 (Accessed June 14, 2022).
108. ClinicalTrials.gov. Tepotinib phase II in NSCLC harboring MET alterations (VISION) (2022). Available at: https://clinicaltrials.gov/study/NCT02864992 (Accessed June 14, 2022).
109. ClinicalTrials.gov. APL-101 study of subjects with NSCLC with c-Met exon 14 skip mutations and c-Met dysregulation advanced solid tumors (SPARTA) (2022). Available at: https://clinicaltrials.gov/study/NCT03175224 (Accessed June 14, 2022).
110. ClinicalTrials.gov. Nivolumab, cabozantinib s-malate, and ipilimumab in treating patients with recurrent stage IV non-small cell lung cancer (2022). Available at: https://clinicaltrials.gov/study/NCT03468985 (Accessed June 14, 2022).
111. ClinicalTrials.gov. Phase II umbrella study directed by next generation sequencing (TRUMP) (2022). Available at: https://clinicaltrials.gov/study/NCT03574402 (Accessed June 14, 2022).
112. ClinicalTrials.gov. Study of TPX-0022 in patients with advanced NSCLC, gastric cancer or solid tumors harboring genetic alterations in MET (SHIELD-1) (2022). Available at: https://clinicaltrials.gov/study/NCT03993873 (Accessed June 14, 2022).
113. ClinicalTrials.gov. Assessment of anti-tumor and safety in glumetinib in patients with c-MET-positive non-small cell lung cancer (2022). Available at: https://clinicaltrials.gov/study/NCT04270591 (Accessed June 14, 2022).
114. ClinicalTrials.gov. Study evaluating efficacy and safety of capmatinib in combination with osimertinib in adult subjects with non-small cell lung cancers as second line therapy (GEOMETRY-E) (2022). Available at: https://clinicaltrials.gov/study/NCT04816214 (Accessed June 14, 2022).
115. ClinicalTrials.gov. Tepotinib with gefitinib in participants with locally advanced or metastatic NSCLC (INSIGHT) (2022). Available at: https://clinicaltrials.gov/study/NCT01982955 (Accessed June 14, 2022).
116. ClinicalTrials.gov. AZD9291 in combination with ascending doses of novel therapeutics (2022). Available at: https://clinicaltrials.gov/study/NCT02143466 (Accessed June 14, 2022).
117. ClinicalTrials.gov. A study of capmatinib (INC280) in NSCLC patients with MET exon 14 alterations who have received prior MET inhibitor (2022). Available at: https://clinicaltrials.gov/study/NCT02750215 (Accessed June 14, 2022).
118. ClinicalTrials.gov. REGN5093 in patients with MET-altered advanced non-small cell lung cancer (2022). Available at: https://clinicaltrials.gov/study/NCT04077099 (Accessed June 14, 2022).
119. Kim TM, Guarneri V, Jye VP, Khaw LB, Yang J-J, Wislez M, et al. OA21.05 Tepotinib + osimertinib in EGFR-mutant NSCLC with MET amplification following 1L osimertinib: INSIGHT 2 primary analysis. J Thorac Oncol (2023) 18(11):S94.
120. Yang J, Zhou Q, Chen H, Wu L, Zhao J, Guo R, et al. A phase I study of cMET inhibitor bozitinib in patients with advanced NSCLC harboring cMET alterations. Cancer Res (2020) 80(16_Supplement):CT127. doi: 10.1158/1538-7445.AM2020-CT127
121. Le X, Paz-Ares LG, Van Meerbeeck J, Viteri S, Galvez CC, Smit EF, et al. Tepotinib in patients with non-small cell lung cancer with high-level MET amplification detected by liquid biopsy: VISION Cohort B. Cell Rep Med (2023) 4:101280. doi: 10.1016/j.xcrm.2023.101280
122. Engelman JA, Zejnullahu K, Mitsudomi T, Song Y, Hyland C, Park JO, et al. MET amplification leads to gefitinib resistance in lung cancer by activating ERBB3 signaling. Science (2007) 316(5827):1039–43. doi: 10.1126/science.1141478
123. Gainor JF, Dardaei L, Yoda S, Friboulet L, Leshchiner I, Katayama R, et al. Molecular mechanisms of resistance to first- and second-generation ALK inhibitors in ALK-rearranged lung cancer. Cancer Discov (2016) 6(10):1118–33. doi: 10.1158/2159-8290.Cd-16-0596
124. Lin JJ, Choudhury NJ, Yoda S, Zhu VW, Johnson TW, Sakhtemani R, et al. Spectrum of mechanisms of resistance to crizotinib and lorlatinib in ROS1 fusion–positive lung cancer. Clin Cancer Res (2021) 27(10):2899–909. doi: 10.1158/1078-0432.CCR-21-0032
125. Yoda S, Lin JJ, Lawrence MS, Burke BJ, Friboulet L, Langenbucher A, et al. Sequential ALK inhibitors can select for lorlatinib-resistant compound ALK mutations in ALK-positive lung cancer. Cancer Discov (2018) 8(6):714–29. doi: 10.1158/2159-8290.Cd-17-1256
126. Tyler LC, Le AT, Chen N, Nijmeh H, Bao L, Wilson TR, et al. MET gene amplification is a mechanism of resistance to entrectinib in ROS1+ NSCLC. Thorac Cancer (2022) 13(21):3032–41. doi: 10.1111/1759-7714.14656
127. Awad MM, Liu S, Rybkin II, Arbour KC, Dilly J, Zhu VW, et al. Acquired resistance to KRASG12C inhibition in cancer. N Engl J Med (2021) 384(25):2382–93. doi: 10.1056/NEJMoa2105281
128. Rivas S, Marín A, Samtani S, González-Feliú E, Armisén R. MET signaling pathways, resistance mechanisms, and opportunities for target therapies. Int J Mol Sci (2022) 23(22):13898. doi: 10.3390/ijms232213898
129. Ko B, He T, Gadgeel S, Halmos B. MET/HGF pathway activation as a paradigm of resistance to targeted therapies. Ann Transl Med (2017) 5(1):4. doi: 10.21037/atm.2016.12.09
130. Qin K, Hong L, Zhang J, Le X. MET amplification as a resistance driver to TKI therapies in lung cancer: Clinical challenges and opportunities. Cancers (Basel) (2023) 15(3):612. doi: 10.3390/cancers15030612
131. Kishi K, Sakai H, Seto T, Kozuki T, Nishio M, Imamura F, et al. First-line onartuzumab plus erlotinib treatment for patients with MET-positive and EGFR mutation-positive non-small-cell lung cancer. Cancer Treat Res Commun (2019) 18:100113. doi: 10.1016/j.ctarc.2018.10.004
132. ClinicalTrials.gov. Clinical study of antibody-drug conjugate MYTX-011 in subjects with non-small cell lung cancer (2023). Available at: https://clinicaltrials.gov/study/NCT05652868 (Accessed November 21, 2023).
133. ClinicalTrials.gov. A study of amivantamab and capmatinib combination therapy in unresectable metastatic non-small cell lung cancer (METalmark) (2023). Available at: https://clinicaltrials.gov/study/NCT05488314 (Accessed November 21, 2023).
134. Gainor JF, Niederst MJ, Lennerz JK, Dagogo-Jack I, Stevens S, Shaw AT, et al. Dramatic response to combination erlotinib and crizotinib in a patient with advanced, EGFR-mutant lung cancer harboring de novo MET amplification. J Thorac Oncol (2016) 11(7):e83–5. doi: 10.1016/j.jtho.2016.02.021
135. AstraZeneca. Tagrisso plus savolitinib demonstrated 49% objective response rate in lung cancer patients with high levels of MET overexpression and/or amplification in SAVANNAH Phase II trial (2022). Available at: https://www.astrazeneca.com/media-centre/press-releases/2022/tagrisso-plus-savolitinib-demonstrated-49-objective-response-rate-in-lung-cancer-patients-in-savannah-phase-ii-trial.html (Accessed January 16, 2022).
Keywords: non-small cell lung carcinoma, epithelial-mesenchymal transition, systematic review, epidemiology, treatment outcome
Citation: Yang M, Mandal E, Liu FX, O’Hara RM Jr, Lesher B and Sanborn RE (2024) Non-small cell lung cancer with MET amplification: review of epidemiology, associated disease characteristics, testing procedures, burden, and treatments. Front. Oncol. 13:1241402. doi: 10.3389/fonc.2023.1241402
Received: 16 June 2023; Accepted: 27 November 2023;
Published: 11 January 2024.
Edited by:
Guodong Zhao, Zhejiang University Kunshan Innovation Institute, ChinaReviewed by:
Shihori Tanabe, National Institute of Health Sciences (NIHS), JapanTimothy F. Burns, University of Pittsburgh, United States
Himanshi Diwan, Rajiv Gandhi Cancer Institute and Research Centre, India
Copyright © 2024 Yang, Mandal, Liu, O’Hara, Lesher and Sanborn. This is an open-access article distributed under the terms of the Creative Commons Attribution License (CC BY). The use, distribution or reproduction in other forums is permitted, provided the original author(s) and the copyright owner(s) are credited and that the original publication in this journal is cited, in accordance with accepted academic practice. No use, distribution or reproduction is permitted which does not comply with these terms.
*Correspondence: Mo Yang, mo.yang@emdserono.com